Abstract
Due to biological and medicinal properties of pyranopyrazoles, synthesis of these bioactive heterocycles has attracted the interest of medicinal and organic chemists. This review focuses on the recent developments in multicomponent synthesis of pyranopyrazole and spiroconjugated pyranopyrazole systems. The present review describes the literature reports for the period 2005 to 2018. These reported approaches were performed in the classical and nonclassical conditions, particularly recent reports includes protocols under a green condition such as using catalyst, green solvents, and solvent-free conditions.
Introduction
Heterocyclic compounds containing the 4H-pyran ring display significant roles in medicinal and synthetic chemistry, and polyfunctionalized 4H-pyran has attracted the attentions of many researchers involved in drug discovery process,Citation1–3 due to its pharmacological and biological characteristics.Citation4 On the other hand, pyrazole and its derivatives find applications as biodegradable agrochemicals and pharmaceutical ingredients.Citation5,Citation6 Compounds containing a pyrazole scaffold have been shown to exhibit interleukin (IL)-1 synthesis inhibition and human immunodeficiency virus (HIV)-1 reverse transcriptase,Citation7 as well as antibacterial, antihyperglycaemic, antiinflammatory, sedative/hypnotic, analgesic and antipyretic activities.Citation8 Pyranopyrazole compounds, oxygen‐ and nitrogen‐ring fused heterocycles, are important group of heterocyclic compounds with natural and synthetic molecules.Citation9 They showed numerous biological activities such as antibacterial,Citation10 antimicrobial,Citation11,Citation12 anticancer,Citation13 anti-inflammatory and analgesicCitation14 properties. Pavlik et al. have also reported that pyranopyrazoles can form dimers under UV lightCitation15 so that, they can act as UV absorbers. In addition, pyranopyrazoles have also a significant role in agrochemical research due to their fungicidal, bactericidal, and herbicidal properties.Citation16,Citation17 Thus, the synthesis of the heterocyclic compounds containing the pyranopyrazole moeity, is of great importance. There are four isomeric structures for pyranopyrazole including: pyrano[2,3-c]pyrazole (1), pyrano[3,2-c]pyrazole (2), pyrano[3,4-c]pyrazole (3), and pyrano[4,3-c]pyrazole (4) (), but pyrano[2,3-c]pyrazoles (1) have so far been the most investigated one. This can be attributed to the biological significance of this isomer. On the other hand, reports on the preparation of other three pepopyrazoles are rare.
Pyrano[2,3-c]pyrazoles have important activities, such as antitumor,Citation18 analgesic, anti-inflammatory,Citation19,Citation20 antimicrobial,Citation21 and molluscicidalCitation22 properties. In addition, these compounds are significant precursors for promising drugs in the field of medicinal chemistry.Citation23,Citation24 They also act as potential inhibitors of human Chk1 kinase ().Citation25
Thus, considering the importance of pyranopyrazole, several synthetic routes have been reported to prepare these biologically valuable heterocycles. The synthetic procedure includes single or multisteps, and two-component or multicomponent reactions (three or more compound) to obtain pyranopyrazole. Multicomponent reaction is the best reported method for preparation of this biologically active heterocycle. The multicomponent reactions (MCRs) are one-pot process in which at least three or more compound react together to form target product without separation and purification of the intermediates, the main advantages of MCRs, in comparison with traditional multistep protocols, are high efficiency, experimental simplicity, low cost, avoidance of large quantities of waste, reducing labor cost, reaction times, and waste production.Citation26 As MCRs are one-pot reaction, they are easier to perform than the multistep reactions. In addition, MCRs are ecofriendly, and often proceed with excellent chemoselectivities.Citation27–Citation29 The design and development of multicomponent synthetic strategies based on green principles and using renewable and recyclable materials, using solvent-free condition, green solvents, and green or reusable catalyst, using nonclassical conditions such as microwave technologies, ultrasonic irradiations give a possibility to achieve an “ideal synthesis.” In addition, the combination of MCRs processes with environmentally benign protocols, such as performing organic reactions in water is a protocol that has become highly interesting for synthetic chemists, “the best solvent is no solvent and if a solvent (diluent) is needed it should preferably be water.”Citation30 Water has emerged as a versatile solvent for organic reactions recently, because of special effects of water such as abundant, eco-friendly benign, hydrogen bonding in the transition state and high cohesive energy density and also negative activation volume. The use of water as a natural and green solvent is emphasized in green chemical processes.Citation31,Citation32 For these reasons, MCRs in water are of prominent value in green chemistry and organic synthesis.Citation33,Citation34
This review will summarize the reported protocols for the preparation of pyranopyrazole and its spiro conjugated derivatives, but as mentioned, most reports include pyrano[2,3-c]pyrazole, so this review covers the preparation of pyrano[2,3-c]pyrazole and spiro- pyrano[2,3-c]pyrazole derivatives by multicomponent reactions using conventional conditions, MW, and ultrasonic irradiations from 2005 to 2018. The most multicomponent reactions (four-component) have been introduced by employing in situ preparation of pyrazolone ring through the reaction between hydrazine and a β-dicarbonyl compounds.
The first approach to synthesize these substances was performed by Otto,Citation35 in which he initiated the reaction sequence by the base-catalyzed cyclization of 4-aryliden-5-pyrazolone. In a further report, Otto and Schmelz showed that weak bases can also be utilized for a Michael-type cyclization.Citation36 This work was extended by Klokol et al. who performed the direct conversion of 3-methyl-3-pyrazolin-5-one with malononitrile in the presence of a weak base.Citation37
The multicomponent synthesis of pyranopyrazoles
Using catalyst-free conditions
Shaabani et al.Citation38 described a three-component reaction for the synthesis of pyranopyrazoles using acetylenedicarboxylate, isocyanides, and 3-methyl-1-phenyl-1H-pyrazol-5(4H)-one (Scheme 1). The reaction afforded the corresponding pyrano[2,3-c]pyrazole derivatives in good yields without using any catalyst.
A catalyst-free synthesis of a series of pyranopyrazoles at the short reaction times (3–11 min) via a four-component reaction between an aromatic aldehyde, hydrazine hydrate, ethyl acetoacetate and malononitrile at room temperature under neat conditions was reportedCitation39 (Scheme 2). In the reaction, pyrazolone ring was generated in situ through the reaction between hydrazine hydrate and ethyl acetoacetate.
Scheme 2. Synthesis of pyrano[2,3-c]pyrazole derivatives under solvent and catalyst-free conditions.
![Scheme 2. Synthesis of pyrano[2,3-c]pyrazole derivatives under solvent and catalyst-free conditions.](/cms/asset/e819d19f-318b-43a8-8ed6-b8f1df908d95/gpol_a_1584576_c0002_b.jpg)
Mandha et al.Citation10 have reported a green approach for the synthesis of substituted pyrano[2,3-c]pyrazoles and spiro-pyrano[2,3-c]pyrazoles via a multicomponent reaction in aqueous ethanol medium under catalyst-free conditions (Schemes 3 and 4). At the outset, synthesis of pyrano[2,3-c]pyrazoles via a multicomponent approach under catalyst-free conditions, as shown in Scheme 3, was envisaged. In the report, when one-pot reaction was attempted by replacing one of the substrates that is, hydrazine hydrate with phenyl hydrazine, reaction was too sluggish. In order to improve the rate of reaction, 3-methyl-1-phenyl-1H-pyrazol-5(4H)-one (6) was isolated by performing an initial condensation reaction between ethylacetoacetate and phenyl hydrazine through a known procedure. This was followed by the addition of carbonyl compound and malononitrile to 3-methyl-1-phenyl-1H-pyrazol-5(4H)-one (6) in aqueous ethanol medium to give the desired products in high yields. Likewise, the isolation of 3-methyl-1H-pyrazol-5(4H)-one (5) and reacting it with carbonyl compound and malononitrile in aqueous ethanol medium resulted in better yields for the synthesis of the desired products, compared to corresponding one-pot four-component reaction. Another feature of this protocol is that the reaction is facile with the ketones that is, isatin and 9-fluorenone (Scheme 4). The synthesized compounds were also evaluated for their antibacterial, anti-inflammatory and cytotoxic activities.
Scheme 3. Preparation of pyrano[2,3-c]pyrazoles in aqueous ethanol medium under catalyst-free conditions.
![Scheme 3. Preparation of pyrano[2,3-c]pyrazoles in aqueous ethanol medium under catalyst-free conditions.](/cms/asset/c2856d14-680f-4568-b7e5-481d675f98dd/gpol_a_1584576_c0003_b.jpg)
Scheme 4. Synthesis of spiro-pyrano[2,3-c]pyrazoles in aqueous ethanol medium under catalyst-free conditions.
![Scheme 4. Synthesis of spiro-pyrano[2,3-c]pyrazoles in aqueous ethanol medium under catalyst-free conditions.](/cms/asset/d9dff35d-39e1-4c06-9226-8319fb2a3df2/gpol_a_1584576_c0004_b.jpg)
In 2012,Citation40 a four-component catalyst-free synthesis of methyl 6-amino-5-cyano-4-aryl-2,4-dihydropyrano[2,3-c]pyrazole-3-carboxylates (7) in water was described (Scheme 5). This protocol involves reaction between dimethyl acetylenedicarboxylate, hydrazine hydrate, malononitrile, and aromatic aldehydes in water.
Scheme 5. Four-component catalyst-free synthesis of methyl 6-amino-5-cyano-4-aryl-2,4-dihydropyrano[2,3-c]pyrazole-3-carboxylates in water.
![Scheme 5. Four-component catalyst-free synthesis of methyl 6-amino-5-cyano-4-aryl-2,4-dihydropyrano[2,3-c]pyrazole-3-carboxylates in water.](/cms/asset/809cbe7b-03f5-498e-88ae-7900c5c8027b/gpol_a_1584576_c0005_b.jpg)
Pore et al.Citation41 have developed an eco-friendly methodology for the synthesis of a class of spiro-pyranopyrazoles from isatin, DEAD/DMAD, hydrazine hydrate and malononitrile under catalyst-free conditions in aqueous medium (H2O: EtOH) (Scheme 6). Pyrazolone was formed in situ from acetylenic esters and hydrazine hydrate at room temperature which on reaction with isatin and malononitrile at reflux conditions produced spiro-pyranopyrazoles. The significant advantages of the reported protocol were simple reaction conditions, easy work-up procedure, simple isolation and purification of the products by nonchromatographic methods.
Bihani et al.Citation42 have developed a green strategy for the synthesis of 6-amino-4-aryl-3-methyl-2,4-dihydropyrano[2,3-c]pyrazolecarbonitriles (8) by a four-component reaction of a mixture of ethyl acetoacetate, hydrazine hydrate, aldehyde, and malononitrile in boiling water (Scheme 7). Similar protocol was carried out with aliphatic aldehydes in water: ethanol (1:1) at reflux temperature, without using any catalyst, to synthesize the desired products.
Scheme 7. Catalyst-free synthesis of 6-amino-4-aryl-3-methyl-2,4-dihydropyrano[2,3-c]pyrazolecarbonitriles in boiling wather.
![Scheme 7. Catalyst-free synthesis of 6-amino-4-aryl-3-methyl-2,4-dihydropyrano[2,3-c]pyrazolecarbonitriles in boiling wather.](/cms/asset/302cc78d-eea6-4997-ba70-1d380894e662/gpol_a_1584576_c0007_b.jpg)
The synthesis of pyrano[2,3-c]pyrazole derivatives has been described by a regio- and chemoselective four-component reaction of dialkyl 3-oxopentanedioate, an active carbonyl compound, hydrazine and malononitrile in water/ethanol as a green medium at 60 °C for 12 h under catalyst-free conditionCitation43 (Scheme 8). Authors have applied this conditions to a series of different aldehydes, such as isatin derivatives and acenaphthenquinone as active carbonyl compounds, malononitrile or ethyl cyanoacetate, hydrazine and dimethyl 3-oxopentanedioate or diethyl 3-oxopentanedioate to synthesize spiro-pyrano[2,3-c]pyrazoles (Scheme 9).
Scheme 9. Catalyst-free synthesis of spiro-pyrano[2,3-c]pyrazole derivatives in water/ethanol at 60 °C.
![Scheme 9. Catalyst-free synthesis of spiro-pyrano[2,3-c]pyrazole derivatives in water/ethanol at 60 °C.](/cms/asset/b4571204-a9e9-407d-99b5-f0fe7516c0bd/gpol_a_1584576_c0009_b.jpg)
A concise synthesis of 6-amino-4-aryl-3-(trifluoromethyl)-1,4-dihydro-1-phenylpyrano[2,3-c]pyrazole-5-carbonitriles (9) was performed effectively in aqueous media without catalyst by the reaction of aryl aldehydes, malononitrile, and 1-phenyl-3-(trifluoromethyl)-1H-pyrazol-5(4H)-one at 90 °CCitation44 (Scheme 10).
Scheme 10. Catalyst-free synthesis of 6-amino-4-aryl-3-(trifluoromethyl)-1,4-dihydro-1-phenylpyrano[2,3-c]pyrazole-5-carbonitriles in aqueous media.
![Scheme 10. Catalyst-free synthesis of 6-amino-4-aryl-3-(trifluoromethyl)-1,4-dihydro-1-phenylpyrano[2,3-c]pyrazole-5-carbonitriles in aqueous media.](/cms/asset/4bd5d0b1-a9c3-4062-b4ec-6e1612cb3d14/gpol_a_1584576_c0010_b.jpg)
The synthesis of dihydropyrano[2,3-c]pyrazoles using ball milling technique without catalyst and solvent was also reported.Citation45 Dihydropyrano[2,3-c]pyrazoles (10) were prepared via a one-pot and four-component reaction of ethyl acetoacetate, hydrazine hydrate, aromatic aldehyde, and malononitrile (Scheme 11). The procedure proceeds without utilization of any catalyst or solvent using ball milling technique at ambient temperature to afford desired dihydropyrano[2,3-c] pyrazole derivatives.
In 2017,Citation46 preparation of pyrazolo[4',3':5,6]Pyrano[2,3-d]Pyrimidines (11) containing both biologically active pyranopyrazole and pyranopyrimidine compounds using a multicomponent reaction of ethyl acetoacetate, hydrazine hydrate, arylaldehydes and thiobarbituric acid without catalyst in ethanol was reported (Scheme 12). All the synthesized compounds showed good to excellent anti-tuberculosis activities.
Water can be magnetized in the presence of an external magnetic field, and due to the magnetization many properties of water, such as penetration, density, specific heat, vaporization enthalpy, refractive index, electric dipole-moment, average cluster size in the bulk water, surface tension and viscosity, change compared with non-magnetic water.Citation47–Citation49 In 2017,Citation50 synthesis of the pyrano[2,3-c]pyrazole (12) and pyrano[4',3':5,6]pyrazolo[2,3-d]pyrimidine (13) derivatives using magnetized water as a green solvent was reported (Scheme 13). In this article as claimed, water kept its magnetization property for up to 4 h, and a reaction performed in water magnetized for some time was as satisfactory as that performed in a freshly-magnetized water, with high yield.
Scheme 13. Synthesis of the pyrano[2,3-c]pyrazole and pyrano[4',3':5,6]pyrazolo [2,3-d]pyrimidine derivatives using magnetized water.
![Scheme 13. Synthesis of the pyrano[2,3-c]pyrazole and pyrano[4',3':5,6]pyrazolo [2,3-d]pyrimidine derivatives using magnetized water.](/cms/asset/a1f9d2ef-09fb-457f-9356-c751fb0ff3d2/gpol_a_1584576_c0013_b.jpg)
Ramesh et al.Citation51 have reported a solvent-free and catalyst-free five-component procedure for the synthesis of thioether containing dihydropyrano[2,3-c]pyrazoles at 120 °C (Scheme 14).
Using acid catalyst
In 2011,Citation52 a series of pyrano[2,3-c]pyrazoles, was efficiently synthesized via one-pot, four component reaction of ethyl acetoacetate, hydrazine hydrate, aldehydes and malononitrile in the presence of a catalytic amount of environmentally friendly silicotungstic acid (H4[SiW12O40]) at 60 °C under solvent-free condition (Scheme 15).
Gein et al.Citation53 have demonstrated preparation of ethyl 6-amino-4-aryl-5-cyano-2,4-dihydropyrano[2,3-c]pyrazole-3-carboxylates (14) via a four-component reaction of the sodium salt of diethyl oxaloacetate, an aromatic aldehyde, hydrazine hydrate and malononitrile in EtOH and in the presence of acetic acid (Scheme 16).
Scheme 16. Synthesis 6-amino-4-aryl-5-cyano-2,4-dihydropyrano[2,3-c]pyrazole-3-carboxylates by a four-component reaction in the presence of acetic acid.
![Scheme 16. Synthesis 6-amino-4-aryl-5-cyano-2,4-dihydropyrano[2,3-c]pyrazole-3-carboxylates by a four-component reaction in the presence of acetic acid.](/cms/asset/a725138a-b622-491c-bc1d-58e5f0bae631/gpol_a_1584576_c0016_b.jpg)
Preyssler heteropoly acid (HPA, H14NaP5W30O120) is an important acid catalyst because it is safe and has substantial other advantages such as high hydrolytic stability (pH = 0–12) and high thermal stability and the availability of 14 acidic hydrogens.Citation54,Citation55 Due to low surface area (7–10 m2/g) and high solubility in polar solvents, it is usually preferable to use in a supported form such as on acid-neutral solids, alumina and silica, activated carbons, acidic ion-exchange resins and zeolites.Citation54–Citation56 Javid et al.Citation57 have investigated the use of preyssler HPA supported onto the silica coated NiFe2O4 MNPs (NiFe2O4@SiO2-Preyssler, abbreviated NFS-PRS) as an efficient and green catalyst for the synthesis of pyrano[2,3-c]pyrazole derivatives via condensation of aromatic aldehydes, hydrazine hydrate, ethyl acetoacetate and malononitrile at room temperature in water (Scheme 17). Excellent yields, short reaction times, and reusability of catalyst are advantages of this procedure.
Scheme 17. Preparation of pyrido[2,3-c]pyrazoles using HPA supported onto the silica coated NiFe2O4 MNPs (NFS-PRS).
![Scheme 17. Preparation of pyrido[2,3-c]pyrazoles using HPA supported onto the silica coated NiFe2O4 MNPs (NFS-PRS).](/cms/asset/14d77bca-e828-48c0-b7d1-e9ed0b66d3eb/gpol_a_1584576_c0017_b.jpg)
Acetic acid functionalized pyridinium salt, namely 1-(carboxymethyl)pyridiniumiodide ([cmpy]I), was applied as reusable (eight runs) catalyst for the green synthesis of 6-amino-4-(4-methoxyphenyl)-5-cyano-3-methyl-1-phenyl-1,4-dihydropyrano[2,3-c]pyrazoles (15) via one-pot tandem four-component condensation reaction of aryl aldehydes with ethyl acetoacetate, malononitrile and hydrazine hydrate at 100 °C under solvent-free conditionsCitation58 (Scheme 18).
Scheme 18. The use of 1-(carboxymethyl)pyridiniumiodide {[cmpy]I} for the green synthesis of 6-amino-4-(4-methoxyphenyl)-5-cyano-3-methyl-1-phenyl-1,4 dihydropyrano[2,3-c]pyrazoles.
![Scheme 18. The use of 1-(carboxymethyl)pyridiniumiodide {[cmpy]I} for the green synthesis of 6-amino-4-(4-methoxyphenyl)-5-cyano-3-methyl-1-phenyl-1,4 dihydropyrano[2,3-c]pyrazoles.](/cms/asset/43880973-8856-4279-bdd3-a1ec5d069b80/gpol_a_1584576_c0018_b.jpg)
Montmorillonite-K10 as a reusable green heterogeneous acid catalyst was applied under eco-friendly condition to prepare pyrano[2,3-c]pyrazolesCitation59 (Scheme 19). In this green protocol, the solid acid catalyst could be reused five times without noticeable loss of activity.
In 2018,Citation60 the synthesis of thioether linked pyranopyrazoles via five-component reaction between 5-phenyl-1,3,4-oxadiazole-2-thiol, ethyl 4-chloro-3-oxobutanoate, phenylhydrazine, malononitrile and aryldehyde, in the presence of montmorillonite K-10 clay in ethanol-water at 70 °C, was reported (Scheme 20). The reaction was also carried out in the absence of catalyst, and the results showed that in the presence of catalyst, the reactions proceeds in higher yield, furnishing all the products without impurities. Authors have also studied the antioxidant and antibacterial properties of the products. Some compounds displayed higher antioxidant activity while some other compounds showed prominent antibacterial activity. In addition, reusability of the catalyst was examined and the catalyst was reused up to four times without significant loss in activity.
Aqueous solution of boric acid, as a green catalytic system, was utilized for tandem four-component condensation reaction of aryl aldehydes with ethyl acetoacetate, malononitrile and hydrazine hydrate at 70 °C in solvent-free conditions to give pyrano[2,3-c]pyrazole derivativesCitation61 (Scheme 21).
Using base catalyst
In 2007, 4,3'-spiro[(6-amino-5-R-3-methyl-2,4-dihydropyrano[2,3-c]-pyrazolo)-2'-oxindoles] were synthesized based on a three-component condensation of isatins, with suitable CH acid (malononitrile or cyanoacetic ester) and 3-methylpyrazolone in the presence of triethanolamine (N(CH2CH2OH)3) as basic catalystsCitation62 (Scheme 22).
Scheme 22. Synthesis of 4,3'-spiro[(6-amino-5-R-3-methyl-2,4-dihydropyrano[2,3-c]-pyrazolo)-2'-oxindoles] using triethanolamine.
![Scheme 22. Synthesis of 4,3'-spiro[(6-amino-5-R-3-methyl-2,4-dihydropyrano[2,3-c]-pyrazolo)-2'-oxindoles] using triethanolamine.](/cms/asset/db6e5b72-c6ac-41cd-acd0-10c89584ae64/gpol_a_1584576_c0022_b.jpg)
Vasuki and KumaravelCitation63 have developed an environmentally benign four-component reaction in aqueous medium at room temperature for the synthesis of 6-amino-5-cyano-3-methyl-4-aryl/heteroaryl-2H,4H-dihydropyrano[2,3-c]pyrazoles (16) (Scheme 23). The reaction was carried out in the presence of different bases (Et3N, Et2NH, pyrrolidine, piperidine, morpholine, piperazine, K2CO3) to optimize the procedure. The reaction in the presence of piperidine produced the maximum yield. The solvent effect was also verified and water proved to be the best solvent compared to common organic solvents.
Scheme 23. Preparation of 6-amino-5-cyano-3-methyl-4-aryl/heteroaryl-2H,4H-dihydropyrano[2,3-c]pyrazoles in the presence of piperidine.
![Scheme 23. Preparation of 6-amino-5-cyano-3-methyl-4-aryl/heteroaryl-2H,4H-dihydropyrano[2,3-c]pyrazoles in the presence of piperidine.](/cms/asset/ca70e44c-d3cc-4c16-9160-ad48366dd2dd/gpol_a_1584576_c0023_b.jpg)
Lehmann et al.,Citation64 in 2008 have reported the preparation of a library of diverse dihydropyrano[2,3-c]pyrazoles in EtOH at room temperature and 3 h reaction time (Scheme 24). The products were prepared in moderate yield, even with aliphatic substituted aldehydes.
Litvinov et al.Citation65 in 2009 have described a four-component synthesis of substituted and spiro-conjugated 6-amino-2H,4Hpyrano[2,3-c]pyrazol-5-carbonitriles from aromatic aldehydes or saturated heterocyclic ketones, malononitrile, ketoesters, and hydrazine hydrate by refluxing in ethanol for 15 min in the presence of Et3N (Schemes 25–27). As was shown in Scheme 25, changing the sequence of reactants to isatin, changes the product. In this report four-component reaction of isatin, produced stable isatine monohydrazone 17, independent of the reaction time (5–30 min), and a three-component reaction of 3-(dicyanomethylene)-2-oxindole 18 with ketoesters and hydrazine also failed to produce the desired pyrano[2,3-c]pyrazole, and instead yielded the same monohydrazone 17 (Scheme 28). However, by changing the reactant addition sequence, pyrano[2,3-c]pyrazole conjugated with isatins was produced (Scheme 24).
Scheme 25. Four-component synthesis of 6-amino-2H,4Hpyrano[2,3-c]pyrazol-5-carbonitriles using aromatic aldehyde.
![Scheme 25. Four-component synthesis of 6-amino-2H,4Hpyrano[2,3-c]pyrazol-5-carbonitriles using aromatic aldehyde.](/cms/asset/34b9efcf-7e22-4ed7-b72c-be026cb4765c/gpol_a_1584576_c0025_b.jpg)
Piperidine catalyzed synthesis of spiro[indoline-3,4'-pyrano[2,3-c]pyrazole]-5'-carbonitriles (19) was reported via one-pot, four-component reaction of β-ketoesters, hydrazine hydrate, malononitrile, and isatines in aqueous media at room temperatureCitation66(Scheme 29). The authors, further explored the potential of this protocol for the synthesis of spiroheterocyclic, by using the reaction of acenaphthylene-1,2-dione instead of isatin and obtained spiro[acenaphthylene-1,4'-pyrano[2,3-c]pyrazole]-5'-carbonitrile 20 in 87% yield (Scheme 30). The products were also investigated in vitro for antibacterial activities.
Scheme 29. Piperidine catalyzed synthesis of spiro[indoline-3,4'-pyrano[2,3-c]pyrazole]-5'-carbonitriles.
![Scheme 29. Piperidine catalyzed synthesis of spiro[indoline-3,4'-pyrano[2,3-c]pyrazole]-5'-carbonitriles.](/cms/asset/237c2d0e-9960-4f26-ab7e-60a86dbcd3c7/gpol_a_1584576_c0029_b.jpg)
Scheme 30. Piperidine catalyzed synthesis of spiro[acenaphthylene-1,4'-pyrano[2,3-c]pyrazole]-5'-carbonitrile.
![Scheme 30. Piperidine catalyzed synthesis of spiro[acenaphthylene-1,4'-pyrano[2,3-c]pyrazole]-5'-carbonitrile.](/cms/asset/f543e933-204a-43fc-af9f-46f722e62176/gpol_a_1584576_c0030_b.jpg)
Ba(OH)2 catalyzed one-pot four-component reaction of Meldrums acid, ethyl acetoacetate, hydrazine hydrate, and aromatic aldehydes to give 3-methyl-4-aryl-4,5-dihydro-1H-pyrano[2,3-c]pyrazol-6-ones (21) in refluxing water, was reportedCitation67 (Scheme 31). The authors also examined this protocol with aliphatic aldehydes to get the corresponding products but there was no product formation even after 10 h under the optimized reaction conditions.
Scheme 31. Synthesis of 3-methyl-4-aryl-4,5-dihydro-1H-pyrano[2,3-c]pyrazol-6-ones in the presence of Ba(OH)2.
![Scheme 31. Synthesis of 3-methyl-4-aryl-4,5-dihydro-1H-pyrano[2,3-c]pyrazol-6-ones in the presence of Ba(OH)2.](/cms/asset/b6b19697-1ae9-44f3-8c5f-0f805d23ff8f/gpol_a_1584576_c0031_b.jpg)
An environmentally benign four-component reaction in aqueous medium in the presence of cetyltrimethylammonium chloride (CTACl) has been reported for the synthesis of 6-amino-3-methyl-4-aryl-/1-phenyl-1,4-dihydropyrano[2,3-c]pyrazole-5-carbonitriles (22) via reaction between ethyl acetoacetate, hydrazine hydrate/phenylhydrazine, aromatic aldehydes, and malononitrileCitation68 (Scheme 32). In this report, the use of cetyltrimethylammonium chloride (CTACl) as phasetransfer catalyst, improve further hydrophobic surfaces, and accelerate the heterogeneous multicomponent reaction rates.
Scheme 32. Preparation of 6-amino-3-methyl-4-aryl-/1-phenyl-1,4-dihydropyrano[2,3-c]pyrazole-5-carbonitriles using cetyltrimethylammonium chloride (CTACl).
![Scheme 32. Preparation of 6-amino-3-methyl-4-aryl-/1-phenyl-1,4-dihydropyrano[2,3-c]pyrazole-5-carbonitriles using cetyltrimethylammonium chloride (CTACl).](/cms/asset/986d9fda-9a42-4a3b-a48c-3eabcda49731/gpol_a_1584576_c0032_b.jpg)
Pal et al.Citation69 have developed a one-pot protocol for the synthesis of various spiro[indoline-3,4'-pyrano[2,3-c]pyrazole]-3'-carboxylate derivatives (23 and 24) by using four-component reaction of isatin, malononitrile, hydrazine derivatives, and dialkyl acetylenedicarboxylates in the presence a base (Scheme 33). The authores optimized the yield of the reaction by screening the same set of reaction with various widely explored organic bases such as DBU, DABCO, DMAP (4-dimethylaminopyridine), and Et3N. The results showed that, Et3N was superior with respect to reaction time and yields. In this process two C-C bonds, two C-N bonds and one C-O bond have been formed in a one-pot reaction.
Scheme 33. Base catalyzed synthesis of spiro[indoline-3,4'-pyrano[2,3-c]pyrazole]-3'-carboxylate derivatives.
![Scheme 33. Base catalyzed synthesis of spiro[indoline-3,4'-pyrano[2,3-c]pyrazole]-3'-carboxylate derivatives.](/cms/asset/0ab84216-d672-4081-bcdb-56513556a600/gpol_a_1584576_c0033_b.jpg)
Sodium benzoate as the mild basic catalyst was used for preparation of pyrano[2,3-c]pyrazole derivatives through the condensation reaction of aryl aldehydes, ethyl acetoacetate, malononitrile, and hydrazine hydrate or phenyl hydrazine in water at room temperatureCitation70 (Scheme 34).
Jayabal and ParamasivanCitation71 have reported regioselective synthesis of pyranopyrazoles from ethyl acetoacetate, hydrazinehydrate, substituted aldehyde with nitroketene-N,S-acetal (25) in the presence of piperidine under solvent-free condition (Scheme 35). This protocol generates biologically significant heterocycles with the formation of C-C, C = C, C-N, C = N, C-O bonds and one stereo-center in a single operation via condensation/Knoevenagel/Michael/annulation sequences. In this report, (E)-N-Methyl-1-(methylthio)-2-nitroethenamine (NMSM) (25) as an ambiphilic synthon which holds both nucleophilic and electrophilic sites has great potential for the development of pyranopyrazoles and it contains four active sites with three functional groups on an ethene motif () which was widely employed in the synthesis of several pharmacological important heterocycles such as ranitidineCitation72,Citation73 and nizatidineCitation74 used for antiulcer drugs. At present report, chromenopyrazoles were also synthesis by this procedure.
Scheme 35. (E)-N-Methyl-1-(methylthio)-2-nitroethenamine (NMSM) as an ambiphilic synthon for the synthesis of pyrano[2,3-c]pyrazoles.
![Scheme 35. (E)-N-Methyl-1-(methylthio)-2-nitroethenamine (NMSM) as an ambiphilic synthon for the synthesis of pyrano[2,3-c]pyrazoles.](/cms/asset/29bf524d-cfc3-41f7-963b-71401ae36980/gpol_a_1584576_c0035_b.jpg)
5-Phenyl-1H-pyrrole-2,3-diones reacted with malononitrile and 1H-pyrazole-5(4H)-ones in anhydrous toluene and in the presence of triethylamine which gave substituted 6-amino-5-cyano-2′-oxo-5′-phenyl-1′,2′-dihydro-1H-spiro[pyrano[2,3-c]pyrazole-4,3′-pyrroles] (26) (Scheme 36).Citation75 In this method pyrroledione was dissolved in anhydrous toluene, malononitrile and triethylamine were added, and the mixture was heated for 5 min under reflux. Pyrazolone, was then added and the mixture was heated for 15 min under reflux to furnish the desired products.
Scheme 36. Preparation of 6-amino-5-cyano-2′-oxo-5′-phenyl-1′,2′-dihydro-1H-spiro[pyrano[2,3-c]pyrazole-4,3′-pyrroles] in the presence of NEt3.
![Scheme 36. Preparation of 6-amino-5-cyano-2′-oxo-5′-phenyl-1′,2′-dihydro-1H-spiro[pyrano[2,3-c]pyrazole-4,3′-pyrroles] in the presence of NEt3.](/cms/asset/673ea8a5-e269-47bb-bad3-a95c500d752b/gpol_a_1584576_c0036_b.jpg)
A series of coumarin based pyrano[2,3-c]pyrazole derivatives (27) was synthesized using DMAP (4-dimethylaminopyridinein) in water-ethanol medium at ambient temparatureCitation76 (Scheme 37). These compounds were screened for their biological activities using array of techniques.
Scheme 37. Synthesis of coumarin based pyrano[2,3-c]pyrazole derivatives using DMAP (4-dimethylaminopyridinein).
![Scheme 37. Synthesis of coumarin based pyrano[2,3-c]pyrazole derivatives using DMAP (4-dimethylaminopyridinein).](/cms/asset/19564201-d469-49ee-b276-eea714b4a237/gpol_a_1584576_c0037_b.jpg)
Diethyl oxalacetate sodium salt as a reagent (carbonyl compound) was used to obtain functionalized spiro[indoline-3,4'-pyrano[2,3-c]pyrazoles] (28).Citation77 This study contains reaction between isatin, malononitrile, hydrazine derivatives and dialkyl acetylenedicarboxylates in the presence of Et3N in EtOH and AcOH at room temperature (Scheme 38). The proposed mechanism is shown in Scheme 39. In the first stage, two parallel reactions occur with the formation of 3-isatinilidenmalononitrile (29) and pyrazolone (30) from the reactions of (i) malononitrile with isatin in the presence of Et3N and (ii) hydrazine hydrate with the diethyl oxalacetate sodium salt in the presence of acetic acid, respectively. Upon mixing, the two reactive intermediates undergo addition with the formation of 31 and subsequent cyclization and isomerization to give the product 28.
Scheme 38. Diethyl oxalacetate sodium salt as a reagent for the synthesis of spiro[indoline-3,4'-pyrano[2,3-c]pyrazoles].
![Scheme 38. Diethyl oxalacetate sodium salt as a reagent for the synthesis of spiro[indoline-3,4'-pyrano[2,3-c]pyrazoles].](/cms/asset/29f55484-aa94-43bd-8201-e3ea43d223d1/gpol_a_1584576_c0038_b.jpg)
Scheme 39. A plausible mechanism for the preparation of spiro[indoline-3,4'-pyrano[2,3-c]pyrazoles].
![Scheme 39. A plausible mechanism for the preparation of spiro[indoline-3,4'-pyrano[2,3-c]pyrazoles].](/cms/asset/62dd66f4-00f6-4f0d-a8e5-54dcc9416c1f/gpol_a_1584576_c0039_b.jpg)
Kanchithalaivan et al.Citation78 applied N,N-diisopropylethylamine (DIPEA) as catalyst for the synthesis of 1,4-dihydropyrano[2,3-c]pyrazol-6-amines (32) via a one-pot four-component domino reactions of ethyl acetoacetate/ethyl benzoylacetate/DMAD with hydrazines, aromatic aldehydes, and (E)-N-methyl-1-(methylthio)-2-nitroethenamine in refluxing EtOH (Scheme 40). This transformation presumably occurs via cyclization–Knoevenagel condensation–Michael addition–tautomerism–intramolecular O-cyclization–elimination sequence of reactions.
Scheme 40. Application of N,N-Diisopropylethylamine (DIPEA) for the synthesis of 1,4-dihydropyrano[2,3-c]pyrazol-6-amines.
![Scheme 40. Application of N,N-Diisopropylethylamine (DIPEA) for the synthesis of 1,4-dihydropyrano[2,3-c]pyrazol-6-amines.](/cms/asset/57c0fd39-b747-424b-bd39-8f9e6f872d13/gpol_a_1584576_c0040_b.jpg)
Khazaei et al.Citation79 have applied N,2-dibromo-6-chloro-3,4-dihydro-2H-benzo[e][1,2,4]thiadiazine-7-sulfonamide-1,1-dioxide (DCDBTSD) as a homogeneous catalyst for the synthesis of 1,4-dihydropyrano[2,3-c]pyrazoles and spiro-pyrano[2,3-c]pyrazoles by multicomponent reaction in water (Schemes 41 and 42). In the report, 4H-pyran, pyranopyrazole, pyrazolo[1,2-b]phthalazine and spirooxindoles were also prepared by this protocol.
N-Methyl morpholine N-oxide (NMO) and silver oxide (Ag2O), were used as catalyst in a four-component reaction of benzyl halide, malanonitrile/ethyl cyanoacetate, diethyl acetylenedicarboxylate (DEAD)/ethyl acetoacetate (EAA) and hydrazine hydrate to prepare pyrano[2,3-c]pyrazolesCitation80 (Scheme 43). In the absence of Ag2O, the reaction failed to produce the desired product, however with a little excess of NMO and silver oxide the reaction rate was increased. The reaction involves the breaking of C-O bond and formation of 2C-C, 2 CN, and a C-O bond leading to the formation of a five and six membered ring in one-pot operation.
Using organocatalyst
Over the last decade, organocatalytic methods have been extensively employed in organic and medicinal chemistry for designing multicomponent and cascade reactions. The methodology avoids the use of toxic and expensive metal atoms. In 2010,Citation81 glycine, an amino acid, as an organocatalyst was used for the fast (5–20 min) and high-yielding (85–95%) synthesis of fused pyranopyrazoles from ethyl acetoacetate, hydrazine hydrate, arylaldehyde and malononitrile in aqueous medium at 25 °C (Scheme 44).
Supramolecular β-cyclodextrin (β-CD) as a highly efficient, biodegradable and reusable catalyst was employed for the synthesis of pyrano[2,3-c]pyrazole and spiro-pyrano[2,3-c]pyrazole derivatives via a one-pot four component reaction of aldehydes, isatins, hydrazine hydrate, malononitrile and β-ketoester in H2O-EtOH (9:1) at 80 °C under neutral condition (Schemes 45 and 46).Citation82 Authors also obtained 6'-amino-1-(4-(6'-amino-5'-cyano-3'-mthyl-2-oxo-1'H-spiro[indoline-3,4'-pyrano[2,3-c]pyrazol]-1-yl)butyl)-3'-methyl-2-oxo-1'H-spiro[indoline-3,4'-pyrano[2,3-c]pyrazole]-5'-carbonitrile (34) from 1,1'-(butane-1,4-diyl)bis(indoline-2,3-dione) (33), malononitrile, hydrazine hydrate, and β-ketoester (Scheme 47) at the same reaction conditions. The catalyst recovery and reusability was also studied (three cycles).
Scheme 47. Synthesis of 6'-amino-1-(4-(6'-amino-5'-cyano-3'-methyl-2-oxo-1'H-spiro[indoline-3,4'-pyrano[2,3-c]pyrazol]-1-yl)butyl)-3'-methyl-2-oxo-1'H-spiro[indoline-3,4'-pyrano[2,3-c]pyrazole]-5'-carbonitrile in the presence of β-CD.
![Scheme 47. Synthesis of 6'-amino-1-(4-(6'-amino-5'-cyano-3'-methyl-2-oxo-1'H-spiro[indoline-3,4'-pyrano[2,3-c]pyrazol]-1-yl)butyl)-3'-methyl-2-oxo-1'H-spiro[indoline-3,4'-pyrano[2,3-c]pyrazole]-5'-carbonitrile in the presence of β-CD.](/cms/asset/7958e0a4-4a5c-491b-b5f4-e787491f0e5f/gpol_a_1584576_c0047_b.jpg)
Per-6-amino-β-cyclodextrin (per-6-ABCD) which acts simultaneously as a supramolecular chiral host and as an efficient solid base catalyst, was used for solvent-free syntheses of various dihydropyrano[2,3-c]pyrazole derivatives via a four-component reaction at room temperatureCitation83 (Scheme 48). The study involves reaction between hydrazine hydrate, ethyl acetoacetate, arylaldehyde/ketone, and malononitrile in the presence of per-6-ABCD under solvent-free conditions at room temperature to afford dihydropyrano[2,3-c]pyrazoles and spiro-pyrano[2,3-c]pyrazoles. The short reaction time (1 min), excellent yields, reusability of the catalyst (six times) and solvent-free conditions, are advantage of this procedure.
Scheme 48. Per-6-amino-β-cyclodextrin (per-6-ABCD) as catalyst for the synthesis of dihydropyrano[2,3-c]pyrazole derivatives.
![Scheme 48. Per-6-amino-β-cyclodextrin (per-6-ABCD) as catalyst for the synthesis of dihydropyrano[2,3-c]pyrazole derivatives.](/cms/asset/0b6732e1-64eb-4ca3-bf1c-2bb5d2eeb8c5/gpol_a_1584576_c0048_b.jpg)
Imidazole as organocatalyst was also used for the synthesis of pyranopyroles. Siddekha et al.Citation84 have described a one-pot four-component synthesis of pyrano[2,3-c]pyroles from aryl aldehydes, ethyl acetoacetate, malononitrile, and hydrazine hydrate in the presence of catalytic amounts of imidazole in water as medium (Scheme 49).
Scheme 41. Synthesis of 1,4-dihydropyrano[2,3-c]pyrazoles using N,2-dibromo-6-chloro-3,4-dihydro-2H-benzo[e][1,2,4] thiadiazine-7-sulfonamide-1,1-dioxide (DCDBTSD).
![Scheme 41. Synthesis of 1,4-dihydropyrano[2,3-c]pyrazoles using N,2-dibromo-6-chloro-3,4-dihydro-2H-benzo[e][1,2,4] thiadiazine-7-sulfonamide-1,1-dioxide (DCDBTSD).](/cms/asset/66fddde5-c10b-423b-8f87-385b3986a078/gpol_a_1584576_c0041_b.jpg)
Urea as a basic organocatalyst was applied for the synthesis of 1,4-dihydropyrano[2,3-c]pyrazoles.Citation85 The reaction proceed via a four-component reaction leading to in situ generation of 3-methyl-1H-pyrazol-5(4H)-one that eventually undergoes condensation with the aldehydes and malononitrile in aqueous ethanol at room temperature to afford the desired products in good to high yield (84 − 90%) (Scheme 50). In this report a diverse range of highly functionalized 2-amino-3-cyano-4H-pyrans and pyran-annulated heterocyclic scaffolds, such as 2-amino-3-cyano-pyrano[3,2-c]-chromen-5(4H)-ones, 2-amino-3-cyano-pyrano[4,3-b]-pyran-5(4H)-ones, 2-amino-3-cyano-7,8-dihydro-4H-chromen-5(6H)-one, 1H-pyrano[2,3-d]pyrimidine-2,4(3H,5H)-diones, 2-amino-3-cyano-5,10-dioxo-5,10-dihydro-4H-benzo[g]chromenes, 2-amino-3-cyano-4H-pyrans, and bis-pyrans were also prepared via tandem Knoevenagel–cyclocondensation of aldehydes, malononitrile, and C − H activated acidic compounds in aqueous ethanol at room temperature in the presence urea.
Gogoi and ZhaoCitation86 have reported enantioselective synthesis of 6-amino-5-cyanodihydropyrano[2,3-c]pyrazoles (35) via a two-component reaction between 2-pyrazolin-5-ones and benzylidenemalononitriles using a cinchona alkaloid catalyst, the reaction may also be carried out in a three-component or a four-component fashion via the in situ formation of these two components from simple and readily available starting materials (Scheme 51). Authors have investigated enantioselective synthesis of these derivatives through a tandem Michael addition-Thorpe-Ziegler reaction, using some readily available cinchona derivatives as the catalyst. Screening of the catalysts (Scheme 51), led to cupreine (37) as the best catalyst for the reaction. The results also showed that the reaction is very sensitive to the subtle changes in the catalyst structure. According to the report, further screening of the reaction conditions revealed that chloroform is also a good solvent for this reaction and when 1 equiv. of Na2SO4 was used, the ee value of the product was improved. The enantioselectivity of this reaction was found to be highly dependent on the reaction conditions and on the structure of the catalysts and the substrates. The desired products were obtained in excellent yields with mediocre to excellent enantioselectivities (up to >99% ee).
Scheme 51. Enantioselective synthesis of 6-amino-5 cyanodihydropyrano[2,3-c]pyrazoles using cupreine (37).
![Scheme 51. Enantioselective synthesis of 6-amino-5 cyanodihydropyrano[2,3-c]pyrazoles using cupreine (37).](/cms/asset/b24c461a-2c21-46cf-a4ef-80eee3aef472/gpol_a_1584576_c0051_b.jpg)
Cocamidopropyl betaine (CAPB) is a biodegradable zwitterionic surfactant with viscoelastic propertiesCitation87 that self-assembles into flexible worm-like micelles, and increases the viscosity of water through a fluid-like polymeric transient network.Citation88,Citation89 These considerable properties are obtained from the presence of both positive and negative charges in the molecule (). Tamaddon and AlizadehCitation90 have applied CAPB in the green synthesis of pyranopyrazoles via a four-component reaction of aldehydes, ethyl acetoacetate, malononitrile, and hydrazine hydrate in water at 50–60 °C (Scheme 52). This zwitterionic surfactant was superior to anionic, cationic, and nonionic alternatives for accessing high yields of pure products without the use of any organic solvent. The reusability of the catalyst, simple isolation of products, mild reaction conditions, low loading of CAPB for critical micelle concentration and short reaction times were mentioned as advantages of this procedure.
Isonicotinic acid, as a dual and biological organocatalyst, at 85 °C under solvent-free conditions was utilized to prepare 1,4-dihydropyrano[2,3-c]pyrazoles via four-component condensation reaction of aryl aldehydes, ethyl acetoacetate, malononitrile, and hydrazine hydrate (Scheme 53).Citation91 In a plausible mechanism, initially, ethyl acetoacetate is activated by isonicotinic acid and hydrazine attacks to the carbonyl group of the activated ethyl acetoacetate. The recyclability of the catalyst was also checked in the model reaction. The results showed that the catalytic activity of the catalyst was restored within the limits of the experimental errors for four successive runs.
Kamble et al.,Citation92 have applied bleaching earth clay (pH 12.5) as heterogeneous catalyst and PEG-400 (polyethylene glycol-400) as green solvent to synthesize pyrano[2,3-c]pyrazole derivatives via reaction of aromatic aldehydes, (4-chlorophenyl)acetonitrile and 3-methyl-1H-pyrazol-5(4H)-one (Scheme 54). The reusability of the catalyst was checked which revieled that the catalyst can be recycled and reused in the reaction, maintaining good activity up to three cycles.
DABCO as basic organocatalyst was used for the synthesis of tricyclic fused pyrazolopyranopyrimidines containing both biologically active pyranopyrazole and pyranopyrimidine templates via a one-pot, four-component reaction involving ethyl acetoacetate, hydrazine hydrate, benzaldehydes, and barbituric acid in waterCitation93 (Scheme 55).
Waghmare et al.Citation94 also applied DABCO for the synthesis of dihydropyrano[2,3-c]pyrazole derivatives by a one pot, four component reaction of ethyl acetoacetate, hydrazine hydrate, malanonitrile, and various aldehydes in aqueous medium (Scheme 56).
In 2018,Citation95 DABCO was applied as a reusable solid base catalyst for the synthesis of bis-spiro pyranopyrazoles via the condensation reaction between bis-isatins, malononitrile, hydrazine hydrate and β-ketoester derivatives in ethanol at reflux conditions (Scheme 57). The desired bis-isatins were obtained via the reaction between isatin (2 mmol) and dibromoalkane (1 mmol) in PEG-400 in the presence of K2CO3 at room temperature. In this protocol, DABCO can be recovered and reused five times without significant loss in the catalytic activity.
A four-component and solvent-free synthesis of 1,4-dihydropyrano[2,3-c]pyrazoles was described,Citation96 by reaction of hydrazine monohydrate, ethyl acetoacetate, arylaldehydes, and malononitrile in the presence of a green catalytic amount of P2O5/SiO2 (7% w/w), H3PO4/Al2O3 (50% w/w), cellulose sulfuric acid and starch sulfuric acid (Scheme 58). The use of nontoxic and inexpensive materials, simple and clean work-up, short reaction times and good yields of the products were mentioned as advantages of this method.
A grinding protocol for the synthesis of dihydropyrano[2,3-c]pyrazole derivatives from acetylene ester, hydrazine hydrate, arylaldehydes, and malononitrile in the presence of L-proline as organocatalyst under solvent-free conditions was achievedCitation97 (Scheme 59). The products were also evaluated for their in vitro antioxidant and antimicrobial activities.
Guo et al.Citation98 have applied meglumine (an amino sugar derived from glucose), a bio-based chemical, as biodegradable and reusable catalyst for the synthesis of a series of dihydroprano[2,3-c]pyrazoles (45) and spiro[indoline-3,4'-pyrano[2,3-c]pyrazole] derivatives (46) via a one-pot, four-component reaction of carbonyl compound/isatin, hydrazine hydrate, malononitrile, and β-keto ester in EtOH-H2O at room temperature (Schemes 60 and 61). This protocol was also applied with aliphatic aldehydes for the synthesis of desired dihydroprano[2,3-c]pyrazoles. Authors were also investigated the use of acenaphtylene-1,2-dione as a substrate to react with hydrazine hydrate, malononitrile, ethyl acetoacetate for preparation of spiro[acenaphthylene-1,4'-pyrano[2,3-c]pyrazole] (47) under the optimized conditions (Scheme 62).
Scheme 62. Preparation of spiro[acenaphthylene-1,4'-pyrano[2,3-c]pyrazole] meglumine using meglumine.
![Scheme 62. Preparation of spiro[acenaphthylene-1,4'-pyrano[2,3-c]pyrazole] meglumine using meglumine.](/cms/asset/246c0018-5815-4742-ab17-b3dcc340c714/gpol_a_1584576_c0062_b.jpg)
Maltose as organocatalyst was utilized for the synthesis of 1,4-dihydropyrano[2,3-c]pyrazoles via one-pot four-component reaction between hydrazine monohydrate or phenylhydrazine, ethyl acetoacetate, malononitrile, and arylaldehydes under solvent-free and classical heatingCitation99 (Scheme 63).
Kangani et al.Citation100 have applied sucrose, as an homogeneous and biodegradable catalyst for the synthesis of 1,4-dihydropyrano[2,3-c]pyrazole derivatives from aromatic aldehydes, malononitrile, ethyl acetoacetate, and hydrazine monohydrate under thermal and solvent-free conditions (Scheme 64). Authors also used maltose and glucose as catalyst for the synthesis of 1,4-dihydropyrano[2,3-c]pyrazoles, under the optimized reaction conditions, which produced similar results.
Ammonium triflate is an organocatalyst which has been used in a variety of reactions. Zhou et al.Citation101 have described a one-pot, four-component reaction of ethyl acetoacetate, hydrazine hydrate, aldehydes, and malononitrile using morpholine triflate (MorT) for preparation of dihydropyrano[2,3-c]pyrazoles (Scheme 65). Authors examined different kinds of ammonium triflates () for the optimization of the reaction conditions. MorT proved to be the most efficient one, giving the highest yield.
Bovine serum albumin (BSA) is an efficient biocatalyst in many organic reactions. In 2016,Citation102 BSA catalytic synthesis of dihydropyrano[2,3-c] pyrazole and spiro[indoline-3,4'-pyrano[2,3-c]pyrazole] derivatives via one-pot, three component reaction of aldehyde/ketone or isatin, malononitrile, and 3-methyl-1H-pyrazol-5 (4H)-one in H2O-EtOH (7:3) at ambient temperature, was reported (Scheme 66). Authors examined different commercially available enzymes (lipase from Candida rugosa, lipase from porcine pancreas,trypsin from bovine, trypsin from porcine pancreas, papain, diastase α-amylase) to screen their role as a catalyst in the synthesis of model product. They also investigated the reusability of catalyst, the results showed that enzyme BSA can be reused up to three runs without significant loss in activity.
In 2017,Citation103 synthesis of spiro[indoline-3,4'-pyrano[2,3-c]pyrazole] derivatives (48) from tandem, four-component reaction of various hydrazines, isatins, β-ketoesters, and malononitrile or methyl(ethyl) cyanoester in the presence of N,N,N',N’-tetrabromobenzene-1,3-disulfonamide [TBBDA] and poly(N,N’-dibromo-N-ethyl-benzene-1,3-disulfonamide) (PBBS) as efficient organocatalysts was reported (Scheme 67). They also revealed that TBBDA and PBBS are stable under atmospheric conditions for two months and also, after completion of the reaction, the catalyst is recovered and can be reused several times without significant decrease in the yield of reaction products.
Scheme 67. Synthesis of spiro[indoline-3,4'-pyrano[2,3-c]pyrazole] derivatives in the presence of TBBDA or PBBS.
![Scheme 67. Synthesis of spiro[indoline-3,4'-pyrano[2,3-c]pyrazole] derivatives in the presence of TBBDA or PBBS.](/cms/asset/bb12a941-3fed-4308-b4fd-9fd288d1d2aa/gpol_a_1584576_c0067_b.jpg)
Moosavi-zare et al.Citation104 have prepared pyrano[2,3-c]pyrazole derivatives by condensation reaction of aryl aldehydes ethyl acetoacetate, malononitrile, and hydrazine hydrate in the presence of Ph3CCl (TrCl) as neutral organocatalyst at 60 °C under solvent-free conditions (Scheme 68). In the protocol, TrCl by in situ generation of trityl carbocation (Ph3C+), promotes the reaction.
In 2017, Fatahpour et al.Citation105 applied acetylsalicylic acid or aspirin for preparation of dihydropyrano[2,3-c]pyrazoles and spiro-pyranopyrazoles under solvent-free conditions at 80 °C (Scheme 69). In this report, 4,4′-(arylmethylene)bis(1H-pyrazol-5-ols) was also obtained by similar protocol.
Using inorganic catalyst
In 2015,Citation106 a one-pot five-component strategy for synthesis of a series of biaryl substituted pyranopyrazole derivatives, involving Suzuki coupling of 4-bromobenzaldehyde and arylboronic acids followed by a four-component reaction from ethyl acetoacetate, malononitrile, and hydrazine hydrate was reported (Scheme 70). Authors examined a variety of bases, such as KF.2H2O, NaOH, CsCO3, K2CO3, Na2CO3, NaOAc, NaHCO3, Na3PO4, Na2HPO4, and Et3N, as well as a variety of solvents, such as EtOH, iPrOH, CH3COCH3, THF, PEG-400, DMF, and effects of temperature in the presence of a catalytic amount of Pd/C (1.0 mol% of Pd). The results proved that KF·2H2O is the best. In this report, electron-donating or electron-withdrawing substituents on the aromatic ring of the arylboronic acids had a significant impact on the conversions. The reactivity of arylboronic acids with electrondonating substituent was higher than those with electronwithdrawing substituent in the para position of aromatic ring.
Scheme 70. Five-component reaction for the synthesis a series of biaryl substituted pyranopyrazoles using Pd/C.
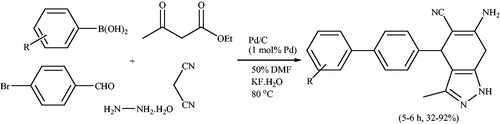
Ebrahimipour et al.Citation107 have applied both nano and bulk forms of [Ni(L)(mimi)] for the synthesis of dihydropyrano[2,3-c]pyrazoles (Scheme 71). They prepared, a mixed-ligand Ni(II) complex, [Ni(L)(mimi)], by the reaction of Ni(OAc)24H2O, 4-bromo-2-[(2-hydroxy-5-methylphenyl]iminomethyl] phenol [H2L] and 1-methylimidazole (mimi) in two forms of bulk and nanoparticle sizes using slow evaporation and ultrasonic irradiation, respectively. Authors characterized the complex by physicochemical and spectroscopic methods, and also by single crystal X-ray diffraction. The Ni(II) center was coordinated by the ONO donor ligand and the nitrogen of 1-methylimidazole. Scanning electron microscopy revealed that the particle size of the synthesized complex using ultrasonic irradiation was below 100 nm. Both the nano and bulk forms of [Ni(L)(mimi)] were effective catalysts for four-component synthesis of dihydropyrano[2,3-c]pyrazoles from a stoichiometric mixture of ethyl acetoacetate, hydrazine hydrate, aldehyde and malononitrile in ethanol, and the result showed that catalytic activity of the nano form of the complex was higher.
Scheme 71. Four-component synthesis of pyranopyrazoles using a mixed-ligand Ni(II) complex [Ni(L)(mimi)].
![Scheme 71. Four-component synthesis of pyranopyrazoles using a mixed-ligand Ni(II) complex [Ni(L)(mimi)].](/cms/asset/f20ccbc3-361a-4f56-b7dd-5c67a6c0a7fe/gpol_a_1584576_c0071_b.jpg)
In 2017, Gangu et al.Citation108 applied Fe-CaOx materials as recyclable heterogeneous catalysts for the synthesis of 2,4-dihydropyrano[2,3-c]pyrazoles (49) via four component condensation of aromatic aldehydes, malononitrile, hydrazine hydrate and dimethyl acetylenedicarboxylate in ethanol with high yields (92–98%) and short reaction times (<20 min) (Scheme 72). The authors prepared Fe-CaOx catalysts by co-precipitation technique using glutamic acid as an additive for crystal growth inhibition. Glutamic acid induced the morphologically of well controlled Fe-CaOx crystals without altering the thermally stable calcium oxalate monohydrate (COM) phase. The characterization results indicate that doped iron occupies the interstitial positions of CaOx crystal. The doping of iron in CaOx enhances the number of catalytic sites which facilitate the Knoevenagel condensation between aromatic aldehyde and malononitrile and providing a rapid synthesis of pyrano[2,3-c]pyrazole derivatives.
Using polymeric catalyst
The resin-bound catalysts are important catalysts in organic synthesis, and are easily removed from the reaction mixture by filtration. Unlike commonly used acid–base catalysts, resin-bound catalysts offer easy recovery of the catalyst. Therefore, resin-bound catalysts can be used several times to drive a reaction into completion without introducing any difficulty to the purification process. Amberlyst A21, is a neutral ion-exchange resin and a solid base, can catalyze organic synthesis. Bihani et al.Citation109 have used Amberlyst A21 as catalyst for the synthesis of a series of 6-amino-4-alkyl/aryl-3-methyl-2,4-dihydropyrano[2,3-c]pyrazole-carbonitriles (50) via reaction of ethyl acetoacetate, hydrazine hydrate, arylaldehyde, and malononitrile in ethanol at room temperature (Scheme 73). The catalytic efficiency of Amberlyst A21 was compared with some other resin-bound free and anionic bases (Amberlite IRA-67, Amberlyst A26 OH, Amberlite IRA-400 OH) , in order to achieve the best catalyst. Amberlyst A21 was also applied for the reaction of acyclic/cyclic ketones, instead of aldehyde, to give corresponding dihydropyrano[2,3-c]pyrazoles or their spirocyclic variants. Easy recovery of the catalyst and its reusability (five times), room temperature reaction conditions, no chromatographic purification, are advantages of this procedure.
Scheme 73. Synthesis of 6-amino-4-alkyl/aryl-3-methyl-2,4-dihydropyrano[2,3-c]pyrazole-carbonitriles using Amberlyst A21.
![Scheme 73. Synthesis of 6-amino-4-alkyl/aryl-3-methyl-2,4-dihydropyrano[2,3-c]pyrazole-carbonitriles using Amberlyst A21.](/cms/asset/9aca63eb-e70b-4fed-85c7-6eeba55ae79a/gpol_a_1584576_c0073_b.jpg)
The use of polymer-supported catalyst for the preparation of pyranopyrazoles was reported.Citation110 A one-pot protocol for the synthesis of dihydropyrano[2,3-c]pyrazole derivatives using polystyrene-supported p-toluenesulfonic acid (PS-PTSA) as an active and reusable heterogeneous catalyst, in water, was developed (Scheme 74). Authors also investigated polystyrene-supported triethylamine (PS-TEA) and polystyrene-supported diisopropylethylamine (PS-DIEA) in the model reaction and the results showed that the catalytic activity of PS-TEA and PS-DIEA was significantly lower than that of PS-PTSA. Reusability of PS-PTSA was tested which maintained its activity with no significant reduction in the yield after five consecutive runs.
Polyethylene glycol (PEG), a biologically acceptable polymer, and modified polyethylene glycol derivatives were employed as a phase-transfer catalyst in various organic transformations.Citation34 In 2015,Citation111 a synthetic protocol has been reported for the synthesis of spiro[indoline-3,4'-pyrano[2,3-c]pyrazole]-3'-carboxylate (51) (Scheme 75) and trifluoromethylated spiro[indole-3,4'-pyrano[2,3-c]pyrazole] (52) (Scheme 76) derivatives via four component reaction using recyclable polyethylene glycol (PEG-400) as a reaction medium.
Using ionic liquid
In recent years, ionic liquids (ILs) have attracted increasing interest and been successfully utilized in a variety of reactions as environmentally benign catalysts and solvents due to their low vapor pressure, relatively low viscosity, and high thermal and chemical stability.Citation112–115 Much attention has currently been focused on the organic reactions with ILs as solvents or catalysts, and many synthetic reactions have been carried out in ILs with excellent outcomes.
Khurana et al.Citation116 have investigated a synthetic protocol of 4H-pyrano[2,3-c]pyrazoles using four-component cyclocondensation of hydrazine monohydrate/phenyl hydrazine, ethyl acetoacetate, aldehydes, and malononitrile in the presence of L-proline and [Bmim]BF4 at 50 °C (Scheme 77). In this study, the model reaction was carried out using equimolar amount of [Bmim]BF4 in the presence of different bases (Et3N, Et2NH, piperidine, K2CO3, L-proline) at 50 °C. [Bmim]BF4. L-Proline was found as the best reaction medium with the highest efficiency at present study.
3-Methyl-1-(4-sulfonic acid)butylimidazolium hydrogen sulfate [(CH2)4SO3HMIM][HSO4], an acidic ionic liquid and as a reusable catalyst (four times) at room temperature and under solvent-free conditions was utilized for preparation of dihydropyrano[2,3-c]pyrazole derivativesCitation117 (Scheme 78).
The use of ionic liquids and solid salts (with an organic cation) as heterogeneous and reusable catalysts for the synthesis of pyranopyrazoles was also reported. Moosavi-zare et al.Citation118 have applied disulfonic acid imidazolium chloroaluminate ([Dsim]AlCl4) as an acidic and heterogeneous catalyst for green synthesis of 6-amino-4-(4-methoxyphenyl)-5-cyano-3-methyl-1-phenyl-1,4-dihydropyrano[2,3-c]pyrazoles (53) by the one-pot multicomponent condensation of arylaldehydes, ethyl acetoacetate, malononitrile and hydrazine hydrate at 80 °C under solvent-free conditions (Scheme 79). In this protocol, disulfonic acid imidazolium chloroaluminate was prepared in two steps. Initially, ionic liquid disulfonic acid imidazolium chloride ([Dsim]Cl) was synthesized, by the reaction of imidazole (1 eq.) with chlorosulfonic acid (2 eq.). Then, [Dsim]AlCl4 was prepared by the reaction of [Dsim]Cl (1 eq.) and aluminum chloride (1 eq.) with high atomic economy.
Scheme 79. Synthesis of 6-amino-4-(4-methoxyphenyl)-5-cyano-3-methyl-1-phenyl-1,4-dihydropyrano[2,3-c]pyrazoles using [Dsim]AlCl4.
![Scheme 79. Synthesis of 6-amino-4-(4-methoxyphenyl)-5-cyano-3-methyl-1-phenyl-1,4-dihydropyrano[2,3-c]pyrazoles using [Dsim]AlCl4.](/cms/asset/754b441c-cdc8-49da-b253-b625e12046eb/gpol_a_1584576_c0079_b.jpg)
The mildly basic ionic liquid N,N,N,N-tetramethylguanidinium acetate [TMG][Ac] was applied as basic catalyst for the one-pot, four-component synthesis of dihydro-1H-pyrano[2,3-c]pyrazol-6-ones via reaction between Meldrums acid, ethyl acetoacetate, hydrazine hydrate and aromatic aldehydes at 80 °C under solvent-free conditionsCitation119 (Scheme 80). The ionic liquid could be recycled several times without loss of efficiency with regards to the reaction times and yields. According to the literature 2H-indazolo[2,1-b]phthalazine-triones was also obtained by this protocol.
1,4-Dimethyl-1-(4-sulphobutyl)piperazinium hydrogen sulfate (IL1), an ionic liquid with both Brønsted acidic and Lewis basic sites was applied to synthesis of pyranopyrazoles at ambient temperature under solvent-free conditions. This“green,” homogeneous and reusable catalyst was also used to prepare benzopyrans at ambient temperature, amino-2-chromenes and dihyropyrano[c]chromenes at 80 °C under solvent-free conditionsCitation120 (Scheme 81). In addition, the double Brønsted acid, 1,4-dimethyl-1,4-bis(4-sulphobutyl)piperazinium hydrogen sulfate (IL2) (Scheme 81), was prepared to evaluate the cooperation efficiency of its Brønsted acidic and Lewis basic sites as compared with the double Brønsted acidic sites in IL1.
Scheme 81. Synthesis of pyranopyrazoles using 1,4-dimethyl-1-(4-sulphobutyl)piperazinium hydrogen sulfate.
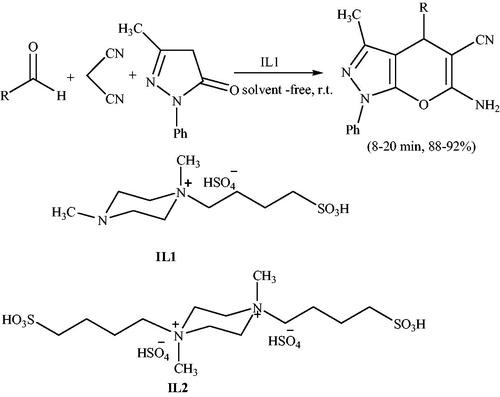
The synthesis of some pyrano[2,3-c]pyrazoles by [ChCl][ZnCl2]2 and [H-NMP]HSO4 as inexpensive and recyclable acidic ionic liquids were investigatedCitation121 (Scheme 82). The comparative study of [ChCl][ZnCl2]2 and [H-NMP]HSO4 ionic liquids was also carried out. [H-NMP]HSO4 showed lower reaction time than the other. It can be attributed to Brønsted acidic property of [H-NMP]HSO4, while [ChCl][ZnCl2]2 is a catalyst with Lewis acidic property.
Mamaghani et al.Citation122 have described an efficient one-pot synthesis of pyrano[2,3-c]pyrazole derivatives and spiro-conjugated pyrano[2,3-c]pyrazole based on Brønsted-acidic ionic liquid catalyst [DMBSI]HSO4 (1,2–dimethyl-N-butanesulfonic acid imidazolium hydrogen sulfate) at 60 °C under solvent-free conditions (Schemes 83 and 84). Interestingly, authors also applied this protocol to synthesis of bis-1,4-dihydro-3-methylpyrano[2,3-c]pyrazole-5-carbonitriles () in high yields. The synthesized compounds were screened for their antibacterial activities against both Gram-negative (Pseudomonas aeruginosa and Escherichia coli) and Gram-positive (Micrococcus luteus and Bacillus subtilis) bacteria. The usability of catalyst was also examined. According to their report, after three runs, catalytic activity of the catalyst was retained without significant loss of activity.
In 2018,Citation123 the SO3H-functionalized ionic liquid ([DMBSI]HSO4) was applied for the synthesis of methyl 4-(aryl)-3-methyl-6-oxo-1,4,5,6-tetrahydropyrano[2,3-c]pyrazole-5-carboxylates (54) via four-component reaction of arylaldehyde, ethyl acetoacetate, hydrazine hydrate and dimethyl malonate at 90 °C under solvent-free condition in high yields (85–95%) and short reaction times (17–24 min) (Scheme 85). This approach was also utilized in preparation of bis-pyrano[2,3-c]pyrazoles () in excellent yields (87-92%) and short reactinon times (17–18 min).
Scheme 85. Methyl 4-(aryl)-3-methyl-6-oxo-1,4,5,6-tetrahydropyrano[2,3-c] pyrazole-5- carboxylates using [DMBSI]HSO4.
![Scheme 85. Methyl 4-(aryl)-3-methyl-6-oxo-1,4,5,6-tetrahydropyrano[2,3-c] pyrazole-5- carboxylates using [DMBSI]HSO4.](/cms/asset/167a453d-871d-4d91-a2e7-f29858dde33f/gpol_a_1584576_c0085_b.jpg)
Zakeri et al.Citation124 have applied 1,3-dimethyl-2-oxo-1,3-bis(4-sulfobutyl) imidazolidine-1,3-diium hydrogen sulfate [DMDBSI].2HSO4 for the four-component reaction of phenylhydrazine, ethyl acetoacetate, benzaldehyde, and malononitrile to prepare pyrano[2,3-c]pyrazoles in H2O at 60 °C (Scheme 86). Authors, in initial experiments, in order to find an effective catalyst and optimize the reaction conditions, screened a series of ILs with respect to both cation and anion counterparts (). They observed that the sulfonic acid ILs effectively catalyze the synthesis of desired pyrano[2,3-c]pyrazole. The optimized reaction conditions were also applied to the synthesis of bis-pyrano[2,3-c]pyrazole derivatives in good yields. Recyclability of the catalyst was also studied, the results showed that the catalyst could be used at least four times without any significant loss in the yield of the model reaction.
Preparation of pyranopyrazoles using supported ionic liquids has also been reported. In 2017,Citation125 synthesis of pyranopyrazoles using silica-supported ionic liquids was studied. Silicabonded 5-n-propyl-octahydro-pyrimido[1,2-α]azepiniumchloride (SBDBU+Cl−), silica-bonded n-propyl-4-aza-1-azoniabicyclo[2.2.2]octane chloride (SB-DABCO+Cl−), and nano silica-bonded 5-n-propyloctahydro-pyrimido[1,2-a]azepinium chloride (NSB-DBU+Cl−) () as silica-supported ionic liquids catalyzed the synthesis of 3-methyl-4-aryl-4,5-dihydro-1H-pyrano[2,3-c]pyrazol-6-ones (55) (Scheme 87) and pyrazolopyranopyrimidines (56) (Scheme 88) via four-component reactions under solvent-free conditions. These green, heterogeneous catalysts are separated from the reaction and recovered for at least four times without significant loss of their catalytic activities. In this article, they also reported preparation of, 1,6-diamino-2-oxo-1,2,3,4-tetrahydropyridine-3,5-dicarbonitrile derivatives using the same catalysts.
Scheme 87. Supported ionic liquids catalyzed synthesis of 3-methyl-4-aryl-4,5-dihydro-1H-pyrano[2,3-c]pyrazol-6-ones.
![Scheme 87. Supported ionic liquids catalyzed synthesis of 3-methyl-4-aryl-4,5-dihydro-1H-pyrano[2,3-c]pyrazol-6-ones.](/cms/asset/116de054-5ecc-4f36-a04c-aced156d50c0/gpol_a_1584576_c0087_b.jpg)
Deep eutectic solvents (DESs) are known as a new class of ionic liquid analogs because they have many properties and characteristics similar to ionic liquids. These are actually two different types of solvent. DESs are formed from a eutectic mixture of Lewis or Brønsted acids and bases which can contain a variety of anionic and/or cationic species. In contrast, ionic liquids are formed from systems composed primarily of one type of discrete anion and cation. Deep eutectic solvents (DESs) have also been used for the preparation of pyranopyrazoles. DES is generally composed of two or three safe and cheap components which are capable of keeping association with each other through hydrogen bond interactions to form a eutectic mixture. The resulting DES is characterized by its melting point, which is lower than of the individual components.Citation126 One of the most significant deep eutectic phenomenons was observed for a mixture of choline chloride (ChCl) and urea (1:2). In 2016,Citation127 synthesis of 6-amino-2H,4H-pyrano[2,3-c]pyrazole-5-carbonitriles (57) was accomplished by cyclocondensation of aryl aldehydes, malanonitrile, ethyl acetoacetate, and hydrazine hydrate in freshly prepared deep eutectic solvent (DES) (choline chloride:urea) (Scheme 89). In the report, stronger hydrogen-bonding capabilities of DES might enhance the electrophilic character of carbonyl carbons of the reactants and thus accelerate formation of the desired products. Authors also examined recyclability of the DES and after three successive cycles, DES was still effective as medium and catalyst.
Scheme 89. Preparation of 6-amino-2H,4H-pyrano[2,3-c]pyrazole-5-carbonitriles using DES (choline chloride:urea).
![Scheme 89. Preparation of 6-amino-2H,4H-pyrano[2,3-c]pyrazole-5-carbonitriles using DES (choline chloride:urea).](/cms/asset/de49e9cd-1e25-4457-b07a-e51aacc3f254/gpol_a_1584576_c0089_b.jpg)
Moshtaghi Zonouz et al.Citation128 have also reported synthesis of dihydropyrano[2,3-c]pyrazole derivatives from aromatic aldehydes, malononitrile, hydrazine hydrate, and ethyl acetoacetate in ChCl–urea (in a 1:2 molar ratio) DES medium (Scheme 90).
The synthesis of 6-amino-4-aryl-2,4-dihydro-3-phenyl pyrano [2,3-c] pyrazole-5-carbonitrile derivatives (58) by one-pot, four-component reaction of arylaldehydes, hydrazine hydrate, ethyl benzoylacetate, and malonitrile using choline chloride based thiourea (ChCl.2Thiourea) has also been reportedCitation129 (Scheme 91). Authors also carried out a number of reactions in the ultrasonic conditions at room temperature. The usability of the catalyst was examined. The catalyst was recovered and reused in the next reaction without loss of activity. In this report, all compounds were subsequently evaluated for their in vitro antibacterial activity compared with Cefazolin by minimum inhibitory concentration, all of these compounds were more active than Cefazolin.
Using nanocatalyst
Heterogeneous catalysts have been extensively used for speeding of organic reactions. The efficiency of heterogeneous catalysis in organic synthesis can be improved by using nanosized catalysts due to their high specific surface area, that lead to an increase of the contact with the reactants.Citation130 These nanoscale particles generally display higher catalytic activity than conventional heterogeneous catalysts. Recently, because of these unique features, the nanoscale catalysts are much utilized in synthetic chemistry. Several methodologies have been also reported for the synthesis of pyranopyrazole using nanoscale catalysts. In 2011,Citation131 a four-component reaction of hydrazine hydrate or phenyl hydrazine, ethyl 3-alkyl-3-oxo-propanoate, aldehydes and malononitrile in the presence of nanosized magnesium oxide as a heterogeneous base catalyst in acetonitrile and at room temperature for preparation of 6-amino-3-alkyl-4-aryl-5-cyano-1,4-dihydropyrano[2,3-c]pyrazoles 59 has been reported (Scheme 92). The reaction was also carried out in the presence of commercially available magnesium oxide (MgO). The catalytic activity of the nanosized MgO particles was compared with the commercially available MgO catalyst. The result showed that the activity of the nanosized MgO was much better, compared to the commercial MgO catalyst.
Scheme 92. Synthesis of 6-amino-3-alkyl-4-aryl-5-cyano-1,4-dihydropyrano[2,3-c]pyrazoles in the presence of magnesium oxide.
![Scheme 92. Synthesis of 6-amino-3-alkyl-4-aryl-5-cyano-1,4-dihydropyrano[2,3-c]pyrazoles in the presence of magnesium oxide.](/cms/asset/8624b593-24e2-4a15-a7ca-cc4577eb3951/gpol_a_1584576_c0092_b.jpg)
Magnetic Fe3O4 nanoparticles as a heterogeneous catalyst, were used for the synthesis of a series of pyranopyrazoles by a four component reaction of a mixture of hydrazine hydrate, ethyl acetoacetate, aldehydes/ketones, and malononitrile in water and at room temperature (Scheme 93).Citation132 Authors examined the model reaction by using three types of catalysts, i.e., Fe3O4, Fe3O4 nanoparticles and recovered Fe3O4 nanoparticles. The highest yield of product, in the lower reaction time was obtained using Fe3O4 nanoparticles. The recovered catalyst was found to be similar to the fresh one.
CuFe2O4 magnetic nanoparticles were synthesized and applied as an efficient catalyst for one-pot synthesis of 3-methyl-1,4-dihydropyrano[2,3-c]pyrazoles (60) in H2OCitation133 (Scheme 94). The four component reaction of a wide variety of substituted hydrazine derivatives, ethyl acetoacetate, dialkyl acetylenedicarboxylates, and alkyl nitrile derivatives (malononitrile and ethyl cyanoacetate) gave the desired dihydropyrano[2,3-c]pyrazoles. When they introduced two equivalents of dialkyl acetylenedicarboxylates instead of ethyl acetoacetate, a new four-component reaction took place providing another type of dihydropyrano[2,3-c]pyrazole derivatives (61) (Scheme 95). In this route, due to the presence of electron withdrawing –CO2R group, the reaction was carried out at room temperature. This reaction was also performed in ethanol media, by introducing EtOH, the yield of the products of this four component reaction changed, authors concluded that in ethanol media, intermediate 63 (releasing MeOH) was formed in greater extent than 64 (releasing EtOH), leaving a greater amount of unreacted diethyl acetylenedicarboxylate which in turn reacted with intermediate 63 to produce the major products (Scheme 96). Unsubstituted hydrazine was very much reactive compared to substituted hydrazines. The catalyst was recycled for six cycles with almost unaltered catalytic activity. This catalyst was also used to prepare pyrano[3,2-c]coumarin and 4H-chromene.
Scheme 94. The use of CuFe2O4 magnetic nanoparticles for the synthesis of 3-methyl-1,4-dihydropyrano[2,3-c]pyrazoles.
![Scheme 94. The use of CuFe2O4 magnetic nanoparticles for the synthesis of 3-methyl-1,4-dihydropyrano[2,3-c]pyrazoles.](/cms/asset/c8e15ac9-f901-44cc-8ac1-bf134ca2fbd6/gpol_a_1584576_c0094_b.jpg)
Scheme 95. Preparation of dihydropyrano[2,3-c]pyrazole-3-carboxylate derivatives in the presence of CuFe2O4 magnetic nanoparticles.
![Scheme 95. Preparation of dihydropyrano[2,3-c]pyrazole-3-carboxylate derivatives in the presence of CuFe2O4 magnetic nanoparticles.](/cms/asset/66121ba6-c376-4a5e-a83f-ade22630ffb4/gpol_a_1584576_c0095_b.jpg)
Titanium dioxide nanosized particles was used in reaction of hydrazine hydrate, ethyl acetoacetate, aryl aldehyde, and malononitrile for preparation of 6-amino-4-aryl-3-methyl-1,4-dihydropyrano[2,3-c]pyrazole-5-carbonitriles (68) at room temperature under solvent-free conditionsCitation134 (Scheme 97).
Scheme 97. The use of nano titanium dioxide for preparation of 6-amino-4-aryl-3-methyl-1,4-dihydropyrano[2,3-c]pyrazole-5-carbonitriles.
![Scheme 97. The use of nano titanium dioxide for preparation of 6-amino-4-aryl-3-methyl-1,4-dihydropyrano[2,3-c]pyrazole-5-carbonitriles.](/cms/asset/09670c99-0801-4bb9-95a6-495680c581e0/gpol_a_1584576_c0097_b.jpg)
Saha et al.Citation135 have utilized ZrO2 nanoparticles as a catalyst in a green solvent (water–ethanol) at room temperature for the synthesis of pyrano[2,3-c]pyrazole (Scheme 98). The surface of ZrO2 NPs possibly contains active hydroxyl, oxide and Zr+4, to act as Lewis acids or bases. The catalytic activity and tetragonal plane of the ZrO2 nanoparticles remained unchanged after the 10th cycle. This protocol was also applied to the synthesis of benzylpyrazolyl coumarin derivatives.
Scheme 98. Application of ZrO2 nanoparticles as catalyst in the synthesis of pyrano[2,3-c]pyrazoles.
![Scheme 98. Application of ZrO2 nanoparticles as catalyst in the synthesis of pyrano[2,3-c]pyrazoles.](/cms/asset/9f072f85-596a-4783-8d36-81a20a4237e0/gpol_a_1584576_c0098_b.jpg)
Magnetic nanoparticles (MNPs) have reported as attractive solid supports for immobilization of homogeneous catalysts. Cesium carbonate supported on hydroxyapatite coated Ni0.5Zn0.5Fe2O4 magnetic nanoparticles (Ni0.5Zn0.5Fe2O4@Hap-Cs2CO3) was utilized as magnetically separable, green and recyclable heterogeneous catalyst for the synthesis of pyranopyrazole derivativesCitation136 (Scheme 99). The reactions were carried out at room temperature in 50:50 water/ethanol. Ni0.5Zn0.5Fe2O4@Hap-Cs2CO3 was separated from the reaction mixture by external magnetic field and efficiently reused at least six runs without any loss of its catalytic activity.
A biochemical and ecofriendly method was reported for the synthesis of Ag nanoparticles using an aqueous leaf extract of readily accessible Cinnamomum tamala as reducing and stabilizing agents.Citation137 These Ag nanoparticles were utilized to catalyze the synthesis of pyranopyrazoles in water (Scheme 100). The authors extended the reaction scope, by performing the reaction with phenylhydrazine instead of hydrazine hydrate, under similar reaction conditions, the reactions were completed at 60 °C. The green nature and ease of recovery and reusability of the catalyst, are the advantages of this protocol.
ZnS nanoparticles, as a heterogeneous catalyst, were applied to the synthesis of 4H-pyrano[2,3-c]pyrazoles at the room temperature using grinding methodCitation138 (Scheme 101). The ZnS nanoparticles were synthesized by hydrothermal method, and can be easily recovered and reused for five runs without significant loss of its catalytic activity.
In 2015,Citation139 BF3 bonded nano Fe3O4 (BF3/MNPs), as heterogeneous and reusable catalyst was used for the preparation of 1,4-dihydropyrano[2,3-c]pyrazole derivatives (Scheme 102). In this report, BF3/MNPs nanoparticles were prepared at three calcination temperature and characterized by various techniques.
Scheme 102. The use of BF3/MNPs nanoparticles for preparation of 1,4-dihydropyrano[2,3-c]pyrazole derivatives.
![Scheme 102. The use of BF3/MNPs nanoparticles for preparation of 1,4-dihydropyrano[2,3-c]pyrazole derivatives.](/cms/asset/22a184ae-71b4-42ea-983d-f5bcf4f14ac1/gpol_a_1584576_c0102_b.jpg)
The Fe3O4@SiO2 core-shell nanocatalyst were prepared and applied for four-component coupling reaction of aromatic aldehydes, malononitrile, ethyl acetoacetate and hydrazine hydrate in water/ethanol mixture at 70 °CCitation140 (Scheme 103). The nanocatalyst was recovered, and reused five times without significant loss in catalytic activity.
Scheme 103. Synthesis of pyran[2,3-c]pyrazoles in the presence of Fe3O4@SiO2 core-shell nanocatalyst.
![Scheme 103. Synthesis of pyran[2,3-c]pyrazoles in the presence of Fe3O4@SiO2 core-shell nanocatalyst.](/cms/asset/27204c74-0239-4c87-bd07-dafd5bde1e2a/gpol_a_1584576_c0103_b.jpg)
The titanomagnetite nanoparticles (Fe3-xTixO4) have been used as support for the synthesis of a magnetic acidic catalyst. These nanoparticles were functionalized with sulfonic acid groups in order to prepare the Fe3-xTixO4@SO3H nanoparticles.Citation141 The synthesized acidic nanoparticles were used as recyclable heterogeneous catalyst for the synthesis of 1,4-dihydropyrano[2,3-c]pyrazoles (Scheme 104). They also used the catalyst for the preparation of 4H-chromenes.
Sadjadi et al.Citation142 have reported a heterogeneous hybrid catalyst by functionalization of halloysite clay nanotubes (HNTs) by γ-aminopropyltriethoxysilane and then immobilization of a Keggin type heteropolyacid, phosphotungstic acid. They applied this hybrid catalyst to synthesis of 3-methyl-4-phenyl-1,4-dihydropyrazolo[4',3':5,6]pyrano[2,3-d]- pyrimidine-5,7-(6H,8H)-dione derivatives (69) via four-component domino reaction of barbituric acid, hydrazine hydrate, ethyl acetoacetate and benzaldehyde in aqueous media (Scheme 105). This heterogeneous catalyst was also separated and reused at least three times with negligible loss of activity. This protocol can be extended to non-conventional methods such as microwave and ultrasonic irradiations.
Scheme 105. Synthesis of 3-methyl-4-phenyl-1,4-dihydropyrazolo[4',3':5,6]pyrano[2,3-d]pyrimidine-5,7(6H,8H)-dione derivatives.
![Scheme 105. Synthesis of 3-methyl-4-phenyl-1,4-dihydropyrazolo[4',3':5,6]pyrano[2,3-d]pyrimidine-5,7(6H,8H)-dione derivatives.](/cms/asset/0dd13a05-413b-4fe3-9354-1d9f5fa56861/gpol_a_1584576_c0105_b.jpg)
In 2017,Citation143 zwitterionic sulfamic acid functionalized nanoclay (MMT-ZSA) was prepared by montmorillonite K-10 as template, 3-aminopropyltriethoxysilane as linker and chlorosulfonic acid as a SO3H source (Scheme 106). MMT-ZSA as a reusable catalyst (five times) was applied to the synthesis of dihydropyrano[2,3-c]pyrazoles and spiro[indoline-3,4'-pyrano[2,3-c]pyrazole] derivatives via the multicomponent reaction of hydrazine hydrate (or phenyl hydrazine), malononitrile, β-keto ester, and carbonyl compounds (1,2-di ketones and benzaldehyde derivatives) under solvent-free conditions (Scheme 107).
Ag/TiO2 nanothin films as a green, robust, and recyclable catalyst was used for preparation of dihydropyrano[2,3-c]pyrazole derivatives in EtOH/H2O at 70 °CCitation144 (Scheme 108). This protocol was also utilized in the synthesis of 4,4-(arylmethylene)bis(1H-pyrazol-5-ol).
In 2017,Citation145 on water chemoselective synthesis of pyrano[2,3-c:6,5-c']dipyrazol]-2-one derivatives (70) was achieved by using Ag NPs decked GO composite (Ag NPs/rGO composite) via reaction of different carbonyl compounds with 3-methyl-1-phenyl-1H-pyrazol-5(4H)-one at room temperature (Scheme 109). In this report “the higher and well-dispersed Lewis acid sites of Ag NPs/GO composite enhances the reactivity of reactant and intermediate towards the Knovengeal-Michael addition sequence followed by ensuing cyclization.” This protocol indicates significant selectivity for pyranodipyrazolones over arylmethylene bispyrazolols and arylmethylenepyrazolones. The catalyst could be recovered and reused at least seven times without significant loss of catalytic activity.
Polyphosphoric acid supported on silica coated Ni0.5Zn0.5Fe2O4 (Ni0.5Zn0.5Fe2O4–PPA) nanoparticles as green and recyclable (at least six times) heterogeneous catalyst was applied to the synthesis of 5-cyano-1,4-dihydropyrano[2,3-c]pyrazoles in waterCitation146 (Scheme 110).
Scheme 110. The use of Ni0.5Zn0.5Fe2O4 (Ni0.5Zn0.5Fe2O4–PPA) for preparation of 5-cyano-1,4-dihydropyrano[2,3-c]pyrazoles.
![Scheme 110. The use of Ni0.5Zn0.5Fe2O4 (Ni0.5Zn0.5Fe2O4–PPA) for preparation of 5-cyano-1,4-dihydropyrano[2,3-c]pyrazoles.](/cms/asset/b86bdf8d-f431-4aa2-9fcf-44f9637c5fbd/gpol_a_1584576_c0110_b.jpg)
In 2018,Citation147 nanosilica from wheat straw-an agricultural waste was utilized as an heterogeneous and recyclable green catalyst for the synthesis of pyrano[2,3-c]pyrazole derivatives in aqueous medium at 80 °C (Scheme 111).
In 2018, Moosavi-zare et al.,Citation148 prepared a pyranopyrazole derivative by condensation reaction of benzaldehyde, ethyl acetoacetate, malononitrile, and hydrazine hydrate in the presence of FeCl2, which then reacted with salicylaldehyde to afford nano‐Fe [phenylsalicylaldiminemethylpyranopyrazole]Cl2 (nano‐[Fe‐PSMP]Cl2) (Scheme 112). The prepared nano‐Schiff base complex was applied as a catalyst for the synthesis of pyranopyrazoles under solvent-free conditions at 100 °C (Scheme 113). The catalyst was recovered and reused successfully for the next run.
Ghorbani-Vaghei et al.149 have prepared hexamethylenetetramine‐functionalized magnetic nanoparticles (Fe3O4@SiO2‐HMTA‐SO3H MNPs) catalyst through the reaction of silanol groups, on the surface of silica‐coated Fe3O4 magnetic nanoparticles, with (3‐chloropropyl)triethoxysilane followed by treating with hexamethylenetetramine and chlorosulfonic acid. The catalyst was applied for the synthesis of pyranopyrazole compounds via reaction of dimethyl acetylenedicarboxylate, malononitrile, aldehydes, and hydrazine hydrate at room temperature and under solvent-free conditions (Scheme 114). The authors studied the recyclability of the catalyst in the model reaction. The results showed that in each cycle the catalyst activity decreased insignificantly.
Arghan et al.Citation150 have reported deposition of polyacrylamide on nanomagnetite surface via in situ polymerization of acryl amide to form n-Fe3O4/PAM nanocomposite. The amide (-CONH2) groups could be converted to amine (-NH2) groups through Hofmann degradation to introduce n-Fe3O4/PVAm as an heterogeneous base catalyst. Authors have applied the obtained organic-inorganic nanocomposite to solvent-free syntheses of dihydropyrano[2,3-c]pyrazole derivatives at room temperature (Scheme 115). In the reaction, n-Fe3O4/PVAm nanocomposite with its free primary amino groups behaves as a base catalyst.
Fe3O4@HNTs-PEI nanocomposite was utilized for the preparation of pyranopyrazole derivatives (Scheme 116).Citation151 For preparation of this heterogeneous catalyst, a green magnetic nanocatalyst based on natural clay was fabricated. Magnetic nanoparticles were loaded on halloysite nanotubes (HNTs) and poly(ethyleneimine) (PEI) was covalently grafted onto the surface of HNTs. This catalyst can be recovered from the reaction mixture by using an external magnet and reused for at least eight times without any significant decrease in catalytic activity.
Scheme 116. synthesis of dihydropyrano[2,3-c]pyrazole derivatives in the presence of Fe3O4@HNTs-PEI nano-catalyst.
![Scheme 116. synthesis of dihydropyrano[2,3-c]pyrazole derivatives in the presence of Fe3O4@HNTs-PEI nano-catalyst.](/cms/asset/9ef02d89-414f-47cb-8adf-422b79039178/gpol_a_1584576_c0116_b.jpg)
Synthesis of spiro[indoline-3,4′-pyrano[2,3-c]pyrazole] and pyranopyrazole derivatives was studied using β-CD/EP as a stationary microvessel and basic heterogeneous catalyst at 100 °C under solvent-free condition (Schemes 117 and 118).Citation152 β-Cyclodextrin-epichlorohydrin nanosponge polymer, β-CD/EP, was prepared by stepwise polymerization of β-cyclodextrin with epichlorohydrin under basic conditions.
Scheme 118. Synthesis of spiro[indoline-3,4′-pyrano[2,3-c]pyrazole] derivatives using β-CD/EP polymer.
![Scheme 118. Synthesis of spiro[indoline-3,4′-pyrano[2,3-c]pyrazole] derivatives using β-CD/EP polymer.](/cms/asset/ea7a4e12-54ef-48bb-996c-12920c479337/gpol_a_1584576_c0118_b.jpg)
Vitamin B1 supported on Fe2O3@SiO2 NPs as a magnetic organo-nanocatalyst was used to prepare 1,4-dihydropyrano[2,3-c]pyrazole and spiro[indoline-3,4'-pyrano[2,3-c]pyrazole derivatives from aromatic aldehyde/isatin, malononitrile, hydrazine hydrate, and ethyl acetoacetate in water-ethanol mixture at room temperature (Scheme 119).Citation153 Vitamin B1 supported on Fe2O3@SiO2 nanoparticles coordinates with carbonyl oxygen and nitrile nitrogen thus increasing the electrophilic character of the respective carbons for facile nucleophilic attack. In this study, after the completion of the reaction, the catalyst was recycled and reused up to six runs without any significant loss of its activity.
The novel Nd-Salen Schiff base complex immobilized mesoporous silica was synthesized by adsorption of NdCl3 on mesoporous-SiO2 (Nd-SM), then was applied, as catalyst, for the synthesis of pyranopyrazoles, at 70 °C under solvent-free conditions (Scheme 120).Citation154 The catalyst was recycled and reused for the next reaction (up to six times).
Using electrolysis
Elinson et al.Citation155 have utilized electrocatalytic system for the preparation of spirocyclic [indole-3,4'-pyrano[2,3-c]pyrazoles] compounds (71) in ethanol in an undivided cell in the presence of sodium bromide as an electrolyte (Scheme 121). The presented mechanism was outlined in Scheme 122. The catalytic cycle begins with the deprotonation of a molecule of alcohol at the cathode, which leads to the formation of an ethoxide anion. The subsequent reaction between the ethoxide anion and malononitrile gives rise to the malononitrile anion which continues synthesis of the target molecule.
Using microwave conditions
In 2015,Citation156 a series of pyranopyrazoles was synthesized by the traditional heating using two-step and one-step protocols and then by microwave-assisted (MWA) multicomponent condensation of ethyl acetoacetate, hydrazine, malonodinitrile, and aldehydes (Scheme 123). Authors compared thier methods first based on the yields and then based on the time taken for the completion of the reactions. They found that although the traditional methods gave slightly better yields, but the MWA syntheses lead to substantial reduction in reaction times.
Scheme 123. Preparation of pyranopyrazoles by the traditional heating techniques and microwave-assisted reaction.
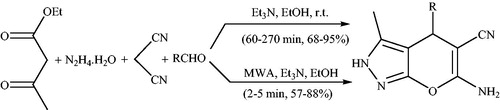
Sharma et al.Citation157 have synthesized a series of compounds having the pyranopyrazole pharmacophore by the traditional method and then by microwave assisted reaction (Scheme 124). The microwave strategy required substantially shorter reaction times (3–5 min) as compared to the conventional method (1–2 h). The prepared molecules were also tested in vitro for their cytotoxic activity against the Hep3B cancer cell lines.
In 2013,Citation158 a regio- and stereoselective procedure was developed for a three-component domino intermolecular Knoevenagel–intermolecular hetero-Diels–Alder reaction, to prepare indolyl- and quinolylpyrano[2,3-c]pyrazoles from corresponding heteroarylaldehyde, pyrazolone and enol ether in ionic liquid triethylammonium acetate (TEAA) under microwave (MW) irradiation (Schemes 125 and 126). Two-dimensional NMR NOESY (nuclear overhauser effect spectroscopy) experiments confirm the cis-orientation of the two pyran ring hydrogens; one is attached to the anomeric carbon and the second to carbon bearing a heteroaryl ring.
Sharifi Aliabadi et al.Citation159 have utilized an imidazole-based ionic liquid, 1-butyl-3-methylimidazolium hydroxide ([bmim][OH]), as a heterogeneous catalyst for the synthesis of pyranopyrazoles (Scheme 127) under microwave irradiation.This organo-solid catalyst was used for the cascade synthesis of colorful pyrano[2,3-c]pyrazoles in a microwave oven at a power of 230 W. The authors also evaluated the antioxidant activity of the products using a 2,2-azinobis(3-ethylbenzothiazoline-sulfonate) (ABTS) assay. The antioxidant activity of the synthesized products was compared with both vitamin E and ascorbic acid. The products showed a higher antioxidant activity using this method.
Using ultrasound technology
Dandia et al.Citation160 have used ZnS nanoparticle in water under ultrasonic irradiation to synthesize spiro[indoline-3,4′-pyrano[2,3-c]pyrazole] derivatives (Scheme 128). After reaction course, the ZnS NPs was recycled and reused without any apparent loss of activity. Spiro[chromene-4,3′-indoline] derivatives were also prepared by the same method.
Zou et al.Citation161 have described spiro[indoline-3,4′-pyrano[2,3-c]pyrazole] derivatives (72) by four-component reaction of hydrazine, β-keto ester, isatin, and malononitrile or ethyl cyanoacetate catalyzed by piperidine under ultrasonic irradiations (Scheme 129). In this report, this synthesis was confirmed to follow the group-assistant-purification chemistry (GAP), which can avoid traditional chromatography and recrystallization purifications.
Scheme 129. Synthesis of spiro[indoline-3,4′-pyrano[2,3-c]pyrazole] derivatives in the presence of piperidine under ultrasonic irradiations.
![Scheme 129. Synthesis of spiro[indoline-3,4′-pyrano[2,3-c]pyrazole] derivatives in the presence of piperidine under ultrasonic irradiations.](/cms/asset/2b80df28-2caf-4fe0-9505-917602848303/gpol_a_1584576_c0129_b.jpg)
In 2013,Citation162 the preparation of multisubstituted dihydropyrano[2,3-c]pyrazole derivatives, via four-component reaction of 4-hydrazinobenzoic acid, β-ketoesters, aromatic aldehydes, and malononitrile under ultrasonic irradiation was reported (Scheme 130).
Scheme 130. Preparation of dihydropyrano[2,3-c]pyrazole derivatives in the presence of CAN and under ultrasonic irradiation.
![Scheme 130. Preparation of dihydropyrano[2,3-c]pyrazole derivatives in the presence of CAN and under ultrasonic irradiation.](/cms/asset/3231d490-158a-442a-b68e-435dbcf21ce9/gpol_a_1584576_c0130_b.jpg)
Gujar et al.Citation163 have described the synthesis of dihydropyrano[2,3-c]pyrazole derivatives using molecular sieves (MS 4 Å) as catalyst in ethanol under reflux and ultrasonic irradiation (Scheme 131). The authors compared the efficiency of the catalyst with other aluminates and silicate based catalysts. The reusability of MS 4 Å, as a heterogeneous catalyst for the same reactants, was examined. It was observed that the recovered catalyst works with the same efficiency up to thrid run, while in the fourth and fifth runs the product yield was decreased slightly.
Scheme 131. Preparation of dihydropyrano[2,3-c]pyrazole using molecular sieves (MS 4 Å) under reflux and ultrasonic irradiation.
![Scheme 131. Preparation of dihydropyrano[2,3-c]pyrazole using molecular sieves (MS 4 Å) under reflux and ultrasonic irradiation.](/cms/asset/039374d1-ed93-4faa-9eaa-9165b96d5554/gpol_a_1584576_c0131_b.jpg)
In 2015,Citation164 a catalyst-free, green synthetic protocol was reported for the synthesis of pyranopyrazoles via multicomponent reaction of aromatic aldehydes, hydrazine monohydrate and ethyl acetoacetate and malononitrile in water under ultrasonic irradiation (Scheme 132). Tetrahydropyrazolopyridine was also prepared by this protocol.
Mn doped zirconia (Mn/ZrO2) was utilized as an environmental-friendly catalyst for an ultrasound mediated four-component synthesis of pyrano[2,3-c]pyrazole-3-carboxylate/pyrano[2,3-c]pyrazole-5-carbonitriles by coupling reaction of dimethylacetylenedicarboxylate/ethyl acetoacetate, hydrazine hydrate, malononitrile, and aromatic aldehyde in aqueous ethanol and at room temperatureCitation165 (Scheme 133). Reusability of the catalyst was checked by the model reaction. The recovered catalyst was employed in six consecutive runs, and the decrease in activity was marginal.
Conclusions
This review has covered the multicomponent strategies reported for the synthesis of pyranopyrazole and spiro-pyranopyrazole derivatives during the period of 2005 to 2018. Since the most reports contains pyrano[2,3-c]pyrazoles, so this review covers reported multicomponent strategies to prepare pyrano[2,3-c]pyrazoles and spiro-pyrano[2,3-c]pyrazoles. Several MCRs for the preparation of pyrano[2,3-c]pyrazoles have been reported. These reactions were performed in classical and nonclassical conditions. In recent years using greener protocols by employing sonochemical conditions, MW-assisted technologies, and using solvent-free conditions and green solvent especially water as a solvent, heterogeneous catalysis especially nanocatalyst, ionic liquids, have made access to these significant heterocyclic compounds.
Additional information
Funding
References
- A. Stachulski, N. Berry, A. Lilian Low, S. Moores, E. Row, D. Warhurst, I. Adagu, and J. Rossignol, “Identification of Isoflavone Derivatives as Effective Anticryptosporidial Agent in Vitro and Vivo,” Journal of Medicinal Chemistry 49, no. 4 (2006): 1450–4.
- W. Sun, L. D. Cama, E. T. Birzin, S. Warrier, L. Locco, R. Mosley, M. L. Hammond, and S. P. Rohrer, “6H-Benzo[c]chromen-6-one Derivatives as Selective ERb Agonists,” Bioorganic & Medicinal Chemistry Letters 16, no. 6 (2006): 1468–72.
- M. Elinson, A. Dorofeev, S. Feducovich, S. Gorbunov, R. Nasybullin, N. Stepanov, and G. Nikishin, “Electrochemically Induced Chain Transformation of Salicylaldehydes and Alkyl Cyanoacetates into Substituted 4H-Chromenes, ”Tetrahedron Letters 47, no. 43 (2006): 7629–32.
- Green, G., J. Evans, and A. Vong, “Pyrans and their Benzo Derivatives: Applications,” in Comprehensive Heterocyclic Chemistry II, edited by A.R. Katritzky, C.W. Rees, E.P.F. Scriven, Vol. 5 (Pergamon Press: Oxford, 1995), 469.
- J. A. Wang, O. Novaro, X. Bokhimi, T. Lopez, R. Gomez, J. Navarrete, M. E. Llanos, and E. L. Salinas, “Structural Defects and Acidic and Basics in Sol-Gel MgO,” The Journal of Physical Chemistry B 101, no. 38 (1997): 7448–51.
- K. L. Kees, J. J. Fitzgerald, K. E. Steine, J. F. Mattes, B. Mihan, T. Tosi, D. Mondoro, and M. L. McCaleb, “New Potent Antihyperglycemic Agents in db/db Mice: Synthesis and Structure-activity Relationship Studies of (4-Substituted Benzyl)(Trifluoromethyl)pyrazoles and-Pyrazolones,” Journal of Medicinal Chemistry 39, no. 20 (1996): 3920–8.
- M. J. Genin, C. Biles, B. J. Keiser, S. M. Poppe, S. M. Swaney, W. G. Tarpley, Y. Yagi, and D. L. Romero, “Novel 1,5-Diphenylpyrazole Nonnucleoside HIV-1 Reverse Transcriptase Inhibitors with Enhanced Activity versus the Delavirdine-Resistant P236L Mutant: Lead Identification and SAR of 3- and 4-Substituted Derivatives,” Journal of Medicinal Chemistry 43, no. 5 (2000): 1034–40.
- S. S. Chobe, G. G. Mandawad, O. S. Yemul, S. S. Kinkar, and B. S. Dawane, “An Efficient One-Pot Synthesis of Substituted Pyrazolo [3,4 b:4',3'e]Pyridine Derivatives via the Hantzch Three Component Condensation Using Bleaching Earth Catalyst and Their InvitroAntimicrobial Evaluation,” International Journal of ChemTech Research 3 (2011): 938–43.
- X. -H. Yang, P. -H. Zhang, Z. -M. Wang, F. Jing, Y. -H. Zhou, and L. -H. Hu, “Of Lignin Related High-Added-Value 2H,4H-Dihydro-Pyrano[2,3-c]Pyrazoles and 1H,4H-Dihydropyrano[2,3-c]Pyrazoles,” Industrial Crops and Production 52 (2014): 413–19.
- S. Mandha, S. Siliveri, M. Alla, V. Bommena, M. Bommineni, and S. Balasubramanian, “Eco-Friendly Synthesis and Biological Evaluation of Substituted Pyrano[2,3-c]Pyrazoles,” Bioorganic & Medicinal Chemistry Letters 22, no. 16 (2012): 5272–8.
- P. W. Smith, S. L. Sollis, P. D. Howes, P. C. Cherry, I. D. Starkey, K. N. Cobley, H. Weston, J. Scicinski, A. Merritt, A. Whittington, et al. “Dihydropyrancarboxamides Related to Zanamivir: A New Series of Inhibitors of Influenza Virus Sialidases. 1. Discovery, Synthesis, Biological Activity, and Structure Activity Relationships of 4-Guanidino- and 4-Amino-4H-pyran-6-Carboxamides,” Journal of Medicinal Chemistry 41, no. 6 (1998): 787–97.
- M. Kidwai, S. Saxena, M. Rahman Khan, and S. Thukral, “Aqua Mediated Synthesis of Substituted 2-Amino-4H-Chromenes and in Vitro Study as Antibacterial Agents,” Bioorganic & Medicinal Chemistry Letters 15, no. 19 (2005): 4295–8.
- S. Patil, J. Wang, X. Li, J. Chen, T. Jones, A. Hosni-Ahmed, R. Patil, W. Seibel, W. Li, and D. Miller, “New Substituted 4H-Chromenes as Anticancer Agents,” Bioorganic & Medicinal Chemistry Letters 22, no. 13 (2012): 4458–61.
- A. Kumar, P. Lohan, D. K. Aneja, G. K. Gupta, D. Kaushik, and O. Prakash, “Design, Synthesis, Computational and Biological Evaluation of Some New Hydrazino Derivatives of DHA and Pyranopyrazoles,” European Journal of Medicinal Chemistry 50 (2012): 81–9.
- J. W. Pavlik, V. Ervithayasuporn, J. C. MacDonald, and S. Tantayanon, “The Photochemistry of Some Pyranopyrazoles,” Arkivoc 8 (2008): 57–68.
- K. Nicolaou, S. Snyder, T. Montagnon, and G. Vassilikogiannakis, “The Diels–Alder Reaction in Total Synthesis,” Angewandte Chemie International Edition 41, no. 10 (2002): 1668–98.
- L. Huang, M. Hour, C. Teng, and S. Kuo, “Synthesis and Antiplatelet Activities of N-Arylmethyl-3,4-dimethylpyrano[2,3-c]pyrazol-6-one Derivatives,” Chemical & Pharmaceutical Bulletin 40, no. 9 (1992): 2547–51.
- J.-L. Wang, D. Liu, Z.-J. Zhang, S. Shan, X. Han, S. M. Srinivasula, C. M. Croce, E. S. Alnemri, and Z. Huang, “Structure-Based Discovery of an Organic Compound That Binds Bcl-2 Protein and Induces Apoptosis of Tumor Cells,” Proceedings of the National Academy of Sciences of the United States of America 97, no. 13 (2000): 7124–9.
- S. C. Kuo, L. J. Huang, and H. Nakamura, “Studies on Heterocyclic Compounds. 6. Synthesis and Analgesic and anti-inflammatory Activities of 3,4-Dimethylpyrano[2,3-c]pyrazol-6-one Derivatives,” Journal of Medicinal Chemistry 27, no. 4 (1984): 539–44.
- M. E. A. Zaki, H. A. Soliman, O. A. Hiekal, and A. E. Rashad, “Pyrazolopyranopyrimidines as a Class of anti-inflammatory Agents,” Zeitschrift Für Naturforschung C 61, no. 1-2 (2006): 1–5.
- E.-S H. El-Tamany, F. A. El-Shahed, and B. H. Mohamed, “Synthesis and Biological Activity of Some Pyrazole Derivatives,” Journal of Serbian Chemical Society 64, (1999): 21–30. https://www.shd.org.rs/JSCS/Vol64/No1.htm#Synthesis.
- F. M. Abdelrazek, P. Metz, N. H. Metwally, and S. F. El-Mahrouky, “Synthesis and Molluscicidal Activity of New Cinnoline and Pyrano [2,3-c]pyrazole Derivatives,” Archiv Der Pharmazie 339, no. 8 (2006): 456–560.
- K. Qvortrup, J. F. Jensen, M. S. Sørensen, I. Kouskoumvekaki, R. K. Petersen, O. Taboureau, K. Kristiansen, and T. E. Nielsen, and “Synthesis and Biological Evaluation of Dihydropyrano-[2,3-c]pyrazoles as a New Class of PPARγ Partial Agonists,” PLoS One 12, no. 2 (2017): e0162642.
- Gesson, J., N. Fonteneau, M.Mondon, S.Charbit, H. Ficheux, and F. Schutze, U. S. Patent, 6, 965,039 B2, 2005.
- N. Foloppe, L. Fisher, R. Howes, A. Potter, A. Robertson, and A. Surgenor, “Identification of Chemically Diverse Chk1 Inhibitors by Receptor-based Virtual Screening,” Bioorganic & Medicinal Chemistry 14, no. 14 (2006): 4792–802.
- R. Maggi, R. Ballini, G. Sartori, and R. Sartorio, “Basic Alumina Catalyzed Synthesis of Substituted 2-Amino-2-chromenes via Three-Component Reaction,” Tetrahedron Letters 45, no. 11 (2004): 2297–9.
- L. F. Tietze, “Domino Reactions in Organic Synthesis,” Chemical Reviews 96, no. 1 (1996): 115–36.
- L. F. Tietze, and F. Haunert, “Domino Reaction in Organic Synthesis. An Approach to Efficiency, Elegance, Ecological Benefit, Economic Advantage and Preservation of our Resources in Chemical Transformations,” in Stimulating Concepts in Chemistry, edited by F. Votle, J. F. Stoddart, M. Shibasaki (Wiley: Weinheim, 2000), 39–64.
- D. Enders, M. R. M. Huttl, C. Grondal, and G. Raabe, “Control of Four Stereocentres in a Triple Cascade Organocatalytic Reaction,” Nature 441, no. 7095 (2006): 861–3.
- R. Sheldon, “Selective Catalytic Synthesis of Fine Chemicals: Opportunities and Trends,” Journal of Moleular Catalysis A 107, no. 1-3 (1996): 75–83.
- Li, C., and T. Chan, Organic Reactions in Aqueous Media (Wiley: New York, NY, 1997).
- P. Grieco, Organic Synthesis in Water (Blackie Academic and Professional: London, 1998).
- K. Kandhasamy, and V. Gnanasambandam, “Multicomponent Reactions in Water,” Current Organic Chemistry 13, (2009): 11820–41.
- K. Z. Andrade, and L. M. Alves, “Environmentally Benign Solvents in Organic Synthesis: Current Topics,” Current Organic Chemistry 9, no. 2 (2005): 195–218.
- H. H. Otto, “Darstellung Einiger 4H‐Pyrano[2.3‐c]Pyrazolderivate,” Archiv Der Pharmazie 307, no. 6 (1974): 444–7.
- H. H. Otto, and H. Schmelz, “Heterocyclen Durch Michael‐Reaktionen, 5. Mitt. Nucleophile Additionen an 4‐Aryliden‐Pyrazolone,” Archiv Der Pharmazie 312, no. 6 (1979): 478–86.
- G. V. Klokol, S. G. Krivokolysko, V. D. Dyachenko, and V. P. Litvinov, “Aliphatic Aldehydes in the Synthesis of Condensed 4-Alkyl(cycloalkyl)-2-amino-3-cyano-4H-Pyrans,” Chemistry of Heterocyclic Compounds 35, no. 10 (1999): 1183–6.
- A. Shaabani, A. Sarvary, A. H. Rezayan, and S. Keshipour, “Synthesis of Fully Substituted Pyrano[2,3-c]pyrazole Derivatives via a Multicomponent Reaction of Isocyanides,” Tetrahedron 65, no. 17 (2009): 3492–5.
- A. S. Nagarajan, and B. S. R. Reddy, “Synthesis of Substituted Pyranopyrazoles under Neat Conditions via a Multicomponent Reaction,” Synlett 12 (2009): 2002–4.
- A. Moshtaghi Zonouz, I. Eskandari, and H. R. Khavasi, “A Green and Convenient Approach for the Synthesis of Methyl 6-Amino-5-cyano-4-aryl-2,4-dihydropyrano[2,3-c]pyrazole-3-carboxylates via a One-Pot, Multicomponent Reaction in Water,” Tetrahedron Lett 53, no. 41 (2012): 5519–22.
- D. Pore, P. Patil, D. Gaikwad, P. Hegade, J. Patil, and K. Undale, “Green Access to Novel Spiro Pyranopyrazole Derivatives,” Tetrahedron Letters 54, no. 44 (2013): 5876–8.
- M. Bihani, P. P. Bora, and Gh Bez, “A Practical Catalyst-Free Synthesis of 6-Amino-4 Alkyl/aryl-3-methyl-2,4-dihydropyrano[2,3-c]pyrazole-carbonitrile in Aqueous Medium,” Journal of Chemistry (2013): Article ID 920719.
- M. Koohshari, M. Dabiri, and P. Salehi, “Catalyst-Free Domino Reaction in Water/Ethanol: An Efficient, Regio- and Chemoselective One-Pot Multicomponent Synthesis of Pyranopyrazole Derivatives,” RSC Advances 4, no. 21 (2014): 10669–71.
- C. Yu, C. Yao, T. Li, and X. Wang, “An Aqueous, Catalyst-Free and Three-Component Synthesis of 6-Amino-3-(Trifluoromethyl)-1,4-dihydro-1-phenyl-4-arylpyrano[2,3-c]pyrazole-5-Carbonitriles,” Research on Chemical Intermediates 40, no. 4 (2014): 1537–44.
- M. G. Dekamin, M. Alikhani, A. Emami, H. Ghafuri, and S. Javanshir, “An Efficient Catalyst- and Solvent-Free Method for the Synthesis of Medicinally Important Dihydropyrano[2,3-c]pyrazole Derivatives Using Ball Milling Technique,” Journal of the Iranian Chemical Society 13, no. 3 (2016): 591–6.
- K. T. Patil, D. K. Jamale, N. J. Valekar, P. T. Patil, P. P. Warekar, G. B. Kolekar, and P. V. Anbhule, “Uncatalyzed Four-component Synthesis of Pyrazolopyranopyrimidine Derivatives and Their Antituberculosis Activities,” Synthetic Communications 47, no. 2 (2017): 111–20.
- B. Baran, O. Berezyuk, and V. Golonzhka, “Water Systems after Magnetic Field Action,” Environmental Research, Engineering and Management 38 (2006): 19–23.
- N. Gang, L. S. St-Pierre, and M. A. Persinger, “Water Dynamics following Treatment by One Hour 0.16 Tesla Static Magnetic Fields Depend on Exposure Volume,” Water 3, (2012) : 122–31.
- K. Higashitani, J. Oshitani, and N. Ohmura, “Effects of Magnetic Field on Water Investigated with Fluorescent Probes,” Colloids and Surfaces A 109, (1996): 167–73. DOI: 10.1016/0927-7757(95)03483-8.
- M. Bakherad, A. Keivanloo, M. Gholizadeh, R. Doosti, and M. Javanmardi, “Using Magnetized Mater as a Solvent for a Green, Catalyst-Free, and Efficient Protocol for the Synthesis of Pyrano[2,3-c]pyrazoles and Pyrano[4',3':5,6]pyrazolo[2,3-d]Pyrimidines,” Research on Chemical Intermediates 43, no. 2 (2017): 1013–29.
- V. Ramesh, S. Shanmugam, and N. S. Devi, “An Efficient Five-Component Synthesis of Thio Ether Containing Dihydropyrano[2,3-c]pyrazoles: A Green Domino Strategy,” New Journal of Chemistry 40, no. 12 (2016): 9993–10001.
- H. V. Chavan, S. B. Babar, R. U. Hoval, and B. P. Bandgar, “Rapid One-Pot, Four-Component Synthesis of Pyranopyrazoles Using Heteropolyacid under Solvent-Free Condition,” Bulletin of the Korean Chemical Society 32, no. 11 (2011): 3963–6.
- V. L. Gein, T. M. Zamaraeva, and P. A. Slepukhin, “A Novel Four-Component Synthesis of Ethyl 6-Amino-4-aryl-5-cyano-2,4-dihydropyrano[2,3-c]pyrazole-3-Carboxylates,” Tetrahedron Letters 55, no. 33 (2014): 4525–8.
- R. Hekmatshoar, M. M. Heravi, S. Sadjadi, H. A. Oskooie, and F. F. Bamoharram, “Catalytic Performance of Preyssler Heteropolyacid, [NaP5W30O110]14− in Liquid Phase Alkylation of Phenol with 1-Octene,” Catalysis Communications 9, no. 5 (2008): 837–41.
- A. Hafizi, A. Ahmadpour, M. M. Heravi, and F. F. Bamoharram, “The Application of Silica-Supported Preyssler HPA as a Heterogeneous and Green Catalyst for the Alkylation of Benzene,” Petroleum Science and Technology 2 (2014): 1022–7.
- Y. B. Gu, R. P. Wei, X. Q. Ren, and J. Wang, “Cs Salts of 12-Tungstophosphoric Acid Supported on Dealuminated USY as Catalysts for Hydroisomerization of n-Heptane,” Catalysis Letters 113, no. 1-2 (2007): 41–5.
- A. Javid, A. Khojastehnezhad, H. Eshghi, F. Moeinpour, F. F. Bamoharram, and J. Ebrahimi, “Synthesis of Pyranopyrazoles Using a Magnetically Separable Modified Preyssler Heteropoly Acid,” Organic Preparations and Procedures International 48, no. 5 (2016): 377–84.
- A. R. Moosavi-Zare, M. A. Zolfigol, R. Salehi-Moratab, and E. Noroozizadeh, “Catalytic Application of 1-(Carboxymethyl)pyridinium Iodide on the Synthesis of Pyranopyrazole Derivatives,” Journal of Molecular Catalysis A: Chemical 415, (2016): 144–50.
- G. M. Reddy, and J. R. Garcia, “Synthesis of Pyranopyrazoles under Eco-Friendly Approach by Using Acid Catalysis,” Journal of Heterocyclic Chemistry 54, no. 1 (2017): 89–94.
- G. M. Reddy, J. R. Garcia, V. H. Reddy, A. K. Kumari, G. V. Zyryanov, and G. Yuvaraja, “An Efficient and Green Approach: One Pot, Multi Component, Reusable Catalyzed Synthesis of Pyranopyrazoles and Investigation of Biological Assays,” Journal of Saudi Chemical Society 23 (2019): 263–273.
- A. R. Moosavi-Zare, H. Afshar-Hezarkhani, and M. M. Rezaei, “Tandem Four-Component Condensation Reaction of Aryl Aldehydes with Ethyl Acetoacetate, Malononitrile, and Hydrazine Hydrate Using Boric Acid in Water as an Efficient and Green Catalytic System,” Polycyclic Aromatic Compounds (2017). doi:10.1080/10406638.2017.1382541.
- R. G. Redkin, L. A. Shemchuk, V. P. Chernykh, O. V. Shishkin, and S. V. Shishkin, “Synthesis and Molecular Structure of Spirocyclic 2-Oxindole Derivatives of 2-Amino-4H-Pyran Condensed with the Pyrazolic Nucleus,” Tetrahedron 63, no. 46 (2007): 11444–50.
- G. Vasuki, and K. Kumaravel, “Rapid Four-Component Reactions in Water: Synthesis of Pyranopyrazoles,” Tetrahedron Letters 49, no. 39 (2008): 5636–8.
- F. Lehmann, M. Holm, and S. Laufer, “Three-Component Combinatorial Synthesis of Novel Dihydropyrano[2,3-c]Pyrazoles,” Journal of Combinatorial Chemistry 10, no. 3 (2008): 364–7.
- Y. M. Litvinov, A. A. Shestopalov, L. A. Rodinovskaya, and A. M. Shestopalov, “New Convenient Four-Component Synthesis of 6-Amino-2,4-dihydropyrano[2,3-c]pyrazol-5-carbonitriles and One-Pot Synthesis of 6-Aminospiro[(3H)-Indol-3,4'-pyrano[2,3-c]pyrazol]-(1H)-2-on-5′-Carbonitriles,” Journal of Combinatorial Chemistry 11, no. 5 (2009): 914–19.
- S. Ahadi, Z. Yasaei, and A. Bazgir, “A Clean and One-Pot Synthesis of Spiroindoline-Pyranopyrazoles,” Journal of Heterocyclic Chemistry 47, no. 5 (2010): 1090–4.
- S. H. S. Azzam, and M. A. Pasha, “Simple and Efficient Protocol for the Synthesis of Novel Dihydro-1H-pyrano[2,3-c]pyrazol-6-ones via a One-Pot Four-Component Reaction,” Tetrahedron Letters 53, no. 50 (2012): 6834–7.
- M. Wu, Q. Feng, D. Wan, and J. Ma, “CTACl as Catalyst for Four-Component, One-Pot Synthesis of Pyranopyrazole Derivatives in Aqueous Medium,” Synthetic Communications 43, no. 12 (2013): 1721–6.
- S. Pal, Md N. Khan, Sh Karamthulla, S. Abbas, and L. H. Choudhury, “One-Pot Four-Component Reaction for the Efficient Synthesis of Spiro[indoline-3,4'-pyrano[2,3-c]pyrazole]-3'-carboxylate Derivatives,” Tetrahedron Letters 54, no. 40 (2013): 5434–40.
- H. Kiyania, H. A. Samimi, F. Ghorbani, and S. Esmaieli, “One-Pot, Four-Component Synthesis of Pyrano[2,3-c]pyrazoles Catalyzed by Sodium Benzoate in Aqueous Medium,” Current Chemistry Letters 2, (2013) : 197–206.
- K. Th. Jayabal, and P. Paramasivan, “An Expedient Four-component Domino Protocol for the Regioselective Synthesis of Highly Functionalized Pyranopyrazoles and Chromenopyrazoles via Nitroketene-N,S-acetal Chemistry under Solvent-Free Condition,” Tetrahedron Letters 55, no. 12 (2014): 2010–14.
- M. J. Daly, and B. J. Price, Progress in Medicinal Chemistry in Ellis, Vol. 20, edited by G. P. West (Elsevier: Amsterdam, 1983), 337.
- Y. Ootsuka, H. Morita, and H. Mori. Jpn. Kokai Tokyo Koho JP 07157465A2, 1995.
- K. P. Moder, Eur. Pat. Appl EP 515121 A1, 1992.
- M. V. Dmitriev, P. S. Silaichev, T. V. Sal’nikova, P. V. Melyukhin, and A. N. Maslivets, “Three-Component Spiro Heterocyclization of 1H-pyrrole-2,3-diones with Malononitrile and Pyrazolones. Crystal and Molecular Structure of a Spiro[pyrano[2,3-c]-Pyrazole-4,3′-Pyrrole],” Russian Journal of Organic Chemistry 51, no. 6 (2015): 884–7.
- B. M. Chougala, S. Samundeeswari, M. Holiyachi, L. A. Shastri, S. Dodamani, S. Jalalpure, S. R. Dixit, Sh, D. Joshi, and V. A. Sunagar, “Synthesis, Characterization and Molecular Docking Studies of Substituted 4-Coumarinylpyrano[2,3-c]pyrazole Derivatives as Potent Antibacterial and anti-inflammatory Agents,” European Journal of Medicinal Chemistry 125, (2017): 101–16.
- V. L. Gein, T. M. Zamaraeva, and P. A. Slepukhin, “Diethyl Oxalacetate Sodium Salt as a Reagent to Obtain Functionalized Spiro[indoline-3,4’-pyrano[2,3-c]pyrazoles],” Tetrahedron Letter 58, no. 2 (2017): 134–6.
- S. Kanchithalaivan, S. Sivakumar, R. R. Kumar, P. Elumalai, Q. N. Ahmed, and A. K. Padala, “Four-Component Domino Strategy for the Combinatorial Synthesis of Novel 1,4-Dihydropyrano[2,3-c]pyrazol-6-Amines,” ACS Combinatorial Science 15, no. 12 (2013): 631–8.
- A. Khazaei, M. A. Zolfigol, F. Karimitabar, I. Nikokar, and A. R. Moosavi-Zare, “N,2-Dibromo-6-chloro-3,4-dihydro-2H-benzo[e][1,2,4] thiadiazine-7-sulfonamide 1,1-dioxide: An Efficient and Homogeneous Catalyst for One-pot Synthesis of 4H-Pyran, Pyranopyrazole and Pyrazolo[1,2-b]phthalazine Derivatives under Aqueous Media,” RSC Advances 5, no. 87 (2015): 71402–12.
- M. Beerappa, and K. Shivashankar, “Four-Component Synthesis of Highly Functionalized Pyrano[2,3-c]pyrazoles from Benzyl Halides,” Synthetic Communications 48, no. 2 (2018): 146–54.
- M. B. Madhusudana Reddy, V. P. Jayashankara, and M. A. Pasha, “Glycine-Catalyzed Efficient Synthesis of Pyranopyrazoles via One-Pot Multicomponent Reaction,” Synthetic Communications 40, (2010): 2930–4.
- Y. A. Tayade, S. A. Padvi, Y. B. Wagh, and D. S. Dalal, “β-Cyclodextrin as a Supramolecular Catalyst for the Synthesis of Dihydropyrano[2,3-c]pyrazole and Spiro[indoline-3,4’-pyrano[2,3-c]pyrazole] in Aqueous Medium,” Tetrahedron Letters 56, no. 19 (2015): 2441–7.
- K. Kanagaraj, and K. Pitchumani, “Solvent-Free Multicomponent Synthesis of Pyranopyrazoles: Per-6-amino-β-cyclodextrin as a Remarkable Catalyst and Host,” Tetrahedron Letters 51, no. 25 (2010): 3312–16.
- A. Siddekha, A. Nizam, and M. A. Pasha, “An Efficient and Simple Approach for the Synthesis of Pyranopyrazoles Using Imidazole (Catalytic) in Aqueous Medium, and the Vibrational Spectroscopic Studies on 6-Amino-4-(4'-methoxyphenyl)-5-cyano-3-methyl-1-phenyl-1,4-dihydropyrano[2,3-c]pyrazole Using Density Functional Theory,” Spectrochimica Acta, Part A 81 (2011): 431–40.
- G. Brahmachari, and B. Banerjee, “Facile and One-pot Access to Diverse and Densely Functionalized 2-Amino-3-cyano-4H-pyrans and Pyran-annulated Heterocyclic Scaffolds via an Eco-Friendly Multicomponent Reaction at Room Temperature Using Urea as a Novel Organo-Catalyst,” ACS Sustainable Chemistry & Engineering 2, no. 3 (2014): 411–22.
- S. Gogoi, and C.-G. Zhao, “Organocatalyzed Enantioselective Synthesis of 6-Amino-5-cyanodihydropyrano[2,3-c]Pyrazoles,” Tetrahedron Letters 50, no. 19 (2009): 2252–5.
- H. Quan, X. Zhang, H. Lu, and Zh Huang, “Synthesis and Acid Solution Properties of a Novel Betaine Zwitterionic Surfactant,” Central Europen Journal of Chemistry 10 (2012) : 1624–32.
- D. P. Acharya, and H. Kunieda, “Wormlike Micelles in Mixed Surfactant Solutions,” Advances in Colloid and Interface Science 123 (2006): 401–13.
- E. Sarmiento-Gomez, D. Lopez-Diaz, and R. Castillo, “Microrheology and Characteristic Lengths in Wormlike Micelles Made of a Zwitterionic Surfactant and SDS in Brine,” The Journal of Physical Chemistry B 114, no. 38 (2010): 12193–202.
- F. Tamaddon, and M. Alizadeh, “A Four-component Synthesis of Dihydropyrano[2,3-c]pyrazoles in a Dew Water-Based Worm-Like Micellar Medium,” Tetrahedron Letters 55, no. 26 (2014): 3588–91.
- M. A. Zolfigol, M. Tavasoli, A. R. Moosavi-Zare, P. Moosavi, H. G. Kruger, M. Shiri, and V. Khakyzadeh, “Synthesis of Pyranopyrazoles Using Isonicotinic Acid as a Dual and Biological Organocatalyst,” RSC Advances 3, no. 48 (2013): 25681–5.
- R. D. Kamble, B. S. Dawane, O. S. Yemul, A. B. Kale, and S. D. Patil, “Bleaching Earth Clay (pH 12.5): a Green Catalyst for Rapid Synthesis of Pyranopyrazole Derivatives via a Tandem Three-Component Reaction,” Research on Chemical Intermediates 39, no. 8 (2013): 3859–66.
- M. M. Heravi, F. Mousavizadeh, N. Ghobadi, and M. Tajbakhsh, “A Green and Convenient Protocol for the Synthesis of Novel Pyrazolopyranopyrimidines via a One-pot, Four-component Reaction in Water,” Tetrahedron Letters 55, no. 6 (2014): 1226–8.
- A. S. Waghmare, and Sh. S. Pandit, “DABCO Catalyzed Rapid One-pot Synthesis of 1,4-Dihydropyrano[2,3-c]pyrazole Derivatives in Aqueous Media,” Journal of Saudi Chemistry Society 21, no. 3 (2017): 286–90.
- E. Safari, and A. Hasaninejad, “One-pot, Multicomponent Synthesis of Novel Bis-Spiro Pyranopyrazole Derivatives in the Presence of DABCO as an Efficient and Reusable Solid Base Catalyst,” Organic Supramolecular Chemistry 3 (2018): 3529–3533.
- H. R. Shaterian, and M. Kangani, “Synthesis of 6-Amino-4-aryl-3-methyl-1,4-dihydropyrano- [2,3-c]pyrazole-5-carbonitriles by Heterogeneous Reusable Catalysts,” Research on Chemical Intermediates 40, no. 5 (2014): 1997–2005.
- S. Ambethkar, V. Padmini, and N. Bhuvanesh, “A Green and Efficient Protocol for the Synthesis of Dihydropyrano [2,3-c]pyrazole Derivatives via a One-pot, Four-component Reaction by Grinding Method,” Journal of Advanced Research 6, no. 6 (2015): 975–85.
- R.-Y. Guo, Z.-M. An, L.-P. Mo, S.-T. Yang, H.-X. Liu, S.-X. Wang, and Z.H. Zhang, “Meglumine Promoted One-pot, Four-component Synthesis of Pyranopyrazole Derivatives,” Tetrahedron 69, no. 47 (2013): 9931–8.
- M. Kangani, N. Hazeri, M. T. Mghsoodlou, S. M. Habibi-Khorasani, and S. Salahi, “Green Synthesis of 1,4-Dihydropyrano[2,3-c]pyrazole Derivatives Using Maltose as Biodegradable Catalyst,” Research on Chemical Intermediates 41, no. 4 (2015): 2513–19.
- M. Kangani, N. Hazeri, M. T. Maghsoodlou, Kh Khandan-Barani, M. Kheyrollahi, and F. Nezhadshahrokhabadi, “Green Procedure for the Synthesis of 1,4-Dihydropyrano[2,3-c]pyrazoles Using Saccharose,” Journal of the Iranian Chemical Society 12, no. 1 (2015): 47–50.
- Ch-F. Zhou, J.-J. Li, and W.-K. Su, “Morpholine Triflate Promoted One-pot, Four-component Synthesis of Dihydropyrano[2,3-c]Pyrazoles,” Chinese Chemical Letters 27, no. 11 (2016): 1686–90.
- K. S. Dalal, Y. A. Tayade, Y. B. Wagh, D. R. Trivedi, D. S. Dalal, and B. L. Chaudhari, “Bovine Serum Albumin Catalyzed One-pot, Three-component Synthesis of Dihydropyrano[2,3-c]pyrazole Derivatives in Aqueous Ethanol,” RSC Advances 6, no. 18 (2016): 14868–79.
- R. Ghorbani-Vaghei, and S. M. Malaekehpoor, “N-Bromosulfonamides Catalyzed Synthesis of New Spiro[indoline-3,4'-pyrano[2,3-c]pyrazole] Derivatives,” Journal of Heterocyclic Chemistry 54, no. 1 (2017): 465–72.
- A. R. Moosavi-Zare, M. A. Zolfigol, and A. Mousavi-Tashar, “Synthesis of Pyranopyrazole Derivatives by in Situ Generation of Trityl Carbocation under Mild and Neutral Media,” Research on Chemical Intermediates 42, no. 10 (2016): 7305–12.
- M. Fatahpour, F. N. Sadeh, N. Hazeri, M. T. Maghsoodlou, and M. Lashkari, “Aspirin: An Efficient Catalyst for Synthesis of Bis(pyrazol-5-ols), Dihydropyrano[2,3-c]pyrazoles and Spiropyranopyrazoles in an Environmentally Benign Manner,” Journal of the Iranian Chemical Society 14, no. 9 (2017): 1945–56.
- Lu Zh, J. Xiao, D. Wang, and Y. Li, “An Efficient One-Pot Five-Component Tandem Sequential Approach for the Synthesis of Pyranopyrazole Derivatives via Suzuki Coupling and Multicomponent Reaction,” Asian Journal of Organic Chemistry 4, (2015) : 482–6.
- S. Y. Ebrahimipour, Z. R. Ranjabr, E. T. Kermani, B. P. Amiri, H. Amiri Rudbari, A. Sacca, and F. Hoseinzade, “A Mixed-Ligand Ternary Complex of Nickel(II): Synthesis, Characterization and Catalytic Investigation for the Synthesis of Pyranopyrazoles,” Transition Metal Chemistry 40, no. 1 (2015): 39–45.
- K. K. Gangu, S. Maddila, S. N. Maddila, and S. B. Jonnalagadda, “Novel Iron Doped Calcium Oxalates as Promising Heterogeneous Catalysts for One-pot Multicomponent Synthesis of Pyranopyrazoles,” RSC Advances 7, no. 1 (2017): 423–32.
- M. Bihani, P. P. Bora, G. Bez, and H. Askari, “Amberlyst A21 Catalyzed Chromatography-Free Method for Multicomponent Synthesis of Dihydropyrano[2,3-c]pyrazoles in Ethanol,” ACS Sustainable Chemistry & Engineering 1, no. 4 (2013): 440–7.
- M. A. Chaudhari, J. B. Gujar1, D. S. Kawade, N. R. Jogdand, and M. S. Shingare, “A Highly Efficient and Sustainable Synthesis of Dihydropyrano[2,3-c]pyrazoles Using Polystyrene Supported p-Toluenesulfonic Acid as Reusable Catalyst,” Cogent Chemistry 1 (2015): 1063830.
- K. Karnakar, K. Ramesh, K. H. V. Reddy, B. S. P. A. Kumar, J. B. Nanubonula, and Y. V. D. Nageswar, “A Novel One-Pot Four-Component Reaction for the Efficient Synthesis of Spiro[indoline-3,4'-pyrano[2,3-c]pyrazole]-3'-carboxylate and Trifluoromethylated Spiro[indole-3,4'-pyrano[2,3-c]pyrazole] Derivatives Using Recyclable PEG-400,” New Journal of Chemistry 39, no. 11 (2015): 8978–83.
- V. Singh, S. Kaur, V. Sapehiyia, J. Singh, and G. L. Kad, “Microwave Accelerated Preparation of [bmim][HSO4] Ionic Liquid: An Acid Catalyst for Improved Synthesis of Coumarins,” Catalysis Communications 6, no. 1 (2005): 57–60.
- R. Zhang, X. Meng, Z. Liu, J. Meng, and C. Xu, “Isomerization of n-Pentane Catalyzed by Acidic Chloroaluminate Ionic Liquids,” Industrial & Engineering Chemistry Research 47, no. 21 (2008): 8205–10.
- A. R. Hajipour, F. Rafiee, and A. E. Ruoho, “Oxidation of Benzylic Alcohols to Their Corresponding Carbonyl Compounds Using KIO4 in Ionic Liquid by Microwave Irradiation,” Synthetic Communications 36, no. 17 (2006): 2563–8.
- A. R. Hajipour, F. Rafiee, and A. E. Ruoho, “Facile and Selective Oxidation of Benzylic Alcohols to Their Corresponding Carbonyl Compounds with Sodium Nitrate in the Presence of Brønsted Acidic Ionic Liquids,” Synlett 2007, no. 7 (2007): 1118–20.
- J. M. Khurana, B. Nand, and S. Kumar, “Rapid Synthesis of Polyfunctionalized Pyrano[2,3-c]pyrazoles via Multicomponent Condensation in Room-Temperature Ionic Liquids.” Synthetic Communications 41, (2011): 405–10.
- J. Ebrahimi, A. Mohammadi, V. Pakjoo, E. Bahramzade, and A. Habibi, “Highly Efficient Solvent-Free Synthesis of Pyranopyrazoles by a Brønsted-Acidic Ionic Liquid as a Green and Reusable Catalyst,” Journal of Chemical Sciences 124, no. 5 (2012): 1013–17.
- A. R. Moosavi-Zare, M. A. Zolfigol, E. Noroozizadeh, M. Tavasoli, V. Khakyzadeh, and A. Zare, “Synthesis of 6-Amino-4-(4-methoxyphenyl)-5-cyano-3-methyl-1-phenyl-1,4-dihydropyrano[2,3-c]pyrazoles Using Disulfonic Acid Imidazolium Chloroaluminate as a Dual and Heterogeneous Catalyst,” New Journal of Chemistry 37, no. 12 (2013): 4089–94.
- H. Veisi, A. A. Manesh, N. Khankhani, and R. Ghorbani-Vaghei, “Protic Ionic Liquid [TMG][Ac] as an Efficient, Homogeneous and Recyclable Catalyst for One-Pot Four-Component Synthesis of 2H-Indazolo[2,1-b]phthalazine-triones and Dihydro-1H-pyrano[2,3-c]pyrazol-6-Ones,” RSC Advances 4, no. 48 (2014): 25057–62.
- D. Habibi, A. Shamsian, and D. Nematollahi, “Synthesis of Pyranopyrazoles, Benzopyrans, Amino-2-chromenes and Dihydropyrano[c]chromenes Using Ionic Liquid with Dual Brønsted Acidic and Lewis Basic Sites,” Chemical Papers 69 (2015): 586–95.
- A. R. Hajipour, M. Karimzadeh, and H. Tavallaei, “Fast Synthesis of Pyrano[2,3-c]pyrazoles: Strong Effect of Brönsted and Lewis Acidic Ionic Liquids,” Journal of the Iranian Chemical Society 12, no. 6 (2015): 987–91.
- M. Mamaghani, R. Hossein Nia, F. Shirini, Kh Tabatabaeian, and M. Rassa, “An Efficient and Eco-friendly Synthesis and Evaluation of Antibactrial Activity of Pyrano[2,3-c]pyrazole Derivatives,” Medicinal Chemistry Research 24, no. 5 (2015): 1916–26.
- P. Farokhian, M. Mamaghani, N. O. Mahmoodi, and Kh Tabatabaeian, “A Green and Practical Method for the Synthesis of Novel Pyrano[2,3-c]pyrazoles and Bis-pyrano[2,3-c]pyrazoles Using Sulfonic Acid-functionalized Ionic Liquid,” Journal of the Iranian Chemical Society 15, no. 1 (2018): 11–16.
- M. Zakeri, M. M. Nasef, T. Kargaran, A. Ahmad, E. Abouzari-Lotf, and J. Asadi, “Synthesis of Pyrano[2,3-c]pyrazoles by Ionic Liquids under Green and Eco-safe Conditions,” Research on Chemical Intermediates 43, no. 2 (2017): 717–28.
- F. Rigi, and H. R. Shaterian, “Prompted One-pot Four-component Synthesis of Pyrazolopyranopyrimidines, 3-Methyl-4-aryl-4,5-dihydro-1H-pyrano[2,3-c]pyrazol-6-ones, and 1,6-Diamino-2-oxo-1,2,3,4-tetrahydropyridine-3,5-Dicarbonitriles,” Polycyclic Aromatic Compounds 37, no. 4 (2017): 314–26.
- E. L. Smith, A. P. Abbott, and K. S. Ryder, “Deep Eutectic Solvents (DESs) and Their Applications,” Chemical Reviews 114, no. 21 (2014): 11060–82.
- M. R. Bhosle, L. D. Khillare, S. T. Dhumal, and R. A. Mane, “A Facile Synthesis of 6-Amino-2H,4H-pyrano[2,3-c]pyrazole-5-4 Carbonitriles in Deep Eutectic Solvent,” Chinese Chemical Letters 27, no. 3 (2016): 370–4.
- A. Moshtaghi Zonouz, and D. Moghani, “Green and Highly Efficient Synthesis of Pyranopyrazoles in Choline Chloride/urea Deep Eutectic Solvent,” Synthetic Communications 46, no. 3 (2016): 220–5.
- M. Gholami Dehbalaei, N. Foroughifar, H. Pasdar, A. Khajeh-Amiri, N. Foroughifar, and M. Alikarami, “Choline Chloride Based Thiourea Catalyzed Highly Efficient, Eco-friendly Synthesis and anti-bacterial Evaluation of Some New 6-Amino-4-aryl-2,4-dihydro-3-phenylpyrano[2,3-c]pyrazole-5-carbonitrile Derivatives,” Research on Chemical Intermediates, 43, no. 5 (2017): 3035–51.
- V. Polshettiwar, and R. S. Varma, “Green Chemistry by Nano-Catalysis,” Green Chemistry 12, no. 5 (2010): 743–54.
- M. Babaie, and H. Sheibani, “Nanosized Magnesium Oxide as a Highly Effective Heterogeneous Base Catalyst for the Rapid Synthesis of Pyranopyrazoles via a Tandem Four-component Reaction,” Arabian Journal of Chemistry 4, no. 2 (2011): 159–62.
- M. A. E. Aleem, and A. A. El-Remaily, “Synthesis of Pyranopyrazoles Using Magnetic Fe3O4 Nanoparticles as Efficient and Reusable Catalyst,” Tetrahedron 70 (2014) : 2971–5.
- K. Pradhan, S. Paul, and A. R. Das, “Magnetically Retrievable Nano Crystalline CuFe2O4 Catalyzed Multicomponent Reaction: A Facile and Efficient Synthesis of Functionalized Dihydropyrano[2,3-c]pyrazole, Pyrano[3,2-c]coumarin and 4H-chromene Derivatives in Aqueous Media,” Catalysis Science & Technology 4, no. 3 (2014): 822–31.
- H. R. Shaterian, and K. Azizi, “Mild, Four-component Synthesis of 6-Amino-4-aryl-3-methyl-1,4-dihydropyrano[2,3-c]pyrazole-5-carbonitriles Catalyzed by Titanium Dioxide Nano-Sized Particles,” Research on Chemical Intermediates 40, no. 2 (2014): 661–7.
- A. Saha, S. Payra, and S. Banerjee, “One-pot Multicomponent Synthesis of Highly Functionalized Bio-active Pyrano[2,3-c]pyrazole and Benzylpyrazolyl Coumarin Derivatives Using ZrO2 Nanoparticles as a Reusable Catalyst,” Green Chemistry 17, no. 5 (2015): 2859–66.
- Farid Moeinpour, and Amir Khojastehnezhad, “Cesium Carbonate Supported on Hydroxyapatite Coated Ni0.5Zn0.5Fe2O4 Magnetic Nanoparticles as an Efficient and Green Catalyst for the Synthesis of Pyrano[2,3-c]Pyrazoles,” Chinese Chemical Letters 26, no. 5 (2015): 575–9.
- S. Yadav, and J. M. Khurana, “Cinnamomum tamala Leaf Extract-Mediated Green Synthesis of Ag Nanoparticles and Their Use in Pyranopyrazles Synthesis,” Chinese Chemical Letters 36, no. 7 (2015): 1042–6.
- A. V. Borhade, and B. K. Uphade, “ZnS Nanoparticles as an Efficient and Reusable Catalyst for Synthesis of 4H-pyrano[2,3-c]Pyrazoles,” Journal of the Iranian Chemical Society 12, no. 6 (2015): 1107–13.
- M. Abdollahi-Alibeik, A. Moaddeli, and K. Masoomi, “BF3 Bonded Nano Fe3O4 (BF3/MNPs): an Efficient Magnetically Recyclable Catalyst for the Synthesis of 1,4-Dihydropyrano[2,3-c]pyrazole Derivatives,” RSC Advances 5, no. 91 (2015): 74932–9.
- E. Soleimani, M. Jafarzadeh, P. Norouzi, J. Dayou, C. S. Sipaut, R. F. Mansa, and P. Saei, “Synthesis of Pyranopyrazoles Using Magnetically Recyclable Heterogeneous Iron Oxide-Silica Core-Shell Nanocatalyst,” Journal of the Chinese Chemical Society 62, no. 12 (2015): 1155–62.
- D. Azarifar, and Y. Abbasi, “Sulfonic Acid-Functionalized Magnetic Fe3-xTixO4 Nanoparticles: New Recyclable Heterogeneous Catalyst for One-pot Synthesis of Tetrahydrobenzo[b]pyrans and Dihydropyrano[2,3-c]pyrazole Derivatives,” Synthetic Communications 46, no. 9 (2016): 745–58.
- S. Sadjadi, M. M. Heravi, and M. Daraie, “Heteropolyacid Supported on Amine-Functionalized Halloysite Nano Clay as an Efficient Catalyst for the Synthesis of Pyrazolopyranopyrimidines via Four-component Domino Reaction,” Research on Chemical Intermediates 43, no. 4 (2017): 2201–14.
- J. Safari, and M. Ahmadzadeh, “ “Zwitterionic Sulfamic Acid Functionalized Nanoclay: A Novel Nanocatalyst for the Synthesis of Dihydropyrano[2,3-c]pyrazoles and Spiro[indoline-3,4´-Pyrano[2,3-c]pyrazole] Derivatives,” Journal of the Taiwan Institute of Chemical Engineers 74 (2017) : 14–24.
- M. Fatahpour, F. Noori Sadeh, N. Hazeri, M. T. Maghsoodlou, M. S. Hadavi, and S. Mahnaei, “Ag/TiO2 Nano-Thin Films as Robust Heterogeneous Catalyst for One-pot, Multicomponent Synthesis of Bis(pyrazol-5-ol) and Dihydropyrano[2,3-c]pyrazole Analogs,” Journal of Saudi Chemical Society 21, no. 8 (2017): 998–1006.
- A. Dandia, S. L. Gupta, A. Indora, P. Saini, V. Parewa, and K. S. Rathore, “Ag NPs Decked GO Composite as a Competent and Reusable Catalyst for ‘ON WATER’ Chemoselective Synthesis of Pyrano[2,3-c:6,5-c']dipyrazol]-2-Ones,” Tetrahedron Letters 58, no. 12 (2017): 1170–5.
- F. Moeinpour, and A. Khojastehnezhad, “Polyphosphoric Acid Supported on Ni0.5Zn0.5Fe2O4 Nanoparticles as a Magnetically-Recoverable Green Catalyst for the Synthesis of Pyranopyrazoles,” Arabian Journal of Chemistry 10 (2017): S3468–S74.
- Kh G. Patel, N. M. Misra, R. H. Vekariya, and R. R. Shettigar, “One-Pot Multicomponent Synthesis in Aqueous Medium of 1,4-Dihydropyrano[2,3-c]pyrazole-5-carbonitrile and Derivatives Using a Green and Reusable Nano-SiO2 Catalyst from Agricultural Waste,” Research on Chemical Intermediates 44, no. 1 (2018): 289–304.
- A. R. H. Moosavi‐Zare, H. Goudarziafshar, and Kh Saki, “Synthesis of Pyranopyrazoles Using Nano‐Fe‐[Phenylsalicylaldiminemethylpyranopyrazole]Cl2 as a New Schiff Base Complex and Catalyst,” Applied Organometallic Chemistry 32 (2018): e3968.
- R. Ghorbani‐Vaghei, and V. Izadkhah, “Preparation and Characterization of Hexamethylenetetramine‐functionalized Magnetic Nanoparticles and Their Application as Novel Catalyst for the Synthesis of Pyranopyrazole Derivatives,” Applied Organometallic Chemistry 32 (2018): e4025.
- M. Arghan, N. Koukabi, and E. Kolvari, “Polyvinyl Amine as a Modified and Grafted Shell for Fe3O4 Nanoparticles: as a Strong Solid Base Catalyst for the Synthesis of Various Dihydropyrano[2,3-c]pyrazole Derivatives and the Knoevenagel Condensation,” Journal of Saudi Chemical Society 23, (2018): 150–61.
- Z. Hajizadeh, and A. Maleki, “Poly(ethylene Imine)-Modified Magnetic Halloysite Nanotubes: A Novel, Efficient and Recyclable Catalyst for the Synthesis of Dihydropyrano[2,3-c]pyrazole Derivatives,” Molecular Catalysis 460, (2018) : 87–93.
- M. Jafari Nasab, A. R. Kiasat, and R. Zarasvandi, “β-Cyclodextrin Nanosponge Polymer: a Basic and Eco-Friendly Heterogeneous Catalyst for the One-Pot Four-Component Synthesis of Pyranopyrazole Derivatives under Solvent-Free Conditions,” Reaction Kinetics, Mechanisms and Catalysis 124, no. 2 (2018): 767–78.
- N. Rahman, G. S. Nongthombam, J. W. S. Rani, R. Nongrum, G. K. Kharmawlong, and R. Nongkhlaw, “An Environment-Friendly Magnetic Organo-Nanomaterial as a Potent Catalyst in Synthesis of Pyranopyrazole Derivatives,” Current Organocatalysis 5, no. 2 (2018): 150–61.
- R. A. Rather, and Z. N. Siddiqui, “Synthesis, Characterization and Application of Nd-Salen Schiff Base Complex Immobilized Mesoporous Silica in Solvent Free Synthesis of Pyranopyrazoles,” Journal of Organomettallic Chemistry 868 (2018): 164–74.
- M. N. Elinson, A. S. Dorofeev, F. M. Miloserdov, and G. I. Nikishin, “Electrocatalytic Multicomponent Assembling of Isatins, 3-Methyl-2-pyrazolin-5-ones and Malononitrile: Facile and Convenient Way to Functionalized Spirocyclic[indole-3,4'-pyrano[2,3-c]pyrazole] System,” Molecular Diversity 13, no. 1 (2009): 47–52.
- P. Shukla, A. Sharma, S. Anthal, and R. Kant, “Synthesis of Functionalized Pyrazolopyran Derivatives: Comparison of Two-step vs. One-step vs. Microwave-Assisted Protocol and X-ray Crystallographic Analysis of 6-Amino-1,4-dihydro-3-methyl-4-phenylpyrano[2,3-c]pyrazole-5-Carbonitrile,” Bulletin of Materials Science 38, no. 5 (2015): 1119–27.
- A. Sharma, R. Chowdhury, S. Dash, B. Pallavi, and P. Shukla, “Fast Microwave Assisted Synthesis of Pyranopyrazole Derivatives as New Anticancer Agents,” Current Microwave Chemistry 3, no. 1 (2016): 78–84.
- N. J. Parmar, H. A. Barad, B. R. Pansuriya, and N. P. Talpada, “A Highly Efficient, Rapid One-pot Synthesis of Some New Heteroaryl Pyrano[2,3-c]pyrazoles in Ionic Liquid under Microwave-Irradiation,” RSC Advances 3, no. 21 (2013): 8064–70.
- R. Sharifi Aliabadi, and N. O. Mahmoodi, “Green and Efficient Synthesis of Pyranopyrazoles Using [bmim][OH-] as an Ionic Liquid Catalyst in Water under Microwave Irradiation and Investigation of Their Antioxidant Activity,” RSC Advances 6, no. 89 (2016): 85877–84.
- A. Dandia, V. Parewa, A. K. Jain, and K. S. Rathore, “Step-Economic, Efficient, ZnS Nanoparticle-Catalyzed Synthesis of Spirooxindole Derivatives in Aqueous Medium via Knoevenagel Condensation Followed by Michael Addition,” Green Chemistry 13, no. 8 (2011): 2135–45.
- Y. Zou, Y. Hu, H. Liu, and D. Shi, “Rapid and Efficient Ultrasound-assisted Method for the Combinatorial Synthesis of Spiro[indoline-3,4′-Pyrano[2,3-c]pyrazole] Derivatives,” ACS Combinatorial Science 14, no. 1 (2012): 38–43.
- K. Ablajan, W. Liju, Y. Kelimu, and F. Jun, “Cerium Ammonium Nitrate (CAN)-Catalyzed Four-component One-pot Synthesis of Multi-substituted Pyrano[2,3-c]pyrazoles under Ultrasound Irradiation,” Molecular Diversity 17, no. 4 (2013): 693–700.
- J. B. Gujar, M. A. Chaudhari, D. S. Kawade, and M. S. Shingare, “Molecular Sieves: An Efficient and Reusable Catalyst for Multicomponent Synthesis of Dihydropyrano [2,3-c] pyrazole Derivatives,” Tetrahedron Letters 55, no. 44 (2014): 6030–3.
- N. Shabalala, R. Pagadala, and S. B. Jonnalagadda, “Ultrasonic-Accelerated Rapid Protocol for the Improved Synthesis of Pyrazoles.” Ultrasonics Sonochemistry 27 (2015): 423–9.
- S. Maddila, S. Gorle, S. Shabalala, O. Oyetade, S. N. Maddila, P. Lavanya, and S. B. Jonnalagadda, “Ultrasound Mediated Green Synthesis of Pyrano[2,3-c]pyrazoles by Using Mn Doped ZrO2,” Arabian Journal of Chemistry (2016). doi:10.1016/j.arabjc.2016.04.016.