Abstract
This study presents indoor and outdoor levels of airborne fine particles (PM2.5), particle bound polycyclic aromatic compounds (PACs) including parent-, alkylated-, nitro-, and oxy-PAHs. Week-long simultaneous measurements were conducted inside and outside 15 occupied homes in southern Sweden during wintertime. The homes were single-family houses or apartments located in urban, semi-urban, and rural areas. The PM2.5 and PACs levels were low compared to studies worldwide. There was great variation in concentrations between sites, which likely is due to proximity to road and traffic intensity. The lower concentrations of nitro and oxy-PAHs compared to parent PAHs in this study, compared to other studies, could possibly be due to lower atmospheric photochemical formation outdoors because the cold climate. This assumption could not be confirmed and need to be further tested. The results point to that particle PAC levels found inside arise primarily from outdoor. This correlation was not as clear for PM2.5. The results of a comparison between residences before and after energy renovation did not indicate an improvement in indoor air regarding PACs. To understand exposure and assess risks it is important to measure wide range of PACs both in gas and particle phase.
Introduction
Air pollution continues to be a significant threat to human health globally. The World Health Organization (WHO) has estimated that around 7 million deaths per year globally are attributable to the joint effects of ambient and household air pollution.Citation1 The impact of air pollution is also significant in Sweden. It has been estimated that exposure to particulate matter (PM) can cause 7600 premature deaths in Sweden.Citation2 PM in the ambient air has been the subject of numerous studies and PM levels have been routinely monitored for many years.Citation2–6 Negative health effects of fine particles (PM2.5) include risk of lung cancer, asthma, as well as increased respiratory and cardiovascular mortality and morbidityCitation6–11 and the International Agency for Research on Cancer (IARC) has classified outdoor pollution particles as carcinogenic to humans (Group 1).Citation12
Gas-particle partitioning has great significance for the environmental fate of organic compounds.Citation13 Temperature, humidity, and wind speed are important environmental parameters for how the gas-particle distribution turns out.Citation13 Number studies point to that not only morphological characteristics of the particle but also the chemical composition, which is then controlled by the gas-particle distribution, plays an important role in the adverse health effects of PM.Citation4 Among the wide range of organic compounds that are associated with PM, polycyclic aromatic hydrocarbons (PAHs), and especially the 16 PAHs defined by the United States Environmental Protection Agency (so called 16 USEPA PAHs) has been studied intensively in different parts of the world.Citation14–25 PAH is a complex mixture of multi-ringed persistent substances with different molecular weights and physicochemical properties. PAHs are formed during the combustion of organic materials, and thus these compounds have many sources both outdoors and indoors, for instance motor vehicle exhaust, domestic burning of wood, coal or biomass, cooking, candle lightening and smoking, and various industrial processes.Citation15,Citation20,Citation26–32 There are several processes that can influence PAHs concentrations; thus, measurements can be challenging. Temperature at sampling location will influence phase change between particle- bound and gas-phase PAHs, there are also several possible depletion mechanisms, such as atmospheric degradation due to photolysis and oxidation processes, where temperature was reported to play a major role.Citation33,Citation34
A group of polycyclic aromatic compounds (PACs) that has been less addressed in environmental studies are the nitrated (nitro-PAH) and oxygenated (oxy-PAH) derivatives of PAHs. This is notable since many nitro- and oxy-PAHs are more toxic than their parent PAHs due to their mutagenicity and carcinogenicity.Citation35,Citation36 These PAH derivatives, like PAHs, can be formed during incomplete combustion processes of organic matter. Once in the atmosphere, they can also be formed through secondary reactions with OH and NO3 radicals and through homogeneous/heterogenous reactions with NO2.Citation37–39 Although, several monitoring campaigns in various regions, have demonstrated the presence of these derivates in the atmosphere,Citation30,Citation37–44 to the best of our knowledge studies on the presence of these PAH derivates in ambient air in Sweden is missing. Furthermore, little attention has been given to indoor environments of these compoundsCitation30 and this can be especially important since people spend most of their time indoors.Citation45 Moreover, several studies have shown that concentration of some air pollutants (e.g. PAH and persistent organic pollutants (POPs)) can be higher indoors than outdoors.Citation28,Citation46 Therefore, in order to evaluate the risk to human health posed by exposure to air toxics, and to identify high-risk populations, it is important to acquire knowledge of contaminant levels and understanding of indoor sources for an increased number of PAH substances.
This study is a part of two bigger projects, one focusing on physicochemical characteristics and toxicity of indoor and outdoor particlesCitation47,Citation48 and People-Environment-Indoor-Renovation-Energy (PEIRE) project, which encompasses different effects of energy renovations and occupants’ behavior on indoor environment quality.Citation49–51 The hypothesis in the bigger projects was that the toxicity of particles indoors (where we spend 90% of the time) is different in comparison to the toxicity of particles outdoors, whereas typically only outdoor particles’ characteristics are used in the epidemiological studies to assess health effect due to exposure to particles. Herein we present the results of a multitude particulate-bound PACs as PACs are important PM component that relates to toxicity of particles and therefore to health effect of exposure to particles. PACs were measured indoors in residences in southern Sweden and were compared with levels measured just outside the buildings. Possible sources of the PACs found in these indoor environments are discussed. Moreover, repeated measurements were made in seven of the residences after energy renovation of the buildings. Levels measured before and after this renovation are compared and discussed.
Material and methods
Sampling campaigns
Week-long simultaneous measurements were conducted inside and just outside 15 occupied homes in southern Sweden during wintertime 2016/2017. The homes were single family houses or apartments. The places of the residences were considered rural, urban, or semi-urban. None of the sites were close to a known outdoor point source where PAH levels can be expected to be elevated, such as an industry, gasoline station or directly next to a motorway. Information of the sampling sites is provided in .
Table 1. Sampling characteristics, indoor and outside 15 residences in southern Sweden.
Two identical sets of instruments for indoor and outdoor measurements were used for a multitude analytical endpoint. Details of the entire instrumental equipment set, and analytical endpoints were presented elsewhere.Citation47 Only the parts relating to sampling relevant for this article are described here. Sampling equipment was placed in specifically designed enclosures to minimize noise and disturbance for the residents, and for the outdoor measurement to protect against weather conditions such as precipitation. Enclosure indoors was typically placed in the living room unless occupants due to practical reasons requested placement in the hall or kitchen. Outdoor enclosure was placed in close vicinity of the indoor sampling site, i.e. either on a balcony or in the garden in case of homes 1–5. In homes 6–15 as these were within the same vicinity, due to practical reasons the outdoor enclosure was kept in one place, i.e. on a balcony of home 9. The distance between indoor and outdoor sampling location for homes 6–15 was within 300 m radius.
Indoors PM2.5 particles were collected on Teflon filters (37 mm, pore size 2 µm, Teflo; Pall Corporation, Port Washington, NY) inside a DustTrack DRX 8533 (2 L min−1 flow, TSI Inc, Shoreview, MN). Outdoors, DustTrack 8520 (TSI Inc., Shoreview, MN) was used, it does not allow simultaneous collection of PM2.5 for post analysis (as in case of DustTrack DRX 8533, used indoors), thus outdoor PM2.5 were collected on Teflon filters (the same brand as describe earlier) in a separate collection line after PM2.5 cyclone at a flow rate of 4 L min−1. Average total air sampling volume per filter was 21.5 m3 for indoor samples and 40.7 m3 for outdoor samples.
Week-long measurements were repeated one year later (winter 2017/2018) in seven of the residences after energy renovation. The renovation was described earlier.Citation49,Citation50 In short, the renovation was focused on reduction of heat losses and balancing the existing mechanical exhaust ventilation (airflows mechanically forced through the kitchen and bathroom fans). Balancing the existing ventilation system was achieved by 1) replacing inlets supplying outdoor air (instead of wide inlets (window like openings) in the living room and bedroom, small inlet vents were installed); 2) better distribution of exhaust fans (situated on the roof) throughout the building (the single exhaust fan on the roof of each building before the renovation was replaced with three, each supplying the area served by one staircase); 3) The kitchen hoods were replaced in all apartments during the renovation. Both before and after the renovation a basic constant airflow was always exhausted via the hood and the residents could manually increase the airflow to a forced flow. Before the renovation the manual control was equipped with timer (switching for 30 or 60 min only), while after renovation there was no time restriction for forced flow control. To reduce the heat losses the following changes were done: 1) extra insulation to the building wall was added to tighten the building envelope and reduce heat transfer; 2) new thermostatic valves were installed on the hydronic radiators and the central heating system was balanced between buildings and flats; 3) the curtain walls, windows, and balcony doors on the first and second floors on the south façade were rebuilt or replaced during the renovation (the windows on the third floor had been replaced a few years earlier). The air exchange rates (AERs) were measured before and after the renovation (details in SI and in Omelekhina et al.Citation49) in 5 apartments out of seven the AER increased slightly after renovation. In the studied apartments AER before the renovation ranged from 0.31 to 0.64 h−1 (median 0.50 h−1) and after renovation from 0.41 to 0.73 h−1 (median 0.55 h−1).
Chemical analysis
The analytical procedures and instrumentation used for PM2.5 and particle-bound PAC analysis are described elsewhereCitation29,Citation52 and in Supplementary Information (SI) and are given here in brief. The filters, diameter 37 mm, pore size 2 µm (Teflo; Pall Corporation, Port Washington, NY) were conditioned for 24 h prior to weighing in a climate chamber controlled for temperature and humidity (23 °C, 50%). All filters were weighed using an MX5 balance (LOD < 11 µg) (Mettler Toledo, Columbus, OH). After gravimetric analysis, the filters were analyzed for their content of particulate PAC compounds. The target PAC compounds were 17 parent PAHs (the 16 priority US EPA PAHs plus perylene), 14 alkylated PAHs, 17 nitrated-PAHs (nitro-PAHs), and 10 oxygenated-PAHs (oxy-PAHs). All chemical classes with target compounds are presented in SI Tables S1 and S2. The filters were extracted by means of a Sonica ultrasonic extractor (Soltec, Milan, Italy) in three mL of dichloromethane for 12 min.Citation29 Two deuterated internal standard (IS) mixtures, containing 16 US EPA PAHs, and four nitro-PAHs, respectively, were added to the samples before extraction. The extract solutions were cleaned through Pasteur pipettes filled with 2 cm of silica gel and sodium sulfate on top, and finally concentrated by purging with gentle stream of nitrogen to a final volume of ca 30 µL.
PAC compounds were separated and detected by means of high-resolution gas chromatography/low-resolution mass spectrometry (HRGC/LRMS). The mass spectrometer (MS) was an Agilent 5975 C coupled to a 7890 A gas chromatograph (GC) (Agilent Technologies, Inc., Santa Clara, CA). The MS was operated in electron impact (EI) ionization mode for parent PAH and alkylated PAH compounds and in electron capture negative chemical ionization (ECNCI) mode for nitro- and oxy-PAHs. The MS was operated in selected ion monitoring mode (SIM) in both cases. Transitions and retention times for all included native and IS compounds are presented in Table S3 (parent and alkylated PAHs) and Table S4 (nitro- and oxy-PAHs). Every PAC target compound was quantified using the relative response factor (RRF)Citation53 to each native compound included in the standard mixtures. More details of the procedure used for quantification of the samples are described in SI.
Quality assurance
Consistent recoveries (40–110%) were obtained for all IS compounds that were added to and used for correction of the samples. Field and laboratory blanks were analyzed in parallel with the samples. Minor residues only of some 2–4 ringed parent PAHs occurred, although the amount occurring was <10% of the amount found in the samples. All results were corrected for the blanks. The limits of detection (LOD) were calculated as three times the standard deviation of the values for the blanks or the background noise of these blanks. Three standard reference materials (SRM 1649a, 1649b and 2975) were used as quality control (QC). The measured levels lie, for most part, within 30% of the certified levels. The results for SRM 1649b are given in SI for 20 parent- and 6 nitro-PAHs.
Results
Introductory remarks on the data
All compound groups (PM2.5, parent-, alkylated-, nitro-, and oxy-PAHs) were detected in most of the samples. This confirms for the PACs in addition to parent and alkylated PAHs but also nitro- and oxy-PAH derivatives may be present in both outdoor and indoor environments in southern Sweden. Although comparison with literature data should be made with caution due to variations in the season sampling, the number of samples collected, and/or the number of targeted PAC compounds among studies, in general, the PAC levels reported herein are low.
The results (mean, median and range) of indoor and residential outdoor measurements can be found in , but also for indoor and outdoor measurements, the results are also shown in Tables S1 and S2 and . Data are presented as range and median in further discussions since the values were not normally distributed. It is important to point out that the temperatures in the sampling enclosures was relatively high, which can have led to some loss of volatile species during sampling, hence some results can be underestimated – see details in limitations.
Figure 1. Concentrations of PM2.5 and polycyclic aromatic compounds (PACs), parent PAHs, alkylated-PAHs, and nitro- and oxy-PAH derivates inside and just outside 15 residences in southern Sweden. (Note different scales on Y-axis for concentrations indoors and outdoors.)
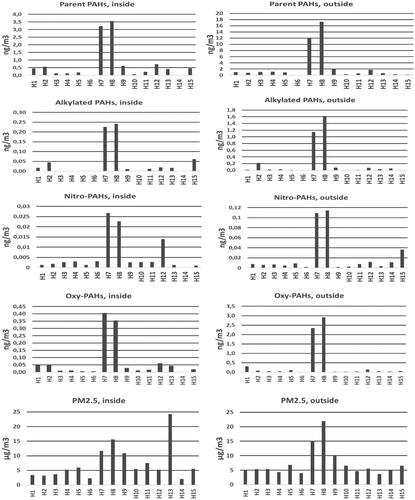
Table 2. Levels (mean, median and range) of PM2.5 (µg m−3) and particulate associated PAC compounds (ng m−3) indoor and outdoor 15 residences in southern Sweden.
PM2.5
The results (mean, median, and range) of indoor and residential outdoor PM2.5 measurements are given in and . The ranges for indoor respective outdoor was 2.1–24 µg m−3 (median 5.5) and 3.7–22 µg m−3 (median 5.3). Thus, there was a factor of 10 between the highest and lowest measured levels indoors and factor of 6 for measurements outdoors. This indicates slightly lower variations in outdoor levels, higher variation indoors with contribution of indoor sources to PM2.5 levels indoors, as reported by Omelekhina et al.Citation48 based on real time PM2.5 measurements indoors and outdoors and information from occupants’ logbooks of activities. Particle mass concentrations in our study were low. They were lower than levels found in central and southern EuropeCitation54 and LithuaniaCitation15 but similar to those found in other Nordic countries viz. Helsinki, FinlandCitation54 Copenhagen, DenmarkCitation55 and Gothenburg, Sweden.Citation4 It is important to note that indoor PM2.5 levels for smokers can be significantly higher compared to nonsmokers.Citation4,Citation56 Even if during recruitment the occupants declared to be nonsmokers in two apartments occasional smoking occurred, namely in apartments 8 and 11. Additionally, as the enclosure was kept on balconies, influence of possible neighbors’ smoking cannot be excluded, measurements took place in real life scenario where we did not have the control what happens in vicinity. Once, during measurements in home 15, in the vicinity an accidental fire of trash happened, however this event did not lead to elevated average PM2.5 concentration.
PACs
Parent PAHs were, as expected, the PAC group that made up the largest part in both indoor and outdoor particles, range 0.015–3.8 ng m−3 (median 0.40) and 0.068–19 ng m−3 (median 0.90). It varied slightly between samples, but parent PAHs accounted typically between 70 and 90% of the total PAC content. There was a large difference, two orders of magnitude, in comparison between the highest measured concentrations, outdoors and indoors, respectively. High molecular weight PAHs with five to six rings, such as benzo(a)anthracene, chrysene, benzo(b)fluoranthene, benzo(k)fluoranthene, benzo(a)pyrene (BaP), indeno(1,2,3-c,d)pyrene, benzo(g,h,i)perylene, and the 3-ring PAH phenanthrene predominated. High concentrations of the 4-ring PAHs, fluoranthene and pyrene, were also observed. These results are expected, since 90% of PAH with 5–6 rings are adsorbed on particles, PAHs with 4-rings are often in similar amounts in both gas (not measured here) and particle phases, while PAH with 2–3 rings is mainly found (>90%) in the gas phase. When the levels of PAH in the gaseous phase are elevated in a place, the levels of PAH with three rings can also be noticeable on particles. Phenanthrene (3-ring PAH) was the dominant PAH in three of the indoor sites.
The concentrations of parent PAHs observed in this study are low compared to other studies in Europe. Comparison of outdoor BaP levels in our study (range 0.0040–0.95 ng m−3) are lower than BaP levels in PM2.5 fraction in Western European cities (0.1–1.1 ng m−3) and in Eastern and Central European cites from 3.0 to 3.2 ng m−3.Citation57 A study from Lithuania reported as high BaP levels as 6.2 ng m−3.Citation15 However, the levels in our study are quite similar compared to another Swedish study, in Gothenburg that reported BaP levels between 0.011 and 0.14 ng m−3.Citation4 It is worth noting that the European limit value for BaP is 1 ng m−3 in PM10. Increasing levels of ambient PM pollution from rapid urbanization and economic development in China and other places from Asia have drawn worldwide attention, especially with its significance for possible health effects.Citation16–21 A study from Beijing, indoors and outdoors, the BaP levels were only slightly higher than those from our study, 1.67 ng m−3 and 1.04 ng m−3, respectively,Citation18 while another study from China (rural area of Guanzhong Basin), reported indoor and outdoor levels as high as 23 and 19 ng m−3.Citation19 Two outdoor studies from the mega city of Bangkok Thailand, the levels were quite the same as in our study (approx. >0.4 ng m−3)Citation16,Citation20 although a study from Chiang-Mai, Thailand where the objective was to study effects of agricultural waste burning on PM2.5-bound PAHs, ambient air levels rendered in as high BaP levels as 9–703 ng m−3.Citation21
The nitro-PAH concentrations ranged indoor and outdoor between LOD and 0.028 ng m−3 (median 0.0027) and 0.0018–0.11 ng m−3 (median 0.0076), respectively, and corresponding results for oxy-PAH were between LOD and 0.41 ng m−3 (median 0.019) and 0.0072–2.9 ng m−3 (median 0.051). The nitro-PAHs concentrations were a magnitude of two lower as those of sum parent PAHs and the corresponding results for oxy-PAHs were one order of magnitude lower. In a study from south of France oxy-PAHs were of the same magnitude as those of parent PAHs while nitro-PAHs concentrations were one to two orders lower.Citation37 Thus, the levels in our study of these PAH derivatives are low compared to total parent PAHs.
Nitro- and oxy-PAHs as well as parent PAHs partition between gaseous and particulate fractions. Most of these derivatives are particle bound; only 1-nitronaphthalene, 2-nitronapthalene and, napthalene-1-aldehyde, 2-naphthaldehyde and p-fluorenone are reportedly almost exclusively in the gaseous phase.Citation37 Out of 17 target nitro-PAHs only seven nitro-PAHs were detected in at least one sample. The highest detectability was 3-nitrofluoranthene, 9-nitrophenanthrene, and 4-nitropyrene, which was detected in almost all samples both indoors and outdoors. 2-Nitrofluorene, 9-nitroanthracene, and 2-nitropyrene were detected in between 40 and 80% of the samples, while 1-nitropyrene was detected in only one indoor and five outdoor samples. All oxy-PAHs were detected in most samples with a detectability of between 80 and 100% for both the indoor and outdoor measurements. Only 1,4-anthraquinone had low detectability and was found in low concentrations in only six samples. The levels of PAH derivatives are very low compared to other studies worldwide. For nitro-PAH, only the two highest levels in our study are in the same range as other studies, e.g. in France,Citation37,Citation42 UKCitation58 and Spain.Citation59 Significantly, higher levels have been reported in Athens, Greece (2.3–5.9 ng m−3)Citation60 and very high levels have been reported indoors and outdoors in four cities in Nepal (4.7–18 ng m−3).Citation30 In addition, oxy-PAHs were low in our study compared to other studies. The two highest levels found outdoor in our study are comparable with previous studies, e.g. Nepal (0.45–6.5 ng m−3)Citation30 France (6.3 ng m−3) and Greece (3.5–6.9 ng m−3).Citation61
The alkylated PAHs were present in most samples both indoors and outdoors. They accounted for approximately between a few to about 10% of total PACs. It is important to point out here that out of the 14 alkylated PAHs that were included in this study, only four were expected to be found as particle bound.Citation62 Alkylated PAHs with two to three rings, which are mainly found in the gaseous phase, were also found in some samples. Alkylated PAHs ranged indoor and outdoor from LOD-0.24 ng m−3 (median 0.012) and LOD-1.6 ng m−3 (median 0.030).
Comparison of parent PAH levels before and after renovation
The results of the seven houses before and after energy renovation, indoors and outdoors, are depicted in . PAHs levels were found to be two times higher outside (average 2.5 ng m−3) than inside (average 0.7 ng m−3) before renovation. After renovation, PAH levels were similar between outside (2.2 ng m−3) and inside (1.8 ng m−3). The PAH levels were similar outside from the sampling before and after renovation (2.5 and 2.2. ng m−3, respectively). This was also confirmed by similar concentrations of BaP before and after renovation. Outside levels of BaP ranged from < LOD to 0.65 ng m−3 (average 0.15 ng m−3) before renovation, and from <0.02 to 0.48 ng m−3 (average 0.14 ng m−3) after renovation.
Figure 2. Concentrations (average) of parent PAHs, outdoors and indoors, seven residences, before and after energy renovation, in southern Sweden.
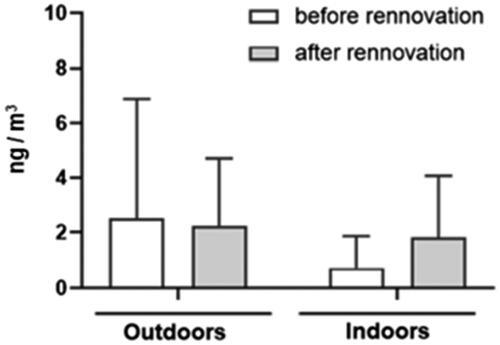
In indoor environments, PAH levels increased slightly after renovation, with an average of 0.7 ng m−3 before renovation and 1.8 ng m−3 after renovation. This was also confirmed by the increase of BaP. Before renovation, BaP levels ranged from < LOD to 0.13 ng m−3 (average 0.03 ng m−3), and after renovation, BaP levels ranged from < LOD to 0.36 ng m−3 (average 0.11 ng m−3).
Discussion
PM2.5 and PAC levels
Ambient PM and PAHs are derived from a variety of sources and have both natural and anthropogenic origin. Anthropogenic sources are attributable to human activities, such as combustion processes, e.g. burning of biomass and fossil fuels, traffic exhaust (petrol and diesel vehicles), and industry.Citation20,Citation27 Major indoor sources include smoking, cookingCitation29 wood stovesCitation28 and candle burning.Citation32 Nitro- and oxy-PAHs can be released together with parent PAHs in incomplete combustion processes but can also be formed through secondary reactions with OH and NO3Citation38,Citation39. Moreover, diesel vehicles have been reported as a source of nitro-PAHsCitation63 and biomass burning has been reported an important source of oxy-PAHs.Citation64 Alkylated PAHs can also be formed as well as parent PAHs by incomplete combustion. Additionally, various motor fuels may contain elevated levels of alkylated PAHs, and elevated levels of these PAHs may occur through direct emissions from the fuel.Citation31 It cannot be ruled out that petrol stations may be a source of these PAHs.
The two outdoor measurement sites (H7 and H8), which are clearly illustrated in , had the highest content of both PM2.5 and all PAC chemical groups. Indeed, for all measurement sites, as can be seen in , both PM2.5, and the PACs alkylated PAHs, nitro- and oxy-PAHs correlated well with parent PAHs (R2 = 0.89 − 0.98; p < 0.001) (alkylated-PAHs not shown). In case of home H8 occasional smoking indoors could have influenced the results, however in case of home H11, where occasional smoking was also reported influence of smoking is not visible. Additionally, the outdoor sampling during measurements in homes H6–H15 were performed on a balcony of home H9, thus occasional smoking inside of H8 could not influence simultaneous outdoor measurements (at different location) at this time. In other words, the influence visible for homes 7 and 8 is due to elevated PM2.5 and PAC outdoor concentrations.
Figure 3. Parent PAHs versus nitro-PAHs, oxy-PAHs, and PM2.5 indoor and just outside 15 residences in southern Sweden.
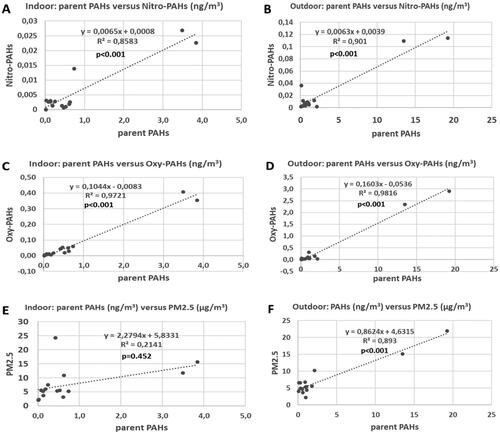
Number of studies show that atmospheric photochemical reactions may significantly influence nitro- and oxy-PAH level in the environment (e.g.Citation30,Citation38) In addition, tropical countries with temperature and stronger solar radiation could possibly favor the atmospheric transformation of PAHs to nitro- and oxy-PAH derivatives.Citation65 This may mean that the low levels of nitro- and oxy-PAHs compared to parent PAHs, in our study, that the dark and cold climate in northern countries does not favor the secondary formation of these PAHs in the atmosphere. Thus, the large variety of PM2.5 and PAC levels between the outdoor locations () but still a clear correlation between all groups (PM2.5 and PACs) can indicate similar combustion sources and the variation in levels can be due to intra-urban variation between the sites that can be related to the different proximities to roads and dissimilarities in traffic density as well as varying in time contribution from long-range transport of pollutants.
Environmental exposures to substances in indoor air which may have toxic potential is important to evaluate, especially that we spend majority of our time in indoor environments. Also, to assess the importance how the outdoor environment affects the indoor air quality. We assumed that if the outdoor air has significantly affected the indoor air, this can be shown in pattern comparisons between indoor and outdoor, and that the different PM2.5 and particulate PACs, respectively, correlated between indoor and outdoor levels. Moreover, ratios between indoor and outdoor air concentrations (I/O ratios) may be used as a tool to indicate whether there are indoor sources (I/O > 1) or outdoor sources (I/O < 1).
There are pattern similarities between the indoor and outdoor samples (). In the samples where the levels are elevated outdoors, they are also indoors. This pattern appears to be clearer for PACs than for PM2.5. This is better clarified by correlation comparison for the respective PAC group and PM2.5 (). Indeed, the PAC groups are correlated (R2 = 0.80–0.95, p < 0.001) (alkylated PAHs are not shown) indicating the significance of the outdoor air for the indoor air for all PAC groups. Contrary, PM2.5 indoors versus outdoors correlated poorly (R2 = 0.16, p = 0.569), which confirms results reported by Omelekhina et al.Citation48,Citation66 (based on real time monitoring indoors and outdoors and logbooks information) that indoor PM2.5 do not originate from outdoors only and indoor sources contribute to observed PM2.5 levels as well, hence the lack of the correlation. That the levels of particulate PAC indoors would have the same source as outdoors is also indicated by the correlation between parent PAH and other PAC groups indoors (; R2 = 0.86–0.97, p < 0.001 (alkylated PAHs not shown)). There is a very weak indoor correlation between parent PAHs and PM2.5 (R2 = 0.21, p = 0.452). Other studies have also shown that indoor and outdoor PM2.5 often do not correlate well and that PAH levels indoors do not correlate with PM2.5 indoors.Citation4,Citation67 This again emphasizes that PACs have the same origin indoors and outdoors but PM2.5 also has significant sources indoors.
Figure 4. Outdoor versus indoor concentrations of parent PAHs, nitro-PAHs, oxy-PAHs, and PM2.5, respectively, in 15 residences in southern Sweden.
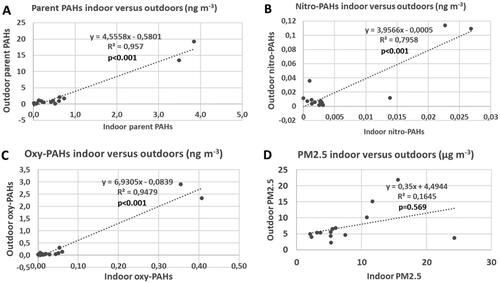
The I/O ratios for PM2.5 and all PAC groupings are shown in Figure S1. The I/O ratios were considerably lower for all parent PAHs in all homes except one (H15). However, the ratio for this home was around one for PM2.5. Thus, there has been indoor source(s) of particulate parent PAH in this home during the measurement period. The ratios of PAH derivatives were lower than one in most homes. However, in four homes, nitro-PAH was higher indoors than outdoors. This indicates that there may also be sources of nitro-PAH indoors. It is important to point out that the levels indoors even in these homes were very low. The PM2.5 I/O ratios were similar to one in most places but higher in some cases. There are many possible indoor sources of PM2.5, e.g. smoking, cooking, wood stoves, candle burning, cleaning, and presence of pets.Citation68 In this study, indoor sources that contributed to indoor PM2.5 namely, candle burning and cooking, were determined on basis of real time PM2.5 monitoring indoors and outdoors and logbook information of occupants’ activities.Citation48,Citation66 Two examples of such indoor sources contributing to PAHs indoors are cookingCitation69 and recently confirmed flickering (stressed) burning of candles.Citation32
Comparison of parent PAH levels before and after renovation
Results demonstrate that PAH levels were slightly increased inside after renovation. This agrees with I/O ratio of PM2.5 reported for seven of the studied apartments before and after renovationCitation48 namely I/O ratios of PM2.5 increased slightly after renovation (median 0.9) in comparison to before renovation (0.77). It can be linked to two processes: 1) increased contribution from outdoors due to slightly increased AER (median AER before the renovation 0.50 h−1 and after renovation 0.55 h−1); 2) contribution from indoor sources to observed PM2.5 levels indoors (indoor sources such as cooking and candle burning, identified in these apartments can contribute to indoor PAH air levels, as described earlier). Due to our small sample number, our results are not conclusive. We cannot show that the efficiency of the energy retrofits could reduce the health risks for the residents with regards to exposure to PM2.5 and PAHs.
BaPeq concentrations
In order to estimate the carcinogenic risks of PAHs mixtures, the equivalents of benzo(a)pyrene (BaPeq), with toxic equivalent factors (TEFs) for BaP set to 1, were evaluated by multiplying concentrations of each PAH with their TEF value.Citation27,Citation70 These authors report TEF for 16 US EPA PAH compounds. TEF factors have been reported for five nitro-PAHs.Citation71 Thus, these parent PAHs and nitro-PAHs are ranked according to cancer potency relative to BaP. Alkylated- and oxy-PAHs, to the best of our knowledge, do not have TEFs but will likely be developed in the future. It is important to point out that alkylated and oxy-PAHs may be more toxic than corresponding parent PAHs and this can underestimate the overall cancer risk.
The European Community has suggested the BaPeq on PM10 at an annual standard in ambient air of 1 ng m−3.Citation72 All measurement sites were below or well below this cancer potency value indoor 0.00038–0.31 ng m−3 (median 0.011) and outdoor 0.006–0.47 ng m−3 (median 0.073). The annual standard BaPeq value (1 ng m−3) have been exceeded in urban sites all over the world, e.g. Athens, Greece (1.6 ng m−3)Citation73 and Zabrze, Poland (7.9 ng m−3)Citation74 and Mexico City, Mexico (2.2 ng m−3).Citation75 None of the nitro-PAHs with TEF factors were found in this study. Thus, these PAH derivatives did not contribute to the cancer potency value indoor and outdoor in this study. Contribution of the cancerogenic potency of BaP alone of the PAH mixtures is commonly >50% (e.g.Citation29,Citation75). BaP, in our study, dominated the carcinogenic potency (>40%) in nine of the indoor and outdoor sites. In addition, the intermediate PAH fluoranthene, with a significant portion also in the gaseous phase accounted for over 50% of the cancer potency in some places. The total PAH content in air is often dominated by PAH with 2–4 rings (>90% in gaseous phase). Although these PAHs have a small TEF factorCitation69 the contribution of these PAHs can be significant if their levels are high.Citation31 This underlines the importance of including both particulate and gas phase PAHs when evaluating risk to human health.
WHOCitation1 has set as an air quality guideline that estimates a unit risk estimate of a BaP level of 0.1 ng m−3, which will cause an extra lifetime cancer risk of 1/100,000. BaP levels found in our study are somewhat higher than this air quality guideline in two indoor and six outdoor measurement sites.
Limitation and uncertainties
There are several limitations in this study. First, the sample size is relatively small. Second, due to the sampling protocol used in this study, the air concentrations were determined without considering gaseous PAH fraction, implying the corresponding conclusion might be unilateral to some extent, especially for low molecular weight PAHs. This can underestimate the concentrations in indoor environment even more as concentrations of gas phase PAH can sometimes be higher indoors than outdoors. Also, the sampling volumes were found to be too small to obtain sufficient mass to quantify, in particular, the nitro-PAHs which were in a very low concentration.
Even though a moderate flow rate was used for the air samplings, the sampling time and volume were still quite high, and the levels of the more volatile PAHs (2–4 rings) may have been somewhat underestimated in the particulate due to losses of these compounds from the filter during sampling. Additionally, the reported results can also be underestimated for both indoor and outdoor measurements, due to temperature difference of ambient air at sampling locations in comparison to temperature in the enclosures where the particles were collected. The average temperature inside the enclosures was relatively high which could have led to some loss of the volatile species. In the enclosures the average temperature was 38.5 °C indoors and 31.3 °C outdoors, while the average ambient temperatures were, respectively, 25.4 and 5.3 °C. The temperature difference between indoor and outdoor week-long collection of the particles inside the enclosures was 7.2 °C. This could have influenced both PM2.5 and PAC concentrations measurements, i.e. some volatile material could have evaporated. Sampled air volume indoors per filter (22 m3) was approximately half in comparison to outdoor sampled volume (40 m3). This volume difference can to some extents have affected the comparison between inside and outside with respect to the limitations mentioned above.
The measurements were conducted in real life scenarios, i.e. in occupied homes thus capturing the living conditions in the studied homes. Occupants were asked to keep logbooks which together with real time PM2.5 measurements allowed to track indoor source contribution to PM2.5 (reported in Omelekhina et al.Citation48) however due to time-integrated samples of PACs, it was not possible to identify specific indoor sources contributing to PAC levels. Location of the measuring equipment in proximity to strong indoor sources, e.g. cooking in the kitchen can result in higher contribution of cooking to PM2.5 and PAC levels in comparison to other sampling locations (living room or hall), measurements in the kitchen were performed in two homes on request of occupants due to practical reasons and might have influenced the concentrations measured. As the outdoor concentrations were monitored in the gardens or balconies, the potential influence of neighbors smoking, barbequing or accidental trash fires could not be controlled or prevented. In some locations, the outdoor measurement was on a balcony on a different floor than the indoor locations. This may have affected the comparisons and contributed to the uncertainty between the indoor and outdoor comparisons for these locations.
The sampling campaign was completed in >3 months. This means an uncertainty in comparing levels between the outdoor locations. This was not the aim of the study either.
Concluding remarks
In summary, the study shows that different PAC chemical classes, viz. parent PAHs, alkylated PAHs, nitro- and oxy-PAHs can be found on particles (PM2.5) both indoors and just outside these homes. The levels were low compared to other studies worldwide. There was, however, great variation in levels between the different sampling sites. Within the framework of this study, it was not possible to investigate specific sources outdoors of PM2.5 and PACs. The low concentrations of nitro- and oxy-compared to parent PAHs, compared to other published studies, could possibly be due to low atmospheric photo formation of nitro- and oxy-PAHs because the cold climate. It is likely that intra-urban variation between the sites, different proximities to roads, dissimilarities in traffic density and varying influence of long-range transport of pollution can partly explain the notable difference in levels found outdoors. However, the results indicate that the content inside the homes is strongly affected by the PAC levels just outside. A comparison of the PAH levels indoors and outdoors after energy renovation does not indicate an improvement of the indoor air regarding the levels of particulate PAC. Although the PAC levels were low, the air levels for BaP were higher inside and outside the residences at some of the measurement sites compared to the air quality guideline set by the WHO.
Supplemental Material
Download MS Word (564 KB)Acknowledgments
The authors thank all participants who let us perform the measurements in their homes as well as LKF, Kraftringen, and Lunds kommun for cooperation. Special thanks to Karen Christensen for technical help with PAC analysis.
Disclosure statement
No potential conflict of interest was reported by the author(s).
Additional information
Funding
References
- WHO. 2021. WHO global air quality guidelines. Particulate matter (PM2.5 and PM10), ozone, nitrogen dioxide, sulfur dioxide and carbon monoxide. ISBN 978-92-4-003422-8 https://apps.who.int/iris/bitstream/handle/10665/345329/9789240034228-eng.pdf (accessed October 25, 2021).
- M. Gustafsson, J. Lindén, L. Tang, B. Forsberg, H. Orru, S. Åström, and K. Sjöberg, “Quantification of Population Exposure to NO2, PM2.5 and PM10 and Estimated Health Impacts,” (Report number C 317 ISBN 978-91-88787-60-6, 2015, Sweden).
- D. W. Dockery, C. A. Pope, X. P. Xu, J. D. Spengler, J. H. Ware, M. E. Fay, B. G. Ferris, and F. E. Speizer, “An Association between Air-Pollution and Mortality in 6 United-States Cities,” The New England Journal of Medicine 329, no. 24 (1993): 1753–9.
- S. Johannesson, P. Gustafson, P. Molnar, L. Barregard, and G. Sällsten, “Exposure to Fine Particles (PM2.5 and PM1) and Black Smoke in the General Population: Personal, Indoor, and Outdoor Levels,” Journal of Exposure Science & Environmental Epidemiology 17, no. 7 (2007): 613–24.
- C. A. Pope, R. T M. Ezzati, and D. W. Dockery, “Fine-Particulate Air Pollution and Life Expectancy in the United States,” The New England Journal of Medicine 360, no. 4 (2009): 376–86.
- D. G. Karottki, M. Spilak, M. Frederiksen, L. Gunnarsen, E. V. Brauner, B. Kolarik, Z. Jovanovic Andersen, T. Sigsgaard, L. Barregard, B. Strandberg, et al, “An Indoor Air Filtration Study in Homes of Elderly: Cardiovascular and Respiratory Effects of Exposure to Particulate Matter,” Environmental Health: A Global Access Science Source 12 (2013): 116.
- N. Li, M. Q. Hao, R. F. Phalen, W. C. Hinds, and A. E. Nel, “Particulate Air Pollutants and Asthma-a Paradigm for the Role of Oxidative Stress in PM-Induced Adverse Health Effects,” Clinical Immunology (Orlando, FL) 109, no. 3 (2003): 250–65.
- A. Nemmar, and I. M. Inuwa, “Diesel Exhaust Particles in Blood Trigger Systemic and Pulmonary Morphological Alterations,” Toxicology Letters 176, no. 1 (2008): 20–30.
- B. Walker, and C. P. Mouton, “Environmental Influences on Cardiovascular Health,” Journal of the National Medical Association 100, no. 1 (2008): 98–103.
- E. Erlandsson, R. Lindgren, Å. Nääv, A. M. Krais, B. Strandberg, T. Lundh, C. Boman, C. Isaxon, S. R. Hansson, and E. Malmqvist, “Exposure to Wood Smoke Particles Leads to Inflammation, Disrupted Proliferation and Damage to Cellular Structures in Human First Trimester Trophoblast Cell Line,” Environmental Pollution (Barking, Essex : 1987) 264 (2020): 114790.
- Å. Nääv, L. Erlandsson, C. Isaxon, E. Åsander Frostner, J. Ehinger, M. K. Sporre, A. M. Krais, B. Strandberg, T. Lundh, E. Elmer, et al, “Urban PM2.5 Induces Cellular Toxicity, Hormone Dysregulation, Oxidative Damage, Inflammation, and Mitochondrial Interference in the HRT8 Trophoblast Cell Line,” Frontiers in Endocrinology 11 (2020): 75.
- “IARC Diesel and Gasoline Engine Exhausts and Some Nitroarenes,” IARC Monographs on the Evaluation of the Carcinogenic Risks to Humans 105 (2012): 1–703.
- J. Dachs, and S. J. Eisenreich, “Asorption onto Aerosol Soot Carbon Dominates Gas-Particle Partitioning of Polycyclic Aromatic Hydrocarbons,” Environmental Science & Technology 34, no. 17 (2000): 3690–7.
- H. Wingfors, A. Sjodin, P. Haglund, and E. Brorstrom-Lundén, “Characteristation and Detemination of Profiles of Polycyclic Aromatic Hydrocarbons in a Traffic Tunnel in Gothenburg,” Atmospheric Environment 35, no. 36 (2001): 6361–9.
- L. Kliucininkas, D. Martuzevicius, E. Krugly, T. Prasauskas, V. Kauneliene, P. Molnar, and B. Strandberg, “Indoor and Outdoor Concentrations of Fine Particles, Particle-Bound PAHs and Volatile Organic Compounds in Kaunas,” Journal of Environmental Monitoring 13, no. 1 (2011): 182–91.
- S. Pongpiachan, M. Hattayanone, O. Suttinun, C. Khumsup, I. Kittikoon, P. Hirunyatrakul, and J. Cao, “Assessing Human Exposure to PM10-Bound Polycyclic Aromatic Hydrocarbons during Fireworks Displays,” Atmospheric Pollution Research 8, no. 5 (2017): 816–27.
- J. Zhang, L. Yang, A. Mellouki, J. Chen, X. Chen, Y. Gao, P. Jiang, Y. Li, H. Yu, and W. Wang, “Diurnal Concentrations, Sources, and Cancer Risk Assessments of PM2.5-Bound PAHs, NPAHs, and OPAHs in Urban, Marine and Mountain Environments,” Chemosphere 209 (2018): 147–55.
- J. Chang, J. Tao, C. Xu, Y. Li, N. Li, Z. Tang, Y. Yang, Z. Liu, Q. Wang, and D. Xu, “Pollution Characteristics of Ambient PM2.5-Bound Benzo(a)Pyrene and Its Cancer Risks in Beijing,” The Science of the Total Environment 654 (2019): 735–41.
- Y. Li, H. Xu, J. Wang, S. Sai Hang Ho, K. He, Z. Shen, Z. Ning, J. Sun, L. Li, R. Lei, et al, “Personal Exposure to PM2.5-Bound Organic Species from Domestic Solid Fuel Combustion in Rural Guanzong Basin, Cina: Characteristics and Health Implication,” Chemosphere 227 (2019): 53–62.
- C. ChooChuay, S. Pongpiachan, D. Tipmanee, O. Suttinun, W. Deelaman, Q. Wang, L. Xing, G. Li, Y. Han, J. Palakun, et al, “Impacts of PM2.5 Sources on Variations in Particulate Chemical Compounds in Ambient Air of Bangkok, Thailand,” Atmospheric Pollution Research 11, no. 9 (2020): 1657–67.
- C. ChooChuay, S. Pongpiachan, D. Tipmanee, W. Deelaman, N. Iadtem, O. Suttinun, Q. Wang, L. Xing, G. Li, Y. Han, et al, “Effects of Agricultural Waste Burning on PM2.5-Bound Polycyclic Aromatichydrocarbons, Carbonaceous Compositions, and Water-Soluble Ionic Species in the Ambient Air of Chiang-Mai,” Polycyclic Aromatic Compounds 42, no. 3 (2022): 749–70.
- M. J. Ehigbor, C. M. A. Iwegbue, O. I. Eguavoen, G. O. Tesi, and B. S. Martincigh, “Occurrence, Sources and Ecological and Human Health Risks of Polycyclic Aromatic Hydrocarbons in Soils from Some Functional Areas of the Nigerian Megacity,” Environmental Geochemistry and Health 42, no. 9 (2020): 2895–923.
- T. Hu, Y. Mao, W. J. Liu, C. Cheng, M. M. Shi, Z. L. Chen, Q. Tian, J. Q. Zhang, S. H. Qi, and X. L. Xing, “Fate of PM2.5-Bound PAHs in Xiangyang, Central Chinaduring 2018 Chinese Spring Festival. Influence of Fireworks Burning and Air-Mass Transport,” Journal of Environmental Sciences (China) 97 (2020): 1–10.
- B. Keshavarzi, H. S. Abbasi, F. Moore, H. Delshab, and N. Soltani, “Polycyclic Aromatic Hydrocarbons in Street Dust of Bushehr City, Iran: Status, Sources, and Human Health Risk Assessment,” Polycyclic Aromatic Compounds 40, no. 1 (2020): 61–75.
- R. Ouyang, S. Yang, and L. Xu, “Analysis and Risk Assessment of PM2.5-Bound PAHs in a Comparison of Indoor and Outdoor Environments in a Middle School: A Case Study in Beijing, China,” Atmosphere 11, no. 9 (2020): 904.
- S. R. Wild, and K. C. Jones, “Polynuclear Aromatic-Hydrocarbons in the United-Kingdom Environment-a Preliminary Source Inventory and Budget,” Environmental Pollution 88, no. 1 (1995): 91–108.
- C. E. Boström, P. Gerde, A. Hanberg, B. Jernström, C. Johansson, T. Kyrklund, A. Rannug, M. Törnqvist, K. Victorin, and R. Westerholm, “Cancer Risk Assessment Indicators, and Guidelines for Polycyclic Aromatic Hydrocarbons in the Ambient Air,” Environmental Health Perspectives 110, no. s3 (2002): 451–89.
- P. Gustafson, C. Östman, and G. Sällsten, “Indoor Levels of Polycyclic Aromatic Hydrocarbons in Homes with or without Wood Burning for Heating,” Environmental Science & Technology 42, no. 14 (2008): 5074–80.
- R. Bramming Jorgensen, B. Strandberg, A.- K. Sjaastad, A. Johansen, and K. Svendsen, “Simulated Restaurant Cook Exposure to Emissions of PAHs, Mutagenic Aldehydes, and Particles from Frying Bacon,” Journal of Occupational and Environmental Hygiene 10, no. 3 (2013): 122–31.
- I. C. Yadav, N. L. Devi, V. K. Singh, J. Li, and G. Zhang, “Concentrations, Sources and Health Risks of Nitrated- and Oxygenated- Polycyclic Aromatic Hydrocarbon in Urban Idoor Air and Dust from Four Cities of Nepal,” The Science of the Total Environment 643 (2018): 1013–23.
- B. Strandberg, C. Österman, H. Koca Akdeva, J. Moldanová, and S. Langer, ” “The Use of Polyurethane Foam (PUF) Passive Air Samplers in Exposure Studies to PAHs in Swedish Seafarers,” Polycyclic Aromatic Compounds 42, no. 2 (2022): 448–59.
- C. Andersen, Y. Omelekhina, B. Brøndum Rasmussen, M. Bennekov, S. Nielsen Skov, M. Køcks, K. Wang, B. Strandberg, F. Mattsson, M. Bilde, et al, “Emissions of Soot, PAHs, Ultrafine Particles, NOx, and Other Health Relevant Compounds from Stressed Burning of Candles in Indoor Air,” Indoor Air 31, no. 6 (2021): 2033–48.
- J. Liu, J. Li, T. Lin, D. Liu, Y. Xu, C. Chaemfa, S. Qi, F. Liu, and G. Zhang, “Diurnal and Nocturnal Variations of PAHs in the Lhasa Atmosphere, Tibetan Plateau: Implication for Local Sources and the Impact of Atmospheric Degradation Processing,” Atmospheric Research 124 (2013): 34–43.
- Q. Mu, M. Shiraiwa, M. Octaviani, N. Ma, A. Ding, H. Su, G. Lammel, U. Poschl, and Y. Cheng, “Temperature Effect on Phase State and Reactivity Controls Atmospheric Multiphase Chemistry and Transport of PAHs,” Science Advances 4, no. 3 (2018): 7314.
- J. L. Durant, A. L. Lafleur, E. F. Plummer, K. Taghizadeh, W. F. Busby, and W. G. Thilly, “Human Lymphoblast Mutagens in Urban Airborne Particles,” Environmental Science & Technology 32, no. 13 (1998): 1894–906.
- M. Y. Chung, R. A. Lazaro, D. Lim, J. Jackson, J. Lyon, D. Rendulic, and A. S. Hasson, “Aerosol-Borne Quinones and Reactive Oxygen Species Generation by Particulate Matter Extracts,” Environmental Science & Technology 40, no. 16 (2006): 4880–6.
- A. Albinet, E. Leoz-Garziandia, H. Budzinski, and E. Viilenave, “Polycyclic Aromatic Hydrocarbons (PAHs), Nitrated PAHs and Oxygenated PAHs in Ambient Air of the Marseilles Area (South of France): Concentrations and Sources,” The Science of the Total Environment 384, no. 1–3 (2007): 280–92.
- J. Ringuet, A. Albinet, E. Leoz-Garziandia, H. Budzinski, and E. Villenave, ” “Reactivity of Polycyclic Aromatic Compounds (PAHs, NPAHs and OPAHs) Adsorbed on Natural Aerosol Particles Exposed to Atmospheric Oxidants,” Atmospheric Environment 61 (2012): 15–22.
- I. J. Keyte, A. Albinet, and R. M. Harrison, “On-Road Traffic Emissions of Polycyclic Aromatic Hydrocarbons and Their Oxy- and Nitro- Derivative Compounds Measured in Road Tunnel Environments,” The Science of the Total Environment 566–567 (2016): 1131–42.
- A. Albinet, E. Leoz-Garziandia, H. Budzinski, E. Villenave, and J.-L. Jaffrezo, “Nitrated and Oxygenated Derivatives of Polycyclic Aromatic Hydrocarbons in the Ambient Air of Two French Alpine Valleys Part 1: Concentrations, Sources and Gas/Particle Partitioning,” Atmospheric Environment 42, no. 1 (2008): 43–54.
- A. Albinet, E. Leoz-Garziandia, H. Budzinski, E. Villenave, and J.-L. Jaffrezo, “Nitrated and Oxygenated Derivatives of Polycyclic Aromatic Hydrocarbons in the Ambient Air of Two French Alpine Valleys Part 2: Particle Size Distribution,” Atmospheric Environment 42, no. 1 (2008): 55–64.
- J. Ringuet, A. Albinet, E. Leoz-Garziandia, H. Budzinski, and E. Villenave, “Diurnal/Nocturnal Concentrations and Sources of Particulate-Bound PAHs, OPAHs and NPAHs at Traffic and Suburban Sites in the Region of Paris (France),” The Science of the Total Environment 437 (2012): 297–305.
- F. Nalin, B. Golly, J.-L. Besombes, C. Pelletier, R. Aujay-Plouzeau, S. Verlhac, A. Dermigny, A. Fievet, N. Karoski, P. Dubois, et al, “Fast Oxidation Processes Fromemission to Ambient Air Introduction of Aerosol Emitted by Residential Log Wood Stoves,” Atmospheric Environment 143 (2016): 15–26.
- S. Tomaz, P. Shahpoury, J. L. Jaffrezo, G. Lammel, e Perraudin, E. Villenave, and A. Albinet, “One-Year Study of Polycyclic Aromatic Compounds at an Urban Site in Grenoble (France): Seasonal Variations, Gas/Particle Partitioning and Cancer Risk Estimation,” The Science of the Total Environment 565 (2016): 1071–83.
- J. A. Leech, W. C. Nelson, R. T. Burnett, S. Aaron, and M. E. Raizenne, “It’s about Time: A Comparison of Canadian and American Time–Activity Patterns,” Journal of Exposure Analysis and Environmental Epidemiology 12, no. 6 (2002): 427–32.
- P. Bohlin, K. C. Jones, H. Tovalin, and B. Strandberg, “Observations on Persistent Organic Pollutants in Indoor and Outdoor Air Using Passive Polyurethane Foam Samplers,” Atmospheric Environment 42, no. 31 (2008): 7234–41.
- A. Wierzbicka, Y. Omelekhina, A. T. Saber, E. Bloom, B. Strandberg, J. Pagels, and N. R. Jacobsen, “Are Airborne Particles inside Occupied Residences More Toxic than Outdoors? Results from 16 Residences in Sweden, 2019” (Proceedings of European Aerosol Conference, Gothenburg, Sweden, 2019).
- Y. Omelekhina, A. Eriksson, F. Canonaco, A. S. Prevot, P. Nilsson, C. Isaxon, J. Pagels, and A. Wierzbicka, “Cooking and Electronic Cigarettes Leading to Large Differences between Indoor and Outdoor Particle Composition and Concentration Measured by Aerosol Mass Spectrometry,” Environmental Science: Processes & Impacts 22, no. 6 (2020): 1382–96.
- Y. Omelekhina, B. Nordquist, G. Alce, H. Caltenco, P. Wallenten, J. Borell, and A. Wierzbicka, “Effect of Energy Renovation and Occupants’ Activities on Airborne Particle Concentrations in Swedish Rental Apartments,” Science of the Total Environment 806 (2022): 149995.
- E. Pedersen, J. Borell, Y. Li, and K. Stålne, "Good indoor environmental quality (IEQ) and high energy efficiency in multifamily dwellings: How do tenants view the conditions needed to achieve both?" Building and Environment 191 (2021): 107581.
- E. Pedersen, C. Gao, and A. Wierzbicka, “Tenant Perceptions of Post-Renovation Indoor Environmental Quality in Rental Housing: Improved for Some, but Not for Those Reporting Health-Related Symptoms,” Building and Environment 189 (2021): 107520.
- L. Gren, V. B. Malmborg, N. R. Jacobsen, P. C. Shukla, K. M. Bendtsen, A. C. Eriksson, Y. J. Essig, A. M. Krais, K. Loeschner, S. Shamun, et al, “Effect of Renewable Fuels and Intake O2 Concentration on Diesel Engine Emission Characteristics and Reactive Oxygen Species (ROS) Formation,” Atmosphere 11, no. 6 (2020): 641.
- S. Pongpiachan, S. Bualert, P. Sompongchaiyakul, and C. Kositanont, “Factors Affecting Sensitivity and Stability of Polycyclic Hydrocarbons Determined by Gas Chromatography Quadrupole Ion Trap Mass Spectrometer,” Analytical Letters 42, no. 13 (2009): 2106–30.
- K. J. Koistinen, O. Hänninen, T. Rotko, R. D. Edwards, D. Moschandreas, and M. J. Jantunen, “Behavioral and Environmental Determinants of Personal Exposure to PM2.5 in EXPOLIS-Helsinki,” Atmospheric Environment 35, no. 14 (2001): 2473–81.
- M. Sorensen, S. Loft, H. V. Andersen, O. Raaschou-Nielsen, L. T. Skovgaard, L. E. Knudsen, I. V. Nielsen, and O. Hertel, “Personal Exposure to PM2.5, Black Smoke and NO2 in Copenhagen: Relationship to Bedroom and Outdoor Concentrations Covering Seasonal Variation,” Journal of Exposure Analysis and Environmental Epidemiology 15 (2005): 413–22.
- L. Oglesby, N. Künzli, M. Röösli, C. Braun-Fahrländer, P. Mathys, W. Stern, M. Jantunen, and A. Kousa, “Validity of Ambient Levels of Fine Particles as Surrogate for Personal Exposure to Outdoor Air Pollution-Results of the European EXPOLIS-EAS Study,” Journal of the Air & Waste Management Association (1995) 50, no. 7 (2000): 1251–61.
- K. Saarnio, M. Sillanpaa, R. Hillamo, E. Sandell, A. S. Pennanen, and R. O. Salonen, “Polycyclic Aromatic Hydrocarbons in Size-Segregated Particulate Matter from Six Urban Sites in Europe,” Atmospheric Environment 42, no. 40 (2008): 9087–97.
- M. S. Alam, I. J. Keyte, J. Yin, C. Stark, A. M. Jones, and R. M. Harrison, “Diurnal Variability of Polycyclic Aromatic Compounds (PAC) Concentrations: Relationship with Meteorological Conditions and Inferred Sources,” Atmospheric Environment 122 (2015): 427–38.
- A. I. Barrado, S. Garcia, M. L. Sevillano, J. A. Rodriguez, and E. Barrado, “Vapor-Phase Concentrations of PAHs and Their Derivatives Determined in a Large City: Correlations with Their Atmospheric Aerosol Concentrations,” Chemosphere 93, no. 9 (2013): 1678–84.
- M. P. Tsakas, I. E. Sitaras, and P. A. Siskos, “Nitro Polycyclic Aromatic Hydrocarbons in Atmospheric Particulate Matter of Athens, Greece,” Chemistry and Ecology 26, no. 4 (2010): 251–61.
- G. Andreou, and S. Rapsomanikis, “Polycyclic Aromatic Hydrocarbons and Their Oxygenated Derivatives in the Urban Atmosphere of Athens,” Journal of Hazardous Materials 172, no. 1 (2009): 363–73.
- T. Harner, K. Su, S. Genualdi, J. Karpowicz, L. Ahrens, C. Mihele, J. Schuster, J.- P. Charland, and J. Narayan, “Calibration and Application of PUF Disk Passive Samplers for Tracking Polycyclic Aromatic Compounds (PACs),” Atmospheric Environment 75 (2013): 123–8.
- S. Hu, J. D. Herner, W. Robertson, R. Kobayashi, M. C. O. Chang, S. M. Huang, B. Zielinska, N. Kado, J. F. Collins, P. Rieger, et al, “Emissions of Polycyclic Aromatic Hydrocarbons (PAHs) and nitro-PAHs from Heavy-Duty Diesel Vehicles with DPF and SCR,” Journal of the Air & Waste Management Association (1995) 63, no. 8 (2013): 984–96.
- G. Shen, S. Tao, S. Wei, Y. Chen, Y. Zhang, H. Shen, Y. Huang, D. Zhu, C. Yuan, H. Wang, et al, “Field Measurement of Emission Factors of PM, EC, OC, Parent, Nitro-, and Oxy-Polycyclic Aromatic Hydrocarbons for Residential Briquette, Coal Cake, and Wood in Rural Shanxi,” Environmental Science & Technology 47, no. 6 (2013): 2998–3005.
- F. Reisen, and J. Arey, “Atmospheric Reactions Influence Seasonal PAH and nitro-PAH Concentrations in the Los Angeles Basin,” Environmental Science & Technology 39, no. 1 (2005): 64–73.
- Y. Omelekhina, “Be Aware of the Indoor Air. Physicochemical Characterization of Airborne Fine Particles in Occupied Homes” (PhD thesis. Lund University, 2022). https://lucris.lub.lu.se/ws/portalfiles/portal/112558840/phd_thesis_151221_final.pdf.
- H. Fromme, T. Lahrz, M. Piloty, H. Gebhardt, A. Oddoy, and H. Ruden, “Polycyclic Aromatic Hydrocarbons inside and outside of Apartments in an Urban Area,” Science of the Total Environment 326, no. 1–3 (2004): 143–9.
- S. Vardoulakis, E. Giagloglou, S. Steinle, A. Davis, A. Sleeuwenhoek, K. S. Galea, K. Dixon, and J. O. Crawford, “Indoor Exposure to Selected Air Pollutants in the Home Environment: A Systematic Review,” International Journal of Environmental Research and Public Health 17, no. 23 (2020): 8972.
- A. Singh, N. K. Chandrasekharan, R. Kamal, V. Bihari, M. K. Gupta, M. K. R. Mudiam, G. N. V. Satyanarayana, A. Raj, I. Haq, N. K. Shukla, et al, “Assessing Hazardous Risks of Indoor Airborne Polycyclic Aromatic Hydrocarbons in the Kitchen and Its Association with Lung Functions and Urinary PAH Metabolites in Kitchen Workers,” Clinica Chimica Acta; International Journal of Clinical Chemistry 452 (2016): 204–13.
- I. T. K. Nisbet, and P. K. LaGoy, “Toxic Equivalence Factors (TEFs) for Polycyclic Aromatic Hydrocarbons (PAHs,” Regulatory Toxicology and Pharmacology 16, no. 3 (1992): 290–300.
- OEHHA. Air Toxics Hot Spots Program Risk Assessment Guidelines. Part II: Technical Support Document for Describing Available Cancer Potency Factors (Sacramento, CA: Office of Environmental Health Hazard Assessment, 2002).
- European Union (EU) Directive "For Nickel, Cadmium, Mercury, and PAHs in Ambient Air." European Union (EU) Directive 2004/107/EC; EU (Brussels, Belgium, 2004). http://eur-lex.europa.eu.
- F. Marino, A. Cecinato, and P. A. Siskos, “Nitro-PAH in Ambient Particulate Matter in the Atmosphere of Athens,” Chemosphere 40, no. 5 (2000): 533–7.
- K. Cwiklak, J. S. Pastuszka, and R. Rogula-Kozlowska, “Influence of Traffic on Particulate-Matter Polycyclic Aromatic Hydrocarbons in Urban Atmosphere of Zabrze, Poland,” Polish Journal of Environmental Studies 18 (2009): 579–85.
- V. Mugica, S. Hernández, M. Torres, and R. García, “Seasonal Variation of Polycyclic Aromatic Hydrocarbon Exposure Levels in Mexico City,” Journal of the Air & Waste Management Association (1995) 60, no. 5 (2010): 548–55.