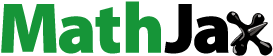
Abstract
Increasing acidity of seawater caused by increasing anthropogenic carbon dioxide absorbed into the seawater attracted the interest of researchers due to increased concern on the deterioration of marine systems and food supply to humans. Total alkalinity (TA) is one of the important parameters in determining carbonate chemistry and is described as the capacity of the sample to neutralize acids. Over the last two decades, many analytical techniques have been developed to determine TA. This article presents a review of different analytical techniques including titration, colorimetric, spectrophotometric, and potentiometric analyses in measuring TA. Among these analytical techniques, potentiometry analysis, which utilizes electrode systems such as glass electrode and ion-selective electrode used as indicator electrodes, is the most used technique. Important features such as principle, limitations, and challenges of the involved technique are discussed in detail.
Introduction
The ocean absorbs almost a quarter of all anthropogenic carbon dioxide (CO2) emissions each year. The absorption of CO2 into the ocean causes calcium carbonate mineral saturation state and seawater pH decline through a process termed ocean acidification. Meanwhile, the exchange of CO2 between the atmosphere and the ocean, as well as the biogeochemical repercussions of this process, is regulated by a set of equilibrium chemical processes and parameters known as the marine carbonate-system.[Citation1] shows the schematic diagram of the carbonate-system.
There are four parameters to characterize the marine carbonate-system, which are total alkalinity (TA), pH, total dissolve inorganic carbon (DIC), and partial carbon dioxide (pCO2).[Citation2,Citation3] One of the most common quantifiable parameters used is TA which can be defined as a chemical measurement of the buffering capacity of water to neutralize acids,[Citation4] which is discussed in this review paper. According to Nand and Ellwood,[Citation5] the alkalinity of seawater is defined as the number of moles of hydrogen ion required to convert bicarbonate to carbonate acid[Citation5–8] and can be expressed using EquationEquation (1)(1)
(1) .
(1)
(1)
TA stands for water quality variables that are important for aquatic ecosystem productivity, aquaculture production, water supply usage, and treatment process for potable water and wastewater. According to Dickson,[Citation9] TA of seawater sample is the number of moles of hydrogen ion equivalent to the excess of proton acceptors over proton donors in 1 kg of a sample.[Citation10]
Alkalinity is the chemical parameter that allows the calculation concentration of bicarbonate system species of CO32−, HCO3−, H2CO3, and CO2 in seawater.[Citation11,Citation12] As a result, alkalinity can be referred to as EquationEquation (2)(2)
(2) .
(2)
(2)
The main sources of alkalinity in surface and subsurface water are carbonic acid-controlled chemical weathering of carbonates, aluminum–silicate minerals such as Mg–Fe–silicates.[Citation13] Silicate minerals are the most common rock-forming minerals. After carbonate weathering, silicates are the dominant buffering mechanisms in natural systems. When silicate minerals dissolve, cations (K+, Na+, Mg2+, Ca2+, Al3+, and Fe2+) are released, and the pH rises. Silicate minerals have the potential to act as long-term sources of alkalinity because their dissolution is slower than that of carbonate minerals.[Citation14] Moreover, TA can be affected by the primary process, which is precipitation and evaporation of the sea surface.[Citation15] The submarine groundwater discharge, river, and formation of dissolution of carbonate minerals can also affect the marine TA locally as well as organic matter cycling, with the degree controlled by runoff and the riverine TA.[Citation16] The measurement of TA has been widely used and routinely conducted as a part of the standard water quality determination in several areas such as in the ocean, marine, and water treatment to interpret and control the process, due to the functionality of the carbonate, bicarbonate, and hydroxide content taken as an indicator of the concentration of this constituent.[Citation17]
Over the past decades, researchers have conducted extensive research using different analytical methods and techniques such as infrared spectroscopy, sample acidification, and single-step acid addition technique to measure TA.[Citation18] However, these techniques have limitations, as the experiment conducted is time-consuming and tedious.[Citation19] Therefore, researchers tried to focus on a new approach and characterize their attractive methodologies using the preferable and current analytical methods such as potentiometric, colorimetric, titration, and spectrophotometric measurements of the sample to improve the system, thus producing a better result. These methods were covered in this review paper because they are the most common analytical techniques to measure TA and they provide reliable and precise measurements of TA.
The ability to measure the TA of water accurately and precisely is important because it is useful in estimating other carbonate-system parameters and characterizing complex biogeochemical processes, because of its stable and conservative nature.[Citation19,Citation20] The common method in determining alkalinity is using the conventional titration method at room temperature with a standard acid solution to calculate the amount of H+ required by titrating a sample to the methyl orange endpoint (pH 4.5). The pH of the titration endpoint is roughly the same as when an amount of H+ is added to react with all of the OH−, CO32−, and HCO3− in the sample to produce CO2 and H2O,[Citation21] which can be expressed using EquationEquation (3)(3)
(3) .
(3)
(3)
However, the measurements were focused more on potentiometry including a dynamic electrochemical approach, which involved monitoring the electrical current to characterize their system and develop interesting methodologies.[Citation22,Citation23]
The purpose of this paper is to review the previous literature as well as current updates on the latest analytical techniques that are commonly used to measure TA including techniques that allow for in-situ measurements. The studies mentioned in this review related to TA measurement aims to reveal the differences between each mentioned technique, for example, the application and materials used for TA measurement with involving analytes. Also, the principle, data converting and other reaction conditions is thoroughly discussed, aiming to provide insight for further development of TA measurement. For example, the color indicators in the titration method were used in getting know the endpoint for TA measurement, meanwhile, the application of the system such as the electrode system used in potentiometric technique is thoroughly discussed. As for the titration method, the titration eliminates the errors due to the sliding endpoint caused by free CO2 in the sample at the time of the titration.[Citation24] For the colorimetric and spectrophotometric techniques, the data converting such as concentration of color compound in solution and intensity of light at a specific wavelength, respectively is also reviewed. This review is organized by grouping the paper into four techniques: titration, colorimetric, spectrophotometric, and potentiometric.
Analytical methods for TA measurement
Titration method
Numerous studies have been reported on the titration method to measure alkalinity. The titration is the addition of certain and precise volumes of reagent to the sample until it reaches a certain pH or endpoint. Often, this method is carried out with standard acid such as hydrochloric acid (HCl) as titrant added until the endpoint pH value is reached, and it was also based on indicators especially phenolphthalein indicator and methyl orange indicator. The endpoint of TA is determined by the color changes of the indicator.[Citation4] However, this method has its limitations such as the time-consuming and the inequality between experimental endpoints and equivalent point.[Citation25]
Tracer-monitored titration (TMT), a new automated titration system for the analysis of seawater TA, free of volumetric and gravimetric measurement, was developed by Martz et al.[Citation26] The alkaline sample was standardized by the mixture of HCl, bromocresol green (BCG), and NaCl at concentrations and ionic strength similar to seawater. TMT is based on dilution factor to quantify the relative proportions of titrant and sample through a chemical tracer (colorimetric indicator) in the titrant. The dilution factors are used in the equivalence point calculation in place of physically metered volume, masses, flow rates, or peak width (time). The sample and titrant are pumped by solenoid pumps to the check valves to prevent reverse breakdown of pump seals. Each pump output is coupled to a low dead volume tee, which connects to the flow cell below the light path, with light collected at the return fiber and split to three dual-band-pass filters. The photocurrent from each photodiode is amplified using a simple two-stage inverting op-amp design. Then the resulting voltage is digitized and logged by the TFX-11 microcomputer (Onset Corp.) control pumps, analog to digital conversion and data collection. The results from sample analysis by examining the sensitivity of the method with the theoretical model showed a good response of the system for the alkalinity determination (R2 = 0.9999), showing that this system is highly accurate and precise.
A new method was developed to calculate TA using ideal Nernst value (ks) with open-cell titration by Okamura et al.[Citation27] The nonlinear least-squares method was conducted by an open-cell alkalinity analyzer with a voltmeter, high input impedance and sensitivity of 0.01 mV. In this research, the seawater sample was acidified with HCl to near pH 3.5 and proceeded until achieved the final pH 3.0. The titration is continued to a pH of about 3 because this enables the equivalency point for the titration to be determined without taking consideration any contributions from carbon dioxide.[Citation9] The total dispensed volume, EMF, and the sample temperature were recorded as well as the temperature of titrant and the E° and TA were calculated by nonlinear least-squares regression. This research shows that TA was successfully calculated using this method.[Citation27]
Zhang et al.[Citation28] presented an automated titration method to simultaneously measure TA and weak organic acid concentrations of the brine with one titration effort. Before the titration alkalinity measurement, the pH of the water sample was measured using a pH electrode, and the filtered sample was sparged with N2 gas containing 1% CO2 until the pH is stable. This indicates that the sample solution is fully saturated with 1% CO2. The N2/CO2 gas was purged into deionized water to avoid water evaporation from the sample. The HCl standard solution (1 N) was delivered into the sample with a slow flow rate of 2 mL h−1 controlled by a syringe pump, so that the solution pH can be restabilized shortly after the introduction of each drop of HCl acid. The pH of the solution was automatically recorded by a data logger. TA and organic acid concentration was determined by obtaining pH value with an amount of HCl added. This software can automatically select 20 evenly spaced points for the curve fitting. The software computes the sum of squared errors of the calculated and observed pH versus the amount of HCl added and minimizes the error by changing the values of alkalinity and THAc via a Newton–Raphson procedure. The automated titration was titrated until pH 3 was achieved, which is the endpoint of the titration method, and the alkalinity equation was modified to accommodate the other types of water samples to include more ionic species encountered in oilfield-produced water. The acetic acid was used as model species of organic acids because of the similar pKa value of approximately 4.7 at standard pressure and temperature conditions. The research shows that the output fits the theoretical equation well with the correlation coefficient (R2) of 0.999 resulting that the automated analytical method can accurately quantify the alkalinity of the solution. Given its accuracy and ease of use, this method could be used in a variety of applications including environment pollution control.[Citation28]
Conventional titrimetric method was used to determine alkalinity against hydrochloric acid using phenolphthalein indicator, followed by methyl orange indicator. Both of the indicators were shown to give direct information on TA and were determined[Citation29] using EquationEquation (4)(4)
(4) :
(4)
(4)
where TA is total alkalinity (mg/L), A is referred to the volume of a titrant with phenolphthalein and methyl orange used during titration, B is referred to the concentration of titrant used during the titration, C is referred to the equivalent weight of CaCO3. With the data obtained, the highest TA value that happened during the winter seasons was concluded as the highest among the sampling places owing to the dissolution of CO2 present in the soil zone, increasing alkaline waters.[Citation29] However, this method is time-consuming and needs further efforts to make them attractive and time-saving.
An autonomous AT system that is the submersible autonomous moored instrument for alkalinity (SAMI-alk) was developed to measure TA in freshwater. This system has the same pattern with Spaulding et al.,[Citation30] which is based on the TMT concept that the acid–base indicator is used to measure both the pH and the amount of acid added. To achieve accurate and precise data, the researchers have extended the utility of SAMI-alk, and it has been thoroughly tested in freshwater conditions and was successfully developed as an in-situ system for freshwater. However, the research faces challenges of using in-situ sensors and analysis in a riverine environment. The excessive amounts of suspended sediments were present in the river, fouling the instruments extensively over the course of the 23 deployments.[Citation31]
TA of filter and unfiltered open-ocean seawater samples were determined using the method described by Hernández et al.[Citation32] Both the filter and unfiltered sample were taken from the same location for comparison. The filtered sample was filtered using a 0.45-µM membrane filter. The accuracy of the two-titration system was verified using several batches of certified reference material (CRM). The data show no differences between filtered and unfiltered samples for open-ocean seawater samples that have been collected during the measurement.[Citation33]
TA measurement of seawater samples was reported by Hunt et al.[Citation34] using a CONTROS HydroFIA in 2021. This method is based on single-point titration of seawater with 0.1 N hydrochloric acid, and bromocresol green (BCG) as an indicator. The instrument collected about 11,000 surfaces TA measurements during six months in 2017 and 2 months in 2018 on the summertime East Coast Ocean Acidification Survey (ECOA-2). The TA sample analysis time required 10 min for the HydroFIA instrument and validate with CRM measurement. The deployment of the CONTROS HydroFIA for TA measurement produced high-quality (2.4-4.1 μmol kg−1) surface TA data and the use of the HydroFIA TA instrument abroad opportunity cruises can significantly increase regional carbonate system monitoring capacity, according to results from 2017 and 2018.[Citation34]
An efficient automated analysis system was developed based on Yao and Bryne’s[Citation35] research, on single-point HCl titration of seawater samples with BCP as an indicator. Relatively, the spectrophotometric titration is indeed accurate and precise, as the result obtained from the sample measurement has a similar standard deviation of 0.03% to the CRMs from the ship’s underway system. However, these methods are needed for further development in alkalinity measurement for the variety of conditions and sample types.[Citation36]
According to Sharp and Bryne,[Citation37] TA can be illustrated through numerical modeling using five TA measurements approaches. Four of them were involved in the analysis of multi-step titration data, and one was involved in the analysis of single-step titration data. The model simulates an alkalinity titration by calculating the concentrations of all acid–base species at the initial conditions specified by the input parameter before replacing them with HCl.[Citation37]
A method by Bruviesh was used for the measurement of total alkalinity in an international experiment organized by the North Pacific Marine Organization (PICES). In this experiment, the Russian Specialist conducted a Bruevish method, using modern burets, Na2CO3 that consists of direct titration of 25 mL seawater with 0.02-N hydrochloric acid in an open-cell. The endpoint titration was determined visually from the solution color changing from greenish to light pink. The method has proved to be easy, requires a minimum length of time, and needs no complicated implementation. This method also proved that there are no errors caused by the imperfect behavior of the measuring electrodes. The results obtained are also in agreement with standard potentiometric titration by Dickson.[Citation9] However, this method needs more attention especially on equivalent points as it needs for the visual fixation.[Citation38]
A description of the bottom-up assessment of the TA, carbonate, and bicarbonate concentrations measurement by acid–base titration with potentiometric detection was presented by Anes et al.[Citation11] where five seawater samples were used to measure TA using the mentioned acid–base titration system. The system consists of a Metrohm 848 Titrino Plus with an Electrode Plus Glass Electrode and a temperature sensor. The HCl solution was used as a titrant in NaCl media to approach seawater ionic strength needed for a stable endpoint detection and to ensure that the activity coefficient remains approximately constant during titration. The titration was monitored by reading the electromotive forces (emf) of the glass electrode. The TA value of the samples was expressed as mean ± standard deviation (repeatability conditioned) and was considered statistically equal with a confidence level of 95% compared with the literature values for TA that have been reported by Millero et al.[Citation11,Citation38,Citation39]
A powerful procedure to measure TA for high-altitude mountain lakes and natural water systems in high-precision and high-accuracy measurement was developed by Kortazar et al.[Citation40] where TA was determined by potentiometric titration, where HCl was standardized using Tris solution and underwent a nonlinear least-squares procedure with volume fitting using the Levenberg–Marquardt algorithm. The authors concluded that the precision of the method is high, showing that this technique might be an attractive methodology for the determination of TA.[Citation40] However, this research needed further investigation because of its limitation in the studied parameter.
Muller and Bleie[Citation7] applied closed-cell, potentiometric titration techniques for Fjord surface water using the previous method proposed by Hernández et al.,[Citation32] for simultaneous determination of TA, pH, TCO2, and organic alkalinity (Org-Alk) of seawater sample. These samples were evaluated using the titration curve. TA is evaluated from the position of the two inflection points in the pH against the volume of the HCl titration plot. Through this research, the technique provides a powerful and hitherto unused tool.[Citation7]
The relationship between the salinity of the sea surface and TA was observed by Jones et al.[Citation41] The authors estimated the TA from salinity at a depth of less than 15 m by routinely taking the water sample for dissolved inorganic carbon (DIC) and calcium carbonate saturation. A dataset of three carbonate-system parameters, measured over 2 years to detect whether TA estimated from salinity and temperature is the reliable predictor and useful parameter for calculating the carbonate-system at depth less than 15 m, was presented, and the data collected were compared with sea surface salinity (SSS) and seawater temperature (SST). The TA measurement was performed using a two-stage, potentiometric, and open-cell titration. Unfortunately, the data indicate that in some coastal environments, estimates of TA based on SSS and SST + SSS do not correlate well, and details of the carbonate-system fluctuations are lost if such estimates are used.[Citation41]
Colorimetric method
Colorimetry is a scientific technique that is used to determine the concentration of colored compounds in solution. It has gained great interest because of their inherent benefits, which include simple operation, adaptable sensitivity, fast response, and long linear range of the quantitative assay that is based on spectrometry.[Citation42] Moreover, this technique is having a color change response visible to the naked eye.[Citation43] According to Zhong et al.[Citation44] in addition to not requiring sophisticated instrumentation, the colorimetric method can convert detection events into color changes that can be observed with the naked eye.[Citation44]
A solid-state sensor couple using colorimetric diffusion titration with modified an ion-sensitive field effect transistor (ISFET) technology for simultaneous measurement of pH and TA was designed by Briggs et al.[Citation45] This optimized sensor for open-ocean seawater over a narrow range of approximately 2200–2500 µmol kg−1, can develop the dual pH-TA sensor with high resistivity and instantaneous with the colorimetric response. Despite these interesting characteristics, it needs further investigation to develop a proper protocol for the evaluation of proton evolution efficiency for the actuator.[Citation45]
An automatic electronic device to measure alkalinity was designed based on visual colorimetric theory. The image detecting technique was combined with discrete curve and automatic identification titration using high-technology software to produce the automatic measurement of TA. The device converts image data from RGB to HSI color space and uses the image saturation value of the solution reaction as a characteristic value to determine when the titration is complete. Phenolphthalein was used as an indicator to measure TA. During the observation, phenolphthalein alkalinity solution pink fade time was used as titration end, and after reaching the titration end, the saturation of the curvature of the curve was calculated to find the inflection point to calculate the titration end. The author concluded that this device has high-precision and can be used to replace visual colorimetric for industrial cooling circulating water alkalinity automatic measurement.[Citation46]
The group of Metzger[Citation47] used an alternative method to measure TA, using the ET probes and adapted the colorimetric technique. In this research, the bases in a sample solution that is water are neutralized by the excess of a weak acid, namely, formic acid, that is mixed with a pH indicator. The active compounds of the colorimetric reagent were represented by the acid that mixed with the pH indicator. These methods were inexpensive and simple. Unfortunately, it was less accurate than the Gran titration method, (about 6% standard deviation).[Citation47] Gran titration was used to determine the endpoint or equivalence point in a potentiometric titration to estimate the TA, which it can replace the currently used first-derivative method for determination of TA. Using this approach, the determination of the endpoint is relatively easy because it requires plotting only the few potential readings that are greater than 300 mV of the data obtained.
Spectrophotometric analysis
Spectrophotometry is the method in which the intensity of light at a specific wavelength is measured and is normally used to identify and quantify raw materials and pharmaceutical products.[Citation48] This technique is used to determine the amount of chemical substance that absorbs light by measuring the intensity of light passing through a sample solution. Each compound absorbs or transmits light over a specific wavelength range, according to the basic principle.[Citation49] Moreover, spectrophotometric methods have several benefits such as being fast, simple, and has low cost.[Citation50]
Yang et al.[Citation51] studied the contributions of Org-Alk to TA in surface water from different coastal environments such as estuary, urban, mangrove, and offshore sites in the Gulf of Mexico. This study is based on spectrophotometric titration for systematic quantification and characterization of organic bases that contribute to TA. The samples were collected for colorimetric measurement of DIC, spectrophotometric pH, and TA. The sample for pH and DIC were collected simultaneously, so that the coupled measurements could be directly used for calculation of the TA of each sample. The TAs for the samples were measured using a single-point spectrophotometric titration method with bromocresol purple (BCP). Throughout each titration, the pH of each sample was continuously monitored by measuring the absorbance ratio (R) of the basic/acidic forms of BCP as the titration work was in progress. The custom-automated titration system used in this research consisted of a USB-4000 fiber optic spectrophotometer, a 665 Dosimat auto-titrator with stir plate and a purging system with high purity N2 gas. The data of pH, DIC, and TA were read by CO2SYS, for carbonate-system calculation, following the same method described by Patsavas et al.[Citation52] where the spectrophotometric pH titration method allows for direct quantification of organic anion contribution to TA. The most negative result (−18.8 µmol kg−1) obtained from the study showed that there are limitations in this indirect approach such as measurement uncertainties or operational errors. However, full-range spectrophotometric pH titration allows for direct quantification of organic anion contributions to TA. The Org-Alk obtained from full-range pH titrations agreed well with the results of indirect measurement. Calculations of the CO2 system involving the DIC–TA pair in the presence of Org-Alk necessitate characterizations of both Org-Alk concentrations and the equilibrium behavior of organic acids/bases that contribute to Org-Alk.[Citation51]
The partial alkalinity (PA) and TA in the anaerobic wastewater were measured and monitored by a new method depending on pH color indicators and read by spectrophotometer. The method was first evaluated offline in manual batch tests on samples taken from an anaerobic reactor (CSTR) system before it was automated in a flow injection analysis (FIA) setup and used for online measurement of PA during overload in a UASB reactor. The PA and TA were measured by offline and online titration measurements. For offline measurements, the reactor was subjected to organic overload, resulting in variations in the partial and TA. For online measurement, the FIA system was used for monitoring the PA. Analyses of PA and TA by titration and by photometry were performed on the supernatants. The authors concluded that there is a good correlation between titration and the photometric method.[Citation53]
A small portable USB-spectrophotometer system was developed by Nand and Ellwood[Citation5] to monitor the alkalinity and pH sample by utilizing the purified bromophenol blue (BPB) as an endpoint indicator. This research was a continuation of work from Okamura et al.[Citation54] by investigating the impact of impurities on the BPB. The comparison of the purified and unpurified BPB, BCG, and BCP were reported, and it was found that the condition of indicator dyes could influence the findings and proved that the impurities of dyes can lower the precision of the results.[Citation5]
Potentiometric analysis
Potentiometric technique is the most widely used technique to determine the TA in water samples. This technique can determine the difference between the potential of two electrodes that are working and indicator electrode respond to the analyte’s activity.[Citation55] It also has benefited from the widespread use of pH electrodes and ion-selective electrodes (ISEs) in many sectors such as environmental, food, clinical, and pharmaceutical applications.[Citation56] This technique provides low cost of samples analysis, time-saving and disposable ISEs, which can improve laboratory’s sample throughput. Examples of ion-selective electrodes for carbonate measurement are screen printed carbon-paste electrode, glassy carbon working electrode and carbonate ion-selective membrane electrode.[Citation57]
Electrochemical sensing based on the liquid ion-selective membrane for direct measurement of TA was studied by Crespo et al.[Citation58] The developed method can be used to determine the three parameters, namely, alkalinity, acidity, and pH with a single membrane. All the parameters measured were different. This is because, by using the same membrane, alkalinity measurement was explored by an anodic current, while acidity measurement was explored using cathodic current and pH was detected by zero current potentiometric.[Citation58]
An electrochemical sensing tool was characterized for the direct measurement of alkalinity and pH by applied current that forces an outward flux of hydrogen ion from an ion-selective membrane into the sample solution. The membrane was synthesized from a commercially available porous polypropylene membrane together with hydrogen ionophore, cation exchanger, lipophilic electrolyte, and plasticizer. This permselective membrane was simultaneously used in chronopotentiometric and zero current potentiometric sensing mode. The predicted chronopotentiogram shows comparable behavior with the obtained chronopotentiogram.[Citation59] This system was consistently being improved by the same research group using an integrated thin-layer electrochemical modulation instrument using two key elements, a selective proton pump membrane and pH probe membrane for in-situ titration. This electrochemical configuration combines dynamic and equilibrium electrochemistry bridged by a thin-layer sample. The researchers concluded that the electrochemical thin-layer proton pump is competent in assessing parameters in-situ without sampling, standardization, and volumetric analysis.[Citation60]
An automated and transportable flow rate system that can conduct continuous TA measurement using an ISFET was developed by Yamamoto et al.[Citation61] The system was altered and equipped with a dual-head peristaltic pump, four-port valve section and custom acrylic plate along with the two plates, DC motor, and mini stirrer system, which make it facilitate easy and rapid in-situ TA measurement to decrease the consumption of electricity and the sizes of the required components. This system is an effective approach as the system measures TA in a shorter time than usual for a titration-based system. A 99% good response was obtained at about 120 s after the sample was switched, and the value remained stable for the next 15 min.[Citation61]
A new fully automated and continuous-flow-through type analyzer to measure rapid changes of TA in coastal zones was developed by Kimoto et al.[Citation62] The method requires continuously adding HCl solution to seawater at controlled flow rates, as well as measuring the acidified solution’s pH with a potentiometric pH meter based on single-step potentiometric procedures. This analyzer was tested on a coral reef flat and achieved a good time resolution that is 1 min. It is also expected that this system can be used for the characterization of rapid temporal changes in the CO2 system in coastal zones.[Citation62]
A novel commercially available flow-through analyzer under laboratory and real field conditions for automated seawater TA measurement was characterized. The experiment was conducted based on single-point open-cell titration with spectrophotometric pH determination, and the performance of the analyzer was compared with the discrete seawater sample results from standard open-cell alkalinity system using potentiometric titration. The overlapping Allan deviation was used to improve confidence of the stability estimate.[Citation10]
Reagentless electroanalytical methodology for automatized acid–base titration of water sample for alkalinity detection was reported. The sample solution that was pumped to the Microfluidic cell consists of top electrode holder; the spacer describing the channel for the thin sample was sandwiched between polyaniline (PANI) film as the pH sensor and a PANI-based electrode as the proton pump and bottom electrode holder to release charge. The pH changes up to pH 4 in the sample were monitored in the pH sensor. The injected charge was translated to a calibration graph for TA determination.[Citation63]
Other analytical methods for TA measurement
A method for correcting measured pore fluid DIC and alkalinity was presented by Sauvage et al.[Citation63] where they presented a technique to quantify in-situ DIC system chemistry in subseafloor sediment. The concept of this research is measurement of three carbonate-system parameters (DIC, TA, and Ca2+) in the pore water permit to mathematically overdetermine the carbonate-system. The method was tested using published pore fluid concentration data from IODP Expedition 329 to the South Pacific gyre. However, this method is valid only if the sediment porewater is saturated with respect to calcium carbonate, or the method will be less accurate.[Citation64]
A unified ion chromatography system combining principle of cation-exchange and acid–base interaction for determination of acidity and alkalinity, respectively, was developed. According to researchers, this developed technique requires a minimum of samples and can be used to determine the other ionic species during the same analysis procedure. Using the HPLC system, lithium dodecyl sulfate (Li-Ds) acts as a stationary phase that is used to determine either free acidity or TA, and the samples were examined three times. According to the authors, this technique is ideal for a variety of applications. However, it was limited to determine the total acidity or the acidity of acids whose corresponding bases were strongly retained by the column.[Citation65]
An automatic online electrochemical cell (EC) to measure alkalinity of wastewater was presented. This method is based on electrolysis of water, where water molecules (H2O) are split into oxygen and hydrogen with the application of sufficient voltage to form H+ and hydroxide ions (OH−) at the anode and cathode of an EC. The detection of online EC is based on in-situ titration caused by anodic proton (H+) generation or hydroxide (OH) consumption. The H+ generation or OH consumption was calculated based on the electron flow, with the assumption that one H+ is generated or one OH is consumed for each electron. The EC setup was formed by separating two acrylic compartments with a cation-exchange membrane. Each compartment was packed with conductive graphite granules and were connected to graphite electrodes. The EC was sequentially connected to an external resistor, and the cell was connected to a power supply with a total voltage output of about 3.5 V. The current and pH in the chambers were recorded using the LabVIEW 7.1 software in conjunction with a national instrument data acquisition card (DAQ). The pH of the solution was monitored online throughout the experiment. There are five solutions that were tested using EC and the alkalinity data of EC for the solutions were compared with the conventional chemical titration method. The data obtained from both methods shown match well and were almost identical. The results show a strong correlation (R2 = 0.9970) between the online EC detection system and the traditional titration method for determining TA. This system can be used further as a simple online titration device to monitor the buffer capacity of various industrial processes as there is no need for the preparation of chemicals.[Citation66]
In general, determination of TA is not only limited to the mentioned technique; hence, a more extended review of total alkalinity determination is also presented in .
Table 1. Summary table of analytical techniques involved for TA measurement.
Challenges and limitation of TA measurement
Several analytical techniques are widely used to measure TA, such as titration, colorimetric, spectrophotometric, and potentiometric analysis. They are considered as the technique to give highly accurate and precise information about carbonate chemistry seawater. However, this technique also has drawbacks when carried out on board. For example, it takes some time to analyze TA because the electrode requires time to modify EMF.[Citation82] Although alkalinity testing can be performed in a variety of techniques, each test variation provides information about the sample in a different way. Alkalinity is primarily found as carbonate (CO32−) and hydroxide (OH) salts above pH 8.3. Alkalinity is mostly found as bicarbonate (HCO3) salts between pH 4.5 and pH 8.3. At pH 4.5, these salts’ buffering capacity is depleted, and it is almost completely converted to carbonic acid (H2CO3). The different alkalinity constituents are present in different proportions depending on pH, and these various forms of alkalinity can be determined by adjusting the titration endpoint. According to Lahav,[Citation82] most laboratories currently measure alkalinity by titration to a predetermined endpoint. The difficulty in measuring alkalinity arises from its low value, which is commonly less than 10 mg/L as CaCO3 and can be as low 1 mg/L, as well as an unknown titration endpoint.[Citation82] Standard methods (1998) propose an approximate method based on titration to an arbitrary endpoint for these waters in between 4.3 and 4.7. However, this leads to large relative errors.[Citation82]
Conclusions
The progress in the measurement technique for total alkalinity has been the main interest for the environmental chemist. Intensive anthropogenic activities have increased the burden on the environment and indirectly increased the concentration of CO2 in the atmosphere. This has led to the increasing trend of pollution monitoring through carbonate chemistry parameters. Over the years, TA has been gaining wide attention for water monitoring due to its stability and accuracy. After reviewing the past and current literature on the analytical technique for TA measurement, several important trends were summarized. First, an increasing number of studies reported the use of potentiometric as the detection system for analysis. Based on the available and latest literature, the use of potentiometric technique is faster compared to other analytical methods, and good response due to the electrode system that provide immediate reaction as described by Yamamoto et al.[Citation61] and Kimoto et al.[Citation62]. Second, an increasing number of researchers reported on the development of titration method in a single-step titration. Numerous titration methods have increased because of these techniques were used as validation and comparison methods as stated in . Third, an increasing trend was seen for in-situ measurement, which can provide time-saving without sample preservation while using less reagent. Based on the discussed analytical techniques for TA measurement, the advantages, disadvantages, and opportunities for each technique will allow improvements in developing the future system to measure TA.
Declaration of competing interest
The authors declare that they have no known competing financial interests or personal relationships that could have appeared to influence the work reported in this paper.
Additional information
Funding
References
- Humphreys, M. P.; Lewis, E. R.; Sharp, J. D.; Pierrot, D. PyCO2SYS v1.8: Marine Carbonate System Calculations in Python. Geosci. Model Dev. 2022, 15, 15–43. DOI: 10.5194/gmd-15-15-2022.
- Seelmann, K.; Steinhoff, T.; Aßmann, S.; Körtzinger, A. Enhance Ocean Carbon Observations: Successful Implementation of a Novel Autonomous Total Alkalinity Analyzer on a Ship of Opportunity. Front. Mar. Sci. 2020, 7, 1–18. DOI: 10.3389/fmars.2020.571301.
- Takeshita, Y.; Johnson, K. S.; Coletti, L. J.; Jannasch, H. W.; Walz, P. M.; Warren, J. K. Assessment of PH Dependent Errors in Spectrophotometric PH Measurements of Seawater. Mar. Chem. 2020, 223, 103801. DOI: 10.1016/j.marchem.2020.103801.
- Dunnivant, F. M. Determination of Alkalinity of Natural Waters. In Environmental Laboratory Exercises for Instrumental Analysis and Environmental Chemistry; John Wiley & Sons, Inc.: Hoboken, NJ, USA, 2005, pp 245–256. DOI: 10.1002/0471660280.ch21.
- Nand, V.; Ellwood, M. J. A Simple Colorimetric Method for Determining Seawater Alkalinity Using Bromophenol Blue. Limnol. Oceanogr. Methods 2018, 16, 401–410. DOI: 10.1002/lom3.10253.
- DOE. Handbook of Methods for the Analysis of the Various Parameters of the Carbon Dioxide System in Sea Water, Version 2.; Dickson, A. G., Goyet, C., Eds. 1997.
- Muller, F. L. L.; Bleie, B. Estimating the Organic Acid Contribution to Coastal Seawater Alkalinity by Potentiometric Titrations in a Closed Cell. Anal. Chim. Acta 2008, 619, 183–191. DOI: 10.1016/j.aca.2008.05.018.
- Middelburg, J. J.; Soetaert, K.; Hagens, M. Ocean Alkalinity, Buffering and Biogeochemical Processes. Rev. Geophys. 2020, 58, 1–28. DOI: 10.1029/2019RG000681.
- Dickson, A. G.; Afghan, J. D.; Anderson, G. C. Reference Materials for Oceanic CO2 Analysis: A Method for the Certification of Total Alkalinity. Mar. Chem. 2003, 80, 185–197. DOI: 10.1016/S0304-4203(02)00133-0.
- Seelmann, K.; Aßmann, S.; Körtzinger, A. Characterization of a Novel Autonomous Analyzer for Seawater Total Alkalinity: Results from Laboratory and Field Tests. Limnol. Oceanogr. Methods 2019, 17, 515–532. DOI: 10.1002/lom3.10329.
- Anes, B.; Bettencourt da Silva, R. J. N.; Oliveira, C.; Filomena Camões, M. Uncertainty Evaluation of Alkalinity Measurements on Seawater Samples. Meas. J. Int. Meas. Confed. 2018, 129, 395–404. DOI: 10.1016/j.measurement.2018.07.042.
- Fine, R. A.; Willey, D. A.; Millero, F. J. Global Variability and Changes in Ocean Total Alkalinity from Aquarius Satellite Data. Geophys. Res. Lett. 2017, 44, 261–267. DOI: 10.1002/2016GL071712.
- Reimer, A.; Arp, G. Alkalinity. In Encyclopedia of Geobiology; Reitner, T., Ed.; Springer: Göttingen, Germany, 2011, pp 20–24. DOI: 10.1007/978-1-4020-9212-1_8.
- Lacroix, E.; Brovelli, A.; Barry, D. A.; Holliger, C. Use of Silicate Minerals for PH Control during Reductive Dechlorination of Chloroethenes in Batch Cultures of Different Microbial Consortia. Appl. Environ. Microbiol. 2014, 80, 3858–3867. DOI: 10.1128/AEM.00493-14.
- Fry, C. H.; Tyrrell, T.; Hain, M. P.; Bates, N. R.; Achterberg, E. P. Analysis of Global Surface Ocean Alkalinity to Determine Controlling Processes. Mar. Chem. 2015, 174, 46–57. DOI: 10.1016/j.marchem.2015.05.003.
- Broullón, D.; Pérez, F. F.; Velo, A.; Hoppema, M.; Olsen, A.; Takahashi, T.; Key, R. M.; Tanhua, T.; González-Dávila, M.; Jeansson, E.; et al. A Global Monthly Climatology of Total Alkalinity: A Neural Network Approach. Earth Syst. Sci. Data. 2019, 11, 1109–1127. DOI: 10.5194/essd-11-1109-2019.
- Omer, N. H. Water Quality Parameters. In Water Quality - Science, Assessments and Policy; IntechOpen: Washington, DC, 2020, pp 1–18. DOI: 10.5772/intechopen.89657.
- Omer, H. E.; Hafez, B.; Alshamsi, M. S.; Ghani, M. A. Online Novel Techniques for Selective Simultaneous Determination of Alkalinity/Bicarbonate and Hardness in Drinking Water by Skalar San++ Segmented Flow Analysis. Moroccan J. Chem. 2020, 8, 124–146. DOI: 10.48317/IMIST.PRSM/morjchem-v8i1.18188.
- Sivashankari, P. R.; Prabaharan, M. Deacetylation Modification Techniques of Chitin and Chitosan. In Chitosan Based Biomaterials Volume 1; Elsevier: Chennai, India. 2017, Vol. 1, pp 117–133. DOI: 10.1016/B978-0-08-100230-8.00005-4.
- Bushinsky, S. M.; Takeshita, Y.; Williams, N. L. Observing Changes in Ocean Carbonate Chemistry: Our Autonomous Future. Curr. Clim. Change Rep. 2019, 5, 207–220. DOI: 10.1007/s40641-019-00129-8.
- Boyd, C. E. Water Quality: An Introduction, Springer: Auburn, AL, 2015; pp 1–357. DOI: 10.1007/978-3-319-17446-4.
- Matamoros, V. Comprehensive Sampling and Sample Preparation; Elsevier: Girona, Spain, 2012, Vol. 1. DOI: 10.1016/B978-0-12-381373-2.10013-4.
- Steininger, F.; Zieger, S. E.; Koren, K. Dynamic Sensor Concept Combining Electrochemical PH Manipulation and Optical Sensing of Buffer Capacity. Anal. Chem. 2021, 93, 3822–3829. DOI: 10.1021/acs.analchem.0c04326.
- Muralikrishna, I. V.; Manickam, V. Analytical Methods for Monitoring Environmental Pollution, 2017; pp 495–570. DOI: 10.1016/b978-0-12-811989-1.00018-x.
- Wang, X.; Bai, X.; Li, Z.; Zhou, X.; Cheng, S.; Sun, J.; Liu, T. Evaluation of Artificial Neural Network Models for Online Monitoring of Alkalinity in Anaerobic Co-Digestion System; Elsevier B.V.: Beijing, PR China, 2018, Vol. 140. DOI: 10.1016/j.bej.2018.09.010.
- Martz, T. R.; Dickson, A. G.; DeGrandpre, M. D. Tracer Monitored Titrations: Measurement of Total Alkalinity. Anal. Chem. 2006, 78, 1817–1826. DOI: 10.1021/ac0516133.
- Okamura, K.; Kimoto, H.; Hatta, M.; Noguchi, T.; Nakaoka, A.; Suzue, T.; Kimoto, T. Potentiometric Open-Cell Titration for Seawater Alkalinity considering Temperature Dependence of Titrant Density and Nernst Response of PH Electrode. Geochem. J. 2014, 48, 153–163. DOI: 10.2343/geochemj.2.0296.
- Zhang, P.; Huang, S.; Zhang, N.; Kan, A. T.; Tomson, M. B. Automated Analytical Method to Determine Solution Alkalinity of Oilfield Brine in the Presence of Weak Organic Acids. Ind. Eng. Chem. Res. 2019, 58, 4667–4673. DOI: 10.1021/acs.iecr.8b05333.
- Varale, A.; Varale, Y. Study of Total Alkalinity Present in the Industrial Effluent (Water Sample) of Nipani Town. J. Chem. Pharm. Res. 2013, 5, 226–229.
- Spaulding, R. S.; Degrandpre, M. D.; Beck, J. C.; Hart, R. D.; Peterson, B.; De Carlo, E. H.; Drupp, P. S.; Hammar, T. R. Autonomous in Situ Measurements of Seawater Alkalinity. Environ. Sci. Technol. 2014, 48, 9573–9581. DOI: 10.1021/es501615x.
- Shangguan, Q.; Lai, C. Z.; Beatty, C. M.; Young, F. L.; Spaulding, R. S.; DeGrandpre, M. D. Autonomous in Situ Measurements of Freshwater Alkalinity. Limnol Oceanogr Methods 2021, 19, 51–66. DOI: 10.1002/lom3.10404.
- Hernández-Ayón, J. M.; Belli, S. L.; Zirino, A. pH, Alkalinity and Total CO2 in Coastal Seawater by Potentiometric Titration with a Difference Derivative Readout. Anal. Chim. Acta. 1999, 394, 101–108. DOI: 10.1016/S0003-2670(99)00207-X.
- Chanson, M.; Millero, F. J. Effect of Filtration on the Total Alkalinity of Open-Ocean Seawater. Limnol. Oceanogr. Methods. 2007, 5, 293–295. DOI: 10.4319/lom.2007.5.293.
- Hunt, C. W.; Salisbury, J. E.; Vandemark, D.; Aßmann, S.; Fietzek, P.; Melrose, C.; Wanninkhof, R.; Azetsu-Scott, K. Variability of USA East Coast Surface Total Alkalinity Distributions Revealed by Automated Instrument Measurements. Mar. Chem. 2021, 232, 103960. DOI: 10.1016/j.marchem.2021.103960.
- Yao, W.; Byrne, R. H. Simplified Seawater Alkalinity Analysis: Use of Linear Array Spectrometers. Deep. Res. Part I Oceanogr. Res. Pap. 1998, 45, 1383–1392. DOI: 10.1016/S0967-0637(98)00018-1.
- Liu, X.; Byrne, R. H.; Lindemuth, M.; Easley, R.; Mathis, J. T. An Automated Procedure for Laboratory and Shipboard Spectrophotometric Measurements of Seawater Alkalinity: Continuously Monitored Single-Step Acid Additions. Mar. Chem. 2015, 174, 141–146. DOI: 10.1016/j.marchem.2015.06.008.
- Sharp, J. D.; Byrne, R. H. Interpreting Measurements of Total Alkalinity in Marine and Estuarine Waters in the Presence of Proton-Binding Organic Matter. Deep. Res. Part I Oceanogr. Res. Pap. 2020, 165, 103338. DOI: 10.1016/j.dsr.2020.103338.
- Pavlova, G. Y.; Tishchenko, P. Y.; Volkova, T. I.; Dickson, A.; Wallmann, K. Intercalibration of Bruevich’s Method to Determine the Total Alkalinity in Seawater. Oceanology 2008, 48, 438–443. DOI: 10.1134/S0001437008030168.
- Millero, F. J.; Lee, K.; Roche, M. Distribution of Alkalinity in the Surface Waters of the Major Oceans. Mar. Chem. 1998, 60, 111–130. DOI: 10.1016/S0304-4203(97)00084-4.
- Kortazar, L.; Duval, B.; Liñero, O.; Olamendi, O.; Angulo, A.; Amouroux, D.; de Diego, A.; Fernandez, L. A. Accurate Determination of the Total Alkalinity and the CO2 System Parameters in High-Altitude Lakes from the Western Pyrenees (France – Spain). Microchem. J. 2020, 152, 104345. DOI: 10.1016/j.microc.2019.104345.
- Jones, J. M.; Sweet, J.; Brzezinski, M. A.; McNair, H. M.; Passow, U. Evaluating Carbonate System Algorithms in a Nearshore System: Does Total Alkalinity Matter? PLoS One. 2016, 11, e0165191. DOI: 10.1371/journal.pone.0165191.
- Zhu, X.; Gao, T. Spectrometry; Elsevier Inc.: Shanghai, P. R. China, 2019. DOI: 10.1016/B978-0-12-815053-5.00010-6.
- Nazifi, M.; Ahmadi, R.; Ramezani, A. M.; Absalan, G. Introducing Hierarchical Hollow MnO2 Microspheres as Nanozymes for Colorimetric Determination of Captopril. Anal. Bioanal. Chem. 2021, 413, 7063–7072. DOI: 10.1007/s00216-021-03672-2.
- Zhong, Q.; Chen, Y.; Su, A.; Wang, Y. Synthesis of Catalytically Active Carbon Quantum Dots and Its Application for Colorimetric Detection of Glutathione. Sensors Actuators, B Chem. 2018, 273, 1098–1102. DOI: 10.1016/j.snb.2018.07.026.
- Briggs, E. M.; Sandoval, S.; Erten, A.; Takeshita, Y.; Kummel, A. C.; Martz, T. R. Solid State Sensor for Simultaneous Measurement of Total Alkalinity and PH of Seawater. ACS Sens. 2017, 2, 1302–1309. DOI: 10.1021/acssensors.7b00305.
- Cao, S.; Huang, D.; Wang, Y.; Li, G. Phenolphthalein Alkalinity Testing Research Based on Computer Vision Technology and Electronic Technology. Lect. Notes Electr. Eng. 2012, 177 LNEE, 381–386. DOI: 10.1007/978-3-642-31516-9_61.
- Metzger, E.; Viollier, E.; Simonucci, C.; Prévot, F.; Langlet, D.; Jézéquel, D. Millimeter-Scale Alkalinity Measurement in Marine Sediment Using DET Probes and Colorimetric Determination. Water Res. 2013, 47, 5575–5583. DOI: 10.1016/j.watres.2013.06.038.
- Akash, M. S. H.; Rehman, K. Essentials of Pharmaceutical Analysis. Springer: Berlin, 2019; pp 1–222. DOI: 10.1007/978-981-15-1547-7.
- Mamqadr, G. S. Spectrophotometric Determination of Diclofenac in Pharmaceutical Preparation; Salahaddin University- Erbil (SUE), Kurdistan Regional Government-Iraq. 2020. DOI: 10.1590/s0103-50532005000600026.
- Nguyen, T. D.; Le, H. B.; Dong, T. O.; Pham, T. D. Determination of Fluoroquinolones in Pharmaceutical Formulations by Extractive Spectrophotometric Methods Using Ion-Pair Complex Formation with Bromothymol Blue. J. Anal. Methods Chem. 2018, 2018, 8436948. DOI: 10.1155/2018/8436948.
- Yang, B.; Byrne, R. H.; Lindemuth, M. Contributions of Organic Alkalinity to Total Alkalinity in Coastal Waters: A Spectrophotometric Approach. Mar. Chem. 2015, 176, 199–207. DOI: 10.1016/j.marchem.2015.09.008.
- Patsavas, M. C.; Byrne, R. H.; Yang, B.; Easley, R. A.; Wanninkhof, R.; Liu, X. Procedures for Direct Spectrophotometric Determination of Carbonate Ion Concentrations: Measurements in US Gulf of Mexico and East Coast Waters. Mar. Chem. 2015, 168, 80–85. DOI: 10.1016/j.marchem.2014.10.015.
- Jantsch, T. G.; Mattiasson, B. A Simple Spectrophotometric Method Based on Ph-Indicators for Monitoring Partial and Total Alkalinity in Anaerobic Processes. Environ. Technol. 2003, 24, 1061–1067. DOI: 10.1080/09593330309385646.
- Okamura, K.; Kimoto, H.; Kimoto, T. Open-Cell Titration of Seawater for Alkalinity Measurements by Colorimetry Using Bromophenol Blue Combined with a Non-Linear Least-Squares Method. Anal. Sci. 2010, 26, 709–713. DOI: 10.2116/analsci.26.709.
- Adeli, A.; Khoshnood, R. S.; Beyramabadi, S. A.; Pordel, M.; Morsali, A. Multivariate Optimization of a Novel Potentiometric Sensor to Determine Silver Ions in Real Water and Pharmacological Product Samples. Monatsh Chem. 2022, 153, 227–235. DOI: 10.1007/s00706-022-02904-0.
- Gil, R. L.; Amorim, C. G.; Montenegro, M. C. B. S. M.; Araújo, A. N. Potentiometric Detection in Liquid Chromatographic Systems: An Overview. J. Chromatogr. A 2019, 1602, 326–340. DOI: 10.1016/j.chroma.2019.06.006.
- Li, G.; Lyu, X.; Wang, Z.; Rong, Y.; Hu, R.; Luo, Z.; Wang, Y. All-Solid-State Carbonate-Selective Electrode Based on Screen-Printed Carbon Paste Electrode. Meas. Sci. Technol. 2017, 28, 025104. DOI: 10.1088/1361-6501/aa52a9.
- Crespo, G. A.; Ghahraman Afshar, M.; Bakker, E. Direct Detection of Acidity, Alkalinity, and PH with Membrane Electrodes. Anal. Chem. 2012, 84, 10165–10169. DOI: 10.1021/ac302868u.
- Afshar, M. G.; Crespo, G. A.; Xie, X.; Bakker, E. Direct Alkalinity Detection with Ion-Selective Chronopotentiometry. Anal. Chem. 2014, 86, 6461–6470. DOI: 10.1021/ac500968c.
- Afshar, M. G.; Crespo, G. A.; Bakker, E. Thin-Layer Chemical Modulations by a Combined Selective Proton Pump and PH Probe for Direct Alkalinity Detection. Angew. Chem. 2015, 127, 8228–8231. DOI: 10.1002/ange.201500797.
- Yamamoto, S.; Kayanne, H.; Fujita, N.; Sato, Y.; Kurihara, H.; Harii, S.; Hemmi, A.; Dickson, A. G. Development of an Automated Transportable Continuous System to Measure the Total Alkalinity of Seawater. Talanta 2021, 221, 121666. DOI: 10.1016/j.talanta.2020.121666.
- Kimoto, H.; Kayanne, H.; Kudo, S.; Nozaki, K.; Negishi, A.; Kato, K. A High Time-Resolution Analyzer for Total Alkalinity of Seawater, Based on Continuous Potentiometric Measurement. Anal. Sci. 2001, 17, i415–i418. https://www.researchgate.net/publication/281609053_A_high_time-resolution_analyzer_for_total_alkalinity_of_seawater_based_on_continuous_potentiometric_measurement.
- Wiorek, A.; Hussain, G.; Molina-Osorio, A. F.; Cuartero, M.; Crespo, G. A. Reagentless Acid-Base Titration for Alkalinity Detection in Seawater. Anal. Chem. 2021, 93, 14130–14137. DOI: 10.1021/acs.analchem.1c02545.
- Sauvage, J.; Spivack, A. J.; Murray, R. W.; D'Hondt, S. Determination of in Situ Dissolved Inorganic Carbon Concentration and Alkalinity for Marine Sedimentary Porewater. Chem. Geol. 2014, 387, 66–73. DOI: 10.1016/j.chemgeo.2014.06.010.
- Hu, W.; Hasebe, K.; Iles, A.; Tanaka, K. A Unified Ion Chromatographic System for the Determination of Acidity and Alkalinity. Anal. Sci. 2001, 17, 1401–1404. DOI: 10.2116/analsci.17.1401.
- Cheng, L.; Charles, W.; Cord-Ruwisch, R. Automatic Online Buffer Capacity (Alkalinity) Measurement of Wastewater Using an Electrochemical Cell. Environ. Technol. 2016, 37, 2467–2472. DOI: 10.1080/09593330.2016.1152307.
- Woosley, R. J.; Millero, F. J.; Takahashi, T. Internal Consistency of the Inorganic Carbon System in the Arctic Ocean. Limnol. Oceanogr. Methods 2017, 15, 887–896. DOI: 10.1002/lom3.10208.
- Yilkal, E.; Zewge, F.; Chandravanshi, B. S. Assessment of the Quality of Bottled Water Marketed in Addis Ababa, Ethiopia. Bull. Chem. Soc. Eth 2019, 33, 21–41. DOI: 10.4314/bcse.v33i1.3.
- Watanabe, A.; Kayanne, H.; Nozaki, K.; Kato, K.; Negishi, A.; Kudo, S.; Kimoto, H.; Tsuda, M.; Dickson, A. G. A Rapid, Precise Potentiometric Determination of Total Alkalinity in Seawater by a Newly Developed Flow-through Analyzer Designed for Coastal Regions. Mar. Chem. 2004, 85, 75–87. DOI: 10.1016/j.marchem.2003.09.004.
- Griffin, A. J.; Anderson, Z.; Ballard, J.; Bates, N. R.; Garley, R.; Johnson, R.; Martz, T.; Pacheco, F.; Takeshita, Y.; Andersson, A. J. Seasonal Changes in Seawater Calcium and Alkalinity in the Sargasso Sea and across the Bermuda Carbonate Platform. Mar. Chem. 2022, 238, 104064. DOI: 10.1016/j.marchem.2021.104064.
- Kuliński, K.; Schneider, B.; Hammer, K.; Machulik, U.; Schulz-Bull, D. The Influence of Dissolved Organic Matter on the Acid-Base System of the Baltic Sea. J. Mar. Syst. 2014, 132, 106–115. DOI: 10.1016/j.jmarsys.2014.01.011.
- Bennett, W. W.; Welsh, D. T.; Serriere, A.; Panther, J. G.; Teasdale, P. R. A Colorimetric DET Technique for the High-Resolution Measurement of Two-Dimensional Alkalinity Distributions in Sediment Porewaters. Chemosphere 2015, 119, 547–552. DOI: 10.1016/j.chemosphere.2014.07.042.
- Metzger, E.; Barbe, A.; Cesbron, F.; Thibault de Chanvalon, A.; Jauffrais, T.; Jézéquel, D.; Mouret, A. Two-Dimensional Ammonium Distribution in Sediment Pore Waters Using a New Colorimetric Diffusive Equilibration in Thin-Film Technique. Water Res. X. 2019, 2, 100023. DOI: 10.1016/j.wroa.2018.100023.
- Gaiao, E. d N.; Martins, V. L.; Lyra, W. d S.; Almeida, L. F. d.; Silva, E. C. d.; Araújo, M. C. U. Digital Image-Based Titrations. Anal. Chim. Acta. 2006, 570, 283–290. DOI: 10.1016/j.aca.2006.04.048.
- Li, Q.; Wang, F.; Wang, Z. A.; Yuan, D.; Dai, M.; Chen, J.; Dai, J.; Hoering, K. A. Automated Spectrophotometric Analyzer for Rapid Single-Point Titration of Seawater Total Alkalinity. Environ. Sci. Technol. 2013, 47, 11139–11146. DOI: 10.1021/es402421a.
- Steinsberger, T.; Kathriner, P.; Meier, P.; Mistretta, A.; Hauser, P. C.; Müller, B. A Portable Low Cost Coulometric Micro-Titrator for the Determination of Alkalinity in Lake and Sediment Porewaters. Sensors Actuators, B Chem. 2018, 255, 3558–3563. DOI: 10.1016/j.snb.2017.09.191.
- Gray, S. M.; Ellis, P. S.; Grace, M. R.; McKelvie, I. D. Underway Determination of Alkalinity in Estuarine Waters by Reagent-Injection Gas-Diffusion Flow Analysis. Talanta 2008, 77, 533–540. DOI: 10.1016/j.talanta.2008.03.020.
- Lancellotti, B. V.; Bercaw, R. J.; Loomis, G. W.; Hoyt, K. P.; Avizinis, E. J.; Amador, J. A. Accuracy of Rapid Tests Used for Analysis of Advanced Onsite Wastewater Treatment System Effluent. Water Air Soil Pollut. 2016, 227, 1–12. DOI: 10.1007/s11270-016-3023-8.
- Priyanka, K.; Shanthi, R.; Poornima, D.; Saravanakumar, A.; Roy, R.; Nagamani, P. V. Long-Term Variability of Satellite Derived Total Alkalinity in the Southwest Bay of Bengal. Quat. Sci. Adv. 2022, 8, 100066. DOI: 10.1016/j.qsa.2022.100066.
- Li, X.; Bellerby, R. G. J.; Wallhead, P.; Ge, J.; Liu, J.; Liu, J.; Yang, A. A Neural Network-Based Analysis of the Seasonal Variability of Surface Total Alkalinity on the East China Sea Shelf. Front. Mar. Sci. 2020, 7, 1–13. DOI: 10.3389/fmars.2020.00219.
- Carter, B. R.; Williams, N. L.; Gray, A. R.; Feely, R. A. Locally Interpolated Alkalinity Regression for Global Alkalinity Estimation. Limnol. Oceanogr. Methods. 2016, 14, 268–277. DOI: 10.1002/lom3.10087.
- Lahav, O.; Morgan, B. E.; Loewenthal, R. E.; Rosich, R. S. Comments on: “Measurement of pH, Alkalinity and Acidity in Ultra-Soft Waters”. WSA 2002, 28, 346–347. DOI: 10.4314/wsa.v28i3.4906.