Abstract
Replacing conventional solvents with deep eutectic solvents (DES) has shown promising effects on the extraction yield of (poly)phenols. DES can be combined with ultrasound-assisted extraction (UAE) to further increase the extraction efficiency of (poly)phenols from natural resources compared to conventional methods. This review discusses the factors associated with DES (composition, solvent-to-sample ratio, extraction duration, and temperature) and UAE (ultrasound frequency, power, intensity, and duty cycle) methods that influence the extraction of (poly)phenols and informs future improvements required in the optimization of the extraction process. For the optimum (poly)phenol extraction from natural resources, the following parameters shall be considered: ultrasound frequency should be in the range of 20–50 kHz, ultrasound intensity in the range of 60–120 W/cm2, ultrasound duty cycle in the range of 40–80%, ultrasound duration for 10–30 minutes, and ultrasound temperature for 25–50 °C. Among the reported DES systems, choline chloride with glycerol or lactic acid, with a solvent-to-sample mass ratio of 10–30:1 shown to be effective. The solvent composition and solvent-to-sample mass ratio should be selected according to the target compound and the source material. However, the high viscosity of DES is among the major limitations. Optimizing these factors can help to increase the yield of extracted (poly)phenols and their applications.
Introduction
(Poly)phenols are naturally occurring substances in plants. Because of the structural diversity, (poly)phenols have been divided into categories based on the similarities between their underlying chemical skeletons. They are classified into six major groups of phenolic acids, flavonoids, tannins, lignans, coumarins, and stilbenes. (Poly)phenols have been gaining growing interest because of their potential health benefits, which include antioxidant,[Citation1,Citation2] anti-bacterial,[Citation3,Citation4] anti-inflammatory,[Citation5,Citation6] anti-osteoporosis,[Citation7,Citation8] and anti-carcinogenic effects.[Citation9,Citation10]
(Poly)phenols have been extracted from numerous natural sources using various processes including maceration, decoction, percolation, infusion, digestion, exhaustive serial extraction, and Soxhlet extraction.[Citation11] However, traditional extraction processes often involve the use of toxic solvents that are not environmentally friendly and can pose safety risks to consumers.[Citation12] Green extraction liquids, also known as eco-friendly solvents, have recently gained considerable attention as replacements for conventional toxic organic solvents especially for food, nutraceutical, cosmeceutical, and pharmaceutical applications.[Citation13] Among the green extraction solvents and technologies, deep eutectic solvents (DES) have recently gained attention as a promising new category of solvents with the advantages of low toxicity, low cost, and biodegradability. DESs are formed by mixing two or more low-molecular-weight compounds to form a new, low-melting-point complex with unique solubility and physicochemical properties.[Citation14] The concept of using eutectic mixtures as solvents has been investigated widely ().[Citation15,Citation16,Citation22–24] For instance, choline chloride (ChCl) and urea (Ur) form a DES with high solubility for several organic compounds.[Citation25]
Table 1. Ultrasound factors that impact the extraction of (poly)phenols using DES.
Ultrasound-assisted extraction (UAE) is a technique that utilizes ultrasonic waves to enhance the extraction of compounds from various matrices.[Citation26] The ultrasound waves create pressure changes that generate cavitation bubbles, leading to the formation and collapse of bubbles, resulting in the release of energy. The collapse of bubbles causes high shear forces and localized high temperatures, leading to cell disruption and improved mass transfer, ultimately increasing the extractability of compounds.[Citation27] Due to its remarkable speed, efficiency, and reduced solvent usage, UAE has emerged as a promising alternative to conventional extraction methods among these green extraction techniques.[Citation28,Citation29] UAE increases the mass transfer rate between the sample and the solvent, resulting in more efficient extraction of target compounds.[Citation30,Citation31] Moreover, ultrasonic energy could be used to improve the extraction of target compounds from plant material.[Citation32] Significant growth in studies investigating the potential of the UAE and its many potential applications may be seen in the last few years, such as food analysis,[Citation33] natural product extraction,[Citation34] and pharmaceuticals.[Citation35] According to these studies, UAE has the potential to be a green alternative to conventional extraction methods, with increased extraction efficiency, reduced solvent usage, and improved target compound extraction. Thus, this literature review aims to review the UAE applications with DESs for extraction (poly)phenols to help researchers understand the considerations that need to be implemented in performing this method.
Introduction to DES properties
DES have garnered considerable attention in recent years due to their unique and versatile attributes, rendering them promising candidates for a multitude of applications in both industry and the scientific realm. A prominent hallmark of DES lies in their remarkable stability, encompassing both chemical and thermal aspects, affording them exceptional resistance to decomposition and the capacity to withstand a broad spectrum of environmental conditions. This intrinsic durability positions them as enduring and dependable choices for practical applications.[Citation36]
Furthermore, DES displays distinctive viscosity and density characteristics, endowing them with a considerable degree of adjustability to fulfill specific application prerequisites. Their viscosity spans the gamut from highly viscous to comparatively low-viscosity states, offering customization opportunities commensurate with the specific requirements of a given application. Concurrently, their density can be fine-tuned to align with the solubility needs of diverse compounds.[Citation37]
The melting temperature of DES assumes paramount importance, profoundly influencing their ease of manipulation and application. DES typically feature lower melting points compared to conventional ionic liquids, rendering them more accessible and cost-effective for deployment across a wider spectrum of applications.[Citation38]
Surface tension stands as another noteworthy attribute of DES, underscoring its pivotal role in mediating interactions with other substances. DES can manifest surface tensions lower than that of water, facilitating their compatibility with a diverse array of organic and inorganic materials, thereby enhancing their wetting and spreading capabilities.[Citation39]
Moreover, DES are acknowledged for their remarkable miscibility with a wide range of compounds, irrespective of their polarity, rendering them outstanding solvents for a diverse array of chemicals. This characteristic extends their utility into various fields, including but not limited to extraction, catalysis, and electrochemistry.[Citation40]
Lastly, the polarity of DES assumes critical significance in comprehending their behavior across diverse applications. DES can be meticulously tailored to exhibit varying degrees of polarity, affording precise control over their solvent efficacy and selectivity.[Citation41] Consequently, they emerge as indispensable tools in the separation and purification of a multitude of compounds. Within the confines of this article, we embark on an in-depth exploration of these extraordinary attributes of deep eutectic solvents, unraveling their implications within the diverse tapestry of scientific and industrial contexts.
Combination of DES with UAE for extraction of (poly)phenols
The theoretical background of deep eutectic solvents-ultrasound assisted extraction (DES-UAE) lies in the principles of ultrasonic cavitation and the properties of DES. Ultrasonic cavitation is a process in which high-frequency sound waves create vapor-filled cavities in a liquid that collapse and generate high temperatures and pressures. This phenomenon has been shown to enhance mass transfer and increase the solubility of target compounds in the solvent, leading to improved extraction efficiency.[Citation42] The variety of DES used, the ultrasonic frequency, the extraction temperature and time, and the plant material are essential factors that can affect the efficiency of DES-UAE extraction.[Citation43] DES are an emerging green solvent class linked to ionic liquids (ILs).[Citation41]
DES with UAE (DES-UAE) is a method that combines the application of ultrasonic waves and DES to increase extraction efficiency and reduce extraction time.[Citation44,Citation45] In general, several DESs are novel biodegradable and environmentally friendly solvents made of ionic liquids and low molecular weight chemicals.[Citation46] DES are created through the powerful bonding of hydrogen bond acceptors (HBAs), such ChCl, quaternary ammonium salts, L-proline (Pro), and betaine (Bet), with hydrogen bond donors (HBDs) like sugars, polymers, metallic salts, and alcohols in the correct molar ratio. Other combinations of HBA:HBD can also be used to produce DES, allowing for the polarity of phases to be easily adjusted and enabling the formation of aqueous biphasic systems.[Citation47] The chemical structure of the most commonly used HBAs and HBDs in preparation for DES is depicted in . Using ultrasonic waves in DES-UAE generates high-frequency mechanical agitation, which improves extraction efficiency via increasing (poly)phenols solubility and mass transfer from plant material to solvent.[Citation48] The extraction efficiency is increased through the application of high-frequency mechanical agitation generated by ultrasonic waves in the DES-UAE process. This mechanical agitation leads to the disruption of plant cell walls and the formation of cavitation bubbles, which create shockwaves that promote the release of (poly)phenols from the plant material. Additionally, the agitation and cavitation bubbles lead to increased solubility of (poly)phenols in the solvent and improved mass transfer from the plant material to the solvent, resulting in increased extraction efficiency.[Citation48]
illustrates the schematic DES-UAE process. There are four stages to this mechanism’s description.[Citation49] First, cavitation bubbles are produced close to the surface of the plant matrix when ultrasound is applied. Second, a high pressure and high temperature microjet is released by crushing the bubbles and heading upwards. Third, the matrix surface is broken, allowing the cellular active ingredients to touch the solvent outside the cell. Lastly, the solvent receives the active ingredients that have been released. Consequently, mass transmission is improved using ultrasonic waves. Typically, a probe from an ultrasonic generator is submerged in the solid/solvent slurry.
Figure 2. Ultrasound-assisted extraction with deep eutectic solvents (DES-UAE) process and extraction mechanism of (poly)phenols.
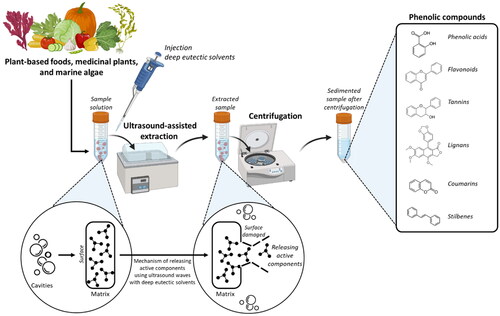
The application of ultrasound for the solid-liquid extraction of bioactive substances is quite common at research institutions, and to a lesser extent, in commercial settings (ultrasonic equipment for industrial UAE is commercially available). In general, the UAE can be seen as a quick, environmentally friendly, straightforward, adaptable, and efficient method. When compared to alternative forms of extraction, its primary benefits can be broken down into the following categories: first, an increase in the yield of material that can be extracted; second, a faster rate of extraction; third, the use of aqueous solutions as the extractant; fourth, greater use for thermolabile compounds; and fifth, fewer resources, both in terms of energy and the material to be extracted.[Citation31]
Furthermore, the factors affecting the DES-UAE process include time, temperature, power, intensity, duty cycle, solvent concentration, and others. The duration of the extraction process is crucial in determining the yield and quality of the extracted (poly)phenols.[Citation50] The temperature has also been shown to play a significant role in extraction efficiency, enhanced yield and the possibility of (poly)phenols breakdown at higher temperatures.[Citation51] Another critical factor in the extraction process is the ultrasonic reactor’s frequency, intensity, power, shape, and size, with various combinations leading to differing extraction efficiencies.[Citation52] Afterwards, the concentration of the DES used in the extraction process can also affect the yield and quality of the extracted (poly)phenols.[Citation53]
Effect of ultrasound factors on the extraction of (poly)phenols using DESs
To provide a better understanding of the UAE-associated factors affecting the extraction efficiency of (poly)phenols when using DESs in the context of UAE, this section describes the four main ultrasound factors that impact the extraction process: ultrasound frequency, power, intensity, and duty cycle. Ultrasound frequency refers to the number of cycles per second and is typically expressed in kilohertz (kHz). Higher frequencies are associated with smaller wavelengths and greater energy delivery, resulting in increased cavitation activity and a higher likelihood of bubble implosion. Ultrasound power, measured in watts (W), refers to the amount of energy delivered per unit of time and is determined by the voltage and current supplied to the transducer. Higher power levels result in more efficient cavitation and bubble collapse, leading to increased extraction efficiency. Ultrasound intensity, expressed in watts per square centimeter (W/cm2), is a measure of the energy density of the ultrasound wave at a given point in the solution. Higher intensities are associated with increased cavitation activity and a higher likelihood of bubble implosion, leading to greater extraction efficiency. Finally, the duty cycle refers to the percentage of time that the ultrasound wave is active during each cycle, with higher duty cycles leading to greater energy delivery and improved extraction efficiency. Understanding the impact of these factors is crucial for optimizing the (poly)phenol extraction process using DESs and UAE.
Ultrasound frequency
The ultrasonic frequency plays a significant role in (poly)phenols extraction using DESs. By optimizing the frequency of the ultrasound waves, the acoustic energy can be tuned to create micro-cavities within the solvent, facilitating the extraction process even in highly viscous solvents.[Citation54] The interaction between frequency and viscosity is believed to be a key factor in this process. For instance, at high frequencies, the material exhibited comparable elastic behavior, whereas, at low frequencies, it demonstrated a viscous behavior.[Citation55] However, in the case of higher boiling point DES solvents, the frequency of the ultrasound waves may need to be adjusted to avoid excessive heating and degradation of the solvent.
Ultrasound is a mechanical wave whose frequencies (more than 20 kHz) lie outside the audible range between 20 Hz and 20 kHz of human hearing. All matter types, including solids, liquids, and gases, are transparent to these waves. When these particles move across a medium, they displace and rearrange molecules. When a sound wave has a high intensity, the negative pressure that occurs during rarefaction becomes more significant than the attraction force that holds the molecules together. This causes the molecules to become separated, resulting in cavitation bubbles forming. These bubbles become larger through a process known as coalescence and then eventually burst during a phase known as compression, which results in the formation of hot spots and extreme local conditions. Temperatures of up to 5000°K and pressures of up to 1000 atmospheres are possible. The metabolic reactions that occur nearby can be sped up by these hot spots.[Citation30,Citation56–58]
During the extraction process, it is helpful to use ultrasound that has a low frequency but a high intensity since this produces a substantial amount of shear and mechanical force, both of which are advantageous. The application of low frequencies ranging from 18 to 40 kHz can improve cell wall disruption and enhance solvent access to cell content, resulting in an increased mass transfer rate. Additionally, the investigation of ultrasound’s impact on the extraction of total phenolics and antioxidant activity demonstrated that the positive effects of acoustic assistance were reduced at higher frequencies (20, 490, and 986 kHz), regardless of the power input.[Citation59] In contrast, ultrasound with a high frequency and a low power density produces many reactive radicals. The majority of research has been done on extracting substances at a constant frequency. Hence, not many studies investigate how changing the frequency of the extraction process affects the yield or quality of the substance.[Citation26] The decision to use a continuous low frequency may have been made because it encourages the creation of fewer cavitation bubbles with greater diameters, which is beneficial to the considerable cavitation effect. This impact is diminished when the ultrasound frequency is raised.[Citation60] This is because the large cavitation effect favors the formation of fewer cavitation bubbles. Cavitation bubbles grow with a minimum compression-rarefaction cycle. The cavitation bubble will not form if the cycle is too short. Ultrasonic waves and rarefaction phase length are inversely proportional. As a result, when the frequency of the ultrasonic waves is high, the cavitation bubble has a very limited amount of time to expand, which reduces the effectiveness of the implosion effect. Because of the huge number of bubbles that are produced when a high frequency is used, there is increased resistance to the transfer of mass.[Citation61]
The effects of multi-frequency ultrasound and DES on the extraction of (poly)phenols from Dendrobium officinale leaves have been investigated.[Citation15] The study examined the effects of different ultrasonic frequency modes, including single frequency (40, 60, and 80 kHz), dual-frequency (40/60, 40/80, and 60/80 kHz), and triple frequency (40/60/80 kHz) on DES extraction. The authors found that ultrasound with a dual-frequency mode of 40/60 kHz was the most effective at increasing the amount of total (poly)phenols. They also observed that single-frequency ultrasound treatments led to an uneven distribution of the acoustic field, which reduced the amount of total (poly)phenols extracted. The lowest amount of total (poly)phenols was obtained with triple-frequency ultrasound of 40/60/80 kHz, possibly due to the resonance field generated in such a mode. The study identified 13 major (poly)phenols in D. officinale leaf extracts, including rutin, vicenin II, and apigenin flavonoids. The study demonstrated that the use of DES combined with the synergistic effect of ultrasound significantly increased the extraction efficiency of (poly)phenols from D. officinale leaves.[Citation15]
Ultrasound power
The viscosity of DES is significantly higher than that of conventional solvents, and this can have a significant impact on the power and power intensity required for ultrasonic treatment. Higher viscosity results in more energy being required to generate cavitation, which is essential for the efficiency of ultrasonic treatment. Additionally, the higher viscosity of DES could affect the behavior of the cavitation bubbles, which could lead to different mechanisms than those observed in conventional solvents.[Citation30,Citation49,Citation62] Ultrasound can cause fragmentation of particles through inter-particle collisions and shockwaves generated by collapsing cavitation bubbles in the liquid. One of the direct outcomes of this particle size reduction is the increase in solid surface area, leading to greater mass transfer and higher extraction yield and rate.[Citation30]
Amplitude percentage (0–100%) and power density (W/mL) have been reported for UAE power.[Citation63] When the ultrasonic amplitude is between 30% and 80% of the maximum power, the relationship between the amplitude and the output power is linear.[Citation64] UAE of (poly)phenols from plants (fruit and vegetable) by-products uses 20–700 W, depending on the chemical and plant matrix.[Citation26,Citation65]
Ultrasonic power and density are considered major factors affecting the performance of UAE. Increased power causes violent cavitation bubbles to collapse more violently. The ultrasonic wave’s power determines the resonant bubble size, which affects implosion. Fragmentation, pore formation, and tissue mixing increase diffusivity and extraction yield.[Citation66] The ultrasonic probe’s mechanical vibration enhances the solid-solvent contact area, enhancing solvent penetration, and yield. Hydrodynamic force disrupts tissues as power increases.[Citation65] As the power increases, the formation of bubbles also increases. However, as the bubble volume becomes larger, the impact of cavitation decreases. The quantity of bubbles promotes non-spherical bubble collision, distortion, and collapse, lowering bubble implosion. The probe tip’s cavitation bubble layer reduces energy transfer into the extraction liquid (saturation effect) and yield. Due to excessive ultrasonic intensity, the bioactive component may degrade.[Citation26]
Several studies have explored the effects of ultrasound power on the extraction of (poly)phenols using DESs, intending to optimize the extraction process as described in . The DES-UAE extraction method was found to be more effective, and timesaving compared to traditional methods like maceration and percolation. The DES-UAE method with ultrasonic power (160, 200, 240, 280, and 320 W) resulted in the extraction of 23 flavonoids, including six components that were identified for the first time in Selaginella uncinata. The extracts also showed potential antioxidant activity based on the results of three antioxidant experiments.[Citation50] A study considering the extraction of (poly)phenols from foxtail millet bran found that optimal extraction conditions are a power input of 200–400 W and a Bet:glycerol (Gly) (1:2) solvent. In these settings, the (poly)phenols extraction yield was much greater than that of maceration or solvent extraction. The study also investigated the bioactivity of the extracted (poly)phenols, finding that they had vigorous antioxidant activities.[Citation16]
Another study investigated the extraction of (poly)phenols from Moringa oleifera leaves. The study found that the optimal extraction conditions included a power input of 80 and 240 W and an Pro:Gly (2:5) sample-to-solvent ratio. The study found that the extracted (poly)phenols had high antioxidant activity. The UAE method was more effective than other methods, such as maceration and stirring-assisted extraction.[Citation17]
Ultrasound intensity
The ultrasonic part of the spectrum is significant in terms of its application and importance to capital cost. Low-intensity sonication (less than one W/cm2) and high-intensity sonication (10–1000 W/cm2) are the two broadest categories. The latter is used for extraction and processing. Ultrasound’s frequency, intensity, medium qualities, and surroundings affect its ability to cause cavitation. The cavitation effect’s high-shear energy is used to modify liquid particles, liberate cellular components and molecular structures, and de-aerate liquids and surfaces. In extraction applications, solvent properties affect cavitation bubble formation and collapse. The fluid’s surface tension and viscosity influence the cavitation’s transitory threshold, whereas the vapor pressure determines collapse severity. Chemically, solvent reactivity determines primary and secondary sonochemical reactions.[Citation67]
According to a recent study that investigated the impact of varying acoustic intensities on the extraction of total phenolic content, it was reported that the percentage increase in extraction of total phenolic content increased by 64%, with increasing acoustic intensity from 20 to 83 W/cm2.[Citation18] The strengthening of the cavitation effect, affecting the extraction efficiency, is a result of the amplification of sonic waves as the power intensity is increased. Additionally, the extract was used to quantify (poly)phenols, with quercetin being the most abundant.
Generally, studies have shown that the intensity of ultrasound has varying effects on the extraction of (poly)phenols from plant sources, depending on several variables such as the specific plant material being used, the solvent system being employed, and the operating conditions of the UAE as shown in .
Ultrasound duty cycle
The duty cycle (DC) of an ultrasonic wave is the fraction of the whole cycle period occupied by the pulses.[Citation26] The term “cycle time” refers to the overall amount of time, which includes both the pulse duration and the pulse interval. In their investigations on pomegranate peel and grapefruit peel, respectively, utilizing pulsed UAE (PUAE). According to Pan et al., there was not much of a difference between PUAE and continuous UAE in terms of the yield and the amount of time needed for extraction; however, PUAE conserved around 50% more electrical energy.[Citation68] The pectin output from grapefruit peel initially increased with a rise in DC, but this increase was followed by a decline when the peak value of 50% was reached. When the DC was low, 20 s of sonication per minute was not enough to break down tissue and eliminate solutes. As was covered in the section on the impact of power, the saturation and inter-bubble collision that occurred at high DC (50–70%) caused the cavitation effect to diminish. The strength of bubble implosion is increased when using pulsed ultrasonic operation, but the quantity of cavitation bubbles produced is reduced. It was determined that a longer sonication duration eliminated saturation and increased the likelihood of an inter-bubble collision, which led to an increase in yield at 80% DC (48 s).[Citation64] recapitulated the effect of ultrasound DC on the extraction of (poly)phenols using DES.
It has been reported that the extraction yield of (poly)phenols from apple pomace, using ChCl:Gly (1:2), was significantly affected by DC.[Citation18] The short sonication duration per minute at low DCs was insufficient to fragment tissue, resulting in low extraction yields. Saturation and inter-bubble collision reduced cavitation when DC was increased to 50–70%. The saturation and inter-bubble collision effects were reduced by increasing the sonication period at high DCs, increasing extraction yield. The optimum DC was determined at 75%, with the total phenolic amount of 5.6 mg/g DW.[Citation18]
Similarly, the consequence of ultrasound DC on the extraction of (poly)phenols from waste mango peels using lactic acid: glucose (5:1). The ultrasound DCs were set at 20%, 40%, 60%, 60%, and 100%, with total (poly)phenols amounts were 48 mg/g dry weight, 58 mg/g dry weight, 62 mg/g dry weight, 56 mg/g dry weight, and 39 mg/g dry weight, respectively. They found that the DC significantly impacted the extraction yield and that a longer DC resulted in higher extraction efficiency. The optimum ultrasound DC was 50%, with a total phenolic amount was 70 mg/g DW.[Citation19]
The extraction of glycyrrhizic acid from Glycyrrhiza glabra was optimized using ChCl:lactic acid (1:1). Findings showed that the ultrasonic DC 50% significantly affected the extraction yield, with the ideal DC resulting in the highest extraction efficiency (55 mg/g DW).[Citation21] In a study by Patil et al., the impact of ultrasound DC on the extraction of curcuminoids from Curcuma longa was investigated.[Citation20] The authors optimized the extraction process by varying the DC (20%, 40%, 60%, 80%, and 100%), sonication time, and temperature. Extraction yields of 77 mg/g DW of curcuminoids were found to be optimal at a DC of 60%. The authors also performed a kinetic study and found that the extraction rate increased with increasing DC up to 50%, after which it remained constant.
In addition, inconsistent results have been found when testing the hypothesis that a longer DC increases extraction efficiency. A higher pulse duration/pulse interval ratio (from 30% to 50%) was associated with a higher yield of pectin extraction from grapefruit peel, and this relationship was shown to be inverse after 50%. An increase followed this in the ratio after it was above 50%.[Citation69] The extraction efficiency of (poly)phenols from pomegranate peel was significantly affected by interactions between ultrasonic power and DC. With a DC of 80%, the authors obtained the highest possible level of extraction efficiency.[Citation70] This suggests that extraction efficiency could be improved by using the DC appropriately, which would benefit the process.[Citation71]
Effect of DES composition on UAE extraction efficiency of (poly)phenols
DESs are comprised of two components: (i) HBA and (ii) HBD and these two are interlinked with each other by hydrogen bonding. Different solvents in different proportions are used to prepare eutectic solvents depending on the polarity, solubility, and desired extraction application. DES-UAE application uses acoustic cavitation to rupture the cell wall matrix and release bioactive compounds, improving extraction efficiency and reducing solvent costs.
Among many other organic compounds due to its low cost, biodegradability, and low toxicity, ChCl was widely used as an organic salt to prepare eutectic solvent mixtures with cheap and safe HBDs such as Ur, Gly, carbohydrate-derived polyols or carboxylic acids. recapitulates the effect of deep eutectic solvent composition on the UAE efficiency of (poly)phenols.
Table 2. Effect of deep eutectic solvent composition on UAE extraction efficiency of (poly)phenols.
Nine different eutectic solvent compositions along with UAE methods were used by Zheng et al. to extract phenolics from foxtail millet bran.[Citation16] The composition of the six eutectic solvents are as follows: ChCl-Oa (Oa = oxalic acid), ChCl-La (La = lactic acid), ChCl-Gly, Pro-Gly, Pro-La (La = lactic acid), Gly-La, Bet-Gly, Gly-Ca (Ca = citric acid) and Sa-Gly (Sa = sodium acetate). By using the nine eutectic solvents they obtained the total phenolics content amount in the following order: Gly-Ca (7.5 mg FAE/g DW) > Bet-Gly (7 mg FAE/g DW) > Gly-La (6.5 mg FAE/g DW) > Sa-Gly (6.25 mg FAE/g DW)> Pro-Gly (4.6 mg FAE/g DW) > Pro-La (1.1 mg FAE/g DW) > ChCl-Gly (0.38 mg FAE/g DW) > ChCl-Oa (0.22 mg FAE/g DW) > ChCl-La (0.13 mg FAE/g DW). This study found that three key factors are intermiscibility, compatibility, polarity, and viscosity of eutectic solvents, which are essential for optimal efficiency. Higher viscosity limits the extraction efficiency of maximum DESs and that is why maximum extraction efficiency can only be achieved through low viscous DES which is Gly-Ca and Bet-Gly here. It has been observed that Bet-Gly possessed slightly higher viscosity due to the presence of a carboxylic group with Bet compared to Gly-Ca. Though viscosity is important for extraction performance, other characteristics such as polarity also play a critical role. Solvents with polarity values near to those of the targeted solutes provide higher solubilization capacity and higher extraction efficiency. Another point is DES composition has been observed to affect the polarity of DES, with organic acid-based DES being the most polar. Bet-Gly is considered an optimum solvent for extraction due to its higher polarity than Gly-Ca.
In another study, three different eutectic solvent compositions were used for the extraction of (poly)phenols from different agricultural wastes.[Citation72] The three eutectic solvent compositions are – Gly-ChCl, Gly-Sa, Gly-Spt-W (Spt = sodium – potassium tartrate, W = Water). In the case of lemon peels, maximum (poly)phenol content was obtained from Gly-ChCl followed by Gly-Spt-W and Gly-Sa, for onion solid wastes maximum (poly)phenol content was obtained from Gly-Spt-W followed by Gly-ChCl and Gly-Sa, for red grape pomace, wheat bran, olive leaves, and spent filter coffee maximum phenolics were obtained from Gly-ChCl followed by Gly-Sa and Gly-Spt-W. In conclusion, it was found that mixtures composed of Gly-ChCl and Gly-Sa show higher extraction efficiency compared with a lower extraction efficiency of Gly-Spt-W and the authors suggested polarity as a key factor of an extraction efficiency of different eutectic solvents.
UAE along with three different eutectic solvent compositions – ChCl-La, ChCl-Ta (Ta = Tartaric acid), ChCl-Aa (Aa = acetic acid) to extract (poly)phenols from kinkeliba (Combretum micranthum G. Don).[Citation73] The best result was obtained by using ChCl-La (23.5 mg GAE/g DW) followed by ChCl-Aa (19 mg GAE/g DW) and ChCl-Ta (17 mg GAE/g DW). To extract (poly)phenols from Lavandula angustifolia flowers, a study by Alasalvar et al. used UAE methods in combination with 18 different eutectic solvent compositions.[Citation74] Among these 18 eutectic solvent compositions, ChCl-Gly was found to have the highest (poly)phenol recovery (44 mg GAE/g DW) and that due to its high polarity.
Twelve different eutectic solvents compositions were used to extract (poly)phenols from sea buckthorn leaves and in all these 12 eutectic solvents ChCl is used as a hydrogen bond acceptor along with 12 different compounds (Ca, La, Ma (malic acid), Etgly (Ethylene glycol), 1,3-but (1,3-butanediol), 1,4-but (1,4-butanediol), 1,6-hex (1,6-hexanediol), 1,2-prop (1,2-propanediol), Gly, Glu (glucose), Fru (fructose) and Su (sucrose)) as HBD.[Citation75] Among these 12 different eutectic solvents, ChCl − 1,4-but exhibited the greatest (poly)phenol extraction ability due to its minimum viscosity that enabled it to maximum penetration of pores in the matrix.
Ten different eutectic solvent compositions were used to extract (poly)phenols from Capparis Ovata var canescens fruit.[Citation76] Among them, four were prepared using ChCl as HBA along with Ur, Gly, La, and Sor (sorbitol) as HBD, three were prepared using Sa as HBA and three were prepared using Sc (sodium citrate) as HBA along with Gly, La, Sor as HBD. Maximum extraction efficiency was observed in ChCl-La eutectic solvent composition due to its higher polarity and solubility factor. In a study by Liu et al., 16 different combinations of deep eutectic solvents were investigated to optimize the (poly)phenols extraction from Paederia scandens (Lour.) Merr.[Citation77] Among the 16 different combination of deep eutectic solvents – eight were prepared using ChCl as HBA along with Ma, Gly, Oa, Xl (xylitol), Lva (levulinic acid), Etgly, Glu, Tgl (triglycol) as HBD, five were prepared by using Pro as HBA along with Gly (with two different ratios), Lva, Etgly, La as HBD, another two were prepared by using Bet as HBD along with Gly, Lva as HBA, last one prepared by using Ca as HBD along with Gly as HBA. ChCl-Etgly combination was found as the optimum deep eutectic solvent having a maximum extraction efficiency of 20 mg CE/g DW. Polarity is a key factor in the comparative study of extraction efficiency of different DES combinations. Optimized combinations of HBA and HBD are selected based on their hydrogen bonding interaction between target chemicals and DES molecules.
To optimize (poly)phenols extraction from waste mango peels, a study by Lanjekar et al. investigated the use of different DES.[Citation78] In this study eight paired of DES were used among which six were prepared by using ChCl as HBA along with Etgly, Gly, Glu, Su, Ma, La as HBD and two were prepared by using lactic acid as HBA along with Sa (salicylic acid) and Glu as HBD. Study shown that La-Glu paired was to have maximum extraction efficiency as it is was capable of extracting polar as well as less polar compounds from the waste mango peel. To extract (poly)phenols from apple pomace seven different combinations of DES were used.[Citation18] Among seven different DES paired, five were made by using ChCl as HBA along with La, Glu, Ur, Ca, and Gly, another two were prepared by using Ur and Ta as HBA along with Gly and Glu as HBD respectively. Among all these pairs, ChCl-Gly was found to have maximum (poly)phenols extraction efficiency due to its solubility phenomena. To optimize (poly)phenols extraction from date palm seven different sets of DES used and all DES were prepared by using ChCl as HBA along with Glu, Su, Xl, Xls (xylose), La, Ma, 1,4-but as HBD.[Citation79] Best result was observed in case of ChCl-La which supported the result of previous study.
To extract (poly)phenols from bayberry, the use of UAE-assisted deep eutectic supramolecular polymers (DESP) has been investigated.[Citation80] In this study, three different combinations of DESPs were used in which β-cyn (β-cyclodextrin) was used as HBA along with three different organic acids such as La, Ma, and Ca as HBD. The best extraction result was observed in the case of β-cyn-La combination where β-cyn-Ma and β-cyn-Ca showed similar results. To extract (poly)phenols from sumac (Rhus coriaria L.) DES-UAE was used for the first time.[Citation73] In this study, three different combinations of DES with a molar ratio of 1:2 were used where ChCl used as HBA along with La, Ta, and Aa as HBD. The recovery of (poly)phenols was highest with ChCl-La. To extract (poly)phenols from Cosmos sulphureus seven DES were used that were based on ChCl as HBA and Glu, Su, Gly, Etgly, La, Ma, and Ur as HBD.[Citation82] Similarly, the greatest recovery was obtained by using ChCl-La combination which is significantly higher than other DESs. To extract (poly)phenols from Pistacia lentiscus seven different sets of DESs were used where several compounds such as ChCl, Gly, Fr, La, Aa and Ur were used as HBD and HBA.[Citation83] Maximum extraction amount was observed in the case of ChCl-Aa combinations which is significantly much higher than all other DESs combinations. Three uncommon combinations of DES were used to extract (poly)phenols from maritime pine residues.[Citation84] Three DES combinations that were used in this study were Lev-Fa (formic acids = Fa), Lev-ChCl, and La-ChCl. Among all these three Lev-Fa showed a significantly much higher extraction amount of (poly)phenols compared to the other two combinations.
From all the studies reported here, it has been observed that ChCl is the most commonly used HBA in DES systems, while different organic acids (in most cases La) are used as HBD. This is because ChCl-based DES performs exceptionally well for (poly)phenolic extraction. These factors have made it the most commonly used HBA in DES systems.
ChCl is abundant and low-cost, making it attractive for industrial applications.
ChCl has low toxicity which makes it a safer choice compared to some other ionic liquids.
ChCl has high thermal and chemical stability, which makes it suitable for use in a wide range of applications.
ChCl acts as a hydrogen bond donor in DESs, helping to stabilize the solvent in high-temperature reactions or harsh environments.
Besides all these advantages, there are some disadvantages also to use ChCl as HBA.
ChCl’s limited solubility in common solvents hinders its effectiveness as an HBA in certain systems.
ChCl is relatively expensive compared to alternative HBAs, restricting its widespread use.
ChCl’s hygroscopic nature makes it sensitive to moisture, potentially interfering with desired hydrogen bonding interactions.
Effect of DES to sample mass ratio on UAE extraction efficiency of (poly)phenols
The DES-UAE method is based on the choice of eutectic solvent composition and the ratio of that eutectic solvent to sample (generally plant cell) mass. The main mechanism for solvent extraction is the penetration of solvent to the cell mass through the cell wall matrix and making the (poly)phenols and other bioactive compounds soluble in that solvent depending on the solubility and polarity of the solvent.[Citation85] The amount of solvent needed to solubilize the maximum (poly)phenols in a solvent depends on the amount of sample. In general, the higher the sample amount, the higher the solvent amount is considered. However, in some cases, for small amount sample, the higher solvent is needed due to hard cell matrix, low diffusivity rate and low solubility.[Citation86] The concentration gradient increases with the solvent-to-sample mass ratio, resulting in higher extraction of solids by the solvent.[Citation14] A high solvent-to-sample mass ratio dilutes the concentration of dissolved (poly)phenols at the surface layer of the cell wall/matrix, accelerating the extraction rate.[Citation87] The solvent-to-sample mass ratio must be low to ensure optimal extraction efficiency and solvent recovery and reusability for a sustainable extraction process. The effect of deep eutectic solvent to sample mass ratio on UAE extraction efficiency of (poly)phenols was summarized in . Solvent (liquid) to sample (solid) ratio were optimized in the DES-UAE of (poly)phenols from green coffee beans of Coffea canephora.[Citation88] In the study, they used three liquid-to-solid ratios, 10:1, 20:1, and 30:1 along with other variables (solvent composition, solvent molar ratio, and extraction time) for optimization purposes by using RSM-Box–Behnken design. From different parameters design combinations, it was found that the highest amount of (poly)phenols was obtained from the 30:1 ratio of liquid to solid followed by 20:1 and 10:1. In this study, the higher the solvent to solid ratio, the higher the (poly)phenols extraction phenomena was observed.
Table 3. Effect of deep eutectic solvent to sample mass ratio on UAE extraction efficiency of (poly)phenols.
To optimize the extraction of (poly)phenols from foxtail millet bran, five different solid-to-liquid ratios were used, 1:5, 1:10, 1:15, 1:20, and 1:25.[Citation19] Here in this study, interesting results were observed that a maximum of 7.6 mg FAE/g (poly)phenol content was observed in cases of 1:10,1:15 and 1:25 and in case of 1:20 the amount was 7.5 mg FAE/g DW followed by 6.8 mg FAE/g DW in 1:5 solid to liquid ratio. The initial increase in the yield observed upon increasing the ratio from 1:5 to 1:10 can be associated with the increase in the contact surface between the matrix and the solvent, as well as an improved concentration gradient that encourages greater diffusion of (poly)phenols from the intracellular sample matrix into the solvent. However, further increase in the volume of solvent showed no enhancing effect, this suggests that it is not always necessary to use high volumes of solvents, especially with DES. Using an excessive amount of extraction solvents could potentially hinder the retrieval of (poly)phenols and lead to unnecessary wastage of reagents.
To get optimum extraction of (poly)phenols from Capparis Ovata var canescens fruit five different liquid to solid ratio were used, 10:1, 20:1, 30:1, 40:1, and 50:1.[Citation76] Here initially the amount of the (poly)phenols was increased with an increase in liquid-to-solid ratio from 10:1 to 30:1 and after the 30:1 ratio, as the ratio increased up to the highest liquid-to-solid ratio of 50:1 the amount of the phenolic remained constant at 24.4 mg GAE/g DW. Though we knew that a higher liquid-to-solid ratio enhances the mass transfer rate, here in this case at a 30:1 liquid-to-solid ratio the extraction reached its equilibrium/saturation point, thus the phenolic amount remained constant. This phenomenon also reduces the solvent’s costs and makes the extraction economically more feasible. Five different (poly)phenols from Morus alba L. leaves were extracted and quantified by using five different solid-to-liquid ratios of 1:10 (10 mg/mL), 1:4 (25 mg/mL), 1:2 (50 mg/mL), 3:4 (75 mg/mL), 1:1 (100 mg/mL).[Citation89] Another interesting phenomenon they observed was that all the (poly)phenols except quercetin and kaempferol showed better extraction efficiency at a solid/liquid ratio of 1:2, and only catechinic acid showed maximum extraction efficiency at a solid/liquid ratio of 1:4. As the (poly)phenol extraction efficiency decreased after the solid/liquid ratio of 1:2, thus the solid/liquid ratio of 1:2 was considered the optimum ratio.
A study by Wu et al. investigated the effect of six different liquid-to-solid ratios (20:1 (20 mL/g), 40:1 (40 mL/g), 60:1 (60 mL/g), 80:1 (80 mL/g), 100:1 (100 mL/g) and 120:1 (120 mL/g)) on the extraction of (poly)phenols from Polygonum aviculare.[Citation90] Here the extracted (poly)phenols increased with increases in liquid-to-solid ratio from 20:1 to 80:1 and reached a maximum of 83 mg GAE/g DW at 100:1 and remained constant at 120:1. The higher the liquid-to-solid ratio, the lower the viscosity, thus the higher extraction efficiency was observed here. By using five different liquid (eutectic solvent) to solid ratios: 22.4:1, 25.5:1, 30:1, 34.5:1 and 37.6:1, were used extracted (poly)phenols from L. angustifolia flowers.[Citation74] Maximum extraction was obtained at 30:1 and beyond that, at 34.5:1 and 37.6:1 the extraction amount was decreased.
In another study, Liu et al., reported that 40 mL/g of DES to solid sample ratio extracted more (poly)phenols in comparison to 10 mL/g, this can indicate that higher DES to sample mass ratio can result in higher extraction yields due to increased solubility of the target compounds in the solvent.[Citation77] To study the extraction frequency of (poly)phenols four different sample-to-DES ratios were used.[Citation19] Among the four solid-to-liquid ratios of 1:10, 1:20, 1:30 and 1:40, it was observed that extraction efficiency was increased from 1:10 to 1:30 ratio due to a higher mass transfer rate created from the difference of concentration gradient. The highest recovery of (poly)phenols was observed at 1:30 ratio and as the ratio further increased to 1:40, the extraction efficiency decreased due to the uneven distribution of the cavitation effect resulting from over dilution effect. To optimize the (poly)phenols extraction from apple pomace was done by using five different solid-to-liquid ratios ranging from 1:10 to 1:50.[Citation18] A similar trend was also observed resulting in maximum extraction at 1:30 solid-to-liquid ratio, further in which it was shown that the (poly)phenols were decreased.
A recent study by Shi et al., combined deep eutectic supramolecular polymers (DESP), instead of DES, with UAE to extract (poly)phenols from bayberry.[Citation80] In this study, five different solid-to-liquid ratios were used 1:75, 1:10, 1:125, 1:50, and 1:175 where the best result was obtained in the 1:75 solid-to-liquid ratio. As the ratio increased further, the (poly)phenols recovery significantly decreased which might be due to lesser cavitation or uneven distribution of cavitation resulting from a higher dilution effect. By using ChCl in five different solid-to-liquid ratios ranging from 10 to 30 mg/L, (poly)phenols were extracted from Cosmos sulphureus where the best extraction was obtained at 20 mg/L liquid-to-solid ratio, beyond which the extraction amount remained more or less constant due to equilibrium factor.[Citation82]
Effect of sonication time on the extraction of (poly)phenols using DES
Sonication time has a crucial role in the extraction process. Long-term sonication time is detrimental to the quality of extracts, as it alters the chemical structure and degrades bioactive compounds, leading to poor recovery.[Citation91] Also, the long sonication time makes the eutectic solvents unstable which hampers their extraction efficiency.[Citation76] Thus, UAE by using eutectic solvent helps to get better optimum extraction results in comparatively low sonication time.
A 4-factor-3-level Box–Behnken design (BBD) based response surface methodology (RSM) was used to investigate single-factor experimental results in the (poly)phenols extraction from Setaria italica (foxtail millet bran) and optimized four independent process variables which were – water content in the DESs, the ultrasonic power used, extraction temperature and extraction time.[Citation16] For finding out the effect of sonication time on the extract amount different sets of sonication times (10–50 minutes) were used. As shown in , from 10 to 30 minutes the (poly)phenol content significantly increases (p < 0.001) with the increase in sonication time. The phenomenon occurred due to the better release of intracellular (poly)phenols through ultrasound and the lower diffusion resistance from the matrix cell wall. Short to medium sonication time (10–30 minutes) exposure also helped stabilize eutectic solvents, resulting in better extraction efficiency. With a further increase in sonication time to 40 minutes, the amount of the (poly)phenols significantly decreased (p < 0.001) due to the maximum solubility of (poly)phenols in the eutectic solvent which caused higher diffusion resistance and with a further increase in sonication time to 50 minutes, more decrease in phenolics was observed due to the higher temperature generation from long sonication time.
Table 4. Ultrasonication-assisted extraction of (poly)phenols by using different eutectic solvents.
A BBD experimental design united with RSM was employed to optimize the percentage of water, the ultrasound amplitude, and the extraction time on five response variables in the extraction of bound (poly)phenols from Garcinia mangostana L. (mangosteen peel).[Citation92] Here three different sonication times (1, 8, and 15 minutes) were used for the optimization of extraction. Analysis of variance (ANOVA) was employed to assess the appropriateness of the fitted model based on water %, ultrasound amplitude, and extraction time. As shown in , 15 minutes of sonication resulted in the highest amount of (poly)phenols which is significantly (p < 0.001) much higher than the other two results obtained at 1 and 8 minutes. Prolonged ultrasonication time (15 minutes) improved the bioavailability and efficacy of compounds by forming smaller particles and uniform distribution. In another study (poly)phenols extraction from Lycium barbarum L. was optimized by using six different sonication times (ranging from 10 to 120 minutes) and the optimized result was observed at 90 minutes. Beyond 90 minutes the result became constant at 120 minutes (). The greatest extraction at 90 minutes is due to the stabilization of eutectic solvent and changes in the crystalline structure of the compound matrix, leading to increased solubility and physical properties. To statistically optimize the ultrasound-assisted DESs for (poly)phenols extraction from Fagopyrum esculentum (Buckwheat sprouts), a central composite design (CCD) with two components and three levels united with RSM was used.[Citation91] Among three sets of sonication time (20, 40, and 60 minutes) the best results were obtained at 40 minutes and beyond 40 minutes the amount was significantly decreased (p < 0.05) at 60 minutes due to prolonged extraction time which may increase the temperature resulting in poor extraction efficiency. BBD united with RSM was applied to extract (poly)phenols from Hippophae rhamnoides (Sea buckthorn leaves) and sonication/extraction time (10, 15, and 20 minutes) was one of the key independent variables.[Citation75] The highest yield was obtained at 15 minutes sonication time and this short sonication time also ensures optimum quality extracts with low energy cost the DES-UAE combination also ensured no detrimental effects on the environment. Maximum (poly)phenols from Capparis Ovata var canescens fruit was reported at a sonication time of 30 minutes over five experimental times (10–50 minutes) and they recommended DES-UAE methods as an alternative to conventional extraction methods due to shorter extraction time and cost-effectiveness.[Citation76]
The DES-UAE method was used to extract (poly)phenols from peaches and pumpkins. For both peaches and pumpkins the highest amount of (poly)phenols was observed at 20 minutes sonication time, and process optimization revealed that 25.7 and 27.9 minutes were the optimal sonication times for both peaches and pumpkins [Citation97] (). In another study by employing the DES-UAE method the highest recovery of (poly)phenols was observed at 30 minutes sonication time compared to 15 and 60 minutes sonication time.[Citation98] The DES-UAE method was used to extract (poly)phenols from Juglans regia L., and the optimum result was achieved at 105 minutes.[Citation95] Higher sonication time is needed for complex cellular structures and low extraction rates. In the DES-UAE method, the highest (poly)phenols content from Morus alba L. (White mulberry) was obtained at a sonication time of 30 minutes..[Citation16,Citation89] Beyond 30 minutes, extract amounts were decreased at 40 and 50 minutes. The reason for this phenomenon is that at a long sonication time, (poly)phenols may form polymer by reacting with eutectic solvents, making them unavailable as extracts. Also, excessive ultrasonication time caused the formation of insoluble aggregates or decomposition products, resulting in poor extraction efficiency.
In the extraction of (poly)phenols from Paederia scandens (Lour.) sonication time was optimized by using response surface methodology.[Citation77] 10 minutes and 40 minutes of sonication time were used to extract (poly)phenols, where 10 minutes resulted 24.9 mg CE/g DW and 40 minutes resulted 20.09 mg CE/g DW. The optimal time was 9.7 minutes. Longer sonication time can lead to higher extraction yield, but can also increase the risk of thermal or chemical degradation. In the extraction of (poly)phenols from apple pomace by using DES-UAE technique nine time interval ranging from 10 to 50 minutes with gap of 5 minutes in each were studied.[Citation18] In this study an interesting trend was observed where (poly)phenols amount increased as the time increased from 10 to 25 minutes then decreased from 25 to 35 minutes, then slightly increased to 40 minutes and then it remained constant till 50 minutes. This indicates Fick’s law of diffusion behavior where the (poly)phenols amount increased due to higher rate of diffusion, but when it reaches equilibrium, it remains constant. Unlike all the previous study, in this study deep eutectic supramolecular polymers along with UAE were used to extract (poly)phenols from bayberry where eight different time segments ranging from 5 to 40 minutes having an interval of 5 minutes each were used.[Citation80] Results showed that as the time increased, the amount of (poly)phenols increased significantly, but decreased after 35 and 40 minutes due to degradation or denaturation of the target compounds. Prolonged sonication time also caused polymorph conversion, resulting in lower extraction efficiency. Seven different DES were used to extract (poly)phenols from Cosmos sulphureus where five time segments ranging from 10 to 50 minutes with 10 minutes gap each were used and best result was obtained at 30 minutes extraction time, after that extraction amount slightly decreased or remained as more or less constant.[Citation82] Though three different time segments 15, 30, and 45 minutes were used to optimize the (poly)phenols extraction from bee pollen by using DES, it was seen that interestingly three different time segments had more or less same extraction efficiency.[Citation96]
Effect of temperature on extraction of (poly)phenols using DES-UAE
Extraction temperature plays a critical role in DES-UAE. The temperature profile influences both the UAE extraction efficiency and the eutectic solvent’s extraction capacity. The DES-UAE mechanism works based on cavitation collapse on the cell wall/matrix, releasing compounds into the extraction solvent.[Citation99] UAE is a favorable extraction method due to its lower extraction temperature, which protects sensitive (Poly)phenols and increases efficiency. The optimum temperature zone is between 30 and 60 °C, with extraction efficiency increasing as the temperature increases.[Citation90] In combination with UAE nowadays eutectic solvents are used to increase extraction efficiency by decreasing viscosity and increasing solubility. This can be achieved by adding solvent or increasing the temperature, which decreases viscosity and increases solubility.[Citation43] Therefore, it has been observed that eutectic solvent combined with UAE extraction methods operating under optimum temperature zone resulted in excellent extraction efficiency. conveys the effect of temperature on the extraction of (poly)phenols using deep eutectic solvents and UAE method.
Table 5. Effect of temperature on yield of (poly)phenols content using DES-UAE method.
(Poly)phenol yield was increased with the increase in temperature up to a maximum of 60 °C that caused higher solubility of (poly)phenols in the eutectic solvent and beyond 60 °C yield was decreasing slightly due to lower thermal stability.[Citation16] Similarly, highest (poly)phenol recovery from L. angustifolia was found at 55 °C.[Citation74] Highest (poly)phenol extraction from Hippophae rhamnoides was observed at 60 °C over a temperature range of 45 to 75 °C and it was also found that increased temperature decreases surface tension and viscosity of eutectic solvent, which increases solubility and penetration power of the eutectic solvent to the cell-matrix.[Citation93]
In another study, maximum (poly)phenol extraction from Capparis Ovata var canescens fruit using DES-UAE was found at 60 °C due to better cavitation, optimum viscosity and lower oxidation.[Citation76] Maximum extraction of (poly)phenols from grape skin observed at 65 °C by employing UAE-eutectic solvent and they found that the increase in temperature caused a decrease in surface tension, resulting in enhanced desorption and dissolution of target (poly)phenols in the solvent.[Citation100] (Poly)phenols were extracted from pumpkins and peaches and in both cases, the optimum temperature was found as 40 °C at which maximum extraction efficiency observed due to increase in diffusivity of the solvent into cells and that enhanced desorption and solubility of target compounds from the cells.[Citation94] In a study maximum (poly)phenol extraction was observed at 50 °C and beyond 50 °C hampered the stability of the extraction system and that resulted in a decrease in extraction amount. As per this study at lower temperatures high viscosity of eutectic solvents caused a low mass-transfer rate that resulted in poor extraction efficiency.[Citation89] Highest (poly)phenol recovery at a temperature of 60 °C was observed due to maximum diffusion of plant metabolites in the solvent and beyond this temperature, no such significant changes were observed in extraction amount.[Citation99] Better extraction of (poly)phenols from olive pomace observed at 60 °C compared to 40 °C due to lower viscosity and surface tension along with higher solubility of (poly)phenols at 60 °C.[Citation43] Increase in (poly)phenols with an increase in temperature observed but within the temperature range of 40 to 70 °C no significant changes in (poly)phenols amount were observed.[Citation90] Similar facts with other studies like a decrease in viscosity with rising in temperature and a solubility increase with a temperature increase were also found here. Two temperature 30 and 60 °C respectively were used for extraction of (poly)phenols from Paederia scandens.[Citation77] In this study, Maximum 24.9 mg CE/g DW (poly)phenols were extracted by using 60 °C of ultrasonication temperature and on the other hand at 30 °C the maximum (poly)phenols were found as 21.17 mg CE/g DW. Six different temperature ranging from 20 to 70 °C were used to optimize the extraction of (poly)phenols from apple pomace and best result was observed at 40 °C.[Citation18] To extract (poly)phenols from Cosmos sulphureus five different temperatures were used ranging from 35 to 55 °C were used and (Poly)phenols were found in increase order till 45 °C after which it drastically decreased due to thermal degradation.[Citation82] To extract (poly)phenols from Melia azedarach, Gly-ammonium acetate solvent was used under three different temperature zones (30, 40, and 50 °C) and maximum extraction was observed at 40 °C.[Citation101]
Limitations and future perspectives
In recent times, the application of the novel UAE method is extensively used to extract different bioactive compounds; however, it has several disadvantages as well.[Citation32,Citation102,Citation103] First of all, the critical optimization process, where all the process parameters such as ultrasound power, ultrasound frequency, ultrasound intensity, sonication time, and sonication temperature are critically interrelated to obtain optimum extraction results. Thus, critical process optimization needs to be carried out with utmost attention to get optimum extraction results. Next, temperature control is very important for better extraction. The optimum temperature has a desirable window and could also be dependent on other factors. It has been reported that long sonication time and higher sonication power resulted in higher temperature and generation of ROS can make (poly)phenols to degrade.[Citation104] Sometimes high sonication frequency can also alter the conformation or molecular structure of some (poly)phenol compounds.[Citation105] In some cases, there may be the possibility of the generation of negative pressure that caused a decrease in the extraction efficiency.[Citation30]
Recently, DES-UAE has been investigated as an alternative green extraction technology for (poly)phenols and other bioactive compounds. DES-UAE has given excellent extraction results as reported in various studies. However, this combined method has also found some limitations as well.[Citation86,Citation106,Citation107] First of all, the main concern of eutectic solvent is its high viscosity and surface tension which lowered its solubility that hampers its extraction efficiency. Secondly, optimization of proper composition formulation of eutectic solvent to get desired (poly)phenols and bioactive compounds to be extracted is another critical factor to be determined to avoid poor results. Sometimes inadequate solid/liquid ratio may result in very poor extraction efficiency. Along with all the UAE parameters, the right eutectic solvent selection with proper solid/liquid ratio requires very good optimization data and at the current time, the numbers of experimental optimized data are very limited. Also, information on the toxicity of some eutectic solvents is very limited and that is a major concern for safety use of resulted in extracts and disposal. On the other hand, high-temperature generation due to high sonication time/power/intensity may hamper the extraction efficiency of the eutectic solvents, which is why all the parameters need to be optimized critically.
The limited publications on optimized DES-UAE systems for specific (poly)phenols and bioactive compounds from different plant sources is the main challenge in this research field. Due to a lack of proper data, the optimization process becomes complex in nature with high costs. Also, while DES-UAE has shown promise as a method for the extraction of (poly)phenols, there is still limited research on its use and effectiveness, particularly in comparison to traditional extraction methods. This makes it challenging to fully understand the potential advantages and limitations of this method for their applications in the industry.[Citation108] The effect of ultrasonication intensity/power/time on conformation, biological activity and molecular structure of bioactive compounds is not very clear to date so those aspects need to be investigated properly through future research. In general, the use of DES-UAE presents both challenges and opportunities for the extraction of (poly)phenols. On one hand, the selection of appropriate DES that can effectively extract the target compounds and minimize the environmental impact is a challenge. Additionally, the optimization of ultrasound parameters such as frequency, power, and time can also be difficult; however, through the application of response surface methodology, this can be overcome. Overall, the combination of ultrasound and DES can enhance the extraction efficiency of (poly)phenols and improve their bioactive properties. Furthermore, ultrasound can reduce the processing time and improve the quality of the extracts by reducing solvent usage and minimizing the degradation of the target compounds. Therefore, the use of UAE with DES presents an opportunity to develop a more sustainable and efficient extraction process for (poly)phenols.[Citation47,Citation109,Citation110]
From a safety point of view, DES have shown their potential in terms of low toxicity and easy biodegradability. More often than not, DES are thought to be less harmful than many organic solvents. Their toxicity, however, might differ based on the particular ingredients included in the eutectic composition and each counterpart of the eutectic composition needs to be assessed to determine their overall toxicity.[Citation111] Martínez et al., reported an interesting study regarding the controversy on the toxic nature of deep eutectic solvents and their possible contribution to environment pollution.[Citation112] The study highlighted about toxicity of significant numbers of DESs which previously seemed to be less toxic. This study also found that the toxicity quantification method that was conventionally used is not accurate for DES due to their low diffusibility resulting from high viscosity. For clarity on toxicity controversies, the authors suggest that more studies about toxicity/biodegradability should be conducted in the near future. From a sustainability point of view, DES are a very good alternative to conventional organic solvents. Though DES have wider acceptance as green solvents, in several studies it has been found that at higher temperatures and low pressure, many DES decomposed which resulted in harmful effects.[Citation113] For industrial applications, this decomposition may cause several detrimental effects such as flammability and explosibility.
In terms of sustainability approach, natural deep eutectic solvents (NADES) are preferable compared to DESs due to its low cost, easy availability, high purity and most importantly low toxicities.[Citation114] NADES are types that are formed by combining nontoxic quaternary ammonium salt as hydrogen receptor (usually choline chloride, ChCl) and a natural low toxic hydrogen donor such as amino acids, carboxylic acids, and sugars.[Citation113] In NADES hydrogen bonding is formed between the HBD and chloride of choline chloride and this hydrogen bonding results in the eutectic point. A study Benvenutti et al. reported a detailed comparative study between DES and NADES.[Citation115] This study suggested NADES as superior to DES due to its low-cost factor and more eco-friendly nature which makes NADES an attractive alternative for food and pharma-based applications.
The current review focuses on the factors associated with DES and UAE that impact the extraction of (poly)phenols from natural resources such as plants and agricultural products. To establish DES as a more diversified dynamic solvent, its extraction efficiency for compounds other than (poly)phenols such as carotenoids, alkaloids and terpenoids shall be explored. A recent review by Suthar et al. reported how the extraction of different biomolecules is affected by DES.[Citation116] In the case of protein extraction using DES it was observed that DES composition, solvent-to-sample molar ratio, and temperature were the vital factors to be considered.[Citation117–119] Considering carbohydrates, DES concentration, liquid-solid ratio, extraction time, temperature, and ultrasound duty cycle were found to be vital factors for overall extraction efficiency.[Citation120–124] The extraction of lipids is affected by the DES composition, solubility and ultrasound power.[Citation125,Citation126] For alkaloids, terpenoids and flavonoids it was observed that ultrasound power, extraction time, liquid-to-solid ratio along with DES composition variation resulted in optimum extraction efficiency.[Citation127–129] It has been observed that similar to (poly)phenols extraction, in the case of other biomolecules extraction the effects of the other associated factors are more or less the same but different structure configurations of different biomolecules ultimately influenced the extraction efficiency to some extent.
Conclusions
DES-UAE method has emerged as a novel and green extraction method for (poly)phenols that offers some advantages in comparison with conventional extraction methods and the use of toxic organic solvents. Ultrasonication helps to achieve extraction with low solvent extraction and low energy cost. Also, ultrasonication does not impact the properties of solvent so much as compared with other traditional extraction methods (i.e. Soxhlet extraction, maceration, and hydro distillation methods).[Citation11,Citation43,Citation100] DES alone or DES-UAE methods further enhanced the extraction efficiency to the maximum level. In general, most tested eutectic solvents possessed several advantages such as low or no toxicity to humans and the environment, reusability, solubility, extraction efficiency, and low cost. The combination of proper eutectic solvents helps to solubilize some (poly)phenols. Thus, the proper selection of eutectic solvents along with other process parameters such as low viscosity and surface tension ensures maximum extraction efficiency. Low to no toxicity of eutectic solvents also makes the extraction process safe for workers and industrial applications. Also, (poly)phenol extracts prepared from nontoxic DES can be used for human consumption once other safety aspects are verified. UAE process optimization is a critical process and thus UAE in combination with eutectic solvents needs critical process optimization, otherwise, optimum results cannot be achieved. To date, there are only limited data available on the optimization of different DES-UAE processes. Optimized UAE parameters for safe and natural eutectic solvent combinations need to be further discovered to enhance (poly)phenol extraction efficiency and use the resulting extracts in nutraceuticals, cosmeceuticals, and food additives among many other industrial applications.
CRediT author statement
Shahida Anusha Siddiqui: Validation, formal analysis, software, data curation, writing - original draft, visualization, resources, project administration. Ali Ali Redha: Conceptualization, writing – review & editing. Molla Salauddin: Writing – original draft. Iskandar Azmy Harahap: Writing – original draft. H. P. Vasantha Rupasinghe: Writing – review & editing, validation.
Disclosure statement
No potential conflict of interest was reported by the authors.
References
- Croft, K. D. Dietary Polyphenols: Antioxidants or Not? Arch. Biochem. Biophys. 2016, 595, 120–124. DOI: 10.1016/j.abb.2015.11.014.
- Fernando, S.; Kim, I. P.; Son, M.; Jeong, K. T.; Jeon, Y. Antioxidant Activity of Marine Algal Polyphenolic Compounds: A Mechanistic Approach. J. Med. Food 2016, 19, 615–628. DOI: 10.1089/jmf.2016.3706.
- Nowacka, N.; Nowak, R.; Drozd, M.; Olech, M.; Los, R.; Malm, A. Antibacterial, Antiradical Potential and Phenolic Compounds of Thirty-One Polish Mushrooms. PLoS One 2015, 10, e0140355. DOI: 10.1371/JOURNAL.PONE.0140355.
- Deng, Y.; Yang, G.; Yue, J.; Qian, B.; Liu, Z.; Wang, D.; Zhong, Y.; Zhao, Y. Influences of Ripening Stages and Extracting Solvents on the Polyphenolic Compounds, Antimicrobial and Antioxidant Activities of Blueberry Leaf Extracts. Food Control 2014, 38, 184–191. DOI: 10.1016/j.foodcont.2013.10.023.
- Joseph, S. V.; Edirisinghe, I.; Burton-Freeman, B. M. Fruit Polyphenols: A Review of anti-Inflammatory Effects in Humans. Crit. Rev. Food Sci. Nutr. 2016, 56, 419–444. DOI: 10.1080/10408398.2013.767221.
- Zhang, H.; Tsao, R. Dietary Polyphenols, Oxidative Stress and Antioxidant and anti-Inflammatory Effects. Curr. Opin. Food Sci. 2016, 8, 33–42. DOI: 10.1016/j.cofs.2016.02.002.
- Luyen, B. T. T.; Tai, B. H.; Thao, N. P.; Lee, S. H.; Jang, H. D.; Lee, Y. M.; Kim, Y. H. Evaluation of the anti-Osteoporosis and Antioxidant Activities of Phenolic Compounds from Euphorbia Maculata. J. Korean Soc. Appl. Biol. Chem. 2014, 57, 573–579. DOI: 10.1007/s13765-014-4157-2.
- Harahap, I. A.; Suliburska, J. Probiotics and Isoflavones as a Promising Therapeutic for Calcium Status and Bone Health: A Narrative Review. Foods 2021, 10 (11), 2685. DOI: 10.3390/FOODS10112685.
- Afsar, T.; Trembley, J. H.; Salomon, C. E.; Razak, S.; Khan, M. R.; Ahmed, K. Growth Inhibition and Apoptosis in Cancer Cells Induced by Polyphenolic Compounds of Acacia Hydaspica: Involvement of Multiple Signal Transduction Pathways. Sci. Rep. 2016, 6, 23077. DOI: 10.1038/srep23077.
- Hasima, N.; Ozpolat, B. Regulation of Autophagy by Polyphenolic Compounds as a Potential Therapeutic Strategy for Cancer. Cell Death Dis. 2014, 5, e1509–e1509. DOI: 10.1038/cddis.2014.467.
- Alara, O. R.; Abdurahman, N. H.; Ukaegbu, C. I. Extraction of Phenolic Compounds: A Review. Curr. Res. Food Sci. 2021, 4, 200–214. DOI: 10.1016/j.crfs.2021.03.011.
- Jha, A. K.; Sit, N. Extraction of Bioactive Compounds from Plant Materials Using Combination of Various Novel Methods: A Review. Trends Food Sci. Technol. 2022, 119, 579–591. DOI: 10.1016/j.tifs.2021.11.019.
- Ali Redha, A. Review on Extraction of Phenolic Compounds from Natural Sources Using Green Deep Eutectic Solvents. J. Agric. Food Chem. 2021, 69, 878–912. DOI: 10.1021/acs.jafc.0c06641.
- Zhang, Q.; De Oliveira Vigier, K.; Royer, S.; Jérôme, F. Deep Eutectic Solvents: Syntheses, Properties and Applications. Chem. Soc. Rev. 2012, 41, 7108–7146. DOI: 10.1039/C2CS35178A.
- Wang, P.; Tian, B.; Ge, Z.; Feng, J.; Wang, J.; Yang, K.; Sun, P.; Cai, M. Ultrasound and Deep Eutectic Solvent as Green Extraction Technology for Recovery of Phenolic Compounds from Dendrobium officinale Leaves. Process Biochem. 2023, 128, 1–11. DOI: 10.1016/j.procbio.2023.02.018.
- Zheng, B.; Yuan, Y.; Xiang, J.; Jin, W.; Johnson, J. B.; Li, Z.; Wang, C.; Luo, D. Green Extraction of Phenolic Compounds from Foxtail Millet Bran by Ultrasonic-Assisted Deep Eutectic Solvent Extraction: Optimization, Comparison and Bioactivities. LWT 2022, 154, 112740. DOI: 10.1016/j.lwt.2021.112740.
- Wu, L.; Li, L.; Chen, S.; Wang, L.; Lin, X. Deep Eutectic Solvent-Based Ultrasonic-Assisted Extraction of Phenolic Compounds from Moringa oleifera L. Leaves: Optimization, Comparison and Antioxidant Activity. Sep. Purif. Technol. 2020, 247, 117014. DOI: 10.1016/j.seppur.2020.117014.
- Rashid, R.; Mohd Wani, S.; Manzoor, S.; Masoodi, F. A.; Masarat Dar, M. Green Extraction of Bioactive Compounds from Apple Pomace by Ultrasound Assisted Natural Deep Eutectic Solvent Extraction: Optimisation, Comparison and Bioactivity. Food Chem. 2023, 398, 133871. DOI: 10.1016/j.foodchem.2022.133871.
- Lanjekar, K.; Gokhale, S.; Rathod, V. Utilization of Waste Mango Peels for Extraction of Polyphenolic Antioxidants by Ultrasound-Assisted Natural Deep Eutectic Solvent (Ua-Nades). Bioresour. Technol. Rep. 2022, 18, 101074. DOI: 10.1016/j.biteb.2022.101074.
- Patil, S. S.; Pathak, A.; Rathod, V. K. Optimization and Kinetic Study of Ultrasound Assisted Deep Eutectic Solvent Based Extraction: A Greener Route for Extraction of Curcuminoids from Curcuma longa. Ultrason. Sonochem. 2021, 70, 105267. DOI: 10.1016/j.ultsonch.2020.105267.
- Lanjekar, K. J.; Rathod, V. K. Application of Ultrasound and Natural Deep Eutectic Solvent for the Extraction of Glycyrrhizic Acid from Glycyrrhiza glabra: Optimization and Kinetic Evaluation. Ind. Eng. Chem. Res. 2021, 60, 9532–9538. DOI: 10.1021/acs.iecr.1c00862.
- Cherif, M. M.; Grigorakis, S.; Halahlah, A.; Loupassaki, S.; Makris, D. P. High-Efficiency Extraction of Phenolics from Wheat Waste Biomass (Bran) by Combining Deep Eutectic Solvent, Ultrasound-Assisted Pretreatment and Thermal Treatment. Environ. Process 2020, 7, 845–859. DOI: 10.1007/s40710-020-00449-0.
- Fanali, C.; Posta, S.; Della; Dugo, L.; Russo, M.; Gentili, A.; Mondello, L.; De Gara, L. Application of Deep Eutectic Solvents for the Extraction of Phenolic Compounds from Extra-Virgin Olive Oil. Electrophoresis 2020, 41, 1752–1759. DOI: 10.1002/elps.201900423.
- Vorobyova, V.; Skiba, M.; Vasyliev, G. Extraction of Phenolic Compounds from Tomato Pomace Using Choline Chloride–Based Deep Eutectic Solvents. Food Measure 2022, 16, 1087–1104. DOI: 10.1007/s11694-021-01238-5.
- Alam, M. A.; Muhammad, G.; Khan, M. N.; Mofijur, M.; Lv, Y.; Xiong, W.; Xu, J. Choline Chloride-Based Deep Eutectic Solvents as Green Extractants for the Isolation of Phenolic Compounds from Biomass. J. Clean. Prod. 2021, 309, 127445. DOI: 10.1016/j.jclepro.2021.127445.
- Kumar, K.; Srivastav, S.; Sharanagat, V. S. Ultrasound Assisted Extraction (UAE) of Bioactive Compounds from Fruit and Vegetable Processing by-Products: A Review. Ultrason. Sonochem. 2021, 70, 105325. DOI: 10.1016/j.ultsonch.2020.105325.
- Kentish, S.; Ashokkumar, M. The Physical and Chemical Effects of Ultrasound. Ann. Rev. Phys. Chem. 2011, 71, 1–12. DOI: 10.1007/978-1-4419-7472-3_1.
- Ali Redha, A.; Siddiqui, S. A.; Ibrahim, S. A. Advanced Extraction Techniques for Berberis Species Phytochemicals: A Review. Int. J. Food Sci. Tech. 2021, 56, 5485–5496. DOI: 10.1111/ijfs.15315.
- Yusoff, I. M.; Mat Taher, Z.; Rahmat, Z.; Chua, L. S. A Review of Ultrasound-Assisted Extraction for Plant Bioactive Compounds: Phenolics, Flavonoids, Thymols, Saponins and Proteins. Food Res. Int. 2022, 157, 111268. DOI: 10.1016/j.foodres.2022.111268.
- Chemat, F.; Rombaut, N.; Sicaire, A. G.; Meullemiestre, A.; Fabiano-Tixier, A. S.; Abert-Vian, M. Ultrasound Assisted Extraction of Food and Natural Products. Mechanisms, Techniques, Combinations, Protocols and Applications. A Review. Ultrason. Sonochem. 2017, 34, 540–560. DOI: 10.1016/j.ultsonch.2016.06.035.
- Lavilla, I.; Bendicho, C. Fundamentals of Ultrasound-Assisted Extraction. In Water Extraction of Bioactive Compounds; Elsevier, 2017; pp 291–316. DOI: 10.1016/B978-0-12-809380-1.00011-5.
- Wen, C.; Zhang, J.; Zhang, H.; Dzah, C. S.; Zandile, M.; Duan, Y.; Ma, H.; Luo, X. Advances in Ultrasound Assisted Extraction of Bioactive Compounds from Cash Crops – A Review. Ultrason. Sonochem. 2018, 48, 538–549. DOI: 10.1016/J.ULTSONCH.2018.07.018.
- Tabaraki, R.; Nateghi, A. Optimization of Ultrasonic-Assisted Extraction of Natural Antioxidants from Rice Bran Using Response Surface Methodology. Ultrason. Sonochem. 2011, 18, 1279–1286. DOI: 10.1016/j.ultsonch.2011.05.004.
- Liao, J.; Zheng, N.; Qu, B. An Improved Ultrasonic-Assisted Extraction Method by Optimizing the Ultrasonic Frequency for Enhancing the Extraction Efficiency of Lycopene from Tomatoes. Food Anal. Methods 2016, 9, 2288–2298. DOI: 10.1007/s12161-016-0419-4.
- Al-Khazrajy, O. S. A.; Boxall, A. B. A. Determination of Pharmaceuticals in Freshwater Sediments Using Ultrasonic-Assisted Extraction with SPE Clean-up and HPLC-DAD or LC-ESI-MS/MS Detection. Anal. Methods 2017, 9, 4190–4200. DOI: 10.1039/C7AY00650K.
- Tian, Y.; Sun, D. W.; Xu, L.; Fan, T. H.; Zhu, Z. Bio-Inspired Eutectogels Enabled by Binary Natural Deep Eutectic Solvents (NADESs): Interfacial anti-Frosting, Freezing-Tolerance, and Mechanisms. Food Hydrocoll. 2022, 128, 107568. DOI: 10.1016/j.foodhyd.2022.107568.
- Li, T.; Song, Y.; Li, J.; Zhang, M.; Shi, Y.; Fan, J. New Low Viscous Hydrophobic Deep Eutectic Solvents in Vortex-Assisted Liquid-Liquid Microextraction for the Determination of Phthalate Esters from Food-Contacted Plastics. Food Chem. 2020, 309, 125752. DOI: 10.1016/j.foodchem.2019.125752.
- Florindo, C.; Branco, L. C.; Marrucho, I. M. Quest for Green‐Solvent Design: From Hydrophilic to Hydrophobic (Deep) Eutectic Solvents. ChemSusChem 2019, 12, 1549–1559. DOI: 10.1002/cssc.201900147.
- Shahbaz, K.; Mjalli, F. S.; Hashim, M. A.; AlNashef, I. M. Prediction of the Surface Tension of Deep Eutectic Solvents. Fluid Phase Equilib. 2012, 319, 48–54. DOI: 10.1016/j.fluid.2012.01.025.
- Ali, M. C.; Chen, J.; Zhang, H.; Li, Z.; Zhao, L.; Qiu, H. Effective Extraction of Flavonoids from Lycium Barbarum L. Fruits by Deep Eutectic Solvents-Based Ultrasound-Assisted Extraction. Talanta 2019, 203, 16–22. DOI: 10.1016/J.TALANTA.2019.05.012.
- Hansen, B. B.; Spittle, S.; Chen, B.; Poe, D.; Zhang, Y.; Klein, J. M.; Horton, A.; Adhikari, L.; Zelovich, T.; Doherty, B. W.; et al. Deep Eutectic Solvents: A Review of Fundamentals and Applications. Chem. Rev. 2021, 121, 1232–1285. DOI: 10.1021/acs.chemrev.0c00385.
- Merouani, S.; Hamdaoui, O.; Rezgui, Y.; Guemini, M. Computational Engineering Study of Hydrogen Production via Ultrasonic Cavitation in Water. Int. J. Hydrogen Energy 2016, 41, 832–844. DOI: 10.1016/j.ijhydene.2015.11.058.
- Chanioti, S.; Tzia, C. Extraction of Phenolic Compounds from Olive Pomace by Using Natural Deep Eutectic Solvents and Innovative Extraction Techniques. Innov. Food Sci. Emerg. Technol. 2018, 48, 228–239. DOI: 10.1016/j.ifset.2018.07.001.
- Juneja, S.; Pandey, S.; Deepika . Water Miscibility, Surface Tension, Density, and Dynamic Viscosity of Hydrophobic Deep Eutectic Solvents Composed of Capric Acid, Menthol, and Thymol. J. Chem. Eng. Data 2022, 67 (11), 3400–3413. DOI: 10.1021/acs.jced.2c00495.
- Bosiljkov, T.; Dujmić, F.; Cvjetko Bubalo, M.; Hribar, J.; Vidrih, R.; Brnčić, M.; Zlatic, E.; Radojčić Redovniković, I.; Jokić, S. Natural Deep Eutectic Solvents and Ultrasound-Assisted Extraction: Green Approaches for Extraction of Wine Lees Anthocyanins. Food Bioprod. Process 2017, 102, 195–203. DOI: 10.1016/j.fbp.2016.12.005.
- Krishnan, A.; Gopinath, K. P.; Vo, D. V. N.; Malolan, R.; Nagarajan, V. M.; Arun, J. Ionic Liquids, Deep Eutectic Solvents and Liquid Polymers as Green Solvents in Carbon Capture Technologies: A Review. Environ. Chem. Lett. 2020, 18, 2031–2054. DOI: 10.1007/s10311-020-01057-y.
- Oke, E. A.; Ijardar, S. P. Advances in the Application of Deep Eutectic Solvents Based Aqueous Biphasic Systems: An up-to-Date Review. Biochem. Eng. J. 2021, 176, 108211. DOI: 10.1016/j.bej.2021.108211.
- Panja, P. Green Extraction Methods of Food Polyphenols from Vegetable Materials. Curr. Opin. Food Sci. 2018, 23, 173–182. DOI: 10.1016/j.cofs.2017.11.012.
- Chemat, F.; Khan, M. K.; Zill-e-Huma . Applications of Ultrasound in Food Technology: Processing, Preservation and Extraction.Ultrason. Sonochem., 2011, 18 (4), 813–835. DOI: 10.1016/j.ultsonch.2010.11.023.
- Qin, G.; Lei, J.; Li, S.; Jiang, Y.; Qiao, L.; Ren, M.; Gao, Q.; Song, C.; Fu, S.; Zhou, J.; et al. Efficient, Green Extraction of Two Biflavonoids from Selaginella uncinata with Deep Eutectic Solvents. Microchem. J. 2022, 183, 108085. DOI: 10.1016/j.microc.2022.108085.
- Wang, X. H.; Wang, J. P. Effective Extraction with Deep Eutectic Solvents and Enrichment by Macroporous Adsorption Resin of Flavonoids from Carthamus tinctorius L. J. Pharm. Biomed. Anal. 2019, 176, 112804. DOI: 10.1016/j.jpba.2019.112804.
- Mehariya, S.; Fratini, F.; Lavecchia, R.; Zuorro, A. Green Extraction of Value-Added Compounds Form Microalgae: A Short Review on Natural Deep Eutectic Solvents (NaDES) and Related Pre-Treatments. J. Environ. Chem. Eng. 2021, 9, 105989. DOI: 10.1016/j.jece.2021.105989.
- Bajkacz, S.; Adamek, J. Evaluation of New Natural Deep Eutectic Solvents for the Extraction of Isoflavones from Soy Products. Talanta 2017, 168, 329–335. DOI: 10.1016/j.talanta.2017.02.065.
- Roselló-Soto, E.; Galanakis, C. M.; Brnčić, M.; Orlien, V.; Trujillo, F. J.; Mawson, R.; Knoerzer, K.; Tiwari, B. K.; Barba, F. J. Clean Recovery of Antioxidant Compounds from Plant Foods, by-Products and Algae Assisted by Ultrasounds Processing. Modeling Approaches to Optimize Processing Conditions. Trends Food Sci. Technol. 2015, 42, 134–149. DOI: 10.1016/j.tifs.2015.01.002.
- Kazemi, M.; Khodaiyan, F.; Labbafi, M.; Hosseini, S. S. Ultrasonic and Heating Extraction of Pistachio by-Product Pectin: Physicochemical, Structural Characterization and Functional Measurement. Food Measure 2020, 14, 679–693. DOI: 10.1007/s11694-019-00315-0.
- Adetunji, L. R.; Adekunle, A.; Orsat, V.; Raghavan, V. Advances in the Pectin Production Process Using Novel Extraction Techniques: A Review. Food Hydrocoll. 2017, 62, 239–250. DOI: 10.1016/j.foodhyd.2016.08.015.
- Zhao, S.; Yao, C.; Liu, L.; Chen, G. Parametrical Investigation of Acoustic Cavitation and Extraction Enhancement in Ultrasonic Microreactors. Chem. Eng. J. 2022, 450, 138185. DOI: 10.1016/j.cej.2022.138185.
- Zinoviadou, K. G.; Galanakis, C. M.; Brnčić, M.; Grimi, N.; Boussetta, N.; Mota, M. J.; Saraiva, J. A.; Patras, A.; Tiwari, B.; Barba, F. J. Fruit Juice Sonication: Implications on Food Safety and Physicochemical and Nutritional Properties. Food Res. Int. 2015, 77, 743–752. DOI: 10.1016/j.foodres.2015.05.032.
- González-Centeno, M. R.; Knoerzer, K.; Sabarez, H.; Simal, S.; Rosselló, C.; Femenia, A. Effect of Acoustic Frequency and Power Density on the Aqueous Ultrasonic-Assisted Extraction of Grape Pomace (Vitis vinifera L.) – A Response Surface Approach. Ultrason. Sonochem. 2014, 21, 2176–2184. DOI: 10.1016/j.ultsonch.2014.01.021.
- Leong, T.; Ashokkumar, M.; Kentish, S. The Fundamentals of Power Ultrasound – A Review. Acoust. Aust. 2002, 30, 88.
- Mason, T. J.; Cobley, A. J.; Graves, J. E.; Morgan, D. New Evidence for the Inverse Dependence of Mechanical and Chemical Effects on the Frequency of Ultrasound. Ultrason. Sonochem. 2011, 18, 226–230. DOI: 10.1016/j.ultsonch.2010.05.008.
- Chemat, F.; Rombaut, N.; Meullemiestre, A.; Turk, M.; Perino, S.; Fabiano-Tixier, A. S.; Abert-Vian, M. Review of Green Food Processing Techniques. Preservation, Transformation, and Extraction. Innov. Food Sci. Emerg. Technol. 2017, 41, 357–377. DOI: 10.1016/j.ifset.2017.04.016.
- Palma, M.; Barroso, C. G. Ultrasound-Assisted Extraction and Determination of Tartaric and Malic Acids from Grapes and Winemaking by-Products. Anal. Chim. Acta 2002, 458, 119–130. DOI: 10.1016/S0003-2670(01)01527-6.
- Xu, Y.; Zhang, L.; Bailina, Y.; Ge, Z.; Ding, T.; Ye, X.; Liu, D. Effects of Ultrasound and/or Heating on the Extraction of Pectin from Grapefruit Peel. J. Food Eng. 2014, 126, 72–81. DOI: 10.1016/j.jfoodeng.2013.11.004.
- Samaram, S.; Mirhosseini, H.; Tan, C. P.; Ghazali, H. M.; Bordbar, S.; Serjouie, A. Optimisation of Ultrasound-Assisted Extraction of Oil from Papaya Seed by Response Surface Methodology: Oil Recovery, Radical Scavenging Antioxidant Activity, and Oxidation Stability. Food Chem. 2015, 172, 7–17. DOI: 10.1016/j.foodchem.2014.08.068.
- Maran, J. P.; Priya, B. Ultrasound-Assisted Extraction of Polysaccharide from Nephelium lappaceum L. Fruit Peel. Int. J. Biol. Macromol. 2014, 70, 530–536. DOI: 10.1016/j.ijbiomac.2014.07.032.
- Tiwari, B. K. Ultrasound: A Clean, Green Extraction Technology. TrAC Trends Anal. Chem. 2015, 71, 100–109. DOI: 10.1016/j.trac.2015.04.013.
- Pan, Z.; Qu, W.; Ma, H.; Atungulu, G. G.; McHugh, T. H. Continuous and Pulsed Ultrasound-Assisted Extractions of Antioxidants from Pomegranate Peel. Ultrason. Sonochem. 2012, 19, 365–372. DOI: 10.1016/j.ultsonch.2011.05.015.
- Xu, Y. X.; Zhang, M.; Fang, Z. X.; Sun, J. C.; Wang, Y. Q. How to Improve Bayberry (Myrica rubra Sieb. et Zucc.) Juice Flavour Quality: Effect of Juice Processing and Storage on Volatile Compounds. Food Chem. 2014, 151, 40–46. DOI: 10.1016/j.foodchem.2013.10.118.
- More, P. R.; Arya, S. S. Intensification of Bio-Actives Extraction from Pomegranate Peel Using Pulsed Ultrasound: Effect of Factors, Correlation, Optimization and Antioxidant Bioactivities. Ultrason. Sonochem. 2021, 72, 105423. DOI: 10.1016/j.ultsonch.2020.105423.
- Kobus, Z.; Krzywicka, M.; Starek-Wójcicka, A.; Sagan, A. Effect of the Duty Cycle of the Ultrasonic Processor on the Efficiency of Extraction of Phenolic Compounds from Sorbus Intermedia. Sci. Rep. 2022, 12, 8311. DOI: 10.1038/s41598-022-12244-y.
- Mouratoglou, E.; Malliou, V.; Makris, D. P. Novel Glycerol-Based Natural Eutectic Mixtures and Their Efficiency in the Ultrasound-Assisted Extraction of Antioxidant Polyphenols from Agri-Food Waste Biomass. Waste Biomass Valor 2016, 7, 1377–1387. DOI: 10.1007/s12649-016-9539-8.
- Zannou, O.; Pashazadeh, H.; Ibrahim, S. A.; Koca, I.; Galanakis, C. M. Green and Highly Extraction of Phenolic Compounds and Antioxidant Capacity from Kinkeliba (Combretum micranthum G. Don) by Natural Deep Eutectic Solvents (NADESs) Using Maceration, Ultrasound-Assisted Extraction and Homogenate-Assisted Extraction. Arab. J. Chem. 2022, 15, 103752. DOI: 10.1016/j.arabjc.2022.103752.
- Alasalvar, H.; Yildirim, Z. Ultrasound-Assisted Extraction of Antioxidant Phenolic Compounds from Lavandula angustifolia Flowers Using Natural Deep Eutectic Solvents: An Experimental Design Approach. Sustain. Chem. Pharm. 2021, 22, 100492. DOI: 10.1016/j.scp.2021.100492.
- Cui, Q.; Liu, J. Z.; Wang, L. T.; Kang, Y. F.; Meng, Y.; Jiao, J.; Fu, Y. J. Sustainable Deep Eutectic Solvents Preparation and Their Efficiency in Extraction and Enrichment of Main Bioactive Flavonoids from Sea Buckthorn Leaves. J. Clean. Prod. 2018, 184, 826–835. DOI: 10.1016/j.jclepro.2018.02.295.
- Ozbek Yazici, S.; Ozmen, İ. Ultrasound Assisted Extraction of Phenolic Compounds from Capparis ovata Var Canescens Fruit Using Deep Eutectic Solvents. Food Process. Preserv. 2022, 46, e16286. DOI: 10.1111/jfpp.16286.
- Liu, Y.; Zhe, W.; Zhang, R.; Peng, Z.; Wang, Y.; Gao, H.; Guo, Z.; Xiao, J. Ultrasonic-Assisted Extraction of Polyphenolic Compounds from Paederia scandens (Lour.) Merr. Using Deep Eutectic Solvent: Optimization, Identification, and Comparison with Traditional Methods. Ultrason Sonochem 2022, 86, 106005. DOI: 10.1016/j.ultsonch.2022.106005.
- Lanjekar, K. J.; Gokhale, S.; Rathod, V. K. Utilization of Waste Mango Peels for Extraction of Polyphenolic Antioxidants by Ultrasound-Assisted Natural Deep Eutectic Solvent. Bioresour. Technol. Rep. 2022, 18, 101074. DOI: 10.1016/j.biteb.2022.101074.
- Osamede Airouyuwa, J.; Mostafa, H.; Riaz, A.; Maqsood, S. Utilization of Natural Deep Eutectic Solvents and Ultrasound-Assisted Extraction as Green Extraction Technique for the Recovery of Bioactive Compounds from Date Palm (Phoenix dactylifera L.) Seeds: An Investigation into Optimization of Process Parameters. Ultrason. Sonochem. 2022, 91, 106233. DOI: 10.1016/j.ultsonch.2022.106233.
- Shi, F.; Hai, X.; Zhu, Y.; Ma, L.; Wang, L.; Yin, J.; Li, X.; Yang, Z.; Yuan, M.; Xiong, H.; Gao, Y. Ultrasonic Assisted Extraction of Polyphenols from Bayberry by Deep Eutectic Supramolecular Polymer and Its Application in Bio-Active Film. Ultrason. Sonochem. 2023, 92, 106283. DOI: 10.1016/j.ultsonch.2022.106283.
- Zannou, O.; Pashazadeh, H.; Galanakis, C. M.; Alamri, A. S.; Koca, I. Carboxylic Acid-Based Deep Eutectic Solvents Combined with Innovative Extraction Techniques for Greener Extraction of Phenolic Compounds from Sumac (Rhus coriaria L.). J. Appl. Res. Med. Aromat. Plants 2022, 30, 100380. DOI: 10.1016/j.jarmap.2022.100380.
- Liu, X. Y.; Ou, H.; Gregersen, H.; Zuo, J. Deep Eutectic Solvent-Based Ultrasound-Assisted Extraction of Polyphenols from Cosmos Sulphureus. J. Appl. Res. Med. Aromat. Plants 2023, 32, 100444. DOI: 10.1016/j.jarmap.2022.100444.
- Tebbi, S. O.; Debbache-Benaida, N.; Kadri, N.; Kadi, R.; Zaidi, S. A Novel Strategy to Improve the Recovery of Phenolic Compounds from Pistacia lentiscus L. Fruits Using Design-Based Statistical Modeling for Ultrasound-Deep Eutectic Solvents Extraction and the Evaluation of Their Antioxidant Potential. Sustain. Chem. Pharm 2023, 31, 100933. DOI: 10.1016/j.scp.2022.100933.
- Duarte, H.; Gomes, V.; Aliaño-González, M. J.; Faleiro, L.; Romano, A.; Medronho, B. Ultrasound-Assisted Extraction of Polyphenols from Maritime Pine Residues with Deep Eutectic Solvents. Foods 2022, 11, 3754. DOI: 10.3390/foods11233754.
- Ivanović, M.; Razboršek, M. I.; Kolar, M. Innovative Extraction Techniques Using Deep Eutectic Solvents and Analytical Methods for the Isolation and Characterization of Natural Bioactive Compounds from Plant Material. Plants (Basel) 2020, 9, 1428. DOI: 10.3390/PLANTS9111428.
- Dheyab, A. S.; Bakar, M. F. A.; Alomar, M.; Sabran, S. F.; Hanafi, A. F. M.; Mohamad, A. Deep Eutectic Solvents (DESs) as Green Extraction Media of Beneficial Bioactive Phytochemicals. Separations 2021, 8, 176. DOI: 10.3390/separations8100176.
- Mišan, A.; Nađpal, J.; Stupar, A.; Pojić, M.; Mandić, A.; Verpoorte, R.; Choi, Y. H. The Perspectives of Natural Deep Eutectic Solvents in Agri-Food Sector. Crit. Rev. Food Sci. Nutr. 2020, 60, 2564–2592. DOI: 10.1080/10408398.2019.1650717.
- Yuniarti, E.; C. Saputri, F.; Mun’im, A. Natural Deep Eutectic Solvent Extraction and Evaluation of Caffeine and Chlorogenic Acid from Green Coffee Beans of Coffea Canephora. ijps 2019, 81, 1062–1069. DOI: 10.36468/pharmaceutical-sciences.604.
- Zhou, P.; Wang, X.; Liu, P.; Huang, J.; Wang, C.; Pan, M.; Kuang, Z. Enhanced Phenolic Compounds Extraction from Morus alba L. Leaves by Deep Eutectic Solvents Combined with Ultrasonic-Assisted Extraction. Ind. Crops Prod 2018, 120, 147–154. DOI: 10.1016/j.indcrop.2018.04.071.
- Wu, L.; Chen, Z.; Li, S.; Wang, L.; Zhang, J. Eco-Friendly and High-Efficient Extraction of Natural Antioxidants from Polygonum aviculare Leaves Using Tailor-Made Deep Eutectic Solvents as Extractants. Sep. Purif. Technol. 2021, 262, 118339. DOI: 10.1016/j.seppur.2021.118339.
- Mansur, A. R.; Song, N. E.; Jang, H. W.; Lim, T. G.; Yoo, M.; Nam, T. G. Optimizing the Ultrasound-Assisted Deep Eutectic Solvent Extraction of Flavonoids in Common Buckwheat Sprouts. Food Chem. 2019, 293, 438–445. DOI: 10.1016/J.FOODCHEM.2019.05.003.
- Plaza, M.; Domínguez-Rodríguez, G.; Sahelices, C.; Marina, M. L. A Sustainable Approach for Extracting Non-Extractable Phenolic Compounds from Mangosteen Peel Using Ultrasound-Assisted Extraction and Natural Deep Eutectic Solvents. Appl. Sci. 2021, 11, 5625. DOI: 10.3390/app11125625.
- Gupta, S.; Pradheep, K. Diversity, Conservation and Use of Underutilized and Minor Fruits in India: An Overview. Acta Hortic. 2020, 1297, 51–60. DOI: 10.17660/ActaHortic.2020.1297.8.
- Altemimi, A.; Watson, D. G.; Choudhary, R.; Dasari, M. R.; Lightfoot, D. A. Ultrasound Assisted Extraction of Phenolic Compounds from Peaches and Pumpkins. PLoS One 2016, 11, e0148758. DOI: 10.1371/JOURNAL.PONE.0148758.
- Vieira, V.; Prieto, M. A.; Barros, L.; Coutinho, J. A. P.; Ferreira, I. C. F. R.; Ferreira, O. Enhanced Extraction of Phenolic Compounds Using Choline Chloride Based Deep Eutectic Solvents from Juglans regia L. Ind. Crops Prod. 2018, 115, 261–271. DOI: 10.1016/j.indcrop.2018.02.029.
- Mansinhos, I.; Gonçalves, S.; Rodríguez-Solana, R.; Ordóñez-Díaz, J. L.; Moreno-Rojas, J. M.; Romano, A. Ultrasonic-Assisted Extraction and Natural Deep Eutectic Solvents Combination: A Green Strategy to Improve the Recovery of Phenolic Compounds from Lavandula pedunculata Subsp. Lusitanica (Chaytor) Franco. Antioxidants (Basel) 2021, 10, 582. DOI: 10.3390/ANTIOX10040582.
- Altemimi, A.; Lakhssassi, N.; Baharlouei, A.; Watson, D. G.; Lightfoot, D. A. Phytochemicals: Extraction, Isolation, and Identification of Bioactive Compounds from Plant Extracts. Plants (Basel) 2017, 6, 42. DOI: 10.3390/plants6040042.
- Çelik, S.; Kutlu, N.; Gerçek, Y. C.; Bayram, S.; Pandiselvam, R.; Bayram, N. E. Optimization of Ultrasonic Extraction of Nutraceutical and Pharmaceutical Compounds from Bee Pollen with Deep Eutectic Solvents Using Response Surface Methodology. Foods 2022, 11, 3652. DOI: 10.3390/foods11223652.
- Naseem, Z.; Zahid, M.; Hanif, M. A.; Shahid, M. Environmentally Friendly Extraction of Bioactive from Mentha arvensis Using Deep Solvent as Green Extraction Media. Pol. J. Environ. Stud. 2020, 29, 3749–3757. DOI: 10.15244/pjoes/114235.
- Cvjetko Bubalo, M.; Ćurko, N.; Tomašević, M.; Kovačević Ganić, K.; Radojcic Redovnikovic, I. Green Extraction of Grape Skin Phenolics by Using Deep Eutectic Solvents. Food Chem. 2016, 200, 159–166. DOI: 10.1016/J.FOODCHEM.2016.01.040.
- Jamshaid, S.; Ahmed, D.; Aydar, A. Y. Ultrasound-Assisted Extraction Optimization of Polyphenols, Flavonoids, and Antioxidant Compounds from Fruit of Melia azedarach Using a Glycerol-Based Green Deep Eutectic Solvent. J. Food Process. Preserv. 2022, 46 (8), e16657. DOI: 10.1111/jfpp.16657.
- Carreira-Casais, A.; Otero, P.; Garcia-Perez, P.; Garcia-Oliveira, P.; Pereira, A. G.; Carpena, M.; Soria-Lopez, A.; Simal-Gandara, J.; Prieto, M. A. Benefits and Drawbacks of Ultrasound-Assisted Extraction for the Recovery of Bioactive Compounds from Marine Algae. IJERPH 2021, 18, 9153. DOI: 10.3390/ijerph18179153.
- Ebringerová, A.; Hromádková, Z. An Overview on the Application of Ultrasound in Extraction, Separation and Purification of Plant Polysaccharides. Cent. Eur. J. Chem. 2010, 8, 243–257. DOI: 10.2478/s11532-010-0006-2.
- Um, M.; Han, T. H.; Lee, J. W. Ultrasound-Assisted Extraction and Antioxidant Activity of Phenolic and Flavonoid Compounds and Ascorbic Acid from Rugosa Rose (Rosa rugosa Thunb.) Fruit. Food Sci. Biotechnol. 2018, 27, 375. DOI: 10.1007/S10068-017-0247-3.
- Naik, A. S.; Suryawanshi, D.; Kumar, M.; Waghmare, R. Ultrasonic Treatment: A Cohort Review on Bioactive Compounds, Allergens and Physico-Chemical Properties of Food. Curr. Res. Food Sci. 2021, 4, 470–477. DOI: 10.1016/J.CRFS.2021.07.003.
- Kalhor, P.; Ghandi, K. Deep Eutectic Solvents for Pretreatment, Extraction, and Catalysis of Biomass and Food Waste. Molecules 2019, 24, 4012. DOI: 10.3390/MOLECULES24224012.
- Lomba, L.; Ribate, M. P.; Sangüesa, E.; Concha, J.; Garralaga, M. P.; Errazquin, D.; García, C. B.; Giner, B. Deep Eutectic Solvents: Are They Safe? Appl. Sci. 2021, 11 (21), 10061. DOI: 10.3390/app112110061.
- Florindo, C.; Lima, F.; Ribeiro, B. D.; Marrucho, I. M. Deep Eutectic Solvents: Overcoming 21st Century Challenges. Curr. Opin. Green Sustain. Chem. 2019, 18, 31–36. DOI: 10.1016/j.cogsc.2018.12.003.
- Dzah, C. S.; Duan, Y.; Zhang, H.; Wen, C.; Zhang, J.; Chen, G.; Ma, H. The Effects of Ultrasound Assisted Extraction on Yield, Antioxidant, Anticancer and Antimicrobial Activity of Polyphenol Extracts: A Review. Food Biosci. 2020, 35, 100547. DOI: 10.1016/j.fbio.2020.100547.
- Ruesgas-Ramón, M.; Figueroa-Espinoza, M. C.; Durand, E. Application of Deep Eutectic Solvents (DES) for Phenolic Compounds Extraction: Overview, Challenges, and Opportunities. J. Agric. Food Chem. 2017, 65, 3591–3601. DOI: 10.1021/acs.jafc.7b01054.
- Halder, A. K.; Cordeiro, M. N. D. S. Probing the Environmental Toxicity of Deep Eutectic Solvents and Their Components: An in Silico Modeling Approach. ACS Sustain. Chem. Eng. 2019, 7, 10649–10660. DOI: 10.1021/ACSSUSCHEMENG.9B01306/ASSET/IMAGES/LARGE/SC-2019-01306A_0004.JPEG.
- Martínez, G. M.; Townley, G. G.; Martínez-Espinosa, R. M. Controversy on the Toxic Nature of Deep Eutectic Solvents and Their Potential Contribution to Environmental Pollution. Heliyon 2022, 8, e12567. DOI: 10.1016/J.HELIYON.2022.E12567.
- Chen, Y.; Mu, T. Revisiting Greenness of Ionic Liquids and Deep Eutectic Solvents. Green Chem. Eng. 2021, 2, 174–186. DOI: 10.1016/j.gce.2021.01.004.
- Hikmawanti, N. P. E.; Ramadon, D.; Jantan, I.; Mun’im, A. Natural Deep Eutectic Solvents (NADES): Phytochemical Extraction Performance Enhancer for Pharmaceutical and Nutraceutical Product Development. Plants (Basel) 2021, 10, 2091. DOI: 10.3390/PLANTS10102091.
- Benvenutti, L.; Zielinski, A. A. F.; Ferreira, S. R. S. Which is the Best Food Emerging Solvent: IL, DES or NADES? Trends Food Sci. Technol. 2019, 90, 133–146. DOI: 10.1016/j.tifs.2019.06.003.
- Suthar, P.; Kaushal, M.; Vaidya, D.; Thakur, M.; Chauhan, P.; Angmo, D.; Kashyap, S.; Negi, N. Deep Eutectic Solvents (DES): An Update on the Applications in Food Sectors. J. Agric. Food Res. 2023, 14, 100678. DOI: 10.1016/j.jafr.2023.100678.
- Lin, Z.; Jiao, G.; Zhang, J.; Celli, G. B.; Brooks, M. S. L. Optimization of Protein Extraction from Bamboo Shoots and Processing Wastes Using Deep Eutectic Solvents in a Biorefinery Approach. Biomass Conv. Bioref. 2021, 11, 2763–2774. DOI: 10.1007/S13399-020-00614-3/METRICS.
- Lin, J.; Xiang, H.; Sun-Waterhouse, D.; Cui, C.; Wang, W. Deep Eutectic Solvents and Alkaline Extraction of Protein from Seabuckthorn Seed Meal: A Comparison Study. Food Sci. Hum. Wellness 2022, 11, 1028–1035. DOI: 10.1016/j.fshw.2022.03.019.
- Rodrigues, L. A.; Leonardo, I. C.; Gaspar, F. B.; Roseiro, L. C.; Duarte, A. R. C.; Matias, A. A.; Paiva, A. Unveiling the Potential of Betaine/Polyol-Based Deep Eutectic Systems for the Recovery of Bioactive Protein Derivative-Rich Extracts from Sardine Processing Residues. Sep. Purif. Technol. 2021, 276, 119267. DOI: 10.1016/j.seppur.2021.119267.
- Cai, C.; Wang, Y.; Yu, W.; Wang, C.; Li, F.; Tan, Z. Temperature-Responsive Deep Eutectic Solvents as Green and Recyclable Media for the Efficient Extraction of Polysaccharides from Ganoderma Lucidum. J. Clean. Prod. 2020, 274, 123047. DOI: 10.1016/j.jclepro.2020.123047.
- Shang, X. C.; Chu, D.; Zhang, J. X.; Zheng, Y. F.; Li, Y. Microwave-Assisted Extraction, Partial Purification and Biological Activity in Vitro of Polysaccharides from Bladder-Wrack (Fucus vesiculosus) by Using Deep Eutectic Solvents. Sep. Purif. Technol. 2021, 259, 118169. DOI: 10.1016/j.seppur.2020.118169.
- Nie, J.; Chen, D.; Lu, Y. Deep Eutectic Solvents Based Ultrasonic Extraction of Polysaccharides from Edible Brown Seaweed Sargassum horneri. JMSE 2020, 8, 440. DOI: 10.3390/jmse8060440.
- Zhu, P.; Gu, Z.; Hong, S.; Lian, H. One-Pot Production of Chitin with High Purity from Lobster Shells Using Choline Chloride–Malonic Acid Deep Eutectic Solvent. Carbohydr. Polym. 2017, 177, 217–223. DOI: 10.1016/J.CARBPOL.2017.09.001.
- Li, H.; Liang, J.; Chen, L.; Ren, M.; Zhou, C. Utilization of Walnut Shell by Deep Eutectic Solvents: Enzymatic Digestion of Cellulose and Preparation of Lignin Nanoparticles. Ind. Crops Prod. 2023, 192, 116034. DOI: 10.1016/j.indcrop.2022.116034.
- Pitacco, W.; Samorì, C.; Pezzolesi, L.; Gori, V.; Grillo, A.; Tiecco, M.; Vagnoni, M.; Galletti, P. Extraction of Astaxanthin from Haematococcus pluvialis with Hydrophobic Deep Eutectic Solvents Based on Oleic Acid. Food Chem. 2022, 379, 132156. DOI: 10.1016/J.FOODCHEM.2022.132156.
- Wils, L.; Leman-Loubière, C.; Bellin, N.; Clément-Larosière, B.; Pinault, M.; Chevalier, S.; Enguehard-Gueiffier, C.; Bodet, C.; Boudesocque-Delaye, L. Natural Deep Eutectic Solvent Formulations for Spirulina: Preparation, Intensification, and Skin Impact. Algal Res. 2021, 56, 102317. DOI: 10.1016/j.algal.2021.102317.
- Mohd Fuad, F.; Mohd Nadzir, M. Ultrasound-Assisted Extraction of Asiaticoside from Centella Asiatica Using Betaine-Based Natural Deep Eutectic Solvent. Ind. Crops Prod. 2023, 192, 116069. DOI: 10.1016/j.indcrop.2022.116069.
- Viñas-Ospino, A.; Panić, M.; Bagović, M.; Radošević, K.; Esteve, M. J.; Radojčić Redovniković, I. Green Approach to Extract Bioactive Compounds from Orange Peel Employing Hydrophilic and Hydrophobic Deep Eutectic Solvents. Sustain. Chem. Pharm. 2023, 31, 100942. DOI: 10.1016/j.scp.2022.100942.
- Jiang, Z. M.; Wang, L. J.; Gao, Z.; Zhuang, B.; Yin, Q.; Liu, E. H. Green and Efficient Extraction of Different Types of Bioactive Alkaloids Using Deep Eutectic Solvents. Microchem. J. 2019, 145, 345–353. DOI: 10.1016/j.microc.2018.10.057.