Abstract
This scoping review aimed to synthesize the analytical techniques used and methodological limitations encountered when undertaking secondary research using residual neonatal dried blood spot (DBS) samples. Studies that used residual neonatal DBS samples for secondary research (i.e. research not related to newborn screening for inherited genetic and metabolic disorders) were identified from six electronic databases: Cochrane Library, Cumulative Index to Nursing and Allied Health Literature (CINAHL), Embase, Medline, PubMed and Scopus. Inclusion was restricted to studies published from 1973 and written in or translated into English that reported the storage, extraction and testing of neonatal DBS samples. Sixty-seven studies were eligible for inclusion. Included studies were predominantly methodological in nature and measured various analytes, including nucleic acids, proteins, metabolites, environmental pollutants, markers of prenatal substance use and medications. Neonatal DBS samples were stored over a range of temperatures (ambient temperature, cold storage or frozen) and durations (two weeks to 40.5 years), both of which impacted the recovery of some analytes, particularly amino acids, antibodies and environmental pollutants. The size of DBS sample used and potential contamination were also cited as methodological limitations. Residual neonatal DBS samples retained by newborn screening programs are a promising resource for secondary research purposes, with many studies reporting the successful measurement of analytes even from neonatal DBS samples stored for long periods of time in suboptimal temperatures and conditions.
Introduction
Neonatal dried blood spot (DBS) screening is routinely carried out within the first few days following birth to detect inherited disorders, including sickle cell disease, cystic fibrosis and congenital hypothyroidism, along with inborn errors of metabolism, such as phenylketonuria (PKU) [Citation1]. Early detection of these serious, but potentially treatable disorders, enables treatment to be commenced before symptom-onset [Citation2]. The process was first described in the early 1960s, when Robert Guthrie demonstrated that phenylalanine concentrations could be measured in neonates using a heel prick blood sample dried on standardized filter paper, providing a simple and convenient diagnostic tool for PKU [Citation3]. Developed countries across the world have since adopted this methodology, establishing comprehensive neonatal screening programs capable of screening for over 50 conditions [Citation1,Citation4].
Following their initial use for screening, neonatal DBS samples are typically retained in storage for possible future clinical or quality control purposes [Citation5]. However, they are also a potential resource for research. Repositories of neonatal DBS samples represent demographically and geographically diverse populations that are useful resources for epidemiology and population-based studies [Citation6]. In contrast, adult cohorts are not typically representative of the general population due to selection and survival bias [Citation7]. Analysis of residual neonatal DBS samples therefore offers a unique opportunity to capture and assess the effects of early life exposures in all individuals, including those who die early and “hard to reach” groups.
The retention and use of residual neonatal DBS samples is subject to ethical and legal considerations, particularly surrounding parental and individual consent [Citation8]. In Denmark, residual neonatal DBS samples can be legally stored without explicit informed consent. However, later use for secondary research purposes is subject to parental consent, although this requirement may be waived by the Research Ethics Committee if the samples are anonymized [Citation9]. Meanwhile, many states in the United States of America (USA) have implemented consent processes for the retention and use of neonatal DBS samples for research purposes, following a lawsuit in Texas that saw five million archived neonatal DBS samples destroyed on the grounds of violating constitutional privacy rights [Citation6]. Nevertheless, public support for the use of DBS samples for secondary research purposes appears high, with the proportion of individuals opting out of DBS sample storage reported to be less than 0.1% in Denmark [Citation9].
A scoping review, published in 2019, reported that residual neonatal DBS samples have been a well-utilized resource for secondary research, with the number of published studies increasing annually [Citation10]. The review further reported that the secondary research uses extended beyond the diseases included in current neonatal screening programs, with analytes, deoxyribonucleic acid (DNA) and enzymes being the main target categories of investigation. However, there is currently no synthesis of the methods that have been used to analyze residual neonatal DBS samples for secondary research purposes (unrelated to newborn screening programs) and their level of success. This information is essential to inform the future storage and use of samples. Therefore, the objectives of this scoping review of the published literature were to ascertain the storage, extraction and testing methodologies used, the analytes measured and any limitations encountered when using residual neonatal DBS samples for secondary research purposes.
Methods
A scoping review of published studies was conducted. The scoping review adhered to the Preferred Reporting Items for Systematic Reviews and Meta-analyses extension for Scoping Reviews (PRISMA-ScR) guidelines [Citation11]. Six electronic databases were searched: Cochrane Library (Wiley; 1996-present), Cumulative Index to Nursing and Allied Health (CINAHL; EBSCOhost; 1981-present), Embase (Ovid; 1947-present), Medline (Ovid; 1966-present), PubMed (United States National Library of Medicine; 1996-present) and Scopus (Elsevier; 1996-present) on 30 August 2023. Databases were searched individually using an adapted search strategy to account for differing subject index terms and keywords used across databases. Variations of search terms used covered the following key concepts: (1) dried bloodspots, Guthrie cards or heel prick tests and (2) archived, residual, stored or biobank samples and (3) newborn, neonates or infants. Advanced search features, including multi-field searches, operators, truncations/wildcards, limits and Boolean terms were used as appropriate. Different spellings, keyword variations, synonyms and related concepts were included to ensure that the search was comprehensive. The full search strategy, including the search terms used for each electronic database, can be found in Supplemental File S1.
Original studies of any design were eligible for inclusion if they described the storage, extraction or testing methods for conducting secondary research using neonatal DBS samples; were written in or translated into English; involved human participants only and were published as full manuscripts from 1973 onwards. Studies were excluded if they were conference abstracts; focused solely on newborn screening, quality improvement in newborn screening or extensions of newborn screening programs (e.g. improving existing screening tests or developing new screening tests) or focused on the immediate diagnosis or initiation of treatment for neonatal conditions. Studies were also excluded if they focused solely on legal, social or ethical issues relating to the use of DBS samples, such as data protection, consent/permission, policy or public opinions; used only samples obtained from umbilical cords or via venipuncture or DBS samples obtained solely from adults or animals.
Following the database searches, records were downloaded to EndNote 21 (Bld 17096, Clarivate) and duplicate records removed. The eligibility of all remaining studies was assessed using the literature review software, DistillerSR (DistillerSR Inc., Ontario, Canada). All studies were screened in duplicate against the predefined eligibility criteria by two independent reviewers (JC and RJS) during both the title/abstract and full text screening stages. Reviewers (JC and RJS) adopted a “Yes-No-Unclear” approach when determining if studies met each eligibility criterion, as recommended by the Joanna Briggs Institute (JBI) framework for scoping reviews [Citation12]. Thus, studies in which the inclusion/exclusion criteria could not be determined from the title and abstract alone (deemed “Unclear”) were included in the full text screening stage for consideration. Disagreements/discrepancies between reviewers were resolved through discussion, with a third reviewer available for further consultation, if required. The level of agreement between reviewers (JC and RJS) was >90% for both title/abstract and full text screening levels. Consultation with a third member of the review team was not required.
The information extracted from eligible studies included the study location and design; storage of DBS samples (duration, temperature and humidity), number and size of DBS samples used in the analyses; the analytes measured and the purpose of measuring them; the laboratory methods used; any reported DBS-specific limitations relating to methods and key study conclusions.
Results
A total of 2,877 studies were identified from the electronic databases. Following the removal of 1,679 duplicates, 1,198 studies underwent title and abstract screening, with 1,061 of these studies excluded for failing to meet the eligibility criteria. Full-text articles were therefore screened for the remaining 137 studies and 67 of these studies were deemed eligible for inclusion in the scoping review () [Citation13–79].
Figure 1. PRISMA 2020 flow diagram.
From: Page MJ, McKenzie JE, Bossuyt PM, et al. The PRISMA 2020 statement: an updated guideline for reporting systematic reviews. BMJ. 2021;372:n71. doi: 10.1136/bmj.n71.
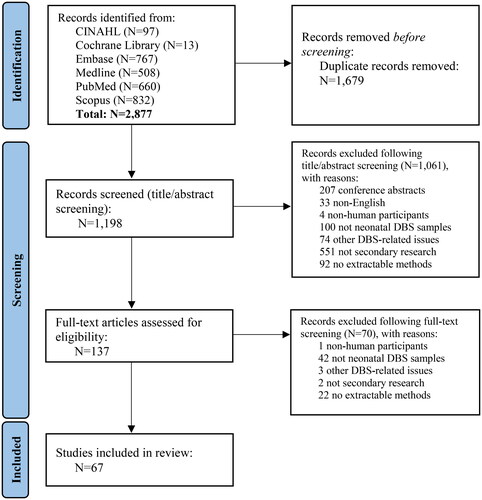
Included studies were published between 1993 and 2023 (). Thirty-one were conducted in the USA [Citation13–16,Citation22,Citation24,Citation28,Citation29,Citation36,Citation37,Citation40,Citation42,Citation44,Citation45,Citation48–52,Citation54–58,Citation63,Citation66,Citation68,Citation69,Citation71,Citation72,Citation77], 16 in Denmark [Citation18,Citation20,Citation25,Citation27,Citation31–34,Citation39,Citation46,Citation61,Citation62,Citation67,Citation74–76], five in Sweden [Citation19,Citation23,Citation30,Citation41,Citation59], five in the UK [Citation17,Citation26,Citation35,Citation43,Citation65], three in Canada [Citation38,Citation64,Citation70], two in Italy [Citation53,Citation78], two in The Netherlands [Citation60,Citation79] and one each in Hungary [Citation73], Japan [Citation47] and Thailand [Citation21]. All neonatal DBS samples used in studies were sourced from the country of study origin, apart from two USA-based studies that used neonatal DBS samples from both the USA and Australia [Citation44,Citation45] and a study based in Sweden that used samples sourced from both Sweden and Denmark [Citation59]. The number of individuals from whom DBS samples were collected ranged from one to 77,639, with the number of individuals not clearly specified in three studies [Citation13,Citation44,Citation45]. Studies were predominately methodological (N = 52) in nature [Citation13–24,Citation26–34,Citation37–47,Citation52–59,Citation63,Citation66,Citation67,Citation70–76,Citation78,Citation79], reporting either the development, validation or comparison of methods. Of the remainder, eight were case-control studies [Citation25,Citation35,Citation36,Citation48,Citation51,Citation61,Citation62,Citation65], six cohort studies [Citation49,Citation50,Citation60,Citation64,Citation68,Citation77] and one cross-sectional [Citation69].
Table 1. Key characteristics of included studies.
Storage conditions
The storage of neonatal DBS samples was highly variable across included studies, with the duration of storage ranging from two weeks to over 40 years (). Neonatal DBS samples were stored solely at room temperature in 28 studies [Citation13–17,Citation21–24,Citation26,Citation35,Citation38,Citation43–45,Citation48,Citation50,Citation53,Citation55,Citation56,Citation63–66,Citation70,Citation72,Citation73,Citation79] and in cold storage (4 °C) in eight [Citation19,Citation30,Citation41,Citation51,Citation57,Citation58,Citation69,Citation78]. Twenty-four studies used frozen DBS samples [Citation18,Citation25,Citation29,Citation31–34,Citation38–40,Citation42,Citation46,Citation47,Citation49,Citation52,Citation54,Citation61,Citation62,Citation67,Citation68,Citation71,Citation74–76], with temperatures ranging from −20 °C to −80 °C. Two studies did not state the exact frozen temperature [Citation42,Citation54]. Storage conditions in three studies changed due to updated storage policies in the respective biobank facilities [Citation28,Citation59,Citation60]. This largely involved moving samples to colder conditions and controlling humidity. Four studies compared the effects of storage temperature and duration by comparing frozen and room temperature-stored samples () [Citation20,Citation27,Citation36,Citation77]. Humidity control was inconsistently reported across studies, with the majority of studies not reporting this aspect. Two studies simply stated that conditions were “humidity-controlled” [Citation19,Citation77], four reported the use of a desiccant to control humidity [Citation36,Citation71,Citation72,Citation78] and three studies provided humidity levels which ranged from <30% to 70% [Citation21,Citation28,Citation59].
Table 2. Summary of methods used in included studies.
Extraction
The number and size of DBS samples used for testing varied. Six studies reported using one or more full DBS samples in their analyses [Citation36,Citation54,Citation56,Citation61,Citation65,Citation79] but the majority of studies used only part of the DBS sample. Most punched between one and 12 disks with diameters ranging from 1 mm to 16 mm () [Citation13–35,Citation37–47,Citation49,Citation50,Citation52,Citation53,Citation57,Citation58,Citation60,Citation62–64,Citation67–78]. One study reported using half the DBS sample [Citation55] and two studies reported using one-quarter [Citation51,Citation66]. Two studies did not specify the number or size of DBS samples used [Citation48,Citation59].
Analytes
Of the 67 studies, 37 measured nucleic acids and nucleic acid types (). Fourteen measured DNA [Citation13,Citation22,Citation24,Citation25,Citation27,Citation28,Citation44,Citation45,Citation47,Citation70–73,Citation77], eight whole genome amplified DNA (wgaDNA) [Citation30–34,Citation74–76], six RNA [Citation14,Citation15,Citation18–20,Citation79], four genomic DNA (gDNA) [Citation21,Citation23,Citation26,Citation29], three methylated DNA [Citation42,Citation43,Citation46], one messenger RNA (mRNA) [Citation16] and one microRNA (miRNA) [Citation17]. These were measured for a variety of purposes, including DNA amplification, gene expression, genotyping, DNA methylation, DNA quantification, DNA sequencing, telomere length measurement and transcriptome analysis. Two studies measured maternally-derived antibodies; one against Group B Streptococcus [Citation35] and the other against Toxoplasma Gondii [Citation36]. Metabolomics were used in five studies to measure a range of 39–1,107 metabolites [Citation37–41]. Six studies measured markers of prenatal exposure to substance use, including alcohol (phosphatidylethanol; PEth) [Citation48,Citation49], cocaine (benzoylecgonine) [Citation50] and tobacco (cotinine [Citation37,Citation51,Citation52] and Cys34 human serum albumin (HSA) adducts [Citation68]). A further six studies measured analytes indicative of prenatal exposure to environmental pollutants, including lead [Citation53,Citation55], hemoglobin adducts of benzene oxide [Citation54], arsenic [Citation55], mercury [Citation55], cadmium [Citation55], polyfluoroalkyl chemicals (PFCs) [Citation56,Citation58] and iodide [Citation57]. A variety of proteins were measured across studies, including oncology-related proteins [Citation59], amino acids [Citation37,Citation41,Citation60], cytokines/hormones [Citation61,Citation63,Citation64,Citation67,Citation69], ferritin [Citation65] and unspecified proteins [Citation62,Citation66]. The antibiotics, piperacillin and tazobactam, were also measured in one study for therapeutic drug monitoring purposes [Citation78]. Studies were largely able to measure the analytes of interest using the methods described (), although a number of studies noted lower quantity and quality of some analytes as a result of the storage methods used ().
Table 3. Summary of analytes successfully measured using neonatal dried blood spot (DBS) samples stored at varying temperatures and their maximum duration of storage as reported in the included studies.
DNA and RNA appeared to be stable in neonatal DBS samples stored at differing temperatures for long periods of time, with storage at −20 °C seemingly improving the preservation of samples [Citation20,Citation27,Citation77]. Proteins were shown to remain detectable after prolonged periods of storage, but storage temperature significantly impacted detectability. Proteins were better preserved when DBS samples were stored frozen, while storage at 4 °C resulted in the degradation of many proteins [Citation59]. Amino acids stored at room temperature were subject to substantial degradation within one year of storage (although aspartate appeared to be stable for four years) [Citation60], while degradation was minimal for amino acids stored at −20 °C [Citation37]. Cytokines were measurable in samples stored at room temperature for ten years [Citation64] and frozen samples for 20 years and appeared to be well preserved [Citation67]. Inflammatory markers and neurotrophins were well preserved and detectable in equal concentrations over time in frozen samples stored for longer than 20 years [Citation67]. Ferritin recovery was low from samples stored at room temperature for more than six months, with expected recovery of ferritin from samples stored for longer periods at room temperature estimated to be around 25% [Citation65]. Adipokines (adiponectin, resistin and adipsin, but not leptin) were measurable in cold storage samples but levels differed from previously reported levels using cord or venous blood [Citation69]. Adiponectin, leptin and insulin were measurable in samples stored at room temperature for up to six months, with similar levels to those observed in serum samples [Citation63]. Antibodies stored at room temperature were subject to degradation within months but may be better preserved for longer when samples are stored at −20 °C [Citation35,Citation36].
Storage of samples at −20 °C was shown to protect against the deterioration of most metabolites studied for almost 30 years, although lipid metabolites appeared less stable [Citation37]. For samples stored at room temperature, the largest changes in metabolite levels were observed after 12 months [Citation38]. Untargeted metabolomics of DBS samples stored for long periods appeared feasible, with the majority of DBS metabolomes stable for up to ten [Citation39] and 30 [40] years if stored at −20 °C. In studies assessing prenatal environmental pollutant exposure, lead was measurable in samples stored at room temperature for up to six months but needed to be extracted from DBS samples in the presence of optimal amounts of EDTA (ethylenediaminetetraacetic acid) [Citation53]. In another study, lead and cadmium (but not mercury) were detectable in samples stored at room temperature for over ten years but arsenic concentrations were low, with potential contamination during blood sample collection and storage noted as an issue [Citation55]. Hemoglobin adducts of benzene oxide, a marker of exposure to the air pollutant benzene, were measurable in neonatal DBS samples after one month of frozen storage [Citation54]. The levels measured were comparable to adult DBS samples and recovery did not appear to decrease over time, although contamination may again be an issue and no longer-term studies of stability were found. Iodide was found to be stable in samples stored at 4 °C for up to eight years [Citation57], although no studies assessing iodide in samples stored frozen or at room temperature were found. PFC extraction was possible using samples stored at room temperature for up to two years but was dependent on the extraction and amplification procedures used [Citation56]. The extraction method used for samples stored at 4 °C for up to five years was deemed reliable but dependent on the number and size of DBS samples used. Background levels of contamination may also cause issues with accurate quantification [Citation58].
A number of studies used neonatal DBS samples to detect prenatal exposures to substances consumed or used by the mother, such as alcohol and tobacco. PEth (a direct ethanol metabolite that can be used to detect prenatal alcohol exposure) was detectable in samples stored at room temperature for two weeks [Citation48] and in samples stored at −80 °C for four months [Citation49], with insignificant degradation noted. However, the stability of PEth in samples stored at these temperatures for longer durations remains unknown. Cotinine and hydroxycotinine (markers of maternal smoking) remained detectable in frozen samples stored for up to 30 years [Citation37]. Measurement of these markers of tobacco was found to be reliable but may be affected by the amount of DBS sample used and background levels of contamination [Citation51,Citation52]. Cotinine stability in DBS samples stored long-term at room temperature or 4 °C is not known, although another study demonstrated that methylated DNA extracted from room temperature samples stored for over 30 years, could be used to indicate maternal smoking [Citation43]. Cys34 HSA adducts (used to investigate in-utero exposure to smoking in the month prior to birth) were measurable in samples stored at −20 °C for over 30 years [Citation68], although it was reported that quantification of Cys34 HSA adducts was harder when hemoglobin was oxidized at room temperature. Samples stored at room temperature showed no decrease in the direct cocaine metabolite, benzoylecgonine, when measured over 30 days [Citation50]. However, no studies assessing long-term stability were found. Finally, the antibiotics, piperacillin and tazobactam, were stable in samples stored at −20 °C but rapid degradation occurred at room temperature [Citation78].
Comparisons
A total of nine studies compared methods used on neonatal DBS samples () [Citation20,Citation23,Citation24,Citation31,Citation33,Citation34,Citation44,Citation45,Citation53]. These studies predominately compared the use of commercially available kits with or without modifications to the available protocol. Such modifications typically improved the efficiency of the method and were recommended for use in future studies. Full descriptions of the methods that were compared along with the results and conclusions/recommendations are contained in .
Table 4. Summary of studies directly comparing methods used on neonatal dried blood spot (DBS) samples.
Limitations
Methodological limitations relating to the use of DBS samples were noted for each study (). Contamination of samples may occur during sample collection, transportation, storage or handling and was a noted limitation in a number of studies. Depending on the analytes being measured, contaminants included DNA [Citation14,Citation70], RNAases [Citation17,Citation79], lipids [Citation37], proteins [Citation13,Citation67], volume of red blood cells (hematocrit) [Citation13,Citation37,Citation67,Citation78] and microbial or viral content [Citation70], although studies largely did not speculate as to the potential sources of contamination. The number and size of DBS samples used further restricted investigations or outputs in a number of studies [Citation18,Citation28,Citation31,Citation33,Citation34,Citation36,Citation41,Citation62,Citation65,Citation74,Citation77]. This was due to finite availability of DBS samples or restrictions imposed by biobank facilities concerning the number of samples/punches that could be used for secondary research purposes. The volume of blood spotted onto DBS samples is largely unknown and may have varied between samples due to DBS size, punch location and filter paper type [Citation41,Citation51,Citation57,Citation58]. Storage duration and conditions were further noted as limitations in included studies. Suboptimal conditions, along with long storage times, may impact the viability of DBS samples and/or recovery of analytes due to degradation [Citation27,Citation32,Citation35,Citation37–39,Citation64,Citation66,Citation68,Citation69]. Results may also be impacted by direct limitations of the methods applied (for example, some methods may be less sensitive when measuring certain analytes), along with a lack of appropriate standards and systematic quality control for sample preparation and assessment [Citation23,Citation69,Citation75].
Discussion
Summary of evidence
This scoping review is the first evidence synthesis, to our knowledge, covering the methodological designs that have been successfully used on residual neonatal DBS samples for secondary research purposes. We have presented the approaches used in the storage, extraction and testing of residual neonatal DBS samples, along with the analytes measured and the limitations encountered, as reported in the published literature. As expected, the methodological designs were highly variable across the included studies. The duration and temperature of neonatal DBS sample storage were notable factors in the detectability of many analytes, with studies emphasizing the need to consider this when choosing optimal methods for planned analyses, particularly when using samples stored for extended periods of time in suboptimal conditions. Contamination, particularly from endogenous components, was a further concern when performing analyses using neonatal DBS samples and appropriate corrections should be made where possible, along with use of quality assurance measures. Further investigation is required to assess the long-term stability of certain analytes, such as those reflecting substance use and environmental pollutant exposure during pregnancy. Additional validation studies using neonatal DBS samples may also be required. For example, the long-term preservation of RNA in neonatal DBS samples stored at room temperature was a particularly intriguing finding given the known instability of RNA. Nevertheless, this review presents encouraging evidence supporting the use of residual neonatal DBS samples for a range of secondary research purposes, with potential for high throughput omics analyses (including DNA, methylation, RNA and proteins) and methods that are scalable to population-level datasets for molecular epidemiology.
Whilst recognizing that issues of consent and access approval for research purposes are yet to be resolved in many jurisdictions [Citation80], using residual neonatal DBS samples for research has a number of advantages. Compared with venous and cord blood samples, DBS samples are more easily transported and stored [Citation81]. Secondary use of neonatal DBS samples collected for screening obviates the need for recruitment and provides large-scale, unselected sampling frames which are continually being expanded. As analytical techniques continue to improve, along with updated biobank policies regarding the optimal storage of samples, analytes may be measurable with high throughput and increased specificity and sensitivity [Citation82], further expanding the scope of research that can be performed. Finally, the linkage of stored DBS samples and healthcare registries would provide resources for studies, including case series, case-control and retrospective cohort studies, to address research questions relating to epidemiology, genotype/phenotype interactions and treatment outcomes, with the potential to inform future policy and practice [Citation83].
Limitations
Due to the broad nature of this scoping review and the breadth of related literature, some articles may have been missed during the search process. However, efforts were made to minimize the risk of unfound articles, such as searching multiple databases using a comprehensive search strategy to identify additional relevant studies, as well as members of the review team performing article screening in duplicate. Likewise, the depth of analysis was limited due to the broad focus of this scoping review that resulted in a large number of included studies. Similarly, this review did not assess risk of bias or quality in the included studies, which is a limitation of scoping reviews in general. Studies were also limited to those published in the English language, although a systematic literature review published in 2012, reported no evidence of systematic bias as a result of English-language restrictions [Citation84].
This scoping review also searched for studies published from 1973 onwards, meaning that relevant studies prior to this year may have been missed. However, newborn screening for inherited inborn errors of metabolism and other metabolic disorders was in its infancy in the 1950s and 1960s, with particular focus on testing for PKU [Citation85,Citation86]. Subsequent neonatal DBS-related research predominately focused on adding conditions to newborn screening panels [Citation87], with the World Health Organization (WHO) commissioning a report in 1968 to develop screening criteria to guide and evaluate the selection of conditions for screening [Citation88]. This focus on the expansion of newborn screening programs may explain the lack of eligible studies prior to 1993 in this scoping review, which was interested in secondary research (unrelated to newborn screening) using neonatal DBS samples. Another reason for the inclusion of studies that were newer in origin may be that advances in methodologies, e.g. the introduction of tandem mass spectrometry in the early 1990s, increased the use of neonatal DBS samples for secondary research purposes by broadening what was technologically possible at this time [Citation85].
The non-reporting of failed experiments and publication bias may also be an issue, in that failed attempts at measuring specific analytes of interest may not have been reported. Delays in sending samples for analysis or in performing clinical testing itself (which remains the priority of neonatal DBS sample collection), may have also impacted the reported storage durations, with such delays unlikely to be reported in studies. Nevertheless, we have attempted to collate the results of studies to demonstrate the feasibility of measuring analytes of interest which may act as an important first step in the standardization of neonatal DBS methodologies for secondary research purposes.
Conclusions
Neonatal DBS samples have been used successfully to measure a variety of analytes for different purposes. Storage duration, temperature and potential contamination limit some potential uses of DBS samples and should be considered when planning research studies and selecting appropriate methods.
Abbreviations | ||
DBS | = | Dried blood spot |
DNA | = | Deoxyribonucleic acid |
gDNA | = | Genomic deoxyribonucleic acid |
HSA | = | Human serum albumin |
mRNA | = | Messenger ribonucleic acid |
miRNA | = | Micro ribonucleic acid |
PEth | = | Phosphatidylethanol |
PFC | = | Polyfluoroalkyl chemical |
PKU | = | Phenylketonuria |
RNA | = | Ribonucleic acid |
USA | = | United States of America |
wgaDNA | = | Whole genome amplified deoxyribonucleic acid |
Supplemental_File_1_finaledits.docx
Download MS Word (16.6 KB)Disclosure statement
JC, RJS, ZM, DJP, CLMS, RC, SP and JPP declare no relevant competing interests. REM is a scientific advisor to Optima Partners and the Epigenetic Clock Development Foundation. MM is a co-founder of Mirvie Inc. (San Fransisco) that creates precise, actionable, noninvasive tests for maternal-fetal health. MEH is a founder of, director of, consultant to and holds shares in Congenica Ltd. and is a consultant to the AstraZeneca Centre for Genomics Research. NS has consulted for and/or received speaker honoraria from Abbott Laboratories, Abbvie, Amgen, AstraZeneca, Boehringer Ingelheim, Eli Lilly, Hanmi Pharmaceuticals, Janssen, Menarini-Ricerche, Novartis, Novo Nordisk, Pfizer, Roche Diagnostics and Sanofi, and received grant support paid to his University from AstraZeneca, Boehringer Ingelheim, Novartis and Roche Diagnostics outside the submitted work.
Additional information
Funding
References
- Therrell BL, Padilla CD, Loeber JG, et al. Current status of newborn screening worldwide: 2015. Semin Perinatol. 2015;39(3):171–187. doi: 10.1053/j.semperi.2015.03.002.
- Wilcken B, Wiley V. Newborn screening. Pathology. 2008;40(2):104–115. doi: 10.1080/00313020701813743.
- Guthrie R, Susi A. A simple phenylalanine method for detecting phenylketonuria in large populations of newborn infants. Pediatrics. 1963;102(1):236–237. doi: 10.1542/peds.32.3.338.
- Benkendorf J, Goodspeed T, Watson MS. Newborn screening residual dried blood spot use for newborn screening quality improvement. Genet Med. 2010;12(12 Suppl):S269–S272. doi: 10.1097/GIM.0b013e3181fea489.
- Caggana M, Jones EA, Shahied SI, et al. Newborn screening: from guthrie to whole genome sequencing. Public Health Rep. 2013;128(Suppl 2):14–19. doi: 10.1177/00333549131280S204.
- Botkin JR, Goldenberg AJ, Rothwell E, et al. Retention and research use of residual newborn screening bloodspots. Pediatrics. 2013;131(1):120–127. doi: 10.1542/peds.2012-0852.
- Kingston A, Jagger C. Review of methodologies of cohort studies of older people. Age Ageing. 2018;47(2):215–219. doi: 10.1093/ageing/afx183.
- Tarini BA. Storage and use of residual newborn screening blood spots: a public policy emergency. Genet Med. 2011;13(7):619–620. doi: 10.1097/GIM.0b013e31822176df.
- Hougaard DM, Bybjerg-Grauholm J, Christiansen M, et al. Response to “newborn dried blood spot samples in Denmark: the hidden figures of secondary use and research participation. Eur J Hum Genet. 2019;27(11):1625–1627. doi: 10.1038/s41431-019-0437-y.
- Rothwell E, Johnson E, Riches N, et al. Secondary research uses of residual newborn screening dried bloodspots: a scoping review. Genet Med. 2019;21(7):1469–1475. doi: 10.1038/s41436-018-0387-8.
- Tricco AC, Lillie E, Zarin W, et al. PRISMA extension for scoping reviews (PRISMA-ScR): checklist and explanation. Ann Intern Med. 2018;169(7):467–473. doi: 10.7326/M18-0850.
- Peters MDJ, Casey M, Tricco AC, et al. Updated methodological guidance for the conduct of scoping reviews. JBI Evid Implement. 2021;19(1):3–10. doi: 10.1097/XEB.0000000000000277.
- Makowski GS, Davis EL, Hopfer SM. The effect of storage on Guthrie cards: implications for deoxyribonucleic acid amplification. Ann Clin Lab Sci. 1996;26(5):458–469.
- Haak PT, Busik JV, Kort EJ, et al. Archived unfrozen neonatal blood spots are amenable to quantitative gene expression analysis. Neonatology. 2009;95(3):210–216. doi: 10.1159/000155652.
- Resau JH, Ho NT, Dykema K, et al. Evaluation of sex-specific gene expression in archived dried blood spots (DBS). Int J Mol Sci. 2012;13(8):9599–9608. doi: 10.3390/ijms13089599.
- Slaughter J, Wei C, Korzeniewski SJ, et al. High correlations in gene expression between paired umbilical cord blood and neonatal blood of healthy newborns on Guthrie cards. J Matern Fetal Neonatal Med. 2013;26(18):1765–1767. doi: 10.3109/14767058.2013.804050.
- Ponnusamy V, Kapellou O, Yip E, et al. A study of microRNAs from dried blood spots in newborns after perinatal asphyxia: a simple and feasible biosampling method. Pediatr Res. 2016;79(5):799–805. doi: 10.1038/pr.2015.276.
- Bybjerg-Grauholm J, Hagen CM, Khoo SK, et al. RNA sequencing of archived neonatal dried blood spots. Mol Genet Metab Rep. 2016;10:33–37. doi: 10.1016/j.ymgmr.2016.12.004.
- Gauffin F, Nordgren A, Barbany G, et al. Quantitation of RNA decay in dried blood spots during 20 years of storage. Clin Chem Lab Med. 2009;47(12):1467–1469. doi: 10.1515/CCLM.2009.351.
- Grauholm J, Khoo SK, Nickolov RZ, et al. Gene expression profiling of archived dried blood spot samples from the Danish neonatal screening biobank. Mol Genet Metab. 2015;116(3):119–124. doi: 10.1016/j.ymgme.2015.06.011.
- Chaisomchit S, Wichajarn R, Janejai N, et al. Stability of genomic DNA in dried blood spots stored on filter paper. Southeast Asian J Trop Med Public Health. 2005;36(1):270–273.
- Hardin J, Finnell RH, Wong D, et al. Whole genome microarray analysis, from neonatal blood cards. BMC Genet. 2009;10(1):38. doi: 10.1186/1471-2156-10-38.
- Klassen TL, Drabek J, Tomson T, et al. Visual automated fluorescence electrophoresis provides simultaneous quality, quantity, and molecular weight spectra for genomic DNA from archived neonatal blood spots. J Mol Diagn. 2013;15(3):283–290. doi: 10.1016/j.jmoldx.2013.01.003.
- Lane JA, Noble JA. Maximizing deoxyribonucleic acid yield from dried blood spots. J Diabetes Sci Technol. 2010;4(2):250–254. doi: 10.1177/193229681000400204.
- Pedersen CB, Bybjerg-Grauholm J, Pedersen MG, et al. The iPSYCH2012 case-cohort sample: new directions for unravelling genetic and environmental architectures of severe mental disorders. Mol Psychiatry. 2018;23(1):6–14. doi: 10.1038/mp.2017.196.
- Rajatileka S, Luyt K, El-Bokle M, et al. Isolation of human genomic DNA for genetic analysis from premature neonates: a comparison between newborn dried blood spots, whole blood and umbilical cord tissue. BMC Genet. 2013;14(1):105. doi: 10.1186/1471-2156-14-105.
- Sjöholm MI, Dillner J, Carlson J. Assessing quality and functionality of DNA from fresh and archival dried blood spots and recommendations for quality control guidelines. Clin Chem. 2007;53(8):1401–1407. doi: 10.1373/clinchem.2007.087510.
- Sok P, Lupo PJ, Richard MA, et al. Utilization of archived neonatal dried blood spots for genome-wide genotyping. PLoS One. 2020;15(2):e0229352. doi: 10.1371/journal.pone.0229352.
- St Julien KR, Jelliffe-Pawlowski LL, Shaw GM, et al. High quality genome-wide genotyping from archived dried blood spots without DNA amplification. PLoS One. 2013;8(5):e64710. doi: 10.1371/journal.pone.0064710.
- Hannelius U, Lindgren CM, Melén E, et al. Phenylketonuria screening registry as a resource for population genetic studies. J Med Genet. 2005;42(10):e60–e60. doi: 10.1136/jmg.2005.032987.
- Hollegaard MV, Grauholm J, Børglum A, et al. Genome-wide scans using archived neonatal dried blood spot samples. BMC Genomics. 2009a;10(1):297. doi: 10.1186/1471-2164-10-297.
- Hollegaard MV, Grove J, Thorsen P, et al. High-throughput genotyping on archived dried blood spot samples. Genet Test Mol Biomarkers. 2009b;13(2):173–179. doi: 10.1089/gtmb.2008.0073.
- Hollegaard MV, Thorsen P, Norgaard-Pedersen B, et al. Genotyping whole-genome-amplified DNA from 3- to 25-year-old neonatal dried blood spot samples with reference to fresh genomic DNA. Electrophoresis. 2009c;30(14):2532–2535. doi: 10.1002/elps.200800655.
- Hollegaard MV, Grove J, Grauholm J, et al. Robustness of genome-wide scanning using archived dried blood spot samples as a DNA source. BMC Genet. 2011;12(1):58. doi: 10.1186/1471-2156-12-58.
- Auma E, Hall T, Chopra S, et al. Using dried blood spots for a Sero-Surveillance study of maternally derived antibody against group B Streptococcus. Vaccines (Basel). 2023;11(2):357. doi: 10.3390/vaccines11020357.
- Mei JV, Li L, Rasmussen SA, et al. Effect of specimen storage conditions on newborn dried blood spots used to assess Toxoplasma gondii immunoglobulin M (IgM). Clin Chim Acta. 2011;412(5-6):455–459. doi: 10.1016/j.cca.2010.11.028.
- He D, Yan Q, Uppal K, et al. Metabolite stability in archived neonatal dried blood spots used for epidemiological research. Am J Epidemiol. 2023;192(10):1720–1730. doi: 10.1093/aje/kwad122.
- Murphy MSQ, Hawken S, Cheng W, et al. Metabolic profiles derived from residual blood spot samples: a longitudinal analysis. Gates Open Res. 2018;2:28. doi: 10.12688/gatesopenres.
- Ottosson F, Russo F, Abrahamsson A, et al. Effects of long-term storage on the biobanked neonatal dried blood spot metabolome. J Am Soc Mass Spectrom. 2023;34(4):685–694. doi: 10.1021/jasms.2c00358.
- Petrick L, Edmands W, Schiffman C, et al. An untargeted metabolomics method for archived newborn dried blood spots in epidemiologic studies. Metabolomics. 2017;13(3):27. doi: 10.1007/s11306-016-1153-z.
- Yu M, Dolios G, Yong-Gonzalez V, et al. Untargeted metabolomics profiling and hemoglobin normalization for archived newborn dried blood spots from a refrigerated biorepository. J Pharm Biomed Anal. 2020;191:113574. doi: 10.1016/j.jpba.2020.113574.
- Asrani K, Shaw GM, Rine J, et al. DNA methylome profiling on the infinium HumanMethylation450 array from limiting quantities of genomic DNA from a single, small archived bloodspot. Genet Test Mol Biomarkers. 2017;21(8):516–519. doi: 10.1089/gtmb.2017.0019.
- Cunningham-Burley S, McCartney DL, Campbell A, et al. Feasibility and ethics of using data from the scottish newborn blood spot archive for research. Commun Med (Lond). 2022;2(1):126. doi: 10.1038/s43856-022-00189-2.
- Ghantous A, Saffery R, Cros MP, et al. Optimized DNA extraction from neonatal dried blood spots: application in methylome profiling. BMC Biotechnol. 2014;14(1):60. doi: 10.1186/1472-6750-14-60.
- Ghantous A, Hernandez-Vargas H, Herceg Z. DNA methylation analysis from blood spots: increasing yield and quality for genome-wide and locus-specific methylation analysis. Methods Mol Biol. 2018;1708:605–619. doi: 10.1007/978-1-4939-7481-8_31.
- Hollegaard MV, Grauholm J, Nørgaard-Pedersen B, et al. DNA methylome profiling using neonatal dried blood spot samples: a proof-of-principle study. Mol Genet Metab. 2013a;108(4):225–231. doi: 10.1016/j.ymgme.2013.01.016.
- Zar Kyaw T, Yamaguchi S, Imai C, et al. The utility of post-test newborn blood spot screening cards for epigenetic association analyses: association between HIF3A methylation and birth weight-for-gestational age. J Hum Genet. 2019;64(8):795–801. doi: 10.1038/s10038-019-0621-5.
- Bakhireva LN, Leeman L, Savich RD, et al. The validity of phosphatidylethanol in dried blood spots of newborns for the identification of prenatal alcohol exposure. Alcohol Clin Exp Res. 2014;38(4):1078–1085. doi: 10.1111/acer.12349.
- Bakhireva LN, Shrestha S, Gutierrez HL, et al. Stability of phosphatidylethanol in dry blood spot cards. Alcohol Alcohol. 2016;51(3):275–280. doi: 10.1093/alcalc/agv120.
- Henderson LO, Powell MK, Hannon WH, et al. Radioimmunoassay screening of dried blood spot materials for benzoylecgonine. J Anal Toxicol. 1993;17(1):42–47. doi: 10.1093/jat/17.1.42.
- Spector LG, Hecht SS, Ognjanovic S, et al. Detection of cotinine in newborn dried blood spots. Cancer Epidemiol Biomarkers Prev. 2007;16(9):1902–1905. doi: 10.1158/1055-9965.
- Yang J, Pearl M, Jacob P, 3rd, et al. Levels of cotinine in dried blood specimens from newborns as a biomarker of maternal smoking close to the time of delivery. Am J Epidemiol. 2013;178(11):1648–1654. doi: 10.1093/aje/kwt182.
- Di Martino MT, Michniewicz A, Martucci M, et al. EDTA is essential to recover lead from dried blood spots on filter paper. Clin Chim Acta. 2004;350(1-2):143–150. doi: 10.1016/j.cccn.2004.07.019.
- Funk WE, Waidyanatha S, Chaing SH, et al. Hemoglobin adducts of benzene oxide in neonatal and adult dried blood spots. Cancer Epidemiol Biomarkers Prev. 2008;17(8):1896–1901. doi: 10.1158/1055-9965.EPI-08-0356.
- Funk WE, McGee JK, Olshan AF, et al. Quantification of arsenic, lead, mercury and cadmium in newborn dried blood spots. Biomarkers. 2013;18(2):174–177. doi: 10.3109/1354750X.2012.750379.
- Kato K, Wanigatunga AA, Needham LL, et al. Analysis of blood spots for polyfluoroalkyl chemicals. Anal Chim Acta. 2009;656(1–2):51–55. doi: 10.1016/j.aca.2009.10.007.
- Kim UJ, Kannan K. Method for the determination of iodide in dried blood spots from newborns by high performance liquid chromatography tandem mass spectrometry. Anal Chem. 2018;90(5):3291–3298. doi: 10.1021/acs.analchem.7b04827.
- Ma W, Kannan K, Wu Q, et al. Analysis of polyfluoroalkyl substances and bisphenol a in dried blood spots by liquid chromatography tandem mass spectrometry. Anal Bioanal Chem. 2013;405(12):4127–4138. doi: 10.1007/s00216-013-6787-3.
- Björkesten J, Enroth S, Shen Q, et al. Stability of proteins in dried blood spot biobanks. Mol Cell Proteomics. 2017;16(7):1286–1296. doi: 10.1074/mcp.RA117.000015.
- Dijkstra AM, de Blaauw P, van Rijt WJ, et al. Important lessons on long-term stability of amino acids in stored dried blood spots. Int J Neonatal Screen. 2023;9(3):34. doi: 10.3390/ijns9030034.
- Klamer A, Skogstrand K, Hougaard DM, et al. Adiponectin levels measured in dried blood spot samples from neonates born small and appropriate for gestational age. Eur J Endocrinol. 2007;157(2):189–194. doi: 10.1530/EJE-06-0710.
- McGuire JN, Eising S, Wägner AM, et al. Screening newborns for candidate biomarkers of type 1 diabetes. Arch Physiol Biochem. 2010;116(4-5):227–232. doi: 10.3109/13813455.2010.501801.
- Mihalopoulos NL, Phillips TM, Slater H, et al. Validity and reliability of perinatal biomarkers of adiposity after storage as dried blood spots on paper. Am J Hum Biol. 2011;23(5):717–719. doi: 10.1002/ajhb.21199.
- Mineyko A, Nettel-Aguirre A, de Jesus P, et al. Association of neonatal inflammatory markers and perinatal stroke subtypes. Neurology. 2020;95(9):e1163–e1173. doi: 10.1212/WNL.0000000000010309.
- Raha-Chowdhury R, Moore CA, Bradley D, et al. Blood ferritin concentrations in newborn infants and the sudden infant death syndrome. J Clin Pathol. 1996;49(2):168–170. doi: 10.1136/jcp.49.2.168.
- Samenuk GM, Kelley AR, Perry G, et al. Rapid method towards proteomic analysis of dried blood spots by MALDI mass spectrometry. Clin Mass Spectrom. 2019;12:30–36. doi: 10.1016/j.clinms.2019.03.002.
- Skogstrand K, Thorsen P, Nørgaard-Pedersen B, et al. Simultaneous measurement of 25 inflammatory markers and neurotrophins in neonatal dried blood spots by immunoassay with xMAP technology. Clin Chem. 2005;51(10):1854–1866. doi: 10.1373/clinchem.2005.052241.
- Yano Y, Grigoryan H, Schiffman C, et al. Untargeted adductomics of Cys34 modifications to human serum albumin in newborn dried blood spots. Anal Bioanal Chem. 2019;411(11):2351–2362. doi: 10.1007/s00216-019-01675-8.
- Yeung EH, McLain AC, Anderson N, et al. Newborn adipokines and birth outcomes. Paediatr Perinat Epidemiol. 2015;29(4):317–325. doi: 10.1111/ppe.12203.
- Durie D, Yeh E, McIntosh N, et al. Quantification of DNA in neonatal dried blood spots by adenine tandem mass spectrometry. Anal Chem. 2018;90(1):801–806. doi: 10.1021/acs.analchem.7b03265.
- Bassaganyas L, Freedman G, Vaka D, et al. Whole exome and whole genome sequencing with dried blood spot DNA without whole genome amplification. Hum Mutat. 2018;39(1):167–171. doi: 10.1002/humu.23356.
- Ding Y, Owen M, Le J, et al. Scalable, high quality, whole genome sequencing from archived, newborn, dried blood spots. NPJ Genom Med. 2023;8(1):5. doi: 10.1038/s41525-023-00349-w.
- Nagy AL, Csáki R, Klem J, et al. Minimally invasive genetic screen for GJB2 related deafness using dried blood spots. Int J Pediatr Otorhinolaryngol. 2010;74(1):75–81. doi: 10.1016/j.ijporl.2009.10.021.
- Hollegaard MV, Grauholm J, Nielsen R, et al. Archived neonatal dried blood spot samples can be used for accurate whole genome and exome-targeted next-generation sequencing. Mol Genet Metab. 2013b;110(1-2):65–72. doi: 10.1016/j.ymgme.2013.06.004.
- Poulsen JB, Lescai F, Grove J, et al. High-quality exome sequencing of whole-genome amplified neonatal dried blood spot DNA. PLoS One. 2016;11(4):e0153253. doi: 10.1371/journal.pone.0153253.
- Winkel BG, Hollegaard MV, Olesen MS, et al. Whole-genome amplified DNA from stored dried blood spots is reliable in high resolution melting curve and sequencing analysis. BMC Med Genet. 2011;12(1):22. doi: 10.1186/1471-2350-12-22.
- Needham BL, Hicken MT, Govia IO, et al. Maternal social disadvantage and newborn telomere length in archived dried blood spots from the Michigan neonatal biobank. Biodemography Soc Biol. 2017;63(3):221–235. doi: 10.1080/19485565.2017.1300520.
- Barco S, Risso FM, Bruschettini M, et al. A validated LC-MS/MS method for the quantification of piperacillin/tazobactam on dried blood spot. Bioanalysis. 2014;6(21):2795–2802. doi: 10.4155/bio.14.205.
- Rovito R, Warnatz HJ, Kiełbasa SM, et al. Impact of congenital cytomegalovirus infection on transcriptomes from archived dried blood spots in relation to long-term clinical outcome. PLoS One. 2018;13(7):e0200652. doi: 10.1371/journal.pone.0200652.
- Cunningham S, O’Doherty KC, Sénécal K, et al. Public concerns regarding the storage and secondary uses of residual newborn bloodspots: an analysis of print media, legal cases, and public engagement activities. J Community Genet. 2015;6(2):117–128. doi: 10.1007/s12687-014-0206-0.
- Zakaria R, Allen KJ, Koplin JJ, et al. Advantages and challenges of dried blood spot analysis by mass spectrometry across the total testing process. EJIFCC. 2016;27(4):288–317.
- Lehmann S, Delaby C, Vialaret J, et al. Current and future use of “dried blood spot” analyses in clinical chemistry. Clin Chem Lab Med. 2013;51(10):1897–1909.) doi: 10.1515/cclm-2013-0228.
- Lombardo S, Seedat F, Elliman D, et al. Policy-making and implementation for newborn bloodspot screening in Europe: a comparison between EURORDIS principles and UK practice. Lan Reg Health Eur. 2023;33:100714. doi: 10.1016/j.lanepe.2023.100714.
- Morrison A, Polisena J, Husereau D, et al. The effect of english-language restriction on systematic review-based meta-analyses: a systematic review of empirical studies. Int J Technol Assess Health Care. 2012;28(2):138–144. doi: 10.1017/S0266462312000086.
- Bhattacharya K, Wotton T, Wiley V. The evolution of blood-spot newborn screening. Transl Pediatr. 2014;3(2):63–70. doi: 10.3978/j.issn.2224-4336.2014.03.08.
- Downing M, Pollitt R. Newborn bloodspot screening in the UK – past, present and future. Ann Clin Biochem. 2008;45(Pt 1):11–17. doi: 10.1258/acb.2007.007127.
- Grüner N, Stambouli O, Ross RS. Dried blood spots–preparing and processing for use in immunoassays and in molecular techniques. J Vis Exp. 2015;(97):e52619. doi: 10.3791/52619.
- Wilson JMG, Jungner G. Principles and practice of screening for disease. Geneva: WHO; 1968. Available from: http://www.who.int/bulletin/volumes/86/4/07-050112BP.pdf