Abstract
Nutritional management of blood glucose levels is a strategic target in the prevention and management of type 2 diabetes mellitus (T2DM). To implement such an approach, it is essential to understand the effect of food on glycemic regulation and on the underlying metabolic derangements. This comprehensive review summarizes the results from human dietary interventions exploring the impact of dietary components on blood glucose levels. Included are the major macronutrients; carbohydrate, protein and fat, micronutrient vitamins and minerals, nonnutrient phytochemicals and additional foods including low-calorie sweeteners, vinegar, and alcohol. Based on the evidence presented in this review, it is clear that dietary components have significant and clinically relevant effects on blood glucose modulation. An integrated approach that includes reducing excess body weight, increased physical activity along with a dietary regime to regulate blood glucose levels will not only be advantages in T2DM management, but will benefit the health of the population and limit the increasing worldwide incidence of T2DM.
INTRODUCTION
Around 366 million people worldwide have diabetes and this is projected to reach 552 million by 2030 (Whiting et al., Citation2011). Diabetes is a leading cause of death in developed countries and is predicted to become epidemic in newly industrialized nations. Additionally, poorly controlled diabetes is a major factor in several serious disorders including macrovascular disease, vision loss, renal failure, neuropathy, and amputations (Diabetes Control and Complications Trial Research Group, Citation1993; Ohkubo et al., Citation1995; Haffner et al., Citation1998; Niskanen et al., Citation1998; UK Prospective Diabetes Study Group, Citation1998; Huxley et al., Citation2006). Nutritional management of blood glucose dysregulation is a strategic target, as there is mechanistic evidence to suggest that elevated blood glucose levels contribute toward development of type 2 diabetes mellitus (T2DM) (Blaak et al., Citation2012). Moreover, high blood glucose levels, including values that are below the cutoff for the diagnosis of diabetes, have been shown to be associated with an increased risk of cardiovascular events. This relationship applies not only to fasting values but also to other markers of glucose homeostasis, which reflect blood glucose control in the postprandial period (after a glucose load or meal) or in a longer time frame, including both fasting and postprandial conditions over a prolonged period (glycated haemoglobin; HbA1c) (Heianza et al., Citation2011). Adoption of a nutritional approach could deliver a cost-effective T2DM prevention and management strategy, applicable across the population. However, to implement a successful strategy and provide clear guidance, it will be essential to understand the effect of food on the underlying metabolic derangements. Particular foods are implicated with increased or reduced incidence of T2DM and their macro-, micro-, and not-nutrient composition along with their energy content are all considered to be contributors to glucose homeostasis (). This comprehensive review will focus on the evidence from human dietary interventions, demonstrating that particular food components have significant and clinically relevant effects on blood glucose regulation.
Figure 1 Potential for dietary factors to reduce blood glucose levels and impact on β-cell function and associated biomarkers.GSIS, glucose-stimulated insulin secretion.
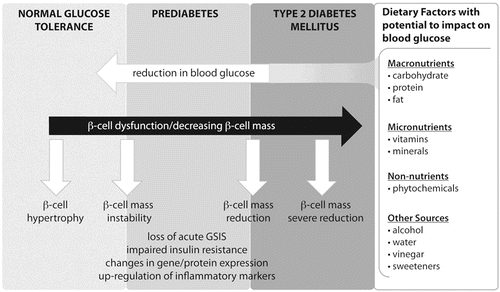
Search Strategy
A systematic and comprehensive review of the literature was undertaken using the highest quality data from the following bibliographic databases: MEDLINE (PubMed) and Web of Knowledge (or Science). These databases were searched for intervention trials investigating the effect of nutrients in postprandial hyperglycemia in T2DM patients or obese or control subjects with an explicit strategy for identifying data for extraction and summarizing the data. The search was conducted by combination (using Boolean operators) of the search terms of: “postprandial” + “specific macronutrient or other substance” and further refined by adding: “glucose” or “hyperglycemia” or “insulin sensitivity” or “insulin resistance.” Outputs were restricted to those completed studies only published in English. Terminology and quality of evidence was assigned according to guidelines from Oxford Centre for Evidence Based Medicine available at http://www.cebm.net/?o=1116. Strategy for identifying data for extraction and summarizing study findings are detailed in Tables and include: Type of Study, Food source (including physical characteristics and delivery), Control, Population Group, Methodology, Study Duration, Primary and Secondary Outcomes, and References. In addition to inclusion of data regarding PPG, outputs considering impact of dietary components on blood glucose regulation were also included, as this was considered to be relevant. The search exclusion criteria were type 1 diabetes mellitus (T1DM), gestational diabetes, diabetes insipidus, infants and pediatric studies, although reference has been made to these studies for discussive purposes.
Table 1 Carbohydrates and carbohydrate foods and postprandial glycemic control in healthy or diabetic individuals
Table 2 Dietary fiber and postprandial glycemic control in diabetic individuals
Table 3 Non digestible carbohydrates with prebiotic properties on blood parameters related to glucose homeostasis
Table 4 Dietary fatty acids and postprandial glycemic control
Table 5 Dietary vitamins and minerals and postprandial control
Table 6 Effect of phytochemical rich sources (cocoa, plant extracts) on postprandial glycemia
Table 7 Effect of ginseng on postprandial glycemia
Table 8 Effect of phytochemical rich sources (nuts and beverages) on postprandial glycemia
Table 9 Effect of miscellaneous food sources (water and low-calorie sweeteners) on postprandial glycemia
Impact of Macronutrients on Blood Glucose Levels
Dietary Carbohydrates and Glycemic Control
By promoting subclinical inflammation, chronic hyperglycemia is a major contributor to endothelial damage, providing a link between diabetes and cardiovascular disease (Ceriello, Citation2004). It has been suggested that oscillatory postprandial glycemic fluctuations are particularly detrimental in relation to interleukin 6 expression, indicating that foods which release their carbohydrates at a slow rate to the blood are to be preferred. Available intervention studies regarding the impact of dietary Glycemic Index (GI), or Glycemic Load (GL = GI × the amount of carbohydrates per portion) on glycemic control in subjects with diabetes was compiled in an extensive Cochrane review in 2009 (Thomas and Elliott, Citation2009). Data from 11 intervention studies lasting four weeks or longer were included and subjected to meta-analysis. The overall conclusion was that low GI diets improved diabetic control and reduced HbA1c to an extent that was comparable to that seen following medication in newly diagnosed T2DM subjects. It was also put forward that a low GI diet may improve whole body peripheral insulin sensitivity (Rizkalla et al., Citation2004), and reduce the number of hypoglycemic events (Gilbertson et al., Citation2001). Of interest in this context is that hypoglycemia may be an additional risk factor for cardiovascular disease in children with type 1 diabetes, independently of HbA1c (Pena et al., Citation2012). Consequently, carbohydrate foods that promote low but sustained blood glucose levels may be considered advantageous with regard to metabolic control of diabetes and possibly reduce risk of future cardiovascular events.
One potential point for criticism of the GI concept is that the glycemic impact of foods may be markedly affected by certain meal components. This has been shown in healthy subjects when adding, e.g., certain proteins or lipids to mixed meals (Wolever and Bolognesi, Citation1996). Consequently, adding whey and/or certain amino acids to a meal may substantially reduce the glycemic excursion in healthy and T2DM subjects (Frid et al., Citation2005; Nilsson et al., Citation2007; Gunnerud et al., Citation2012a, Citation2012b). However, for foods and meals rich in carbohydrates it appears as if the GI of mixed meals can be predicted from the component foods in healthy subjects indicating that for carbohydrate-rich meals the effects of additive components of GI are minor. This has also been demonstrated in T2DM subjects (Järvi et al., Citation1995; Robert and Ismail, Citation2012). The authors concluded that generally, the single foods were ranked similarly to the mixed meals, supporting the utility of the GI concept also for mixed meals in T2DM subjects (Robert and Ismail, Citation2012).
The GI of foods is influenced by the type and amount of dietary fiber (DF). In a study by Järvi and coworkers (Järvi et al., Citation1999), a four-week intervention was performed in T2DM subjects comparing a high versus low GI diet, using a cross-over design. The differences in GI of the diets were achieved by manipulating the food structure, thus essentially maintaining the same food composition- including that of the DF, but with different rates of digestion and absorption of the available carbohydrates. It was concluded, that the low GI diet with slow release carbohydrates lowered the glucose and insulin responses throughout the day and improved the lipid profile and capacity for fibrinolysis, suggesting a therapeutic potential of such a diet in diabetes.
The impact of carbohydrate foods on blood glucose levels is affected by a range of food factors, e.g., the type of carbohydrate, the food form, type and amount of DF, and the presence of certain food components capable of interfering with the digestive and/or absorptive mechanisms. As for the roles of sugars on glycemic regulation in diabetic subjects, Wheeler and Pi-Sunyer published a review in 2008, which is still valid (Wheeler and Pi-Sunyer, Citation2008). One of their messages was that adding an amount of sugar up to 10% of total energy to the diet did not negatively influence glycemic regulation as measured by HbA1c. Another message put forward by the same authors was that high-fructose corn syrup should be considered as sucrose rather than fructose when included in the diabetic diet, since it most often consist of equal amounts of glucose and fructose (Wheeler and Pi-Sunyer, Citation2008). Regarding the effects of fructose on glycemic control in diabetes, Cozma et al., recently published a meta-analysis (Cozma et al., Citation2012). Their aggregated analyses of short-term controlled feeding trials revealed that isocaloric fructose replacement of other carbohydrates (mainly starch and/or sucrose) resulted in clinically significant improvements in glycemic control, without significant effects on insulin, when including both type 1 and type 2 diabetic individuals. In stratified analysis, however, the reduction in glycated albumin and/or haemoglobin was only significant in people with type 1 diabetes. There are certainly trends toward positive outcomes on glycemia regulation also for individuals with T2DM, but larger and longer trials of higher quality are warranted to establish such associations (Cozma et al., Citation2012). However, it should be taken into account that a higher intake of sucrose or fructose should not be recommended to people with diabetes or other conditions of impaired blood glucose regulation, in view of a potential detrimental impact on body weight, insulin sensitivity, and blood lipids (Johnson et al., Citation2009; Stanhope et al., Citation2009).
Effect of Carbohydrate Structure and Properties
Chemical composition as well as the degree of gelatinization may play a role for glycemic responses to starchy foods. Most of the knowledge concerning the differences in PPG to carbohydrates and carbohydrate foods stem from studies in healthy subjects. Information regarding the GI features of carbohydrate foods is available in the international GI tables (Atkinson et al., Citation2008), where studies in diabetic subjects are presented.
Amylose-amylopectin ratio in starch: In a study of parboiled rice of a high-amylose (27%) variety, it was suggested that a combination of the high-amylose rice and formation of stable amylose-lipid complexes of the complex II type were responsible for substantial lowering of GI (Larsen et al., Citation2000). Furthermore, the results indicated that the severity of processing during parboiling may be an important tool to reduce the GI of rice. In bread products, the use of wheat (Hallstrom, Sestili et al., Citation2011), barley (Liljeberg, Åkerberg et al., Citation1996), and corn (Granfeldt, Drews et al., Citation1995) varieties with elevated amylose contents have been reported to have increased contents of resistant starch (RS). In addition to the formation of an enzyme-resistant starch fraction in bread with elevated amylose content, a slowly digestible starch fraction appears to be formed, as judged from decreased PPG in healthy subjects. When combining the use of cereal varieties with elevated amylose contents with baking at pumpernickel conditions (low temperature-long time), the formation of RS is promoted even more (Hallström et al., Citation2011). Some suggested mechanisms behind the increased RS-formation are: a compact structure that results in restricted starch swelling and gelatinization, formation of amylose-lipid, or amylose-protein complexes, or encapsulation of gelatinized starch between layers of RS.
Native versus gelatinized starch: Processing by heat is common in the manufacturing of cereal products. During heat treatment the starch is more or less completely gelatinized. However, although the degree of gelatinization may be rather low, the rate of amylolysis and thus the glycemic response appear to increase rapidly. This has been shown for, e.g., oat flakes in healthy subjects (Granfeldt, Hagander et al., Citation1995; Granfeldt, Eliasson et al., Citation2000). A possibility to lower the glycemic response to starchy food is by using native starches, rather than gelatinized or partly gelatinized forms. The use of uncooked corn starch to prevent nocturnal hypoglycemia in diabetic children is one example of how glycemic features of a food product can be optimized by a conscious choice of ingredients. Thus, in a study by Kaufman and Devgan (Citation1996), the frequency of nocturnal and before breakfast hypoglycemic episodes was significantly reduced in type 1 diabetic subjects after having uncooked corn starch included in an evening snack, compared with their normal snack without such starch.
Chemically modified starch: Hydrogenated starch hydrolysates (HSH) are used as bulking and nonreducing sweetening agents in for example, candy and gums. When comparing the glycemic response to glucose and two varieties of HSH in both insulin- and noninsulin dependent diabetic subjects as well as healthy individuals, the glycemia was significantly reduced after the HSH-meals (Wheeler et al., Citation1990). Furthermore, when combining all the subjects, a difference in glycemic responses between the two HSH-varieties could be detected, pointing in favor of the HSH with shorter hydrogenated saccharide chains (HSH, 5875).
Intact botanical structure or physical form: As mentioned above, studies in T2DM subjects have clearly indicated the importance of food structure on PPG (Järvi et al., Citation1995). In an earlier study (d’Emden et al., Citation1987) the glycemic responses to equi-carbohydrate portions of bread made from either white wheat or semolina, respectively, or spaghetti made from white and whole grain wheat, respectively, were tested in a group of noninsulin dependent diabetic subjects. No differences were found in glycemic response between either forms of bread, or either type of spaghetti, but both bread products showed higher glucose responses than the spaghetti meals. Thus, it was concluded that the physical form of the food was a major factor influencing the glycemic response. Similarly, maintaining the botanical structure of cereal kernels (Liljeberg et al., Citation1992) as well as rice (Panlasigui and Thompson, Citation2006) intact has been shown to reduce PPG. The importance of botanical structure has recently been investigated in two semiacute studies in healthy subjects (Nilsson et al., Citation2008a; Nilsson et al., Citation2008b). Interestingly, it was revealed that the semiacute benefits of barley kernels on daylong glycemia was lost when the kernels were milled into flour and consumed as porridge (Nilsson et al., Citation2008a). On the other hand, by mimicking the content of DF and RS in barley kernel bread, a flour-based bread product improved glucose tolerance in an overnight perspective study (10 h), compared with a white wheat bread (Nilsson et al., Citation2008b). The benefits observed on semiacute glucose tolerance are mediated by mechanisms emanating from colonic fermentation of the indigestible carbohydrate fraction.
In an earlier attempt to investigate the potential of depletion and disruption of dietary fiber, Haber et al. (Citation1977) compared the postprandial blood glucose levels in healthy subjects after intake of apples, fiber-disrupted apple purée and fiber-free apple juice. The results showed that plasma glucose rose to the same extent after all three meals, but there was a substantial difference in the rebound fall with the apple juice causing a marked hypoglycemia between approximately 60–120 min after the meal. The postprandial glucose response after intact apples did not fall below the fasting level at any time point. In addition to its detrimental effect on glucose regulation, the disruption and removal of fiber also favored faster and easier ingestion, which may promote over-eating of such products.
Course of glycemia: After reviewing the literature regarding effects of available carbohydrates on blood glucose levels in diabetic patients it is concluded that there are surprisingly few studies focusing on carbohydrate foods. However, as reported in the International tables of glycemic index (Atkinson et al., Citation2008), there is a high correlation between GI-values derived from studies performed in healthy and diabetic subjects, respectively. Therefore, much of the information on the glycemic response of different foods gained in healthy people may be relevant also to predict the impact of these foods on blood glucose levels in diabetic patients. Another important aspect to consider when discussing acute and semiacute glycemia is to acknowledge not only the GI-value of a food product but the course of glycemia. In 2009, the concept of glycemic profile (GP) was introduced and is defined as the duration of the incremental postprandial blood glucose response divided by the blood glucose incremental peak (min mM−1) (Rosen et al., Citation2009). Rye products generally produced high GI-values despite low insulin indices. When examining the course of glycemia after rye products, there was a sustained blood glucose increment beyond the 120 min used for GI-calculations, resulting in a higher than expected GI-value. It has been suggested that GP is more strongly correlated with insulin response (both insulin index and insulin incremental peak) compared with GI (Rosen et al., Citation2009; Rosen, Östman et al., Citation2011). Taking into account also the avoidance of hypoglycemia after high-GP food, this GP measure describing the course of glycemia may also be interesting to apply to PPG and glycemic regulation in diabetic subjects.
Dietary Fiber and Glycemic Control
Dietary fiber has traditionally been defined as edible plant polysaccharides and lignin naturally occurring in foods, and which are resistant to hydrolysis by human digestive enzymes (Trowell et al., Citation1976). Later definitions of fiber in nutrition have also included isolated fibers from food raw materials or edible synthetic carbohydrates that are resistant to digestion and absorption in the human small intestine but are partly or completely fermentable in the colon, and display beneficial physiological effects, i.e., improved bowel function, blood cholesterol, and blood glucose attenuation (Raninen et al., Citation2011). These beneficial effects, i.e., improvements in metabolic and disease control, are essentially based on the dominant attributes of different fiber types when passing through the gastrointestinal tract.
Types of dietary fiber: Dietary fiber is chemically a heterogeneous group of compounds with variable molecular size and different physicochemical properties, such as water solubility, viscosity, cation exchange properties, organic acid absorption and water-holding capacity (Eastwood and Morris, Citation1992; Guillon and Champ, Citation2000). Several classifications for fiber have been established (Slavin et al., Citation2009; Raninen et al., Citation2011) in which the traditional one is based on water solubility dividing fiber into soluble (pectins, gums, mucilages) and insoluble (cellulose, hemicelluloses, lignin) fiber types. Although solubility per se is an essential determinant of the physiological responses, viscosity and fermentability are likely to play a more pronounced role in the physiological benefits in humans.
Major dietary sources of DF are whole grain cereals, legumes, vegetables, and fruits, which include a wide variety of different types of fiber. The strongest and most consistent effects on reduced risk of developing T2DM are observed with diets high in insoluble and often only moderately fermentable cereal fiber, whereas fruits and vegetables, which are more important sources for soluble/fermentable fiber, do not show any protective effect on this outcome in meta-analyses of prospective cohort studies (Weickert et al., Citation2006; de Munter et al., Citation2007; Schulze et al., Citation2007).
However, most commonly used fiber supplements are primarily soluble fiber types, such as guar gum, glucomannan, xanthan gum, psyllium, pectin, alginate, β-glucan concentrates, and various fiber combinations (Vuksan et al., Citation2009; Papathanasopoulos and Camilleri, Citation2010). To date, it has not been investigated in long-term controlled human interventions whether these agents influence the risk of developing T2DM. Due to the diversity of DF in natural fiber sources it is generally recommended to adhere to these food sources to improve daily DF intake, thereby also assuring the additional nutritional qualities of a healthy diet. Even so, it is usually challenging to meet the recommended DF intake. Therefore, fiber supplements can play an important role in increasing DF intake but also in managing daily glucose levels both in healthy individuals and individuals with impaired glucose metabolism. However, although the potential health benefits of different types of DF are known, there is still no clear consensus about the ideal composition and proportion for the consumption of the major sources of DF.
Amount of fiber: In addition to the type of DF, the amount of DF plays a marked role in acute glycemic and insulinemic responses. For example, findings from studies using β-glucan have shown that 4 g of soluble β-glucan appear to be a threshold for a significant reduction in postprandial glucose and insulin responses in healthy individuals (Granfeldt et al., Citation2008; Juvonen et al., Citation2011), whereas in T2DM subjects somewhat lower amounts may be sufficient (Tapola et al., Citation2005). However, the amount of fiber does not appear to explain the lower postprandial insulin response observed after a meal containing rye bread as compared to white wheat bread (Juntunen et al., Citation2003). Furthermore, the food matrix, i.e., liquid or solid food forms in which fiber is consumed and macronutrient content in mixed meals can markedly affect postprandial glucose and insulin responses, as described elsewhere in the text.
Postprandial trials on fiber foods and supplements: Recently, an obvious reappearance in the research interest on the role of DF in the regulation of glycemic and insulinemic responses in individuals with T2DM has emerged. Until now, a large number of postprandial studies involving different fiber types, either in the form of unprocessed foods or as isolated DF supplements, have been carried out in people with T2DM ( & 2). Additionally, data are derived from a few longer-term dietary interventions in which either modified total diets or individual fiber products were served to individuals with T2DM for periods from two weeks up to one year ().
The randomized short-term trials clearly indicate that especially soluble fibers with viscous properties (e.g., glucomannan, guar gum, psyllium, β-glucan) exert acute improvements in glucose and insulin responses in individuals with T2DM (). Guar gum (GG), an example of such a water-soluble nonstarch polysaccharide, has shown both glucose and insulin lowering properties (Ellis et al., Citation1991; Golay et al., Citation1995), and psyllium fiber administered to NIDDM patients immediately before breakfast and dinner lowered postprandial glucose by 14% at breakfast and 20% at dinner compared to placebo. A second meal effect was observed at lunch (served 5 h after breakfast), where glucose was 31% lower compared with placebo. Psyllium's ability to reduce glycemia was not affected by the patients mode of therapy (diet-treated or on oral hypoglycemic agents) (Pastors et al., Citation1991).
In the majority of these postprandial studies, relatively high doses of single DF types have been used to produce significant postprandial reduction especially in glucose but also in insulin responses when compared with no or low-fiber test products (Tappy et al., Citation1996; Östman et al., Citation2006; Kim et al., Citation2009). The significant effects of DF on postprandial glucose are not necessarily accompanied with similar insulin responses which may partly result from the DF type or amount used in these studies. Insoluble cereal fiber is nonviscous/gel-forming and, therefore, does not directly influence GI. However, cereal fiber has been shown to improve whole-body insulin sensitivity, an effect that can be observed as short as 24 h after intake, i.e., second meal effect (Weickert et al., Citation2005; Nilsson et al., Citation2008c, Citation2008d, Citation2008e, Citation2008f). Since many low-GI foods are also rich in insoluble cereal fiber interpretation of the results is often not straight forward (Weickert and Pfeiffer, Citation2009). It is also worth to note that the food matrix in which the fiber is incorporated may actually modulate the postprandial responses (Brennan, Citation2005) so that the effect of the added fiber may be partly masked (Sels et al., Citation1992). These examples highlight the difficulties when trying to investigate the metabolic effects of single components of complex foods (Weickert, Citation2012).
Semiacute effects: The presence of intrinsic DF in certain cereal products has been shown to influence PPG in healthy humans at a subsequent meal, through a mechanism involving gut fermentation (Nilsson et al., Citation2008a, Citation2008b). Consequently, provision of evening meals based on barley kernel bread significantly lowered blood glucose excursions at a subsequent standardized breakfast compared with an evening meal with white bread. Also, markers of inflammation and insulin resistance were lowered, and the incretin hormone GLP-1 increased at breakfast (Nilsson et al., Citation2008a). In the case of barley, similar benefits on over-night glucose tolerance could be mimicked by adding a similar amount and ratio of barley DF and RS to white wheat bread. In contrast, a flour-based whole grain barley evening meal failed to induce benefits the subsequent morning (Nilsson et al., Citation2008c), indicating a role of the combined barley DF and RS in case of the kernel-based barley meal. Additionally, ingestion of boiled cereal kernels from rye and barley in the morning, not only reduced the acute PPG, compared with white bread, but additionally improved PPG at two subsequent standardized meals in healthy subjects. It is clear that cereal products rich in intrinsic DF may improve day-long PPG (Nilsson et al., Citation2008b). It is put forward that whereas the benefits of certain kernel-based products in the 10 h perspective is related to gut fermentation; benefits from breakfast to lunch are probably mediated by the latent features of the breakfast meal. In contrast to barley, most rye meals are characterized by improved PPG, including both flour-based and kernel-based products (Rosen et al., Citation2011). Interestingly, in the case of rye, evidence of rapid gut fermentation is present as judged from early postprandial increment in breath hydrogen excretion. Taken together, the botanical structure and intrinsic composition of fermentable substrates seems important for PPG at subsequent meals.
Longer-term interventions: Evidence from longer-term DF interventions in T2DM subjects shows that relatively high fiber levels can be consumed when given as unprocessed foods (legumes, vegetables, whole grain cereals) without causing gastrointestinal problems (). Both de Natale et al. (Citation2009) and Chandalia et al. (Citation2000) noticed improved glycemic control in T2DM subjects after feeding 4–6 weeks of diets supplying 50–53 g of fiber per day. No adverse side effects were reported. Studies lasting 6–12 months with diets containing low-GI foods have produced rather negative results on fiber effects, showing no effect on glycated hemoglobin levels in T2DM subjects (Jenkins et al., Citation2008a, Citation2008b; Wolever et al., Citation2008).
An 18-week randomized controlled intervention in healthy subjects having increased intake of insoluble cereal fiber, supplemented by cereal fiber extracts twice daily, significantly improved whole-body insulin sensitivity, as measured using euglycemic-hyperinsulinemic clamps (Weickert et al., Citation2011a, Citation2011b). A previous intervention by Pereira and colleagues showed comparable results when investigating the effects of whole grain diets on whole-body insulin sensitivity (Pereira et al., Citation2002). Interestingly, the consumption of insoluble resistant starch appears to result in comparable effects on insulin sensitivity in healthy adults (Robertson et al., Citation2003; Robertson et al., Citation2005) and in subjects with metabolic syndrome (Johnston et al., Citation2010). It is worth noting that in some studies different sorts of moderately fermentable insoluble cereal fiber and insoluble resistant starch resulted in comparable improvements of estimated insulin sensitivity 24 h after consumption of the cereal. Fiber/starch extracts consumed in a second meal test, indicated that colonic fermentation with the production of SCFA was not the key factor conveying improved insulin sensitivity in this setting (Weickert et al., Citation2005). Longer-term studies with isolated fiber products have yielded conflicting results. Schwab et al. (Citation2006) gave 24–30 g soluble fiber in the form of sugar beet pectin or synthetic polydextrose mixed with drink for three months to 66 subjects of which 10 were T2DM subjects, the rest having problems with glucose metabolism (IGT, IFG). They found no effect on fasting or on PPG after a standardized breakfast. However, supplemental fructooligosaccharides (FOS), 20 g/d, given to subjects with idiopathic hyperglycemia reduced the glucose excursions (Sorensen and Johansen, Citation2010).
Possible mechanisms of fiber effects: Soluble viscous fibers play a marked role in managing postprandial glucose and insulin levels via forming viscous gels by absorbing water and thereby reducing gastric emptying (Marciani et al., Citation2001; Darwiche et al., Citation2003; Sanaka et al., Citation2007) and rate of glucose absorption (Dikeman and Fahey, Citation2006). Other suggested explanations why high viscosity products reduce PPG are altered intestinal motility, slower diffusion rate of starch digestion products and reduced α-amylase accessibility (Leclere et al., Citation1994). In addition, it has been shown in vitro that guar gum inhibits α-amylase in a direct, noncompetitive way in the first stage of the enzymatic degradation of starch (Slaughter et al., Citation2002).
The mechanisms underlying the association of increased fiber intake with improved glycemic control in longer term studies (Hu et al., Citation2001; Schulze et al., Citation2007; Livesey et al., Citation2008) are not fully understood, but may include also colonic effects such as short chain fatty acid production by colonic fermentation and concurrent liberation of phenolic compounds in addition to the above mentioned altered postprandial gastric and small intestinal functions (CitationLappi et al., in press; Vitaglione et al., Citation2008). Improved whole-body insulin sensitivity by insoluble cereal fiber was suggested to be related to interference with the digestion and/or absorption of dietary protein and consequently reduced amino acid induced activation of the mammalian target of rapamycin (mTOR) S6 kinase 1 (S6K1) signaling pathway (Weickert et al., Citation2006, Citation2011a, Citation2011b) that directly results in insulin resistance both in animal models and human intervention studies (Krebs et al., Citation2002; Um et al., Citation2004; Tremblay et al., Citation2005). Further mechanisms that may be involved could be cereal-fiber induced binding of bile acids and fiber-induced effects on gut hormones and metabolite profiles but this will need to be investigated in future studies.
Thus, these results suggest that acute consumption of foods rich in soluble fiber or soluble fiber supplements is beneficial especially for the postprandial glycemic control but also for the insulinemic response. However, the number of studies investigating the effects of DF on acute glucose and insulin responses in T2DM subjects is still limited which limits interpretation of the effects. Moreover, the relevance of acute postprandial effects needs to be studied with regard to long-term health outcome; in this context also insoluble dietary fiber seem to play an important role by improving glucose and/or insulin homeostasis (Blaak et al., Citation2012).
Gut Microbiota and Glycemic Control
The gut microbiota is increasingly considered as a symbiotic partner for the maintenance of health. The human gut harbours vast numbers of bacteria, around 1011 bacterial cells per mL of contents (Diamant et al., Citation2011; Qin et al., Citation2010). Specific strains of gut bacteria can be classified upon their sequence analogy into phyla, gender, species, and subspecies. More recently, Arumugam et al., have “clusterized” the gut microbiota of individuals from different countries and continents into three “enterotypes” identified by the variation at the level of one of the three following genera: Bacteroides, Prevotella, and Ruminococcus (Arumugam et al., Citation2011). Long-term dietary pattern may influence the proportion of those enterotypes, the Prevotella group being associated with carbohydrates and fiber intake (Wu et al., Citation2010). Bifidobacterium spp. represents an important and complex group of bacteria whose presence is often associated with beneficial health effects (Boesten and de Vos, Citation2008; Turroni et al., Citation2009; Delzenne and Cani, Citation2011a, Citation2011b). The Bifidobacterium genus was poorly represented in the faecal samples of diabetic subjects compared with healthy individuals (Wu et al., Citation2010). Another interesting bacterial species is Faecalibacterium prausnitzii, which level is found to be decreased in subjects with diabetes compared to nondiabetic obese subjects. Additionally, F. prausnitzii is negatively associated with inflammatory markers measured in the serum of obese individuals before and after roux- and Y-gastric bypass surgery (Furet et al., Citation2010). Related to the gut microbiota, components of the bacterial membrane have been incriminated in the development of inflammation and this is associated with lipopolysaccharides (LPS), present in gram negative bacteria (for review, see Cani and Delzenne, Citation2011). The level of serum LPS is increased approximately two-fold in obese subjects and those with diabetes, or fed a high-fat diet. This is considered to involve processes including an increase in chylomicron formation (upon high fat diet feeding), a decrease in gut barrier integrity, and/or a decrease in alkaline phosphatase activity, which is the enzyme responsible for the cleavage of the LPS in the intestine. It is likely that the metabolic function of the gut microbiota could be important in the control of blood glucose, even if much of the data have been mainly obtained in animal studies. The promoting effect of the gut microbiota on intestinal glucose absorption has been suggested in germ-free mice colonized with the saccharolytic Bacteroides thetaiotaomicron: this effect supports the fact that events occurring upon bacterial fermentation may have an effect in the upper part of the gut, and can modulate digestible carbohydrates availability (Hooper et al., Citation2001). The short chain fatty acids produced upon carbohydrate fermentation in the gut, reach host tissues where they may act as metabolic regulators or substrates. If propionate acts as gluconeogenic substrate in the liver, it is also able, at least in vitro, to counteract gluconeogenesis from lactate, thereby decreasing hepatic glucose production. Dietary supplementation of butyrate can prevent and treat diet-induced insulin resistance in mouse. The mechanism of butyrate action is related to promotion of energy expenditure and induction of mitochondria function (Gao et al., Citation2009). On the other hand, the G-protein coupled receptors, GPR43 and 41, are both expressed in endocrine L cells in the colon, and when activated by their physiological ligands, the short chain fatty acids, they promote proglucagon expression, GLP-1 secretion, and could thereby control insulin secretion and glucose homeostasis (Tolhurst et al., Citation2012).
If the gut microbiota plays a role in the control of glycemia, it would be interesting to modulate the gut microbiota composition. The transplantation of gut microbiota from diabetic animals to germ-free mice transfers the diabetic and obese phenotype, thereby suggesting that the characteristics of the gut microbiota of diabetic individuals per se could play a role in the metabolic response to diet, and that gut microbial transfer is effective (Delzenne et al., Citation2011). The proof of this concept has recently been shown in humans. An allogenic faecal transplantation assay was performed from a nonlean male donor to individuals with metabolic syndrome, and this “treatment” was shown to improve glucose tolerance and insulin sensitivity after six weeks. Specific changes in the gut microbiota composition were associated with the improvement of health (Vrieze et al., Citation2012). It is clear that dietary approaches influence the composition of the gut microbiota in humans and for this reason, the following sections will cover the potential interest of prebiotics and probiotics to control blood glucose levels.
Prebiotics and Blood Glucose Regulation
Among dietary fiber, some oligo- and polysaccharides exhibit prebiotic properties, a concept originally described by Gibson and Roberfroid (Citation1995) and recently revisited at the initiative of the ILSI prebiotic task force (Roberfroid et al., Citation2010). This “prebiotic concept” is defined as the selective stimulation of one or a limited number of microbial genus(era)/species/strain(s) in the gut microbiota that confer(s) health benefits to the host. Inulin-type fructans were the first studied prebiotics that have repeatedly demonstrated the capacity to stimulate the growth of bifidobacteria in both animals and in humans. Even if other bacteria may be promoted or modulated on fructan administration, there is no proof that the bifidobacteria are responsible per se for the health effect of fructans, the bifidogenic effect appears as the “microbial signature” of those prebiotics. Other oligosaccharides such as galactans, arabinoxylans and glucans have now been considered as dietary fibers with prebiotic properties (Delzenne et al., Citation2011).
The modulation of gut microbiota by using dietary prebiotics in the control of blood glucose homeostasis has been evaluated in experimental animal models of hyperglycemia, associated or not with obesity (streptozotocin treatment rats, obese leptin deficient or leptin resistant mice of rats, high fat/ and or high glycemic index diet). The data have been detailed in a previous paper (Roberfroid et al., Citation2010). A decrease in postprandial or post-oral glucose tolerance test is observed in most of the models. The implication of gut peptides such as an increase in L cell differentiation leading to an increase portal GLP-1 may be involved in this effect, as shown in high fat fed mice or rats (Delzenne et al., Citation2011), or more recently in ob/ob mice (Everard et al., Citation2011). Data obtained in high-fat fed mice treated with fructan-type prebiotics revealed an improvement of hepatic insulin sensitivity and a decrease in fasting insulinemia that correlated with the increase in caecal Bifidobacteria (Cani et al., 2008). A more detailed genomic analysis of the gut microbiota in ob/ob mice treated with fructans as prebiotics highlighted novel bacteria that could be associated with the improvement of gut endocrine function (L cell number), such as Faecalibacterium praustnitzii or Akkermansia muciniphila (Everard et al., Citation2011). The increase in GLP-2 by L cells occurring in prebiotic-treated mice could, in turn, reinforce the gut barrier integrity and thereby decreases inflammation in the liver tissue. This effect also contributes to improved glucose metabolism (Cani et al., Citation2009). However, in the Profimet study whole-body insulin sensitivity (Weickert, 2012) was significantly improved independent of various markers of colonic fermentation, SCFA profiles in faecal samples, and dominant groups of the gut microbiota (Weickert et al., Citation2011a, Citation2011b), indicating that further factors were involved in conveying improved insulin sensitivity upon the consumption of insoluble cereal fiber.
Few papers have been published, which have focused on the influence of prebiotics on glucose homeostasis in humans (). Luo et al. (Citation1996) were the first to show that 20 g of short chain fructans given for four weeks in healthy subjects decreased basal hepatic glucose production, but had no detectable effect on insulin-stimulated glucose metabolism. In T2DM subjects, no significant modification of glucose homeostasis (plasma glucose level or hepatic glucose production) occurred in prebiotic-treated patients (Luo et al., 2000). In a similar study conducted in hypercholesterolemic patients, fructan-prebiotics reduced the PPI response, but the clinical relevance of this effect remains unclear (Giacco et al., Citation2004). A two-week supplementation with 16 g/day inulin-type fructans versus the same amount of maltodextrin as placebo, was able to increase GLP-1 production, and to lessen postprandial glucose response after a standardized breakfast (Cani et al., 2009a). Increases in gut peptides have also been shown in obese individuals treated with prebiotics. This effect was related to improvement of satiety, but the relation with glucose homeostasis was not reported in this study (Parnell and Reimer 2009). Glycemia was not significantly modified in obese individuals subjected to low-calorie diet completed with Jerusalem artichoke concentrate containing oligofructose as prebiotic, whereas an improvement of HOMA-IR was observed in treated obese adult women (Antal et al., Citation2008). If there are some positive effects of a prebiotic intervention, there is a lack of information relating the improvement of glucose response and to changes in gut microbiota composition and/or activity in humans or in diabetic/obese subjects. Those studies are needed to evaluate the relevance of microbiota in the management of glucose homeostasis.
Probiotics and Blood Glucose Regulation
A number of observations have led to speculation that probiotics, defined as “live microbes which, when administered in adequate amounts, confer a health benefit on the host” (FAO/WHO, Citation2002), may have potential benefits in the maintenance of healthy glucose metabolism. However, despite encouraging preclinical studies, the clinical research in this field is still only starting to emerge. In this review no human clinical studies assessing the effect of probiotic bacteria specifically on postprandial glycemia were identified. However, a limited number of clinical studies addressing the effect of probiotics on related outcomes such as fasting glycemia and insulin resistance were identified.
Some studies have investigated the effect of probiotics on fasting glycemia. Moroti et al. (Citation2012) reported that consumption of probiotics and fructo-oligosaccharides reduced fasting glycemia in elderly subjects with T2DM, whereas consumption of a placebo product did not. Similar results on probiotics were reported by Ejtahed et al. (Citation2011; Citation2012), but Chang et al. (Citation2011) and Schaafsma and coworkers (Schaafsma et al., Citation1998) did not observe reduced glycemia in their interventions. Probiotics combined with dietary counseling have also been suggested to improve glucose regulation during pregnancy (Laitinen et al., Citation2009). Probiotics may also regulate glucose by targeting insulin action; Andreasen et al. (Citation2010) reported an improvement in insulin sensitivity in diabetic and nondiabetic volunteers following consumption of probiotics. A bilberry drink with oatmeal fermented with probiotics has also been suggested to lead to reduced insulin demand upon glycemic response (Granfeldt and Bjorck, Citation2011). In addition to human studies, several animal studies have yielded promising results on the efficacy of probiotics in the maintenance of healthy fasting and postprandial blood glucose levels and related outcomes (Andersson et al., Citation2010; Amar et al., Citation2011a, Citation2011b; Chen et al., Citation2011).
Dietary Protein and Blood Glucose Regulation
High protein diets appear to have beneficial effects on weight loss, body composition, and certain blood lipids, at least in the short term (Hession et al., Citation2009). Satiating effects of dietary protein, a reduced choice of foods, and an aversion against dietary fat in the absence of carbohydrates have all been attributed to better weight loss with high protein diets (Weickert et al., Citation2005). Lowering the percent protein of the diet from 15% to 10% increases total energy intake (Gosby et al., Citation2011), further indicating that a higher intake of dietary protein may help to reduce energy intake. In a recent review, it is concluded that dietary protein by acting on satiety and energy expenditure in negative energy balance may prevent weight cycling effects (Westerterp-Plantenga et al., Citation2012), indicating a beneficial role on weight regulation. Furthermore, a combination of high-protein and low glycemic load foods, in a hypo-caloric diet, significantly increased insulin sensitivity and decreased markers of inflammation in overweight and obese women with polycystic ovary syndrome (Mehrabani et al., Citation2012). Insulin resistance following amino acid infusion has been reported in healthy humans (Tremblay et al., Citation2005). One of the mechanisms of protein-induced insulin resistance appears to be inhibition of glucose uptake through phosphorylation of downstream factors of the insulin signaling cascade by the translation initiation factor serine-kinase-6-1 (S6K1) (Tremblay et al., Citation2005). On the other hand, it has been put forward that branched-chain amino acids (BCAA), in particular leucine, may help to counteract “anabolic” resistance” which may increase availability of AA for muscle protein synthesis, reduce muscle protein breakdown, and enhance glucose disposal to help maintain blood glucose homeostasis in T2DM (Manders et al., Citation2012). Recent data on metabolomic profiling in obese adults have suggested associations between BCAA and future risk of T2DM (Newgard et al., Citation2009a, Citation2009b), whereas the opposite has been found in youth (Michaliszyn et al., Citation2012a, Citation2012b). In fact in youth increased plasma AA concentrations were positively associated with β-cell function. In animal models particularly the intake of BCAA appears to lead to unfavorable metabolic effects including insulin resistance, although in rodents the background of a high fat diet is required to promote these negative effects (Newgard, Citation2012).
Longer term intake of high protein diets in human has been shown to result in whole-body insulin resistance (Linn et al., Citation2000; Weickert et al., Citation2006), associated with up-regulation of factors involved in the mammalian-target-of-rapamycin (mTOR)/S6K1 signaling pathway (Linn et al., Citation2000; Weickert et al., Citation2006), increased stimulation of insulin and glucagon within the endocrine pancreas, stimulation of gluconeogenesis (Linn et al., Citation2000; Weickert et al., Citation2006), and high glycogen turnover (Linn et al., Citation2000; Weickert, Citation2012). Furthermore, protein induced deterioration of insulin sensitivity has been observed in humans under conditions of restricted fat intake (30% of energy intake), both on whole-body and liver level, as measured using euglycemic hyperinsulinemic clamps combined with stable isotope techniques (Weickert et al., Citation2011a, Citation2011b). Interestingly, the way of protein administration seems to be of importance, and supplementation of insulin resistant rats with leucine through gavage, that is intermittent feeding, significantly lowered fasting glycemia compared with continuous administration through the drinking water (Zanchi et al Citation2012).
In the short-term, these unfavorable effects on insulin resistance could be compensated by high protein diet induced weight loss, and, at least in physically active people, an increase of the in lean muscle mass that is also mediated via the mTOR/S6K1 pathway. In the Diet, Obesity and Genes (DiOGenes) European multicentre trial with 548 completers, maintenance of weight loss was marginally better with the high protein diet despite controlled conditions, with no significant difference between groups in the full model (Larsen et al., Citation2010). Furthermore, DiOGenes revealed that both, a high protein intake or increased intake of high-GI carbohydrates, may increase low-grade inflammation (Gögebakan et al., Citation2011), which could be further related to worsening of whole-body insulin resistance (Möhlig et al., Citation2006). In further agreement that high protein-diets may have deleterious effects on glucose metabolism, a recent large prospective cohort with 10-years follow-up revealed that consuming 5% of energy from animal protein, but also from total protein at the expense of fat or carbohydrates increases diabetes risk as much as 30% (Sluijs et al., Citation2010). Furthermore, low carbohydrate high protein diets, used on a regular basis and without consideration of the source of proteins or the nature of carbohydrates is associated with increased risk of cardiovascular disease (Lagiou et al., Citation2012), thereby suggesting a link between high-protein Western diets, cardiovascular risk and T2DM. Given these concerns further research is advised before high protein diets should be routinely introduced as a tool in the treatment of patients with T2DM or at risk of developing diabetes. However, in subjects with intact β-cell function protein/amino acid induced insulin resistance may be compensated by the also observed amino acid mediated increase in insulin secretion, as discussed in the next paragraph.
Amino Acid Induced Insulin Secretion
In a state of impaired glucose tolerance or T2DM, endogenous insulin secretion shows multiple abnormalities. Secretory defects include an attenuated early insulin secretory response to glucose, reduced ability of the β-cell to fully compensate for the degree of whole-body insulin resistance, impaired glucose sensing ability of the β-cell, and a shift to the right in the dose-response curve between glucose and insulin secretion, all of which are indicative of a progressive insensitivity of the β-cell to glucose (Polonsky et al., Citation1996). These defects involve glucose-sensing and signaling pathways in the β-cell. Even though insulin secretion in response to the prevailing glucose concentration may be blunted in T2DM subjects, insulin secretion in response to other stimuli remains functional (van Loon et al., Citation2003). Besides glucose, amino acids can also act as potent stimuli for the secretion of insulin from the pancreatic β-cell (Newsholme et al., Citation2005). In vitro studies using incubated primary islet cells or β-cell lines have described strong insulinotropic properties for arginine, leucine, isoleucine, alanine, and phenylalanine (Floyd et al., Citation1963; Sener et al., Citation1981; Pipeleers et al., Citation1985; Blachier et al., Citation1989; Malaisse et al., Citation1991; Schwanstecher et al., Citation1998; Lajoix et al., Citation2001; Xu et al., Citation2001a, Citation2001b; Salehi et al., Citation2012). The various mechanisms, by which these amino acids promote and/or enhance insulin secretion from the pancreatic β-cell, are diverse and have not yet been fully elucidated (Newsholme et al., Citation2005). In the presence of glucose, amino acids like arginine seem to be able to stimulate insulin secretion by depolarizing the plasma membrane (Blachier et al., Citation1989). The latter results in the opening of voltage-activated Ca2+ channels, resulting in the influx of Ca2+, which triggers insulin exocytosis (Newsholme et al., Citation2005). Other amino acids tend to induce their insulinotropic properties by activating the Ca2+ channels, through their cotransport with Na+ (Sener and Malaisse, Citation2002). Furthermore, similar to glucose-mediated insulin secretion (Dean and Matthews, Citation1970), intracellular catabolism of the metabolizable amino acids in the pancreatic β-cell will increase the intracellular energy status (ATP/ADP ratio), which closes ATP-sensitive K+ channels, leading to the depolarization of the plasma membrane (Dunne et al., Citation1990; Brennan et al., Citation2002; Newsholme et al., Citation2005). In addition, leucine-induced insulin secretion is mediated both through its oxidative decarboxylation, as well as its ability to allosterically activate glutamate dehydrogenase (Newsholme et al., Citation2005; Xu et al., Citation2001a, Citation2001b). The latter has also been reported for other amino acids, like phenylalanine (Kofod et al., Citation1986). A simplified overview on some of the proposed mechanisms by which amino acids are likely to stimulate insulin secretion in the pancreatic β-cell is provided in .
Figure 2 Overview of proposed mechanisms by which glucose and leucine stimulate insulin secretion. GK, glucokinase; PDC, pyruvate dehydrogenase complex; PC, pyruvate carboxylase; TCA, tricarboxylic acid; α-KG, α-ketoglutarate; AT, aminotransferase; BCKDH, branched-chain a-keto-acid dehydryogenase; GDH, glutamate dehydrogenase; KIC, a-ketoisocaproate. Adopted form van Loon et al; Current opinion in clinical nutrition and metabolic care, 2012, 15: 71–77.
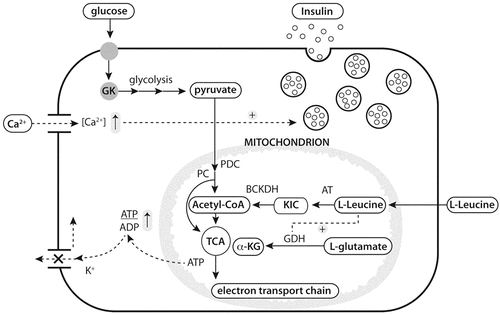
In accordance with the in vitro data on incubated β−cells, in vivo studies in humans have shown increased plasma insulin concentrations following the intravenous infusion of amino acids in both healthy (Floyd et al., Citation1966; Floyd et al., Citation1970) and T2DM patients subjects (Floyd, 1968). In line with those findings, nutritional studies in humans already reported the synergistically stimulating effect of the combined ingestion of carbohydrate and protein on plasma insulin concentrations in the 1960s (Rabinowitz et al., Citation1966; Pallotta and Kennedy, Citation1968), which were later confirmed in both healthy (Nuttall et al., Citation1985) and T2DM subjects (Nuttall et al., Citation1984; Gannon et al., Citation1992; Gannon et al., Citation1998). A series of studies trying to define the in vivo insulinotropic potential of the ingestion of various free amino acids and protein in combination with carbohydrate, report a mixture containing a protein (hydrolysate) with the addition of free leucine to be most potential (van Loon et al., Citation2000a, Citation2000b). The latter was later shown to allow a —two–four-fold greater increase in postprandial insulin release in both healthy and T2DM subjects when compared with the ingestion of carbohydrate only (van Loon et al., Citation2003; Manders et al., Citation2005; Manders et al., Citation2006a, Citation2006b; Manders et al., Citation2008). Reduction in PPG was observed following intake of whey and soy protein with or without supplementation with amino acids (isoleucine, leucine, lysine, threonine, and valine) in healthy volunteers. This reduction was mediated by an early postprandial insulinaemia (PPI) response and correlated with presence of plasma GLP-1 (Gunnerud et al., Citation2012a, Citation2012b). Increased plasma amino acid concentrations was also shown to be positively associated with β-cell function relative to insulin sensitivity in adolescents (Michaliszyn et al., Citation2012a, Citation2012b).
The insulinotropic properties of amino acids, and leucine in particular, may be of relevance as a means to stimulate endogenous insulin release and, as such, to improve postprandial glycemic control in T2DM subjects (van Loon et al., Citation2003; Manders et al., Citation2006a, Citation2006b). In agreement, various studies have reported lower postprandial glucose concentrations in both healthy, normoglycemic and T2DM subjects following co-ingestion of protein (concentrate/hydrolysate) and/or free amino acids (Nuttall et al., Citation1984; Nuttall et al., Citation1985; Gannon et al., Citation1992; Gannon et al., Citation1998; Gannon et al., Citation2001; Frid et al., Citation2005). The greater postprandial insulin response following protein and amino acid co-ingestion has been proven functional, stimulating blood glucose uptake, and reducing postprandial hyperglycemia in T2DM subjects (Manders et al., Citation2005). Co-ingestion of a small amount of protein hydrolysate and leucine with each main meal can be applied to improve 24 h glycemic control by reducing the prevalence of postprandial hyperglycemia (Manders et al., Citation2006a, Citation2006b) despite a higher energy intake. Protein and/or amino acid ingestion strongly stimulates postprandial insulin release in both healthy and T2DM subjects and, as such, can strongly affect postprandial glycemic control. Dietary intervention strategies to improve PPG should not only consider the glycemic load of a meal, but should also consider the protein/amino acid content of the meal.
Dietary Fatty Acids and Blood Glucose Regulation
A significant positive correlation exists between circulating free fatty acid levels and insulin resistance in tissues. Twenty years ago, Storlien et al. (Citation1991) reported that high saturated fat-fed rats developed insulin resistance, whereas those fed with diets high in n-3, with a low n-6/n-3 ratio, maintained insulin action at normal levels. Subsequent studies of platelet membrane phospholipid fatty acid content in Indian Asians who are at increased risk of metabolic syndrome showed a greater proportion of n-6 polyunsaturated fatty acid (PUFA) linoleic acid, and arachidonic acid in combination with a reduced proportion of the n-3 long chain (LC)-PUFAs, eicosapentanoic acid (EPA), and docosahexaenoic acid (DHA) in Indian Asians than in whites (Lovegrove et al., Citation2004). These effects have variably been attributed to PUFA-mediated alterations of the membrane phospholipid characteristics, such as fluidity (Rustan et al., Citation1997) or through the saturated fatty acid (SAFA)-driven accumulation of ceramide and diacylglycerol, which inhibit Akt/PKB activation. Latterly, an increase in ceramide content in skeletal muscles of obese insulin resistant humans (Adams et al., Citation2004) and in subjects who showed insulin resistance after lipid infusion (Straczkowski et al., Citation2004) ends further support to its role, possibly through alterations in lipid raft formation and stability (Xu et al., Citation2001a, Citation2001b) or increased reactive oxygen species (ROS) generation from mitochondrial uncoupling. In vitro experiments have confirmed that the presence of the monounsaturated fatty acid, oleate, can divert palmitate away from ceramide synthesis and prevent ceramide accumulation and insulin resistance (Gao et al., Citation2009; Gao et al., Citation2012). Since dietary fat and the resulting plasma fatty acids profiles are corelated, dietary intervention is predicted to influence insulin resistance.
A systematic analysis of the literature for studies reporting on the impact on blood glucose and insulin of interventions on fatty acid intake in the diet of adult humans with either obesity or T2DM has been undertaken here. We have identified 20 relevant studies for inclusion in systematic review, of which 3 considered fatty acid length and degree of esterification (Scalfi et al., Citation1991; Li et al., Citation2008), 4 examined n-3 PUFAs (Zambon et al., Citation1992; Brady et al., Citation2004; West et al. Citation2005, Madigan et al., Citation2000; Jans et al., Citation2012), 14 investigated effects of cis-MUFA (Lerman-Garber et al., Citation1994; Christiansen et al., Citation1997; McCargari et al., Citation1998; Madigan et al., Citation2000; Thomsen et al., Citation2003; Piers et al., Citation2003; Manning et al., Citation2004; West et al., Citation2005; Lefevre et al., Citation2005; Paniagua et al., Citation2007; de Natale et al., Citation2009; Sloth et al., Citation2009; Li et al., Citation2010; Lopez et al., 2011; Jans et al., Citation2012) three considered trans-MUFA (Christiansen et al., Citation1997; Lefevre et al., Citation2005; de Natale et al., Citation2009) with 7 describing effects of SAFA (Rivellese 1996; Christiansen et al., Citation1997; Thomsen et al., Citation2003; Piers et al., Citation2003; Manning et al., Citation2004; Devaraj et al., Citation2008; Lopez et al., Citation2011). In total, dietary effects on postprandial glucose or insulin were reported in 689 participants. Key features of these studies are reported in . Overall, there was no effect of varying triacylglycerol, diacylglycerol or chain length on insulin resistance in study participants although some benefits for lipid metabolism were noted. In contrast, closer examination of PUFA invention studies showed mixed results; one study reported a decrease in PPI concentration (n = 10, 12 weeks PUFA intervention) whereas a second reported no benefit (n = 18, US T2DM subjects, single PUFA meal) a third (n = 11, T2DM subjects in Ireland, two weeks PUFA intervention) reported higher PPI concentrations after compared with post-MUFA diets and a fourth reported improved AUC over 6 h for glucose and insulin after PUFA and MUFA compared to SFA (n = 10, Dutch insulin resistant, single meal). The variability in duration of dietary change may be significant in determining effect, with benefit being observed in the study with longest intervention. In the two studies that described an investigation of trans-MUFA, one did not show any significant difference to MUFA in outcomes and the second showed comparable effects to a saturated fatty acid diet. This is consistent with a review by Thompson et al. (Citation2011) that there is very little convincing evidence that habitual exposure to dietary trans-fatty acid as part of a standard western diet has a significant contribution to risk of diabetes or insulin resistance. However, studies in FABP2Thr54 carriers (at increased risk for T2DM complications) have shown increased postprandial glucose following trans-MUFA challenge compared to noncarriers of this allele (Lefevre et al., Citation2005). The majority of studies identified systematically in this present review have described cis-MUFA interventions on insulin resistance involving 277 subjects in 12 studies. Only 24 subject interventions showed no significant benefit, with the remaining 10 studies of 253 participants describing significant reductions in either postprandial glucose and/or insulin compared to control diets. Taking the systematic analysis of dietary fatty acid modulation together strongly supports the benefit of replacement of fats with MUFAs in the diet of T2DM or obese insulin-resistant subjects for the improvement of their glucose/insulin metabolism.
Impact of Micronutrients on Blood Glucose Levels
Dietary Vitamins and Glycemic Control
Micronutrients are nutrients required in small quantities for a whole range of physiological functions, but which the organism itself cannot produce. Micronutrients include dietary trace minerals, which are generally less than 100 mg day−1 and vitamins. Vitamins, organic molecules that are vital for normal physiology in any organism, are known to improve health when supplemented during specific deficiency states. However, less is known of the clinical value for supplementing to improve the outcomes of diseases that are associated with lower plasma vitamin levels. Concerns exist that vitamin supplementation for health benefit may be distributed according to a bell-shaped curve, where deficiency contributes to disease but excessive vitamin intake may also have adverse health outcomes for some individuals. However, the association between vitamin E and all cause mortality is now disputed (Gerss, Citation2009). A number of clinical trials are registered presently that are investigating vitamin supplementation in early diabetic states to prevent onset of T2DM, or in T2DM subjects to improve outcome measurements such as HbA1c level. A limited number of studies have completed which describe vitamin effects on PPG or PPI and these are summarized and discussed.
The complex of B vitamins serves as cofactors principally associated with metabolism and circulating plasma levels of B vitamins are reduced in some diabetic populations (Mitri et al., Citation2011; Page et al., Citation2011). Thiamine (vitamin B1) is an essential coenzyme for the transketolase enzyme and the dehydrogenase complexes for pyruvate, α-ketoglutarate, and branched-chain keto acids which is at lower plasma concentration in T2DM (Page et al., Citation2011). Benfotiamine, a lipid-soluble allithiamine derivative that has better intestinal absorption and improved bioavailability than thiamine, has been shown to afford postprandial benefit on vascular function following meals rich in advanced glycation end-products in T2DM. Plasma glucose levels were lowered in the benfotiamine supplemented group but not significantly in the postprandial period after and AGE meal (Stirban et al., Citation2006), but the effects of benfiotamine on PPG were significant after a cooked meat meal (Stirban et al., Citation2007). The pharmacologic effects of niacin (nicotinic acid; vitamin B3) on cholesterol lowering were first reported in 1955 (Altschul et al., Citation1955). It is potent in lowering fasting triglycerides and postprandial triglycerides, however, extended-release niacin increased postprandial insulin concentrations by 54% by 2 h after a high-fat meal indicating a decrease in insulin sensitivity (Plaisance et al., Citation2008). Nevertheless, there remain some significant benefits of niacin for management of cardiovascular complications and the most recent summarized evidence suggests that the cardiovascular benefit outweighs the reduction in glycemic control (Goldberg and Jacobson, Citation2008; Bays et al., Citation2011). However, it was observed that treatment with high dose, extended-release niacin did not reduce the risk of cardiovascular events (including heart attacks and stroke) in people with heart and vascular disease receiving intensive statin therapy (Boden et al., Citation2011). The cofactor, biotin (vitamin B7) works in synergy with insulin and independently increases the activity of glucokinase. No studies meeting the inclusion criteria for this review were identified using biotin supplementation alone. However, in moderately obese subjects with T2DM and with impaired glycemic control supplemented with chromium and biotin combination for four weeks, glucose levels decreased at 1 h and 2 h and glucose area under the curve and fructosamine level were significantly decreased (Geohas et al., Citation2007).
As a lipid soluble vitamin whose levels are expected to correlate to an extent with lipid levels, the interpretation of the association that exists between plasma vitamin D and diabetes is complicated (Muscogiuri et al., Citation2011). Nevertheless, daily intake of a vitamin D-fortified yogurt drink (500 IU vitamin D3), either with or without added calcium, improved glycemic status in T2DM patients measured as HOMA-IR (Nikooyeh et al., Citation2011). In contrast, supplementation with cholecalciferol did not improve glycemic control in T2DM whose serum 25-hydroxyvitamin D levels were in the normal range at baseline (Jorde and Figenschau, Citation2009). Similarly, non-T2DM subjects with lower than normal plasma vitamin D levels did not show any difference in glucose homeostasis post glucose tolerance test (Tai et al., Citation2008). Using 2H NMR analysis of monoacetone glucose (MAG) after tracer administration to understand what effect vitamin D3 may have on hepatic glucose metabolism revealed no changes in the percentage contribution of glycogenolysis (O’Sullivan et al., Citation2011). At present there is a lack of evidence to support any intervention with vitamin D on PPG and this was compounded by the early termination of the Thiazolidinedione Intervention with vitamin D Evaluation (TIDE) randomized controlled trial due to concerns over increased cardiovascular events with rosiglitazone (Punthakee et al., Citation2012).
High plasma and cellular nutrients promote the formation of reactive oxygen species (ROS) from membrane NADPH oxidase and mitochondria. It has been proposed that ROS play an important role in insulin resistance and, therefore, vitamins as dietary antioxidants may act as important mitigants of metabolic stress, promoting glucose and fatty acid utilization. Antioxidants frequently act in concert, at least in vitro, each operating within defined redox couples and transferring radicals to yield less reactive/damaging products. The principal dietary antioxidants include a number of vitamins (C-ascorbate, E-tocopherols and A-carotenoids) which are essential for human health, and phytochemicals such as lycopene. Recently, we have undertaken a meta-analysis and shown that HbA1C levels were significantly reduced by antioxidant supplementation, suggesting that antioxidants may have some benefit in protecting against the complications of T2DM (Akbar et al., Citation2011). Our analysis showed that vitamin C or E supplementation did not affect plasma glucose or insulin levels, and here we have supplemented these studies by examining the effect of antioxidant vitamins on postprandial glucose/insulin.
Four studies (Nappo et al., Citation2002; Carroll and Schade Citation2003; Evans et al., Citation2003; Neri et al., Citation2005) were identified that met inclusion criteria for the study investigating antioxidant effects in 77 subjects with all studies incorporating vitamin C, three also including vitamin E and one also including N-acetyl cysteine. Summary information is shown in . Supplement duration varied from immediately prior to the test fatty meal up to 14 days of supplementation prior to meal test. None of the studies reported any benefit on plasma glucose excursions in the postprandial period following antioxidant intervention. More recent interest in vitamin E has been directed toward gamma tocopherol as the active constituent which is reported to have anti-inflammatory and gene regulatory effects. Masterjohn et al. (Citation2012) have investigated effects of vitamin E supplementation enriched with (500 mg day−1) gamma tocopherol on PPG in 12 healthy, normoglycemic, college students. They did not see any benefit for AUC glucose or insulin following five-day ingestion of a supplement enriched in γ-tocopherol.
Dietary Minerals and Blood Glucose Regulation
Deficiencies of several dietary minerals (such as chromium, magnesium, and zinc) have been reported in T2DM subjects. As there is no evidence of beneficial effects of mineral supplementation on diabetic subjects without underlying deficiencies, the following review will be focused on the evidence of three most reported micronutrients on glucose control ().
Chromium. Chromium is a trace mineral which plays an important role in whole body glucose homeostasis by enhancing the binding of insulin to insulin receptor (Cefalu and Hu, Citation2004). Beneficial roles of chromium supplementation on glucose metabolism have been demonstrated in T2DM subjects. For example, chromium supplementation (500 μg/d chromium picolinate) for four-months in T2DM subjects showed a significant reduction of fasting and postprandial glucose levels (Anderson et al., Citation1997; Cheng et al., Citation1999) and this beneficial effect existed after chromium supplementation for a 10-month period. Moreover, an acute chromium supplementation at 400 μg and 800 μg to a white bread meal showed a substantial reduction in PPG in young, apparently healthy adults compared with the white bread meal supplemented with a placebo (Frauchiger et al., Citation2004).
Magnesium. Magnesium is a mineral which plays important roles in glucose transport and, therefore, blood glucose metabolism. A reduced plasma magnesium levels has been often reported in subjects with T2DM. A previous study in young diabetes subjects in Bangladeshis showed that more than half the subjects were hypomagnesemic (Khan et al., Citation1999). Furthermore, a recent cross sectional study showed lowered mean magnesium intake, urine magnesium, plasma magnesium, and erythrocyte magnesium in T2DM subjects with poor glucose control (Sales et al., Citation2011). However, because the lack of randomized interventional studies, the role of magnesium in hyperglycemia in T2DM subjects is not clear and future studies are required.
Zinc. Zinc is a mineral that plays important roles in the synthesis and insulin sensitivity (Roth and Kirchgessner, Citation1981; Park et al., Citation1986; Brun et al., Citation1995). Previous studies have shown that plasma and tissue zinc concentrations in humans and animal models with T2DM are lower than in nondiabetic subjects due to the increased urinary zinc excretion by uncontrolled diabetes (Kinlaw et al., Citation1983). Supplementation of zinc is found to be beneficial in alleviating the hyperglycemia of ob/ob and streptozotocin-induced diabetic mice models (Chan et al., Citation1998; Chen et al., Citation2000). Also, a randomized study evaluated the effect of zinc with other antioxidants on blood glucose in human diabetic subjects on long-term follow-up. They showed that oral zinc sulfate (22 mg/day) supplementation with a multivitamin/mineral preparation (vitamin A, vitamin D3, vitamin E, magnesium, manganese, copper, and selenium) for a four-month period significantly reduced both fasting and postprandial glucose levels in previously diagnosed (for at least two years) subjects from Sri Lanka with T2DM compared to the placebo group (Gunasekara et al., Citation2011). Another study in ob/ob mice also showed that administration (by gavage) of arachidonic acid plus zinc significantly increased glucose disposal in ob/ob mice and effectively decreased blood glucose levels in obese mice from acute treatment (30 min; 3 h) to up to 14 days prolonged treatment (Hwang et al., Citation2002). Further human studies are required to fully evaluate the effect of zinc supplementation alone on glucose metabolism in diabetic subjects.
Impact of Phytochemicals on Blood Glucose Regulation
Phytochemicals are plant secondary metabolites broadly classified according to the pathway from which they are derived. Very few studies have directly attributed specific phytochemicals as to having an effect on PPG. Much of the data discussed concern the observation that a phytochemical-rich food has been effective in modulating the glycemic response and the foods include: berries, nuts, soy, cinnamon, seaweed, tea, ginseng, beans, chocolate, and the herbal extract ‘`salacia’’ (). The phytochemicals associated with fiber are an important and likely effective group and this has been discussed above. The most widely studied phytochemical rich foods studied for their effect on postprandial metabolism are berries, nuts, cocoa, and beverages (tea and coffee). This is due to their strong epidemiological association of these products with cardiovascular health.
The compounds that appear to be of particular importance are phenylpropanoid-derived and comprise predominantly the flavonoids and phenolic acids (). Many preclinical and in vitro studies have shown these molecules can attenuate the postprandial glycemic response, improve acute insulin secretion, and insulin sensitivity and these have been extensively reviewed (Hanhineva et al., Citation2012). No human studies to date have demonstrated a direct effect of the flavonoids or phenolic acids on PPG. However, resveratrol, a phenylpropanoid-derived stilbene found in grape skins has been shown to reduce plasma glucose and improve insulin resistance in volunteers with T2DM, when administered in a capsule (Brasnyo et al., Citation2011). The seed extract of grapes also had an effect on blood glucose regulation by lowering fructosamine in T2DM volunteers (Kar et al., Citation2009). In this study, an improvement in endothelial function, antioxidant status, and C-reactive protein (CRP) was described. Other soft fruits found to decrease plasma glucose levels were fig (Serraclara et al., Citation1998), sea buckthorn berries (Lehtonen et al., Citation2010), and mixed puree containing blackcurrant, bilberry, cranberry, and strawberry (Torronen et al., Citation2010). Again, no direct effect of an individual phytochemical could be attributed. Few human studies have explored vegetables as a potential source of phytochemicals which could effect PPG. A Flaxinius species extract was found to have an acute effect postprandial glucose levels in healthy male volunteers (Visen et al., Citation2009). Another particularly phytochemical rich plant source found to effect PPG in healthy volunteers was seaweed (Goni et al., Citation2000). Seaweed is also a rich source of vitamins, minerals, and amino acids, and the causative effect needs further investigation.
Of the foods containing these phytochemicals, flavan-3-ol rich chocolate and tea are widely studied. In healthy and IGT volunteers (with no symptoms of diabetes) chocolate had an effect on blood glucose levels when in response to an OGTT (Grassi et al., Citation2005, Citation2008), although no effect was observed on insulin sensitivity measured by the glucose clamp method in mild-moderate hypertensive volunteers (Muniyappa et al., Citation2008). Tea is the second most commonly consumed beverage worldwide after water (). Results of epidemiological studies have suggested that consumption of tea (mainly green tea) could lower the risk of T2DM (Iso et al., Citation2006) as well as cardiovascular risk through decrease of CRP (Steptoe et al., Citation2007; Rebello et al., Citation2011), serum amyloid A and haptoglobin (De Bacquer et al., Citation2006). Maki and coauthors did not confirm the link between tea consumption and CRP, but daily users of green tea represented more than 80% of the sample, and they others were potentially coffee drinkers (Maki et al., Citation2010). So, it is difficult to see the clear link with CRP in a population where tea and coffee are consumed daily. Animal studies showed a clear effect of green tea extracts on the improvement of glucose tolerance either in induced diabetic rats or in genetic models of rodents with T2DM (Sae-tan et al., Citation2011). Despite preclinical evidence (Tsuneki et al., Citation2004), green tea, when consumed as a beverage had no effect on postprandial glucose or insulin response when consumed within a meal or one hour prior to a meal in healthy human volunteers (Louie et al., Citation2008; Josic et al., Citation2010). Green tea has been particularly studied for health interest because of its high content on polyphenolic compounds (especially catechins). Consumption of an epigallocatechin gallate (EGCG; > 97%) enriched extract by overweight volunteers also showed no change in glucose levels, insulin sensitivity or secretion (Brown et al., Citation2009). However, there was some correlation of green-tea phenolic intake and insulin levels in healthy humans (Fukino et al., Citation2005) and a catechin-rich green tea increased insulin levels in volunteers with T2DM subjects (Nagao et al., Citation2009). Another cross-sectional study linked green tea consumption to the lowering of fasting blood glucose and fructosamine (Maruyama et al., Citation2009). In a longer term study it was demonstrated that daily supplementation of green tea-extract powder (as an equivalent of 456 mg of catechins daily) for two months lowered the HbA1c levels in subjects with borderline diabetes (Fukino et al., Citation2008) and green tea was inversely associated with plasma C-reactive protein concentrations (Rebello et al., Citation2011). Some potential mechanism of action of the catechins could be the modulation of certain glucose metabolism enzymes in the liver (increase of glucokinase expression and decrease phosphoenolpyruvate carboxykinase), as well as lipid metabolism enzymes in the liver and adipose tissue (acyl-CoA oxidase-1 and carnitinepalmitoyl transferase-1), and potentially on some adipose and muscle glucose receptors (GLUT-4 mRNA or translocation) (Sae-tan et al., Citation2011). Other types of tea are less well studied (i.e., dark tea, Oolong tea). However, it was observed that black tea exhibited a late phase plasma glucose response in healthy humans with a corresponding increase in insulin (Bryans et al., Citation2007). In conclusion, controversial results have been obtained on the consumption of tea and its impact on glucose metabolism. Discrepancy of results may be due to type of tea. However, the interest of phenolic compounds present in green tea seems to be associated with a positive impact of glucose metabolism.
Coffee is among the most widely consumed beverages in the world (). Knowledge on both the positive and negative health effects of coffee has been shown and it has been extensively studied in relation to various diseases (Van Dam and Hu, Citation2005). Several systematic reviews associated the habitual consumption of caffeinated or decaffeinated coffee with a substantially lower risk in T2DM (Van Dam and Hu, Citation2005; Tunnicliffe et al., 2008; Floegel et al., Citation2012). Higher consumption of coffee was consistently associated with a lower prevalence of newly detected hyperglycemia, particularly postprandial hyperglycemia (Van Dam and Hu, Citation2005). Some interesting additional inverse associations were found between coffee consumption and inflammatory markers; CRP, Tumor Necrosis Factor alpha (TNF-α) (Williams et al., Citation2008; Maki et al., Citation2010) and some interleukins (Wedick et al., Citation2011). The potential beneficial chronic effects of coffee may be related to antioxidant compounds including chlorogenic acid (Johnston et al., Citation2003; van Dam Citation2006; Tunniclifeet and Shearer, Citation2008). However, no direct link has been established so far. The acute effect of coffee may be different if consumed within a meal or before the meal, and if it is decaffeinated or caffeinated coffee. It has been shown that caffeinated coffee elicited acute insulin insensitivity when ingested around one hour before a carbohydrate meal compared to decaffeinated coffee (Moisey et al., Citation2008). Additionally, co-ingestion of caffeinated coffee with a high carbohydrate meal increased the blood glucose response, without modifying postprandial insulin response. Three hours later, an OGTT showed decreased insulin sensitivity after the caffeinated coffee compared to water of decaffeinated coffee (Moisey et al., Citation2010). Based on the current knowledge, the controversy between deleterious acute effect and beneficial chronic effect of caffeinated coffee has not been clarified. However, decaffeinated coffee could be another alternative to provide a beneficial impact on the glycemic response.
Nuts in general contain very little available carbohydrate and, therefore, contribute little to the postprandial glycemic response. Addition of nuts (almonds, pistachios, and mixed nuts) to carbohydrate rich foods has been shown to blunt the glycemic response (Jenkins et al., Citation2006) in a dose dependent manner (Josse et al., Citation2007; Kendall et al., Citation2011a, Citation2011b) up to 55% (90 g) in healthy people (). Also, in T2DM subjects, mixed nut intake decreased the PPG response dose dependently, although the effect was half of the effect seen in nondiabetic subjects (Kendall et al., Citation2011a). In another study in T2DM subjects ingestion of 28 g almonds before a high-starch meal lowered PPG by 30%, but did not demonstrate this response in healthy volunteers. In contrast to the former studies, in this study, the control meal contained added fat (butter), and the amount of butter was reduced to compensate for the fat content of the almonds in the treatment meal. In impaired glucose tolerant adults, incorporation of whole almonds into a breakfast meal containing 75 g of carbohydrate also resulted in a lower PPG response and blunted the glucose levels throughout the day. The mechanism of action of nuts on PPG may be due to a reduction in gastric emptying rate as a consequence of the additional energy from fat and protein. However, when the energy and fat contents of meals were controlled, a reduction in PPG was observed (Cohen and Johnston, Citation2011). Alternatively, the phytochemicals in nuts (phytates and phenolics) could play a role by reducing amylase activity, the enzyme that hydrolyzes starch. Addition of nuts to meals resulted in decreased (Jenkins et al., Citation2006) (healthy volunteers) or unchanged (Cohen and Johnston, Citation2011; Mori et al., Citation2011) (IGT, DM2) insulin response and increased insulin levels after a second meal (Mori et al., Citation2011) (IGT). Furthermore, addition of nuts to a meal may result in less oxidative protein damage (Jenkins et al., Citation2006). Only a limited number of longer term trials with nuts on glycemic control have been conducted in people with T2DM. Three relatively small and short-term studies have generally not found significant improvements in markers of glycemic control with nut consumption (for overview, see, Kendall et al., Citation2010). However, a recent pilot trial in which ingestion of one serving of almonds 5 days week−1 resulted in a 4% reduction of HbA1c (Cohen and Johnston, Citation2011). In addition a very recent well-powered study of 117 subjects with T2DM, consumption of mixed nuts (73 g/d) for 12 weeks significantly reduced HbA1c levels and improved blood lipid risk factors for CHD (Jenkins et al., Citation2011). Although, no compounds could be individually attributed to the effects observed, nuts are rich sources of phenolics and phytosterols, as well as macro- (e.g., MUFA) and micronutrients (e.g., α-tocopherol). More studies are required to make definite conclusions on the role of nuts in glycemic control.
With reference to more specific phytochemicals, various herbal extracts have been shown to be effective. This includes Ginseng, both Asian (Panax ginseng) and American (Panax quinquefolius) and Korean Red (Panax ginseng; heat treated) species (). The two most popular types of ginseng, namely Panax quinquefolius L (American ginseng) and Panax ginseng CA Meyer (Asian ginseng) are most widely studied with respect to their hypoglycemic effects. In a series of randomized, placebo controlled studies, American ginseng has been shown to reduce PPG by up to 39% in healthy volunteers (Vuksan et al., Citation2000a, Citation2000b; Vuksan et al., Citation2001; Dascalu et al., Citation2007) and up to 22% in T2DM subjects (Vuksan et al., 2000a; Vuksan et al., Citation2000c). In one study with healthy volunteers, only a trend for hypoglycemic effects was found and one study did not find any effects of American ginseng on PPG (Sievenpiper et al., Citation2003a). The effects of Asian ginseng are mixed, with some studies showing increasing (Sievenpiper et al., Citation2003a), no (Sievenpiper et al., Citation2003a, Citation2003b; Reay et al., Citation2006; Reay et al., Citation2009) or decreasing effects up to 29% (Sievenpiper et al., Citation2006; De Souza et al., Citation2011) on indices of PPG in healthy volunteers. The high variability in efficacy on PPG may be secondary to the variability in the ginseng source (species, batch, preparation, part of the plant, and variety) and its composition, as represented by the measured ginsenoside profile. For example, Korean red ginseng rootlets have been shown to decrease PPG (Vuksan et al., Citation2008), while the root body has not (Sievenpiper et al., Citation2006; De Souza et al., Citation2011). This inconsistency limits the generalizability of the effects from one ginseng source to another and thereby, the use of ginseng as a hypoglycemic agent by subjects with T2DM. However, it has been shown in a so called “acute to chronic testing model” that an acute clinical postprandial model used to select the most efficacious ginsenoside profile, dose, and time of administration is able to identify sources of ginseng with safety and efficacy on long term glycemic parameters in T2DM subjects (Vuksan et al., Citation2000d; Vuksan et al., Citation2003). Two other long-term studies in T2DM subjects, with some (methodological) limitations, also showed positive effects of ginseng on glycemic control (Sotaniemi et al., Citation1995). In 18 healthy volunteers (placebo controlled, double-blind, cross over study), 200 mg Panax ginseng extract during eight weeks had no effect on any gluco-regulatory parameter investigated. (Reay et al., Citation2009) The mechanism behind the hypoglycemic effects of ginseng are unknown but may include modulation of digestion of food and thereby carbohydrate absorption. Modulation of glucose transport and insulin secretion have also been proposed (Vuksan et al., Citation2000a). It is hypothesized that the ginsenosides may be responsible for the effect, but phytochemicals such as the alkaloids or other plant components may be involved. There have also been various studies on cinnamon with no conclusive results. A recent study where a cinnamon extract was administered to healthy volunteers had no effect on PPG (Markey et al., Citation2011). However, several human studies with the herbal extract of Salacia oblonga have demonstrated significant decreases in postprandial glucose levels in both healthy and T2DM subjects (Collene et al., Citation2005; Heacock et al., Citation2004; Williams et al., Citation2007). The mechanism of action is considered to be through competitive inhibition of α-glucosidase activity and two bioactive phytochemicals kotalanol and salacinol have been isolated (Matsuda et al., Citation2005).
The mechanism of action of nonnutrients on PPG is mostly unknown due to poor classification of the phytochemical composition of the foods studied. Potential mechanisms are mostly based on in vitro, preclinical and human ex vivo data. Some general mechanisms that have been proposed include: inhibition/retardation of carbohydrate digestion, modulation of glucose release, uptake and absorption, stimulation of insulin secretion (pancreatic β-cells), activation of receptors, modulation of signaling/gene expression, inhibition of lipolysis, decrease in oxidative stress and inflammation. It may be that the level required of certain plant products may be too high to tolerate. For example, some interventions have been shown to be ineffective at sustainable product levels (Josic et al., Citation2010; Clegg et al., Citation2011) and the food may have to be provided as a purified extract. For this reason, herbs, spices, and certain plant extracts (with high phytochemical content) are likely to be more effective. There is also little consideration regarding the phytochemicals associated with fiber and protein rich plants such as cereal crops and soya. Phytochemicals bound to these fractions can be released within the gastrointestinal tract and influence PPG, but few studies address their metabolism and bioavailability. It is clear that phytochemical-rich foods have potential to influence PPG, but in order to understand the impact of individual components on glucose metabolism it will be essential that the bioactive metabolites are identified.
Impact of Miscellaneous Food Components on Blood Glucose Regulation
Low-calorie Sweeteners and Glycemic Response
People with diabetes need to monitor their carbohydrate intake in order to control blood glucose levels. Low-calorie sweeteners are added to foods, beverages, and used as table-top sweeteners to provide sweetness without the calories or carbohydrates. As a caloric sweetener replacement, they are added in smaller quantities; hence, they provide few or zero calories. Low-calorie sweeteners do not affect the glycemic response and can help control overall carbohydrate intake by substituting for higher energy yielding sweeteners (Duffy and Sigman-Grant, Citation2004; Fitch and Keim, Citation2012).
A recent review (Fernstrom et al., 2012) concluded that ingestion of low-calorie sweeteners by animals and humans does not cause the hypothesized changes in blood glucose or hormone levels. An animal study observed that rats given acesulfame-K saccharin, stevia or sucralose by gavage showed no change in blood concentrations of GLP-1, GIP, and glucose. Also, low-calorie sweeteners by gavage did not influence the rise in blood glucose during an oral glucose tolerance test (Fujita et al., Citation2009). The influence of drinking solutions containing acesulfame-K (165 mg), aspartame (165 mg), cyclamate (800 mg) or saccharin (75 mg) on blood insulin and glucose levels in human volunteers over the following 2 h in comparison with sucrose (30 g) or water controls was investigated (Härtel et al., Citation1993). In comparison with the water control, and in contrast to the dose of sucrose, it was concluded that the ingestion of acesulfame-K, aspartame, cyclamate or saccharin (at moderate doses) did not increase blood glucose and insulin over the 2-h study period.
Further information is available from clinical research on the more recently developed sweeteners such as sucralose and stevioside/rebaudioside A (commonly known as stevia). A high single oral dose of sucralose (1000 mg) given orally to individuals with noninsulin-dependent diabetes mellitus and individuals with insulin-dependent diabetes did not influence plasma C-peptide or glucose concentrations (Mezitis et al., Citation1996). A single oral dose of sucralose (10 mg/kg) did not affect the glucose-induced changes in blood glucose levels when given with a large test dose of sucrose (100 g) (Roberts, Citation1999). Furthermore, a randomized, double-blind, placebo-controlled study in which 128 subjects with T2DM were given either sucralose (667 mg/d for 13 weeks) or placebo showed no effect on fasting plasma glucose, HbA1c or fasting serum C-peptide, demonstrating no effect of chronic ingestion of sucralose on glucose homeostasis (Grotz et al., Citation2003). A similar effect was observed when the low calorie sweetener, stevioside/rebaudioside A was consumed in a chronic study by patients with diabetes (Maki et al., Citation2008).
In summary, the above randomized human studies that varied from 1 to 16 weeks in duration found no significant difference between the effects of low-calorie sweeteners and various comparisons (sucrose, starch or placebo) on standard measures of glycemic and in general did not detect clinically relevant effects (). Furthermore, in the last two years more data have emerged. Anton and colleagues (Citation2010) looked at the effects of preloads containing stevia, aspartame, and sucrose on food intake, satiety, and postprandial glucose and insulin levels in both lean and obese individuals. They concluded that stevia preloads reduced postprandial blood glucose and insulin levels, suggesting stevia may assist with glucose regulation. Raben et al. (Citation2011) looked at importance of exchanging sucrose for sweeteners, on risk factors for diabetes development. Healthy overweight subjects were randomized to consume drinks and foods sweetened with either sucrose or sweeteners as supplements to their usual diet. After 10 weeks, a diet rich in low-calorie sweeteners had no effect on postprandial glucose, insulinemia, and lipidemia. Lastly, a study by Brown (2012) concluded that drinking diet soda could increase the amounts of a hormone that has been previously shown to be beneficial for people with diabetes when it comes to appetite and insulin secretion. More research, however, is needed to determine if this finding may impact future treatment of individuals with diabetes (Brown et al., Citation2012). In conclusion, the use of low-calorie sweeteners and the products that contain them by subjects, with or without diabetes, do not affect blood glucose and are tools to help people reduce and control their caloric intake. Reducing calories could help to attain and maintain a healthy body weight, and thereby lower the risk of heart disease and diabetes. Leading health groups such as American Heart Association, American Diabetes Association (Gardner et al., Citation2012), and the Academy of Nutrition and Dietetics (Fitch and Keim, Citation2012) also agree that substituting low-calorie sweeteners for added sugars in beverages and other foods has the potential to help people reach and maintain a healthy body weight and help people with diabetes with glucose control.
Water and Glycemic Control
Addition of water to a test meal resulted in a 40% increased glucose response in 12 well controlled T2DM subjects (). In poorly controlled diabetic subjects, however, no significant effect was found, probably due to the varying fasting glycemia in these subjects (Torsdottir and Andersson, Citation2009). In another study in T2DM subjects, similar glucose responses were found to test meals with either 90 or 600 mL of tap water (Gregersen et al., Citation1990).
Alcohol and Glycemic Control
Epidemiological studies showed a U-shaped relationship between alcohol consumption and T2DM in western countries (Carlsson et al., Citation2005), showing that moderate alcohol consumption is associated with an increase in insulin sensitivity (Facchini et al., Citation1994; Kiechl et al., Citation1996; Kroenke et al., Citation2003). However, it is also suggested that unhealthy diets which include alcohol consumption are associated to metabolic disease genesis. Alcohol consumption of 10 g was associated with an increase in Low-Density Lipoprotein (LDL) oxidation, which is implicated in the development of cardiovascular disease (Schroder et al., Citation2006). Some inconsistent results may be attributed to the difference in beverage types, drinking frequency, and other dietary factors. The specific interest of phytochemical content in some alcoholic beverages such as wine has been addressed above. In a recent prospective cohort study, moderate alcohol consumption from 0 to 11 g/day of ethanol as beer, wine or liquors has been studied in around 3000 postmenopausal women with T2DM (Rajpathak et al., Citation2010). It was found that an inverse association between quantity and frequency of alcohol intake in relation to incident CHD (Rajpathak et al., Citation2010), was consistent with previous results obtained with nondiabetic postmenopausal women (Joosten et al., Citation2008). In an acute setting, moderate alcohol consumption (around 20 g of alcohol) may decrease the glycemic response when consumed within the meal, or before the meal (around 2 h prior to ingestion), independently of the type of beverage (Brand-Miller et al., Citation2007). However, the consumption of moderate alcohol may not modify the glycemic response of the breakfast when consumed during dinner (Godley et al., Citation2009). One potential mechanism of action is the inhibition of the gluconeogenesis and the decrease of glucose output from the liver, as well as potential inhibition of α-amylase and α-glucosidase. However, this transitory impact of alcohol on glycemia seems to produce deleterious metabolic and hormonal consequence in the long term. Animal studies showed that chronic alcohol consumption increased plasma glucose and serum leptin, resistin, and adiponectin which are involved in insulin resistance genesis (Pravdova et al., Citation2009). Thus, moderate alcohol consumption may have some metabolic interest, including improvement of insulin sensitivity, and the reduction of some cardiovascular risk factors, but some critical questions are pending regarding the potential beneficial quantity, and which type of alcohol.
Vinegar/Acetic Acid and Glycemic Control
The interest on the effects of vinegar on the glycemic response has been studied because of the organic acid content, mainly acetic acid. Several years ago, organic acids such as acetic and propionic acids were shown to lower the glucose and insulin responses when added to white bread meals in healthy subjects (Liljeberg and Bjorck, Citation1998; Darwiche et al., Citation2001; Östman et al., Citation2005). More recently, addition of vinegar in a carbohydrate rich meal decreased significantly the glycemic and insulin responses in T2DM subjects only after a high GI meal (Liatis et al., Citation2010). The blood glucose and insulin kinetics were not modified after the low GI meal. A recent paper did not confirm the decrease of glucose response in a sucrose supplemented rice meal with addition of acetic acid (Mettler et al., Citation2009). Although, the authors could not find a clear explanation to justify this result, it may be due to the elevation of glycemia with rice was not large enough to observe the decremental effect of acetic acid, as some rice have a low GI. The potential mechanism of action proposed for acetic acid to decrease postprandial glucose and insulin responses is by slowing down the gastric emptying phase (Liljeberg and Bjorck, Citation1998; Darwiche et al., Citation2001), but this was not confirmed (Hlebowicz et al., Citation2008). Thus, addition of vinegar or acetic acid in carbohydrate rich meal may have a short-term positive impact by decreasing the postprandial glucose and insulin responses of high GI meals. There is no information regarding this addition in longer term studies.
DISCUSSION
Lifestyle modifications with the highest impact on the risk of diabetes and on blood glucose regulation in the long term are weight reduction and increased habitual physical activity. As for diet composition, the strongest correlation of a dietary component with reduced risk of developing T2DM is with insoluble and moderately fermentable cereal-based fiber (Hu et al., Citation2001; Schulze et al., Citation2007). Diets rich in fruit and vegetables, which are a good source of soluble/fermentable fiber, have also shown a protective effect. However, the evidence is less consistent, particularly if their impact on the risk of T2DM is evaluated independently of their effects on body weight (Cooper et al., Citation2012). Many studies utilize soluble fiber in the context of a healthy diet and these have been shown to potentially play a role in managing daily glucose levels both in healthy individuals and individuals with impaired glucose metabolism. An additional factor, which appears to influence glycemic and insulinemic responses in addition to the amount of fiber in the diet, is the food matrix of carbohydrate rich foods. In particular, soluble fiber has been shown to alter the physical food form, structure, and viscosity of test foods (Tappy et al., Citation1996; Kim et al., Citation2009), impacting on the rate of carbohydrate digestion and absorption, which, in turn, is reflected in the postprandial glucose and insulin responses (Juvonen et al., Citation2011; Karhunen et al., Citation2010). The overall glycemic impact of foods may be markedly affected by the individual meal components. However, when single foods were ranked in relation to their glycemic index, they showed a similar ranking of blood glucose responses when included in a mixed meal (Robert and Ismail Citation2012). In general, the results suggest that carbohydrate foods that promote low but sustained blood glucose levels may be considered advantageous with regard to metabolic control of diabetes (Järvi et al., Citation1999), with acute consumption of foods rich in soluble fiber being beneficial for both postprandial glycemic control and the insulinemic response.
Despite encouraging preclinical studies demonstrating that control of glycemia is likely to involve interaction with gut microbes, evidence from human clinical studies is only just starting to emerge. There are some positive effects of including prebiotics in the habitual diet and a limited number of clinical studies addressing the effect of probiotics on related outcomes such as fasting glycemia and insulin resistance were identified. Amino acids function as nutritional signals regulating various metabolic processes and can stimulate secretion of insulin from the pancreatic β-cells (Newsholme et al., Citation2005), with intravenous infusion of amino acids resulting in increased plasma insulin concentrations (Floyd et al., Citation1966; Floyd et al., Citation1968; Floyd et al., Citation1970). In particular, leucine can strongly stimulate endogenous insulin release and has potential to improve postprandial glycemic control (van Loon et al., Citation2003; Manders et al., Citation2006a, Citation2006b). Protein and/or amino acid co-ingestion can improve PPG (Nuttall et al., Citation1984; Nuttall et al., Citation1985; Gannon et al., Citation1992; Gannon et al., Citation1998; Gannon et al., Citation2001). However, it should be noted that longer term intake of high protein diets has been shown to result in whole-body insulin resistance (Linn et al., Citation2000; Weickert et al., Citation2006). Although these unfavorable effects on insulin resistance could be compensated in the short term by high protein diet induced weight loss, anabolic effects resulting in increased lean/muscle mass, and an amino acid induced increase in insulin secretion in subjects with intact β-cell function, a clearer understanding of the impact of protein consumption on blood glucose levels is required. High fat diets are associated with reduced insulin sensitivity, whereas with lower fat intake the impact appears to be dependent on the fatty acid composition. The data regarding dietary fatty acid modulation strongly supports the benefit of replacement of saturated fats with MUFAs in the diet of T2DM or obese insulin-resistant subjects for the reduction of insulin resistance and improvement of blood glucose levels.
Data regarding the impact of micronutrients on blood glucose regulation is beginning to emerge. Although, there is currently no data supporting the effect of vitamins on blood glucose levels it has been shown that plasma concentrations of B vitamins are reduced in some diabetic populations (Mitri et al., Citation2011; Page et al., Citation2011) and that regular vitamin D intake improved glycemic control in T2DM subjects (Nikooyeh et al., Citation2011). Reduced circulating levels of minerals such as magnesium and zinc have been observed in T2DM subjects (Kinlaw et al., Citation1983) and there is some evidence that mineral supplementation can be effective in improving glucose metabolism (Anderson et al., Citation1997; Cheng et al., Citation1999; Gunasekara et al., Citation2011). However, intervention data from human studies are severely limited. There is a large and increasing body of evidence to suggest that phytochemical-rich foods can improve glucose metabolism. Unfortunately, in general these studies suffer from poor characterization of the phytochemical composition of the foods studied and limited human interventions with single compounds and this decreased the strength of the data obtained.
Based on the evidence presented in this review, it is clear that dietary components can modulate blood glucose levels. Although closely related, fasting and postprandial blood glucose levels are regulated by mechanisms that are to some extent, different. In fact, while postprandial blood glucose concentrations are largely dependent on meal composition, fasting values are only minimally influenced by the amount and/or rate of glucose absorption during the previous meal, and reflect the rate of glucose production in the liver (the two key processes being glycogenolysis and gluconeogenesis). Among the various dietary constituents, the one with the strongest influence on blood glucose levels in the postprandial period is the amount of digestible carbohydrate in the diet. Digestible carbohydrates include monosaccharides (glucose, fructose), disaccharides (sucrose, lactose) and certain polysaccharides (starch), which are digested and absorbed in the human intestine, thus contributing to the glucose in flow to the blood stream. However, the impact of dietary carbohydrates on glucose metabolism depends not only on the amount consumed, as believed in the past, but also on some specific food properties which can profoundly influence the metabolic effects. Among these, also the food structure and some specific physico-chemical characteristics of carbohydrates present in the food can have a relevant role in modulating blood glucose response. The ratio between mono-, di- and polysaccharides is no longer regarded as important in relation to the effects on postprandial blood glucose since amylase and disaccharidase activities in the human duodenum are sufficient to hydrolyse starch and disaccharides within minutes. The meal content of protein and fat, although able to influence postprandial glucose values, has limited practical significance because the magnitude of these effects is rather small.
More important are all dietary factors able to delay the process of digestion and/or absorption of carbohydrates in the intestine, thus reducing the glycemic response to carbohydrate-rich food. Although, the overall mechanisms of action are not yet clear, the nature of the effective dietary components suggests that they are likely to include delayed gastric emptying and accessibility of the available carbohydrates (Thomas and Pfeiffer, Citation2012). As already mentioned, soluble viscous types of fiber are those with the largest impact on the postprandial glucose and insulin response after a meal. The knowledge of nutritional factors influencing blood glucose metabolism in the fasting state is still not complete. Liver glucose production, the major determinant of fasting glucose levels, is under the control of insulin and, therefore, not adequately suppressed when insulin resistance is present; thus nutritional factors influencing fasting plasma glucose concentrations are primarily those acting on insulin resistance. The strongest corroboration of efficacy for dietary components improving glucose metabolism in the fasting state is available for nondigestible carbohydrates (i.e., dietary fiber, resistant starch and fructo-oligosaccarides) and MUFAs (as replacement of saturated fat) with weaker but substantial evidence that certain phytochemicals, predominantly phenolic compounds are likely to be effective. Nondigestible carbohydrates escape digestion in the small intestine and are fermented by colonic bacteria in the large bowel generating SCFAs and other metabolites such as phenolic compounds (CitationLappi et al., in press; Vitaglione et al., Citation2008). This may explain the available, albeit mostly preclinical evidence that suggests that the gut microbiota plays an important role in metabolic regulation of glucose metabolism. These metabolites could influence liver glucose production and, thus, the fasting glucose concentration. Moreover, they act as prebiotics influencing the composition of gut microbiota. Finally, alcohol intake also has significant, clinical effects on plasma glucose levels. In fact, alcohol acutely suppresses hepatic glucose production thus lowering plasma glucose levels. Conversely, if habitually consumed in large amounts it impairs insulin sensitivity, thus deteriorating glucose tolerance and increasing plasma glucose levels.
In conclusion, T2DM is a lifestyle illness that can be managed and prevented by reducing excess body weight, increasing physical exercise and modifying diet composition as outlined in this review. Intensive interventions should be undertaken in individuals at high risk and, in particular, in those with impaired blood glucose regulation, in whom they have proven to be effective. This is especially desirable since high blood glucose levels, even if they are below the diagnostic threshold for diabetes, are also linked with a high risk for CVD. Therefore, lifestyle measures effective in improving blood glucose regulation are useful for prevention of cardiovascular disease as well as of T2DM. New emphasis on prevention might limit the dramatic worldwide increase in the incidence of T2DM expected in the next decades which is also going to impact on health and longevity of the population.
DECLARATION OF INTEREST
Ms Athanasia Baka is employed by ILSI Europe.
ABBREVIATIONS
PPG | = | Postprandial glycemia |
PPI | = | Postprandial insulinaemia |
T2DM | = | Type 2 diabetes mellitus |
GI | = | Glycemic index |
GL | = | Glycemic load |
GP | = | Glycemic profile |
DF | = | Dietary fiber |
HSH | = | Hydrogenated starch hydrolysates |
RS | = | Resistant starch |
SFA | = | Saturated fatty acid |
PUFA | = | Polyunsaturated fatty acid |
MUFA | = | Monounsaturated fatty acid |
TFA | = | Trans fatty acid |
EPA | = | Eicosapentaenoic acid |
DHA | = | Docosahexaenoic acid |
GG | = | Guar gum |
SCFA | = | Short chain fatty acid |
IGT | = | Impaired glucose tolerance |
IFG | = | Impaired fasting glucose |
GLP | = | Glucagon-like peptide |
GPR | = | G-protein couple receptor |
ACKNOWLEDGMENTS
This work was conducted by an expert group of the European branch of the International Life Sciences Institute (ILSI Europe).
The Expert Group is grateful to Professor Massimo Massi-Benedetti for his invaluable advice and guidance throughout the project and to John Sievenpiper and Cyril Kendall for critically reviewing the sections on ginseng and nuts, respectively.
The opinions expressed herein and the conclusions of this publication are those of the authors and do not necessarily represent the views of ILSI Europe nor those of its member companies. Industry members of this task force are listed on the ILSI Europe website at www.ilsi.eu. For further information about ILSI Europe, please email [email protected] or call +32 2 771 00 14.
Funding
The expert group received funding from the ILSI Europe Metabolic Syndrome and Diabetes Task Force.
REFERENCES
- Adams, J. M., Pratipanawatr, T., Berria, R., Wang, E., DeFronzo, R. A., Sullards, M. C. and Mandarino, L. J. (2004). Ceramide content is increased in skeletal muscle from obese insulin-resistant humans. Diabetes. 53:25–31.
- AIM-HIGH Investigators, Boden, W. E., Probstfield, J. L., Anderson, T., Chaitman, B. R., Desvignes-Nickens, P., Koprowicz, K., McBride, R., Teo, K. and Weintraub, W. (2011) Niacin in patients with low HDL cholesterol levels receiving intensive statin therapy. N. Engl. .J Med. 365(24):2255–2267.
- Akbar, S., Bellary, S. and Griffiths, H. R. (2011) Dietary antioxidant interventions in type 2 diabetes patients: A meta-analysis. Br. J. Diabetes Vascular Dis. 11(2):62–66.
- Altschul, R., Hoffer, A. and Stephen, J. (1955). Influence of nicotinic acid on serum cholesterol in man. Arch. Biochem. Biophys. 54:558–559.
- Amar, J., Chabo, C., Waget, A., Klopp, P., Vachoux, C., Bermudez-Humaran, L. G., Smirnova, N., Berge, M., Sulpice, T., Lahtinen, S., et al. (2011a). Intestinal mucosal adherence and translocation of commensal bacteria at the early onset of type 2 diabetes: Molecular mechanisms and probiotic treatment. EMBO Mol. Med. 3:559–572.
- Amar, J., Serino, M., Lange, C., Chabo, C., Iacovoni, J., Mondot, S., Lepage, P., Klopp, C., Mariette, J., Bouchez, O., et al. (2011b). Involvement of tissue bacteria in the onset of diabetes in humans: Evidence for a concept. Diabetologia. 54:3055–3061.
- Anderson, R. A., Cheng, N., Bryden, N. A., Polansky, M. M., Cheng, N., Chi, J. and Feng, J. (1997). Elevated intakes of supplemental chromium improve glucose and insulin variables in individuals with type 2 diabetes. Diabetes. 46(11):1786–1791.
- Andersson, U., Branning, C., Ahrne, S., Molin, G., Alenfall, J., Onning, G., Nyman, M. and Holm, C. (2010). Probiotics lower plasma glucose in the high-fat fed C57BL/6J mouse. Benef. Microbes. 1:189–196.
- Andreasen, A. S., Larsen, N., Pedersen-Skovsgaard, T., Berg, R. M., Moller, K., Svendsen, K. D., Jakobsen, M., and Pedersen, B. K. (2010). Effects of Lactobacillus acidophilus NCFM on insulin sensitivity and the systemic inflammatory response in human subjects. Br. J. Nutr. 104:1831–1838.
- Antal, M., Regöly-Mérei, A., Biró, L., Arató, G., Schmidt, J., Nagy, K., Greiner, E., Lásztity, N., Szabó, C., Péter, S. and Martos, E. (2008). Effects of oligofructose containing diet in obese persons. Orv. Hetil. 149(42):1989–1995.
- Anton, S. D., Martin, C. K., Han, H., Coulon, S., Cefalu, W. T., Geiselman, P. and Williamson, D. A. (2010). Effects of stevia, aspartame, and sucrose on food intake, satiety, and postprandial glucose and insulin levels. Appetite. 55(1):37–43.
- Arumugam, M., Raes, J., Pelletier, E., Le, P. D., Yamada, T., Mende, D. R., Fernandes, G. R., Tap, J., Bruls, T., Batto, J. M., Bertalan, M., Borruel, N., Casellas, F., Fernandez, L., Gautier, L., Hansen, T., Hattori, M., Hayashi, T., Kleerebezem, M., Kurokawa, K., Leclerc, M., Levenez, F., Manichanh, C., Nielsen, H. B., Nielsen, T., Pons, N., Poulain, J., Qin, J. and Sicheritz-Ponten T (2011). Enterotypes of the human gut microbiome. Nature. 473/7346:174–180.
- Atkinson, F. S. and Foster-Powell, K. et al. (2008). International tables of glycemic index and glycemic load values. 2008. Diabetes Care. 31(12):2281–2283.
- Bays, H., Shah, A., Dong, Q., McCrary Sisk, C. and Maccubbin, D. (2011). Extended-release niacin/laropiprant lipid-altering consistency across patient subgroups. Int. J. Clin. Practice. 65:436–445.
- Blaak, E. E., Antoine, J. M., Benton, D., Björck, I., Bozzetto, L., Brouns, F., Diamant, M., Dye, L., Hulshof, T., Holst, J. J., Lamport, D. J., Laville, M., Lawton, C. L., Meheust, A., Nilson, A., Normand, S., Rivellese, A. A., Theis, S., Torekov, S. S. and Vinoy, S. (2012) Impact of postprandial glycaemia on health and prevention of disease. Obes. Rev. 13(10):923–984.
- Blachier, F., Leclercq Meyer, V., Marchand, J., Woussen Colle, M. C., Mathias, P. C., Sener, A., and Malaisse, W. J. (1989). Stimulus-secretion coupling of arginine-induced insulin release. Functional response of islets to L-arginine and L-ornithine. Biochim. Biophys. Acta. 1013:144–151.
- Boesten, R. J. and de Vos, W. M. (2008). Interactomics in the human intestine: Lactobacilli and Bifidobacteria make a difference. J. Clin. Gastroenterol. 42(3):S163–S167.
- Braaten, J. T., Scott, F. W., Wood, P. J., Riedel, K. D., Wolynetz, M. S., Brulé, D., Collins, M. W. (1994). High beta-glucan oat bran and oat gum reduce postprandial blood glucose and insulin in subjects with and without type 2 diabetes. Diabet Med. 11(3):312–318.
- Brady, L. M., Lovegrove, S. S., Lesauvage, S. V., Gower, B. A., Minihane, A.-M., Williams, C. M. and Lovegrove, J. A. (2004). Increased nÂ-6 polyunsaturated fatty acids do not attenuate the effects of long-chain nÂ-3 polyunsaturated fatty acids on insulin sensitivity or triacylglycerol reduction in Indian Asians. Ame. J. Clin. Nutr. 79:983–991.
- Brand-Miller, J. C, Fatima, K., Middlemiss, C., Bare, M., Liu, V., Atkinson, F. and Petocz, P. (2007). Effect of alcoholic beverages on postprandial glycemia and insulinemia in lean, young, healthy adults. Amer. J. Clin. Nutr. 85(6):1545–1551.
- Brasnyo, P., Molnar, G. A., Mohas, M., Marko, L., Laczy, B., Cseh, J., Mikolas, E., Szijarto, I. A., Merei, A., Halmai, R., Meszaros, L. G., Sumegi, B. and Wittmann, I. (2011). Veratrol improves insulin sensitivity, reduces oxidative stress and activates the Akt pathway in type 2 diabetic patients. Br. J. Nutr. 106(3):383.
- Brennan, C. S. (2005). Dietary fibre, glycaemic response, and diabetes. Mol. Nutr. Food Res. 49(6):560–570.
- Brennan, L., Shine, A., Hewage, C., Malthouse, J. P., Brindle, K. M., McClenaghan, N., Flatt, P. R. and Newsholme, P. (2002). A nuclear magnetic resonance-based demonstration of substantial oxidative L-alanine metabolism and L-alanine-enhanced glucose metabolism in a clonal pancreatic beta-cell line: Metabolism of L-alanine is important to the regulation of insulin secretion. Diabetes. 51:1714–1721.
- Brown, A. L., Lane, J., Coverly, J., Stocks, J., Jackson, S., Stephen, A., Bluck, L., Coward, A. and Hendrickx, H. (2009). Effects of dietary supplementation with the green tea polyphenol epigallocatechin-3-gallate on insulin resistance and associated metabolic risk factors: Randomized controlled trial. Br. J. Nutr. 101(6):886–894.
- Brown, R. J., Walter, M. and Rother, K. I. (2012). Effects of DietSoda on gut hormones in youths with diabetes. Diabetes Care. 35:959–964.
- Brun, J. F., Guintrand-Hugret, R., Fons, C. et al. (1995). Effects of oral zinc gluconate on glucose effectiveness and insulin sensitivity in humans. Biol. Trace Elem. Res. 47:385–391.
- Bryans, J. A., Judd, P. A. and Ellis, P. R. (2007). The effect of consuming instant black tea on postprandial plasma glucose and insulin concentrations in healthy humans. J. Amer. Coll. Nutr. 26(5):471–477.
- Cani, P. D., Delzenne, N. M. (2009). The role of the gut microbiota in energy metabolism and metabolic disease. Curr Pharm Des. 15(13):1546–1558.
- Cani, P. D. and Delzenne, N. M. (2011). The gut microbiome as therapeutic target. Pharmacol. Ther. 130:202–212.
- Cani, P. D., Lecourt, E., Dewulf, E. M., Sohet, F. M., Pachikian, B. D., Naslain, D., De, B. F., Neyrinck, A. M. and Delzenne, N. M. (2009). Gut microbiota fermentation of prebiotics increases satietogenic and incretin gut peptide production with consequences for appetite sensation and glucose response after a meal. Am. J. Clin. Nutr. 90:1236–1243.
- Cani, P. D., Rottier, O., Goiot, Y., Neyrinck, A., Geurts, L., Delzenne, N. (2008). Changes in gut microbiota control intestinal permeability-induced inflammation in obese and diabetic mice through unexpected dependent mechanisms. Diabetologia 51:S34–S35.
- Carlsson, S., Hammar, N. and Grill, V. (2005). Alcohol consumption and type 2 diabetes. Meta-analysis of epidemiological studies indicates a U-shaped relationship. Diabetologia. 48:1051–1054.
- Carroll, M. F. and Schade, D. S. (2003). Timing of antioxidant vitamin ingestion alters postprandial proatherogenic serum markers. Circulation. 108:24–31.
- Cefalu, W. and Hu, F. B. (2004). Role of chromium in human health and in diabetes. Diabetes Care. 27(11):2741–2751.
- Ceriello, A. (2004). Impaired glucose tolerance and cardiovascular disease: The possible role of post-prandial hyperglycemia. Am. Heart. J. 147(5):803–807.
- Chandalia, M., Garg, A., Lutjohann, D., von Bergmann, K., Grundy, S. M. and Brinkley, L. J. (2000). Beneficial effects of high dietary Fibre intake in patients with type 2 diabetes mellitus. N. Engl. J. Med. 342:1392–1398.
- Chang, B. J., Park, S. U., Jang, Y. S., Ko, S. H., Joo, N. M., Kim, S. I., Kim, C. H. and Chang DK. (2011). Effect of functional yogurt NY-YP901 in improving the trait of metabolic syndrome. Eur. J. Clin. Nutr. 65:1250–1255.
- Chan, M. D., Liou, S. J., Lin, P. Y. et al. (1998). Effects of zinc sup-plementation on the plasma glucose level and insulin activity in genetically obese (ob/ob) mice. Biol. Trace Elem. Res. 61:303–311.
- Chearskul, S., Sangurai, S., Nitiyanant, W., Kriengsinyos, W., Kooptiwut, S., Harindhanavudhi, T. (2007). Glycemic and lipid responses to glucomannan in Thais with type 2 diabetes mellitus. J Med Assoc Thai. 90(10):2150–2157.
- Cheng, N., Zhu, X., Shi, H., Wu, W., Chi, J., Cheng, J. and Anderson, R. A. (1999). Follow-up survery of people in China with type 2 diabetes mellitus consuming supplemental chromium. Trace Elements Exp. Res. 12:55–60.
- Chen, M. D., Song, Y. M. and Lin, P. Y. (2000). Zinc effects on hyper-glycemia and hypoleptinemia in streptozotocin-induced diabetic mice. Horm. Metab. Res. 32:107–109.
- Chen, J. J., Wang, R., Li, X. F. and Wang RL. (2011). Bifidobacterium longum supplementation improved high-fat-fed-induced metabolic syndrome and promoted intestinal Reg I gene expression. Exp. Biol. Med. (Maywood). 236:823–831.
- Christiansen, E., Schnider, S., Palmvig, B., Tauber-Lassen, E. and Pedersen, O. (1997). Intake of a diet high in trans monounsaturated fatty acids or saturated fatty acids: Effects on postprandial insulinemia and glycemia in obese patients with NIDDM. Diabetes Care. 20:881–887.
- Clegg, M. E., Pratt, M., Meade, C. M. and Henry, C. J. K. (2011). The addition of raspberries and blueberries to a starch-based food does not alter the glycaemic response. Br. J. Nutr. 106(3):335–338.
- Cohen, A. E. and Johnston, C. S. (2011). Almond ingestion at mealtime reduces postprandial glycemia and chronic ingestion reduces hemoglobin A(1c) in individuals with well-controlled type 2 diabetes mellitus. Metabolism. 60:1312–1317.
- Collene, A. L., Hertzler, S. R., Williams, J. A. and Wolf, B. W. (2005). Effects of a nutritional supplement containing Salacia oblonga extract and insulinogenic amino acids on postprandial glycemia, insulinemia, and breath hydrogen responses in healthy adults. Nutrition. 21/7-8:848–854.
- Cooper, A. J., Forouhi, N. G., Ye, Z., Buijsse, B., Arriola, L., Balkau, B., Barricarte, A., Beulens, J. W., Boeing, H., Büchner, F. L., Dahm, C. C., de Lauzon-Guillain, B., Fagherazzi, G., Franks, P. W., Gonzalez, C., Grioni, S., Kaaks, R., Key, T. J., Masala, G., Navarro, C., Nilsson, P., Overvad, K., Panico, S., Ramón Quirós, J., Rolandsson, O., Roswall, N., Sacerdote, C., Sánchez, M. J., Slimani, N., Sluijs, I., Spijkerman, A. M., Teucher, B., Tjonneland, A., Tumino, R., Sharp, S. J., Langenberg, C., Feskens, E. J., Riboli, E., Wareham, N. J., The InterAct Consortium. (2012). Fruit and vegetable intake and type 2 diabetes: EPIC-InterAct prospective study and meta-analysis. Eur. J. Clin. Nutr. 66(10):1082–1092.
- Cozma, A. I., Sievenpiper, J. L., de Souza, R. J., Chiavaroli, L., Ha, V., Wang, D. D., Mirrahimi, A., Yu, M. E., Carleton, A. J., Di Buono, M., Jenkins, A. L., Leiter, L. A., Wolever, T. M., Beyene, J., Kendall, C. W. and Jenkins, D. J. (2012). Effect of fructose on glycemic control in diabetes: A systematic review and meta-analysis of controlled feeding trials. Diabetes Care. 7:1611–1620.
- Darwiche, G., Björgell, O. and Almér LO. (2003). The addition of locust bean gum but not water delayed the gastric emptying rate of a nutrient semisolid meal in healthy subjects. BMC Gastroenterol. 6(3):12.
- Darwiche, G., Stman, E. M., Liljeberg, H. G. M., Kallinen, N., Björgell, O., Björck, I. M. E. and Almer, L.-O. (2001). Measurements of the gastric emptying rate by use of ultrasonography: Studies in humans using bread with added sodium propionate. Am. J. Cli. Nutr. 74:254–258.
- Dascalu, J. L., Sievenpiper, A. L., Jenkins, M. P., Stavro, L. A., Leiter, J. T., Arnason, et al. (2007). Five batches representative of Ontario-grown American ginseng root produce comparable reductions of postprandial glycemia in healthy individuals. Can. J. Physiol. Pharmacol. 85:856–864.
- Daubioul, C. A., Horsmans, Y., Lambert, P., Danse, E., and Delzenne, N. M. Effects of oligofructose on glucose and lipid metabolism in patients with nonalcoholic steatohepatitis: results of a pilot study. Eur. J. Clin. Nutr. 59, 723–726 (2005).
- Dean, P. M., and Matthews, E. K. (1970). Glucose-induced electrical activity in pancreatic islet cells. J. Physiol. 210:255–264.
- De Bacquer, D., Clays, E., Delanghe, J. and De Backer, G. (2006). Epidemiological evidence for an association between habitual tea consumption and markers of chronic inflammation. Atherosclerosis. 189(2):428–435.
- Delzenne, N. M. and Cani, P. D. (2011a). Gut microbiota and the pathogenesis of insulin resistance. Curr. Diabetes Rep. 11(3):154–159.
- Delzenne, N. M. and Cani, P. D, (2011b). Interaction between obesity and the gut microbiota: Relevance in nutrition. Annu. Rev. Nutr. 31:15–31.
- Delzenne, N. M., Neyrinck, A. M., Bäckhed, F. and Cani, P. D (2011). Targeting gut microbiota in obesity: Effects of prebiotics and probiotics. Nat. Rev. Endocrinol. 7/11:639–646.
- Del Toma, E., Lintas, C., Clementi, A., Marcelli, M. (1988a). Soluble and insoluble dietary fibre in diabetic diets. Eur J Clin Nutr.42(4):313–319.
- Del Toma, E., Lintas, C., Clementi, A., Marcelli, M. Cappelloni, M., Lintas, C. (1988b). Food fiber choices for diabetic diets. Am J Clin Nutr. 47(2):243–246.
- de Munter, J. S., Hu, F. B., Spiegelman, D., Franz, M. and van Dam, R. M. (2007). Whole grain, bran, and germ intake and risk of type 2 diabetes: A prospective cohort study and systematic review. PLoS Med. 4:e261.
- de Natale, C., Annuzzi, G., Bozzetto, L., Mazzarella, R., Costabile, G., Ciano, C., Riccardi, G. and Rivellese, A. A. (2009). Effects of a plant-based high-carbohydrate/high-Fibre diet versus high–monounsaturated fat/low-carbohydrate diet on postprandial lipids in type 2 diabetic patients. Diabetes Care. 32:2168–2173.
- De Souza, L. R., Jenkins, A. L., Sievenpiper, J. L., Jovanovski, E., Rahelic, D. and Vuksan, V. (2011). Korean red ginseng (Panax ginseng C.A. Meyer) root fractions: Differential effects on postprandial glycemia in healthy individuals. J. Ethnopharmacol. 137:245–250.
- Devaraj, S., Wang-Polagruto, J., Polagruto, J., Keen, C. L. and Jialal, I. (2008). High-fat, energy-dense, fast-food—style breakfast results in an increase in oxidative stress in metabolic syndrome. Metab. Clin. Experl. 57:867–870.
- Devaraj, S., Wang-Polagruto, J., Polagruto, J., Keen, C. L., Jialal, I. (2008). High-fat, energy-dense, fast-food-style breakfast results in an increase in oxidative stress in metabolic syndrome. Metabolism. 57(6):867–870
- Diabetes Control and Complications Trial (DCCT) Research Group. (1993) The effect of intensive treatment of diabetes on the development and progression of long-term complications in insulindependent diabetes mellitus. N. Engl. J. Med. 329/14:977–986.
- Diamant, M., Blaak, E. E. and de Vos, W. M. (2011). Do nutrient-gut-microbiota interactions play a role in human obesity, insulin resistance and type 2 diabetes? Obes. Rev. 12(4):272–281.
- Dikeman, C. L. and Fahey, G. C. J. (2006). Viscosity as related to dietary Fibre: A review. Crit. Rev. Food. Sci. Nutr. 46:649–663.
- Duffy, V. B. and Sigman-Grant, M. (2004). Position of the American Dietetic Association. Use of nutritive and non-nutritive sweeteners. J. Amer. Dietetic. Assoc. 104:255–275.
- Dunne, M. J., Yule, D. I., Gallacher, D. V. and Petersen, O. H. (1990). Effects of alanine on insulin-secreting cells: Patch-clamp and single cell intracellular Ca2 +measurements. Biochim. Biophys. Acta. 1055:157–164.
- d’Emden, M. C., Marwick, T. H., Dreghorn, J., Howlett, V. L. and Cameron, D. P. (1987). Post-prandial glucose and insulin responses to different types of spaghetti and bread. Diabetes Res. Clin. Pract. 4:221–226.
- Eastwood, M. A. and Morris, E. R. (1992). Physical properties of dietary fibre that influence physiological function: A model for polymers along the gastrointestinal tract. Am. J. Clin. Nutr. 55:436–442.
- Ejtahed, H. S., Mohtadi-Nia, J., Homayouni-Rad, A., Niafar, M., Asghari-Jafarabadi, M. and Mofid, V. (2012). Probiotic yogurt improves antioxidant status in type 2 diabetic patients. Nutrition. 28:539–543.
- Ejtahed, H. S., Mohtadi-Nia, J., Homayouni-Rad, A., Niafar, M., Asghari-Jafarabadi, M., Mofid, V. and Akbarian-Moghari, A. (2011). Effect of probiotic yogurt containing Lactobacillus acidophilus and Bifidobacterium lactis on lipid profile in individuals with type 2 diabetes mellitus. J. Dairy Sci. 94:3288–3294.
- Elin Östmana, E., Rossia, E., Larssonc, H., Brighentib, F. and Björck, I. (2006). Glucose and insulin responses in healthy men to barley bread with different levels of (1→3;1→4)-β-glucans; predictions using fluidity measurements of in vitro enzyme digests. J. Cereal. Sci. 43(2):230–235.
- Ellis, P. R., Dawoud, F. M. et al. (1991). Blood glucose, plasma insulin and sensory responses to guar-containing wheat breads: Effects of molecular weight and particle size of guar gum. Br. J. Nutr. 66(3):363–379.
- Evans, M., Anderson, R. A., Smith, J. C., Khan, N., Graham, J. M., Thomas, A. W., Morris, K., Deely, D., Frenneaux, M. P., Davies, J. S. and Rees, A. (2003). Effects of insulin lispro and chronic vitamin C therapy on postprandial lipaemia, oxidative stress and endothelial function in patients with type 2 diabetes mellitus. Eur. J. Clin. Inv. 33:231–238.
- Everard, A., Lazarevic, V., Derrien, M., Girard, M., Muccioli, G. G., Neyrinck, A. M., Possemiers, S., Van Holle, A., François, P., de Vos, W. M., Delzenne, N. M., Schrenzel, J. and Cani, P. D. (2011). Responses of gut microbiota and glucose and lipid metabolism to prebiotics in genetic obese and diet-induced leptin-resistant mice. Diabetes. 60(11):2775–2786.
- Facchini, F., Chen, Y. D. and Reaven, G. M. (1994). Light-to-moderate alcohol intake is associated with enhanced insulin sensitivity. Diabetes Care. 17(2):115–119.
- FAO/WHO. (2002). Guidelines for the Evaluation of Probiotics in Food. Joint FAO/WHO Working Group Report on Drafting for the Evaluation of Probiotics in Food. London, Ontario, Canada
- Fernstrom, J. D., Munger, S. D., Sclafani, A., de Araujo, I. E., Roberts, A. and Molinary, S. (2012). Mechanisms for sweetness. J Nutr. 142(6):1134S–1141S.
- Fitch, C. and Keim, K. S. (2012). Academy of Nutrition and Dietetics. Position of the Academy of Nutrition and Dietetics: Use of nutritive and nonnutritive sweeteners. J. Acad. Nutr. Diet. 112(5):739–758.
- Flammang, A. M., Kendall, D. M., Baumgartner, C. J., Slagle, T. D., Choe, Y. S. (2006). Effect of a viscous fiber bar on postprandial glycemia in subjects with type 2 diabetes. J Am Coll Nutr. 25(5):409–414.
- Floegel, A., Pischon, T., Bergmann, M. M., Teucher, B., Kaaks, R. and Boing, H. (2012). Coffee consumption and risk of chronic disease in European Prospective Investigation into Cancer and Nutrition (EPIC)-Germany study. Am. J. Clin. Nutr. 95(4):901–908.
- Floyd, J. C., Jr., Fajans, S. S., Conn, J. W., Knopf, R. F. and Rull, J. (1966). Stimulation of insulin secretion by amino acids. J. Clin. Invest. 45:1487–1502.
- Floyd, J. C., Jr., Fajans, S. S., Conn, J. W., Thiffault, C., Knopf, R. F. and Guntsche, E. (1968). Secretion of insulin induced by amino acids and glucose in diabetes mellitus. J. Clin. Endocrinol. Metab. 28:266–276.
- Floyd, J. C., Jr., Fajans, S. S., Knopf, R. F. and Conn, J. W. (1963). Evidence that insulin release is the mechanism for experimentally induced leucine hypoglycemia in man. J. Clin. Invest. 42:1714–1719.
- Floyd, J. C., Jr., Fajans, S. S., Pek, S., Thiffault, C. A., Knopf, R. F. and Conn, J. W. (1970). Synergistic effect of essential amino acids and glucose upon insulin secretion in man. Diabetes. 19:109–115.
- Frauchiger, M. T., Wenk, C. and Colombani, P. C. (2004). Effects of acute chromium supplementation on postprandial metabolism in healthy young men. J. Am. Coll. Nutr. 23(4):351–357.
- Frid, A. H., Nilsson, M., et al. (2005). “Effect of whey on blood glucose and insulin responses to composite breakfast and lunch meals in type 2 diabetic subjects.” Amer. J. Clin. Nutr. 82(1):69–75.
- Fujita, Y., Wideman, R. D., Speck, M., Asadi, A., King, D. S., Webber, T. D., Haneda, M. and Kieffer, T. J. (2009). Incretin release from gut is acutely enhanced by sugar but not by sweeteners in vivo. Am. J. Physiol. Endocrinol. Metab. 296:E473–E479.
- Fukino, Y., Ikeda, A., Maruyama, K., Aoki, N., Okubo T and Iso, H. (2008). Randomized controlled trial for an effect of green tea-extract powder supplementation on glucose abnormalities. Eur. J. Clin. Nutr. 62:953–960.
- Fukino, Y., Shimbo, M., Aoki, N., Okubo, T. and Iso, H. (2005). Randomized controlled trial for an effect of green tea consumption on insulin resistance and inflammation markers. J. Nutr. Sci. Vitaminol. (Tokyo). 51(5):335–342.
- Furet, J. P., Kong, L. C., Tap, J., Poitou, C., Basdevant, A., Bouillot, J. L., Mariat, D., Corthier, G., Dore, J., Henegar, C., Rizkalla, S. and Clement, K. (2010). Differential adaptation of human gut microbiota to bariatric surgery-induced weight loss: Links with metabolic and low-grade inflammation markers. Diabetes. 59:3049–3057.
- Gannon, M. C., Nuttall, J. A., Damberg, G., Gupta, V. and Nuttall, F. Q. (2001). Effect of protein ingestion on the glucose appearance rate in people with type 2 diabetes. J. Clin. Endocrinol. Metab. 86:1040–1047.
- Gannon, M. C., Nuttall, F. Q., Grant, C. T., Ercan-Fang, S. and Ercan-Fang, N. (1998). Stimulation of insulin secretion by fructose ingested with protein in people with untreated type 2 diabetes. Diabetes Care. 21:16–22.
- Gannon, M. C., Nuttall, F. Q., Lane, J. T. and Burmeister, L. A. (1992). Metabolic response to cottage cheese or egg white protein, with or without glucose, in type II diabetic subjects. Metabolism. 41:1137–1145.
- Gao, D., Griffiths, H. R. and Bailey, C. J. (2009). Oleate protects against palmitate-induced insulin resistance in L6 myotubes. Br. J. Nutr. 102:1557–1563.
- Gao, D., Pararasa, C., Dunston, C. R., Bailey, C. J. and Griffiths, H. R. (2012). Palmitate promotes monocyte atherogenicity via de novo ceramide synthesis. Free Rad. Biol. Med. 53:796–806.
- Garcia, A.L. et al. (2007). Arabinoxylan consumption decreases postprandial serum glucose, serum insulin and plasma total ghrelin response in subjects with impaired glucose tolerance. Eur. J. Clin. Nutr. 61, 334–341.
- Gardner, C., Wylie-Rosett, J., Gidding, S. S., Steffen, L. M., Johnson, R. K., Reader, D. and Lichtenstein, A. H. on behalf of American Heart Association Nutrition Committee of the Council on Nutrition, Physical Activity and Metabolism, Council on Arteriosclerosis, Thrombosis and Vascular Biology, Council on Cardiovascular Disease in the Young; American Diabetes Association (2012). Nonnutritive sweeteners: Current use and health perspectives. A scientific statement from the American Heart Association and the American Diabetes Association. Diabetes Care. 35(8):1798–1808.
- Gatenby, S. J., Ellis, P. R., Morgan, L. M., Judd, P. A. (1996). Effect of partially depolymerized guar gum on acute metabolic variables in patients with non-insulin-dependent diabetes. Diabet Med. 13(4):358–364.
- Geohas, J., Daly, A., Juturu, V., Finch, M. and Komorowski, J. (2007). Chromium picolinate and biotin combination reduces atherogenic index of plasma in patients with type 2 diabetes mellitus: A placebo-controlled, double-blinded, randomized clinical trial. Amer. J. Medl. Sci. 333:145–153.
- Gerss, J., K. W. (2009). The questionable association of vitamin E supplementation and mortality–Inconsistent results of different meta-analytic approaches. Cell. Mol. Biol. (Noisy-le-grand). 55:1111–1120.
- Giacco, R., Clemente, G., Luongo, D., Lasorella, G., Fiume, I., Brouns, F., Bornet, F., Patti, L., Cipriano, P., Rivellese, A. A. and Riccardi, G. (2004). Effects of short-chain fructo-oligosaccharides on glucose and lipid metabolism in mild hypercholesterolaemic individuals. Clin. Nutr. 23(3):331–340.
- Gibson, G. R. and Roberfroid, M. B. (1995). Dietary modulation of the human colonic microbiota: Introducing the concept of prebiotics. J. Nutr. 125(6):1401–1412.
- Gilbertson, H. R., Brand-Miller, J. C. et al. (2001). The effect of flexible low glycemic index dietary advice versus measured carbohydrate exchange diets on glycemic control in children with type 1 diabetes. Diabetes Care. 24(7):1137–1143.
- Godley, R., Brown, R. C., Williams, S. M. and Green, T. J. (2009). Moderate alcohol consumption the night before glycaemic index testing has no effect on glycaemic response. Eur. J. Clin. Nutr. 63:692–694.
- Golay, A., Schneider, H. et al. (1995). The effect of a liquid supplement containing guar gum and fructose on glucose-tolerance in non-insulin-dependent diabetic-patients. Nutr. Met. Cardiovascular Dis. 5/2:141–148.
- Goldberg, R. B. and Jacobson, T. A. (2008). Effects of niacin on glucose control in patients with dyslipidemia. Mayo. Clinic. Proc. 83:470–478.
- Goni, I., Valdivieso, L. and Garcia-Alonso, A. (2000). Nori seaweed consumption modifies glycemic response in healthy volunteers. Nutr. Res. 20(10):1367–1375.
- Gosby, A. K., Conigrave, A. D., Lau, N. S., Iglesias, M. A., Hall, R. M., Jebb, S. A., Brand-Miller, J., Caterson, I. D., Raubenheimer, D. and Simpson, S. J. (2011). Testing protein leverage in lean humans: A randomised controlled experimental study. PLoS One. 6:e25929.
- Granfeldt, Y. E. and Bjorck, I. M. (2011) A bilberry drink with fermented oatmeal decreases postprandial insulin demand in young healthy adults. Nutr. J. 10:57.
- Granfeldt, Y., Drews, A. et al. (1995). Arepas made from high amylose corn flour produce favorable low glucose and insulin responses in healthy humans. J. Nutr. 125(3):459–465.
- Granfeldt, Y., Eliasson, A.-C. et al. (2000). An examination of the possibility of lowering the glycemic index of oat and barley flakes by minimal processing. J. Nutr. 130(9):2207–2214.
- Granfeldt, Y., Hagander, B. et al. (1995). Metabolic responses to starch in oat and wheat products. On the importance of food structure, incomplete gelatinization or presence of viscous dietary fibre. Eur. J. Clin. Nutr. 49:189–199.
- Granfeldt, Y., Nyberg, L. and Björck, I. (2008). Muesli with 4 g oat beta-glucans lowers glucose and insulin responses after a bread meal in healthy subjects. Eur. J. Clin. Nutr. 62(5):600–607.
- Grassi, D., Desideri, G., Necozione, S., Lippi, C., Casale, R., Properzi, G., Blumberg, J. B. and Ferri, C. (2008). Blood pressure is reduced and insulin sensitivity increased in glucose-intolerant, hypertensive subjects after 15 days of consuming high-polyphenol dark. J. Nutr. 138(9):1671–1676.
- Grassi, D., Lippi, C., Necozione, S., Desideri, G. and Ferri, C. (2005). Short-term administration of dark chocolate is followed by a significant increase in insulin sensitivity and a decrease in blood pressure in healthy persons. Amer. J. Clin. Nutr. 81(3):611–614.
- Gregersen, S., Rasmussen, O., Winther, E. and Hermansen, K. (1990). Water volume and consumption time: Influence on the glycemic and insulinemic responses in non-insulin-dependent diabetic subjects. Am. J. Clin. Nutr. 52:515–518.
- Grotz, V. L., Henry, R. F., McGill, J. B., Prince, M. J., Shamoon, H., Trout, J. R. and Pi-Sunyer, F. X. (2003). Lack of effect of sucralose on glucose homeostasis in subjects with type 2 diabetes. J. Am. Diet. Assoc. 103:1607–1612.
- Guévin, N., Jacques, H., Nadeau, A., and Galibois, I. (1996). Postprandial glucose, insulin, and lipid responses to four meals containing unpurified dietary fiber in non-insulin-dependent diabetes mellitus (NIDDM), hypertriglyceridemic subjects. J Am Coll Nutr. 15(4):389–396.
- Guillon, F. and Champ, M. (2000). Structural and physical properties of dietary fibres and consequences of processing on human physiology. Food Res. Int. 33:233–245.
- Gunasekara, P., Hettiarachchi, M., Liyanage, C. and Lekamwasam, S. (2011). Effects of zinc and multimineral vitamin supplementation on glycemic and lipid control in adult diabetes. Diabetes Metab. Syndr. Obes. 26(4):53–60.
- Gunnerud, U. J., Heinzle, C., Holst, J. J., Östman, E. M. and Björck, I. M. (2012a). Effects of pre-meal drinks with protein and amino acids on glycemic and metabolic responses at a subsequent composite meal. PLoS One. 7(9):e44731.
- Gunnerud, U. J., Heinzle, C., Holst, J. J., Östman, E. M. and Björck, I. M. (2012b). Effects of pre-meal drinks with protein and amino acids on glycemic and metabolic responses at a subsequent composite meal. PLoS One. 7(9):e44731.
- Gögebakan, O., Kohl, A., Osterhoff, M. A., van Baak, M. A., Jebb, S. A., Papadaki, A., Martinez, J. A., Handjieva-Darlenska, T., Hlavaty, P., Weickert, M. O., Holst, C., Saris, W. H., Astrup, A. and Pfeiffer, A. F. (2011). Effects of weight loss and long-term weight maintenance with diets varying in protein and glycemic index on cardiovascular risk factors: The diet, obesity, and genes (DiOGenes) study: A randomized, controlled trial. Circulation. 124:2829–2838.
- Haber, G. B., Heaton, K. W. et al. (1977). Depletion and disruption of dietary fibre, effects on satiety, plasma-glucose, and serum-insulin. Lancet. 679–682.
- Haffner, S. M., Lehto, S., Ronnemaa, T., Pyorala, K. and Laakso, M. (1998). Mortality from coronary heart disease in subjects with type 2 diabetes and in nondiabetic subjects with and without prior myocardial infarction. N. Engl. J. Med. 339(4):229–234.
- Hagander, B., Scherstén, B., Asp, N. G., Sartor, G., Agardh, C. D., Schrezenmeir, J., Kasper, H., Ahrén, B., Lundquist, I. (1984). Effect of dietary fibre on blood glucose, plasma immunoreactive insulin, C-peptide and GIP responses in non insulin dependent (type 2) diabetics and controls. Acta Med Scand. 215(3):205–213
- Hallstrom, E., Sestili, F. et al. (2011). A novel wheat variety with elevated content of amylose increases resistant starch formation and may beneficially influence glycaemia in healthy subjects. Food Nutr. Res. 55:doi: 10.3402/fnr.v55i0.7074.
- Hanhineva, K., Torronen, R., Bondia-Pons, I., Pekkinen, J., Kolehmainen, M., Mykkanan, H. and Poutanen, K. (2012). Impact of dietary polyphenols on carbohydrate metabolism. Int. J. Mol. Sci. 11:1365–1402.
- Heacock, P. M., Hertzler, S. R., Williams, J. A. and Wolf, B. W. (2004). Effects of a medical food containing an herbal alpha-glucosidase inhibitor on postprandial glycemia and insulinemia in healthy adults. J. Amer. Diatetic Ass. 105(1):65–71.
- Heianza, Y., Hara, S., Arase, Y., Saito, K., Fujiwara, K., Tsuji, H., Kodama, S., Hsieh, S. D., Mori, Y., Shimano, H., Yamada, N., Kosaka, K. and Sone, H. (2011). HbA1c 5·7–6·4% and impaired fasting plasma glucose for diagnosis of prediabetes and risk of progression to diabetes in Japan (TOPICS 3): A longitudinal cohort study. Lancet. 9786:147–155.
- Hession, M., Rolland, C., Kulkarni, U., Wise, A. and Broom, J. (2009). Systematic review of randomized controlled trials of low-carbohydrate vs. low-fat/low-calorie diets in the management of obesity and its comorbidities. Obes. Rev. 10:36–50.
- Hlebowicz, J., Lindstedt, S., Björgell, O., Höglund, P., Almér, L. O. and Darwiche, G. (2008). The botanical integrity of wheat products influences the gastric distention and satiety in healthy subjects. Nutr. J. 27:7–12.
- Hooper, L. V., Wong, M. H., Thelin, A., Hansson, L., Falk, P. G. and Gordon, J. I.(2001). Molecular analysis of commensal host-microbial relationships in the intestine. Science. 291:881–884.
- Hu, F. B., Manson, J. E., Stampfer, M. J., Colditz, G., Liu, S., Solomon, C. G. and Willett, W. C. (2001). Diet, lifestyle, and the risk of type 2 diabetes mellitus in women. N. Engl. J. Med. 345:790–797.
- Huxley, R., Barzi, F. and Woodward, M. (2006). Excess risk of fatal coronary heart disease associated with diabetes in men and women: Meta-analysis of 37 prospective cohort studies. Br. Med. J. 332/7533:73–78.
- Hwang, I. K., Go, V. L., Harris, D. M., Yip, I. and Song, M. K. (2002). Effects of arachidonic acid plus zinc on glucose disposal in genetically diabetic (ob/ob) mice. Diabetes Obes. Metab. 4/2:124–131.
- Härtel, B., Graubaum, H. L. and Schneider, B. (1993). The influence of sweetener solutions on the secretion of insulin and the blood glucose level. Ernährungsumschau. 40:152–155.
- Iso, H., Date, C., Wakai, K., Fukui, M. and Tamakoshi, A. (2006). The relationship between green tea and total caffeine intake and risk for self-reported type 2 diabetes among Japanese adults. Ann. Intern. Med. 144(8):554–562.
- Jans, A., Konings, E., Goossens, G. H., Bouwman, F. G., Moors, C. C., Boekschoten, M. V., et al. (2012). PUFAs acutely affect triacylglycerol-derived skeletal muscle fatty acid uptake and increase postprandial insulin sensitivity. Amer. J. Clin. Nutr. 95(4):825–836.
- Jenkins, D. J., Goff, D. V., Leeds, A. R., Alberti, K. G., Wolever, T. M., Gassull, M. A., Hockaday, T. D. (1976). Unabsorbable carbohydrates and diabetes: Decreased post-prandial hyperglycaemia. Lancet. 4;2(7978):172–174.
- Jenkins, A. L., Jenkins, D. J., Zdravkovic, U., Würsch, P., Vuksan V. (2002). Depression of the glycemic index by high levels of beta-glucan fiber in two functional foods tested in type 2 diabetes. Eur J Clin Nutr. 56(7):622–628.
- Jenkins, A. L., Jenkins, D. J., Wolever, T. M., Rogovik, A. L., Jovanovski, E., Bozikov, V., Rahelić, D., Vuksan, V. (2008). Comparable postprandial glucose reductions with viscous fiber blend enriched biscuits in healthy subjects and patients with diabetes mellitus: acute randomized controlled clinical trial. Croat Med J. 49(6):772–782.
- Jenkins, D. J., Kendall, C. W., Banach, M. S., Srichaikul, K., Vidgen, E., Mitchell, S., Parker, T., Nishi, S., Bashyam, B., de Souza, R., Ireland, C. and Josse, R. G. (2011). Nuts as a replacement for carbohydrates in the diabetic diet. Diabetes Care. 34:1706–1711.
- Jenkins, D. J., Kendall, C. W., Josse, A. R., Salvatore, S., Brighenti, F., Augustin, L. S. et al. (2006). Almonds decrease postprandial glycemia, insulinemia, and oxidative damage in healthy individuals. J. Nutr. 136:2987–2992.
- Jenkins, D. J., Kendall, C. W., McKeown-Eyssen, G., et al. (2008a). Effect of a low glycemic index or a high-cereal Fibre diet on type 2 diabetes: A randomized trial. JAMA. 300:2742–2753.
- Jenkins, D. J., Kendall, C. W., McKeown-Eyssen, G., Josse, R. G., Silverberg, J., Booth, G. L., Vidgen, E., Josse, A. R., Nguyen, T. H., Corrigan, S., Banach, M. S., Ares, S. and Mitchell, S. (2008b). Effect of a low-glycemic index or a high-cereal fiber diet on type 2 diabetes: A randomized trial. Jenkins, JAMA. 23:2742–2753.
- Johnson, R. K., Appel, L. J., Brands, M., Howard, B. V., Lefevre, M., Lustig, R., Sacks, F., Steffen, L. M. and Judith Wylie-Rosett, J. on behalf of the American Heart Association Nutrition Committee of The Council on AHA. (2009). Dietary sugars intake and cardiovascular health: A scientific statement from the Amer Heart Association. Circulation. 120:1011–1020.
- Johnston, K. L., Clifford, M. N. and Morgan, L. M. (2003). Coffee acutely modifies gastrointestinal hormone secretion and glucose tolerance in humans: Glycemic effects of chlorogenic acid and caffeine. Amer. J. Clin. Nutr. 78(4):728–733.
- Johnston, K. L., Thomas, E. L., Bell, J. D., Frost, G. S. and Robertson, M. D. (2010). Resistant starch improves insulin sensitivity in metabolic syndrome. Diabet Med. 27:391–397.
- Joosten, M. M., Beulens, J. W., Kersten, S. and Hendriks, H. F. (2008). Moderate alcohol consumption increases insulin sensitivity and ADIPOQ expression in postmenopausal women: A randomised, crossover trial. Diabetologia. 51:1375–1381.
- Jorde, R. and Figenschau, Y. (2009). Supplementation with cholecalciferol does not improve glycaemic control in diabetic subjects with normal serum 25-hydroxyvitamin D levels. Eur J Nutr. 48:349–354.
- Josic, J., Olsson, A. T., Wickeberg, J., Lindstedt, S. and Hlebowicz, J. (2010). Does green tea affect postprandial glucose, insulin and satiety in healthy subjects: A randomized controlled trial. Nutr. J. 9:63.
- Josic, J., Olsson, A. T., Wickeberg, J., Lindstedt, S. and Hlebowicz, J. (2010). Does green tea affect postprandial glucose, insulin and satiety in healthy subjects: A randomized controlled trial. Nutr. J. 9:63.
- Josse, A. R., Kendall, C. W., Augustin, L. S., Ellis, P. R. and Jenkins, D. J. (2007). Almonds and postprandial glycemia—A dose-response study. Metabolism. 56:400–404.
- Juntunen, K. S., Laaksonen, D. E., Autio, K., Niskanen, L. K., Holst, J. H., Savolainen, K. H., Liukkonen, K.-H., Poutanen, K. S. and Mykkänen, H. M. (2003). Structural differences between rye and wheat bread but not total fiber content may explain the lower postprandial insulin response to rye bread. Am. J. Clin. Nutr. 78:957–964.
- Juvonen, K. R., Salmenkallio-Marttila, M., Lyly, M., Liukkonen, K. H., Lähteenmäki, L., Laaksonen, D. E., Uusitupa, M. I., Herzig, K. H., Poutanen, K. S. and Karhunen, L. J. (2011). Semisolid meal enriched in oat bran decreases plasma glucose and insulin levels, but does not change gastrointestinal peptide responses or short-term appetite in healthy subjects. Nutr. Metab. Cardiovasc Dis. 21:748–756.
- Järvi, A. E., Karlström, B. E. et al. (1995). The influence of food structure on postprandial metabolism in patients with non-insulin-dependent diabetes mellitus. Am. J. Clin. Nutr. 61:837–842.
- Järvi, A. E., Karlström, B. E. et al. (1999). Improved glycemic control and lipid profile and normalized fibrinolytic activity on a low-glycemic index diet in type 2 diabetic patients. Diabetes Care. 22(1):10–18.
- Karhunen, L. J., Juvonen, K. R., Flander, S. M., Liukkonen, K. H., Lähteenmäki, L., Siloaho, M., Laaksonen, D. E., Herzig, K. H., uusitupa, M. I. and Poutanen, K. S. (2010). A psyllium fiber-enriched meal strongly attenuates postprandial gastrointestinal peptide release in healthy young adults. J. Nutr. 140:737–744.
- Kar, P., Laight, D., Rooprai, H. K., Shaw, K. M. and Cummings, M. (2009). Effects of grape seed extract in Type 2 diabetic subjects at high cardiovascular risk: A double blind randomized placebo controlled trial examining metabolic markers, vascular tone, inflammation, oxidative stress and insulin sensitivity. Diabetic. Med. 26(5):526–531.
- Kaufman, F. R. and Devgan, S. (1996). Use of uncooked cornstarch to avert nocturnal hypoglycemia in children and adolescents with type I diabetes. J. Diabetes Complicat. 10(2):84–87.
- Kelley, D. S., Adkins, Y., Woodhouse, L. R., Swislocki, A., Mackey, B. E. and Siegel, D. (2012). Docosahexaenoic acid supplementation improved lipocentric but not glucocentric markers of insulin sensitivity in hypertriglyceridemic men. Metab. Syndr. Relat. Disord. 10(1):32–38.
- Kendall, C. W., Esfahani, A., Josse, A. R., Augustin, L. S., Vidgen, E. and Jenkins, D. J. (2011a). The glycemic effect of nut-enriched meals in healthy and diabetic subjects. Nutr. Metab. Cardiovasc. Dis. 21(1):S34–S39.
- Kendall, C. W., Josse, A. R., Esfahani, A. and Jenkins, D. J. (2010). Nuts, metabolic syndrome and diabetes. Br. J. Nutr. 104:465–473.
- Kendall, C. W., Josse, A. R., Esfahani, A. and Jenkins, D. J. (2011b). The impact of pistachio intake alone or in combination with high-carbohydrate foods on post-prandial glycemia. Eur. J. Clin. Nutr. 65:696–702.
- Khan, L. A., Alam, A. M., Ali, L., Goswami, A., Hassan, Z., Sattar, S., Banik, N. G. and Khan, A. K. (1999). Serum and urinary magnesium in young diabetic subjects in Bangladesh. Am. J. Clin. Nutr. 69(1):70–73.
- Kiechl, S., Willeit, J., Poewe, W., Egger, G., Oberhollenzer, F., Muggeo, M. and Bonora, E. (1996). Insulin sensitivity and regular alcohol consumption: Large, prospective, cross-sectional population study (Bruneck study). Br. Med. J. 313:1040–1044.
- Kim, H., Stote, K. S., Behall, K. M., Spears, K., Vinyard, B. and Conway, J. M. (2009). Glucose and insulin responses to whole grain breakfasts varying in soluble Fibre, beta-glucan: A dose response study in obese women with increased risk for insulin resistance. Eur. J. Nutr. 48(3):170–175.
- Kinlaw, W. B., Levine, A. S., Morley, J. E. et al. (1983). Abnormal zinc metabolism in type II diabetes mellitus. Am. J. Med. 75:273–277.
- Kofod, H., Lernmark, A. and Hedeskov, C. J. (1986). Potentiation of insulin release in response to amino acid methyl esters correlates to activation of islet glutamate dehydrogenase activity. Acta. Physiol. Scand. 128:335–340.
- Krebs, M., Krssak, M., Bernroider, E., Anderwald, C., Brehm, A., Meyerspeer, M., Nowotny, P., Roth, E., Waldhausl, W. and Roden, M. (2002). Mechanism of amino acid-induced skeletal muscle insulin resistance in humans. Diabetes. 51:599–605.
- Kroenke, C. H., Chu, N. F., Rifai, N., Spiegelman, D., Hankinson, S. E., Manson, J. E. and Rimm, E. B. (2003). A cross-sectional study of alcohol consumption patterns and biologic markers of glycemic control among 459 women. Diabetes Care. 26:1971–1978.
- Lagiou, P., Sandin, S., Lof, M., Trichopoulos, D., Adami, H. O. and Weiderpass, E. (2012). Low carbohydrate-high protein diet and incidence of cardiovascular diseases in Swedish women: Prospective cohort study. Br. Med. J. 344:e4026.
- Laitinen, K., Poussa, T. and Isolauri, E. (2009). Probiotics and dietary counselling contribute to glucose regulation during and after pregnancy: A randomised controlled trial. Br. J. Nutr. 101:1679–1687.
- Lajoix, A. D., Reggio, H., Chardes, T., Peraldi-Roux, S., Tribillac, F., Roye, M., Dietz, S., Broca, C., Manteghetti, M., Ribes, G., Wollheim, C. B. and Gross, R. (2001). A neuronal isoform of nitric oxide synthase expressed in pancreatic beta-cells controls insulin secretion. Diabetes. 50:1311–1323.
- Lappi, J., Kolehmainen, M., Mykkänen, H. and Poutanen, K. (2012). Do large intestinal events explain the protective effects of whole grain foods against type 2 diabetes? Crit. Rev. Food Sci. Nutr. In press.
- Larsen, T. M., Dalskov, S. M., van Baak, M., Jebb, S. A., Papadaki, A., Pfeiffer, A. F., Martinez, J. A., Handjieva-Darlenska, T., Kunesova, M., Pihlsgard, M., Stender, S., Holst, C., Saris, W. H. and Astrup, A. (2010). Diets with high or low protein content and glycemic index for weight-loss maintenance. N. Engl. J. Med. 363:2102–2113.
- Larsen, H. N., Rasmussen, O. W., Rasmussen, P. H., Alstrup, K. K., Biswas, S. K., Tetens, I., Thilsted, S. H. and Hermansen, K. (2000). Glycaemic index of parboiled rice depends on the severity of processing: Study in type 2 diabetic subjects. Eur. J. Clin. Nutr. 5:380–385.
- Leclere, C., Champ, M. et al. (1994). Role of viscous guar gums in lowering the glycemic response after a solid meal. Amer. J. Clin. Nutr. 59(4):914–921.
- Lefevre, M., Lovejoy, J. C., Smith, S. R., DeLany, J. P., Champagne, C., Most, M. M., Denkins, Y., de Jonge, L., Rood, J. and Bray, G. A. (2005). Comparison of the acute response to meals enriched with cis- or trans-fatty acids on glucose and lipids in overweight individuals with differing FABP2 genotypes. Metab. Clin. Exper. 54:1652–1658.
- Lehtonen, H. M., Jarvinen, R., Linderborg, K., Viitanen, M., Venojarvi, M., Alanko, H. and Kallio, H. (2010). Postprandial hyperglycemia and insulin response are affected by sea buckthorn (Hippophae rhamnoides ssp turkestanica) berry and its ethanol-soluble metabolites. Eur. J. Clin. Nutr. 64(12):1465–1471.
- Lerman-Garber, I., Ichazo-Cerro, S., Zamora-González, J., Cardoso-Saldaña, G. and Posadas-Romero, C. (1994). Effect of a high-monounsaturated fat diet enriched with avocado in NIDDM patients. Diabetes Care. 4:311–315.
- Li, S. C., Liu, Y. H., Liu, J. F., Chang, W. H., Chen, C. M. and Chen, C. Y. (2011). Almond consumption improved glycemic control and lipid profiles in patients with type 2 diabetes mellitus. Metabolism. 60(4):474–479.
- Liatis, S., Grammatikou, S., Poulia, K. A., Perrea, D., Makrilakis, K., Diakoumopoulou, E. and Katsilambros, N. (2010). Vinegar reduces postprandial hyperglycaemia in patients with type II diabetes when added to a high, but not to a low, glycaemic index meal. Eur. J. Clin. Nutr. 64(7):727–732.
- Librenti, M. C., Cocchi, M., Orsi, E., Pozza, G., Micossi, P. (1992). Effect of soya and cellulose fibers on postprandial glycemic response in type II diabetic patients. Diabetes Care. 15(1):111–113.
- Liljeberg, H. G. M., Åkerberg, A. K. E. et al. (1996). Resistant starch formation in bread as influenced by choice of ingredients or baking conditions. Food Chem. 56(4):389–394.
- Liljeberg, H. G. M. and Bjorck, I. M. E. (1998). Delayed gastric emptying rate may explain improved glycaemia in healthy subjects to a starchy meal with added vinegar. Eur. J. Clin. Nutr. 52:368–371.
- Liljeberg, H. G. M., Granfeldt, Y. E. et al. (1992). Metabolic response to starch in bread containing intact kernels versus milled flour. Eur. J. Clin. Nutr. 46:561–575.
- Linn, T., Santosa, B., Gronemeyer, D., Aygen, S., Scholz, N., Busch, M. and Bretzel, R. G. (2000). Effect of long-term dietary protein intake on glucose metabolism in humans. Diabetologia. 43:1257–1265.
- Livesey, G., Taylor, R., Hulshof, T. and Howlett, J. (2008). Glycemic response and health—A systematic review and meta-analysis: Relations between dietary glycemic properties and health outcomes. Am. J. Clin. Nutr. 87(suppl):258S–268S.
- Li, D., Xu, T., Takase, H., Tokimitsu, I., Zhang, P., Wang, Q., Yu, X. and Zhang, A. (2008). Diacylglycerol-induced improvement of whole-body insulin sensitivity in type 2 diabetes mellitus: A long-term randomized, double-blind controlled study. Clin. Nutr. (Edinburgh, Scotland). 27:203–211.
- Lopez, S., Bermudez, B., Ortega, A., Varela, L. M., Pacheco, Y. M., Villar, J., Abia, R. and Muriana, F. J. (2011). Effects of meals rich in either monounsaturated or saturated fat on lipid concentrations and on insulin secretion and action in subjects with high fasting triglyceride concentrations. Amer. J. Clin. Nutrn. 93:494–499.
- Louie, J. C., Atkinson, F., Petocz, P. and Brand-Miller, J. C. (2008). Delayed effects of coffee, tea and sucrose on postprandial glycemia in lean, young, healthy adults. Asia Pac. Clin. Nutr. 17(4):657–662.
- Lovegrove, J. A., Lovegrove, S. S., Lesauvage, S. V., Brady, L. M., Saini, N., Minihane, A. M. and Williams, C. M. (2004). Moderate fish-oil supplementation reverses low-platelet, long-chain n–3 polyunsaturated fatty acid status and reduces plasma triacylglycerol concentrations in British Indo-Asians. Amer. J. Clin. Nutr. 79:974–982.
- Lu, Z. X., Walker, K. Z., Muir, J. G., and O'Dea, K. (2004). Arabinoxylan fibre improves metabolic control in people with Type II diabetes. Eur. J. Clin. Nutr. 58, 621–628.
- Luo, J. et al. (2000). Chronic consumption of short-chain fructooligosaccharides does not affect basal hepatic glucose production or insulin resistance in type 2 diabetics. J. Nutr. 130, 1572–1577.
- Luo, J., Rizkalla, S. W., Alamowitch, C., Boussairi, A., Blayo, A., Barry, J. L., Laffitte, A., Guyon, F., Bornet, F. R. and Slama, G. (1996). Chronic consumption of short-chain fructooligosaccharides by healthy subjects decreased basal hepatic glucose production but had no effect on insulin-stimulated glucose metabolism. Am. J. Clin. Nutr. 63(6):939–945.
- Madigan, C., Ryan, M., Owens, D., Collins, P. and Tomkin, G. H. (2000). Dietary unsaturated fatty acids in type 2 diabetes: Higher levels of postprandial lipoprotein on a linoleic acid-rich sunflower oil diet compared with an oleic acid-rich olive oil diet. Diabetes Care. 23:1472–1477.
- Maki, K. C., Curry, L. L., Reeves, M. S., Toth, P. D., McKenney, J. M., Farmer, M. V., Schwartz, S. L., Lubin, B. C., Boileau, A. C., Dicklin, M. R., Carakostas, M. C. and Tarka, S. M. (2008). Chronic consumption of rebaudioside A, a steviol glycoside, in men and women with type 2 diabetes mellitus. Food Chem. Toxicol. 46(7): S47–S53.
- Maki, T., Pham, N. M., Yoshida, D., Yin, G., Ohnaka, K., Takayanagi, R. and Kono, S. (2010). The relationship of coffee and green tea consumption with high-sensitivity C-reactive protein in Japanese men and women. Clin. Chem. Lab. Med. 48(6):849–854.
- Maki, K .C., Reeves, M. S., Carson, M. L., Miller, M. P., Turowski, M., Rains, T. M., Anderson, K., Papanikolaou, Y., Wilder, D. M. (2009). Dose-response characteristics of high-viscosity hydroxypropylmethylcellulose in subjects at risk for the development of type 2 diabetes mellitus. Diabetes Technol Ther. 11(2):119–225.
- Malaisse, W. J., Plasman, P. O., Blachier, F., Herchuelz, A. and Sener, A. (1991). Stimulus-secretion coupling of arginine-induced insulin release: Significance of changes in extracellular and intracellular pH. Cell. Biochem. Funct. 9:1–7.
- Manders, R. J., Koopman, R., Beelen, M., Gijsen, A. P., Wodzig, W. K., Saris, W. H. and van Loon, L. J. (2008). The muscle protein synthetic response to carbohydrate and protein ingestion is not impaired in men with longstanding type 2 diabetes. J. Nutr. 138:1079–1085.
- Manders, R. J., Koopman, R., Sluijsmans, W. E., van den Berg, R., Verbeek, K., Saris, W. H., Wagenmakers, A. J. and van Loon, L. J. (2006a). Co-ingestion of a protein hydrolysate with or without additional leucine effectively reduces postprandial blood glucose excursions in Type 2 diabetic men. J. Nutr. 136:1294–1299.
- Manders, R. J., Little, J. P., Forbes, S. C. and Candow, D. G. (2012). Insulinotropic and muscle protein synthetic effects of branched-chain amino acids: Potential therapy for type 2 diabetes and sarcopenia. Nutrients. 4/11:1664–1678
- Manders, R. J., Praet, S. F., Meex, R. C., Koopman, R., de Roos, A. L., Wagenmakers, A. J., Saris, W. H. and van Loon, L. J. (2006b). Protein hydrolysate/leucine co-ingestion reduces the prevalence of hyperglycemia in type 2 diabetic patients. Diabetes Care. 29:2721–2722.
- Manders, R. J., Wagenmakers, A. J., Koopman, R., Zorenc, A. H., Menheere, P. P., Schaper, N. C., Saris, W. H. and van Loon, L. J. (2005). Co-ingestion of a protein hydrolysate and amino acid mixture with carbohydrate improves plasma glucose disposal in patients with type 2 diabetes. Am. J. Clin. Nutr. 82:76–83.
- Manning, P. J., Sutherland, W. H., Hendry, G., de Jong, S. A., McGrath, M. and Williams, S. M. (2004). Changes in circulating postprandial proinflammatory cytokine concentrations in diet-controlled type 2 diabetes and the effect of ingested fat. Diabetes Care. 27:2509–2511.
- Marciani, L., Gowland, P. A., Spiller, R. C., Manoj, P., Moore, R. J., Young, P. and Fillery-Travis, A. J. (2001). Effect of meal viscosity and nutrients on satiety, intragastric dilution, and emptying assessed by MRI. Am. J. Physiol. Gastrointest Liver Physiol. 280(6):G1227–G1233.
- Markey, O., McClean, C. M., Medlow, P., Davison, G. W., Trinick, T. R., Duly, E. and Shafat, A. (2011). Effect of cinnamon on gastric emptying, arterial stiffness, postprandial lipemia, glycemia, and appetite responses to high-fat breakfast. Cardiovascular Dis. 10:78.
- Maruyama, K., Iso, H., Sasaki, S. and Fukino, Y. (2009). The association between concentrations of green tea and blood glucose levels. J. Clin. Biochem. Nutr. 44(1):41–45.
- Masterjohn, C., Mah, E., Guo, Y., Koo, S. I. and Bruno, R. S. (2012). Gamma-Tocopherol abolishes postprandial increases in plasma methylglyoxal following an oral dose of glucose in healthy, college-aged men. J. Nutr. Bioch. 23(3):292–298.
- Matsuda, H., Yoshikawa, M., Morikawa, T., Tanabe, G. and Muraoka, O. (2005). Antidiabetogenic constituents from Salacia species. J. Trad. Med. 22(1):145–153.
- McCargar, L. J., Innis, S. M., Bowron, E., Leichter, J., Dawson, K., Toth, E. and Wall, K. M. (1998). Effect of enteral nutritional products differing in carbohydrate and fat on indices of carbohydrate and lipid metabolism in patients with NIDDM. Mol. Cell Biochem. 188:81–89.
- McIvor, M. E., Cummings, C. C., Leo, T. A., Mendeloff, A. I. (1985). Flattening postprandial blood glucose responses with guar gum: Acute effects. Diabetes Care. 8(3):274–278.
- Mehrabani, H. H., Salehpour, S., Amiri, Z., Farahani, S. J., Meyer, B. J. and Tahbaz, F. (2012). Beneficial effects of a high-protein, low-glycemic-load hypocaloric diet in overweight and obese women with polycystic ovary syndrome: A randomized controlled intervention study. J. Am. Coll. Nutr. 31(2):117–125.
- Mettler, S., Schwarz, I. and Colombani, P. C. (2009). Additive postprandial blood glucose-attenuating and satiety-enhancing effect of cinnamon and acetic acid. Nutr. Res. 29(10):723–727.
- Mezitis, N. H., Maggio, C. A., Koch, P., Quddoos, A., Allison, D. B. and Pi-Sunyer, F. X. (1996). Glycemic effect of a single high oral dose of the novel sweetener sucralose in patients with diabetes. Diabetes Care. 19:1004–1005.
- Michaliszyn, S. F., Sjaarda, L. A., Mihalik, S. J., Lee, S., Bacha, F., Chace, D. H., De Jesus, V. R., Vockley, J. and Arslanian, S. A. (2012a). Metabolomic profiling of amino acids and β-cell function relative to insulin sensitivity in youth. J. Clin. Endocrinol. Metab. 97(11): E2119–E2124
- Michaliszyn, S. F., Sjaarda, L. A., Mihalik, S. J., Lee, S., Bacha, F., Chace, D. H., De Jesus, V. R., Vockley, J., and Arslanian, S. A. (2012b). Metabolomic profiling of amino acids and β-cell function relative to insulin sensitivity in youth. J. Clin. Endocrinol. Metab. 11:E2119–E2124.
- Mitri, J., Muraru, M. D. and Pittas, A. G. (2011). Vitamin D and type 2 diabetes: A systematic review. Eur. J. Clin. Nutr. 65:1005–1015.
- Moisey, L. L., Kacker, S., Bickerton, A. C., Robinson, L. E. and Graham, T. E. (2008). Caffeinated coffee consumption impairs blood glucose homeostasisin response to high and low glycemic index meals in healthy men. Am. J. Clin. Nutr. 87:1254–1261.
- Moisey, L. L., Robinson, L. E. and Graham, T. E. (2010). Consumption of caffeinated coffee and a high carbohydrate meal affects postprandial metabolism of a subsequent oral glucose tolerance test in young, healthy males. Br. J. Nutr. 103(6):833–841.
- Mori, A. M., Considine, R. V. and Mattes, R. D. (2011). Acute and second-meal effects of almond form in impaired glucose tolerant adults: A randomized crossover trial. Nutr. Metab. 8/1:6.
- Moroti, C., Souza Magri, L. F., de Rezende Costa, M., Cavallini, D. C. and Sivieri, K. (2012). Effect of the consumption of a new symbiotic shake on glycemia and cholesterol levels in elderly people with type 2 diabetes mellitus. Lipids. Health Dis. 11:29.
- Muniyappa, R., Hall, G., Kolodziej, T. L., Karne, R. J., Crandon, S. K. and Quon, M. J. (2008). Cocoa consumption for 2 wk enhances insulin-mediated vasodilatation without improving blood pressure or insulin resistance in essential hypertension. Amer. J. Clin. Nutr. 88(6):1685–1696.
- Muscogiuri, G., Sorice, G. P., Prioletta, A., Policola, C., Casa, S. D., Pontecorvi, A. and Giaccari, A. (2011). Will vitamin D reduce insulin resistance? Still a long way to go. Amer. J. Clin. Nutr. 93:672–673.
- Möhlig, M., Freudenberg, M., Bobbert, T., Ristow, M., Rochlitz, H., Weickert, M. O., Pfeiffer, A. F. and Spranger, J. (2006). Acetylsalicylic acid improves lipid-induced insulin resistance in healthy men. J. Clin. Endocrinol. Metab. 91:964–967.
- Nagao, T., Meguro, S., Hase, T., Otsuka, K., Komikado, M., Tokimitsu, I., Yamamoto, T. and Yamamoto, K. A. (2009). Catechin-rich beverage improves obesity and blood glucose control in patients with type 2. Diabetes Obes. 17(2):310–317.
- Nappo, F., Esposito, K., Cioffi, M., Giugliano, G., Molinari, A. M., Paolisso, G., Marfella, R. and Giugliano, D. (2002). Postprandial endothelial activation in healthy subjects and in type 2 diabetic patients: Role of fat and carbohydrate meals. J. Amer. Coll Cardiol. 39:1145–1150.
- Nazare, J. A., Normand, S., Oste Triantafyllou, A., Brac de la Perrière, A., Desage, M., Laville, M. (2009). Modulation of the postprandial phase by beta-glucan in overweight subjects: effects on glucose and insulin kinetics. Mol. Nutr. Food Res. 53(3):361–369.
- Neri, S., Signorelli, S. S., Torrisi, B., Pulvirenti, D., Mauceri, B., Abate, G., Ignaccolo, L., Bordonaro, F., Cilio, D., Calvagno, S. and Leotta, C. (2005). Effects of antioxidant supplementation on postprandial oxidative stress and endothelial dysfunction: A single-blind, 15-day clinical trial in patients with untreated type 2 diabetes, subjects with impaired glucose tolerance, and healthy controls. Clin. Therap. 27:1764–1773.
- Newgard, B. C. (2012). Interplay between lipuds and branched chain amino acids in development of insulin resistance. Cell Metab. 15:606–614.
- Newgard, C. B., An, J., Bain, J. R., Muehlbauer, M. J., Stevens, R. D., Lien, L. F., Haqq, A. M., Shah, S. H., Arlotto, M., Slentz, C. A., Rochon, J., Gallup, D., Ilkayeva, O., Wenner, B. R., Yancy, W. S., Jr, Eisenson, H., Musante, G., Surwit, R. S., Millington, D. S., Butler, M. D., and Svetkey, L. P. (2009a). A branched-chain amino acid-related metabolic signature that differentiates obese and lean humans and contributes to insulin resistance. Cell Metab. 9/4:311–326.
- Newgard, C. B., An, J., Bain, J. R., Muehlbauer, M. J., Stevens, R. D., Lien, L. F., Haqq, A. M., Shah, S. H., Arlotto, M., Slentz, C. A., Rochon, J., Gallup, D., Ilkayeva, O., Wenner, B. R., Yancy, W. S., Jr., Eisenson, H., Musante, G., Surwit, R. S., Millington, D. S., Butler, M. D., and Svetkey, L. P. (2009b). Cell Metab. 9:311–326.
- Newsholme, P., Brennan, L., Rubi, B. and Maechler, P. (2005). New insights into amino acid metabolism, beta-cell function and diabetes. Clin. Sci. (Lond). 108:185–194.
- Nikooyeh, B., Neyestani, T. R., Farvid, M., Alavi-Majd, H., Houshiarrad, A., Kalayi, A., Shariatzadeh, N., Gharavi, A. a., Heravifard, S., Tayebinejad, N., Salekzamani, S. and Zahedirad, M. (2011). Daily consumption of vitamin D or vitamin D calcium “fortified yogurt drink improved glycemic control in patients with type 2 diabetes: A randomized clinical trial. Amer. J. Clin. Nutr. 93:764–771.
- Nilsson, M., Holst, J. J. et al. (2007). Metabolic effects of amino acid mixtures and whey protein in healthy subjects: Studies using glucose-equivalent drinks. Amer. J. Clin. Nutr. 85(4):996–1004.
- Nilsson, A. C., Östman, E. M. et al. (2008a). Effect of cereal test breakfasts differing in glycemic index and content of indigestible carbohydrates on daylong glucose tolerance in healthy subjects. Amer. J. Clin. Nutr. 87(3):645–654.
- Nilsson, A. C., Östman, E. M. et al. (2008b). Including indigestible carbohydrates in the evening meal of healthy subjects improves glucose tolerance, lowers inflammatory markers, and increases satiety after a subsequent standardized breakfast. J. Nutr. 138(4):732–739.
- Nilsson, A. C., Östman, E. M., Granfeldt, Y. and Bjorck, I. M. (2008c). Effect of cereal test breakfasts differing in glycemic index and content of indigestible carbohydrates on daylong glucose tolerance in healthy subjects. Am. J. Clin. Nutr. 87:645–654.
- Nilsson, A. C., Östman, E. M., Granfeldt, Y. and Björck, I. M. (2008d). Effect of cereal test breakfasts differing in glycemic index and content of indigestible carbohydrates on daylong glucose tolerance in healthy subjects. Am. J. Clin. Nutr. 87(3):645–654.
- Nilsson, A. C., Östman, E. M., Holst, J. J. and Björck, I. M. (2008e). Including indigestible carbohydrates in the evening meal of healthy subjects improves glucose tolerance, lowers inflammatory markers, and increases satiety after a subsequent standardized breakfast. J. Nutr. 138(4):732–739.
- Nilsson, A., Östman, E., Preston, T. and Björck, I. (2008f). Effects of GI vs content of cereal fibre of the evening meal on glucose tolerance at a subsequent standardized breakfast. Eur. J. Clin. Nutr. 62(6):712–720.
- Niskanen, L., Turpeinen, A., Penttila, I. and Uusitupa, M. I. (1998) Hyperglycemia and compositional lipoprotein abnormalities as predictors of cardiovascular mortality in type 2 diabetes: A 15-year follow-up from the time of diagnosis. Diabetes Care 21(11):1861–1869.
- Nuttall, F. Q., Gannon, M. C., Wald, J. L. and Ahmed, M. (1985). Plasma glucose and insulin profiles in normal subjects ingesting diets of varying carbohydrate, fat, and protein content. J. Am. Coll. Nutr. 4:437–450.
- Nuttall, F. Q., Mooradian, A. D., Gannon, M. C., Billington, C. and Krezowski, P. (1984). Effect of protein ingestion on the glucose and insulin response to a standardized oral glucose load. Diabetes Care. 7:465–470.
- Ohkubo, Y., Kishikawa, H., Araki, E., Miyata, T., Isami, S., Motoyoshi, S. et al. (1995) Intensive insulin therapy prevents the progression of diabetic microvascular complications in Japanese patients with noninsulin-dependent diabetes mellitus: A randomized prospective 6-year study. Diabetes Res. Clin. Pract. 28(2):103–117.
- Östman, E., Granfeldt, Y., Persson, L., and Björck, I. (2005). Vinegar supplementation lowers glucose and insulin responses and increases satiety after a bread meal in healthy subjects. Eur. J. Clin. Nutr. 59(9):983–988.
- O’Sullivan, A., Balducci, D., Paradisi, F., Cashman, K. D., Gibney, M. J. and Brennan, L. (2011). Effect of supplementation with vitamin D3 on glucose production pathways in human subjects. Mol. Nutr. Food Res. 55:1018–1025.
- Page, G. L. J., Laight, D. and Cummings, M. H. (2011). Thiamine deficiency in diabetes mellitus and the impact of thiamine replacement on glucose metabolism and vascular disease. Int. J. Clin. Practice. 65:684–690.
- Pallotta, J. A. and Kennedy, P. J. (1968). Response of plasma insulin and growth hormone to carbohydrate and protein feeding. Metabolism. 17:901–908.
- Paniagua, J. A., de la Sacristana, A. G., Sánchez, E., Romero, I., Vidal-Puig, A., Berral, F. J., Escribano, A., Moyano, M. J., Peréz-Martinez, P., López-Miranda, J. and Pérez-Jiménez, F. (2007). A MUFA-rich diet improves posprandial glucose, lipid and GLP-1 responses in insulin-resistant subjects. J. Amer. Coll. Nutr. 26:434–444.
- Panlasigui, L. N. and Thompson, L. U. (2006). Blood glucose lowering effects of brown rice in normal and diabetic subjects. Int. J. Food Sci. Nutr. 57/3–4:151–158.
- Papathanasopoulos, A. and Camilleri, M. (2010). Dietary fibre supplements: Effects in obesity and metabolic syndrome and relationship to gastrointestinal functions. Gastroenterology. 138(1):65–72.
- Park, J. H., Grandjean, C. J., Hart, M. H. et al. (1986). Effect of pure zinc deficiency on glucose tolerance and insulin and glucagon levels. Am. J. Physiol. 251:E273±E278.
- Parnell, J. A. and Reimer, R. A. (2009). Weight loss during oligofructose supplementation is associated with decreased ghrelin and increased peptide YY in overweight and obese adults. Am. J. Clin. Nutr. 89(6):1751–1759.
- Pastors, J. G., Blaisdell, P. W. et al. (1991). Psyllium Fibre reduces rise in postprandial glucose and insulin concentrations in patients with non-insulin-dependent diabetes. Am. J. Clin. Nutr. 53(6):1431–1435.
- Pena, A. S., Couper, J. J. et al. (2012). Hypoglycemia, but not glucose variability, relates to vascular function in children with type 1 diabetes. Diabetes Technol. Ther. 14(6):457–462.
- Pereira, M. A., Jacobs, D. R., Jr., Pins, J. J., Raatz, S. K., Gross, M. D., Slavin, J. L. and Seaquist, E. R. (2002). Effect of whole grains on insulin sensitivity in overweight hyperinsulinemic adults. Am. J. Clin. Nutr. 75:848–855.
- Piers, L. S., Walker, K. Z., Stoney, R. M., Soares, M. J. and O’Dea, K. (2003). Substitution of saturated with monounsaturated fat in a 4-week diet affects body weight and composition of overweight and obese men. Br. J. Nutr. 90:717–727.
- Pipeleers, D. G., Schuit, F. C., in't Veld, P. A., Maes, E., Hooghe-Peters, E. L., Van de Winkel, M., and Gepts, W. (1985). Interplay of nutrients and hormones in the regulation of insulin release. Endocrinology. 117:824–833.
- Pi-Sunyer, F. X. (2005). Weight loss in type 2 diabetic patients. Diabetes Care. 28:1526–1527.
- Plaisance, E. P., Mestek, M. L., Mahurin, A. J., Taylor, J. K., Moncada-Jimenez, J. and Grandjean, P. W. (2008). Postprandial triglyceride responses to aerobic exercise and extended-release niacin. Amer. J. Clin. Nutr. 88:30–37.
- Polonsky, K. S., Sturis, J. and Bell, G. I. (1996). Seminars in medicine of the Beth Israel Hospital, Boston. Non-insulin-dependent diabetes mellitus—A genetically programmed failure of the beta cell to compensate for insulin resistance. N. Engl. J. Med. 334:777–783.
- Pravdova, E., Macho, L. and Fickova, M. (2009). Alcohol intake modifies leptin, adiponectin and resistin serum levels and their mRNA expressions in adipose tissue of rats. Endocrine. Reg. 43(3):117–125.
- Punthakee, Z., Bosch, J., Dagenais, G., Diaz, R., Holman, R., Probstfield, J. L., Ramachandran, A., Riddle, M. C., Rydén, L. E., Zinman, B., Afzal, R., Yusuf, S., Gerstein, H. C.; TIDE Trial Investigators. (2012). Design, history and results of the thiazolidinedione intervention with vitamin D evaluation (TIDE) randomised controlled trial. Diabetologia. 55:36–45.
- Qin, J., Li, R., Raes, J., Arumugam, M., Burgdorf, K. S., Manichanh, C., Nielsen, T., Pons, N., Levenez, F., Yamada, T., Mende, D. R., Li, J., Xu, J., Li, S., Li, D., Cao, J., Wang, B., Liang, H., Zheng, H., Xie, Y., Tap, J., Lepage, P., Bertalan, M., Batto, J. M., Hansen, T., Le, P. D., Linneberg, A., Nielsen, H. B., Pelletier, E., Renault, P., Sicheritz-Ponten, T., Turner, K., Zhu, H., Yu, C., Li, S., Jian, M., Zhou, Y., Li, Y., Zhang, X., Li, S., Qin, N., Yang, H., Wang, J., Brunak, S., Dore, J., Guarner, F., Kristiansen, K., Pedersen, O., Parkhill, J., Weissenbach, J., Bork, P., Ehrlich, S. D. and Wang J (2010). A human gut microbial gene catalogue established by metagenomic sequencing. Nature. 464:59–65.
- Raben, A., Møller, B. K., Flint, A., Vasilaris, T. H., Christina Møller, A., Juul Holst, J. and Astrup, A. (2011). Increased postprandial glycaemia, insulinemia, and lipidemia after 10 weeks’ sucrose-rich diet compared to an artificially sweetened diet: A randomised controlled trial. Food Nutr. Res. 55: doi:10.3402/fnr.v55i0.5961.
- Rabinowitz, D., Merimee, T. J., Maffezzoli, R. and Burgess, J. A. (1966). Patterns of hormonal release after glucose, protein, and glucose plus protein. Lancet. 2:454–456.
- Rajpathak, S. N., Freiberg, M. S., Wang, C., Wylie-Rosett, J., Wildman, R. P., Rohan, T. E., Robinson, J. G., Liu, S. and Wassertheil-Smoller, S. (2010). Alcohol consumption and the risk of coronary heart disease in postmenopausal women with diabetes: Women's Health Initiative Observational Study. Eur. J. Nutr. 4:211–218.
- Raninen, K., Lappi, J., Mykkanen, H. and Poutanen, K. (2011). Type of dietary Fibre reflects the physiological functionality – comparison of grain fibre, inulin and polydextrose. Nutr. Abstr. Rev. 1:9–21.
- Reay, J. L., Kennedy, D. O. and Scholey, A. B. (2006). The glycaemic effects of single doses of Panax ginseng in young healthy volunteers. Br. J. Nutr. 96:639–642.
- Reay, J. L., Scholey, A. B., Milne, A., Fenwick, J. and Kennedy, D. O. (2009). Panax ginseng has no effect on indices of glucose regulation following acute or chronic ingestion in healthy volunteers. Br. J. Nutr. 101:1673–1678.
- Rebello, S. A., Chen, C. H., Naidoo, N., Xu, W., Lee, J., Chia, K. S., Tai, E. S. and van Dam, R. M. (2011). Coffee and tea consumption in relation to inflammation and basal glucose metabolism in a multi-ethnic Asian population: A cross-sectional study. Nutr. J. 2:10:61.
- Rendell, M., Vanderhoof, J., Venn, M., Shehan, M. A., Arndt, E., Rao, C. S., Gill, G., Newman, R. K., Newman, C. W. (2005). Effect of a barley breakfast cereal on blood glucose and insulin response in normal and diabetic patients. Plant Foods Hum Nutr. 60(2):63–67.
- Rivellese, A. A., Maffettone, A., Iovine, C., Di Marino, L., Annuzzi, G., Mancini, M. and Riccardi, G. (1996). Long-term effects of fish oil on insulin resistance and plasma lipoproteins in NIDDM patients with hypertriglyceridemia. Diabetes Care. 11:1207–1213.
- Rizkalla, S. W., Taghrid, L. et al. (2004). Improved plasma glucose control, whole-body glucose utilization, and lipid profile on a low-glycemic index diet in type 2 diabetic men: A randomized controlled trial. Diabetes Care. 27(8):1866–1872.
- Roberfroid, M., Gibson, G. R., Hoyles, L., McCartney, A. L., Rastall, R., Rowland, I., Wolvers, D., Watzl, B., Szajewska, H., Stahl, B., Guarner, F., Respondek, F., Whelan, K., Coxam, V., Davicco, M. J., Leotoing, L., Wittrant, Y., Delzenne, N. M., Cani, P. D., Neyrinck, A. M. and Meheust, A. (2010). Prebiotic effects: Metabolic and health benefits. Br. J. Nutr. 104(2):S1–S63.
- Robert, S. D. and Ismail, A. A. (2012). Glycemic responses of patients with type 2 diabetes to individual carbohydrate-rich foods and mixed meals. Ann. Nutr. Metab. 60(1):27–32.
- Roberts, A. (1999). Sucralose and diabetes. Food Food Ingred. Jpn. 182:49–55.
- Robertson, M. D., Bickerton, A. S., Dennis, A. L., Vidal, H. and Frayn, K. N. (2005). Insulin-sensitizing effects of dietary resistant starch and effects on skeletal muscle and adipose tissue metabolism. Am. J. Clin. Nutr. 82:559–567.
- Robertson, M. D., Currie, J. M., Morgan, L. M., Jewell, D. P. and Frayn, K. N. (2003). Prior short-term consumption of resistant starch enhances postprandial insulin sensitivity in healthy subjects. Diabetologia. 46:659–665.
- Rosén, L. A., Östman, E. M., and Björck, I. M. (2011). Effects of cereal breakfasts on postprandial glucose, appetite regulation and voluntary energy intake at a subsequent standardized lunch; focusing on rye products. Nutr. J. 10:7.
- Rosen, L. A. H., Silva, L. O. B. et al. (2009). Endosperm and whole grain rye breads are characterized by low post-prandial insulin response and a beneficial blood glucose profile. Nutr. J. 25(8):42.
- Rosen, L. A., Östman, E. M. et al. (2011). Effects of cereal breakfasts on postprandial glucose, appetite regulation and voluntary energy intake at a subsequent standardized lunch; focusing on rye products. Nutr. J. 19(10):7.
- Roth, H. P. and Kirchgessner, M. (1981). Zinc and insulin metabolism. Biol. Trace Elem. Res. 3:13–32.
- Rustan, A. C., Nenseter, M. S. and Drevon, C. A. (1997). Omega-3 and Omega-6 fatty acids in the insulin resistance syndrome. Ann. NY Acad. Sci. 827:310–326.
- Sae-tan S, Grove, K. A., and Lambert, J. D. (2011). Weight control and prevention of metabolic syndrome by green tea. Pharmacol. Res. 64:146–154.
- Salehi, A., Gunnerud, U., Muhammed, S. J., Östman, E., Holst, J. J., Björck, I. and Rorsman, P. (2012). The insulinogenic effect of whey protein is partially mediated by a direct effect of amino acids and GIP on β-cells. Nutr. Metab. 9(1):48.
- Sales, C. H., Pedrosa, L. F., Lima, J. G., Lemos, T. M. and Colli, C. (2011). Influence of magnesium status and magnesium intake on the blood glucose control in patients with type 2 diabetes. Clin. Nutr. 30(3):359–364.
- Sanaka, M., Yamamoto, T., Anjiki, H., Nagasawa, K. and Kuyama, Y. (2007). Effects of agar and pectin on gastric emptying and post-prandial glycaemic profiles in healthy human volunteers. Clin. Exp. Pharmacol. Physiol. 34(11):1151–1155.
- Scalfi, L., Coltorti, A. and Contaldo, F. (1991). Postprandial thermogenesis in lean and obese subjects after meals supplemented with medium-chain and long-chain triglycerides. Ame. J. Clin. Nutr. 53:1130–1133.
- Schaafsma, G., Meuling, W. J., van Dokkum, W. and Bouley, C. (1998). Effects of a milk product, fermented by Lactobacillus acidophilus and with fructo-oligosaccharides added, on blood lipids in male volunteers. Eur. J. Clin. Nutr. 52:436–440.
- Schroder, H., Marrugat, J., Fito, M., Weinbrenner, T. and Covas, M. I. (2006). Alcohol consumption is directly associated with circulating oxidized low-density lipoprotein. Free Radic Biol. Med. 40(8):1474–1481.
- Schulze, M. B., Schulz, M., Heidemann, C., Schienkiewitz, A., Hoffmann, K. and Boeing, H. (2007). Fibre and magnesium intake and incidence of type 2 diabetes: A prospective study and meta-analysis. Arch. Intern. Med. 167:956–965.
- Schwab, U., Louheranta, A., Törrönen, A. and Uusitupa, M. (2006). Impact of sugar beet pectin and polydextrose on fasting and postprandial glycemia and fasting concentrations of serum total and lipoprotein lipids in middle-aged subjects with abnormal glucose metabolism. Eur. J. Clin. Nutr. 60:1073–1080.
- Schwanstecher, C., Meyer, M., Schwanstecher, M. and Panten, U. (1998). Interaction of N-benzoyl-D-phenylalanine and related compounds with the sulphonylurea receptor of beta-cells. Br. J. Pharmacol. 123:1023–1030.
- Sels, J. P., De Bruin, H., Camps, M. H., Postmes, T. J., Menheere, P., Wolfenbuttel, B. H. and Kruseman, A. C. (1992). Absence of guar efficacy in complex spaghetti meals on postprandial glucose and C-peptide levels in healthy control and non-insulin-dependent diabetes mellitus subjects. Horm. Metab. Res. Suppl. 26:52–58.
- Sener, A., Hutton, J. C. and Malaisse, W. J. (1981). The stimulus-secretion coupling of amino acid-induced insulin release. Synergistic effects of L-glutamine and 2-keto acids upon insulin secretion. Biochim. Biophys. Acta. 677:32–38.
- Sener, A. and Malaisse, W. J. (2002). The stimulus-secretion coupling of amino acid-induced insulin release. Insulinotropic action of L-alanine. Biochim. Biophys. Acta. 1573:100–104.
- Serraclara, A., Hawkins, F., Perez, C., Dominguez, E., Campillo, J. E. and Torres, M. D. (1998). Hypoglycemic action of an oral fig-leaf decoction in type-I diabetic patients. Diabetes Res. Clin. Pract. 39(1):19–22.
- Sievenpiper, J. L., Arnason, J. T., Leiter, L. A. and Vuksan, V. (2003a). Null and opposing effects of Asian ginseng (Panax ginseng C.A. Meyer) on acute glycemia: Results of two acute dose escalation studies. J. Am. Coll. Nutr. 22:524–532.
- Sievenpiper, J. L., Arnason, J. T., Leiter, L. A. and Vuksan, V. (2003b). Variable effects of American ginseng: A batch of American ginseng (Panax quinquefolius L.) with a depressed ginsenoside profile does not affect postprandial glycemia. Eur. J. Clin. Nutr. 57:243–248.
- Sievenpiper, J. L., Arnason, J. T., Leiter, L. A. and Vuksan, V. (2004). Decreasing, null and increasing effects of eight popular types of ginseng on acute postprandial glycemic indices in healthy humans: The role of ginsenosides. J. Am. Coll. Nutr. 23:248–258.
- Sievenpiper, J. L., Sung, M. K., Di Buono, M., Seung-Lee, K., Nam, K. Y., Arnason, J. T. et al. (2006). Korean red ginseng rootlets decrease acute postprandial glycemia: Results from sequential preparation- and dose-finding studies. J. Am. Coll. Nutr. 25:100–107.
- Slaughter, S. L., Ellis, P. R., et al. (2002). The effect of guar galactomannan and water availability during hydrothermal processing on the hydrolysis of starch catalysed by pancreatic [alpha]-amylase. Biochim. Biophys. Acta. General Subjects 1571/1:55–63.
- Slavin, J. L., Savarino, V., Paredes-Diaz, A. and Fotopoulos, G. (2009). A review of the role of soluble Fibre in health with specific reference to wheat dextrin. J. Int. Med. Res. 37(1):1–17.
- Sloth, B., Due, A., Larsen, T. M., Holst, J. J., Heding, A. and Astrup, A. (2009). The effect of a high-MUFA, low-glycaemic index diet and a low-fat diet on appetite and glucose metabolism during a 6-month weight maintenance period. Br. J. Nutr. 101:1846–1858.
- Sluijs, I., Beulens, J. W., van der, A.D., Spijkerman, A. M., Grobbee, D. E. and van der Schouw, Y. T. (2010). Dietary intake of total animal and vegetable protein and risk of type 2 diabetes in the European Prospective Investigation into Cancer and Nutrition (EPIC)-NL study. Diabetes Care. 33:43–48.
- Sorensen, M. and Johansen, O. E. (2010). Idiopathic reactive hypoglycaemia—Prevalence and effect of fibre on glucose excursions. Scan. J. Clin. Lab. Invest. 70:385–391.
- Sotaniemi, E. A., Haapakoski, E. and Rautio, A. (1995). Ginseng therapy in non-insulin-dependent diabetic patients. Diabetes Care. 18:1373–1375.
- Stanhope, K. L., Schwarz, J. M., Keim, N. L., Griffen, S. C., Bremer, A. A., Graham, J. L., Hatcher, B., Cox, C. L., Dyachenko, A., Zhang, W., McGahan, J. P., Seibert, A., Krauss, R. M., Chiu, S., Schaefer, E. J., Ai, M., Otokozawa, S., Nakajima, K., Nakano, T., Beysen, C., Hellerstein, M. K., Berglund, L. and Havel, P. J (2009). Consuming fructose-sweetened, not glucose-sweetened, beverages increases visceral adiposity and lipids and decreases insulin sensitivity in overweight/obese humans. J. Clin. Invest. 119(5):1322–1334.
- Steptoe, A., Gibson, E. L., Vuononvirta, R., Hamer, M., Wardle, J., Rycroft, J. A., Martin, J. F. and Erusalimsky, J. D. (2007). The effects of chronic tea intake on platelet activation and inflammation: A double-blind placebo controlled trial. Atherosclerosis. 193(2):277–282.
- Stirban, A., Negrean, M., Stratmann, B., Gawlowski, T., Horstmann, T., Götting, C., Kleesiek, K., Mueller-Roesel, M., Koschinsky, T., Uribarri, J., Vlassara, H. and Tschoepe, D. (2006). Benfotiamine prevents macro- and microvascular endothelial dysfunction and oxidative stress following a meal rich in advanced glycation end products in individuals with type 2 diabetes. Diabetes Care. 29:2064–2071.
- Stirban, A., Negrean, M., Stratmann, B., Götting, C., Salomon, J., Kleesiek, K. and Tschoepe, D. (2007). Adiponectin decreases postprandially following a heat-processed meal in individuals with type 2 diabetes. Diabetes Care. 30:2514–2516.
- Storlien, L. H., Jenkins, A. B., Chisholm, D. J., Pascoe, W. S., Khouri, S. and Kraegen, E. W. (1991). Influence of dietary fat composition on development of insulin resistance in rats. Relationship to muscle triglyceride and omega-3 fatty acids in muscle phospholipid. Diabetes. 40:280–289.
- Straczkowski, M., Kowalska, I., Nikolajuk, A., Dzienis-Straczkowska, S., Kinalska, I., Baranowski, M., Zendzian-Piotrowska, M., Brzezinska, Z. and Gorski, J. (2004). Relationship between insulin sensitivity and sphingomyelin signaling pathway in human skeletal muscle. Diabetes. 53:1215–1221.
- Tai, K., Need, A. G., Horowitz, M. and Chapman, I. M. (2008). Glucose tolerance and vitamin D: Effects of treating vitamin D deficiency. Nutr. (Burbank, Los Angeles County, Calif.). 24:950–956
- Tapola, N., Karvonen, H., Niskanen, L., Mikola, M. and Sarkkinen, E. (2005). Glycemic responses of oat bran products in type 2 diabetic patients. Nutr. Metab. Cardiovasc Dis. 15(4):255–261.
- Tappy, L., Gügolz, E. and Würsch, P. (1996). Effects of breakfast cereals containing various amounts of beta-glucan Fibres on plasma glucose and insulin responses in NIDDM subjects. Diabetes Care. 19(8):831–834.
- Thomas, D. and E. J. Elliott (2009). Low glycaemic index, or low glycaemic load, diets for diabetes mellitus. Cochrane Database Syst. Rev. (1):CD006296.
- Thomas, T. and Pfeiffer, A. F. H. (2012). Foods for the prevention of diabetes: How do they work? Diabetes Metab. Res. Rev. 2:25–49.
- Thompson, AK., Minihane, AM. and Williams, CM. (2011). Trans fatty acids, insulin resistance and diabetes. Eur. J. Clin. Nutr. 65:553–564.
- Thomsen, C., Storm, H., Holst, J. J. and Hermansen, K. (2003). Differential effects of saturated and monounsaturated fats on postprandial lipemia and glucagon-like peptide 1 responses in patients with type 2 diabetes. Amer. J. Clin. Nutr. 77:605–611.
- Tolhurst, G., Heffron, H., Lam, Y. S., Parker, H. E., Habib, A. M., Diakogiannaki, E., Cameron, J., Grosse, J., Reimann, F. and Gribble, F. M. (2012). Short-chain fatty acids stimulate glucagon-like peptide-1 secretion via the G-protein-coupled receptor FFAR2. Diabetes. 61(2):364–371.
- Torronen, R., Sarkkinen, E., Tapola, N., Hautaniemi, E., Kilpi, K. and Niskanen, L. (2010). Berries modify the postprandial plasma glucose response to sucrose in healthy subjects. Br. J. Nutr. 103(8):1094.
- Torsdottir and Andersson, H. (2009). Effect on the postprandial glycaemic level of the addition of water to a meal ingested by healthy subjects and type 2 (non-insulin-dependent) diabetic patients. Diabetologia. 32:231–235.
- Torsdottir, I., Alpsten, M., Holm, G., Sandberg, A. S., and Tölli, J. (1991). A small dose of soluble alginate-fiber affects postprandial glycemia and gastric emptying in humans with diabetes. J Nutr. 121(6):795–799.
- Tremblay, F., Krebs, M., Dombrowski, L., Brehm, A., Bernroider, E., Roth, E. and Nowotny, P. (2005). Overactivation of S6 kinase 1 as a cause of human insulin resistance during increased amino acid availability. Diabetes. 54(9):2674–2684.
- Trowell, H. C., Southgate, D. A. T., Wolever, T. M. S., Leeds, A. R., Gassull, M. A., and Jenkins, D. J. A. (1976). Dietary fibre redefined. Lancet. 307/7966:967.
- Tsuneki, H., Ishizuka, M., Terasawa, M., Wu, J.-B., Sasaoka, T., and Kimura, I. (2004). Effect of green tea on blood glucose levels and serum proteomic patterns in diabetic (db/db) mice and on glucose metabolism in healthy humans. BMC Pharmacol. 4:18.
- Tunnicliffe, J. M. and Shearer, J. (2008). Coffee, glucose homeostasis, and insulin resistance: Physiological mechanisms and mediators. Appl. Physiol. Nutr Metab. 33(6):1290–1300.
- Turroni, F., Marchesi, J. R., Foroni, E., Gueimonde, M., Shanahan, F., Margolles, A., van, S. D. and Ventura, M. (2009). Microbiomic analysis of the bifidobacterial population in the human distal gut. ISME J. 3:745–751.
- UK Prospective Diabetes Study (UKPDS) Group. (1998) Intensive blood-glucose control with sulphonylureas or insulin compared with conventional treatment and risk of complications in patients with type 2 diabetes (UKPDS 33). Lancet. 352/9131:837–853.
- Um, S. H., Frigerio, F., Watanabe, M., Picard, F., Joaquin, M., Sticker, M. and Fumagalli, S. (2004). Absence of S6K1 protects against age- and diet-induced obesity while enhancing insulin sensitivity. Nature. 431/7005:200–205.
- van Dam, R. M. (2006). Coffee and type 2 diabetes: From beans to beta-cells. Nutr. Metab. Cardiovasc Dis. 16(1):69–77.
- van Dam, R. M. and Hu, F. B. (2005). Coffee consumption and risk of type 2 diabetes a systematic review. JAMA. 294:97–104.
- van Loon, L. J., Kruijshoop, M., Menheere, P. P., Wagenmakers, A. J., Saris, W. H. and Keizer, H. A. (2003). Amino acid ingestion strongly enhances insulin secretion in patients with long-term type 2 diabetes. Diabetes Care. 26:625–630.
- van Loon, L. J., Kruijshoop, M., Verhagen, H., Saris, W. H., and Wagenmakers, A. J. (2000a). Ingestion of protein hydrolysate and amino acid-carbohydrate mixtures increasespostexercise plasma insulin responses in men. J. Nutr. 130:2508–2513.
- van Loon, L. J., Saris, W. H., Verhagen, H. and Wagenmakers, A. J. (2000b). Plasma insulin responses after ingestion of different amino acid or protein mixtures with carbohydrate. Am. J. Clin. Nutr. 72:96–105.
- Visen, P., Saraswat, B., Visen, A., Roller, M., Bily, A., Mermet, C., He, K., Bai, N. I. S., Lemaire, B., Lafay, S. and Ibarra, A. (2009). Acute effects of Fraxinus excelsior L. seed extract on postprandial glycemia and insulin secretion on healthy. J. Ethnopharmacol. 126(2):226–232.
- Vitaglione, P., Napolitano, A. and Fogliano, V. (2008). Cereal dietary fibre: A natural functional ingredient to deliver phenolic compounds into the gut. Trends Food Sci. Tech. 19:451–463.
- Vrieze, A., van Nood, E., Holleman, F., Salojärvi, J., Kootte, R. S., Bartelsman, J. F., Dallinga-Thie, G. M., Ackermans, M. T., Serlie, M. J., Oozeer, R., Derrien, M., Druesne, A., van Hylckama Vlieg, J. E., Bloks, V. W., Groen, A. K., Heilig, H. G., Zoetendal, E. G., Stroes, E. S., de Vos, W. M., Hoekstra, J. B. and Nieuwdorp, M. (2012). Transfer of intestinal microbiota from lean donors increases insulin sensitivity in individuals with metabolic syndrome. Gastroenterology. 143(4):913–916.
- Vuksan, V., Rogovik, A. L., Jovanovski, E. and Jenkins, A. L. (2009). Fibre facts: Benefits and recommendations for individuals with type 2 diabetes. Curr. Diab. Rep. 9(5):405–411.
- Vuksan, V., Sievenpiper, J. L., Koo, V. Y., Francis, T., Beljan-Zdravkovic, U., Xu, Z. et al. (2000a). American ginseng (Panax quinquefolius L) reduces postprandial glycemia in nondiabetic subjects and subjects with type 2 diabetes mellitus. Arch. Intern. Med. 160:1009–1013.
- Vuksan, V., Sievenpiper, J. L., Sung, M. K., Di Buono, M., Stavro, M. P., Jenkins, A. L. et al. (2003). Safety and efficacy of Korean red ginseng intervention (SAEKI): Results of a double-blind, placebo-controlled crossover trial in type 2 diabetes. Diabetes. 52(1):A:137.
- Vuksan, V., Sievenpiper, J. L., Wong, J., Xu, Z., Beljan-Zdravkovic, U., Arnason, J. T. et al. (2001). American ginseng (Panax quinquefolius L.) attenuates postprandial glycemia in a time-dependent but not dose-dependent manner in healthy individuals. Am. J. Clin. Nutr. 73:753–758.
- Vuksan, V., Stavro, M. P., Sievenpiper, J. L., Koo, V. Y., Wong, E., Beljan-Zdravkovic, U. et al. (2000b). American ginseng improves glycemia in individuals with normal glucose tolerance: Effect of dose and time escalation. J. Am. Coll. Nutr. 19:738–744.
- Vuksan, V., Stavro, M. P., Sievenpiper, J. L., Beljan-Zdravkovic, U., Leiter, L. A., Josse, R. G. et al. (2000c). Similar postprandial glycemic reductions with escalation of dose and administration time of American ginseng in type 2 diabetes. Diabetes Care. 23:1221–1226.
- Vuksan, V., Sung, M. K., Sievenpiper, J. L., Stavro, P. M., Jenkins, A. L., Di Buono, M, Lee, K. S., Leiter, L. A., Nam, K. Y., Arnason, J. T., Choi, M. and Naeem, A. (2008). Korean red ginseng (Panax ginseng) improves glucose and insulin regulation in well-controlled, type 2 diabetes: Results of a randomized, double-blind, placebo-controlled study of efficacy and safety. Nutr. Metab. Cardiovascular Dis. 18(1):46–56.
- Vuksan, V., Xu, Z., Jenkins, A. L., Beljan-Zdravkovic, U., Sievenpiper, J. L., Leiter, L. A. et al. (2000d). American ginseng improves long-term glycemic control in type 2 diabetes: Double-blind placebo controlled crossover trial (abstract). Diabetes. 49(1):A:95.
- Vuksan, V., Sievenpiper, J. L., Koo, V. Y., Francis, T., Beljan-Zdravkovic, U., Xu, Z. et al. (2000a). American ginseng (Panax quinquefolius L) reduces postprandial glycemia in nondiabetic subjects and subjects with type 2 dia-Q31 betes mellitus. Arch. Intern. Med. 160:1009–1013.
- Wedick, N. M., Brennan, A. M., Sun, Q., Hu, F. B., Mantzoros, C. S. and van Dam, R. M. (2011). Effects of caffeinated and decaffeinated coffee on biological risk factors for type 2 diabetes: A randomized controlled trial. Nutr. J. 10:93
- Weickert, M. O. (2012). What dietary modification best improves insulin sensitivity and why? Clin Endocrinol (Oxf). 2012 77(4):508–512.
- Weickert, M. O., Arafat, A. M., Blaut, M., Alpert, C., Becker, N., Leupelt, V., Rudovich, N., Mohlig, M. and Pfeiffer, A. F. (2011a). Changes in dominant groups of the gut microbiota do not explain cereal-Fibre induced improvement of whole-body insulin sensitivity. Nutr. Metab. (Lond). 8:90.
- Weickert, M. O., Möhlig, M., Koebnick, C., Holst, J. J., Namsolleck, P., Ristow, M., Osterhoff, M., Rochlitz, H., Rudovich, N., Spranger, J. and Pfeiffer, A. F. (2005). Impact of cereal fibre on glucose-regulating factors. Diabetologia. 48:2343–2353.
- Weickert, M. O., Möhlig, M., Schöfl, C., Arafat, A. M., Otto, B., Viehoff, H., Koebnick, C., Kohl, A., Spranger, J. and Pfeiffer, A. F. (2006). Cereal Fibre improves whole-body insulin sensitivity in overweight and obese women. Diabetes Care. 29:775–780.
- Weickert, M. O. and Pfeiffer, A. F. (2008). Metabolic effects of dietary Fibre consumption and prevention of diabetes. J. Nutr. 138:439–442.
- Weickert, M. O. and Pfeiffer, A. F. (2009). Low-glycemic index vs high-cereal Fibre diet in type 2 diabetes. Jama. 301:1538.
- Weickert, M. O., Roden, M., Isken, F., Hoffmann, D., Nowotny, P., Osterhoff, M., Blaut, M., Alpert, C., Gögebakan, O., Bumke-Vogt, C., Mueller, F., Machann, J., Barber, T. M., Petzke, K. J., Hierholzer, J., Hornemann, S., Kruse, M., Illner, A. K., Kohl, A., Loeffelholz, C. V., Arafat, A. M., Möhlig, M. and Pfeiffer, A. F. (2011b). Effects of supplemented isoenergetic diets differing in cereal Fibre and protein content on insulin sensitivity in overweight humans. Am. J. Clin. Nutr. 94:459–471.
- Westerterp-Plantenga, M. S., Lemmens, S. G. and Westerterp, K. R. (2012). Dietary protein—Its role in satiety, energetics, weight loss and health. Br. J. Nutr. 108(2):S105–S112.
- West, S., Hecker, K., Mustad, V., Nicholson, S., Schoemer, S., Wagner, P., Hinderliter, A., Ulbrecht, J., Ruey, P. and Kris-Etherton, P. (2005). Acute effects of monounsaturated fatty acids with and without omega-3 fatty acids on vascular reactivity in individuals with type 2 diabetes. Diabetologia. 48:113–122.
- Wheeler, M. L. and Fineberg, S. E., et al. (1990). Metabolic response to oral challenge of hydrogenated starch hydrolysate versus glucose in diabetes. Diabetes Care. 13(7):733–740.
- Wheeler, M. L. and Pi-Sunyer, F. X. (2008). Carbohydrate Issues: Type and Amount. J. Amer. Dietetic Ass. 108(4):S34–S39.
- Whiting, D. R., Guariguata, L., Weil, C. and Shaw, J. (2011). IDF diabetes atlas: Global estimates of the prevalence of diabetes for 2011and 2030. Diabetes Res. Clin. Pract. 94(3):311–321.
- Williams, J. A., Choe, Y. S., Noss, M. J., Baumgartner, C. J. and Mustad, V. A. (2007). Extract of Salacia oblonga lowers acute glycemia in patients with type 2 diabetes. Amer. J. Clin. Nutr. 86(1):124–130.
- Williams, C. J., Fargnoli, J. L., Hwang, J. J., van Dam, R. M., Blackburn, G. L., Hu, F. B. and Mantzoros, C. S. (2008). Coffee consumption is associated with higher plasma adiponectin concentrations in women with or without type 2 diabetes: A prospective cohort study. Diabetes Care. 31(3):504–507.
- Wolever, T. M. S. and Bolognesi, C. (1996). Prediction f glucose and insulin responses of normal subjects after consuming mixed meals varying in energy, protein, fat, carbohydrate and glycemic index. J. Nutr. 126:2807–2812.
- Wolever, T. M., Gibbs, A. L., Mehling, C., et al. (2008). The Canadian Trial of Carbohydrates in Diabetes (CCD), a 1-y controlled trial of low-glycemic-index dietary carbohydrate in type 2 diabetes: No effect on glycated hemoglobin but reduction in C-reactive protein. Am. J. Clin. Nutr. 87:114–125.
- Wu, X., Ma, C., Han, L., Nawaz, M., Gao, F., Zhang, X., Yu, P., Zhao, C., Li, L., Zhou, A., Wang, J., Moore, J. E., Millar, B. C. and Xu, J. (2010). Molecular characterisation of the faecal microbiota in patients with type II diabetes. Curr. Microbiol. 61:69–78.
- Xu, X., Bittman, R., Duportail, G., Heissler, D., Vilcheze, C. and London, E. (2001a). Effect of the structure of natural sterols and sphingolipids on the formation of ordered sphingolipid/sterol domains (Rafts). J. Biol. Chem. 276:33540–33546.
- Xu, G., Kwon, G., Cruz, W. S., Marshall, C. A. and McDaniel, M. L. (2001b). Metabolic regulation by leucine of translation initiation through the mTOR-signaling pathway by pancreatic beta-cells. Diabetes. 50:353–360.
- Zambon, S., Friday, K., Childs, M., Fujimoto, W., Bierman, E. and Ensinck, J. (1992). Effect of glyburide and omega 3 fatty acid dietary supplements on glucose and lipid metabolism in patients with non-insulin-dependent diabetes mellitus. Amer. J. Clin. Nutr. 56:447–454.
- Zanchi, N. E., Guimarães-Ferreira, L., Siqueira-Filho, M. A., Gabriel Camporez, J. P., Nicastro, H., Seixas Chaves, D. F., Campos-Ferraz, P., Lancha, A. H., Jr., and de Oliveira Carvalh, C. R. (2012). The possible role of leucine in modulating glucose homeostasis under distinct catabolic conditions. Med. Hypotheses. 79(6):883–888.