Abstract
Pulses are nutrient-dense foods that have for a long time been empirically known to have beneficial effects in human health. In the last decade, several studies have gathered evidence of the metabolic benefits of pulse intake. However, it remains unclear at what amounts these effects may be attained. This study aimed to systematically review the scientific outputs of the last two decades regarding health benefits of pulse consumption and the amounts necessary for positive outcomes to be achieved. A PubMed search including keywords [(“dietary pulses”, “pulses”, “legumes”, “grain legumes”, “bean”, “chickpea”, “pea”, “lentil”, “cowpea”, “faba bean”, “lupin”) and (“inflammation”, “inflammatory markers”, “C-reactive protein”, “blood lipids”, “cholesterol”, “cardiometabolic health”, “cardiovascular disease”, “diabetes”, “glycaemia”, “insulin”, “HOMA-IR”, “body weight”, “body fat”, “obesity”, “overweight”, “metabolome”, “metabolic profile”, “metabolomics”, “biomarkers”, “microbiome”, “microbiota”, “gut”)] was performed. Only English written papers referring to human dietary interventions, longer than one day, focusing on whole pulses intake, were included. Most of the twenty eligible publications reported improvements in blood lipid profile, blood pressure, inflammation biomarkers, as well as, in body composition, resulting from pulse daily amounts of 150 g (minimum-maximum: 54-360 g/day; cooked). Concerns regarding methodological approaches are evident and the biochemical mechanisms underlying such effects require further investigation.
Introduction
The Food and Agriculture Organization of the United Nations (FAO) defines the term “pulses” as edible seeds of members of the Leguminosae family (FAO Citation1994). There are numerous varieties of pulses and FAO recognizes 11 main types: beans, broad beans, peas, chickpeas, cowpeas, pigeon peas, lentils, bambara beans, vetches, lupins, and other “minor” pulses (pulses other than those included in previous categories and with minor relevance at the international level). Nevertheless, beans, peas, lentils and chickpeas are amongst the most commonly known and frequently consumed pulses worldwide and have been so for a long time (Marinangeli et al. Citation2017).
In the last few years, pulses have drawn attention, not only for their main role as ecologically sustainable protein food sources (Mcdermott and Wyatt Citation2017; Foyer et al. Citation2016), compared to animal-based foods, but also for their unique nutrient-rich profile (Hall, Hillen, and Robinson Citation2017), in particular, their high protein content. Generally, pulse protein contents range between 17% and 30% (dry weight) (Boye, Zare, and Pletch Citation2010). A recent report from the European Commission to the Council and the European Parliament on the development of plant proteins in the European Union preconizes a change in consumer behavior and preferences, including rebalancing plant versus animal protein in human consumption (European Commission Citation2018). Pulses have a high content of the essential amino acid lysine (∼64 ± 10 mg/g of protein) (Young and Pellett Citation1994), which is often found in low levels in cereal grains, thus, consumers following plant-based diets may fall short in meeting lysine needs if pulses are not included in their eating habits (Messina Citation2014).
Pulses are also characterized by having relatively low energy density, providing approximately 1.3 kcal/g (based on a cooked serving) (Mudryj, Yu, and Aukema Citation2014). Even though ∼50–65% of their weight represents carbohydrate content (Havemeier, Erickson, and Slavin Citation2017), they are slowly digested, which contributes for their lower glycaemic index (GI) when compared to other carbohydrate-rich foods such as rice, white bread or potatoes (Rizkalla, Bellisle, and Slama Citation2002). Adding to this is the fact that pulses can provide up to 30 g of fiber per 100 g dry weight (beans: 23–32 g; chickpeas: 18–22 g; lentils: 18–20 g; peas: 14–26 g), the majority of which as insoluble fiber (beans: 20–28 g; chickpeas: 10–18 g; lentils: 11–17 g; peas: 10–15 g per 100 g of dry weight) (Tosh and Yada Citation2010). Hence, pulses are generally considered fiber-rich foods, although the precise amount varies depending on the type of grain legume. Another positive characteristic of pulses is their low-fat content and healthier lipid profile, since they are sources of mono- and polyunsaturated fats (Grela et al. Citation2017), as well as of plant sterols (Singh, Singh, and Singh Citation2016). Pulses also stand as excellent food sources of micronutrients, including minerals such as potassium, iron and zinc (Grela et al. Citation2017), as well as vitamins like thiamin, niacin, folate, riboflavin, pyridoxine, vitamin E and A (Mudryj, Yu, and Aukema Citation2014). Finally, pulses provide important dietary bioactive compounds to the diet (e.g. phenolic acids, tannins, and flavonoids) known for their antioxidant potential, amongst other health-protective effects (Singh, Singh, and Singh Citation2016).
Accordingly, it is hypothesized that the pulses’ nutrient composition, especially their protein, fiber, mineral and phytochemical contents, may contribute to important health benefits. Growing bodies of research provide low- to moderate-quality evidence that pulse rich diets favorably affect conditions such as cardiovascular diseases (Arnoldi et al. Citation2015), diabetes (Sievenpiper et al. Citation2009), as well as obesity and its related co-morbidities (Kim et al. Citation2016; Rebello, Greenway, and Finley Citation2014; Li et al. Citation2014). Thus, efforts have been carried out to maintain the presence of such foods in traditional pulse-eating communities, as well as to foster their reintroduction into more western diet-based countries (Calles Citation2016). Particularly in the latter, it becomes urgent to adopt more plant based food patterns in order to counteract serious global health, food insecurity and sustainability problems (Mcdermott and Wyatt Citation2017; Foyer et al. Citation2016).
For the reasons above, pulses feature in many official dietary guidelines for healthy eating and disease management, such as the Mediterranean Diet (Bach-Faig et al. Citation2011), The Dietary Approaches to Stop Hypertension (DASH) (Harsha et al. Citation1999) and even the Cancer Prevention Recommendations from the World Cancer Research Fund International and the American Institute for Cancer Research (World Cancer Research Fund International Citation2018). However, there seems to be great variability in pulse intake recommendations and it remains unclear at what amounts health beneficial effects may be achieved (Marinangeli et al. Citation2017). Therefore, this review aims to gather the scientific output of the last two decades regarding the metabolic benefits of whole pulse consumption and clarify the necessary amounts for health positive outcomes to be achieved through increased pulse intake.
Methods
To conduct the present systematic review of literature, a slightly modified PRISMA (Preferred Reporting Items for Systematic reviews and Meta-Analyses) checklist was followed (Moher et al. Citation2009). The identification of papers was conducted through a search on PubMed with the following key-words or expressions [(“dietary pulses” OR “pulses” OR “legumes” OR “grain legumes” OR “bean” OR “beans” OR “chickpea” OR “pea” OR “lentil” OR “cowpea” OR “faba bean” OR “lupin”) AND (“inflammation” OR “inflammatory markers” OR “C-reactive protein” OR “blood lipids” OR “cholesterol” OR “cardiometabolic health” OR “cardiovascular disease” OR “diabetes” OR “glycaemia” OR “insulin” OR “HOMA-IR” OR “body weight” OR “body fat” OR “obesity” OR “overweight” OR “metabolome” OR “metabolic profile” OR “metabolomics” OR “biomarkers” OR “microbiome” OR “microbiota” OR “gut”)]. The search was performed from December 2017 to July 2018, considering the following inclusion criteria: human based clinical trials, published since 1997 and written in English. Relevant papers found alongside the systematic search described, for example, retrieved from cited references or PubMed online suggestions, were also reviewed (n = 8). In total, the search retrieved 371 publications.
After a first screening, 326 papers were excluded based on abstract reading (Figure1), considering the following exclusion criteria: clinical trials without an oral food-based intervention or with unsuitable endpoints (n = 192), food-based interventions focusing on specific diets or food patterns (n = 27), food-based interventions focusing on foods other than pulses (n = 48), food-based interventions focusing on pulse-based food products or extracts (n = 59). Notably, whenever the focus of the manuscript was not clear on the title and/or abstract sections, the full text was examined in order to decide about inclusion or exclusion. The remaining papers (n = 45) were analyzed, and 25 further publications were excluded accordingly to the following criteria: food-based interventions focusing on pulse-based food products or extracts (n = 6), pulse-based interventions with unsuitable endpoints (n = 18) and a study protocol (n = 1). Finally, 20 articles were considered, fulfilling the inclusion criteria, comprising human dietary interventions, at least for two weeks and focusing on whole pulses intake (Figure1). The search and papers selection were conducted by the first author, however when doubts arose regarding the inclusion/exclusion of a paper, the decision was taken together with the other authors.
Results
Study design
In line with the inclusion criteria, all studies reviewed (n = 20; 100%) comprised human dietary interventions, focusing on whole pulses intake and their potential metabolic effects. The most significant amount of papers was published between the year of 2005 and 2015 (n = 18; 90.0%). Overall, dietary interventions took place in several countries around the world, however more expressively in Iran (n = 6; 30.0%), followed by the United States of America (n = 5; 25.0%). All studies were randomized controlled food trials, yet around half of them (n = 11; 55.0%) also applied a cross-over design.
Regarding the studied populations, all dietary interventions were performed on adults but only one (Fernando et al. Citation2010) exclusively specifically considered healthy individuals, according to the authors’ definition. Considering the remaining publications, subjects can be grouped as follows: overweight/obese with or without other CVD risk factors (n = 12, 60.0%) (Duane Citation1997; Crujeiras et al. Citation2007; Winham, Hutchins, and Johnston Citation2007; Abete, Parra, and Martinez Citation2009; Pittaway, Robertson, and Ball Citation2008; Venn et al. Citation2010; Trinidad et al. Citation2010; Hermsdorff et al. Citation2011; Abeysekara et al. Citation2012; Alizadeh, Gharaaghaji, and Gargari Citation2014; Tonstad, Malik, and Haddad Citation2014; Safaeiyan et al. Citation2015); at risk for diabetes or diabetic patients (n = 5; 25.0%) (Jenkins et al. 2012; Hosseinpour-Niazi, Mirmiran, Hedayati, et al. Citation2015; Hosseinpour-Niazi, Mirmiran, Fallah-Ghohroudi, et al. Citation2015; Saraf-Bank et al. Citation2016, Citation2015); at risk for colorectal cancer (n = 2; 10.0%) (Hartman et al. Citation2010; Zhang et al. Citation2010). Sample size varied significantly between publications, with a minimum number of n = 9 and a maximum of n = 108. Nevertheless, the majority of papers studied the effect of pulse consumption on subjects from both genders (n = 14; 70.0%).
Dietary interventions
Where pulse intake is concerned, publications implied an average amount of 150 g (minimum-maximum: 54-360 g/day; cooked) of pulses per day (n = 12; 60.0%) and most trial diets (n = 12; 60.0%) lasted between 4 to 8 weeks (1-2 months). Only 3 of the 20 reviewed papers focused on the metabolic effect of specific grain legumes (Pittaway, Robertson, and Ball Citation2008; Winham, Hutchins, and Johnston Citation2007; Fernando et al. Citation2010), whereas the other 17 studies (85.0%) tested the simultaneous effect of different types of pulses. However, trial diets differed significantly between studies and generally ranged within the following groups: controlled diets with meals provided by the research team (n = 3; 15.0%) (Duane Citation1997; Hartman et al. Citation2010; Zhang et al. Citation2010); usual diets plus pulses provided by the research team (n = 5; 25.0%) (Winham, Hutchins, and Johnston Citation2007; Pittaway, Robertson, and Ball Citation2008; Fernando et al. Citation2010; Trinidad et al. Citation2010; Abeysekara et al. Citation2012); prescription of specific healthy dietary guidelines plus pulses provided by the research team (n = 2; 10.0%) (Saraf-Bank et al. Citation2015, Citation2016); prescription of energy restricted (n = 5; 25.0%) (Crujeiras et al. Citation2007; Abete, Parra, and Martinez Citation2009; Hermsdorff et al. Citation2011; Alizadeh, Gharaaghaji, and Gargari Citation2014; Safaeiyan et al. Citation2015) or nutrient modified (n = 1; 5.0%) (Tonstad, Malik, and Haddad Citation2014) diets including pulses; prescription of specific healthy dietary guidelines including pulses (n = 3; 15.0%) (Venn et al. Citation2010; Hosseinpour-Niazi, Mirmiran, Fallah-Ghohroudi, et al. Citation2015; Hosseinpour-Niazi, Mirmiran, Hedayati, et al. Citation2015); prescription of pulse intake target plus add libitum diets (n = 1; 5.0%) (Jenkins et al. 2012).
Metabolic outcomes
Considering the analytical methods applied, the metabolic impact was accessed mainly through common standard biofluid analysis and blood was the most common biological sample collected (n = 19; 95%). In terms of endpoints, the majority of the publications focused essentially on the assessment of changes on four key individual features: body composition (n = 19; 95.0%), blood lipid profile (n = 17; 85.0%), blood pressure (n = 14; 70.0%) and glycaemic control (n = 14; 70.0%). Main intervention outcomes () reported meaningful improvements in blood lipid profile (n = 13; 65.0%), blood pressure (n = 4; 20.0%), inflammation biomarkers (n = 4; 20.0%), as well as, anthropometric measurements, such as body weight (n = 4; 20.0%) and waist circumference (n = 4; 20.0%). Three (15.0%) publications reported the occurrence of adverse events within the dietary intervention, namely, upset stomach, flatulence, bloating, heartburn and diarrhea (Abeysekara et al. Citation2012; Jenkins et al. 2012; Tonstad, Malik, and Haddad Citation2014). Intervention studies are summarized in and results are briefly described below. Information was grouped and presented according to each study objective, design and population studied, starting with the results on healthy subjects for gut microbiota modulation, followed by weight loss management objectives, CVD risk management approaches, and finally diabetes risk reduction.
Figure 1. Diagram of systematic literature search (Search time frame: 1997-2018; database: PubMed; language: English; study design: human based food clinical trials).
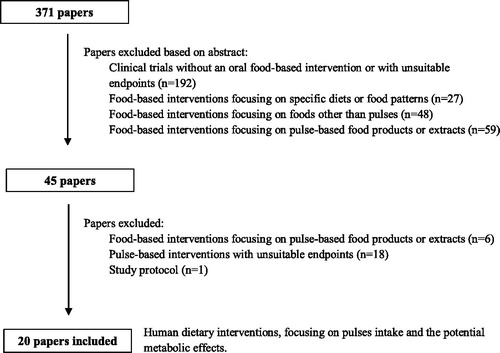
Figure 2. Main metabolic outcomes observed after whole pulse consumption (n = 20; HbA1c – hemoglobin A1c; ↓ - decreased; ↑ - increased).
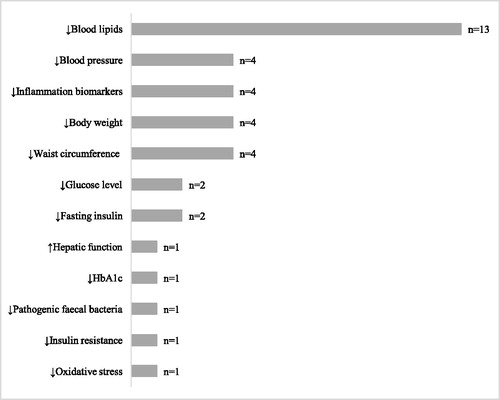
Table 1. Summary of characteristics of the trials included in the present systematic review of human dietary interventions assessing the effect of dietary pulses on metabolism and health.
Gut microbiota modulation approach
In a parallel randomized control trial, the fortification of the usual diet of 12 healthy adults with 200 g per day of canned chickpea (≈ 2 servings per day; cooked), for 3-week periods, led to a lower number of individuals testing positive pathogenic gut bacteria species and putrefactive gut bacteria. Less intestinal colonization by a high ammonia-producing bacterial isolate was also observed for the chickpea group (42%), in relation to the other two treatments (raffinose group: 92%; control: 83%) (Fernando et al. Citation2010).
Weight loss management approach
All energy-restricted dietary approaches were tested within parallel randomized control designs. Greater weight reduction was observed in thirty obese subjects who followed an 8-week energy-restricted non-soy legume-enriched diet (4 servings/week; cooked) as compared to control, namely, −7.7 ± 3.0% versus −5.3 ± 2.7% (p = 0.023) (Crujeiras et al. Citation2007). Such decrease in body weight correlated with diet fiber content (r = 0.46; p = 0.014). In addition, plasma total cholesterol (TC) concentration reductions were significantly higher in comparison to control diet, which is −14.4 ± 10.6% versus −3.9 ± 10.7% (p < 0.001). Indeed, the decrease in TC appeared to be directly correlated with weight reduction (r = 0.50; p = 0.006) and increases in fiber intake (r = 0.44; p = 0.022). Legume-enriched diet induced a statistically significant decline as well in lipid peroxidation biomarkers, namely, plasma malondialdehyde (MDA) (p = 0.008) and urinary 8-isoprostane- F2α (8-iso-PGF2α) (p = 0.035), as compared to baseline, despite no statistical differences between control diet were seen. Such changes were positively associated with reductions in TC concentrations (r = 0.50; p = 0.006). A similar work found an 8-week legume-rich diet (4 servings/week) to be able to produce as well one of the highest percentage of weight loss (-8.4 ± 1.2%) among thirty-five obese men, compared with the control diet (−5.5 ± 2.5%; p = 0.042) (Abete, Parra, and Martinez Citation2009). The legume diet phase was also associated with reductions in waist circumference (WC) (baseline vs. endpoint: −7.0 ± 3.0%; p < 0.05) and fat mass (FM) (baseline vs. endpoint: −15.1 ± 6.6%; p < 0.05) but with no statistically significant differences from other dietary groups (control vs. high-protein diet vs. fatty fish diet). Nevertheless, a significantly 30.1 ± 2.5% higher percentage of mitochondrial oxidation was observed for the legume-rich diet when compared to control (+26.4 ± 4.6%; p < 0.021). Noteworthy, significant improvements in blood pressure measurements were only observed for the legume approach, significantly different from control diet as well. For instance, systolic blood pressure (SBP) reduced about −9.6 ± 5.1% versus −2.5 ± 4.8% within control group (p < 0.05). Statically significant changes in blood lipid profiles were also almost exclusive to the legume phase, with reductions at endpoint of −19.5 ± 9.3% for TC and of −25,5 ± 11.8% for LDL cholesterol (LDL-C), in relation to baseline (p < 0.05). Such type of diet (8-week legume-based hypocaloric diet; 4 servings/week) also seemed to improve the proinflammatory status and metabolic features in thirty overweight subjects (Hermsdorff et al. Citation2011). Compared to a legume-free control diet, the legume-rich phase induced larger bodyweight loss, that is, −7.8 ± 2.9% versus −5.3 ± 2.7 (p = 0.024). Decreases in FM percentage and WC were perceived as well, but not statistically different between experimental groups. Additionally, the legume diet produced significantly greater reductions in C-reactive protein (CRP) and complement C3 (C3) concentrations, compared to both baseline (CRP: 2.7 ± 2.4 vs. 1.6 ± 0.9 mg/L, p < 0.01; C3: 1.5 ± 0.2 vs. 1.1 ± 0.2 g/L, p < 0.05) and control assessments (CRP: −1.2 ± 3.2 vs. +0.4 ± 2.8 mg/dL, p < 0.044; C3: −0.8 ± 0.7 vs. +0.4 ± 0.4 g/L, p < 0.044), even after adjusting for weight loss. Noteworthy, the legume-rich diet was the only approach inducing significant reductions in SBP (baseline: 115 ± 13 vs. endpoint: 106 ± 10 mg/L; p < 0.01), TC (baseline: 215 ± 27 vs. endpoint: 182 ± 27 mg/dL; p < 0.01), LDL-C (baseline: 142.2 ± 41 vs. endpoint: 121 ± 28 mg/dL; p < 0.01) and HDL cholesterol (HDL-C) (baseline: 49 ± 10 vs. endpoint: 44 ± 7 mg/dL; p < 0.05) measures. Also, reductions in plasma CRP concentrations seen during the legume phase were positively associated with decreases in SBP (intervention: r = 0.78, p = 0.001; control: r=−0.11, p = 0.721), as well as with TC levels (intervention: r = 0.81, p = 0.001; control: r=-0.47, p = 0.750). Results indicate as well that a shorter intervention, that is a 6-week hypocaloric diet enriched in non-soy legumes (1 cup or 2 servings per day; cooked), was able to reduce WC by 4.6% (p < 0.001) and SBP by 8% (p = 0.009) among a group of 34 women with central obesity (Alizadeh, Gharaaghaji, and Gargari Citation2014). In the legume group, percent of the decrease in triglycerides (TG) between 3rd and 6th weeks and 1st and 6th weeks was statistically significant, that is, 9% (p = 0.009) and 12% (p = 0.05), respectively. Both dietary approaches significantly increased fasting insulin (FI) concentration after 3 weeks (intervention: 31%, p = 0.039; control: 39%, p = 0.03), but their significant effects disappeared after 6 weeks. Homeostatic Model Assessment of Insulin Resistance (HOMA-IR) was statistically increased in both groups in the first 3 weeks as well (intervention: 35%, p = 0.002; control: 38%, p = 0.049) but the legume-based intervention returned it to basal levels in the subsequent 3 weeks (29%; p = 0.031). Lastly, the legume approach produced statistically significant decreases in relevant hepatic enzymes levels, namely, aspartate aminotransferase (AST) and alanine aminotransferase (ALT), between the 3rd and 6th weeks, that is, 30% (p < 0.001) and 46% (p = 0.038), respectively. In contrast, other publication reveals that 6-week hypocaloric diets with or without non-soy legumes (1 cup or 2 servings per day; cooked) were equally capable of producing statistically significant reductions on CRP level, among thirty-four premenopausal women with central obesity (Safaeiyan et al. Citation2015). This specific effect was seen in the first 3 weeks, despite returning to basal levels after 6 weeks. The legume diet was associated with higher total antioxidant capacity after the first 3 weeks of the trail (+4.0%; p = 0.050). Also, a 16-week high-fiber bean-rich diet (½ cup or 1 serving per day; cooked) was proved to be as effective as a low-carbohydrate diet for weight loss among a group of a hundred and twenty-three obese participants (Tonstad, Malik, and Haddad Citation2014). Still, the bean-rich diet alone was capable of lowering atherogenic lipids, namely TC (intervention: −0.2 ± 0.6 vs. control: 0.1 ± 0.6 mmol/L; p < 0.038) and LDL-C (intervention: −0.2 ± 0.6 mmol/L vs. control: 0.1 ± 0.6 mmol/L; p < 0.045).
CVD risk management approach
In a parallel randomized control trial, daily consumption of 180 g of canned pulses (≈ 2 servings per day) for 18 months, revealed a decrease in WC of −2.8 cm (p < 0.05) among 108 volunteers. Other clinically relevant parameters, such as blood pressure, TG level, and glycaemic load (GL) were lessened as well compared to baseline, but with no statistically significant differences from the control group (Venn et al. Citation2010). Where cross-over randomized control trials are concerned, nine male subjects living on the metabolic ward showed a significant reduction in the mean serum LDL-C during a 6-week pulse rich diet (360 g or 4 servings per day; cooked), namely, 138 mg/dl versus 126 mg/dl (p = 0.039), associated with higher mean cholesterol saturation index of gallbladder bile (1.07 to 1.26; p = 0.016), as well as, greater mean cholesterol hepatic secretion (90.2 μmol/h to 100.8 μmol/h; p = 0.042) (Duane Citation1997). Moreover, significant improvements were reported in total and LDL-C profile among sixteen free-living mildly insulin-resistant adults, following an 8-week pinto bean-enriched diet (½ cup or 1 serving per day; canned) in relation to placebo, namely, −19 ± 5 mg/dl versus 1 ± 5 mg/dl (p = 0.011) and −14 ± 4 versus 1 ± 4 mg/dL (p = 0.013), respectively (Winham, Hutchins, and Johnston Citation2007). Similarly, mean serum TC and LDL-C was found to be less in a group of forty five free-living adults, after the consumption of a 12-week chickpea-enriched diet (104 g or ≈ 1 serving per day; cooked), namely, −7.7 mg/dL (p = 0.002) and −7.3 mg/dL (p = 0.01), respectively (Pittaway, Robertson, and Ball Citation2008). The intervention diet was also able to reduce FI by 0.75 μIU/mL (p = 0.045) and HOMA-IR by 0.21 (p = 0.01) in this group. Within the Legume Inflammation Feeding Experiment (Hartman et al. Citation2010), a 4-week legume-enriched (250 g or ≈3 servings per day; cooked; beans) low-GI diet, was equally capable of favorably improving CRP (-20.2 vs. −18.3%; p > 0.05) and soluble tumor necrosis factor-α receptor I (sTNFRI) (-3.7 vs. −4.4%; p > 0.05) concentrations, compared to a healthy American diet, among sixty-four middle-aged men, characterized for colorectal adenomas and insulin resistance status. In this context, the adenoma status seam not to interact with dietary treatments. Within the same experiment, another publication (Zhang et al. Citation2010) reported that the legume diet led to greater declines in both fasting serum TC and LDL-C, that is, -11 ± 3 mg/dL (p < 0.001) and -9 ± 3 mg/dL (p < 0.01), respectively. Insulin-sensitive subjects showed larger reductions in CVD lipid risk factors, such as, TC (-14 ± 4 mg/dL, p < 0.001), LDL-C (-10 ± 4 mg/dL, p < 0.01), TC/HDL-C (-0.30 ± 0.10, p < 0.01), and LDL-C/HDL-C (-0.20 ± 0.08, p = 0.02) after the legume diet, in relation to control. Also, daily intake (1 serving) of kidney beans for just 2 weeks, significantly lowers both total (-6.0%; p < 0.05) and LDL-C (-9.0%; p < 0.05) among twenty moderately hypercholesterolemic individuals (Trinidad et al. Citation2010). An 8-week pulse-based diet (250 g or ≈3 servings per day; cooked) seem able to reduce CVD risk factors specifically among individuals with fifty years or older (Abeysekara et al. Citation2012). Compared to the regular diet, the pulse-based diet seem to be able to decrease TC by 8.3% (intervention: 4.57 ± 0.93 to 4.11 ± 0.91 mmol/L; control: 4.47 ± 0.94 to 4.39 ± 0.97 mmol/L; p < 0.001) as well as LDL-C by 7.9% (intervention: 2.93 ± 0.84 to 2.55 ± 0.75 mmol/L; control: 2.96 ± 0.86 to 2.81 ± 0.83 mmol/L; P = 0.01), in a group of eighty-seven participants. Other dependent variables measured from blood sampling showed no significant changes between diets.
Diabetes risk management approach
Regarding parallel randomized control trials, the inclusion of 190 g per day (2 servings) of cooked legumes (beans, chickpeas or lentils) as part of a 12-week low-glycaemic index diet helped improve both glycaemic control and reduced calculated coronary heart disease (CHD) risk score among sixty type 2 Diabetes Mellitus patients (Jenkins et al. 2012). Results show weight loss appeared significantly higher for the legume group (−2.7 kg vs. −2.0 kg; p = 0.002) in relation to a high wheat fiber control diet. Reduction in WC was significantly superior in the intervention group as well (−1.4 cm; p = 0.007), as happened in TC level (−8 mg/dL; p = 0.005). Moreover, BP and heart rate (HR) were reduced during the legume diet, namely, SBP lowered −4.5 mmHg (p < 0.001), Diastolic Blood Pressure (DBP) reduced −3.1 mmHg (p < 0.001) and HR lowered −3.1 beats per minute (p < 0.001). Likewise, the relative reduction in HbA1c values within the legume group was higher by −0.2% (p < 0.001) in relation to the control group. All in all, the legume diet reduced the CHD risk by −0.8% (p = 0.003), largely owing to a higher relative decrease in SBP compared to the control diet. On the other hand, considering cross-over randomized control trials, the substitution of red meat with non-soy legumes (3 cups/week or ≈ 1 serving/day; cooked) within the Therapeutic Lifestyle Change Diet helped improve cardiometabolic risk factors in a group of thirty-one overweight type 2 diabetes patients (Hosseinpour-Niazi, Mirmiran, Fallah-Ghohroudi, et al. Citation2015; Hosseinpour-Niazi, Mirmiran, Hedayati, et al. Citation2015). Indeed, the 8-week legume-based diet significantly decreased fasting blood glucose (FBG) (-28.7 ± 6.7 vs. −19.5 ± 5.5 mg/dL; p < 0.001), FI (-3.5 ± 0.4 vs. −1.5 ± 0.5 μIU/mL; p = 0.006), TG (-38.5 ± 6.6 vs. −19.5 ± 6.4 mg/dL; p = 0.02) and LDL-C (-15.6 ± 5.1 vs. −8.7 ± 2.7 mg/dL; p = 0.02), compared to the control legume-free approach. Additionally, there was a reduction in inflammatory biomarkers among intervention group, namely, CRP (-1.7 ± 1.2 vs. −1.3 ± 1.1 mg/L; p = 0.019), Interleukin-6 (IL-6) (-1.6 ± 1.1 vs. −1.2 ± 1.0 pg/mL; p = 0.018) and Tumor Necrosis Factor-α (TNF-α) (-1.8 ± 1.1 vs. −1.3 ± 1.1 pg/mL; p = 0.018). Such effects were seen under weight-maintenance conditions. In turn, four servings of non-soy legumes per week (111.12 ± 10.53 g/day; cooked), over a 6-week period, among twenty-six individuals with family history of diabetes, had no significant effect on anthropometric measurements and most biochemical parameters, though it could marginally reduce SBP (baseline: 113.65 ± 2.69 vs. endpoint: 109.05 ± 2.57 mmHg; p = 0.08) and DBP (baseline: 77.88 ± 1.54 vs. endpoint: 75.00 ± 1.79 mmHg; p = 0.07) (Saraf-Bank et al. Citation2015, Citation2016). However, the legume-rich diet was able to significantly reduce CRP level compared to the habitual diet, namely, −4.86 ± 1.86% versus 3.55 ± 1.97% (p = 0.002).
Discussion
Regarding main intervention outcomes (see ), most trials reported the indirect effects of pulse consumption on CVD risk factors. Results consistently emphasize the blood lipid-lowering potential of pulses as part of health promoting diets and even if for some situations, the variations in metabolic markers seem not to reach statistical significance (Abeysekara et al. Citation2012; Hartman et al. Citation2010; Venn et al. Citation2010; Saraf-Bank et al. Citation2016), they may sometimes reveal themselves as clinically relevant. For example, in the absence of statistically significant differences, a pulse-based diet was capable of placing subjects within more favorable cardiovascular risk categories, where pharmacologic treatment could indeed be delayed, such as the use of statins for the management of blood lipid disorders (Abeysekara et al. Citation2012).
As far as negative impact is concerned, few publications (n = 3; 15.0%) reported the occurrence of adverse events, mainly gastrointestinal symptoms, considered related to pulse-rich diets (Abeysekara et al. Citation2012; Jenkins et al. 2012; Tonstad, Malik, and Haddad Citation2014). Noteworthy, researchers highlighted both the low frequency and the lack in severity of the adverse events observed and affirmed that none of the participants withdrew from the studies because of these symptoms (Abeysekara et al. Citation2012; Jenkins et al. 2012). Such results help restore pulses’ good reputation as the occurrence of intestinal discomfort becomes an overstated motif for their exclusion from the everyday diet (Messina Citation2014; Havemeier, Erickson, and Slavin Citation2017).
Numerous explanations have been proposed for the observed metabolic outcomes that mainly spring from the nutrient-dense profile of grain legumes, namely, high content of fiber and other non-digestible fermentable components (e.g. resistant starches), several vital minerals (e.g. magnesium), as well as, diverse bioactive compounds (e.g. phenolic acids) (Safaeiyan et al. Citation2015; Saraf-Bank et al. Citation2016; Hosseinpour-Niazi, Mirmiran, Hedayati, et al. Citation2015).
Bodyweight, glycaemic control and lipid profile
Commonly, a serving of pulses provides significant amounts of both soluble and insoluble fiber (Crujeiras et al. Citation2007). Scientific literature indicates that pulse intake benefits are closely linked to the ability of dietary fiber to i) act as a physiological obstacle to energy and nutrient intake and ii) suffer gut microbial fermentation action. On one hand, it is suggested that fiber, in particular the insoluble part, could physically block energy intake, displacing available calories and nutrients from the diet, increasing satiety and decreasing the absorption efficiency of, for example, carbohydrates and fats (Crujeiras et al. Citation2007; Trinidad et al. Citation2010; Zhang et al. Citation2010; Abeysekara et al. Citation2012; Saraf-Bank et al. Citation2016). Pulse intake is therefore associated with hypocaloric and low glycaemic index/load diets, which in turn are widely advocated for their weight-loss promoting properties and ability to improve blood lipid profile, glycaemic control and overall inflammatory status (Crujeiras et al. Citation2007; Trinidad et al. Citation2010; Abete, Parra, and Martinez Citation2009; Hermsdorff et al. Citation2011; Jenkins et al. 2012; Tonstad, Malik, and Haddad Citation2014; Safaeiyan et al. Citation2015). In fact, it appears that hypocaloric diets containing pulses, along with high-protein hypocaloric diets, are more effective than a regular calorie-restrictive diet for reducing body weight and also improving some cardiovascular risk factors (Abete, Parra, and Martinez Citation2009). According to the authors, such diets tended to decrease more body fat mass which could be linked to, not only changes in insulin concentrations, since less insulin promotes free fatty acid mobilization from body fat storages, but also activation of mitochondrial oxidation (Abete, Parra, and Martinez Citation2009). In turn, it is claimed that the lower GI and GL of the pulse diet plays an important role in favorably altering blood lipid concentrations by reducing insulin resistance (Zhang et al. Citation2010). Since insulin inhibits the mobilization of free fatty acids from adipose tissue, it can induce a reduction in hepatic production of very low density lipoprotein (VLDL) and maintain low levels of TC and LDL-C (Zhang et al. Citation2010; Saraf-Bank et al. Citation2016).
On the other hand, evidence indicates that the soluble part of fiber has the capacity to bind specifically to bile acids and cholesterol in the intestinal tract, during intraluminal formation of micelles (Crujeiras et al. Citation2007; Hermsdorff et al. Citation2011; Abeysekara et al. Citation2012; Hosseinpour-Niazi, Mirmiran, Hedayati, et al. Citation2015). A study of the effect of pulse consumption on sterol metabolism also revealed an increase in the secretion of cholesterol into bile, which may add to the negative cholesterol balance caused by the blocked absorption (Duane Citation1997). However, the exact mechanism by which pulse intake intensifies secretion of cholesterol into bile is not clear yet. Researchers hypothesize that some component of grain legumes, independent of their capacity to reduce bile acid absorption, such as saponins, once hydrolyzed by intestinal bacteria may directly stimulate biliary cholesterol secretion (Duane Citation1997; Hermsdorff et al. Citation2011).
Another proposed mechanism for the effects seen is the inhibition of fatty acid synthesis in the liver, mediated by fiber fermentation products, such as acetate, butyrate and propionate, also known as short chain fatty acids (Crujeiras et al. Citation2007; Abeysekara et al. Citation2012; Saraf-Bank et al. Citation2016). For instance, pulses contain high amounts of resistant starches and one of the end-products of resistant starches fermentation is propionate, a short chain fatty acid which has been shown to inhibit hepatic cholesterol synthesis in animal models (Winham, Hutchins, and Johnston Citation2007). In this context, it was reported that the prebiotic potential of pulses may truly positively modulate fecal microbial composition in healthy adults, associated with an increase in Bifidobacterium spp. and a decrease in pathogenic and putrefactive bacteria (e.g. Clostridium), adding to the previously mentioned overall metabolic benefits of dietary fiber alone (Fernando et al. Citation2010).
Blood pressure
The mineral content of pulses may account for some of their cardio-protective effects, through the reduction of hypertension risk (Hermsdorff et al. Citation2011; Alizadeh, Gharaaghaji, and Gargari Citation2014; Jenkins et al. 2012; Saraf-Bank et al. Citation2016; Abete, Parra, and Martinez Citation2009). Grain legumes are not only low in sodium but also represent excellent sources of other minerals, such as potassium, which is believed to help reduce blood pressure (Saraf-Bank et al. Citation2016). In fact, a study comparing four hypocaloric dietary obesity treatments (Abete, Parra, and Martinez Citation2009), found that the diet that included pulses was the only one that produced a significant reduction in this cardiovascular risk factor, although all diets tended toward a decrease in blood pressure. It is proposed that along with low sodium content, the high potassium intake conveyed by pulse consumption may contribute to sodium excretion, suppressing the renin–angiotensin system and reducing vasoconstriction (Saraf-Bank et al. Citation2016).
Oxidative stress and inflammatory status
Besides their attributed roles in reducing hypertension risk, the benefits of pulses might also be explained, at least to some extent, by oxidative stress and inflammatory status improvements. It was observed that the inclusion of non-soybean legumes 4 days/week in the nutritional treatment of obesity by a moderate calorie-restricted diet, had the ability to mitigate oxidative stress associated with lipids (Crujeiras et al. Citation2007). Results suggest that weight loss coupled with declines in circulating cholesterol could be linked to the reduction of lipid peroxidation biomarkers, such as oxidized-LDL and MDA (Crujeiras et al. Citation2007). Indeed, the total antioxidant capacity of the pulse diet seemed to be associated with lower urinary excretion of 8-iso-F2α, a widely-accepted indicator of overall lipid peroxidation, showing the potential health benefits of such dietary obesity treatment, beyond weight loss alone (Crujeiras et al. Citation2007). Similarly, the consumption of pulses (4 servings/week) within a hypocaloric diet, resulted in a specific reduction in pro-inflammatory markers, such as CRP and C3, among other clinically relevant improvements, in overweight/obese subjects, in some cases independently from weight loss (Hermsdorff et al. Citation2011). Identical results regarding CRP were obtained by another publication (Saraf-Bank et al. Citation2015), studying first-degree relatives of patients with diabetes, following a 6-week legume-enriched diet.
Apart from weight loss and hypocholesterolemic effects, four more hypotheses have been advanced to explain the inflammation-related outcomes (Hosseinpour-Niazi, Mirmiran, Fallah-Ghohroudi, et al. Citation2015): (1) higher fiber intake through pulse consumption could improve overall glucose homeostasis and subsequently lessen hyperglycemia, hyperinsulinemia and insulin resistance events, which in turn may, for example through the presence of advanced glycation end-products, elevate acute-phase markers of subclinical inflammation, like CRP; (2) bioactive compounds that can be present in legume grains, such as phenolic acids, flavonoids, and anthocyanins, have been found to inhibit Necrose Factor-kB signaling, as well as, cellular enzyme activity, such as phospholipase A2, cyclo-oxygenase and lipoxygenase, impairing the production of pro-inflammatory metabolites, like arachidonic acid, prostaglandins and leukotrienes; (3) pulse intake may promote higher magnesium uptake which has been suggested to participate in the down-regulation of genes related to inflammatory pathways, including C1q and Tumor Necrose Factor-related protein 9 and pro-platelet basic protein; 4) substitution of animal protein with plant protein could encourage lower inflammation grades. In this context, Hosseinpour-Niazi et al. reported that replacing two servings of red meat with non-soya legumes within an isoenergetic therapeutic diet, in overweight diabetic patients, for a period of 3 d/week, reduced plasma concentrations of CRP, IL-6, and TNF-α (Hosseinpour-Niazi, Mirmiran, Fallah-Ghohroudi, et al. Citation2015) and improved glycaemic control and lipid profile (Hosseinpour-Niazi, Mirmiran, Hedayati, et al. Citation2015), both independent of weight modification. Although these results are in line with previous works, the authors sustain further investigation is needed regarding the mechanisms behind the effects seen on inflammatory biomarkers synthesis.
Methodologic concerns
The authors suggest numerous explanations for the observed results that can basically be linked to the nutrient content of pulses. Still, concerns arise in relation to the ability to isolate the effect of pulse consumption from possible confounding factors (e.g. overall dietary intake, physical activity or weight status), particularly amongst trials that are performed within more free-living conditions (Pittaway, Robertson, and Ball Citation2008; Winham, Hutchins, and Johnston Citation2007; Hartman et al. Citation2010; Zhang et al. Citation2010; Hermsdorff et al. Citation2011; Jenkins et al. 2012; Abeysekara et al. Citation2012; Alizadeh, Gharaaghaji, and Gargari Citation2014; Hosseinpour-Niazi, Mirmiran, Fallah-Ghohroudi, et al. Citation2015; Saraf-Bank et al. Citation2015; Safaeiyan et al. Citation2015; Hosseinpour-Niazi, Mirmiran, Hedayati, et al. Citation2015; Saraf-Bank et al. Citation2016). However, less-controlled approaches may strengthen the external validity of results, since they would be more similar to everyday living conditions (Abeysekara et al. Citation2012). Nevertheless, the positive outcomes of pulse intake found within more controlled dietary settings were coupled with the implementation of moderate calorie-restricted diets where weight loss, among other health improvements, happen to be manifest consequences (Venn et al. Citation2010; Crujeiras et al. Citation2007; Abete, Parra, and Martinez Citation2009). Still, metabolic improvements were found independently from bodyweight variances (Hermsdorff et al. Citation2011; Saraf-Bank et al. Citation2015; Zhang et al. Citation2010) thus there may be growing data supporting pulse intake benefits apart from solely weight loss-related outcomes.
Moreover, the use of positive controls may minimize the opportunity to see treatment differences (Jenkins et al. 2012). Also, the fact that most studies (n = 17; 85.0%) were performed on health-impaired subjects may have overestimated the positive results of pulse intake, as well as, minimized the possibility to extrapolate the same expected benefits to the general population. Nevertheless, these results may help validate the inclusion of pulses amongst therapeutic and health-promoting diets.
It would be very useful to note if the legume-rich diets have a more pronounced impact in healthy subjects or if, in opposite, the impact is stronger on diabetic and/or obese patients. However, considering the heterogeneity of study designs in what concerns frequency and dosage of pulses consumed, the duration of intervention and the outcomes measured it is not possible to state clearly these impacts. Finally, since most trial diets included a mixture of pulses, it is not clear if the observed benefits resulted from the cumulative effect of different types of pulses and/or from the effect of a specific variety.
As far as intake quantity is concerned, here we found that it is difficult to estimate the actual amount of pulses that were consumed, since sometimes the exact value in food weight was not referred. Nevertheless, in the present publication, an effort was made to estimate pulse intake despite all bias. Therefore, when the study indicated the quantity of pulses as cups (Winham, Hutchins, and Johnston Citation2007; Alizadeh, Gharaaghaji, and Gargari Citation2014; Tonstad, Malik, and Haddad Citation2014; Hosseinpour-Niazi, Mirmiran, Hedayati, et al. Citation2015; Hosseinpour-Niazi, Mirmiran, Fallah-Ghohroudi, et al. Citation2015; Safaeiyan et al. Citation2015) or servings (Crujeiras et al. Citation2007; Abete, Parra, and Martinez Citation2009; Trinidad et al. Citation2010), the amount was calculated assuming that ≈190 g equals 1 cup or 2 servings of cooked pulses (Jenkins et al. 2012). In turn, when the amount was referred as “dry weight”, the “wet weight” was calculated assuming pulses triple their weight once cooked, as suggested by the Portuguese Food Guide (25 g dry weight = 80 g wet weight) (Rodrigues et al. Citation2006). Consequently, it was visible that positive results were obtained based on the average daily intakes of 150 g (54–360 g) or ≈ 1½ servings of cooked pulses per day.
Doubts arise as well regarding the analytical methods utilized in the reviewed publications. Most studies aimed to access changes in selected metabolic biomarkers, performing essentially targeted analyses. In this sense, metabolic profiling could emerge as a powerful tool to help gain mechanistic insight into nutrition research (Brignardello, Holmes, and Garcia-Perez Citation2017; Sébédio Citation2017) and in particular, regarding pulse consumption. In fact, by exploiting thousands of measured metabolites in easily accessed biofluid samples (e.g. blood, urine, and feces), reflecting together physiological status, food intake, gut microbiota metabolism and environmental exposure (Brignardello, Holmes, and Garcia-Perez Citation2017; Sébédio Citation2017), metabolomics approaches, such as Nuclear Magnetic Resonance (NMR) spectroscopy, may successfully demonstrate variable metabolites profiles in response to different dietary interventions, including plant-based versus omnivorous diets (Wu et al. Citation2016; Bertram et al. Citation2007; Jakobsen et al. Citation2017; Hecke et al. 2016; Zheng et al. Citation2015). Another significant advantage of the use of metabolomics methods is the capacity to perform both targeted and untargeted biochemical assessments, which allows researchers to gain a broader view of metabolic responses to food intake, taking into consideration eventual interpersonal variability as well (Brignardello, Holmes, and Garcia-Perez Citation2017; Sébédio Citation2017). Moreover, these extensive analytic approaches would not only help overcome one crucial limitation of most food trials, which is the self-report nature of food intake data, but also, optimize diet compliance assessment (Madrid-Gambin et al. Citation2017; Guasch-Ferré, Bhupathiraju, and Hu Citation2018; Hanhineva Citation2015; Perera et al. Citation2015). Notwithstanding, to our knowledge there are few publications to date that have focused specifically on the assessment of the metabolic impact of pulse consumption through a metabolomic perspective, leaving room for more extensive research and further intervention studies contemplating such analytical tools.
It is evident that there is growing, yet insufficient, scientific data supporting the health benefits of pulse intake, especially regarding the biochemical mechanisms underlying such effects. Regarding the publication time span, it is clear that the number of papers has increased between 2005 and 2015, in comparison to previous time frames. We believe that these lines of work and respective results, amongst others, have added strength to the scientific data supporting the declaration of 2016 as the International Year of Pulses (United Nations Citation2013; Havemeier, Erickson, and Slavin Citation2017). Curiously, the studies selected were developed in a small number of countries, with 6 studied (30.0%) conducted in Iran. Pulses are highly consumed in this country, as grain legumes are part of Iranians traditional diet (Saraf-Bank et al. Citation2016) and this can justify this finding partially, however, this also enforces the need for more research in this field. However, interventions also took place in countries where pulse consumption is not so widespread (e.g. USA, Canada, etc.), perhaps not only as a result of the increasingly value attributed to these foods over the years, but also as an attempt to promote the adoption of healthier and more sustainable food patterns, especially amongst more western diet-based nations (Mcdermott and Wyatt Citation2017; Foyer et al. Citation2016; Calles Citation2016).
Limitations
The use of only one database could be a limitation of this study and the search filters may have also influenced the retrieved results. A meaningful amount of studies (n = 65) focusing on pulse-based food products (e.g. pulse-based breads and biscuits; flakes; flours) or extracts (e.g. protein hydrolysates; fiber extracts) were excluded, which did not correspond to the purpose of this review, but still considered grain legumes as the main study subject. Nevertheless, we intended that our findings would be consistent with global pulse-promotion campaigns (FAO Citation2016) and dietary recommendations (Marinangeli et al. Citation2017), where whole pulse consumption, in their natural form, is highly advocated as part of healthy and sustainable dietary patterns.
Conclusions
There is growing, yet insufficient, scientific data supporting the health benefits of whole pulses. The majority of papers published in the last two decades report indirect positive outcomes on cardiovascular risk factors, such as, blood lipid profile, glycaemic control, inflammatory status, oxidative stress, as well as, gut microbiota composition and activity. However, concerns regarding the lack of control over food intake, short trial duration and low compliance rates, are evident. Hence it remains unclear whether pulse consumption alone improves metabolic health, and it is still not evident in which population or at what amounts a maximum beneficial effect may be attained. Notwithstanding, positive results were observed with intakes of ≈ 1½ servings (150 g) of cooked pulses per day. As so, data supports the continued presence of such foods in traditional pulse-eating communities, together with their reintroduction into more western diet-based countries. Also, pulses should feature within most dietary treatments, since they seem to potentiate the results of traditional nutritional approaches applied to the management of world-wide prevalent health conditions, such as obesity, diabetes or cardiovascular diseases.
Pulse nutrient-dense profile, including high-fiber content, is believed to be the key factor of the observed health promoting properties. Thus, longer-term randomized controlled trials that are specifically targeted to pulses are necessary to clarify their role in health promotion and disease prevention. In this context, we strongly advocate that more advanced cutting-edge analytical techniques could be explored, for instance, the use of metabolomics tools, more precisely NMR spectroscopy, for its undeniable rising potential within nutrition sciences research.
Acknowledgments
This work was supported by National Funds from FCT through projects UID/Multi/50016/2019 and CICECO-Aveiro Institute of Materials, FCT Ref. UID/CTM/50011/2019 and POCI-01-0145-FEDER-007679, financed by national funds through the FCT/MCTES. Transition paths to sustainable legume-based systems in Europe (TRUE), has received funding from the European Union’s Horizon 2020 research and innovation program under grant agreement No. 727973. H. Ferreira would like to acknowledge FCT for doctoral grant ref. SFRH/BDE/132240/2017.
Disclosure statement
The authors declare no conflicts of interest.
References
- Abete, I., D. Parra, and J. A. Martinez. 2009. Legume-, fish-, or high-protein-based hypocaloric diets: Effects on weight loss and mitochondrial oxidation in obese men. Journal of Medicinal Food 12 (1):100–8. https://www.liebertpub.com/doi/10.1089/jmf.2007.0700.
- Abeysekara, S., P. D. Chilibeck, H. Vatanparast, and G. A. Zello. 2012. A pulse-based diet is effective for reducing total and LDL-cholesterol in older adults. British Journal of Nutrition 108 (S1):S103–S10. doi: 10.1017/S0007114512000748.
- Alizadeh, M., R. Gharaaghaji, and B. P. Gargari. 2014. The effects of legumes on metabolic features, insulin resistance and hepatic function tests in women with central obesity: A randomized controlled trial. International Journal of Preventive Medicine 5 (6):710–20. https://www.ncbi.nlm.nih.gov/pubmed/25013690.
- Arnoldi, A., C. Zanoni, C. Lammi, and G. Boschin. 2015. The role of grain legumes in the prevention of hypercholesterolemia and hypertension. Critical Reviews in Plant Sciences 34 (1-3):144–68. (March):. doi: 10.1080/07352689.2014.897908.
- Bach-Faig, A., E. M. Berry, D. Lairon, J. Reguant, A. Trichopoulou, S. Dernini, F. X. Medina, M. Battino, R. Belahsen, G. Miranda, et al. 2011. Mediterranean diet pyramid today. Science and cultural updates. Public Health Nutrition 14 (12A):2274–84. doi: 10.1017/S1368980011002515.
- Bertram, H. C., C. Hoppe, B. O. Petersen, J. Duus, C. Mølgaard, and K. F. Michaelsen. 2007. An NMR-based metabonomic investigation on effects of milk and meat protein diets given to 8-year-old boys. British Journal of Nutrition 97 (4):758–63. doi: 10.1017/S0007114507450322.
- Boye, J., F. Zare, and A. Pletch. 2010. Pulse proteins : Processing, characterization, functional properties and applications in food and feed. Food Research International 43 (2):414–31. doi: 10.1016/j.foodres.2009.09.003.
- Brignardello, J., E. Holmes, and I. Garcia-Perez. 2017. Metabolic phenotyping of diet and dietary intake. In Advances in food and nutrition research. 1st ed. Vol. 81. Elsevier Inc. 10.1016/bs.afnr.2016.12.002.
- Calles, T. 2016. The international year of pulses: What are they and why are they important? Agriculture for Development - Tropical Agriculture Association, 1–3. http://www.fao.org/3/a-bl797e.pdf
- Crujeiras, A. B., D. Parra, I. Abete, and J. A. Martinez. 2007. A hypocaloric diet enriched in legumes specifically mitigates lipid peroxidation in obese subjects. Free Radical Research 41 (4):498–506. doi: 10.1080/10715760601131935.
- Duane, W. C. 1997. Effects of legume consumption on serum cholesterol, biliary lipids, and sterol metabolism in humans. Journal of Lipid Research 38 (6):1120–8. https://www.ncbi.nlm.nih.gov/pubmed/9215540.
- European Commission. 2018. Report from the commission to the council and the European Parliament on the Development of Plant Proteins in the European Union, Brussels.
- FAO. 1994. Definition and classification of commodities: Pulses and derived products. http://www.fao.org/es/faodef/fdef04e.htm#4.01.
- FAO. 2016. Let the countdown to the international year of pulses begin! http://www.fao.org/zhc/detail-events/en/c/358100/.
- Fernando, W., J. E. Hill, G. A. Zello, R. T. Tyler, W. J. Dahl, and A. G. Van Kessel. 2010. Diets supplemented with chickpea or its main oligosaccharide component raffinose modify faecal microbial composition in healthy adults. Beneficial Microbes 1 (2):197–207. doi: 10.3920/BM2009.0027.
- Foyer, C. H., H.-M. Lam, H. T. Nguyen, K. H. M. Siddique, R. K. Varshney, T. D. Colmer, W. Cowling, H. Bramley, T. A. Mori, J. M. Hodgson, et al. 2016. Neglecting legumes has compromised human health and sustainable food production. Nature Plants 2 (8):1–10. doi: 10.1038/nplants.2016.112.
- Grela, E. R., W. Samolińska, B. Kiczorowska, R. Klebaniuk, and P. Kiczorowski. 2017. Content of minerals and fatty acids and their correlation with phytochemical compounds and antioxidant activity of leguminous seeds. Biological Trace Element Research 180 (2):338–48. doi: 10.1007/s12011-017-1005-3.
- Guasch-Ferré, M., S. N. Bhupathiraju, and F. B. Hu. 2018. Use of metabolomics in improving assessment of dietary intake. Clinical Chemistry 64 (1):82–98. doi: 10.1373/clinchem.2017.272344.
- Hall, C., C. Hillen, and J. G. Robinson. 2017. Composition, nutritional value, and health benefits of pulses. Cereal Chemistry Journal 94 (1):11–31. doi: 10.1094/CCHEM-03-16-0069-FI.
- Hanhineva, K. 2015. Application of metabolomics to assess effects of controlled dietary interventions. Current Nutrition Reports 4 (4):365–76. doi: 10.1007/s13668-015-0148-0.
- Harsha, D. W., P. H. Lin, E. Obarzanek, N. M. Karanja, T. J. Moore, and B. Caballero. 1999. Dietary approaches to stop hypertension: A summary of study results. DASH Collaborative Research Group. Journal of the American Dietetic Association 99 (8):S35–S39.(99)00414-9. doi: 10.1016/S0002-8223(99)00414-9.
- Hartman, T. J., P. S. Albert, Z. Zhang, D. Bagshaw, P. M. Kris-Etherton, J. Ulbrecht, C. K. Miller, G. Bobe, N. H. Colburn, and E. Lanza. 2010. Consumption of a legume-enriched, low-glycemic index diet is associated with biomarkers of insulin resistance and inflammation among men at risk for colorectal cancer. The Journal of Nutrition 140 (1):60–7. doi: 10.3945/jn.109.114249.
- Havemeier, S., J. Erickson, and J. Slavin. 2017. Dietary guidance for pulses: the challenge and opportunity to be part of both the vegetable and protein food groups. Annals of the New York Academy of Sciences 1392 (1):58–66. doi: 10.1111/nyas.13308.
- Hecke, T. V., L. M. A., Jakobsen, E. Vossen, F. Guéraud, F. De Vos, F. Pierre, H. C. S. Bertra, and and S. De Smet. 2016. Short-term beef consumption promotes systemic oxidative stress, TMAO formation and inflammation in rats, and dietary fat content modulates these effects. 10.1039/C6FO00462H.
- Hermsdorff, H. H., M. A. Zulet, I. Abete, and J. A. Martinez. 2011. A legume-based hypocaloric diet reduces proinflammatory status and improves metabolic features in overweight/obese subjects. European Journal of Nutrition 50 (1):61–9. doi: 10.1007/s00394-010-0115-x.
- Hosseinpour-Niazi, S., P. Mirmiran, A. Fallah-Ghohroudi, and F. Azizi. 2015. Non-Soya Legume-Based Therapeutic Lifestyle Change Diet Reduces Inflammatory Status in Diabetic Patients: A Randomised Cross-over Clinical Trial. British Journal of Nutrition 114 (2):213–9. doi: 10.1017/S0007114515001725.
- Hosseinpour-Niazi, S., P. Mirmiran, M. Hedayati, and F. Azizi. 2015. Substitution of red meat with legumes in the therapeutic lifestyle change diet based on dietary advice improves cardiometabolic risk factors in overweight type 2 diabetes patients: a cross-over randomized clinical trial. European Journal of Clinical Nutrition 69 (5):592–7. doi: 10.1038/ejcn.2014.228.
- Jakobsen, L. M. A., C. C. Yde, T. Van Hecke, R. Jessen, J. F. Young, S. De Smet, and H. C. Bertram. 2017. Impact of red meat consumption on the metabolome of rats. Molecular Nutrition & Food Research 61 (3):1–10. doi: 10.1002/mnfr.201600387.
- Jenkins, D. J. A., C. W. C. Kendall, L. S. A. Augustin, S. Mitchell, S. Sahye-Pudaruth, S. Blanco Mejia, L. Chiavaroli, A. Mirrahimi, C. Ireland, B. Bashyam, et al. 2012. Effect of legumes as part of a low glycemic index diet on glycemic control and cardiovascular risk factors in type 2 diabetes mellitus: A randomized controlled trial. Archives of Internal Medicine 172 (21):1653–60. doi: 10.1001/2013.jamainternmed.70.
- Kim, S. J., R. J. de Souza, V. L. Choo, V. Ha, A. I. Cozma, L. Chiavaroli, A. Mirrahimi, S. Blanco Mejia, M. Di Buono, A. M. Bernstein, et al. 2016. Effects of dietary pulse consumption on body weight : A systematic review and meta-analysis of randomized controlled trials. The American Journal of Clinical Nutrition 103 (5):1213–23. 10.3945/ajcn.115.124677.1.
- Li, S. S., C. W.C. Kendall, R. J. de Souza, V. H. Jayalath, A. I. Cozma, V. Ha, A. Mirrahimi, L. Chiavaroli, L. S.A. Augustin, S. Blanco Mejia, et al. 2014. Dietary pulses, satiety and food intake: A systematic review and meta-analysis of acute feeding trials. Obesity 22 (8):1773–80. doi: 10.1002/oby.20782.
- Madrid-Gambin, F., R. Llorach, R. Vázquez-Fresno, M. Urpi-Sarda, E. Almanza-Aguilera, M. Garcia-Aloy, R. Estruch, D. Corella, and C. Andres-Lacueva. 2017. Urinary1H nuclear magnetic resonance metabolomic fingerprinting reveals biomarkers of pulse consumption related to energy-metabolism modulation in a subcohort from the PREDIMED study. Journal of Proteome Research 16 (4):1483–91. doi: 10.1021/acs.jproteome.6b00860.
- Marinangeli, C. P. F., J. Curran, S. I. Barr, J. Slavin, S. Puri, S. Swaminathan, L. Tapsell, and C. A. Patterson. 2017. Enhancing nutrition with pulses : Defining a recommended serving size for adults. Nutrition Review 75 (12):990–1006. doi: 10.1093/nutrit/nux058.
- Mcdermott, J., and A. J. Wyatt. 2017. The role of pulses in sustainable and healthy food systems. Annals of the New York Academy of Sciences 1392 (1):30–42. doi: 10.1111/nyas.13319.
- Messina, V. 2014. Nutritional and health benefits of dried beans. The American Journal of Clinical Nutrition 100 (suppl_1):437S–42S. doi: 10.3945/ajcn.113.071472.
- Moher, D., A. Liberati, J. Tetzlaff, D. G. Altman, and P. Grp. 2009. Preferred reporting items for systematic reviews and meta-analyses: The PRISMA statement (Reprinted from Annals of Internal Medicine). Physical Therapy 89 (9):873–80. doi: 10.1093/ptj/89.9.873.
- Mudryj, A. N., N. Yu, and H. M. Aukema. 2014. Nutritional and health benefits of pulses. Applied Physiology, Nutrition, and Metabolism 39 (11):1197–204. doi: 10.1139/apnm-2013-0557.
- Perera, T., M. R. Young, Z. Zhang, G. Murphy, N. H. Colburn, E. Lanza, T. J. Hartman, A. J. Cross, and G. Bobe. 2015. Identification and monitoring of metabolite markers of dry bean consumption in parallel human and mouse studies. Molecular Nutrition & Food Research 59 (4):795–806. doi: 10.1002/mnfr.201400847.
- Pittaway, J. K., I. K. Robertson, and M. J. Ball. 2008. Chickpeas may influence fatty acid and fiber intake in an ad libitum diet, leading to small improvements in serum lipid profile and glycemic control. Journal of the American Dietetic Association 108 (6):1009–13. doi: 10.1016/j.jada.2008.03.009.
- Rebello, C. J., F. L. Greenway, and J. W. Finley. 2014. A review of the nutritional value of legumes and their effects on obesity and its related co-morbidities. Obesity Reviews 15 (5):392–407. doi: 10.1111/obr.12144.
- Rizkalla, S. W., F. Bellisle, and G. Slama. 2002. Health benefits of low glycaemic index foods, such as pulses, in diabetic patients and healthy individuals. British Journal of Nutrition 88 (S3):255–S262. doi: 10.1079/BJN2002715.
- Rodrigues, S., B. Franchini, P. Graça, and M. D. V. de Almeida. 2006. A new food guide for the Portuguese population: Development and technical considerations. Journal of Nutrition Education and Behavior 38 (3):189–95. doi: 10.1016/j.jneb.2006.01.011.
- Safaeiyan, A., B. Pourghassem-Gargari, R. Zarrin, J. Fereidooni, and M. Alizadeh. 2015. Randomized controlled trial on the effects of legumes on cardiovascular risk factors in women with abdominal obesity. ARYA Atherosclerosis 11 (2):117–25. https://www.ncbi.nlm.nih.gov/pubmed/26405440.
- Saraf-Bank, S., A. Esmaillzadeh, E. Faghihimani, and L. Azadbakht. 2015. Effect of non-soy legume consumption on inflammation and serum adiponectin levels among first-degree relatives of patients with diabetes: A randomized, crossover study. Nutrition 31 (3):459–65. doi: 10.1016/j.nut.2014.09.015.
- Saraf-Bank, S., A. Esmaillzadeh, E. Faghihimani, and L. Azadbakht. 2016. Effects of legume-enriched diet on cardiometabolic risk factors among individuals at risk for diabetes: A crossover study. Journal of the American College of Nutrition 35 (1):31–40. 10.1080/07315724.2014.931262.
- Sébédio, J. L. 2017. Metabolomics, nutrition, and potential biomarkers of food quality, intake, and health status. Advances in Food and Nutrition Research 82:83–116. doi: 10.1016/bs.afnr.2017.01.001.
- Sievenpiper, J. L., C. W. C. Kendall, A. Esfahani, J. M. W. Wong, A. J. Carleton, H. Y. Jiang, R. P. Bazinet, E. Vidgen, and D. J. A. Jenkins. 2009. Effect of non-oil-seed pulses on glycaemic control: A systematic review and meta-analysis of randomised controlled experimental trials in people with and without diabetes. Diabetologia 52 (8):1479–95. doi: 10.1007/s00125-009-1395-7.
- Singh, B., Singh, J. P. K., and N. Singh. 2016. Bioactive constituents in pulses and their health benefits. Journal of Food Science and Technology. 54 (4):858–70. doi: 10.1007/s13197-016-2391-9.
- Tonstad, S., N. Malik, and E. Haddad. 2014. A high-fibre bean-rich diet versus a low-carbohydrate diet for obesity. Journal of Human Nutrition and Dietetics 27 (Suppl 2):109–16. doi: 10.1111/jhn.12118.
- Tosh, S. M., and S. Yada. 2010. Dietary fibres in pulse seeds and fractions : Characterization, functional attributes, and applications. Food Research International 43 (2):450–60. doi: 10.1016/j.foodres.2009.09.005.
- Trinidad, T. P., A. C Mallillin, A. S. Loyola, R. S. Sagum, and R. R. Encabo. 2010. The potential health benefits of legumes as a good source of dietary fibre. British Journal of Nutrition 103 (4):569–74. doi: 10.1017/S0007114509992157.
- United Nations. 2013. Resolution adopted by the general assembly on 20 December 2013 - 68/231. International Year of Pulses, 2016, 45331, 13–4.
- Venn, B. J., T. Perry, T. J. Green, C. M. Skeaff, W. Aitken, N. J. Moore, J. I. Mann, A. J. Wallace, J. Monro, A. Bradshaw, et al. 2010. The effect of increasing consumption of pulses and wholegrains in obese people: A randomized controlled trial. Journal of the American College of Nutrition 29 (4):365–72. https://www.ncbi.nlm.nih.gov/pubmed/21041811. doi: 10.1080/07315724.2010.10719853.
- Winham, D. M., A. M. Hutchins, and C. S. Johnston. 2007. Pinto bean consumption reduces biomarkers for heart disease risk. Journal of the American College of Nutrition 26 (3):243–9. https://www.ncbi.nlm.nih.gov/pubmed/17634169. doi: 10.1080/07315724.2007.10719607.
- World Cancer Research Fund International. 2018. ‘Recommendations and Public Health and Policy Implications’. https://www.wcrf.org/sites/default/files/Cancer-Prevention-Recommendations-2018.pdf.
- Wu, G. D., C. Compher, E. Z. Chen, S. A. Smith, R. D. Shah, C. Chehoud, L. G. Albenberg, et al. 2016. Comparative metabolomics in vegans and omnivores reveal constraints on diet-dependent gut microbiota metabolite production. Gut 65 (1):63–72. doi: 10.1136/gutjnl-2014-308209.
- Young, R., and L. Pellett. 1994. Plant proteins in relation to human and amino acid nutrition. American Journal of Clinical Nutrition 59:1203–12.
- Zhang, Z., E. Lanza, P. M. Kris-Etherton, N. H. Colburn, D. Bagshaw, M. J. Rovine, J. S. Ulbrecht, G. Bobe, R. S. Chapkin, and T. J. Hartman. 2010. A high legume low glycemic index diet improves serum lipid profiles in men. Lipids 45 (9):765–75. doi: 10.1007/s11745-010-3463-7.
- Zheng, H., Yde, C. C. T. K. Dalsgaard, K. Arnberg, Christian Mølgaard, K. F. Michaelsen, A. Larnkjaer, and H. C. Bertram. 2015. Nuclear magnetic resonance ‑ Based metabolomics reveals that dairy protein fractions affect urinary urea excretion differently in overweight adolescents. European Food Research and Technology 240:489–97. doi: 10.1007/s00217-014-2347-0.