Abstract
Cocoa beans, the seeds of the tree Theobroma cacao L., are the key raw material for chocolate production that implies an extensive post-harvest process. Chocolate properties can vary depending on cocoa origin, composition and manufacturing procedure, which will give unique sensory properties to the final product. On the other hand, the high global consumption of cocoa products, long recognized as a major source of dietary polyphenols with important health benefits, has increased interest in tracking the geographical origin of cocoa and authenticating chocolate to guarantee product quality and reveal possible commercial fraud. However, the sustainable production of high-quality cocoa is still far from reality, and the cocoa sector continues to face many challenges in this field. This review provides an update on the progress toward the authenticity, traceability and sustainability of cocoa products, issues that chocolate producers still need to resolve.
1. Introduction
Cocoa beans, the seeds of the tree Theobroma cacao L., are the basic raw material for chocolate production. Cocoa trees grow in a limited geographical zone within approximately 10° either side of the Equator (in particular Central America, West Indian islands, South America, and Africa) (Caligiani et al. Citation2014). There are three main varieties of cocoa beans, Criollo, Forastero, and Trinitario, each with a distinct chemical composition, as well as textural and organoleptic properties (Żyżelewicz et al. Citation2018). Cocoa and chocolate are among the most consumed luxury foods worldwide (approximately 9 kg/year/person in Western European countries) (Beckett Citation2008). The popularity of chocolate is essentially due to its pleasant sensory properties (Magagna et al. Citation2017) and the positive emotions its consumption engenders (Magagna et al. Citation2017; Konar et al. Citation2016), such as joy, pleasure and a stress reduction (Meier, Noll, and Molokwu Citation2017). However, eating chocolate can also be associated with negative emotions, such as longings and unease (Fletcher et al. Citation2007). On the other hand, the consumption of polyphenol-rich cocoa products is thought to contribute to improving overall health and prevent a wide range of chronic diseases. It is reported to reduce blood pressure, hyperglycemia, insulin resistance, and other symptoms of diabetes and obesity in humans (Almoosawi et al. Citation2010). Furthermore, improvement in vascular and platelet function, increased cerebral blood flow, potential cancer prevention, and anti-inflammatory and antioxidant activity have been demonstrated (Decroix et al. Citation2018).
Chocolate production implies an extensive post-harvest process that includes fermentation, drying, roasting and grinding of cocoa beans, the mixing of ingredients, conching, and tempering (Barišić et al. Citation2019). The key ingredients in chocolate formulation are cocoa liquor (obtained by grinding cocoa beans), cocoa butter (CB, obtained by pressing cocoa liquor), sugar, emulsifiers, aroma, and milk components if needed (Li et al. Citation2014; Afoakwa, Paterson, and Fowler Citation2007). Different contents of cocoa solids, milk fat and CB define the primary chocolate categories known as dark, milk and white (Konar et al. Citation2016). In addition to nutrients (carbohydrates, fat, proteins, peptides and amino acids), cocoa beans also contain bioactive compounds, above all polyphenols, including flavanols (epicatechin, catechin, procyanidins), flavonols (quercetin and its glycosides) and phenolic acids (gallic acid) (Żyżelewicz et al. Citation2018). Cocoa beans also have significant amounts of alkaloids (mainly theobromine and caffeine), methylxanthine peptides and N-phenylpropenoyl-L-amino acids (Fayeulle et al. Citation2018). Constituents of cocoa and chocolate products include volatile compounds derived from aroma precursors generated during bean fermentation and drying. Additionally, cocoa beans are a good source of dietary fiber, such as cellulose, hemicellulose and pectic substances (Lecumberri et al. Citation2007). During chocolate production, numerous chemical reactions (aldol condensation, polymerization, cyclization, and alkalization) take place, which enhance flavor, color, shelf life, bioavailability and nutritional value (Afoakwa, Paterson, and Fowler Citation2007).
The cocoa supply chain is complex, involves numerous stakeholders, and each stage (the production of raw material, processing and distribution of the final product) is separated by long distances (Saltini, Akkerman, and Frosch Citation2013). Many characteristics of chocolate, including health-promoting properties, antioxidants, flavor and economic value, depend on its geographical origin (Bertoldi et al. Citation2016). Consumers, increasingly concerned about food safety, now demand to be informed about all the processes in the food supply chain (Zhang, Mankad, and Ariyawardana Citation2020). The problem of food fraud involves criminal adulteration or misrepresentation of food, food ingredients or packaging, on local, regional and global levels, mainly motivated by economic gain (Kendall et al. Citation2019). A way that food industries and governments can enhance consumer confidence in food safety is to adopt a traceability system able to track food from ‘farm to fork’ (Zhang, Mankad, and Ariyawardana Citation2020). The chocolate industry has used this approach to guarantee the flavor and quality of their products and preserve stakeholder confidence, especially in the context of a growing consumer demand for single origin chocolate and interest in sustainable production (Rottiers et al. Citation2019).
Sustainable cocoa implies the production of high quality cocoa beans in terms of nutritional composition, flavor volatiles, polyphenolic content and fermentative quality (Kongor et al. Citation2016). However, current cocoa farming practices are causing extensive deforestation, with consequent loss of biodiversity (Wessel and Foluke Quist-Wessel Citation2015), and greenhouse emissions from transportation also have negative effects on the environment (Saltini, Akkerman, and Frosch Citation2013; Vogel et al. Citation2020). The economic vulnerability of cocoa-farming households (Vogel et al. Citation2020) also undermines efforts toward achieving sustainable cocoa production, and poor governance in most of the cocoa-producing regions complicates the guarantee of traceability (Saltini, Akkerman, and Frosch Citation2013). There is therefore a strong need to find new approaches that can ensure a viable future for cocoa-producing countries (Vogel et al. Citation2020). The importance of quality control, and assessment of authenticity and traceability has resulted in the development of promising analytical tools, which will be described in depth in this review.
2. Cocoa processing: how does it affect the chemical composition of chocolate?
Cocoa processing has an impact on the bioactive compound composition of cocoa beans (Wollgast and Anklam Citation2000; de Brito et al. Citation2001; Radojc, Markovic, and Jolic Citation2011), potentially yielding hundreds of products through a diversity of reactions (Ryan et al. Citation2016). Cocoa processing procedures induce a wide range of chemical rearrangements, including aldol condensation, polymerization, cyclization, and alkalization, which enhance the flavor, color, shelf life, bioavailability and nutritional value of chocolate (Afoakwa Citation2016). Each of these processes can modify the structure and function of the chocolate components or generate interactions between them and other ingredients.
The first steps in cocoa bean processing usually take place in the country of origin, where traditional local cultivation processes, and specific soil and climatic conditions can have an effect on the chemical composition of cocoa (Kongor et al. Citation2016). As mentioned in the Introduction, the chocolate production process consists of fermentation, drying, roasting, and grinding of cocoa beans, mixing the ingredients (cocoa mass, sugar, CB, emulsifiers, aroma, and milk components if needed), conching, and tempering (Barišić et al. Citation2019). Various other techniques such as pressing, or alkalization are applied to produce the desired final product. The overall processing of cocoa beans is shown in .
Figure 1. Cocoa processing and impact on composition. Adapted from Frauendorfer and Schieberle (Citation2008); Miller et al. (Citation2008); Afoakwa et al. (Citation2009); Kongor et al. (Citation2016), and Barišić et al. (Citation2019).
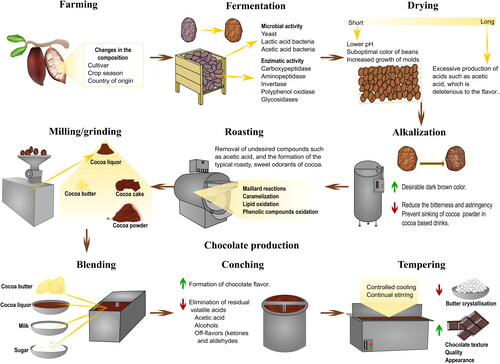
Cocoa beans are typically fermented by environmental microbiota, in a process that lasts from 4 to 7 days and involves temperatures of up to 50 °C and a pH below 4. The combination of acidic conditions and high temperatures leads to major changes in cocoa beans. During this step, endogenous enzymes play a crucial role in the production of flavor precursors and degradation of pigments (Hansen, Olmo, and Burri Citation1998), while the addition of commercial enzymes, such as pectinase, accelerates the fermentation process, produces a high ratio of fermented beans, and improves the testing scores of the final product (Gil et al. Citation2016). Besides flavor precursors such as free amino acids, peptides and reducing sugars (Kongor et al. Citation2016), fermentation generates nonvolatile and volatile flavor compounds such as alcohols, short-chain organic acids and esters (Frauendorfer and Schieberle Citation2008). The potential for microbial synthesis of methylpyrazines (Jinap et al. Citation1998) and the formation of aldehydes (Frauendorfer and Schieberle Citation2008) has also been reported. Fermented cocoa beans are rich in free amino acids, metabolites released by the action of microbial peptidases during fermentation (Ardhana and Fleet Citation2003; Rohsius, Matissek, and Lieberei Citation2006). Moreover, fermentation leads to a significant reduction in the concentration of polyphenols, which undergo oxidation and polymerization to insoluble high molecular weight compounds such as tannins, thereby reducing the bitterness and astringency of the beans (Wollgast and Anklam Citation2000; Barišić et al. Citation2019; Kongor et al. Citation2016).
Drying follows the stage of fermentation and also plays an important role in reducing astringency and bitterness (Jinap, Thien, and Yap Citation1994). Dried cocoa beans are less acidic due to the outward migration of volatile acids as well as biochemical oxidation of acetic acid (Kongor et al. Citation2016). The content of alcohols, esters and pyrazines increases during the sun-drying process, whereas acids, aldehydes and ketones decrease (Rodriguez-Campos et al. Citation2011). Oxidization and polymerization further reduce the polyphenol concentration (Kongor et al. Citation2016).
During roasting, which lasts from 10 to 35 min at temperatures between 120 and 140 °C, all the precursors formed in the previous phases react and generate numerous compounds. In this part of the process, the contents of undesirable components are reduced and the chocolate-specific aroma and flavor are produced (Barišić et al. Citation2019). Flavor precursors, namely free amino acids, short-chain peptides, and reducing sugars, undergo the Maillard reaction and Strecker degradation to produce desirable flavor compounds (Kongor et al. Citation2016). These reactions also result in a significant increase of acids, aldehydes, ketones and methylpyrazines (Pertanian Citation1994). The sourness and bitterness of the cocoa beans are reduced even more by the evaporation of volatile acids (Kongor et al. Citation2016). The high roasting temperatures and cocoa bean dehydration lead to sugar caramelization and thus to the formation of the corresponding aroma, flavor and color of cocoa products. During roasting, high processing temperatures can enhance lipid oxidation and non-enzymatic browning by reducing essential fatty acids (FA) and essential amino acids, thus decreasing the nutritional value of cocoa beans. Vitamins can also be affected and protein digestibility reduced (Djikeng et al. Citation2018). The desired brown pigments of chocolate are mainly created during this process, due to the oxidation and polymerization of polyphenols, protein degradation, the Maillard reaction and dextrinization of starch (Vítová et al. Citation2009).
Some manufacturers include an additional step of alkalization, also known as Dutching, which is applied to the cocoa beans, cocoa liquor or cocoa powder (Miller et al. Citation2008) to obtain the desirable dark brown color, reduce bitterness and astringency, and prevent the sinking of cocoa powder in cocoa-based drinks (Miller et al. Citation2008). When cocoa is alkalized, after heating in a closed mixing vessel, a warm alkali solution is added for a specific reaction time, and excess moisture is removed by heating or drying (Li et al. Citation2014). The degree of alkalization, and consequently the mild taste and darkness of cocoa, depends on the strength of the alkali solution, alkali type, length of the reaction stage, and the process temperature (Kostic Citation1997). Alkalization also affects the content of polyphenols, theobromine, caffeine, amino acids and the volatile compounds, affecting the unique flavor and color of the end products (Li et al. Citation2012).
The polyphenol content and composition of the final chocolate product is also affected by milling, refining and conching, mainly because of heat exposure and the presence of oxygen (Wollgast and Anklam Citation2000). Conching is conducted to achieve a proper viscosity, remove excess moisture, and develop a desirable color (Barišić et al. Citation2019), and it eliminates the residual volatile acids such as acetic acid, alcohols (mainly linalool and 2-phenylethanol), and other off-flavors (e.g., ketones and aldehydes) (Afoakwa et al. Citation2008). In the initial stage of conching, volatile polyphenols are lost due to evaporation, together with water and short-chain FA (SFA) (Barišić et al. Citation2019). Finally, tempering is essential for the fat crystallization behavior of cocoa butter, which influences the quality properties of the final product such as color, hardness, handling, finish and shelf life characteristics (Afoakwa et al. Citation2009).
3. Sensorial perception of cocoa: what do dark chocolate lovers expect?
While the popularity of chocolate is mainly due to its pleasant sensory properties, recent evidence of various health benefits opens up prospects of new markets and the possible use of cocoa in functionalized foods (Magagna et al. Citation2017). Although dark chocolate is still significantly less popular than milk chocolate, accounting for 31% versus 51% of the global market share, it has attracted growing consumer interest on the basis of these positive findings (Afoakwa Citation2016). Dark chocolate differs considerably from milk chocolate in terms of its sensory profile (Kennedy and Heymann Citation2009), partly due to the absence of dairy ingredients and a lower sugar content. The main difference, however, is a higher content of cocoa, whose bitter taste and astringency, caused mainly by flavan-3-ols (procyanidins, epicatechin, catechin), may limit consumer acceptance and preference (Sun-Waterhouse and Wadhwa Citation2013).
The sensory profile (aroma, flavor, mouthfeel and texture) is crucial in obtaining top quality products suited to consumer preferences. The main cocoa flavor (aroma and taste) components are alcohols, ethers, hydrocarbons, furans, thiazoles, pyridines, acids, esters, aldehydes, ketones, imines, amines, oxazoles, pyrazines, and pyrroles (Counet et al. Citation2002). Flavors are developed from complex biochemical and chemical reactions that occur post-harvest and vary according to genotype, geographical origin, environmental conditions, agricultural practices, and technological processing, as mentioned above (Section 2). With a growing demand for sustainably produced high-quality cocoa beans, a greater understanding of the factors contributing to flavor variations would have significant commercial implications.
But which attributes attract chocolate lovers the most? The sensory characteristics of dark chocolate have been studied for many years, and the characteristics of the four sensory modalities (appearance, aroma, texture and flavor), which are decisive in consumer choice of chocolate products, were recently identified (Pelsmaeker et al. Citation2019). In another study, the key sensory descriptors of chocolate provided by two panels from different countries were bitter, sweet, acidic, cocoa, and melting behavior (Thamke, Dürrschmid, and Rohm Citation2009).
Cocoa flavor components, sensory properties, and the processes involved in flavor generation are essential for determining consumer preferences. According to a study published in 2012, the effect of processing conditions on the acceptability of chocolate varies according to the origin of the cocoa beans (Torres-Moreno, Tarrega, Costell, et al. Citation2012). The level of acceptability of dark chocolate for most consumers depended above all on the flavor. Specifically, the differences in preference corresponded to the interaction effect between roasting time and cocoa origin. In the case of samples from Ghana, it was found that a long roasting time reduced chocolate acceptability. However, for another group of consumers, the most acceptable samples of dark chocolate were unrelated to the geographical origin of the cocoa and were associated only with specific combinations of processing factors. In their case, the preferred chocolate was processed by a short roasting and long conching, or a long roasting with a short conching time.
The sensory impact of CB substitutes has also been the subject of study. In 2017, it was demonstrated that chocolate with a palm oil-based CB substitute had significantly different sensory characteristics in terms of taste, hardness and overall acceptability compared to the CB chocolate (Biswas et al. Citation2017). In another study, it was demonstrated that the shape of dark chocolate pieces has an impact on consumer perceptions of texture and flavor and that in-mouth melting is not the sole factor influencing cocoa flavor intensity (Lenfant et al. Citation2013). Artisanal dark chocolate scored higher than industrial dark chocolate for smell, texture, and taste in a blind test (Caponio et al. Citation2020).
The information provided on the label of dark chocolate also affects consumer expectations, acceptance and purchase intention. A study found that after reading the label, but without tasting the chocolate, consumer expectations were mainly affected by the brand, whereas in a blind tasting test, the most important factor was product type and the samples with a high percentage of cocoa were the least preferred. Finally, when the consumers tasted the products after reading the label, the most acceptable dark chocolate depended on both brand and product type. Consumers had higher expectations of premium than store brand chocolate, but both were judged equally acceptable when tasted. Labels claiming a high percentage of cocoa and single cocoa origin did not raise higher expectations than standard dark chocolate (Torres-Moreno, Tarrega, Torrescasana, et al. Citation2012).
4. Authenticity and traceability of cocoa and chocolate products
The aim of food authentication testing is to confirm the validity of information on the product label regarding origin, production method and processing technologies. It protects consumers by guaranteeing food safety and quality, but also benefits industries interested in protecting their brands. The issue of origin, which is covered by national and international legislation, is a key factor in food authentication (Aung and Chang Citation2014). In Europe, there is a growing trend among consumers to associate a particular product origin with quality. Therefore, accurate, standardized food authentication techniques are essential in the food industry (Posudin, Peiris, and Kays Citation2015; Georgiou and Danezis Citation2015).
The labeling and composition of chocolate products are controlled by the Cocoa and Chocolate Products Regulations 2003 in accordance with the EC Directive 2000/36/EC2. However, authentication testing of chocolate is hampered by a complex matrix and the long production chain. During the radical transformation of the raw material into the finished product, there are many opportunities for adulteration, one of the most common being mixing under-fermented with fermented cocoa beans (Caligiani et al. Citation2014). The quality of beans strongly depends on the fermentation process, which, as mentioned above, reduces astringency and bitterness. Chocolate may also contain allergy-producing adulterants such as peanut and egg (Khuda et al. Citation2015). During tempering, which is performed to improve chocolate texture, quality, and appearance, the crystallization of end products is affected by the chocolate fat content (Afoakwa et al. Citation2009), and can potentially mask allergen detection chocolate (Khuda et al. Citation2015). The main fat in cocoa and chocolate is CB, but cheaper fats can be used to reduce production costs, negatively affecting the quality of chocolate (Naik and Kumar Citation2014).
Chocolate adulteration, the practice of achieving desired properties by introducing foreign ingredients, which may or may not replace natural or synthetic ingredients, represents a serious health threat for consumers (Lakshmi Citation2012). With increasing adulteration trends, faster and more reliable analytical methods are required to address authentication challenges, ensure product quality and avoid economic fraud.
An overview of the methods presented throughout this review to evaluate cocoa and chocolate authenticity and traceability is shown in .
Table 1. Overview of presented methods to evaluate cocoa and chocolate authenticity and traceability.
4.1. Variety and geographic traceability of cocoa beans
Cocoa beans are produced in a limited geographical region within approximately 10° to the north and south of the Equator in Africa (75%), Central and South America (18%), and Asia and Oceania (7%) (Caligiani et al. Citation2014; Afoakwa Citation2016). The most common varieties are Forastero, Criollo and Trinitario (Caligiani et al. Citation2014; Afoakwa Citation2016). The highest quality cocoa beans are the Criollo variety and the native Forastero variety known as National, which grows mainly in Venezuela, Ecuador and Mexico. The price generally depends on the variety, seasonal weather conditions, the total crop production and the geographical origin, among other factors (Żyżelewicz et al. Citation2018; Afoakwa Citation2016).
The composition profile of the fermented cocoa beans, one of the most important factors influencing the flavor and price of cocoa products, depends on the variety but above all on geographical origin (Roelofsen Citation1958). As the higher quality cocoa beans are more expensive, the ability to identify their real geographical provenance is desirable for consumers, producers, retailers and administrative authorities in order to assess product authenticity and disclose possible commercial frauds.
Increasing effort has been dedicated to developing robust scientific methods for the traceability of cocoa beans, including high performance liquid chromatography (HPLC) (D’Souza et al. Citation2017), Raman spectroscopy (Vargas Jentzsch et al. Citation2016), nuclear magnetic resonance (NMR) (Caligiani et al. Citation2014; Marseglia et al. Citation2016), and isotope ratio monitoring using mass spectrometry coupled to an elemental analyzer (IRMS-EA) (Diomande et al. Citation2015). These methodologies will be briefly discussed below. Some of these strategies have shown that, after exhaustive statistical evaluation of the data obtained, it is possible to classify the samples according to the three main varieties of cocoa (Forastero, Criollo and Trinitario) and to distinguish between various growing regions, at the level of continent, country and even town. Another approach to the authentication of cocoa and chocolate is through the new DNA barcoding technique (Guiltinan et al. Citation2008), which is described in Section 4.1.5.
4.1.1. Raman and Fourier transform infrared spectroscopy
The expensive National cocoa variety, also known as Fino de Aroma or Arriba, is used to produce the highest quality chocolate. Although Ecuador is the most important producer of this variety (Carrera Almeida Citation2014), the CCN-51 variety is also extensively cultivated in this country due to its resistance to diseases and high crop production (Herrmann et al. Citation2014). Due to the differences in productivity and price, sometimes both cocoa beans are fraudulently mixed to increase profits from the cocoa cultivar. The National and the CCN-51 cocoa beans can be distinguished, but this requires the expertise of a well-trained technician, who evaluates the bean shape, color, odor and taste. Raman spectroscopy, which is a powerful analytical technique with numerous applications in chemistry, physics and biology (Petry, Schmitt, and Popp Citation2003; Craig, Franca, and Irudayaraj Citation2013), was assessed for its ability to distinguish between Ecuadorian cocoa bean varieties in 2016 (Vargas Jentzsch et al. Citation2016). Spectra of 20 shell samples from fermented cocoa beans of the National and CCN-51 varieties were obtained. The most important Raman bands were assigned to the principal shell components and the spectroscopic data were analyzed with chemometric methods to assess the cocoa bean variety. The variety-differentiation results obtained from the average Raman spectra, using a support vector machine (SVM), had a total accuracy of 91.8%.
Whereas Raman spectroscopy is a dispersion process, near infrared (NIR) spectroscopy is based on absorption and uses harmonics and combinations of bands of molecular vibrational spectra. Fourier transform (FT)-NIR spectroscopy has also been used to perform qualitative and quantitative analyses in agricultural and food-processing industries (Chen, Zhao, and Lin Citation2009; Ribeiro, Ferreira, and Salva Citation2011). NIR spectroscopy was initially used in cocoa beans to quantify fat, nitrogen and moisture (Veselá et al. Citation2007) and predict the content of proanthocyanidins (Whitacre et al. Citation2003). It was also applied to determine the content of fats, proteins and carbohydrates in cocoa (Kaffka et al. Citation1982). In 2013, in an authentication study, FT-NIR spectroscopy was used to analyze a total of 194 cocoa bean samples from seven Ghanaian cocoa growing regions (Ashanti, Brong Ahafo, Central, Eastern, Volta, Western north and Western south) (Teye et al. Citation2013). Four multivariate classification methods were compared and cross-validated: linear discriminant analysis (LDA), K-nearest neighbors (KNN), back propagation artificial neural network (BPANN) and SVM. Due to a greater capacity for self-learning and self-adjustment, the two non-linear models (BPANN and SVM) were found to be superior to the two linear methods (LDA and KNN). Furthermore, the SVM model was superior to all the mathematical methods with a geographical discrimination rate of 100% in both the training and prediction sets after pre-processing with Mean centering.
4.1.2. Mineral element and isotope profiles to determine geographical provenance
The study of stable isotope distribution is an efficient approach extensively used in agrarian food traceability, as isotopes reflect both meteorological events (precipitation, condensation and evaporation) and geographical location (altitude, latitude and continent). The ratio of stable isotopes of hydrogen (δ2H) and oxygen (δ18O) in plants is influenced by both latitude and altitude of the growth site. The ratio of nitrogen isotopes (δ15N) is affected by agricultural practices (Amundson et al. Citation2003), while the ratio of carbon isotopes (δ13C) is highly dependent on the environment. Isotope content is measured by IRMS. In 2010, the specific influence of soil geology and contamination sources in the growing and manufacturing areas of cocoa was studied (Manton Citation2010). The author proposed the isotopic analysis of lead (Pb), neodymium (Nd), samarium (Sm), and strontium (Sr) to identify the geographical region of cocoa beans by soil protolith ages.
Multi-element stable isotope ratios were also assessed by Diomande et al. (Citation2015) to identify cocoa agricultural regions. Sixty-one samples of cocoa beans collected in 2008–2010 from 24 geographical origins covering four continents were analyzed by IRMS-EA. The isotope ratios and C and N percentage composition were measured in cotyledons and shells, as well as in fermented cocoa bean extracts. Differences between fermented cocoa beans were assessed using principal component analysis (PCA) of parameters measured in whole beans and their various derivatives. The dataset was then handled using partial least squares discriminant analysis (PLS-DA) models, prioritizing the geographical origin to optimize the separation between sample groups (Szymańska et al. Citation2012), as shown in .
Figure 2. Main results in the statistical analyses of nitrogen (δ15N) and carbon (δ13C) isotope signatures in cocoa beans and cocoa-containing products. Adapted from Diomande et al. (Citation2015).
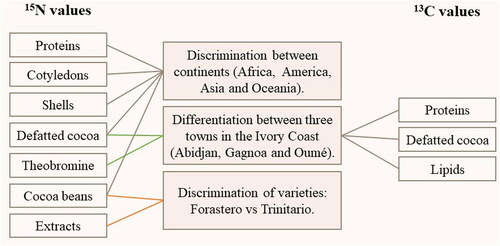
The combination of stable isotope ratios of oxygen (δ18O), hydrogen (δ2H) and sulfur (δ34S) with carbon (δ13C) and nitrogen (δ15N) was tested as a traceability tool on a wide selection of cocoa beans of different geographical origin (fifty-three samples from Africa, Asia, Central and South America) (Perini et al. Citation2016). For statistical evaluation, the samples were grouped into five different macro-areas. The differences between the isotopic ratios of cocoa beans were determined using Tukey’s honestly significant difference test and the Pearson correlation test (Abdi and Williams Citation2010) was applied to verify the correlations between the parameters. The ability of the isotopic profile to distinguish between cocoa beans based on their origin was assessed by canonical discriminant analysis (CDA). To test the predictive power of discrimination and the stability of the model, a cross validation was used, in which 84% of the samples were allocated to the right geographical region. In samples from Papua New Guinea, São Tomé and Peru, classification was 100% correct.
In addition to isotopes, the profiling of trace elements is another very useful technique to trace the origin of agricultural food, as edaphic and environmental factors such as fertilization, soil type, climate and temperature easily change metallic elements. Both inductively coupled plasma MS (ICP-MS) and atomic spectroscopy are employed for the quantitative determination of trace elements. Element profiling has been successfully used to establish the origin of honey, onion, black tea and wine (Coetzee, Van Jaarsveld, and Vanhaecke Citation2014; D’Archivio et al. Citation2014; Madejczyk and Baralkiewicz Citation2008; Pilgrim, Watling, and Grice Citation2010; Šelih, Šala, and Drgan Citation2014). In 2016, Bertoldi et al. (Citation2016) developed a multi-elemental chemometric technique to fingerprint cocoa beans, using ICP-MS to generate an elemental-profile model for their geographic traceability. To find the average mineral content, the concentration of 56 macro-, micro- and trace elements was determined for 61 cocoa bean samples from 5 different macro-areas (West Africa, East Africa, Asia, Central America and South America). Tukey's honestly significant difference test was applied to identify different elemental concentrations in cocoa beans, whereas PCA, and canonical and forward stepwise discriminant analysis were carried out to estimate the power of the method to distinguish geographical provenance. Finally, 29 elements were selected for their optimal ability to reclassify cocoa beans (100% of samples) according to the macro-area of origin, when sufficient samples were available for statistical analysis, confirming the usefulness of mineral profiles for geographic traceability. After removing heavy metals, potentially present because of environmental contamination, the same authors tested the effectiveness of the model by carrying out a leave-one-out cross-validation and 97% of the samples were reclassified to the correct macro-area of origin.
4.1.3. Metabolic profile of cocoa beans to assess the geographical origin
4.1.3.1. Polyphenolic fingerprinting by HPLC
A comprehensive analysis of polyphenols in cocoa beans was carried out in 2017 to catalogue systematic differences related to origin as well as fermentation status (D’Souza et al. Citation2017). In this study, several oligomeric proanthocyanidins and their glycosides, as well as various previously unreported compounds, were identified and quantified using ultra HPLC coupled with ultra-high-resolution time-of-flight MS (UHPLC-qToF-MS). The large sample set employed (86 different bean samples from six countries: Ivory Coast, Tanzania, Malaysia, Indonesia, Ecuador, and Brazil) allowed statistically significant variations in cocoa chemical compounds to be determined. All detected compounds were assigned based on high resolution MS data. Subsequent MS/MS measurements yielded accurate mass information on fragment ions, which led to the identification of 66 compounds by comparison with authentic standards or data in the literature. Multivariate statistical analysis of the full sample set revealed a series of key biomarkers that could distinguish between unfermented and fermented beans. Furthermore, beans of certain origins also showed good separation within the loading plots, which was corroborated by comparing two different origins using PCA. Samples from the Ivory Coast (characterized by a higher level of citric acid and 12-hydroxyjasmonic acid–O-sulfate) and Indonesia (with an increased level of certain proanthocyanidin oligomer polyphenols) were accurately distinguished.
4.1.3.2. Fatty acid profiling of cocoa beans by GC-MS
FA of different cocoa bean samples from Ecuador and Ghana were identified and quantified by gas chromatography–MS (GC-MS) analyses in 2015 (Torres-Moreno et al. Citation2015). The FA profile was studied in depth to assess provenance, using the corresponding fatty acid methyl esters (FAMEs) (AOAC International Citation1990). According to the dataset obtained, the FA profile was similar in all the cocoa beans studied and only a few significant differences were observed: Ecuadorian cocoa beans had a higher content of C16:0 and C16:1 than the samples from Ghana, and a lower content of C14:0, C17:0, C18:0 and C17:1. On the other hand, traces of C12:0 were detected in Ecuadorian beans, but not in samples from Ghana.
4.1.3.3. Composition profile of cocoa beans by 1H NMR and high-resolution magic angle spinning-NMR
The team of Augusta Caligiani has long studied the metabolic profile of cocoa beans by NMR techniques. In 2010, they provided exhaustive qualitative and quantitative data on the chemical composition of cocoa beans by the full assignment of the 1H NMR spectra of hydro-alcoholic extracts (Caligiani et al. Citation2010). In successive studies, the NMR approach was extended to a larger number of samples, including unfermented and under-fermented cocoa beans, applying chemometric techniques to determine the variables that could discriminate among different bean varieties (Caligiani et al. Citation2014). More recently, geographical tracing was attempted by determining a complete metabolite profile (both lipophilic and hydrophilic components) using HRMAS-NMR (Marseglia et al. Citation2016).
In the variety-traceability approach of Caligiani and coworkers, 1H NMR spectra of hydro-alcoholic extracts of 57 well-fermented, under-fermented and unfermented cocoa bean samples were initially compared (Caligiani et al. Citation2014). The data obtained from the integration of the complete 1H NMR spectra were treated with PCA using a matrix of 39 variables, and the principal components were identified with the correlation method, followed by a manual integration of the most important NMR signals. The results confirmed literature reports that cocoa bean fermentation causes a loss of polyphenols (Nazaruddin et al. Citation2006). In the variety assessment, the metabolic profiles were analyzed using only well-fermented cocoa beans. As before, after performing an explorative PCA (a matrix of 39 variables for 31 samples), the principal components were obtained with the correlation method. In the score plot, the bean samples were qualitatively separated into three groups: National, Forastero (plus Trinitario), and Criollo. Notably, high levels of carbohydrates, epicatechin and methylxanthines were found in the National variety, which is related to their short fermentation times, whereas the Criollo variety was characterized by high levels of amino acids (precursors of aroma compounds) and fermentation metabolites such as lactic acid and 2,3-butanediol.
A few years later, the same team tested HRMAS 1H NMR for its ability to determine the geographical origins of 60 samples of Forastero cocoa powder from 23 different cocoa-producing countries (Marseglia et al. Citation2016). Without any chemical, physical or biological manipulation, this technique offers the opportunity to obtain high resolution NMR spectra, and the simultaneous detection of polar and apolar metabolites (Valentini et al. Citation2011). The study compared the spectra of cocoa beans recorded by 1H NMR from hydroalcoholic extracts and by HRMAS 1H NMR directly from cocoa powder. The detection of amino acids, polyalcohols, organic acids, sugars, methylxanthines, and catechins allowed a metabolic fingerprinting of the samples. An advantage of HRMAS is that it can be used to detect lipids, which are absent in the aqueous extract sample for 1H NMR. The combination of the HRMAS 1H NMR and 1H NMR data sets with chemometric techniques successfully discriminated between African and American cocoa samples ().
Figure 3. Potential markers to distinguish between African and American samples. Adapted from Valentini et al. (Citation2011).
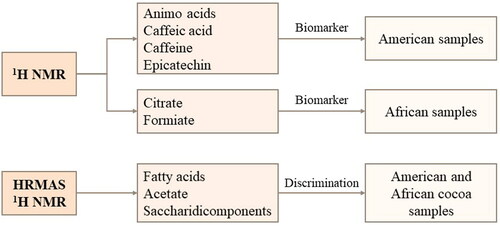
4.1.4. Synergistic effects of fuzing 1H NMR fingerprints with multi-element stable isotope abundance data
The results produced by a single analytical method are often insufficient for an accurate statistical evaluation, whereas the use of multiple methods can provide more information. When large data sets from various analytical instruments are combined, statistical methods such as PCA, CDA or PLS-DA are effective tools for product characterization.
To demonstrate the progress in both precision and accuracy of cocoa authenticity testing, a recent study analyzed 48 samples of cocoa beans from 20 countries in a multi-method approach using 1H NMR fingerprints and abundances of certain stable isotopes (measured by IRMS) and element concentrations (Bindereif et al. Citation2019). Chemometric analysis of the combined data sets of both techniques achieved a better sample separation compared to the classification based on data from each individual method.
Specifically, stable isotope data (δ13C, δ15N, δ18O and δ2H) and element concentrations (%C, %N, %O and %H) were found to be very effective in discriminating between countries, while 1H NMR fingerprints contributed significantly to varietal classification and the separation of regions within individual countries.
4.1.5. Authentication of cocoa products and chocolate by DNA barcoding
The new DNA barcoding technique can be applied to authenticate cocoa and chocolate (Guiltinan et al. Citation2008). Cocoa authentication is particularly challenging in that the DNA profile needs to be established for individual beans rather than leaves or other tissues, as different varieties of cocoa beans of variable quality may be mixed at the fermentation, drying or packaging stages and sold as one lot. The authenticity of a cocoa variety is therefore verified by using the bean coat to establish genetic identity and trace the mother tree. Though still a costly technology, with equipment and reagent requirements that make it logistically demanding and often unpractical for controls in the producing areas and customs, DNA fingerprinting of cocoa beans may be useful for manufacturers of high-quality chocolate made from single-origin cocoa.
4.1.6. Toward a reliable analytical method for geographical tracing of cocoa beans
In response to the growing interest of consumers and producers in food quality and traceability, especially in the case of high value products such as chocolate, larger datasets are needed to build a solid and reliable discriminant model for geographical origin. Differentiation between varieties and geographical provenance of cocoa beans is particularly complex because of the major role the fermentation process plays in their composition.
Among the most useful analytical tracing techniques for foods are those based on the natural abundance of isotope and element variation. However, although the observed differences in isotope values can be mainly explained by altitude and precipitation parameters, the effect of fermentation on the isotope ratios of the analyzed elements still needs to be defined. Nevertheless, the isotopic analysis of nitrogen and carbon from cocoa beans can differentiate between geographical origins, tracing not only the continent of the cocoa cultivar, but also smaller regions (even to the level of towns). It can also discriminate between cocoa varieties.
Alternatively, fingerprinting techniques based on the vibrational mode of molecules offer several benefits, as they are nondestructive, rapid and accurate analytical tools, respectful with the environment, and require little or no sample preparation. In this context, the availability and ease of use of handheld Raman spectrometers opens new perspectives for the assessment of cocoa bean varieties, whereas NIR spectroscopy coupled with an SVM has the potential to discriminate between the geographical origins of cocoa beans at a regional level.
NMR-based food metabolomics analysis has recently attracted scientific interest due to its multiple advantages. This approach is nondestructive, robust, avoids derivation and separation steps, allows the simultaneous detection of all major classes of organic compounds, can identify new compounds, and provide quantitative results. Although the use of HRMAS 1H NMR provides a complete metabolite profile (both lipophilic and hydrophilic components), the sample preparation, specifically the ratio between cocoa powder and deuterated solvents, can cause considerable variation in spectra. This variability leads to unreliable data and excludes the possibility of obtaining quantitative results. Another drawback is that this technique does not discriminate between samples from Asia and Oceania due to their genetic similarity. Crucially, the strong influence of fermentation on the metabolic profile of cocoa increases the complexity of geographic assessment of beans with a short fermentation.
Analysis based on the FA and polyphenolic profiles of cocoa beans is limited in that it can only distinguish between two countries at a time. Although polyphenolic fingerprints using HPLC successfully differentiated between the Ivory Coast and Indonesia, more extensive studies are needed to test this strategy on a wider geographical basis.
4.2. Chocolate authentication through traceability measurement
4.2.1. Chocolate quality based on cocoa polyphenol fingerprinting
Polyphenol fingerprinting, described in 4.1.3.1 to classify origin and fermentation status of cocoa beans, was also used on the chocolate product to classify underlying cocoa beans accordingly. Sensorial quality and phenolic composition of the final product was recently studied with 60 samples of cocoa beans from different origins and harvest years (Fayeulle et al. Citation2019). The cocoa beans were separated into four groups based on the sensory data of the chocolate prepared with the analyzed samples. After averaging each mass spectrum of the cocoa polyphenol extracts, obtained by liquid chromatography - low resolution MS, the provided polyphenolic fingerprints were combined in a comparative matrix and processed with chemometrics. The results allowed the selection of the most significant molecules to discriminate between chocolate sensory groups. Variations in intensity of the average spectra recorded with low-resolution MS were found to be precise and robust, thus providing a fast, efficient and reproducible fingerprint method. To verify the relevance of the 29 selected variables, they were tested on a larger set of cocoa bean samples and the data were treated with PCA. Control samples showed that the results were reproducible and that the selected variables were significant, even when applied to a wide range of cocoa beans from different origins and years of production.
4.2.2. Detection of vegetable fats and lard in chocolate formulation
CB is an expensive raw material and a vital component in the production of chocolate and related confectionery (Chaiseri and Dimick Citation1989). In some countries, chocolate manufacturers choose to mix vegetable fats with CB to reduce production costs. Fractions of palm oil and other vegetable fats of tropical origin (shea, sal, illipe) are used to make CB equivalents, although they can interfere with the normal process of triglyceride crystallization. The current European legislation allows the addition of vegetable fats to chocolate up to a level of 5% of the product weight, if the addition is correctly indicated on the label (Council of the European Union, Directive 73/241/EEC). In addition to the difficulty of identifying a suitable biomarker to establish the authenticity of fats (Kamm et al. Citation2001), the European directive does not specify any method of analysis to test for compliance. Other cheaper fats such as butter and lard are also sometimes employed to reduce production costs, but they negatively affect the quality of chocolate, as they do not melt in the mouth easily (Bahri and Che Man Citation2016). Besides fats, collagen is added to chocolate by some manufacturers as an anti-ageing nutrient to attract health-conscious consumers, which in some countries requires authentication (Shariff and Lah Citation2014).
Some of the strategies developed so far to detect and quantify non-CB vegetable fats in chocolate use triglycerides, FAs, sterols and vitamins as indicators (Buchgraber, Ulberth, and Anklam Citation2004; Lipp and Anklam Citation1998). Triacylglycerol (TAG) compositional analyses have been extensively employed to detect adulterations with oils and fats. Unadulterated CB can be authenticated by its TAG fingerprint, which is composed of only three major TAG species, namely 1,3-dipalmitoyl-2-oleyl-glycerol, 1-palmitoyl-2-oleoyl-3-stearoyl-glycerol, and 1,3-distearoyl-2-oleoyl-glycerol (Lipp and Anklam Citation1998). Sterols, such as 4-methylsterols, triterpene alcohols and sterol degradation products, have also been used (Crews, Calvet-Sarrett, and Brereton Citation1997). The detection of several CB equivalents added to CB is possible through bulk stable carbon isotope composition analysis and δ13C compound specific measurements of FAs, even when the FA composition is similar (Spangenberg and Dionisi Citation2001). However, this approach seems unable to detect illipe fat in CB. For this reason, and also for greater precision in the quantification of vegetable fats added to CB, it should be used in combination with other established qualitative and quantitative methods.
A model based on the detection and quantification of the palm mid-fraction (PMF) added to CB mixtures was reported in 2008. HPLC was used to determine the tocopherol and tocotrienol profiles in PMF and CB samples from different manufacturers (Moazami Farahany et al. Citation2008). Alfa-tocotrienol, found only in PMF, is proposed as a promising indicator for the detection and quantification of PMF and thus also for the addition of PMF to CB.
More recently, Fourier transform infrared (FTIR) spectroscopy was applied to detect lard used in the production of commercial chocolate and to develop a calibration and validation model to determine the amount of lard added to these products (Bahri and Che Man Citation2016). The spectral bands associated with lard, CB and their mixtures were recorded, interpreted and identified. Fingerprints of functional groups by FTIR, widely used to authenticate adulteration in food analysis (Bendini et al. Citation2007; Rohman and Che Man Citation2010; De Luca et al. Citation2011; Gallardo-Velázquez et al. Citation2009), were demonstrated to be a fast and effective analytical tool for the quantitative determination of lard in chocolate, suitable for routine quality control checks.
4.2.3. Differentiation of chocolate according to the cocoa geographical origin
Chocolate samples produced with cocoa from different geographical origins (Caribbean, Madagascar, Africa and South America) were successfully differentiated by an analytical method based on volatile compounds (Cambrai et al. Citation2010). The characteristic aroma of chocolate is due to constituents (pyrazines, esters and aldehydes phenolics) strongly correlated with volatile compounds. As a result of the heat treatments during chocolate production, some of the most volatile compounds in cocoa are lost (Counet et al. Citation2002). However, as chocolate has a high fat content, it retains volatile compounds that can be analyzed by chromatographic methods. In this study, hydrodistillation was chosen as an extraction technique, and GC was used for compound separation, followed by MS (GC-MS) or flame ionization detection (GC-FID) (Cambrai et al. Citation2010). After two statistical analyses and a blind test carried out on the chemical composition of the chocolate samples, two independent groups for Africa and Madagascar were discernable. This approach also clearly separated Caribbean chocolate from that of other origins. The method developed (hydrodistillation, GC analysis and statistical treatment) has the potential to improve the control of the geographical origin of chocolate during its long production process. Furthermore, seven chemical compounds identified in the chocolate samples could be used as biomarkers of the continental origin of cocoa.
The same study also assessed the geographical origin of chocolate by FA profiling (Torres-Moreno et al. Citation2015). Similar to FA profiling used to decipher the geographical origin of cocoa beans as presented in 4.1.3.2, GC-MS was used in this study to determine the nutritional composition and FA profile of dark chocolate of various geographical origins and subjected to different processing conditions. Regarding the composition of chocolate, significant associations with origin were only observed for carbohydrates (higher in Ghanaian cocoa) and fats (higher in Ecuadorian cocoa). Analysis of variance was performed on three factors (origin, roasting, and conching time) to study if they affected the FA profile of the chocolate samples. The results indicated that only the origin had a significant effect on certain FA: the Ecuadorian samples had a significantly higher content of C16:0, C18:1 and C18:2 than the samples from Ghana, and a lower content of C18:0. FA profiles of the chocolate samples seemed largely unchanged by processing, as they were similar to those obtained for unroasted cocoa beans. Differences in the total percentage of SFA, monounsaturated FA and polyunsaturated FA were also found between the chocolate samples from the two geographic regions: Ecuadorian chocolate had a healthier FA profile and SFA/unsaturated FA ratio than the Ghanian samples, with higher amounts of unsaturated FA and lower amounts of SFA.
5. Sustainability of cocoa: a key issue for the chocolate sector
The pressure to increase cocoa production to meet the growing worldwide demand for chocolate has had negative impacts on the environment, economy and society of the producing countries. There is a need to achieve a sustainable production of high-quality cocoa (Pope, Annandale, and Morrison-Saunders Citation2004), as outlined in the sustainable development goals of the United Nations (Desa Citation2016). However, cocoa and chocolate traceability is challenging since the cocoa supply chain is complex, involving numerous stakeholders, and multiple steps (the production of raw material, processing and distribution of the final product) often spread in different regions. Solutions can be difficult to implement due to the poor governance systems in most of the cocoa-producing regions (Saltini, Akkerman, and Frosch Citation2013).
Despite initiatives to enhance sustainable agriculture, studies reveal that the cocoa sector still has many issues to resolve in this respect (Ngoucheme et al. Citation2016). Although training programs for farmers have been introduced, proper dissemination of good agricultural practices remains limited. One of the main problems is the unavailability of high-quality planting materials for smallholder farmers, which results in poor quality cocoa and low profits. In addition, cocoa-producing countries are suffering extensive deforestation and consequent loss of biodiversity (Wessel and Foluke Quist-Wessel Citation2015), which is exacerbated by microclimatic changes triggered by the large-scale removal of trees (Ruf, Schroth, and Doffangui Citation2015).
A strategy for advancing the economic conditions of cocoa farmers is through certification schemes, which aim to improve labor conditions and the profit share for farmers, with benefits both for local communities and the environment (Melykh and Melykh Citation2016). The announcement that European markets will be purchasing only certified cocoa from 2020 onwards has stimulated the development of this market niche and motivated exporters to certify their farmers. Nevertheless, the changes are slow, and more incentives are needed to support the transition toward certification. A drawback is that the price of the final product is likely to be higher, which might reduce sales, unless accompanied by sustainability-oriented marketing and labeling, targeting green consumers. In this context, chocolate packaging is also important, needing to be simple in both design and material to effectively signal sustainability. The environmental costs of packaging have long been underestimated, with focus placed primarily on food safety.
More efforts are required to bring about sustainability changes in the cocoa sector, which is suffering the negative impacts of failed governance. New organizational structures without negative effects on farming households, such as certification schemes, need further implementation.
6. Conclusions
Authenticity and traceability testing of cocoa and chocolate products is constantly evolving, and, in this review, we have attempted to provide an overview of recent updates in the field, focusing on the development of analytical tools with promising application in the cocoa sector. Nevertheless, before the sustainable production of high-quality cocoa can be achieved, many negative impacts on the environment, economy and society need to be resolved. Although manufacturers have generally welcomed the application of a traceability system that can track food from ‘farm to fork’ as a tool to reestablish and amplify consumer confidence in food safety, the chocolate industry still faces many challenges in this regard.
Acknowledgments
A. Lopez-Yerena thanks the Consejo Nacional de Ciencia y Tecnología (CONACYT) of Mexico for the doctoral scholarship. A. Vallverdú-Queralt thanks the Ministry of Science Innovation and Universities for the Ramon y Cajal contract (RYC-2016-19355). We also thank Professor Lamuela-Raventós for her support in this work.
References
- Abdi, H., and L. J. Williams. 2010. Tukey’s honestly signiflcant difierence (HSD) test. In Encyclopedia of research design, ed. N. J. Salkind, 1–5. Thousand Oaks, CA: Sage. https://www.researchgate.net/publication/237426041.
- Afoakwa, E. O. 2016. World production, processing and chocolate consumption pattern. In Chocolate science and technology, ed. E. O. Afoakwa, 2nd ed., 17–48. West Sussex, UK: John Wiley & Sons, Ltd.
- Afoakwa, E. O., A. Paterson, and M. Fowler. 2007. Factors influencing rheological and textural qualities in chocolate—A review. Trends in Food Science & Technology 18 (6):290–8. doi: https://doi.org/10.1016/j.tifs.2007.02.002.
- Afoakwa, E. O., A. Paterson, M. Fowler, A. Ryan, E. Ohene, A. Paterson, M. Fowler, A. Ryan, M. Fowler, and A. Ryan. 2008. Flavor formation and character in cocoa and chocolate: A critical review. Critical Reviews in Food Science and Nutrition 48 (9):840–57. doi: https://doi.org/10.1080/10408390701719272.
- Afoakwa, E. O., A. Paterson, M. Fowler, and J. Vieira. 2009. Fat bloom development and structure-appearance relationships during storage of under-tempered dark chocolates. Journal of Food Engineering 91 (4):571–81. doi: https://doi.org/10.1016/j.jfoodeng.2008.10.011.
- Almoosawi, S., L. Fyfe, C. Ho, and E. Al-Dujaili. 2010. The effect of polyphenol-rich dark chocolate on fasting capillary whole blood glucose, total cholesterol, blood pressure and glucocorticoids in healthy overweight and obese subjects. The British Journal of Nutrition 103 (6):842–50. doi: https://doi.org/10.1017/S0007114509992431.
- Amundson, R., A. T. Austin, E. A. G. Schuur, K. Yoo, V. Matzek, C. Kendall, A. Uebersax, D. Brenner, and W. T. Baisden. 2003. Global patterns of the isotopic composition of soil and plant nitrogen. Global Biogeochemical Cycles 17 (1):1031. doi: https://doi.org/10.1029/2002GB001903.
- AOAC International. 1990. AOAC fatty acid in oils and fats preparation of methyl ester boron trifluoride method. AAOC Vol. 15th ed. Washington, DC: AOAC International.
- Ardhana, M. M., and G. H. Fleet. 2003. The microbial ecology of cocoa bean fermentations in Indonesia. International Journal of Food Microbiology 86 (1–2):87–99. doi: https://doi.org/10.1016/S0168-1605(03)00081-3.
- Aung, M. M., and Y. S. Chang. 2014. Traceability in a food supply chain: Safety and quality perspectives. Food Control 39 (1):172–84. doi: https://doi.org/10.1016/j.foodcont.2013.11.007.
- Bahri, S. S., and Y. B. Che Man. 2016. Rapid detection of lard in chocolate and chocolate-based food products using Fourier transform infrared spectroscopy. Journal of Tropical Agriculture and Food Science 44 (2):253–63.
- Barišić, V., M. Kopjar, A. Jozinović, I. Flanjak, Đ. Ačkar, B. Miličević, D. Šubarić, S. Jokić, and J. Babić. 2019. The chemistry behind chocolate production. Molecules 24 (17):3163. doi: https://doi.org/10.3390/molecules24173163.
- Beckett, S. T. 2008. The science of chocolate. 2nd ed., 39–79. Cambridge, UK: Royal Society of Chemistry.
- Bendini, A., L. Cerretani, F. Di Virgilio, P. Belloni, M. Bonoli-Carbognin, and G. Lercker. 2007. Preliminary evaluation of the application of the FTIR spectroscopy to control the geographic origin and quality of virgin olive oils. Journal of Food Quality 30 (4):424–37. doi: https://doi.org/10.1111/j.1745-4557.2007.00132.x.
- Bertoldi, D., A. Barbero, F. Camin, A. Caligiani, and R. Larcher. 2016. Multielemental fingerprinting and geographic traceability of Theobroma cacao beans and cocoa products. Food Control 65:46–53. doi: https://doi.org/10.1016/j.foodcont.2016.01.013.
- Bindereif, S. G., F. Brauer, J.-M. Schubert, S. Schwarzinger, and G. Gebauer. 2019. Complementary use of 1H NMR and multi-element IRMS in association with chemometrics enables effective origin analysis of cocoa beans (Theobroma cacao L.). Food Chemistry 299:125105. doi: https://doi.org/10.1016/j.foodchem.2019.125105.
- Biswas, N., Y. Lin Cheow, C. P. Tan, and L. F. Siow. 2017. Physical, rheological and sensorial properties, and bloom formation of dark chocolate made with cocoa butter substitute (CBS). LWT - Food Science and Technology 82:420–8. doi: https://doi.org/10.1016/j.lwt.2017.04.039.
- Buchgraber, M., F. Ulberth, and E. Anklam. 2004. Interlaboratory evaluation of injection techniques for triglyceride analysis of cocoa butter by capillary gas chromatography. Journal of Chromatography. A 1036 (2):197–203. doi: https://doi.org/10.1016/j.chroma.2004.03.011.
- Caligiani, A., D. Acquotti, M. Cirlini, and G. Palla. 2010. 1H NMR study of fermented cocoa (Theobroma cacao L.) beans. Journal of Agricultural and Food Chemistry 58 (23):12105–11. doi: https://doi.org/10.1021/jf102985w.
- Caligiani, A., L. Palla, D. Acquotti, A. Marseglia, and G. Palla. 2014. Application of 1H NMR for the characterisation of cocoa beans of different geographical origins and fermentation levels. Food Chemistry 157:94–9. doi: https://doi.org/10.1016/j.foodchem.2014.01.116.
- Cambrai, A., C. Marcic, S. Morville, P. Sae Houer, F. Bindler, and E. Marchioni. 2010. Differentiation of chocolates according to the cocoa’s geographical origin using chemometrics. Journal of Agricultural and Food Chemistry 58 (3):1478–83. doi: https://doi.org/10.1021/jf903471e.
- Caponio, G. R., M. P. Lorusso, G. T. Sorrenti, V. Marcotrigiano, G. Difonzo, E. De Angelis, R. Guagnano, A. D. Ciaula, G. Diella, A. F. Logrieco, et al. 2020. Chemical characterization, gastrointestinal motility and sensory evaluation of dark chocolate: A nutraceutical boosting consumers’ health. Nutrients 12 (4):939–20. doi: https://doi.org/10.3390/nu12040939.
- Carrera Almeida, M. L. 2014. Análisis Sobre El Desarrollo de La Comercialización Internacional Del Cacao Nacional Fino o de Aroma Del 2002 Al 2012, Su Producción e Impacto Político, Económico y Social. Pontificia Universidad Católica del Ecuador.
- Chaiseri, S., and P. S. Dimick. 1989. Lipid and hardness characteristics of cocoa butters from different geographic regions. Journal of the American Oil Chemists' Society 66 (12):1771–6. doi: https://doi.org/10.1007/BF02660745.
- Chen, Q., J. Zhao, and H. Lin. 2009. Study on discrimination of roast green tea (Camellia sinensis L.) according to geographical origin by FT-NIR spectroscopy and supervised pattern recognition. Spectrochimica Acta. Part A, Molecular and Biomolecular Spectroscopy 72 (4):845–50. doi: https://doi.org/10.1016/j.saa.2008.12.002.
- Coetzee, P. P., F. P. Van Jaarsveld, and F. Vanhaecke. 2014. Intraregional classification of wine via ICP-MS elemental fingerprinting. Food Chemistry 164:485–92. doi: https://doi.org/10.1016/j.foodchem.2014.05.027.
- Counet, C., D. Callemien, C. Ouwerx, and S. Collin. 2002. Use of gas chromatography-olfactometry to identify key odorant compounds in dark chocolate. Comparison of samples before and after conching. Journal of Agricultural and Food Chemistry 50 (8):2385–91. doi: https://doi.org/10.1021/jf0114177.
- Craig, A. P., A. S. Franca, and J. Irudayaraj. 2013. Surface-enhanced Raman spectroscopy applied to food safety. Annual Review of Food Science and Technology 4:369–80. doi: https://doi.org/10.1146/annurev-food-022811-101227.
- Crews, C., R. Calvet-Sarrett, and P. Brereton. 1997. The analysis of sterol degradation products to detect vegetable fats in chocolate. Journal of the American Oil Chemists' Society 74 (10):1273–80. doi: https://doi.org/10.1007/s11746-997-0057-5.
- D’Archivio, A. A., A. Giannitto, A. Incani, and S. Nisi. 2014. Analysis of the mineral composition of Italian saffron by ICP-MS and classification of geographical origin. Food Chemistry 157:485–9. doi: https://doi.org/10.1016/j.foodchem.2014.02.068.
- D’Souza, R. N., S. Grimbs, B. Behrends, H. Bernaert, M. S. Ullrich, and N. Kuhnert. 2017. Origin-based polyphenolic fingerprinting of Theobroma cacao in unfermented and fermented beans. Food Research International (Ottawa, Ont.) 99 (Pt 1):550–9. doi: https://doi.org/10.1016/j.foodres.2017.06.007.
- de Brito, E. S., N. H. P. García, M. I. Gallão, A. L. Cortelazzo, P. S. Fevereiro, and M. R. Braga. 2001. Structural and chemical changes in cocoa (Theobroma cacao L q) during fermentation, drying and roasting. Journal of the Science of Food and Agriculture 81 (2):281–8. doi: https://doi.org/10.1002/1097-0010(20010115)81:2<281::AID-JSFA808>3.0.CO;2-B.
- De Luca, M., W. Terouzi, G. Ioele, F. Kzaiber, A. Oussama, F. Oliverio, R. Tauler, and G. Ragno. 2011. Derivative FTIR spectroscopy for cluster analysis and classification of morocco olive oils. Food Chemistry 124 (3):1113–8. doi: https://doi.org/10.1016/j.foodchem.2010.07.010.
- Decroix, L., D. D. Soares, R. Meeusen, E. Heyman, and C. Tonoli. 2018. Cocoa flavanol supplementation and exercise: A systematic review. Sports Medicine (Auckland, N.Z.) 48 (4):867–92. doi: https://doi.org/10.1007/s40279-017-0849-1.
- Desa, U. N. 2016. Transforming our world: The 2030 agenda for sustainable development, 12–4. New York, NY: Division for Sustainable Development Goals.
- Diomande, D., I. Antheaume, M. Leroux, J. Lalande, S. Balayssac, G. S. Remaud, and I. Tea. 2015. Multi-element. Food Chemistry 188:576–82. doi:https://doi.org/10.1016/j.foodchem.2015.05.040.
- Djikeng, F. T., W. T. Teyomnou, N. Tenyang, B. Tiencheu, A. T. Morfor, B. A. H. Touko, S. N. Houketchang, G. T. Boungo, M. S. L. Karuna, F. Z. Ngoufack, et al. 2018. Effect of traditional and oven roasting on the physicochemical properties of fermented cocoa beans. Heliyon 4 (2):e00533. doi: https://doi.org/10.1016/j.heliyon.2018.e00533.
- Fayeulle, N., E. Meudec, J. C. Boulet, A. Vallverdu-Queralt, C. Hue, R. Boulanger, V. Cheynier, and N. Sommerer. 2019. Fast discrimination of chocolate quality based on average-mass-spectra fingerprints of cocoa polyphenols. Journal of Agricultural and Food Chemistry 67 (9):2723–31. doi: https://doi.org/10.1021/acs.jafc.8b06456.
- Fayeulle, N., A. Vallverdu-Queralt, E. Meudec, C. Hue, R. Boulanger, V. Cheynier, and N. Sommerer. 2018. Characterization of new flavan-3-ol derivatives in fermented cocoa beans. Food Chemistry 259:207–12. doi: https://doi.org/10.1016/j.foodchem.2018.03.133.
- Fletcher, B. C., Pine, K. J. W. Zoe, and N. Avril. 2007. How visual images of chocolate affect the craving and guilt of female dieters. Appetite 48 (2):211–7. doi: https://doi.org/10.1016/j.appet.2006.09.002.
- Frauendorfer, F., and P. Schieberle. 2008. Changes in key aroma compounds of Criollo cocoa beans during roasting. Journal of Agricultural and Food Chemistry 56 (21):10244–51. doi: https://doi.org/10.1021/jf802098f.
- Gallardo-Velázquez, T., G. Osorio-Revilla, M. Zuñiga de Loa, and Y. Rivera-Espinoza. 2009. Application of FTIR-HATR spectroscopy and multivariate analysis to the quantification of adulterants in Mexican honeys. Food Research International 42 (3):313–8. doi: https://doi.org/10.1016/j.foodres.2008.11.010.
- Georgiou, C. A., and G. P. Danezis. 2015. Elemental and isotopic mass spectrometry. Comprehensive Analytical Chemistry 68:131–243. doi: https://doi.org/10.1016/B978-0-444-63340-8.00003-0.
- Gil, M., F. Orrego, E. Cadena, R. Alegria, and J. Londono-Londono. 2016. Effect of pectin lyase enzyme on fermentation and drying of cocoa (Theobroma cacao L.): An alternative to improve raw material in the industry of chocolate. Food and Nutrition Sciences 7 (4):215–26. doi: https://doi.org/10.4236/fns.2016.74023.
- Guiltinan, M. J., J. Verica, D. Zhang, and A. Figueira. 2008. Genomics of Theobroma cacao, ‘the food of the gods’. In Genomics of tropical crop plants, edited by Paul H. Moore and Ray Ming, 145–70. New York, NY: Springer.
- Hansen, C. E., M. Olmo, and C. Burri. 1998. Enzyme activities in cocoa beans during fermentation. Journal of the Science of Food and Agriculture 77 (2):273–81. doi: https://doi.org/10.1002/(SICI)1097-0010(199806)77:2<273::AID-JSFA40>3.0.CO;2-M.
- Herrmann, L., I. Haase, M. Blauhut, N. Barz, and M. Fischer. 2014. DNA-based differentiation of the Ecuadorian cocoa types CCN-51 and arriba based on sequence differences in the chloroplast genome. Journal of Agricultural and Food Chemistry 62 (50):12118–27. doi: https://doi.org/10.1021/jf504258w.
- Jinap, S., J. Thien, and T. N. Yap. 1994. Effect of drying on acidity and volatile fatty acids content of cocoa beans. Journal of the Science of Food and Agriculture 65 (1):67–75. doi: https://doi.org/10.1002/jsfa.2740650111.
- Jinap, S., W. I. Wan Rosli, A. R. Russly, and L. M. Nordin. 1998. Effect of roasting time and temperature on volatile component profiles during nib roasting of cocoa beans (Theobroma cacao). Journal of the Science of Food and Agriculture 77 (4):441–8. doi: https://doi.org/10.1002/(sici)1097-0010(199808)77:4 < 441::aid-jsfa46 > 3.0.co;2-%23.
- Kaffka, K. J., K. H. Norris, F. Kulcsar, and I. Draskovits. 1982. Attempts to determine fat, protein and carbohydrate content in cocoa powder by the NIR technique [near infrared]. Acta Alimentaria (Hungary) 11 (3):271–88.
- Kamm, W., F. Dionisi, C. Hischenhuber, and K. H. Engel. 2001. Authenticity assessment of fats and oils. Food Reviews International 17 (3):249–90. doi: https://doi.org/10.1081/FRI-100104702.
- Kendall, H., B. Clark, C. Rhymer, S. Kuznesof, J. Hajslova, M. Tomaniova, P. Brereton, and L. Frewer. 2019. A systematic review of consumer perceptions of food fraud and authenticity: A European perspective. Trends in Food Science & Technology 94:79–90. doi: https://doi.org/10.1016/j.tifs.2019.10.005.
- Kennedy, J., and H. Heymann. 2009. Projective mapping and descriptive analysis of milk and dark chocolates. Journal of Sensory Studies 24 (2):220–33. doi: https://doi.org/10.1111/j.1745-459X.2008.00204.x.
- Khuda, S. E., L. S. Jackson, T. Jen Fu, and K. M. Williams. 2015. Effects of processing on the recovery of food allergens from a model dark chocolate matrix. Food Chemistry 168:580–7. doi: https://doi.org/10.1016/j.foodchem.2014.07.084.
- Konar, N., O. S. Toker, S. Oba, and O. Sagdic. 2016. Improving functionality of chocolate: A review on probiotic, prebiotic, and/or synbiotic characteristics. Trends in Food Science & Technology 49:35–44. doi: https://doi.org/10.1016/j.tifs.2016.01.002.
- Kongor, J. E., M. Hinneh, D. V. de Walle, E. O. Afoakwa, P. Boeckx, and K. Dewettinck. 2016. Factors influencing quality variation in cocoa (Theobroma cacao) bean flavour profile: A review. Food Research International 82:44–52. doi: https://doi.org/10.1016/j.foodres.2016.01.012.
- Kostic, M. J. 1997. Cocoa alkalization. Manufacturing Confectioner 77:128–30.
- Lakshmi, V. 2012. Food adulteration. International Journal of Sciences Inventions Today 1 (2):106–13.
- Lecumberri, E., R. Mateos, M. Izquierdo-Pulido, P. Rupérez, L. Goya, and L. Bravo. 2007. Dietary fibre composition, antioxidant capacity and physico-chemical properties of a fibre-rich product from cocoa (Theobroma cacao L.). Food Chemistry 104 (3):948–54. doi: https://doi.org/10.1016/j.foodchem.2006.12.054.
- Lenfant, F., C. Hartmann, B. Watzke, O. Breton, C. Loret, and N. Martin. 2013. Impact of the shape on sensory properties of individual dark chocolate pieces. LWT - Food Science and Technology 51 (2):545–52. doi: https://doi.org/10.1016/j.lwt.2012.11.001.
- Li, Y., Y. Feng, S. Zhu, C. Luo, J. Ma, and F. Zhong. 2012. The effect of alkalization on the bioactive and flavor related components in commercial cocoa powder. Journal of Food Composition and Analysis 25 (1):17–23. doi: https://doi.org/10.1016/j.jfca.2011.04.010.
- Li, Y., S. Zhu, Y. Feng, F. Xu, J. Ma, and F. Zhong. 2014. Influence of alkalization treatment on the color quality and the total phenolic and anthocyanin contents in cocoa powder. Food Science and Biotechnology 23 (1):59–63. doi: https://doi.org/10.1007/s10068-014-0008-5.
- Lipp, M., and E. Anklam. 1998. Review of cocoa butter and alternative fats for use in chocolate—Part B. Analytical approaches for identification and determination. Food Chemistry 62 (1):99–108. doi: https://doi.org/10.1016/S0308-8146(97)00161-1.
- Madejczyk, M., and D. Baralkiewicz. 2008. Characterization of Polish rape and honeydew honey according to their mineral contents using ICP-MS and F-AAS/AES. Analytica Chimica Acta 617 (1–2):11–7. doi: https://doi.org/10.1016/j.aca.2008.01.038.
- Magagna, F., A. Guglielmetti, E. Liberto, S. E. Reichenbach, E. Allegrucci, G. Gobino, C. Bicchi, and C. Cordero. 2017. Comprehensive chemical fingerprinting of high-quality cocoa at early stages of processing: Effectiveness of combined untargeted and targeted approaches for classification and discrimination. Journal of Agricultural and Food Chemistry 65 (30):6329–41. doi: https://doi.org/10.1021/acs.jafc.7b02167.
- Manton, W. I. 2010. Determination of the provenance of cocoa by soil protolith ages and assessment of anthropogenic lead contamination by Pb/Nd and lead isotope ratios. Journal of Agricultural and Food Chemistry 58 (2):713–21. doi: https://doi.org/10.1021/jf9029207.
- Marseglia, A., D. Acquotti, R. Consonni, L. R. Cagliani, G. Palla, and A. Caligiani. 2016. HR MAS 1H NMR and chemometrics as useful tool to assess the geographical origin of cocoa beans—Comparison with HR 1H NMR. Food Research International (Ottawa, Ont.) 85:273–81. doi: https://doi.org/10.1016/j.foodres.2016.05.001.
- Meier, B. P., S. W. Noll, and O. J. Molokwu. 2017. The sweet life: The effect of mindful chocolate consumption on mood. Appetite 108:21–7. doi: https://doi.org/10.1016/j.appet.2016.09.018.
- Melykh, K., and O. Melykh. 2016. Implication of environmental certification and CSR for companies’ sustainable performance in developing countries. Journal of Sustainable Development 9 (3):160–9. doi: https://doi.org/10.5539/jsd.v9n3p160.
- Miller, K. B., W. J. Hurst, M. J. Payne, D. A. Stuart, J. Apgar, D. S. Sweigart, and B. Ou. 2008. Impact of alkalization on the antioxidant and flavanol content of commercial cocoa powders. Journal of Agricultural and Food Chemistry 56 (18):8527–33. doi: https://doi.org/10.1021/jf801670p.
- Moazami Farahany, E., J. Selamat, Y. B. Che Man, and N. A. Idris. 2008. Detection and quantification of palm mid-fraction in a chocolate model system. International Journal of Food Science & Technology 43 (6):1083–7. doi: https://doi.org/10.1111/j.1365-2621.2007.01570.x.
- Naik, B., and V. Kumar. 2014. Cocoa butter and its alternatives: A reveiw. Journal of Bioresource Engineering and Technology 2:1–11.
- Nazaruddin, R., L. K. Seng, O. Hassan, and M. Said. 2006. Effect of pulp preconditioning on the content of polyphenols in cocoa beans (Theobroma cacao) during fermentation. Industrial Crops and Products 24 (1):87–94. doi: https://doi.org/10.1016/j.indcrop.2006.03.013.
- Ngoucheme, R., C. B. Kamdem, P. Jagoret, and M. Havard. 2016. Impact de la certification sur les performances agro-economiques des producteurs de cacao du centre Cameroun. Addis Ababa, 5th International Conference of AAAE 310-2016–5, 23–6.
- Pelsmaeker, S. D., G. De Clercq, X. Gellynck, and J. J. Schouteten. 2019. Development of a sensory wheel and lexicon for chocolate. Food Research International (Ottawa, Ont.) 116:1183–91. doi: https://doi.org/10.1016/j.foodres.2018.09.063.
- Perini, M., L. Bontempo, L. Ziller, A. Barbero, A. Caligiani, and F. Camin. 2016. Stable isotope composition of cocoa beans of different geographical origin. Journal of Mass Spectrometry: JMS 51 (9):684–9. doi: https://doi.org/10.1002/jms.3833.
- Pertanian, U. 1994. Formation of methyl pyrazine during cocoa bean fermentation. Pertanika 17:27.
- Petry, R., M. Schmitt, and J. Popp. 2003. Raman spectroscopy: A prospective tool in the life sciences. Chemphyschem 4 (1):14–30.
- Pilgrim, T. S., R. J. Watling, and K. Grice. 2010. Application of trace element and stable isotope signatures to determine the provenance of tea (Camellia sinensis) samples. Food Chemistry 118 (4):921–6. doi: https://doi.org/10.1016/j.foodchem.2008.08.077.
- Pope, J., D. Annandale, and A. Morrison-Saunders. 2004. Conceptualising sustainability assessment. Environmental Impact Assessment Review 24 (6):595–616. doi: https://doi.org/10.1016/j.eiar.2004.03.001.
- Posudin, Y. I., K. S. Peiris, and S. J. Kays. 2015. Non-destructive detection of food adulteration to guarantee human health and safety. Ukrainian Food Journal 4 (2):207–353.
- Radojc, I., K. Markovic, and S. M. Jolic. 2011. Changes of phenolic compounds and antioxidant capacity in cocoa beans processing. International Journal of Food Science & Technology 46 (9):1793–800. doi: https://doi.org/10.1111/j.1365-2621.2011.02670.x.
- Ribeiro, J. S., M. M. C. Ferreira, and T. J. G. Salva. 2011. Chemometric models for the quantitative descriptive sensory analysis of Arabica coffee beverages using near infrared spectroscopy. Talanta 83 (5):1352–8. doi: https://doi.org/10.1016/j.talanta.2010.11.001.
- Rodriguez-Campos, J., H. B. Escalona-Buendía, I. Orozco-Avila, E. Lugo-Cervantes, and M. E. Jaramillo-Flores. 2011. Dynamics of volatile and non-volatile compounds in cocoa (Theobroma cacao L.) during fermentation and drying processes using principal components analysis. Food Research International Journal 44 (1):250–8. doi: https://doi.org/10.1016/j.foodres.2010.10.028.
- Roelofsen, B. Y. P. A. 1958. Fermentation, drying, and storage of cacao beans. In Advances in food research, edited by E. M. Mrak and G. F. Stewart, 225–96. Cambridge, MA: Academic Press.
- Rohman, A., and Y. B. Che Man. 2010. Fourier transform infrared (FTIR) spectroscopy for analysis of extra virgin olive oil adulterated with palm oil. Food Research International 43 (3):886–92. doi: https://doi.org/10.1016/j.foodres.2009.12.006.
- Rohsius, C., R. Matissek, and R. Lieberei. 2006. Free amino acid amounts in raw cocoas from different origins. European Food Research and Technology 222 (3–4):432–8. doi: https://doi.org/10.1007/s00217-005-0130-y.
- Rottiers, H., D. Amelia, T. Sosa, L. Van De Vyver, M. Hinneh, H. Everaert, J. De Wever, K. Messens, and K. Dewettinck. 2019. Discrimination of cocoa liquors based on their odor fingerprint: A fast GC electronic nose suitability study. Food Analytical Methods 12 (2):475–88. doi: https://doi.org/10.1007/s12161-018-1379-7.
- Ruf, F., G. Schroth, and K. Doffangui. 2015. Climate change, cocoa migrations and deforestation in West Africa: What does the past tell us about the future? Sustainability Science 10 (1):101–11. doi: https://doi.org/10.1007/s11625-014-0282-4.
- Ryan, C. M., W. Khoo, L. Ye, J. D. Lambert, S. F. O. Keefe, and A. P. Neilson. 2016. Loss of native flavanols during fermentation and roasting does not necessarily reduce digestive enzyme-inhibiting bioactivities of cocoa. Journal of Agricultural and Food Chemistry 64 (18):3616–25. doi: https://doi.org/10.1021/acs.jafc.6b01725.
- Saltini, R., R. Akkerman, and S. Frosch. 2013. Optimizing chocolate production through traceability: A review of the influence of farming practices on cocoa bean quality. Food Control 29 (1):167–87. doi: https://doi.org/10.1016/j.foodcont.2012.05.054.
- Šelih, V. S., M. Šala, and V. Drgan. 2014. Multi-element analysis of wines by ICP-MS and ICP-OES and their classification according to geographical origin in Slovenia. Food Chemistry 153:414–23. doi: https://doi.org/10.1016/j.foodchem.2013.12.081.
- Shariff, S. M., and N. A. A. Lah. 2014. Halal certification on chocolate products: A case study. Procedia - Social and Behavioral Sciences 121:104–12. doi: https://doi.org/10.1016/j.sbspro.2014.01.1112.
- Spangenberg, J. E., and F. Dionisi. 2001. Characterization of cocoa butter and cocoa butter equivalents by bulk and molecular carbon isotope analyses: Implications for vegetable fat quantification in chocolate. Journal of Agricultural and Food Chemistry 49 (9):4271–7. doi: https://doi.org/10.1021/jf001509g.
- Sun-Waterhouse, D., and S. S. Wadhwa. 2013. Industry-relevant approaches for minimising the bitterness of bioactive compounds in functional foods: A review. Food and Bioprocess Technology 6 (3):607–27. doi: https://doi.org/10.1007/s11947-012-0829-2.
- Szymańska, E., E. Saccenti, A. K. Smilde, and J. A. Westerhuis. 2012. Double-check: Validation of diagnostic statistics for PLS-DA models in metabolomics studies. Metabolomics 8 (Suppl 1):3–16. doi: https://doi.org/10.1007/s11306-011-0330-3.
- Teye, E., X. Huang, H. Dai, and Q. Chen. 2013. Rapid differentiation of Ghana cocoa beans by FT-NIR spectroscopy coupled with multivariate classification. Spectrochimica Acta. Part A, Molecular and Biomolecular Spectroscopy 114:183–9. doi: https://doi.org/10.1016/j.saa.2013.05.063.
- Thamke, I., K. Dürrschmid, and H. Rohm. 2009. Sensory description of dark chocolates by consumers. LWT - Food Science and Technology 42 (2):534–9. doi: https://doi.org/10.1016/j.lwt.2008.07.006.
- Torres-Moreno, M., A. Tarrega, E. Costell, and C. Blanch. 2012. Dark chocolate acceptability: Influence of cocoa origin and processing conditions. Journal of the Science of Food and Agriculture 92 (2):404–11. doi: https://doi.org/10.1002/jsfa.4592.
- Torres-Moreno, M., A. Tarrega, E. Torrescasana, and C. Blanch. 2012. Influence of label information on dark chocolate acceptability. Appetite 58 (2):665–71. doi: https://doi.org/10.1016/j.appet.2011.12.005.
- Torres-Moreno, M., E. Torrescasana, J. Salas-Salvadó, and C. Blanch. 2015. Nutritional composition and fatty acids profile in cocoa beans and chocolates with different geographical origin and processing conditions. Food Chemistry 166:125–32. doi: https://doi.org/10.1016/j.foodchem.2014.05.141.
- Valentini, M., M. Ritota, C. Cafiero, S. Cozzolino, L. Leita, and P. Sequi. 2011. The HRMAS-NMR tool in foodstuff characterisation. Magnetic Resonance in Chemistry 49 (Suppl 1):S121–S5. doi: https://doi.org/10.1002/mrc.2826.
- Vargas Jentzsch, P., V. Ciobotă, W. Salinas, B. Kampe, P. M. Aponte, P. Rösch, J. Popp, and L. A. Ramos. 2016. Distinction of Ecuadorian varieties of fermented cocoa beans using Raman spectroscopy. Food Chemistry 211:274–80. doi: https://doi.org/10.1016/j.foodchem.2016.05.017.
- Veselá, A., A. S. Barros, A. Synytsya, I. Delgadillo, J. Čopíková, and M. A. Coimbra. 2007. Infrared spectroscopy and outer product analysis for quantification of fat, nitrogen, and moisture of cocoa powder. Analytica Chimica Acta 601 (1):77–86. doi: https://doi.org/10.1016/j.aca.2007.08.039.
- Vítová, E., B. Loupancová, H. Štoudková, I. Macku, J. Zemanová, and L. Babák. 2009. Effect of fat composition on some physico-chemical parameters and sensorial evaluation of dark chocolate. Journal of Food and Nutrition Research 48 (2):72–9.
- Vogel, C., S. Mathé, M. Geitzenauer, H. T. Ndah, S. Sieber, M. Bonatti, and M. Lana. 2020. Stakeholders’ perceptions on sustainability transition pathways of the cocoa value chain towards improved livelihood of small-scale farming households in Cameroon. International Journal of Agricultural Sustainability 18 (1):55–69. doi: https://doi.org/10.1080/14735903.2019.1696156.
- Wessel, M., and P. M. Foluke Quist-Wessel. 2015. Cocoa production in West Africa, a review and analysis of recent developments. NJAS - Wageningen Journal of Life Sciences 74–75:1–75. doi: https://doi.org/10.1016/j.njas.2015.09.001.
- Whitacre, E., J. O. Liver, R. Broek, P. Engelen, B. K. Remers, B. Horst, M. S. Tewart, and A. Jansen-Beuvink. 2003. Predictive analysis of cocoa procyanidins using near-infrared spectroscopy techniques. Journal of Food Science 68 (9):2618–22. doi: https://doi.org/10.1111/j.1365-2621.2003.tb05779.x.
- Wollgast, J., and E. Anklam. 2000. Review on polyphenols in Theobroma cacao: Changes in composition during the manufacture of chocolate and methodology for identification and quantification. Food Research International 33 (6):423–47. doi: https://doi.org/10.1016/S0963-9969(00)00068-5.
- Zhang, A., A. Mankad, and A. Ariyawardana. 2020. Establishing confidence in food safety: Is traceability a solution in consumers’ eyes? Journal of Consumer Protection and Food Safety 15 (2):99–107. doi: https://doi.org/10.1007/s00003-020-01277-y.
- Żyżelewicz, D., G. Budryn, J. Oracz, H. Antolak, D. Kręgiel, and M. Kaczmarska. 2018. The effect on bioactive components and characteristics of chocolate by functionalization with raw cocoa beans. Food Research International (Ottawa, Ont.) 113:234–44. doi: https://doi.org/10.1016/j.foodres.2018.07.017.