Abstract
As a notorious food-borne pathogen, Staphylococcus aureus can readily cause diseases in humans via contaminated food. Biofilm formation on various surfaces can increase the capacity of viable S. aureus cells for self-protection due to the stubborn structure of the biofilm matrix. Increased disease risk and economic losses caused by biofilm contamination in the food industry necessitate the urgent development of effective strategies for the inhibition and removal of S. aureus biofilms. Natural products have been extensively used as important sources of “eco-friendly” antibiofilm agents to avoid the side effects of conventional strategies on human health and the environment. This review discusses biofilm formation of S. aureus in food industries and focuses on providing an overview of potential promising target-oriented natural products and their mechanisms of S. aureus biofilm inhibition or removal. Hoping to provide valuable information of attractive research targets or potential undeveloped targets to screen potent natural anti-biofilm agents in food industries.
Introduction
In recent years, food safety has become a worldwide focus of public health. As one of the most common food safety issues, microbial contamination causes food spoilage and foodborne illness, not only seriously affecting people’s health but also incurring huge economic losses. Bacterial biofilms are the main culprits of sanitary problems and economic losses in the food industry. Bacteria can remain in food and attach to various surfaces by building three-dimensional rigid biofilm structures. Biofilm formation can enhance bacterial resistance to environmental stresses, which leads to increased cleaning difficulty and poses challenges for food hygiene (Avila-Novoa et al. Citation2018). In the food industry, the formation of biofilms in piping, equipment or heat exchangers can lead to the potential for metal corrosion, a decrease in operational efficiencies of equipment and an increase in maintenance costs (Xu et al. Citation2018). On the global scale, the annual impact of biofilms is incalculable, resulting in the loss of millions of dollars or more in food industries (Alonso, Harada, and Kabuki Citation2020; Cattò and Cappitelli Citation2019). Furthermore, biofilm formation occurs not only on environmental processing surfaces but also on food itself, leading to potential for cross-contamination and foodborne illness. Staphylococcus aureus is one of the most common foodborne pathogens related to foodborne disease outbreaks, especially in the meat and dairy industries (Guo et al. Citation2020). Thus, removal or inhibition strategies for S. aureus biofilms are an area of growing worldwide concern for public health.
In recent years, significant research efforts have been devoted to discovering biofilm inhibition and removal strategies, which have yielded several promising achievements. Traditional cleaning methods, including heat, detergents, surfactants, and disinfectants (Simões, Simões, and Vieira Citation2010). To avoid the negative effects of conventional chemical and physical strategies on humans and the environment, such as high energy consumption, high toxicity, the emergence of resistance, etc., efforts are focused on natural products to develop “green” anti-biofilm agents (Elmasri et al. Citation2015; Xu et al. Citation2017; Wu et al. Citation2019a).
Much attention has been directed toward target-based screening for natural antibiofilm agents over the past two decades. For example, quorum sensing (QS) systems are typical research target related to biofilms (Truchado et al. Citation2015; Chen et al. Citation2018). Although some natural products have been found which can inhibit S. aureus biofilm by disturbing QS system, further studies are still necessary because the mechanisms are still not fully understood. Additionally, an increasing number of new antibiofilm targets have been studied for S. aureus via various methods (e.g. computer virtual screening, molecular biology methods, etc.) (Yan et al. Citation2019; Sayed et al. Citation2020; Liang et al. Citation2019). These findings provide the basis for screening effective and “green” antibiofilm agents.
This review discusses common food environments where S. aureus biofilms easily occur; subsequently, we focus on the promising natural products of target-based screening and discuss their antibiofilm mechanisms. In addition, research limitations and future prospects for natural antibiofilm product target-oriented screening are provided.
S. aureus biofilms in food industries
In food processing operations and storage, in addition to existing in raw materials, most bacteria are associated with food contact surfaces and easily accumulate to form biofilms, which induce cross-contamination for transmitting pathogens to food. S. aureus can develop biofilms on different surfaces with nutrient-rich food residues, especially in the dairy and meat industries ().
In dairy industry, numerous instances of contamination have been reported. MRSA or MSSA biofilm-forming strains were isolated from raw milk or milk products such as cheese (Kang et al. Citation2020; Dai et al. Citation2019; Basanisi et al. Citation2017). Throughout the whole dairy supply chain, production involves milk and cheese in contact with the walls of the equipment. Because biofilms easily attach to stainless steel surfaces of equipment once contaminated, they can secrete exotoxin, which is a challenge for food safety in the dairy industry (Dutra et al. Citation2018; Avila-Novoa et al. Citation2018). More importantly, in dairy equipment, the development of biofilms is very rapid: 4–12 h (Meesilp and Mesil Citation2019). Furthermore, biofilm formation and dispersion behavior of foodborne pathogens are different in different dairy substrates due to varied composition and chemical alterations, such as pH. Alonso et al observed a greater dispersion of biofilm cells in skimmed milk (Alonso and Kabuki Citation2019). In addition, Carvalho et al. found that S. aureus has the capacity to form biofilms in low-density polyethylene packages when stored at 5 °C in the presence of Minas Frescal cheese whey, which indicates that packaging may be a potential source for dairy contamination (Carvalho et al. Citation2021).
In the meat industry, contamination caused by S. aureus biofilms mainly involves three aspects: (i) raw materials: as one of the most common pathogens originating from animals consumed as food, S. aureus has been discovered and isolated from pork, chickens, raw fish (Ali et al. Citation2021; Carvalho et al. Citation2020; Wu and Su Citation2014; Ou et al. Citation2020; Sullivan et al. Citation2020; Lin et al. Citation2019). Under appropriate conditions, initial attachment of biofilms on the surface may occur and induce cross-contamination through cutting boards, knives, and working surfaces (Llorens et al. Citation2012); (ii) food processing environment and equipment: in addition to slaughterhouses, operator bodies and clothes, which are often ignored, provide the perfect places for bacterial adhesion. Once bacteria are present on these surfaces due to incomplete cleaning, biofilm formation easily occurs (Al-Shabib et al. Citation2017a; de Souza et al. Citation2014); (iii) transportation and storage occur throughout the food chain, from transportation to sale, and bacterial biofilm formation easily occurs due to unsanitary environments or nonstandard worker operations (Miao et al. Citation2017).
Target-based screening for natural products
Biofilm formation is manipulated by various regulators involved in a complex regulatory network. Thus, to screen effective natural antibiofilm agents, it is necessary to research and determine one or multiple molecular targets. The biofilm formation process contains 4 stages: (1) initial biofilm attachment, (2) accumulation of layers, (3) biofilm maturation production of extracellular polymeric substrate (EPS), and (4) detachment of cells, as shown in . Natural products can control biofilms by targeting these stages of the process. In the following discussion, we focus on promising natural products based on different molecular targets involved in the process.
Figure 2. Scheme of the network pathways governing biofilm formation and disruption in S. aureus. PIA, Polysaccharide intercellular adhesin; eDNA, Extracellular DNA; QS, Quorum sensing; LuxS, S-ribosylhomocysteine lyase; AI-2, Interspecies autoinducer; Agr, Accessory gene regulator protein locus; Spx, Global transcriptional regulator Spx; IcaR, Biofilm operon icaADBC HTH-type negative transcriptional regulator; SarA, Transcriptional regulator; SarR, HTH-type transcriptional regulator; σB, RNA polymerase sigma factor; PSMs, phenol-soluble modulins; CidA, Holin-like protein.
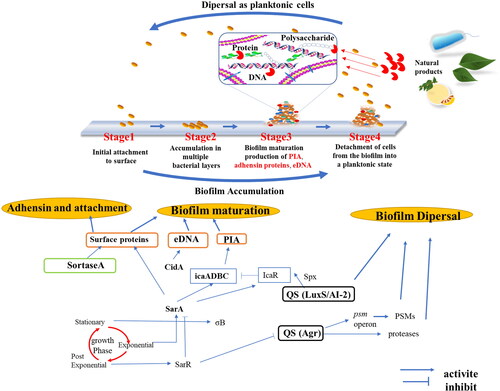
Target adhesion and attachment stage
At the beginning of biofilm formation, adhesion to various surfaces is a major strategy by S. aureus cells to remain in food or invade host cells. As adhesive matrix molecules, a vast array of Staphylococcal surface proteins are mobilized in this process, such as fibronectin-binding proteins FnbpA, FnbpB and ClfA. These proteins can be regarded as research targets for anti-biofilm agents discovery. As approved edible spice by national standard in China (GB1886.270-2016), tea tree oil showed high biofilm removal activity and eradicate pre-formed biofilm by 75% at minimum inhibitory concentration (MIC) concentration for 48 h, which targets surface proteins FnbB, as shown in (Zhao et al. Citation2018). Thus, it can be considered as a potential promising natural product for development of anti-biofilm agents.
Table 1. Natural Products screening for inhibiting biofilm formation or removing pre-formed biofilm targeted adhesion proteins.
Some of these surface proteins carry a C-terminal LPXTG motif, which can be recognized by the membrane cysteine transpeptidase sortase A (SrtA). Briefly, the transpeptidation reaction mediated by SrtA is indispensable for anchoring these surface proteins on the cell wall (). As important drug targets for controlling some attached proteins that mediate biofilm accumulation and adhesion, SrtA is not essential for bacterial growth or viability (Nitulescu et al. Citation2019). Thus, its inhibition should produce mild evolutionary pressure that will not favor the emergence of resistance. Inhibitors targeting this process are divided into two categories: (i) blocking SrtA activity directly by interacting with the catalytic active site. Chlorogenic acid has been found to bind to C184 and G192 in SrtA (Wang et al. Citation2015). Maresso et al. found that small molecules aryl (β-amino) ethyl ketones inhibit SrtA through irreversible, covalent modification of their active site cysteine (Maresso et al. Citation2007). Promising natural products, such as kaempferol, have been found to inhibit S. aureus biofilm formation (>80% inhibition rate at a sub-MIC 64 µg/mL, 18 h) by decreasing SrtA activity and the expression of the fnbpA, fnbpB, clfA, clfB and sarA genes (). Some other natural products, such as myricetin, esculein and palmatine chlorides, showed potent inhibitory activity on SrtA by virtual screening studies (Nitulescu et al. Citation2017; Nitulescu et al. Citation2016). A subsequent study proved that myricetin has biofilm inhibitory activity (64 µg/mL, 24 h, ≈70% inhibition rate) (), which indicates the reliability of a structure-based virtual screening strategy for target-oriented inhibitor discovery. (ii) SrtA can also recognize and couple synthetic molecules containing LPXTG motifs onto the peptidoglycan layer of S. aureus (Nelson et al. Citation2010). Therefore, molecules containing the LPXTG motif may be potential competitive inhibitors that interfere with the recognition of the LPXTG motif on surface proteins.
Figure 3. Mechanism of surface protein anchoring to the cell wall mediated by sortase A. Surface proteins precursors P1 with a C-terminal LPXTG motif are produced in the cytoplasm. SrtA is responsible for cleaving the amide bond between the threonine and glycine of the motif and catalyzing the formation of amide bond to form a Gly5 cross-bridge between the surface protein fragment and the peptidoglycan biosynthesis intermediate lipid II (Gao et al. Citation2016). Key molecule can be seen as antibiofilm and antibacterial targets, encircled in red. P1, P2, P3 represent Surface proteins precursors in different stages. MSCRAMMs: microbial surface components recognizing adhesive matrix molecule.
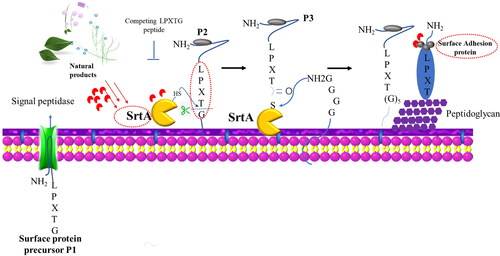
Target maturation stage
In S. aureus mature biofilms, the matrix accounts for more than 90% of the dry mass, whereas the microorganisms account for less than 10%. The EPS matrix comprises various types of polymeric substrates, including polysaccharides, nucleic acids (such as extracellular DNA), proteins, lipids, etc., and is indispensable for the construction of a three-dimensional biofilm architecture scaffold (). Many natural products were found to reduce biofilms by destroying or decreasing biofilm matrix EPS directly, as presented in .
Table 2. Natural Products screening for inhibiting biofilm formation or removing pre-formed biofilm targeted EPS.
Strategy against polysaccharide intercellular adhesin (PIA)
As a major fraction of the EPS matrix, exopolysaccharides are essential for biofilm formation. The biosynthesis of PIA is mediated by the intercellular adhesion (IcaADBC) locus (Archer et al. Citation2011). The natural product (+)-nootkatone (edible spices, GB 2760-2014) from grapefruit significantly prevented the biofilm formation (inhibition rate 99.8%, 100 μg/mL) of S. aureus by inhibiting the IcaA operon, as listed in . Another natural product gallic acid used as antioxidant which is regarded as safe in the food industry also exhibited anti-biofilm activity and regulated ica operon expression (Oliveira et al. Citation2020; M. Liu et al. Citation2017). Furthermore, the transcriptional regulator SarA can upregulate the production of biofilms by modulating ica transcription and the expression of poly-N-acetylglucosamine (PIA precursor) (Arciola et al. Citation2012). The biofilm inhibition activity of licochalcone A and emodin has been identified by decreasing the expression of sarA (Yan et al. Citation2017). Additionally, Ma et al. proposed that the S-ribosylhomocysteine lyase LuxS can reduce cell-to-cell adhesion by repressing rbf expression and inducing the downregulation of PIA. Thus, rbf might be considered as a potential research target (Ma et al. Citation2017).
Strategy against extracellular DNA (eDNA)
Another component in S. aureus biofilms, eDNA, requires the presence of matrix proteins to adhere to the cells, playing a role as an electrostatic net to hold cells together in large clumps (DeFrancesco et al. Citation2017). Indeed, biofilms can be reduced by adding DNase I exogenously, indicating the importance of eDNA in biofilm formation. Additionally, the CidA was indicated to positively increase the release of DNA during the development of a biofilm (Mann et al. Citation2009). Thus, eDNA release, or CidA, can be seen as a target for anti-biofilm agent screening (DeFrancesco et al. Citation2017). Some promising plant-derived compounds, including licochalcone A and emodin, were discovered to inhibit or disturb preformed biofilms by suppressing cidA, as shown in (Shen et al. Citation2015; Yan et al. Citation2017). Although they have not been approved for use as food additives, their high inhibitory activity at low doses shows their potential for development as antibiofilm agents in the food industry.
Strategy against extracellular proteins
Considerable amounts of extracellular proteins within the biofilm matrix, including enzymes and nonenzymatic proteins, can far exceed the polysaccharide content. Numerous extracellular enzymes in biofilms can break down biopolymers to low-molecular-mass products and promote the detachment of bacteria from biofilms. The serine proteases SspA and SplA-F, cysteine proteases SspB and ScpA and metalloprotease Aur promote biofilm dispersal in S. aureus mediated by the global regulators SarA and SigB (Lister and Horswill Citation2014).
Nonenzymatic proteins, consisting of cell surface proteins and extracellular carbohydrate-binding proteins (biofilm-associated surface protein Bap, surface protein G SasG, fibronectin binding proteins FnBPs, clumping factor ClfA, enolase, etc.), are involved in the formation and stabilization of the biofilm matrix network and contribute to connecting the bacterial surface with extracellular EPS related to biofilm formation contribute to the attachment of bacterial cells on different surfaces. Natural products, such as emodin from P. cuspidatum, suppress biofilm formation by inhibiting these surface adhesion proteins, as shown in .
Target quorum sensing involved in dispersal stage
The quorum sensing (QS) system participates in bacterial cell-to-cell communication behavior, including biofilm formation. Thus, it is regarded as an important target for the development of antibiofilm agents. Interference with the QS system in S. aureus was achieved in two ways: disturbance of the Agr/AIP system and of the LuxS/AI-2 system, which are involved in intraspecific and interspecies communication, respectively.
Disrupting the agr/AIP system
In response to autoinducer peptide (AIP) signaling, the agr system regulates cell communication and virulence secretion via a series of cascading reactions. Briefly, AIP is first processed and secreted by the precursor peptide AgrD and integral membrane endopeptidase AgrB. Subsequently, extracellular AIP binds to the cognate transmembrane-bound receptor histidine kinase AgrC and promotes the phosphorylation of AgrA (). Agr quorum-sensing activation leads to the production of enzyme proteins such as proteases, lipases, and nucleases that participate in the biofilm dispersal process. AgrA also directly activates the transcription of the psmα and psmβ genes, which encode phenol-soluble modulins (PSMs) that participate in biofilm dispersal (Horswill and Gordon Citation2020; Kim et al. Citation2017; Boles and Horswill Citation2008). From this perspective, activation of the agr system might be considered a more attractive strategy for biofilm inhibition (Horswill and Gordon Citation2020). However, most efforts to date have concentrated on the development of quorum inhibitors to reduce virulence because agr can positively increase virulence factors, such as enterotoxin and hemolysin secretion (Wang and Muir Citation2016). It is a challenge to precisely manipulate the Agr quorum sensing system to inhibit biofilm formation while not increasing virulence. This factor hinders the development of biofilm inhibitors targeting the agr quorum for S. aureus.
Figure 4. Mechanism of quorum sensing system (QS) Agr/AIP for biofilm regulation in S. aureus. The precursor of autoinducer peptide (AIP) is produced in the cytoplasm mediated by AgrD, Subsequently, AgrB is involved in the externalization of mature AIP. AgrC-AgrA two component system is activated at an AIP threshold concentration. Phosphorylated AgrA activates transcription of RNAII and RNAIII. The RNAIII inhibits biofilm by down- regulating adhesion surface proteins. AgrA increases transcription of the psmα and psmβ operons, encoding PSM peptides which improve biofilm dispersion. Key molecule can be seen as antibiofilm and antibacterial targets, encircled in red (Xie et al. Citation2019; Srivastava et al. Citation2014; Nicod et al. Citation2014).
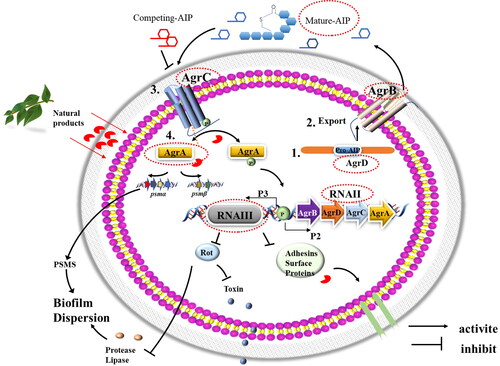
Currently, the activation of agr has been proposed as a biofilm dispersal promoting strategy (Wang and Muir Citation2016). The first identified agr activation agents are the AIPs themselves. Activation of agr through AIP addition in established biofilms can trigger biofilm dispersal (Boles and Horswill Citation2008). The AIPs produced by one strain can cross-inhibit a strain producing a different agr class, which are competing AIPs (Cech and Horswill Citation2013). AIPs may also be regarded as potential targets; however, their roles in biofilm formation still need to be determined. Furthermore, Xie et al. reported that the activation of AgrC involves disruption of an intrasteric inhibitory docking interaction in the AgrC dimer, which provided a potential mechanistic strategy for inhibiting biofilms via the activation of AgrC (Xie et al. Citation2019). Qin et al proposed that resveratrol can enhance MRSA agr function at the RNA level to inhibit biofilm formation (Qin et al. Citation2014). Brazilin isolated from Caesalpinia sappan L inhibits S. aureus biofilm formation (32 µg/mL, 60% inhibition rate) by upregulating AgrA mRNA expression, as shown in . In contrast, some natural products have been found to have antibiofilm activity by downregulating the quorum-sensing system regulator AgrA in S. aureus (Sharifi et al. Citation2018; Chen et al. Citation2016). The results contradict the mechanism discussed above. This is possibly due to the complicated regulatory network involved in the multifactor response to biofilm removal or inhibition.
Table 3. Natural products screening for inhibiting biofilm formation or removing pre-formed biofilm targeted QS system.
Interfering with LuxS/AI-2
The interspecies autoinducer AI-2 synthesized by the LuxS enzyme, which is involved in QS sensing, participates in interspecies cell communication, including biofilm formation. In Vibrio harveyi, Escherichia coli and Salmonella typhimurium, the pathways involved in AI-2 receptors and the transduction process have been well researched (Rajamani and Sayre Citation2011; Chen et al. Citation2002), but the mechanism of AI-2-mediated signal transduction in S. aureus remains inadequate. The inactivation of LuxS has been identified in S. aureus to result in increased biofilm formation and PIA production. The LuxS/AI-2 system regulates biofilm formation by modulating the transcriptional regulation of the ica locus in S. aureus (Ma et al. Citation2017; Rowe et al. Citation2016) (). Cluzel et al. first demonstrated an AI-2-producing enzyme regulated by Ser/Thr phosphorylation (Cluzel et al. Citation2010). This has been seen as a potential strategy for perturbing AI-2 signaling for anti-biofilm and anti-virulence agent development (M. Guo et al. Citation2013). Natural products, such as surfactin of microbial origin, were identified as antibiofilm compounds targeted LuxS/AI-2 QS system with increased AI-2 activity. The 70% inhibition was achieved at 1/2 MIC (16 μg/mL), and 90% removal was achieved for the established biofilm after just 4 hours at 2 mg/mL (). Although the mechanism has not been clarified, the LuxS/AI-2 QS system can be a hypothetical competitive target due to its important role in intercellular communication.
Figure 5. Mechanism of quorum sensing system (QS) LuxS/AI-2 for biofilm regulation in S. aureus. Biosynthesis of Autoinducer 2 (AI-2) is catalyzed by LuxS and repressed the expression of rbf. Rbf could bind to the SarX and rbf promoters to upregulate their expression. Rbf can improves PIA-dependent biofilm formation via the repression of icaR expression. Polysaccharide intercellular adhesin (PIA) is synthesized regulated via proteins IcaA, IcaD, IcaB, and IcaC, encoded within the intercellular adhesin (ica) operon. IcaA and IcaD synergistically synthesize UDP-N-acetylglucosamine and exported via IcaC. Subsequently, IcaB regulates the partial deacetylation of PIA, improving adhesion via increased positive charge. Key molecule can be seen as antibiofilm and antibacterial targets, encircled in red.
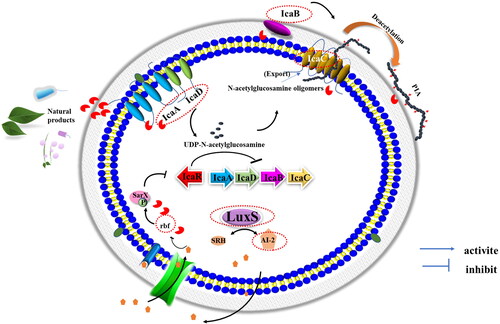
The QS system is the key regulator of dispersal in biofilms by S. aureus. Biofilm dispersion, which is the last step in the biofilm formation process, is no less critical than the previous steps. At this stage, planktonic cells detach from biofilms and dissolve in neighboring spaces, determining the beginning of a new biofilm and becoming a new source for food contamination (Alonso and Kabuki Citation2019). Increased antibiotic susceptibility has been observed with the occurrence of the dissociation of the three-dimensional structure of the biofilm. Thus, several studies have proposed that the induction of biofilm dispersal may be a promising strategy (Landini et al. Citation2010; Lister and Horswill Citation2014). However, there are opposite voices. If biofilms accumulate on the contact surfaces of food packaging or equipment, induced dispersal could result in cross infections if the antibacterial agent fails to eradicate the released cells, and bacterial cells can detach from the surfaces, increasing the microbial load on the food product (Rossi et al. Citation2020). Although targeting QS system is a promising strategy, studies will need to address these challenges.
As depicted in and , a number of natural products ((+)-nootkatone, tea tree oil, emodin and myricetin) target SrtA, SarA and EPS substrates simultaneously. In addition, natural products carvacrol and surfactin extracted from Bacillus subtilis () disturb QS system while reducing EPS production. Indeed, it is a coherent process for biofilm formation from the initial adhesion stage to the dissociation stage which involves complex pathway crosstalk. Multi-target compounds screening is an effective strategy for potent anti-biofilm agents discovery. Furthermore, according to , most natural compounds that target EPS (e.g. tea tree oil, essential oils of Pogostemon heyneanus, Baicalein, Carvacrol, etc.) are found to exhibit not only high biofilm formation inhibitory/prevention activity at sub-MIC concentrations but also well pre-formed biofilm removal activity at higher concentrations. However, few natural products target adhesin proteins show biofilm removal activity, as shown in Table1. Possibly because targeting EPS substrates is more conducive to break up the stable matrix facilitating the removal of mature biofilm. Moreover, essential oils from plants (e.g. tea tree oil, essential oils of Pogostemon heyneanus, Thymus daenensis, Eucalyptus globulus, etc.) () all show biofilm inhibitory and scavenging activity which may be due to the synergy result from interactions between various components. Obviously, these products can serve as potential candidates of promising anti-biofilm agents.
Conclusions
In this review, most natural products have the ability to prevent biofilm formation at sub-MIC concentrations. This fact highlights that the capability of natural products to inhibit biofilms is linked to mechanisms other than growth inhibition. Indeed, an ideal antibiofilm inhibitor is expected to exert no pressure on bacterial growth to develop resistance. In addition, appropriate dosages and contact times are important for avoiding the overuse of antibiofilm agents that could be toxic to the end consumer. Thus, the present review summarizes useful information on the mechanisms, targets and dosage of natural compounds against biofilms.
The development of natural products urgently requires further exploration of their antibiofilm mechanism. Alternatively, to improve the potency and avoid negative effects, structural optimization of natural products is required. In view of drug resistance alleviation and increasing efficiency, the combination of different natural products or multitarget screening are promising strategy to inhibit or eradicate S. aureus biofilms. Within this review, many natural products against S. aureus biofilms have been discovered by targeting various substrates which involve in biofilm regulation. Overall, this review provides valuable information on promising targets for screening anti-biofilm agents. In addition, these natural products provide attractive candidates for the application and development of anti-biofilm agents against S. aureus in the food industry.
Disclosure statement
No potential conflict of interest was reported by the authors.
Additional information
Funding
References
- Ali, S. S., M. S. Moawad, M. A. Hussein, M. Azab, E. A. Abdelkarim, A. Badr, J. Sun, and M. Khalil. 2021. Efficacy of metal oxide nanoparticles as novel antimicrobial agents against multi-drug and multi-virulent Staphylococcus aureus isolates from retail raw chicken meat and giblets. International Journal of Food Microbiology 344:109116. doi: 10.1016/j.ijfoodmicro.2021.109116.
- Alonso, V. P. P., A. M. M. Harada, and D. Y. Kabuki. 2020. Competitive and/or Cooperative interactions of Listeria monocytogenes with Bacillus cereus in dual-Species Biofilm formation. Frontiers in Microbiology 11:177. doi: 10.3389/fmicb.2020.00177.
- Alonso, V. P. P., and D. Y. Kabuki. 2019. Formation and dispersal of biofilms in dairy substrates. International Journal of Dairy Technology 72 (3):472–8. doi: 10.1111/1471-0307.12587.
- Al-Shabib, N. A., F. M. Husain, I. Ahmad, and M. H. Baig. 2017a. Eugenol inhibits quorum sensing and biofilm of toxigenic MRSA strains isolated from food handlers employed in Saudi Arabia. Biotechnology & Biotechnological Equipment 31 (2):387–96. doi: 10.1080/13102818.2017.1281761.
- Al-Shabib, N. A., F. M. Husain, I. Ahmad, M. S. Khan, R. A. Khan, and J. M. Khan. 2017b. Rutin inhibits mono and multi-species biofilm formation by foodborne drug resistant Escherichia coli and Staphylococcus aureus. Food Control 79:325–32. doi: 10.1016/j.foodcont.2017.03.004.
- Archer, N. K., M. J. Mazaitis, J. W. Costerton, J. G. Leid, M. E. Powers, and M. E. Shirtliff. 2011. Staphylococcus aureus biofilms: Properties, regulation, and roles in human disease. Virulence 2 (5):445–59. doi: 10.4161/viru.2.5.17724.
- Arciola, C. R., D. Campoccia, P. Speziale, L. Montanaro, and J. W. Costerton. 2012. Biofilm formation in Staphylococcus implant infections. A review of molecular mechanisms and implications for biofilm-resistant materials. Biomaterials 33 (26):5967–82. doi: 10.1016/j.biomaterials.2012.05.031.
- Avila-Novoa, M. G., M. Iñíguez-Moreno, O. A. Solís-Velázquez, J. P. González-Gómez, P. J. Guerrero-Medina, and M. Gutiérrez-Lomelí. 2018. Biofilm Formation by Staphylococcus aureus isolated from food contact surfaces in the dairy industry of Jalisco. Journal of Food Quality 2018:1–8. doi: 10.1155/2018/1746139.
- Basanisi, M. G., G. La Bella, G. Nobili, I. Franconieri, and G. La Salandra. 2017. Genotyping of methicillin-resistant Staphylococcus aureus (MRSA) isolated from milk and dairy products in South Italy. Food Microbiology 62:141–6. doi: 10.1016/j.fm.2016.10.020.
- Bazargani, M. M., and J. Rohloff. 2016. Antibiofilm activity of essential oils and plant extracts against Staphylococcus aureus and Escherichia coli biofilms. Food Control 61:156–64. doi: 10.1016/j.foodcont.2015.09.036.
- Boles, B. R., and A. R. Horswill. 2008. Agr-mediated dispersal of Staphylococcus aureus biofilms. PLoS Pathogens 4 (4):e1000052. doi: 10.1371/journal.ppat.1000052.
- Burt, S. A., V. T. A. Ojo-Fakunle, J. Woertman, and E. J. A. Veldhuizen. 2014. The natural antimicrobial carvacrol inhibits quorum sensing in chromobacterium violaceum and reduces bacterial biofilm formation at sub-lethal concentrations. PLoS One. 9 (4):e93414. doi: 10.1371/journal.pone.0093414.
- Carvalho, L. G., M. M. A. Alvim, R. L. Fabri, and A. C. M. Apolônio. 2021. Staphylococcus aureus biofilm formation in Minas Frescal cheese packaging. International Journal of Dairy Technology 74 (3):575–80. doi: 10.1111/1471-0307.12783.
- Carvalho, J. S., A. F. L. Neto, I. M. Melo, L. M. Varjão, C. A. D. N. Andrade, D. E. Xavier, N. C. Leal, and R. C. De Castro Almeida. 2020. Occurrence of methicillin-resistant Staphylococcus aureus in ready-to-eat raw fish from Japanese Cuisine Restaurants in Salvador, Brazil. Journal of Food Protection 83 (6):991–5. doi: 10.4315/0362-028X.JFP-19-375.
- Cattò, C., and F. Cappitelli. 2019. Testing anti-biofilm polymeric surfaces: Where to start?International Journal of Molecular Sciences 20 (15):3794–854. doi: 10.3390/ijms20153794.
- Cech, N. B., and A. R. Horswill. 2013. Small-molecule quorum quenchers to prevent Staphylococcus aureus infection. Future Microbiology 8 (12):1511–4. doi: 10.2217/fmb.13.134.
- Chen, Y., T. Liu, K. Wang, C. Hou, S. Cai, Y. Huang, Z. Du, H. Huang, J. Kong, and Y. Chen. 2016. Baicalein inhibits Staphylococcus aureus biofilm formation and the quorum sensing system in vitro. PLoS ONE 11 (4):e0153468. doi: 10.1371/journal.pone.0153468.
- Chen, X., S. Schauder, N. Potier, A. Van Dorsselaer, I. Pelczer, B. L. Bassler, and F. M. Hughson. 2002. Structural identification of a bacterial quorum-sensing signal containing boron. Nature 415 (6871):545–9. doi: 10.1038/415545a.
- Chen, X., L. Zhang, M. Zhang, H. Liu, P. Lu, and K. Lin. 2018. Quorum sensing inhibitors: A patent review (2014-2018). ). Expert Opinion on Therapeutic Patents 28 (12):849–65. doi: 10.1080/13543776.2018.1541174.
- Cluzel, M. E., I. Zanella-Cléon, A. J. Cozzone, K. Fütterer, B. Duclos, and V. Molle. 2010. The Staphylococcus aureus autoinducer-2 synthase LuxS is regulated by Ser/Thr phosphorylation. Journal of Bacteriology 192 (23):6295–301. doi: 10.1128/JB.00853-10.
- Dai, J., S. Wu, J. Huang, Q. Wu, F. Zhang, J. Zhang, J. Wang, Y. Ding, S. Zhang, X. Yang, et al. 2019. Prevalence and characterization of Staphylococcus aureus isolated from pasteurized milk in China. Frontiers in Microbiology 10 (641):1–10. doi: 10.3389/fmicb.2019.00641.
- de Souza, E. L., Q. G. S. Meira, I. de, M. Barbosa, A. J. A. A. Athayde, M. L. da Conceição, and J. P. de Siqueira. 2014. Biofilm formation by Staphylococcus aureus from food contact surfaces in a meat-based broth and sensitivity to sanitizers. Brazilian Journal of Microbiology 45 (1):67–75. doi: 10.1590/s1517-83822014000100010.
- DeFrancesco, A. S., N. Masloboeva, A. K. Syed, A. DeLoughery, N. Bradshaw, G. W. Li, M. S. Gilmore, S. Walker, and R. Losick. 2017. Genome-wide screen for genes involved in eDNA release during biofilm formation by Staphylococcus aureus. Proceedings of the National Academy of Sciences of the United States of America 114 (29):E5969–E5978. doi: 10.1073/pnas.1704544114.
- Dong, G., H. Liu, X. Yu, X. Zhang, H. Lu, T. Zhou, and J. Cao. 2018. Antimicrobial and anti-biofilm activity of tannic acid against Staphylococcus aureus. Natural Product Research 32 (18):2225–8. doi: 10.1080/14786419.2017.1366485.
- Dutra, T. V., M. d S. Fernandes, M. R. F. G. Perdoncini, M. M. dos Anjos, and B. A. d Abreu Filho. 2018. Capacity of Escherichia coli and Staphylococcus aureus to produce biofilm on stainless steel surfaces in the presence of food residues. Journal of Food Processing and Preservation 42 (4):e13574. doi: 10.1111/jfpp.13574.
- Elmasri, W. A., T. Yang, P. Tran, M. E. F. Hegazy, A. N. Hamood, Y. Mechref, and P. W. Paré. 2015. Teucrium polium phenylethanol and iridoid glycoside characterization and flavonoid inhibition of biofilm-forming staphylococcus aureus. Journal of Natural Products 78 (1):2–9. doi: 10.1021/np5004092.
- Espina, L., D. Berdejo, P. Alfonso, D. García-Gonzalo, and R. Pagán. 2017. Potential use of carvacrol and citral to inactivate biofilm cells and eliminate biofouling. Food Control. 82:256–65. doi: 10.1016/j.foodcont.2017.07.007.
- Farha, A. K., Q. Q. Yang, G. Kim, D. Zhang, V. Mavumengwana, O. Habimana, H. Bin Li, H. Corke, and R. Y. Gan. 2020. Inhibition of multidrug-resistant foodborne Staphylococcus aureus biofilms by a natural terpenoid (+)-nootkatone and related molecular mechanism. Food Control. 112:107154. doi: 10.1016/j.foodcont.2020.107154.
- Gao, C., I. Uzelac, J. Gottfries, and L. A. Eriksson. 2016. Exploration of multiple sortase A protein conformations in virtual screening. Scientific Reports 6 (1):20413. doi: 10.1038/srep20413.
- Guo, M., S. Gamby, Y. Zheng, and H. O. Sintim. 2013. Small molecule inhibitors of AI-2 signaling in bacteria: State-of-the-art and future perspectives for anti-quorum sensing agents. International Journal of Molecular Sciences 14 (9):17694–728. doi: 10.3390/ijms140917694.
- Guo, L., Y. Wang, X. Bi, K. Duo, Q. Sun, X. Yun, Y. Zhang, P. Fei, and J. Han. 2020. Antimicrobial activity and mechanism of action of the Amaranthus tricolor crude Extract against Staphylococcus aureus and potential application in cooked meat. Foods 9 (3):359. doi: 10.3390/foods9030359.
- Guo, N., X. Zhao, W. Li, C. Shi, R. Meng, Z. Liu, and L. Yu. 2015. The synergy of berberine chloride and totarol against Staphylococcus aureus grown in planktonic and biofilm cultures. Journal of Medical Microbiology 64 (8):891–900. doi: 10.1099/jmm.0.000106.
- Horswill, A. R., and C. P. Gordon. 2020. Structure-activity relationship studies of small molecule modulators of the staphylococcal accessory gene regulator. Journal of Medicinal Chemistry 63 (6):2705–30. doi: 10.1021/acs.jmedchem.9b00798.
- Jabra-Rizk, M. A., M. Shirtliff, C. James, and T. Meiller. 2006. Effect of farnesol on Candida dubliniensis biofilm formation and fluconazole resistance. FEMS Yeast Research 6 (7):1063–73. doi: 10.1111/j.1567-1364.2006.00121.x.
- Kang, J., W. Jin, J. Wang, Y. Sun, X. Wu, and L. Liu. 2019. Antibacterial and anti-biofilm activities of peppermint essential oil against Staphylococcus aureus. LWT 101:639–45. doi: 10.1016/j.lwt.2018.11.093.
- Kang, S., F. Kong, X. Shi, H. Han, M. Li, B. Guan, M. Yang, X. Cao, D. Tao, Y. Zheng, et al. 2020. Antibacterial activity and mechanism of lactobionic acid against Pseudomonas fluorescens and Methicillin-resistant Staphylococcus aureus and its application on whole milk. Food Control. 108:106876. doi: 10.1016/j.foodcont.2019.106876.
- Kim, M. K., A. Zhao, A. Wang, Z. Z. Brown, T. W. Muir, H. A. Stone, and B. L. Bassler. 2017. Surface-attached molecules control Staphylococcus aureus quorum sensing and biofilm development. Nature Microbiology 2 (8):17080. doi: 10.1038/nmicrobiol.2017.80.
- Kot, B., H. Sytykiewicz, I. Sprawka, and M. Witeska. 2020. Effect of trans-Cinnamaldehyde on methicillin-resistant Staphylococcus Aureus biofilm formation: Metabolic activity assessment and analysis of the biofilm-associated genes expression. International Journal of Molecular Sciences 21 (1):102–16. doi: 10.3390/ijms21010102.
- Landini, P., D. Antoniani, J. G. Burgess, and R. Nijland. 2010. Molecular mechanisms of compounds affecting bacterial biofilm formation and dispersal. Applied Microbiology and Biotechnology 86 (3):813–23. doi: 10.1007/s00253-010-2468-8.
- Lemos, A. S. O., L. M. Campos, L. Melo, M. C. M. R. Guedes, L. G. Oliveira, T. P. Silva, R. C. N. Melo, V. N. Rocha, J. A. K. Aguiar, A. C. M. Apolônio, et al. 2018. Antibacterial and antibiofilm activities of psychorubrin, a pyranonaphthoquinone isolated from Mitracarpus frigidus (Rubiaceae). Frontiers in Microbiology 9:724–35. doi: 10.3389/fmicb.2018.00724.
- Li, W. L., X. C. Zhao, Z. W. Zhao, Y. J. Huang, X. Z. Zhu, R. Z. Meng, C. Shi, L. Yu, and N. Guo. 2016. In vitro antimicrobial activity of honokiol against Staphylococcus aureus in biofilm mode. Journal of Asian Natural Products Research 18 (12):1178–85. doi: 10.1080/10286020.2016.1194829.
- Liang, Z., Y. Qi, S. Guo, K. Hao, M. Zhao, and N. Guo. 2019. Effect of AgWPA nanoparticles on the inhibition of Staphylococcus aureus growth in biofilms. Food Control 100:240–6. doi: 10.1016/j.foodcont.2019.01.030.
- Lin, M. H., F. R. Chang, M. Y. Hua, Y. C. Wu, and S. T. Liu. 2011. Inhibitory effects of 1,2,3,4,6-penta-O-galloyl-beta-D-glucopyranose on biofilm formation by Staphylococcus aureus. Antimicrobial Agents and Chemotherapy 55 (3):1021–7. doi: 10.1128/AAC.00843-10.
- Lin, Q., H. Sun, K. Yao, J. Cai, Y. Ren, and Y. Chi. 2019. The Prevalence, Antibiotic resistance and biofilm formation of Staphylococcus aureus in bulk ready-to-eat foods. Biomolecules 9 (10):524–35. doi: 10.3390/biom9100524.
- Lister, J. L., and A. R. Horswill. 2014. Staphylococcus aureus biofilms: Recent developments in biofilm dispersal. Frontiers in Cellular and Infection Microbiology 4:178–87. doi: 10.3389/fcimb.2014.00178.
- Liu, J., W. Li, X. Zhu, H. Zhao, Y. Lu, C. Zhang, and Z. Lu. 2019. Surfactin effectively inhibits Staphylococcus aureus adhesion and biofilm formation on surfaces. Applied Microbiology and Biotechnology 103 (11):4565–74. doi: 10.1007/s00253-019-09808-w.
- Liu, T., J. Wang, X. Gong, X. Wu, L. Liu, and F. Chi. 2020. Rosemary and tea tree essential oils exert antibiofilm activities in vitro against staphylococcus aureus and Escherichia coli. Journal of Food Protection 83 (7):1261–7. doi: 10.4315/0362-028X.JFP-19-337.
- Liu, M., X. Wu, J. Li, L. Liu, R. Zhang, D. Shao, and X. Du. 2017. The specific anti-biofilm effect of gallic acid on Staphylococcus aureus by regulating the expression of the ica operon. Food Control. 73:613–8. doi: 10.1016/j.foodcont.2016.09.015.
- Liu, X., Y. Yue, Y. Wu, K. Zhong, Q. Bu, and H. Gao. 2021. Discovering the antibacterial mode of action of 3-p-trans-coumaroyl-2-hydroxyquinic acid, a natural phenolic compound, against Staphylococcus aureus through an integrated transcriptomic and proteomic approach. Journal of Food Safety 41 (1):1–11. doi: 10.1111/jfs.12861.
- Llorens, A., E. Lloret, P. A. Picouet, R. Trbojevich, and A. Fernandez. 2012. Metallic-based micro and nanocomposites in food contact materials and active food packaging. Trends in Food Science & Technology 24 (1):19–29. doi: 10.1016/j.tifs.2011.10.001.
- Lopes, L. A. A., J. B. dos Santos Rodrigues, M. Magnani, E. L. de Souza, and J. P. de Siqueira-Júnior. 2017. Inhibitory effects of flavonoids on biofilm formation by Staphylococcus aureus that overexpresses efflux protein genes. Microbial Pathogenesis 107:193–7. doi: 10.1016/j.micpath.2017.03.033.
- Ma, R., S. Qiu, Q. Jiang, H. Sun, T. Xue, G. Cai, and B. Sun. 2017. AI-2 quorum sensing negatively regulates rbf expression and biofilm formation in Staphylococcus aureus. International Journal of Medical Microbiology 307 (4–5):257–67. doi: 10.1016/j.ijmm.2017.03.003.
- Mann, E. E., K. C. Rice, B. R. Boles, J. L. Endres, D. Ranjit, L. Chandramohan, L. H. Tsang, M. S. Smeltzer, A. R. Horswill, and K. W. Bayles. 2009. Modulation of eDNA release and degradation affects Staphylococcus aureus biofilm maturation. PLoS ONE 4 (6):e5822. doi: 10.1371/journal.pone.0005822.
- Maresso, A. W., R. Wu, J. W. Kern, R. Zhang, D. Janik, D. M. Missiakas, M. E. Duban, A. Joachimiak, and O. Schneewind. 2007. Activation of inhibitors by sortase triggers irreversible modification of the active site. The Journal of Biological Chemistry 282 (32):23129–39. doi: 10.1074/jbc.M701857200.
- Meesilp, N., and N. Mesil. 2019. Effect of microbial sanitizers for reducing biofilm formation of Staphylococcus aureus and Pseudomonas aeruginosa on stainless steel by cultivation with UHT milk. Food Science and Biotechnology 28 (1):289–96. doi: 10.1007/s10068-018-0448-4.
- Merghni, A., E. Noumi, O. Hadded, N. Dridi, H. Panwar, O. Ceylan, M. Mastouri, and M. Snoussi. 2018. Assessment of the antibiofilm and antiquorum sensing activities of Eucalyptus globulus essential oil and its main component 1,8-cineole against methicillin-resistant Staphylococcus aureus strains. Microbial Pathogenesis 118:74–80. doi: 10.1016/j.micpath.2018.03.006.
- Miao, J., Y. Liang, L. Chen, W. Wang, J. Wang, B. Li, L. Li, D. Chen, and Z. Xu. 2017. Formation and development of Staphylococcus biofilm: With focus on food safety. Journal of Food Safety 37 (4):1–12. doi: 10.1111/jfs.12358.
- Ming, D., D. Wang, F. Cao, H. Xiang, D. Mu, J. Cao, B. Li, L. Zhong, X. Dong, X. Zhong, et al. 2017. Kaempferol inhibits the primary attachment phase of biofilm formation in Staphylococcus aureus. Frontiers in Microbiology 8:2263–74. doi: 10.3389/fmicb.2017.02263.
- Mu, D., Y. Luan, L. Wang, Z. Gao, P. Yang, S. Jing, Y. Wang, H. Xiang, T. Wang, and D. Wang. 2020. The combination of salvianolic acid A with latamoxef completely protects mice against lethal pneumonia caused by methicillin-resistant Staphylococcus aureus. Emerging Microbes & Infections 9 (1):169–79. doi: 10.1080/22221751.2020.1711817.
- Mu, D., H. Xiang, H. Dong, D. Wang, and T. Wang. 2018. Isovitexin, a potential candidate inhibitor of sortase a of staphylococcus aureus USA300. Journal of Microbiology and Biotechnology 28 (9):1426–32. doi: 10.4014/jmb.1802.02014.
- Nelson, J. W., A. G. Chamessian, P. J. McEnaney, R. P. Murelli, B. I. Kazmierczak, B. I. Kazmiercak, and D. A. Spiegel. 2010. A Biosynthetic strategy for re-engineering the Staphylococcus aureus cell wall with non-native small molecules. ACS Chemical Biology 5 (12):1147–55. doi: 10.1021/cb100195d.
- Nicod, S. S., R. O. J. Weinzierl, L. Burchell, A. Escalera-Maurer, E. H. James, and S. Wigneshweraraj. 2014. Systematic mutational analysis of the LytTR DNA binding domain of Staphylococcus aureus virulence gene transcription factor agrA. Nucleic Acids Research 42 (20):12523–36. doi: 10.1093/nar/gku1015.
- Nitulescu, G., D. P. Mihai, I. M. Nicorescu, O. T. Olaru, A. Ungurianu, A. Zanfirescu, G. M. Nitulescu, and D. Margina. 2019. Discovery of natural naphthoquinones as sortase A inhibitors and potential anti-infective solutions against Staphylococcus aureus. Drug Development Research 80 (8):1136–45. doi: 10.1002/ddr.21599.
- Nitulescu, G., I. M. Nicorescu, O. T. Olaru, A. Ungurianu, D. P. Mihai, A. Zanfirescu, G. M. Nitulescu, and D. Margina. 2017. Molecular docking and screening studies of new natural sortase A inhibitors. International Journal of Molecular Sciences 18 (10):2217–32. doi: 10.3390/ijms18102217.
- Nitulescu, G., A. Zanfirescu, O. T. Olaru, I. M. Nicorescu, G. M. Nitulescu, and D. Margina. 2016. Structural analysis of sortase A inhibitors. Molecules 21 (11):1591–601. doi: 10.3390/molecules21111591.
- Oliveira, A. P. D. d., M. M. da Costa, D. M. Nogueira, and F. S. Dias. 2020. Characterisation of Staphylococcus Aureus strains from Milk and Goat Cheese and evaluation of their inhibition by gallic acid, nisin and velame of the Brazilian caatinga. International Journal of Dairy Technology 73 (2):345–56. doi: 10.1111/1471-0307.12673.
- Ou, C., D. Shang, J. Yang, B. Chen, J. Chang, F. Jin, and C. Shi. 2020. Prevalence of multidrug-resistant Staphylococcus aureus isolates with strong biofilm formation ability among animal-based food in Shanghai. Food Control. 112:107106. doi: 10.1016/j.foodcont.2020.107106.
- Ouyang, P., X. He, Z. W. Yuan, Z. Q. Yin, H. Fu, J. Lin, C. He, X. Liang, C. Lv, G. Shu, et al. 2018. Erianin against staphylococcus aureus infection via inhibiting sortase A. Toxins 10 (10):385–99. doi: 10.3390/toxins10100385.
- Parai, D., E. Islam, J. Mitra, and S. K. Mukherjee. 2017. Effect of Bacoside A on growth and biofilm formation by Staphylococcus aureus and Pseudomonas aeruginosa. Canadian Journal of Microbiology 63 (2):169–78. doi: 10.1139/cjm-2016-0365.
- Park, J. H., J. H. Lee, C. J. Kim, J. C. Lee, M. H. Cho, and J. Lee. 2012. Extracellular protease in actinomycetes culture supernatants inhibits and detaches Staphylococcus aureus biofilm formation. Biotechnology Letters 34 (4):655–61. doi: 10.1007/s10529-011-0825-z.
- Peng, D., A. Chen, B. Shi, X. Min, T. Zhang, Z. Dong, H. Yang, X. Chen, Y. Tian, and Z. Chen. 2018. Preliminary study on the effect of Brazilin on biofilms of Staphylococcus aureus. Experimental and Therapeutic Medicine 16 (3):2108–18. doi: 10.3892/etm.2018.6403.
- Qin, N., X. Tan, Y. Jiao, L. Liu, W. Zhao, S. Yang, and A. Jia. 2014. RNA-Seq-based transcriptome analysis of methicillin-resistant staphylococcus aureus biofilm inhibition by ursolic acid and resveratrol. Scientific Reports 4 (1):5467. doi: 10.1038/srep05467.
- Rajamani, S., and R. T. Sayre. 2011. A sensitive fluorescence reporter for monitoring quorum sensing regulated protease production in Vibrio harveyi. Journal of Microbiological Methods 84 (2):189–93. doi: 10.1016/j.mimet.2010.11.017.
- Rossi, C., C. Chaves-López, A. Serio, M. Casaccia, F. Maggio, and A. Paparella. 2020. Effectiveness and mechanisms of essential oils for biofilm control on food-contact surfaces: An updated review. Critical Reviews in Food Science and Nutrition Advance online publication. doi: 10.1080/10408398.2020.1851169.
- Rowe, S. E., C. Campbell, C. Lowry, S. T. O’Donnell, M. E. Olson, J. K. Lindgren, E. M. Waters, P. D. Fey, and J. P. O’Gara. 2016. AraC-type regulator Rbf controls the Staphylococcus epidermidis biofilm phenotype by negatively regulating the icaADBC repressor SarR. Journal of Bacteriology 198 (21):2914–24. doi: 10.1128/JB.00374-16.
- Rubini, D., S. F. Banu, B. N. Veda Hari, D. Ramya Devi, S. Gowrishankar, S. Karutha Pandian, and P. Nithyanand. 2018a. Chitosan extracted from marine biowaste mitigates staphyloxanthin production and biofilms of Methicillin- resistant Staphylococcus aureus. Food and Chemical Toxicology 118:733–44. doi: 10.1016/j.fct.2018.06.017.
- Rubini, D., S. F. Banu, P. Nisha, R. Murugan, S. Thamotharan, M. J. Percino, P. Subramani, and P. Nithyanand. 2018b. Essential oils from unexplored aromatic plants quench biofilm formation and virulence of Methicillin resistant Staphylococcus aureus. Microbial Pathogenesis 122:162–73. doi: 10.1016/j.micpath.2018.06.028.
- Sayed, A. M., H. A. Alhadrami, S. S. El‐Hawary, R. Mohammed, H. M. Hassan, M. E. Rateb, U. R. Abdelmohsen, and W. Bakeer. 2020. Discovery of two brominated oxindole alkaloids as staphylococcal DNA gyrase and pyruvate kinase inhibitors via inverse virtual screening. Microorganisms 8 (2):293–307. doi: 10.3390/microorganisms8020293.
- Sharifi, A., A. Mohammadzadeh, T. Z. Salehi, and P. Mahmoodi. 2018. Antibacterial, antibiofilm and antiquorum sensing effects of Thymus daenensis and Satureja hortensis essential oils against Staphylococcus aureus isolates. Journal of Applied Microbiology 124 (2):379–88. doi: 10.1111/jam.13639.
- Shen, F., X. Tang, Y. Wang, Z. Yang, X. Shi, C. Wang, Q. Zhang, Y. An, W. Cheng, K. Jin, et al. 2015. Phenotype and expression profile analysis of Staphylococcus aureus biofilms and planktonic cells in response to licochalcone A. Applied Microbiology and Biotechnology 99 (1):359–73. doi: 10.1007/s00253-014-6076-x.
- Silva, L. N., G. C. A. Da Hora, T. A. Soares, M. S. Bojer, H. Ingmer, A. J. Macedo, and D. S. Trentin. 2017. Myricetin protects Galleria mellonella against Staphylococcus aureus infection and inhibits multiple virulence factors. Scientific Reports 7 (1):2823–49. doi: 10.1038/s41598-017-02712-1.
- Simões, M., L. C. Simões, and M. J. Vieira. 2010. A review of current and emergent biofilm control strategies. LWT—Food Science and Technology 43 (4):573–83. doi: 10.1016/j.lwt.2009.12.008.
- Srivastava, S. K., K. Rajasree, A. Fasim, G. Arakere, and B. Gopal. 2014. Influence of the AgrC-AgrA complex on the response time of Staphylococcus aureus quorum sensing. Journal of Bacteriology 196 (15):2876–88. doi: 10.1128/JB.01530-14.
- Sullivan, D. J., S. Azlin-Hasim, M. Cruz-Romero, E. Cummins, J. P. Kerry, and M. A. Morris. 2020. Antimicrobial effect of benzoic and sorbic acid salts and nano-solubilisates against Staphylococcus aureus, Pseudomonas fluorescens and chicken microbiota biofilms. Food Control. 107:106786. doi: 10.1016/j.foodcont.2019.106786.
- Truchado, P., M. Larrosa, I. Castro-Ibáñez, and A. Allende. 2015. Plant food extracts and phytochemicals: Their role as quorum sensing inhibitors. Trends in Food Science & Technology 43 (2):189–204. doi: 10.1016/j.tifs.2015.02.009.
- Unlu, A., T. Sar, G. Seker, A. G. Erman, E. Kalpar, and M. Y. Akbas. 2018. Biofilm formation by Staphylococcus Aureus strains and their control by selected phytochemicals. International Journal of Dairy Technology 71 (3):637–46. doi: 10.1111/1471-0307.12520.
- Vijayakumar, K., and T. Ramanathan. 2018. Antiquorum sensing and biofilm potential of 5-Hydroxymethylfurfural against Gram positive pathogens. Microbial Pathogenesis 125:48–50. doi: 10.1016/j.micpath.2018.09.008.
- Wang, L., C. Bi, H. Cai, B. Liu, X. Zhong, X. Deng, T. Wang, H. Xiang, X. Niu, and D. Wang. 2015. The therapeutic effect of chlorogenic acid against Staphylococcus aureus infection through sortase A inhibition. Frontiers in Microbiology 6:1031–43. doi: 10.3389/fmicb.2015.01031.
- Wang, B., and T. W. Muir. 2016. Regulation of virulence in Staphylococcus aureus: Molecular mechanisms and remaining puzzles. Cell Chemical Biology 23 (2):214–24. doi: 10.1016/j.chembiol.2016.01.004.
- Wu, S. C., F. Liu, K. Zhu, and J. Z. Shen. 2019a. Natural products that target virulence factors in antibiotic-resistant Staphylococcus aureus. Journal of Agricultural and Food Chemistry 67 (48):13195–211. doi: 10.1021/acs.jafc.9b05595.
- Wu, X., and Y. C. Su. 2014. Effects of frozen storage on survival of Staphylococcus aureus and enterotoxin production in precooked tuna meat. Journal of Food Science 79 (8):M1554–1559. doi: 10.1111/1750-3841.12530.
- Wu, Y. P., X. Y. Liu, J. R. Bai, H. C. Xie, S. L. Ye, K. Zhong, Y. N. Huang, and H. Gao. 2019b. Inhibitory effect of a natural phenolic compound, 3-p-trans-coumaroyl-2-hydroxyquinic acid against the attachment phase of biofilm formation of Staphylococcus aureus through targeting sortase A. RSC Advances 9 (56):32453–61. doi: 10.1039/C9RA05883D.
- Xie, Q., A. Zhao, P. D. Jeffrey, M. K. Kim, B. L. Bassler, H. A. Stone, R. P. Novick, and T. W. Muir. 2019. Identification of a molecular latch that regulates Staphylococcal virulence. Cell Chemical Biology 26 (4):548–58. doi: 10.1016/j.chembiol.2019.01.006.
- Xu, Y., C. Shi, Q. Wu, Z. Zheng, P. Liu, G. Li, X. Peng, and X. Xia. 2017. Antimicrobial activity of punicalagin against Staphylococcus aureus and its effect on biofilm formation. Foodborne Pathogens and Disease 14 (5):282–7. doi: 10.1089/fpd.2016.2226.
- Xu, S., T. Sun, Q. Xu, C. Duan, Y. Dai, L. Wang, and Q. Song. 2018. Preparation and antibiofilm properties of zinc oxide/porous anodic alumina composite films. Nanoscale Research Letters 13 (1):201–12. doi: 10.1186/s11671-018-2568-4.
- Yan, X., S. Gu, X. Cui, Y. Shi, S. Wen, H. Chen, and J. Ge. 2019. Antimicrobial, anti-adhesive and anti-biofilm potential of biosurfactants isolated from Pediococcus acidilactici and Lactobacillus plantarum against Staphylococcus aureus CMCC26003. Microbial Pathogenesis 127:12–20. doi:10.1016/j.micpath.2018.11.039.
- Yan, X., S. Gu, Y. Shi, X. Cui, S. Wen, and J. Ge. 2017. The effect of emodin on Staphylococcus aureus strains in planktonic form and biofilm formation in vitro. Archives of Microbiology 199 (9):1267–75. doi: 10.1007/s00203-017-1396-8.
- Zhang, J., H. Liu, K. Zhu, S. Gong, S. Dramsi, Y.-T. Wang, J. Li, F. Chen, R. Zhang, L. Zhou, et al. 2014. Antiinfective therapy with a small molecule inhibitor of Staphylococcus aureus sortase. Proceedings of the National Academy of Sciences of the United States of America 111 (37):13517–22. doi:10.1073/pnas.1408601111.
- Zhang, B., Z. Teng, X. Li, G. Lu, X. Deng, X. Niu, and J. Wang. 2017. Chalcone Attenuates Staphylococcus aureus virulence by targeting sortase A and alpha-hemolysin. Frontiers in Microbiology 8:1715–27. doi: 10.3389/fmicb.2017.01715.
- Zhao, X., Z. Liu, Z. Liu, R. Meng, C. Shi, X. Chen, X. Bu, and N. Guo. 2018. Phenotype and RNA-seq-based transcriptome profiling of Staphylococcus aureus biofilms in response to tea tree oil. Microbial Pathogenesis 123:304–13. doi: 10.1016/j.micpath.2018.07.027.