Abstract
Alzheimer’s disease (AD) is a progressive neurodegenerative disorder. Recently, sustained neuroinflammatory response in microglia and astrocytes has been found to cause the deposition of amyloid beta plaques and the hyperphosphorylation of tau protein, thereby accelerating AD progression. The lipoxin A4-transcription factor nuclear factor-kappa B and mitogen-activated protein kinase pathways have been shown to play important roles in the regulation of inflammatory processes. There is growing research-based evidence suggesting that dietary whole-plant foods, such as mushrooms and berries, may be used as inhibitors for anti-neuroinflammation. The beneficial effects of whole-plant foods were mainly attributed to their high contents of functional macromolecules including polysaccharides, polyphenols, and bioactive peptides. This review provides up-to-date information on important molecular signaling pathways of neuroinflammation and discusses the anti-neuroinflammatory effects of whole-plant foods. Further, a critical evaluation of plants’ macromolecular components that have the potential to prevent and/or relieve AD is provided. This work will contribute to better understanding the pathogenetic mechanism of neuroinflammation in AD and provide new approaches for AD therapy.
Introduction
Alzheimer’s disease (AD) is a major health concern increasing dramatically due to the rapid increase in aging population (Takeda Citation2019). Currently, there are about 50 million cases worldwide and it is expected the number of cases will triple to 152 million by 2050 (Sharma et al. Citation2019). The main pathological hallmarks of AD are characterized by the deposition of amyloid-beta (Aβ) plaques and the formation of neurofibrillary tangles (NFTs) (Busche and Hyman Citation2020). Aβ deposition is promoted by the sequential cleavage of the amyloid precursor protein (APP) by the β-secretase (BACE1) and the γ-secretase, as well as the accumulation of NFTs that consist of the phosphorylated tau in the brain (Shi, Sabbagh, and Vellas Citation2020). However, these classical Aβ and tau theories do not seem to hold for all AD cases. Recent studies indicated that neuroinflammation could appear even before the classical markers and cognitive symptoms of the disease, as demonstrated by positron emission tomography (PET) imaging in AD patients at prodromal stage (Hamelin et al. Citation2016; Rodriguez-Vieitez et al. Citation2016). Up-regulation of APP and BACE1 expressions has been shown to increase Aβ deposition (Domingues, da Cruz e Silva, and Henriques Citation2017; Lin et al. Citation2020; Jorda et al. Citation2020). In particular, inflammatory cytokines could up-regulate BACE1 expression and enzymatic activity, resulting in increased Aβ production (Alasmari et al. Citation2018). Moreover, multiple proinflammatory cytokines have been shown to up-regulate APP expression in the mice brain and the human brain (Domingues, da Cruz e Silva, and Henriques Citation2017; Alasmari et al. Citation2018). Similar to Aβ deposition, neuroinflammation could also accelerate tau phosphorylation and dispersion. For example, several cytokines such as interleukin-1β (IL-1β), interleukin-6 (IL-6), and tumor necrosis factor-α (TNF-α) have been found to promote tau phosphorylation, which potentially affects its function and accelerate NFTs formation leading to the development of AD symptoms (Decourt, Lahiri, and Sabbagh Citation2017; Kaur, Sharma, and Deshmukh Citation2019; Lau, Fu, and Ip Citation2021). These findings suggest that neuroinflammation plays a prominent role in the pathogenesis of AD () and several mechanisms are potentially involved. Therefore, elucidating the neuroinflammatory pathways involved in the progression of AD would be beneficial to explain potential mechanisms that contribute to AD and examine opportunities to delay its onset by functional foods.
Figure 1. The role of neuroinflammation in the progress of AD. Pro-inflammatory cytokines released by activated microglia can increase Aβ deposition through upregulating APP and BACE1 expression, and promote tau phosphorylation accelerating NFTs formation. APP, amyloid precursor protein; Aβ, amyloid-beta; BACE1, β-secretase; NFTs, neurofibrillary tangles.
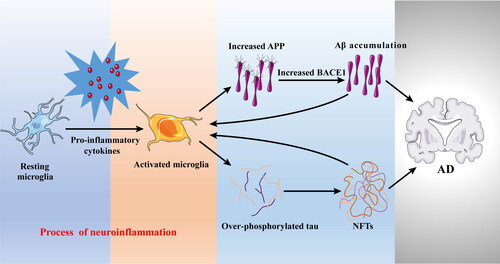
The transcription factor nuclear factor-kappa B (NF-κB) signaling pathway and the mitogen-activated protein kinase (MAPK) signaling pathway are the primary pathways of regulating inflammatory progress in neuronal cells (Jayaraj et al. Citation2019; Zhou et al. Citation2020). Many natural products such as mushrooms (Trovato Salinaro et al. Citation2018) and berries (Gasparrini et al. Citation2021) can regulate these two pathways and thus improve AD condition. Further studies have indicated that bioactive components such as polysaccharides, polyphenols, and peptides in these natural products can control neuroinflammation (Welcome Citation2020). However, because of their complicated and variable structures and properties, the actual biochemical interactions and mechanisms of action are not conclusive. The effects of some micro-molecules, such as steroids and terpenoids, on modulating neuroinflammation to improve AD have been summarized and reviewed (Yilmaz et al. Citation2019; Jin et al. Citation2019; Olajide and Sarker Citation2020). In contrast, macromolecules like polysaccharides and peptides have rarely been reviewed. Moreover, few studies have discussed potential relationships among whole-plant foods, their bioactive compounds, and regulation of neuroinflammation.
This review mainly focuses on two significant pathways, lipoxin A4 (LXA4)-NF-κB and MAPK, of regulating neuroinflammatory progress in AD. In addition, the underlying mechanisms of anti-neuroinflammation of whole-plant foods (mushrooms, berries, Curcuma longa L., and garlic) and their bioactive macromolecules including polysaccharide, polyphenol, and peptide are discussed and compared. This review provides new sights into AD progression and some possible interventions to control AD with natural products.
Key signaling pathways of neuroinflammation in AD
Inflammation is a defensive response to cellular injury in the body intended to be protective, but an excessive inflammatory response will cause tissue damage and lead to pathological diseases (Long and Holtzman Citation2019). Neuroinflammation was defined as the inflammatory response in the central nervous system (CNS) secondary to neuronal insult (Leng and Edison Citation2021). Astrocytes and microglial cells are the target of the inflammatory response in the CNS (Hansen, Hanson, and Sheng Citation2018). The inflammatory conditions in activated microglia have been found to be regulated by intracellular signaling pathways, mainly by NF-κB and MAPK pathways (Leng and Edison Citation2021). Also, LXA4 is an endogenous anti-inflammatory molecule that could modulate the levels of various transcription factors such as NF-κB (Jaén et al. Citation2021). In the following section, we comprehensively summarize and discuss the LXA4-NF-κB and MAPK pathways in the inflammatory processes, which are conducive to revealing some insights on the mechanism of AD.
The role of LXA4-NF-κB pathway in AD development
LXA4 acts as an endogenous “braking signal” in the inflammatory process, which can inhibit neutrophil recruitment and activation, and reduce many cell responses such as neutrophils and macrophages by blocking pro-inflammatory cytokines, thereby promoting resolution of inflammation (Kantarci et al. Citation2018). The lipoxin (LX) biosynthesis has been divided into three routes (Tiberi and Chiurchiù Citation2021). The first route of LX biosynthesis involves the major lipoxygenases (LO): 5-LO and 12-LO. In this case, arachidonic acid (AA) attaches to 5-LO to produce leukotriene A4 (LTA4), and then 12-LO catalyzes the conversion of LTA4 into a delocalized cation, which is hydrolyzed to give LXA4 and LXB4 (Serhan and Sheppard Citation1990). The second route is where AA is firstly utilized by 15-LO to yield 15S-hydroxy eicosatetraenoic acid (15S-HpETE), which is then transformed by 5-LO into the 5S, 6S, 15S-epoxytetraene, and then generate LXA4 and B4 (Serhan, Hamberg, and Samuelsson Citation1984). The third route is an aspirin-dependent way (Clària and Serhan Citation1995). Briefly, after treatment with aspirin, cyclooxygenase (COX)-2 produces 15R-HpETE, which is used by 5-LO, and transformed into 15-epi-LXA4 and 15-epi-LXB4. The end products termed aspirin-triggered lipoxin (ALT) are LX epimers with the alcohol at C15 in the R configuration and have similar functions to LX (Romano et al. Citation2015).
Several studies have indicated that endogenous LXA4 possesses inhibiting activity against AD progression. It is responsible for down-regulating the gene expression and protein expression of IL-1β and TNF-α induced by β-amyloid both in the cortex and hippocampus of mice and in Aβ-stimulated BV2 cells (Wu et al. Citation2011). Furthermore, the administration of LXA4 in mice was found to improve AD, as indicated by the reduction in the physiological indices of Aβ pathology and reversal in the inflammation process. Further studies suggested that LXA4 mediates the NF-κB signaling pathway involved in the modulation of inflammation (Kantarci et al. Citation2018). It is now well-established that the NF-κB plays a major role in neuroinflammation-mediated AD. It is regarded as a master regulator of inflammatory gene transcription expressed in the AD brains (Ju Hwang et al. Citation2019). Under normal conditions, NF-κB is bound to its inhibitory protein κB (IκB) within the cytoplasm in an inactive state, whereas it can be activated by various extracellular stimuli such as pro-inflammatory cytokines and bacterial lipopolysaccharides (LPS) (Yu et al. Citation2020). The stimulation initiates an intracellular signaling cascade, resulting in the phosphorylation of IκB by IκB kinase (IKK), and then the IκB is degraded (Sun Citation2017). Once liberated from its inhibitory protein, NF-κB translocates into the nucleus, where it conducts the transcription of several cytokines and chemokines. Moreover, aspirin-triggered LXA4 (ATL) inhibits the LPS-evoked activation of NF-κB and the DNA-binding activities of NF-κB in lung tissues (Liu et al. Citation2018). Hence, the LXA4-NF-κB pathway would be an important pathway for bioactive substances to regulate neuroinflammation ().
Figure 2. The anti-inflammatory effect of natural products via LXA4-NF-κB and MAPKs. Under normal conditions, NF-κB is bound to its inhibitory protein κB (IκB) within the cytoplasm in an inactive state. Once activated, IκB is degraded and NF-κB translocates into the nucleus, where it conducts the transcription of pro-inflammatory cytokines. However, LXA4 can inhibit activation of NF-κB signaling pathway. The activation of JNK and p38 MAPK also can promote transcription of pro-inflammatory cytokines through AP-1. Whole-plants food and macromolecules can inhibit inflammation via LXA4-NF-κB and MAPKs. LXA4, lipoxin A4; ALX/FPR2, G-protein coupled formyl peptide receptor 2; TLR4, toll-like receptor 4; NF-κB, nuclear factor kappa-B; IκBα, NF-kappa-B inhibitor alpha; MKK, mitogen-activated protein kinases kinases; JNK, c-Jun NH2-terminal kinase; AP-1, activated protein 1; IL-1β, interleukin 1β; IL-6, interleukin 6; TNF-α, tumor necrosis factor alpha; iNOS, inducible nitric oxide synthase.
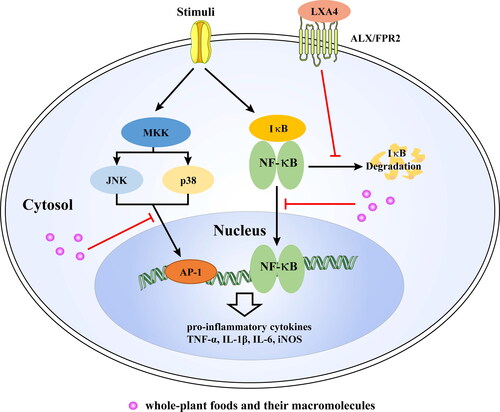
It should be noted that the endogenous LXA4 prevents the release of excessive inflammatory cytokines by the inhibition of microglial activation (Wu et al. Citation2019). However, it is unclear whether microglial activation in AD is a damaging or protective step even though it is associated with disease pathology in AD. For example, activated microglia are beneficial through the phagocytosis of debris and Aβ, whereas uncontrolled activation of microglia can release excessive pro-inflammatory factors that induce neurotoxicity (Lu et al. Citation2019). Recently, a novel viewpoint that modifying microglial activation, instead of inhibiting it, seems to represent a reasonable alternative to enhance Aβ clearance and reduce amyloid deposition in the AD brain (Yang, Kuboyama, and Tohda Citation2019). In particular, treatment with ATL resulted in improved cognition, reduced Aβ levels, and enhanced microglial phagocytic activity in Tg2576 transgenic AD mice (Dunn et al. Citation2015). In addition, ATL did not alter the production of Aβ, but microglia in the brains of ATL-treated Tg2576 mice presented an alternative activation phenotype that enhanced their phagocytic activity of Aβ42 and the down-regulation in inducible nitric oxide synthase (iNOS) expression (Medeiros et al. Citation2013).
The role of MAPK pathway in AD development
MAPKs are crucial signal transducers, which have evolved in a wide array of extracellular signals and intracellular signal cascades. The diverse MAPK molecules and their respective signal transducers are categorized into three distinct functional groups, including the extracellular signal-regulated kinase (ERK), p38, and c-Jun NH2-terminal kinase (JNK) (Guo et al. Citation2020). Substaintial evidence indicates that MAPK signaling pathways are involved in the inhibition of neuroinflammation in neurological disorders (Plastira et al. Citation2020). The MAPKs signaling pathway might play a pivotal role in LPS-, cytokine-, and Aβ-induced neuroinflammation (Kheiri et al. Citation2018). Studies have provided evidence that overexpression of cytokines such as IL-1β and TNF-α is mediated by activating p38 MAPK, contributing to cognitive decline (Falcicchia et al. Citation2020). Furthermore, the use of p38 MAPK inhibitor in transgenic mice decreased neuroinflammatory conditions and Aβ deposits, and improved spatial memory (Gee et al. Citation2020). These findings provided evidence linking p38 MAPK to neuroinflammation. Further studies have shown that activation of p38 MAPK is positively correlated with the amount of aggregated tau (Lee and Kim Citation2017). Additionally, p38 MAPK can activate the NF-κB pathway in neuroinflammation-induced AD. Thus, the p38 MAPK is considered a potential molecular target for novel AD treatment ().
Figure 3. Novel strategies of macromolecules on alleviation of neuroinflammation. Nanoparticle- and gut microbiota-based approaches greatly enhance the anti-neuroinflammatory activity of macromolecules, and the change of gut microbiota composition and metabolites can modulate neuroinflammation via gut-brain axis.
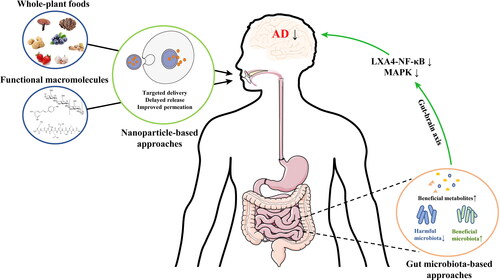
JNK3 is an important member of the JNK family that can be used as a regulatory target to treat AD. The JNK3 was found to mainly expresses in brain and heart (de Los Reyes Corrales, Losada-Pérez, and Casas-Tintó Citation2021). In clinical studies, a significant increase of JNK3 levels was observed in the cortex and cerebrospinal fluid (CSF) from AD patients’ brains compared with controls (Hepp Rehfeldt et al. Citation2020). A high level of JNK3 in the CSF was highly correlated to cognitive decline based on the linear mixed models. Furthermore, JNK3 is a key kinase for APP phosphorylation, suggesting that JNK3 plays an important role in AD via regulating Aβ production (Musi et al. Citation2020). Like the p38 MAPK, JNK signaling is an important pathway contributing to neuroinflammation (Anfinogenova et al. Citation2020). Inhibition of JNK activation was found to reduce productions of TNF-α IL-6 and IL-1β, and inhibited microglia activation to attenuate neuroinflammation (Zheng et al. Citation2020).
Whole-plant foods beneficially improve neuroinflammation in AD
Despite the outstanding progress in modern medicine, there are still no effective drugs to treat neurodegenerative diseases such as AD. Thus, there is an urgent need to find preventive substances with low side effects to treat AD. Whole-plant foods are highly appreciated by consumers as a source of nutrients to maintain and improve health and wellbeing. Consumption of whole-plant foods has been shown to have anti-neuroinflammatory activity in both human and animal models (Shukitt-Hale et al. Citation2019; Rutledge et al. Citation2019). This section summarizes the effects of whole-plant foods, i.e., mushrooms, berries, Curcuma longa L., and garlic, on the neuroinflammation in AD. The representative studies on the effects of whole-plant foods and their extracts on neuroinflammation are summarized in .
Table 1. Effects of whole-plant foods on neuroinflammation.
Mushrooms
Edible mushrooms have attracted a great deal of attention due to their high nutritional content and diverse bioactivities that will support overall health maintenance and disease prevention. Mushrooms have antioxidant (Lu et al. Citation2018), anti-inflammatory (Muszyńska et al. Citation2018), and anti-diabetic activities (Khursheed et al. Citation2020). Among those, the anti-inflammatory activity of mushrooms has been studied in depth. For example, ethanolic extract of Hericium erinaceus suppressed LPS-induced secretion of NO production in BV2 cells (Kushairi et al. Citation2019). In addition, Bennett et al. (Citation2013) indicated that APPswe/PS1dE9 mice treated with vitamin D2-enriched Agaricus bisporus powder (VDM, 5% wt/wt, 7 months) displayed higher levels of IL-10, which have substantial anti-inflammatory activities. They also found that VDM significantly reduced the content and size of Aβ plaque in the cortex or hippocampus. Similar results were observed by Tsai-Teng et al. (Citation2016), in which Hericium erinaceus mycelia and its ethanol extracts (300 mg/kg, 30 days) reduced activated microglia and astrocytes, thereby attenuating cerebral Aβ plaque burden and the number of whole plaques. These findings suggest that mushrooms may reduce Aβ plaque by regulating neuroinflammation.
Recently, the role of mushrooms in regulating neuroinflammation has been comprehensively reviewed (Kushairi et al. Citation2020). It has been found that extracts and compounds from serval medicinal mushrooms have anti-neuroinflammatory activities via TLR4/NF-κB pathway. Ethanol extract of Ganoderma lucidum (GLE, 0.5 mg/mL) has shown to inhibit pro-inflammatory cytokines (IL-1α, murine monocyte chemoattractant protein-5 (MCP5), and macrophage inflammatory protein-3α (MIP3α)) via NF-κB and MAPK pathways in LPS-treated BV2 cells (Hilliard, Mendonca, and Soliman Citation2020). Moreover, it has been reported that mushrooms can significantly up-regulate LXA4 expression in brains and can ameliorate AD symptoms (Trovato Salinaro et al. Citation2018). Coriolus versicolor biomass powder (200 mg/kg), soluble in saline, treatment of adult male rats for 30 days resulted in a significant increase in the expression levels of LXA4 in the brain regions, especially in the cerebral cortex and hippocampus (Trovato, et al. Citation2016). Similarly, after oral administration of Hericium erinaceus biomass (200 mg/kg for 3 months) in adult male rats, the expression of LXA4 in brain was up-regulated, with the highest level in the cerebral cortex, followed by the hippocampus (Trovato et al. Citation2016). Interestingly, LXA4 could impact many endogenous processes such as NF-κB translocation and chemokines production. For example, LXA4 inhibited IL-1β and TNF-α induction by LPS in BV2 cells and suppressed microglial activation (Wu et al. Citation2019). Collectively, mushrooms can regulate both MAPK and NF-κB signaling pathways, but mainly depend on LXA4-NF-κB to reverse neuroinflammation in AD.
Berries
Berries, well-known functional fruits, are excellent source of micronutrients and nonessential phytochemicals, especially polyphenols (Gasparrini et al. Citation2021). Previous studies indicated that most berries, e.g., blueberries, strawberries, and Goji, could significantly inhibit LPS-induced inflammatory factors such as TNF-α and IL-6 in animal and human models (Ma et al. Citation2019; Gasparrini et al. Citation2021). In addition, studies investigated strategies to counteract AD that demonstrated dietary intake of berries was able to improve memory and protect the brain against cognitive loss both in vitro and in vivo experiments (Boespflug et al. Citation2018; Ma et al. Citation2019; Liu and Du Citation2020; Whyte et al. Citation2021). These results suggested that berries might improve AD symptoms in association with regulating neuroinflammation.
Recently, it has been shown that strawberry extracts (50 and 100 g/mL) can reduce the level of proinflammatory cytokines in LPS-induced human dermal fibroblast cells (Gasparrini et al. Citation2018). Further studies indicated that consumption with one or more servings of strawberries per week had a 34% lower risk of developing AD, suggesting that the anti-neuroinflammatory effect of strawberries is positively related to improving cognitive function (Agarwal et al. 2019). Some studies found that blueberry juice consumption (444–621 mL/d, 12 weeks) could improve the memory of senior people who had early memory decline (Krikorian et al. Citation2010). And blueberries (24 g/d, 90 d) could effectively improve the cognitive function of subjects (Miller et al. 2017). Rutledge et al. (Citation2019) indicated that both blueberries and strawberries regulated inflammation in old adults and LPS-treated BV2 cells, and the bioactive components such as polyphenols in berries were still active even during fasting periods. Furthermore, the functions of whole berries might depend on their metabolites in the host. For example, the anti-inflammatory effects of both blueberry and strawberry have been shown to be significantly related to the retention time of the metabolite phenolic acid in the blood (Sandhu et al. Citation2017; Sandhu et al. Citation2018). Interestingly, the impact of blueberries on improving cognition depended on individual cognitive baseline status. Short-term daily consumption of blueberries (20 g/kg diet, 8 weeks) was more beneficial to subjects with poor cognitive performance than good cognitive group, which may be due to the physiological states of the good performers are more stable than those in the poor performers, and there is no much room to improve in good performers (Shukitt-Hale et al. Citation2019). However, the mechanism underlying the anti-neuroinflammatory effect of berries remains obscure. Therefore, the topic is emerging, and it will be interesting to conduct experiments to investigate the LXA4-NF-κB and MAPK pathways in berries treated models and their effects on neuroinflammation ().
Table 2. Effects of phytochemicals on neuroinflammation.
Curcuma longa L
Curcuma longa L. is a well-known edible and medicinal plant that possesses in vitro and in vivo multiple biological activities, including antioxidant, anti-inflammation, and antidementia (Eun et al. Citation2017; Flores Citation2017; Yuliani, Mustofa, and Partadiredja Citation2018). Numerous studies have investigated the effect of Curcuma longa L. on AD symptoms. For instance, experimental evidence demonstrated its effectiveness in decreasing neuroinflammation and β-amyloid plaques, and improving the cognitive function (Adalier and Parker Citation2016; Berry et al. Citation2021). In scopolamine-treated mice, fermented Curcuma longa L. (50, 100 and 200 mg/kg) improved memory deficits through suppression of acetylcholinesterase (AchE) activity (Eun et al. Citation2017). AchE activity was found to control the levels of TNF-a, IL-6, and IL-1β via the cholinergic anti-inflammatory pathway, and thus it was used as a biomarker for neuroinflammation (Tyagi et al. Citation2008). In addition, after treatment with Curcuma longa L. (15 and 30 mg/kg) for six weeks, the memory performance in D-galactose-treated mice has been evaluated (Kumar, Prakash, and Dogra Citation2011). The result showed that Curcuma longa L. decreased AchE activity, attenuated oxidative stress, and was found to improve impaired learning and memory. These results indicated that Curcuma longa L. could beneficially improve AD symptoms. However, less evidence is available about the modulation of Curcuma longa L. on the neuroinflammation in AD. In a recent study, fermented Curcuma longa L. (100 and 200 mg/kg) containing 1.44% curcuminoids, suppressed the production of inflammatory biomarkers such as TNF-α via regulating the expression levels of COX-2 and iNOS in LPS-treated BV2 cells and prevented glioma C6 cell death induced by H2O2 (Eun et al. Citation2017). Collectively, these results imply that Curcuma longa L. has the potential to improve nerve-related diseases by regulating neuroinflammation. Thus, further studies are warranted to determine the effect of Curcuma longa L. on neuroinflammation as well as identifying the pathways that may be involved in regulating neuroinflammation.
Garlic (Allium sativum)
Garlic (Allium sativum) has been well recognized for its culinary and medicinal value, and different food and nutraceutical products have been developed from garlic. Raw garlic extract displayed great anti-neuroinflammatory activity (Ho and Su Citation2014). It inhibited the production of NO, IL-1β, TNF-α, and monocyte chemoattractant protein-1 (MCP-1) by suppressing NF-κB activation in BV2 cells. Based on the anti-neuroinflammatory activity of fresh garlic, many garlic products have been developed for neuro-protection. Aged garlic extract (AGE, 125, 250, and 500 mg/kg for 65 days), an odorless garlic preparation containing S-allylcysteine (SAC) as its most abundant compound (Colin-Gonzalez et al. Citation2012), was found to restore the density of cholinergic neurons in the brains of Aβ-treated rats and significantly improve working memory at a dose of 250 mg/kg body weight (Thorajak et al. Citation2017). However, recent studies suggested AGE modulated inflammation to prevent AD. For instance, AGE at doses of 250 and 500 mg/kg body weight for 56 days significantly reduced the inflammatory response by decreasing the activation of microglia and IL-1β to the levels found in the untreated control group, thereby improving short-term recognition memory in cognitively impaired rats (Nillert et al. Citation2017). Additionally, AGE could reduce the expression levels of neuroinflammatory mediators, including IL-1β and toll-like receptor 4 (TLR4) via the NF-κB pathway, and show an excellent AD prevention effect (Sripanidkulchai Citation2020). Interestingly, the crosstalk of anti-inflammation and antioxidant of AGE contributes to its neuroprotection effect. AGE extends antioxidant properties via nuclear factor erythroid 2-related factor 2 pathway in microglial and Aβ-induced neuroinflammation in rats (Song et al. Citation2016; Thorajak et al. Citation2017). Garlic oil (GO), another garlic product, also shows neuroprotective effects due to its antioxidant effect. Administration of GO (46 mg/kg body weight) markedly attenuated impaired short-term memory and motor coordination after global cerebral ischemia for 10 min followed by reperfusion for 24 h in mice (Gupta, Singh, and Sharma Citation2003). A recent study reported that the neuroprotective effect of garlic could be attributed to organosulfur components (Song et al. Citation2020). SAC down-regulated hippocampal nuclear NF-κB and attenuated oxidative stress, resulting in mitigating cognitive deficits (Zarezadeh et al. Citation2017). Alliin, the main functional component of raw garlic, and alliin-derived sulfides exerted anti-neuroinflammatory capacity via the NF-κB pathway that contributed to the effect of garlic against AD (Ho and Su Citation2014).
Effects of functional macromolecules present in whole-plant foods on neuroinflammation
The improvement of neuroinflammation by whole-plant foods was mainly attributed to functional macromolecules such as polysaccharides, polyphenols, and peptides found in these whole-plant foods. Due to the anti-inflammatory, antioxidant, and neuroprotective properties of their functional macromolecules with minimal side effects, these functional macromolecules may improve and prevent neurodegeneration in AD (Cooper and Ma Citation2017). In the following section, we summarize and discuss the anti-neuroinflammatory activities of functional macromolecules from multiple plants in both in vitro and in vivo studies with evidence of the mechanistic pathways involved. In addition, comparisons were also made among the various sources of the bioactive compounds and the different AD models used to identify any limitations in models or specific interactions.
Polysaccharides
Dietary polysaccharides, a complex group of carbohydrate macromolecules, are the main bioactive components of many natural plants. Based on the available references, the anti-neuroinflammatory polysaccharides were found to mainly derived from mushrooms (Ganoderma lucidum, Flammulina velutipes and Phellinus linteus) and herbs (Astragalus membranaceus, Lycium barbarum, Acorus tatarinowii, Schisandra chinensis, Dendrobium huoshanense and Sophorae tonkinensis) (Cai, Li, and Pei Citation2017; Su et al. Citation2018; Hu et al. Citation2018; Huang et al. Citation2017; Lam et al. Citation2015; Zhong et al. Citation2020; Xu et al. Citation2020; Ge et al. Citation2018; Cai et al. Citation2018). Most of them are heteropolysaccharides and are generally named by their sources. For example, backbones of [→2)-α-D-Galp-(1→4)-α-D-Galp-(1→6)-α-D-Glcp-(1→3)-β-D-Glcp-(1→] in F. velutipes polysaccharide (FVP, Mw: 268 kDa) (Yang et al. Citation2012), [(1→2,4)-β-D-Xylp, (1→4)-α-D-Glcp and (1→5)-β-D-Araf] residues in P. linteus polysaccharide (Mw: 343,000 kDa) (Hu et al. Citation2018), [→3)-α-L-Araf-(1→5)-α-L-Araf-(1→3,4)-α-L-Rhap-(1→3,4)-α-D-Xylp-(1→2)-α-L-Araf-(1→4)-β-D-GlcpA-(1→3)-β-D-Galp-(1→3)-β-DgalpA-(1→6)-β-D-Galp-(1→2,3,6)-β-D-Glcp-(1→4,6)-β-D-Manp-(1→3,6)-β-D-Galp-(1→3,4,6)-β-D-Galp-(1→] in A. tatarinowii polysaccharide (Mw: 87.3 kDa) (Zhong et al. Citation2020), [→4)-β-Galp-(1→4)-α-Glcp-(1→] in S. chinensis polysaccharide (Mw: 5,388 kDa) (Xu et al. Citation2020), and [(1→4)-Manp, (1→4)-Glcp, (1→4,6)-Manp and 1-Glcp] residues in D. huoshanense polysaccharide (intrinsic viscosity [η]: 3.76 ± 0.412 dL/g) (Ge et al. Citation2018) were revealed. However, a minor part of the polysaccharides are homopolysaccharides, such as (1→4)-α-D-glucans (Mw: 12–36 kDa) existed in A. membranaceus (Fu et al. Citation2014). These structural differences may be due to different types of raw materials, growth environments, extraction technology, and parts of the plant (Li et al. Citation2018; Zhu et al. Citation2021).
The beneficial effects of most polysaccharides were based in the LPS- and Aβ-induced neuroinflammation models, where the regulation of inflammatory cytokines was confirmed via the pathways of MAPK and NF-κB. In addition, microglia as the major mediator of neuroinflammation is closely linked to the release of inflammatory cytokines and Aβ production; thus, microglial cells treated by LPS are usually used as an in vitro model. For example, polysaccharides from G. lucidum (0.01, 0.1 and 1 μg/mL) were found to inhibit LPS-induced (1 μg/mL) pro-inflammatory cytokines IL-1β, IL-6, and iNOS expressions in the microglial model in vitro in a dose-dependent manner (Cai, Li, and Pei Citation2017). In addition, Astragalus polysaccharide (APS) (200 μg/mL) treatment for 1 h was found to reduce the iNOS and NO production significantly, and suppress COX-2, TNF-α and IL-1β mRNA expressions by inhibiting NF-κB activation in LPS-stimulated BV2 microglia (Luo et al. Citation2015). Treating LPS-treated BV2 microglial cells with 600 μg/mL of L. barbarum polysaccharides (LBPs) effectively suppressed the expression of TNF-α, and p65, as well as inhibited the activation of NF-κB and microglia (Teng et al. Citation2013). These results suggest that the anti‐inflammatory effects of LBPs may be associated with the inhibition of NF-κB signaling. It should be noted that LPS treatment alone for 30 min augmented NF-κB p65 phosphorylation in BV2 microglial cells, which was accompanied by increased translocation of NF-κB p65 from the cytoplasm to the nucleus (Zhong et al. Citation2020). A recent study found that pretreatment with A. tatarinowii polysaccharides (2.5, 5, and 10 μM) can inhibit LPS-induced IKKα/β activation and IκBα degradation by blocking NF-κB p65 nuclear translocation in BV2 microglial cells in a concentration-dependent manner (Zhong et al. Citation2020). A purified S. chinensis polysaccharides (SCP2-1) reversed the phenotypes of M1/M2 polarization BV2 cells stimulated with LPS at various concentrations (6.25 μg/mL and 12.5 μg/mL) (Xu et al. Citation2020). The results demonstrated that SCP2-1 inhibited M1 microglia and promoted cells M2 polarization that was based on the level of inflammatory cytokines. These studies suggest that plant-derived polysaccharides from different sources regulate neuroinflammation in LPS-induced microglial cells, with particular relevance to NF-κB pathways.
Compared to cell models, several animal models can be used to evaluate the anti-neuroinflammation activities of polysaccharides, including LPS, scopolamine, Aβ-treated mice or rats, transgenic AD mice and other diseases-induced cognitive impaired mice. The polysaccharide SCP2-1 was investigated in an LPS-induced cognitive dysfunction mice model at 28 and 56 mg/kg for 3 weeks and was found to suppress M1 polarization in the hippocampus of mice stimulated with LPS (Xu et al. Citation2020). Furthermore, SCP2-1 suppressed NF-κB and JNK pathway, and improved cognitive dysfunction in Y-maze and NOR tests. The study showed that SCP2-1 treatment (260 mg/kg body weight for 28 days) in an Aβ1–42-induced mice relieved the levels of IL-1β, IL-6 and TNF-α, and reduced the activation of astrocytes and microglia, which ameliorated excessive Aβ1-42 deposition, resulting in improving short-term learning memory and cognitive capacity (Xu et al. Citation2019).
Scopolamine is widely used in models for studying cognitive-related illnesses since it can impair memory function in humans and rodents, particularly working memory and learning acquisition (Tang Citation2019). Su et al. (Citation2018) reported that polysaccharides from F. velutipes (400 g/kg body weight, 63 days) suppressed IL-6, IL-1β and TNF-α expressions, promoted the anti-inflammatory mediator IL-10, and improved the behavioral performance in the Morris Water Maze (MWM) test in mice with an injection of 5 mg/kg body weight scopolamine. Water-soluble ginseng oligosaccharides (40 and 80 mg/kg body weight, 10 days) showed significant inhibition of neuroinflammation, by down-regulating the expression of IL-1β and IL-6 mRNA and inhibiting astrocyte activation in the hippocampus of mice treated with 3 mg/kg body weight scopolamine (Xu et al. Citation2016).
Apart from chemical induced cognitive impaired models, transgenic mice are considered a valuable animal model to study AD. APP/PS1 double transgenic mice are widely used to express mouse/human amyloid precursor protein (APPswe) and a mutant human presenilin 1 (PS1-dE9), both directed to CNS neurons. These mice may be helpful in studying neurological disorders of the brain, specifically AD (Hashimoto and Saido Citation2018). Huang et al. (Citation2017) conducted experiments that utilized a combination of a high-fat diet and a low-dose of streptozotocin (HFSTZ)-induced APP/PS1 double transgenic mice. The model further could aggravate astrocyte reactivity and cognitive impairments of APP/PS1 double transgenic mice. APS (500 mg/kg body weight, 11 weeks) supplementation in HFSTZ-induced APP/PS1 double transgenic mice significantly reduced the accumulation of reactive astrocytes and microglia around plaque, and decreased the nesting construction time prolonged by HFSTZ treatment. In addition to the models mentioned above, there are other AD models. For example, studies suggest that chronic intermittent hypoxia (CIH) can induce oxidative stress and inflammation that is correlated with early stages of neurodegenerative disease (Snyder et al. Citation2017). Before the daily CIH treatment, SD rats fed with LBPs solution in a dose of 1 mg/kg body weight for 7 days. The results showed that LBPs suppressed the expression levels of IL-1β and COX-2, and inhibited NF-κB activation in the hippocampus. Thus, LBPs can counteract the effects of CIH-induced hippocampal injury (Lam et al. Citation2015). Collectively, these findings indicate that polysaccharides from plants can inhibit neuroinflammation via NF-κB and/or JNK pathways, and are potential candidates to attenuate AD.
The bioactivities of polysaccharides are highly correlated to their chemical structures, such as constituent monosaccharides, glycosidic linkages, and molecular weights (Wang, et al. Citation2019). SCP2-1 was composed of glucose (87%) which was the major contributor to its anti-inflammatory activity (Xu et al. Citation2020). Water-soluble ginseng oligosaccharides rich in D-glucose molecules also inhibited neuroinflammation in mice (Xu et al. Citation2016), indicating that anti-neuroinflammatory activity may depend on its relatively high glucose content. Moreover, the glycosidic linkages are related to the function. SCP2-1 with 1,4-linked-Glcp and 1,4-linked-Galp, and P. linteus polysaccharides with 1,4-α-Glcp linkage had significant anti-inflammatory activity (Xu et al. Citation2020; Hu et al. Citation2018). Polysaccharides from D. huoshanense, A. tatarinowii, and S. tonkinensis Radix had similar α-glycosidic bonds, and exhibited great anti-inflammatory activity (Ge et al. Citation2018; Cai et al. Citation2018; Zhong et al. Citation2020). These results give us a hint that 1,4-α-Glcp linkage may play an important role in the anti-inflammatory activity of these polysaccharides.
Polyphenols
Polyphenols are important micronutrients obtained from the diet and are widely found in plants. According to their chemical structure, they are generally characterized by consisting of one or more hydroxyl groups linked to a benzene ring and classified as flavonoids and nonflavonoids (Serra, Almeida, and Dinis Citation2018). Flavonoids are a group of polyphenolic compounds with a typical structure (two benzene rings linked through a linear three-carbon chain), such as curcumin (1,7-bis-(4-hydroxy-3-methoxyphenyl)-hepta-1,6-diene-3,5-dione), quercetin (3,3′,4′,5,7-penthahydroxy-2-phenyl-chromen-4-one), and mangiferin (3,3′,4′,5,7-penthahydroxy-2-phenyl-chromen-4-one). The nonflavonoid group includes structurally different compounds, such as phenolic acids and resveratrol (trans-3,4,5-trihydroxystilbene). The antioxidant activities of polyphenol are associated with their beneficial effects against AD (Shavandi et al. Citation2018; Potì et al. Citation2019). The antioxidant capacity of polyphenols has been attributed mainly to their free radical scavenging ability and metal ion chelating activity (Huyut, Beydemir, and Gulcin Citation2017). In addition, they exhibit indirect antioxidant effects by interacting and regulating the activity of antioxidant enzymes (Domıńguez-Avila et al. Citation2015). The structure of polyphenols is a crucial determinant of their antioxidant activities (Dajas et al. Citation2015). With the degree of hydroxylation increasing, the antioxidant activity of polyphenols increases (Russo Citation2018). The presence of ortho-dihydroxy structure contributes to the high stability of phenoxy radicals, which participates in electron delocalization (Dajas et al. Citation2015). Additionally, ortho-dihydroxy structure and carbonyl in benzene ring contribute to metal ion chelating activity (Dajas et al. Citation2015). However, many different polyphenols have recently been reported to improve AD symptoms via regulating levels of host inflammatory factors such as IL-1β and TNF-α, which will be discussed below.
Curcumin found in Curcuma longa (turmeric) is the most investigated natural neuroprotective polyphenol for AD treatment. Curcumin (10 μM, 2 h) has been reported to lead a nearly 50% reduction in LPS-induced pro-inflammatory cytokines (IL-6, IL-1β, and iNOS), and promote anti-inflammatory cytokines (IL-10 and IL-4) as well as decrease the expression level of IκB-α in BV2 cells (Zhang et al. Citation2019). These results suggest that curcumin could inhibit neuroinflammation in association with the down-regulation of NF-κB. Similar results were also observed in LPS-induced rats (Khan et al. Citation2019). For instance, curcumin treatment could remarkably inhibit the overexpression of TNF-α and IL-1β, and then improve memory dysfunctions in the hippocampal tissue of LPS-treated adult rats at a dose of 300 mg/kg body weight for 14 days (Khan et al. Citation2019). Another study has shown that curcumin (250 mg/kg body weight, 28 days) can alleviate activated astrocytes with signs of necrosis and brain tissue damage, and improve signs of inflammation through down-regulation of NF-κB in LPS-treated SD rats (Sunny et al. Citation2019). These results suggest that curcumin as the main bioactive component of Curcuma longa can effectively inhibit neuroinflammation and have the potential against AD.
Resveratrol (80 mg/kg body weight, 12 weeks), a common polyphenol found in grapes and berries, reduced the plasma levels of pro-inflammatory makers including IL-1β, IL-12, and TNF-α by down-regulating the expression of NF-κB, and slowed progressive diminishing of cognition via protecting the integrity of the blood-brain barrier (BBB) in rats treated with D-galactose (Zhao et al. Citation2015). In addition, PS19 transgenic mice, expressing the P301S mutant form of human tau, were treated with resveratrol (40 mg/kg body weight, five weeks), and their cognitive performance was improved nearly to normal level (Sun et al. Citation2019). The results showed that resveratrol might improve cognitive deficits via reducing pro-inflammatory cytokines such as TNF-α and IL-1β. Resveratrol also could decrease mRNA levels of TNF-α, IL-6, and IL-1β in BV2 cells (Qi et al. Citation2018). Human trials to evaluate the efficacy of resveratrol in improving cognitive function have reflected the results achieved in vitro and in animal models of AD. For example, a recent clinical study by Moussa et al. (Citation2017) demonstrated that intake of resveratrol (500 mg/d, 52 weeks) by patients with AD could modulate neuroinflammation-associated mediators such as IL-8 and Aβ40, and improve the performance in mini-mental score examination compared to baseline in a placebo group. These findings suggested that resveratrol could slow the progressive cognitive loss and functional decline associated with neuroinflammation.
In addition to the well-known polyphenols, curcumin, and resveratrol, some other polyphenols such as quercetin and mangiferin have demonstrated neuroprotective activities via anti-neuroinflammation. Quercetin is a ubiquitous anti-inflammatory and antioxidant natural flavonol found in many fruits, vegetables, and seeds (Sato and Mukai Citation2020). Compared to LPS-injected mice, intake of quercetin (30 mg/kg body weight, 2 weeks) for two weeks significantly reduced levels of TNF-α, COX-2, iNOS, and IL-1β to prevent neuroinflammation by inhibiting the expression of NF-κB in the cortex and hippocampus of adult mice (Khan et al. Citation2018). Mangiferin is a potent medicinal compound isolated from plants and fruits such as Mangifera indica L. (Feng et al. Citation2019). Mangiferin (50 mg/kg body weight, 22 weeks) was shown to have a remarkable reduction of tau hyperphosphorylation and neuronal injury by regulating inflammatory progress in APP/PS1 mice (Infante-Garcia et al. Citation2017). The above information indicated that mangiferin might inhibit inflammation through the NF-κB pathway, but further research is required to confirm the involvement of this pathway. Taken together, polyphenols are considered to be one of the important natural products for inhibiting neuroinflammation in AD, especially in pre-clinical models of AD. In addition, many studies have shown that the NF-κB signaling pathway is believed to be the central pathway of polyphenols action in regulating neuroinflammation.
Peptides
Bioactive peptides derived from various plant-derived foods have shown beneficial anti-inflammation activities (Guha and Majumder Citation2019; Wong et al. Citation2020). For example, treatment with soybean peptides Maillard reaction products (SMRPs; 200, 400, or 800 mg/kg body weight, 6 weeks), mainly containing favor peptides with different molecular weights, amino acids and volatile compounds, significantly reduced the level of pro-inflammatory cytokines, such as TNF-α, IL-1β, and IL-6 in D-galactose-induced aging mice in a dose-dependent manner (Zhang et al. Citation2020). SMRPs intake reduced AchE activity by 16.56%, 31.57%, and 44.91% at different doses, respectively, indicating SMRPs could alleviate neurocognitive impairment. The neuroprotection effect of SMRPs may be related to anti-inflammatory activity. Furthermore, some peptides have been demonstrated to possess the ability to alleviate AD. For instance, a bioactive peptide (PPKNW, 80 and 400 mg/kg body weight, 12 weeks) from walnut protein hydrolysate was found to inhibit Aβ plaques aggregation and improve cognition in APP/PS1 mice (Wang, et al. Citation2019). In addition, walnut peptides (200, 400, and 800 mg/kg body weight, 5 weeks), enzymatic hydrolysis by pancreatin, were also found to ameliorate cognitive deficits and memory impairment in Aβ25-35-induced mice, as well as improve neuroinflammation via NF-κB pathway (Zou et al. Citation2016). Thus, bioactive peptides have great potential to inhibit the progress of AD, which may be due to their anti-inflammatory activities.
A number of studies have indicated that bioactive peptides have shown promise in inhibiting inflammation, indicating their potential effect on neuroprotection (Guha and Majumder Citation2019; Wong et al. Citation2020). For example, a novel bioactive peptide FK2 (TMKLLLVTL), a 9-amino-acid-residue peptide derived from trypsin hydrolysis of corn silk, exhibited about 60% improvement on inflammation compared to the LPS-treated group, which was associated with the inhibition of the NF-κB signaling pathway (Ho et al. Citation2017). Peptides (10 mg/mL) from in vitro gastrointestinal digestion of soybean proteins displayed anti-inflammatory activity as indicated by its ability to inhibit by about 45% NO production in LPS-stimulated RAW 264.7 macrophages (González-Montoya et al. Citation2018). The walnut protein hydrolysate (666 mg/kg, 21 days) mainly composed of Glu, Arg, and Asp was found to inhibit the level of pro-inflammatory cytokines in brain, thereby ameliorating learning and memory impairment in LPS-treated mice (Wang et al. Citation2020). Future studies of these bioactive peptides should test their potentials on alleviating neurodegenerative diseases, such as AD.
The amino acid compositions of a peptide are critically important for its bioactive function (Moayedi et al. Citation2017). Most of peptides with anti-inflammatory activities were found to be rich in hydrophobic amino acids, such as P, V, L, A, F, and W (Katayama, Corpuz, and Nakamura Citation2021). In addition, the peptides LPF, DVYY, and APTLW from walnut exhibited substantial anti-inflammatory activities in LPS-treated BV-2 cells at 0.1 mM, which were partially contributed to the relative abundance of hydrophobic amino acids in peptide sequences (Wang et al. Citation2020). Similar findings were observed in the anti-inflammatory activities of the peptides GVSLLQQFFL from Mytilus coruscus and LDAVNR and MMLDF from Spirulina maxima (Vo, Ryu, and Kim Citation2013; Kim et al. Citation2013). Apart from the amino acid composition, molecular weights play a role in the bioactive function of peptides. For example, peptides of <3 kDa from Spirulina maxima have been found to exhibit the most superior anti-inflammatory activity than those of >10, 5–10, and 3–5 kDa (Vo, Ryu, and Kim Citation2013). Nevertheless, further investigation is needed to identify the precise causality between the structure of peptides and anti-neuroinflammatory activity.
Comparison among dietary patterns, whole-plant foods, and isolated major components of whole-plant foods in modulating neuroinflammation
In recent years, accumulating evidence indicates a link between lifestyles such as dietary habits and the occurrence of AD (Solfrizzi et al. Citation2017). Following some specific healthy dietary patterns such as Mediterranean diet (MeDi) and Dietary Approach to Stop Hypertension (DASH) may lower the risks of cognitive decline based on recent review and meta-analysis (Solfrizzi et al. Citation2017; Szczechowiak, Diniz, and Leszek Citation2019). Moreover, the Mediterranean-DASH Intervention for Neurodegenerative Delay (MIND) diet, a hybrid of MeDi and DASH, has been associated with dementia prevention and slows cognitive decline in observational studies (Cherian et al. Citation2019). These three dietary patterns are characterized by high consumption of fruits, vegetables, legumes and cereals, and low consumption of red meat and dairy products (Solfrizzi et al. Citation2017; Szczechowiak, Diniz, and Leszek Citation2019). The efficacy of these dietary patterns is attributed to their wide range of neuroprotective active ingredients. For example, green leafy vegetables are sources of folate, vitamin E, and flavonoids, related to a lower risk of cognitive decline (Morris et al. Citation2015). Berries, as we discussed above, are rich in polyphenols with anti-neuroinflammatory activities (Gasparrini et al. Citation2021). Nuts contain various bioactive peptides, and seafood, especially fish, are rich sources of long-chain n-3 fatty acids, which have shown Aβ cleaning and anti-oxidant activities (Morris et al. Citation2015). In contrast, milk and dairy products as the main dietary pattern had a higher risk of cognitive impairment due to high saturated fat in these foods (Kepka et al. Citation2020). Apart from kinds of nutrients, the effects of dietary patterns may also be affected by gender. For example, adherence to MeDi was inversely associated with cognitive impairment in men but positively associated in women, revealing potential behavioral differences and exposures between genders (Katsiardanis et al. Citation2013). The possible sex-specific effect needs further investigation.
When evaluating the effects of target materials on neuroinflammation, whether the effects were attributed to the consumption of whole-plant foods or major isolated components must be considered. Since each kind of whole-plant foods has complex nutrients and different proportions of nutrients, and the interactions among various compounds are complicated, the bioactivities of final products can be additive, negative, or neutral (Phan et al. Citation2018). It should be stressed that studies on potential interactions between the isolated extracts and other bioactive components in whole-plant foods are now an overlooked area, which may hinder a clear understanding of their effects. Hence, the potentiality of these interactions needs to be studied.
Nevertheless, separate studies on the anti-neuroinflammation of whole-plant foods and their bioactive components have given some valuable hints. For example, although both HE-My and HE-Et ameliorated AD in APPswe/PS1dE9 mice, the HE-My significantly reduced the level of soluble Aβ1–42 while the HE-Et did not (Tsai-Teng et al. Citation2016). Furthermore, HE-My had a more significant effect on decreasing microglia activation and improving the impairment in multiple brain regions than HE-Et. In a similar line of investigating a combined of compounds that potentially co-exist in food, the resveratrol-curcumin combination was shown to more effective on inhibiting neuroinflammation than resveratrol or curcumin alone (Zaky et al. Citation2017). In particular, the combination significantly inhibited inflammation and ameliorated aluminum chloride-induced damage in the brain that was recovered almost to regular status. These results suggest that individual parts from whole-plant foods on modulating neuroinflammation may exert synergistic effects when different components interact with others. The water extracts of coffee and ginger both had antiradical potential (Durak, Gawlik-Dziki, and Kowalska Citation2015). On examining the antioxidant ability of the water-soluble compounds of coffee in combination with ginger, a synergistic effect was observed. The results showed that the interactions between bioactive components played an important role in the bioactivity of coffee and ginger. Interestingly, coffee and ginger extracts mixtures acted antagonistically in anti-inflammation (Durak, Gawlik-Dziki, and Kowalska Citation2015). After in vitro digestion, extracts acted synergistically, suggesting digestion changed the type of interaction between bioactive compounds. Conclusively, the way of interactions is complicated, which would lead to distinct activities by different processing. However, some studies have found that a specific component may play a major role in a specific activity. For example, all the polyphenolic-protein-polysaccharide complexes from Hovenia dulcis extracted using different methods extended great antioxidant abilities in vitro, while the higher bioactivities were observed in the high content of polyphenolics in the complexes (Wu et al. Citation2020). The above findings suggest that interactions between the different components in whole-plant foods are common and extremely complex, and the results of these effects will directly impact on their bioactivities. However, the effects of interactions between different compounds found in whole-plant foods on anti-neuroinflammation remain largely unknown and warrant further investigations.
Challenges in the neuroinflammation regulation of macromolecules: novel strategies
The whole-plant foods and their macromolecules that can directly alleviate neuroinflammation in the CNS need to firstly across the BBB. The main structural and dynamic components of the BBB are capillary endothelial cells, supported by pericytes and a network of astrocytes (Curcio et al. Citation2020). Together with the efflux proteins on the BBB surface, these cells constitute a “security wall” for regulating the foreign substances to enter the brain. Different structural characteristics of foreign substances across the BBB in different ways. Namely, lipophilic ingredients in the whole-plant foods can cross the apical and basal membranes of endothelial cells by passive diffuse pathway to enter the brain (Feng Citation2002), while hydrophilic ingredients need specific cell membrane transporters such as peptide/histidine transporter 1 for carrying Gly-Sar and L-type amino acid transporter 1 for carrying Tyr-Pro to help them enter the brain (Tanaka et al. Citation2019; Čvorović et al. Citation2018). Notably, cell membrane transporters are not suitable for macromolecular ingredients, which cross the endothelium through a vesicular route by energy-independent passive penetration or energy-dependent endocytotic penetration pathways (Ciura and Dziomba Citation2020; Guidotti, Brambilla, and Rossi Citation2017). Nevertheless, evidence points to a limited degree of macromolecules enter the brain (Leclerc, Dudonné, and Calon Citation2021). The low bioavailability is a common problem of these bioactive macromolecules. Besides, easy degradation tendency, non-target, and other limitations hinder their effectiveness. Some novel molecular engineering techniques have been used to overcome these limitations. Due to their small sizes, multiple particle shapes, and transport ligands, nanoparticle-based approaches have been widely studied for bioactive components delivery, such as peptide and polyphenol (Acar et al. Citation2017; Ahmadi, Mohammadinejad, and Ashrafizadeh Citation2019). Targeted delivery effectively improves the bioaccessibility and bioavailability of the bioactive ingredients in normal intake, thereby providing a significant amount of efficacy. For instance, solid lipid NPs for oral delivery of resveratrol produced an 8.035-fold improvement in bioavailability and exhibited more significant protective effects than free resveratrol in Parkinsonian rats (Wang et al. Citation2011; Pandita et al. Citation2014). In addition, the NPs were functionalized with target-specific ligand or antibody at their surface to contribute bioactive ingredients directly reach the specific location of action without diffusion (Ashfaq et al. Citation2017). However, little is known about the delivery of bioactive components to target neuroinflammation. In this aspect, nanoparticle-based approaches may be an ideal way to improve the application of whole-plant foods or macromolecules for anti-neuroinflammation.
Apart from their direct actions on the targets, macromolecules can be utilized by gut microbiota to produce beneficial metabolites and change the composition of gut microbiota that modulate the nervous system through the gut-brain axis. A recent comprehensive review from our group on the effects of bioactive peptides on controlling neurodegenerative diseases via gut microbiota-gut-axis (Wu et al. Citation2021) demonstrated that bioactive peptides that modulate the gut microbiota could be used as a novel strategy for the control of neurodegenerative diseases. Many studies have focused on the undigested polysaccharides because their interaction with the gut microbiota is a curial way to exert their functional activities. FVP had been found to improve learning and memory impairment in scopolamine-treated mice (Su et al. Citation2018). FVP altered gut microbiota composition and inhibited inflammation. In addition, FVP modulated gut microbiota that were closely associated with the behavior. Fecal microbiota transplantation from FVP-treated mice had similar effects. The results indicated that FVP affected the gut microbiota instead of having a direct effect on the host, which inhibited inflammation and improved cognitive impairment. Therefore, natural products that improve neurodegenerative diseases by regulating gut microbiota and associated metabolites provide a new clue to reveal the potential mechanism for their functions.
There is a gap in understanding which kinds of gut microbiota and their metabolites contribute to the effects of macromolecules on regulating neuroinflammation. Currently, a major challenge that hinders our understanding of the phenomena is that it is difficult to determine the structure and content of intermediates or metabolites of macromolecules degraded by the gut microbiota and the level at which they can be available to the host. In addition, the individual or combined bacteria and intermediates/metabolites that play the role of anti-neuroinflammatory effects are still unknown and require urgent research to obtain better knowledge on these issues to design better approaches to maximize the benefits gained from these compounds. Potential solutions to close this research gap are (a) using cutting-edge technology such as Q-TOF-MS/MS to establish a reliable analysis method for determining the fine structure and quantitative detection, which will help in the elucidation of the degradation process of macromolecules; and (b) adopting gene knockout mouse model combined with multi-omics such as metagenomics, transcriptomics, and proteomics to determine the effective substances involved in the regulation of signaling pathways of anti-neuroinflammatory activity.
Conclusions
Neuroinflammation is considered to be the major driver of AD. The principal signaling pathways involved in the regulation of neuroinflammation, LAX4-NF-κB and MAPK, appear to be the most critical mechanisms exerted by dietary whole-plant foods and/or the bioactive compounds. Dietary whole-plant foods, such as mushrooms, berries, Curcuma longa L., and garlic, could effectively improve neuroinflammation by regulating the signaling targets, and therefore can be recommended as part of a diet program to prevent AD progression. Polysaccharides, polyphenols, and peptides are the three major types of bioactive components responsible for the regulatory effects of whole-plant foods on neuroinflammation. Interactions among different components in whole-plant foods may produce synergistic effects to regulate neuroinflammation, but fewer studies have been conducted. Furthermore, nanoparticle- and gut microbiota-based approaches greatly enhance the anti-neuroinflammatory activity of macromolecules and promote the discovery of effective bioactive substances for macromolecules regulating neuroinflammation, respectively. A highly interdisciplinary and dynamic approach with special consideration to the physiological relevance of the experimental models is important to further elucidate the potential mechanism of anti-neuroinflammation of whole-plant foods and their macromolecules in alleviating AD.
Author contributions
R. Huang and Z. Zhu: conceptualization, formal analysis, investigation; manuscript preparation; and visualization. Q. Wu and A.E.A. Bekhit: formal analysis, writing-review and editing. S. Wu, M. Chen and J. Wang: investigation and discussion. Yu Ding: resources, conceptualization, formal analysis, writing-review and editing, supervision, and project administration. All authors listed approved the final version of the manuscript for publication.
Disclosure statement
The authors declare no conflicts of interest.
Additional information
Funding
References
- Acar, H., J. M. Ting, S. Srivastava, J. L. LaBelle, and M. V. Tirrell. 2017. Molecular engineering solutions for therapeutic peptide delivery. Chemical Society Reviews 46 (21):6553–69. doi: 10.1039/C7CS00536A.
- Adalier, N., and H. Parker. 2016. Vitamin E, turmeric and saffron in treatment of Alzheimer’s disease. Antioxidants 5 (4):40. doi: 10.3390/antiox5040040.
- Agarwal, P., T. M. Holland, Y. Wang, D. A. Bennett, and M. C. Morris. 2019. Association of strawberries and anthocyanidin intake with alzheimer’ s dementia risk. Nutrients 11 (12):6553–69. doi: 10.3390/nu11123060. PMID:31847371.
- Ahmadi, Z., R. Mohammadinejad, and M. Ashrafizadeh. 2019. Drug delivery systems for resveratrol, a non-flavonoid polyphenol: Emerging evidence in last decades. Journal of Drug Delivery Science and Technology 51:591–604. doi: 10.1016/j.jddst.2019.03.017.
- Alasmari, F., M. A. Alshammari, A. F. Alasmari, W. A. Alanazi, and K. Alhazzani. 2018. Neuroinflammatory cytokines induce amyloid beta neurotoxicity through modulating amyloid precursor protein levels/metabolism. BioMed Research International 2018:3087475. doi: 10.1155/2018/3087475.
- Anfinogenova, N. D., M. T. Quinn, I. A. Schepetkin, and D. N. Atochin. 2020. Alarmins and c-Jun N-terminal kinase (JNK) signaling in neuroinflammation. Cells 9 (11):2350. doi: 10.3390/cells9112350.
- Ashfaq, U. A., M. Riaz, E. Yasmeen, and M. Z. Yousaf. 2017. Recent advances in nanoparticle-based targeted drug-delivery systems against cancer and role of tumor microenvironment. Critical Reviews in Therapeutic Drug Carrier Systems 34 (4):317–53. doi: 10.1615/CritRevTherDrugCarrierSyst.2017017845.
- Bennett, L., C. Kersaitis, S. L. Macaulay, G. Münch, G. Niedermayer, J. Nigro, M. Payne, P. Sheean, P. Vallotton, D. Zabaras, et al. 2013. Vitamin D2-enriched button mushroom (Agaricus bisporus) improves memory in both wild type and APPswe/PS1dE9 transgenic mice. PLoS One 8 (10):e76362. doi: 10.1371/journal.pone.0076362.
- Berry, A., B. Collacchi, R. Masella, R. Varì, and F. Cirulli. 2021. Curcuma Longa, the “golden spice” to counteract neuroinflammaging and cognitive decline-what have we learned and what needs to be done. Nutrients 13 (5):1519. doi: 10.3390/nu13051519.
- Boespflug, E. L., J. C. Eliassen, J. A. Dudley, M. D. Shidler, W. Kalt, S. S. Summer, A. L. Stein, A. N. Stover, and R. Krikorian. 2018. Enhanced neural activation with blueberry supplementation in mild cognitive impairment. Nutritional Neuroscience 21 (4):297–305. doi: 10.1080/1028415X.2017.1287833.
- Busche, M. A., and B. T. Hyman. 2020. Synergy between amyloid-β and tau in Alzheimer’s disease. Nature Neuroscience 23 (10):1183–93. doi: 10.1038/s41593-020-0687-6.
- Cai, L., S. Zou, D. Liang, and L. Luan. 2018. Structural characterization, antioxidant and hepatoprotective activities of polysaccharides from Sophorae tonkinensis Radix. Carbohydrate Polymers 184:354–65. doi: 10.1016/j.carbpol.2017.12.083.
- Cai, Q., Y. Y. Li, and G. Pei. 2017. Polysaccharides from Ganoderma lucidum attenuate microglia-mediated neuroinflammation and modulate microglial phagocytosis and behavioural response. Journal of Neuroinflammation 14 (1):63. doi: 10.1186/s12974-017-0839-0.
- Cherian, L., Y. Wang, K. Fakuda, S. Leurgans, N. Aggarwal, and M. Morris. 2019. Mediterranean-Dash intervention for neurodegenerative delay (MIND) diet slows cognitive decline after stroke. The Journal of Prevention of Alzheimer’s Disease 6 (4):267–73. doi: 10.14283/jpad.2019.28.
- Ciura, K., and S. Dziomba. 2020. Application of separation methods for in vitro prediction of blood–brain barrier permeability—The state of the art. Journal of Pharmaceutical and Biomedical Analysis 177:112891. doi: 10.1016/j.jpba.2019.112891.
- Clària, J., and C. N. Serhan. 1995. Aspirin triggers previously undescribed bioactive eicosanoids by human endothelial cell-leukocyte interactions. Proceedings of the National Academy of Sciences of the United States of America 92 (21):9475–9. doi: 10.1073/pnas.92.21.9475.
- Colín-González, A. L., R. A. Santana, C. A. Silva-Islas, M. E. Chánez-Cárdenas, A. Santamaría, and P. D. Maldonado. 2012. The antioxidant mechanisms underlying the aged garlic extract- and S-allylcysteine-induced protection. Oxidative Medicine and Cellular Longevity 2012:907162. doi: 10.1155/2012/907162.
- Cooper, E. L., and M. J. Ma. 2017. Alzheimer disease: Clues from traditional and complementary medicine. Journal of Traditional and Complementary Medicine 7 (4):380–5. doi: 10.1016/j.jtcme.2016.12.003.
- Curcio, M., G. Cirillo, J. R. C. Rouaen, F. Saletta, F. P. Nicoletta, O. Vittorio, and F. Iemma. 2020. Natural polysaccharide carriers in brain delivery: Challenge and perspective. Pharmaceutics 12 (12):1183. doi: 10.3390/pharmaceutics12121183.
- Čvorović, J., L. Ziberna, S. Fornasaro, F. Tramer, and S. Passamonti. 2018. Chapter 22: Bioavailability of flavonoids: The role of cell membrane transporters. In Polyphenols: Mechanisms of action in human health and disease. 2nd ed., eds. R. R. Watson, V. R. Preedy and S. Zibadi. Cambridge, MA: Academic Press.
- Dajas, F., J. A. Abin-Carriquiry, F. Arredondo, F. Blasina, C. Echeverry, M. Martinez, F. Rivera, and L. Vaamonde. 2015. Quercetin in brain diseases: Potential and limits. Neurochemistry International 89:140–8. doi: 10.1016/j.neuint.2015.07.002.
- de Los Reyes Corrales, T., M. Losada-Pérez, and S. Casas-Tintó. 2021. JNK pathway in CNS pathologies. International Journal of Molecular Sciences 22 (8):3883. doi: 10.3390/ijms22083883.
- Decourt, B., D. K. Lahiri, and M. N. Sabbagh. 2017. Targeting tumor necrosis factor alpha for Alzheimer’s disease. Current Alzheimer Research 14 (4):412–25. doi: 10.2174/1567205013666160930110551.
- Deng, Z., C. Yuan, J. Yang, Y. Peng, W. Wang, Y. Wang, and W. Gao. 2019. Behavioral defects induced by chronic social defeat stress are protected by Momordica charantia polysaccharides via attenuation of JNK3/P I3K/AKT neuroinflammatory pathway. Annals of Translational Medicine 7 (1):6. doi: 10.21037/atm.2018.12.08.
- Domingues, C., O. A. B. da Cruz e Silva, and A. G. Henriques. 2017. Impact of cytokines and chemokines on Alzheimer’s disease neuropathological hallmarks. Current Alzheimer Research 14 (8):870–82. doi: 10.2174/1567205014666170317113606.
- Domínguez-Avila, J. A., E. Alvarez-Parrilla, J. A. Lopez-Diaz, I. E. Maldonado-Mendoza, M. del C. Gómez-Garcia, and L. A. de la Rosa. 2015. The pecan nut (Carya illinoinensis) and its oil and polyphenolic fractions differentially modulate lipid metabolism and the antioxidant enzyme activities in rats fed high-fat diets. Food Chemistry 168:529–37. doi: 10.1016/j.foodchem.2014.07.092.
- Dunn, H. C., R. R. Ager, D. Baglietto-Vargas, D. Cheng, M. Kitazawa, D. H. Cribbs, and R. Medeiros. 2015. Restoration of lipoxin A4 signaling reduces Alzheimer’s disease-like pathology in the 3xTg-AD mouse model. Journal of Alzheimer’s Disease: JAD 43 (3):893–903. doi: 10.3233/JAD-141335.
- Durak, A., U. Gawlik-Dziki, and I. Kowalska. 2015. Coffee with ginger—Interactions of biologically active phytochemicals in the model system. Food Chemistry 166:261–9. doi: 10.1016/j.foodchem.2014.06.075.
- Eun, C. S., J. S. Lim, J. Lee, S. P. Lee, and S. A. Yang. 2017. The protective effect of fermented Curcuma longa L. on memory dysfunction in oxidative stress-induced C6 gliomal cells, proinflammatory-activated BV2 microglial cells, and scopolamine-induced amnesia model in mice. BMC Complementary and Alternative Medicine 17 (1):367. doi: 10.1186/s12906-017-1880-3.
- Falcicchia, C., F. Tozzi, O. Arancio, D. M. Watterson, and N. Origlia. 2020. Involvement of p38 MAPK in synaptic function and dysfunction. International Journal of Molecular Sciences 21 (16):5624. doi: 10.3390/ijms21165624.
- Feng, M. R. 2002. Assessment of blood-brain barrier penetration: In silico, in vitro and in vivo. Current Drug Metabolism 3 (6):647–57. doi: 10.2174/1389200023337063.
- Feng, S. T., Z. Z. Wang, Y. H. Yuan, H. M. Sun, N. H. Chen, and Y. Zhang. 2019. Mangiferin: A multipotent natural product preventing neurodegeneration in Alzheimer’s and Parkinson’s disease models. Pharmacological Research 146:104336. doi: 10.1016/j.phrs.2019.104336.
- Flores, G. 2017. Curcuma longa L. extract improves the cortical neural connectivity during the aging process. Neural Regeneration Research 12 (6):875–80. doi: 10.4103/1673-5374.208542.
- Fu, J., Z. Wang, L. Huang, S. Zheng, D. Wang, S. Chen, H. Zhang, and S. Yang. 2014. Review of the botanical characteristics, phytochemistry, and pharmacology of Astragalus membranaceus (Huangqi). Phytotherapy Research : PTR 28 (9):1275–83. doi: 10.1002/ptr.5188.
- Gasparrini, M., F. Giampieri, T. Y. Forbes-Hernandez, S. Afrin, D. Cianciosi, P. Reboredo-Rodriguez, A. Varela-Lopez, J. J. Zhang, J. L. Quiles, B. Mezzetti, et al. 2018. Strawberry extracts efficiently counteract inflammatory stress induced by the endotoxin lipopolysaccharide in Human Dermal Fibroblast. Food and Chemical Toxicology : An International Journal Published for the British Industrial Biological Research Association 114:128–40. doi: 10.1016/j.fct.2018.02.038.
- Gasparrini, M., T. Y. Forbes-Hernandez, D. Cianciosi, J. L. Quiles, B. Mezzetti, J. Xiao, F. Giampieri, and M. Battino. 2021. The efficacy of berries against lipopolysaccharide-induced inflammation: A review. Trends in Food Science & Technology doi: 10.1016/j.tifs.2021.01.015.
- Ge, J. C., X. Q. Zha, C. Y. Nie, N. J. Yu, Q. M. Li, D. Y. Peng, J. Duan, L. H. Pan, and J. P. Luo. 2018. Polysaccharides from Dendrobium huoshanense stems alleviates lung inflammation in cigarette smoke-induced mice. Carbohydrate Polymers 189:289–95. doi:10.1016/j.carbpol.2018.02.054.
- Gee, M. S., S. H. Son, S. H. Jeon, J. Do, N. Kim, Y.-J. Ju, S. J. Lee, E. K. Chung, K.-S. Inn, N.-J. Kim, et al. 2020. A selective p38α/β MAPK inhibitor alleviates neuropathology and cognitive impairment, and modulates microglia function in 5XFAD mouse. Alzheimer’s Research & Therapy 12 (1):45. doi:10.1186/s13195-020-00617-2.
- González-Montoya, M., B. Hernández-Ledesma, J. M. Silván, R. Mora-Escobedo, and C. Martínez-Villaluenga. 2018. Peptides derived from in vitro gastrointestinal digestion of germinated soybean proteins inhibit human colon cancer cells proliferation and inflammation. Food Chemistry 242:75–82. doi: 10.1016/j.foodchem.2017.09.035.
- Guha, S., and K. Majumder. 2019. Structural-features of food-derived bioactive peptides with anti-inflammatory activity: A brief review. Journal of Food Biochemistry 43 (1):e12531. doi:10.1111/jfbc.12531.
- Guidotti, G., L. Brambilla, and D. Rossi. 2017. Cell-penetrating peptides: From basic research to clinics. Trends in Pharmacological Sciences 38 (4):406–24. doi:10.1016/j.tips.2017.01.003.
- Guo, Y. J., W. W. Pan, S. B. Liu, Z. F. Shen, Y. Xu, and L. L. Hu. 2020. ERK/MAPK signalling pathway and tumorigenesis. Experimental and Therapeutic Medicine 19 (3):1997–2007. doi: 10.3892/etm.2020.8454.
- Gupta, R., M. Singh, and A. Sharma. 2003. Neuroprotective effect of antioxidants on ischaemia and reperfusion-induced cerebral injury. Pharmacological Research 48 (2):209–15. doi:10.1016/s1043-6618(03)00102-6.
- Hamelin, L., J. Lagarde, G. Dorothée, C. Leroy, M. Labit, R. A. Comley, L. C. de Souza, H. Corne, L. Dauphinot, M. Bertoux, et al. 2016. Early and protective microglial activation in Alzheimer’s disease: A prospective study using 18F-DPA-714 PET imaging. Brain : a Journal of Neurology 139 (Pt 4):1252–64. doi:10.1093/brain/aww017.
- Hansen, D. V., J. E. Hanson, and M. Sheng. 2018. Microglia in Alzheimer’s disease. Journal of Cell Biology 217 (2):459–72. doi: 10.1083/jcb.201709069.
- Hashimoto, S., and T. C. Saido. 2018. Critical review: Involvement of endoplasmic reticulum stress in the aetiology of Alzheimer’s disease. Open Biology 8 (4):180024. doi:10.1098/rsob.180024.
- Hepp Rehfeldt, S. C., F. Majolo, M. I. Goettert, and S. Laufer. 2020. c-Jun N-Terminal Kinase inhibitors as potential leads for new therapeutics for Alzheimer’s diseases. International Journal of Molecular Sciences 21 (24):9677. doi: 10.3390/ijms21249677.
- Hilliard, A., P. Mendonca, and K. F. A. Soliman. 2020. Involvement of NF-κB and MAPK signaling pathways in the preventive effects of Ganoderma lucidum on the inflammation of BV-2 microglial cells induced by LPS. Journal of Neuroimmunology 345:577269. doi:10.1016/j.jneuroim.2020.577269.
- Ho, S. C., and M. S. Su. 2014. Evaluating the anti-neuroinflammatory capacity of raw and steamed garlic as well as five organosulfur compounds. Molecules 19 (11):17697–714. doi: 10.3390/molecules191117697.
- Ho, T. Y., C. C. Li, H. Y. Lo, F. Y. Chen, and C. Y. Hsiang. 2017. Corn silk extract and its bioactive peptide ameliorated lipopolysaccharide-induced inflammation in mice via the nuclear factor-kappaB signaling pathway. Journal of Agricultural and Food Chemistry 65 (4):759–68. doi: 10.1021/acs.jafc.6b03327.
- Hu, T., Q. Lin, T. Guo, T. Yang, W. Zhou, X. Deng, J. K. Yan, Y. Luo, M. Ju, and F. Luo. 2018. Polysaccharide isolated from Phellinus linteus mycelia exerts anti-inflammatory effects via MAPK and PPAR signaling pathways. Carbohydrate Polymers 200:487–97. doi:10.1016/j.carbpol.2018.08.021.
- Huang, Y. C., H. J. Tsay, M. K. Lu, C. H. Lin, C. W. Yeh, H. K. Liu, and Y. J. Shiao. 2017. Astragalus membranaceus-polysaccharides ameliorates obesity, hepatic steatosis, neuroinflammation and cognition impairment without affecting amyloid deposition in metabolically stressed APPswe/PS1dE9 mice. International Journal of Molecular Sciences 18 (12):2746. doi:10.3390/ijms18122746.
- Huyut, Z., S. Beydemir, and I. Gulcin. 2017. Antioxidant and antiradical properties of selected flavonoids and phenolic compounds. Biochemistry Research International 2017:7616791. doi:10.1155/2017/7616791.
- Infante-Garcia, C., J. J. Ramos-Rodriguez, I. Delgado-Olmos, C. Gamero-Carrasco, M. T. Fernandez-Ponce, L. Casas, C. Mantell, and M. Garcia-Alloza. 2017. Long-term Mangiferin extract treatment improves central pathology and cognitive deficits in APP/PS1 Mice. Molecular Neurobiology 54 (6):4696–704. doi:10.1007/s12035-016-0015-z.
- Jaén, R. I., S. Sánchez-García, M. Fernández-Velasco, L. Boscá, and P. Prieto. 2021. Resolution-based therapies: The potential of lipoxins to treat human diseases. Frontiers in Immunology 12:658840. doi:10.3389/fimmu.2021.658840.
- Jayaraj, R. L., S. Azimullah, R. Beiram, F. Y. Jalal, and G. A. Rosenberg. 2019. Neuroinflammation: Friend and foe for ischemic stroke. Journal of Neuroinflammation 16 (1):142. doi:10.1186/s12974-019-1516-2.
- Jin, X., M. Y. Liu, D. F. Zhang, X. Zhong, K. Du, P. Qian, H. Gao, and M. J. Wei. 2019. Natural products as a potential modulator of microglial polarization in neurodegenerative diseases. Pharmacological Research 145:104253. doi:10.1016/j.phrs.2019.104253.
- Jorda, A., J. Campos-Campos, A. Iradi, M. Aldasoro, C. Aldasoro, J. M. Vila, and S. L. Valles. 2020. The role of chemokines in Alzheimer’s disease. Endocrine, Metabolic & Immune Disorders - Drug Targets 20 (9):1383–90. doi: 10.2174/1871530320666200131110744.
- Ju Hwang, C., D. Y. Choi, M. H. Park, and J. T. Hong. 2019. NF-κB as a key mediator of brain inflammation in Alzheimer’s disease. CNS & Neurological Disorders Drug Targets 18 (1):3–10. doi:10.2174/1871527316666170807130011.
- Kantarci, A., N. Aytan, I. Palaska, D. Stephens, L. Crabtree, C. Benincasa, B. G. Jenkins, I. Carreras, and A. Dedeoglu. 2018. Combined administration of resolvin E1 and lipoxin A4 resolves inflammation in a murine model of Alzheimer’s disease. Experimental Neurology 300:111–20. doi: 10.1016/j.expneurol.2017.11.005.
- Katayama, S., H. M. Corpuz, and S. Nakamura. 2021. Potential of plant-derived peptides for the improvement of memory and cognitive function. Peptides 142:170571. doi:10.1016/j.peptides.2021.170571.
- Katsiardanis, K., A. A. Diamantaras, N. Dessypris, T. Michelakos, A. Anastasiou, K. P. Katsiardani, P. Kanavidis, F. C. Papadopoulos, C. Stefanadis, D. B. Panagiotakos, et al. 2013. Cognitive impairment and dietary habits among elders: The Velestino Study. Journal of Medicinal Food 16 (4):343–50. doi: 10.1089/jmf.2012.0225.
- Kaur, D., V. Sharma, and R. Deshmukh. 2019. Activation of microglia and astrocytes: A roadway to neuroinflammation and Alzheimer’s disease. Inflammopharmacology 27 (4):663–77. doi:10.1007/s10787-019-00580-x.
- Kepka, A., A. Ochocinska, M. Borzym-Kluczyk, E. Skorupa, B. Stasiewicz-Jarocka, S. Chojnowska, and N. Waszkiewicz. 2020. Preventive role of L-carnitine and balanced diet in Alzheimer’s disease. Nutrients 12 (7):1987. doi:10.3390/nu12071987.
- Khan, A., T. Ali, S. U. Rehman, M. S. Khan, S. I. Alam, M. Ikram, T. Muhammad, K. Saeed, H. Badshah, and M. O. Kim. 2018. Neuroprotective effect of quercetin against the detrimental effects of LPS in the adult mouse brain. Frontiers in Pharmacology 9:1383. doi:10.3389/fphar.2018.01383.
- Khan, M. S., T. Muhammad, M. Ikram, and M. O. Kim. 2019. Dietary supplementation of the antioxidant curcumin halts systemic LPS-induced neuroinflammation-associated neurodegeneration and memory/synaptic impairment via the JNK/NF-κB/Akt signaling pathway in adult rats. Oxidative Medicine and Cellular Longevity 2019:7860650. doi:10.1155/2019/7860650.
- Kheiri, G., M. Dolatshahi, F. Rahmani, and N. Rezaei. 2018. Role of p38/MAPKs in Alzheimer’s disease: Implications for amyloid beta toxicity targeted therapy. Reviews in the Neurosciences 30 (1):9–30. doi:10.1515/revneuro-2018-0008.
- Khursheed, R., S. K. Singh, S. Wadhwa, M. Gulati, and A. Awasthi. 2020. Therapeutic potential of mushrooms in diabetes mellitus: Role of polysaccharides. International Journal of Biological Macromolecules 164:1194–205. doi:10.1016/j.ijbiomac.2020.07.145.
- Kim, E. K., Y. S. Kim, J. W. Hwang, S. H. Kang, D. K. Choi, K. H. Lee, J. S. Lee, S. H. Moon, B. T. Jeon, and P. J. Park. 2013. Purification of a novel nitric oxide inhibitory peptide derived from enzymatic hydrolysates of Mytilus coruscus. Fish & Shellfish Immunology 34 (6):1416–20. doi:10.1016/j.fsi.2013.02.023.
- Krikorian, R., M. D. Shidler, T. A. Nash, W. Kalt, M. R. Vinqvist-Tymchuk, B. Shukitt-Hale, and J. A. Joseph. 2010. Blueberry supplementation improves memory in older adults. Journal of Agricultural and Food Chemistry 58 (7):3996–4000. doi:10.1021/jf9029332.
- Kumar, A., A. Prakash, and S. Dogra. 2011. Protective effect of curcumin (Curcuma longa) against D-galactose-induced senescence in mice. Journal of Asian Natural Products Research 13 (1):42–55. doi: 10.1080/10286020.2010.544253.
- Kushairi, N., C. W. Phan, V. Sabaratnam, P. David, and M. Naidu. 2019. Lion’s mane mushroom, Hericium erinaceus (Bull.: Fr.) Pers. suppresses H2O2-induced oxidative damage and LPS-induced inflammation in HT22 hippocampal neurons and BV2 microglia. Antioxidants (Basel) 8 (8):261. doi: 10.3390/antiox8080261.
- Kushairi, N., N. A. K. A. Tarmizi, C. W. Phan, I. Macreadie, V. Sabaratnam, M. Naidu, and P. David. 2020. Modulation of neuroinflammatory pathways by medicinal mushrooms, with particular relevance to Alzheimer’s disease. Trends in Food Science & Technology 104:153–62. doi: 10.1016/j.tifs.2020.07.029.
- Lam, C. S., G. L. Tipoe, K. F. So, and M. L. Fung. 2015. Neuroprotective mechanism of Lycium barbarum polysaccharides against hippocampal-dependent spatial memory deficits in a rat model of obstructive sleep apnea. PLoS One 10 (2):e0117990. e0117990. doi: 10.1371/journal.pone.0117990.
- Lau, S. F., A. K. Y. Fu, and N. Y. Ip. 2021. Cytokine signaling convergence regulates the microglial state transition in Alzheimer’s disease. Cellular and Molecular Life Sciences: CMLS 78 (10):4703–12. doi:10.1007/s00018-021-03810-0.
- Leclerc, M., S. Dudonné, and F. Calon. 2021. Can natural products exert neuroprotection without crossing the blood-brain barrier?International Journal of Molecular Sciences 22 (7):3356. doi: 10.3390/ijms22073356.
- Lee, J. K., and N. J. Kim. 2017. Recent advances in the inhibition of p38 MAPK as a potential strategy for the treatment of Alzheimer’s disease. Molecules (Basel, Switzerland) 22 (8):1287. doi: 10.3390/molecules22081287.
- Leng, F., and P. Edison. 2021. Neuroinflammation and microglial activation in Alzheimer disease: Where do we go from here?Nature Reviews. Neurology 17 (3):157–72. doi: 10.1038/s41582-020-00435-y.
- Li, Z., X. He, F. Liu, J. Wang, and J. Feng. 2018. A review of polysaccharides from Schisandra chinensis and Schisandra sphenanthera: Properties, functions and applications. Carbohydrate Polymers 184:178–90. doi: 10.1016/j.carbpol.2017.12.058.
- Lin, G., X. Li, X. Cheng, N. Zhao, and W. Zheng. 2020. Manganese exposure aggravates beta-amyloid pathology by microglial activation. Frontiers in Aging Neuroscience 12:556008. doi:10.3389/fnagi.2020.556008.
- Liu, D., and D. Du. 2020. Mulberry fruit extract alleviates cognitive impairment by promoting the clearance of amyloid-beta and inhibiting neuroinflammation in Alzheimer’s disease mice. Neurochemical Research 45 (9):2009–19. doi: 10.1007/s11064-020-03062-7.
- Liu, M., S. Chen, Y. Shang, and S. Yao. 2018. Aspirin-triggered lipoxin A4 attenuates lipopolysaccharide-induced acute lung injury by inhibiting activation of mitogen-activated protein kinases and NF-κB in mice. International Journal of Clinical and Experimental Pathology 11 (5):2570–8. doi:
- Long, J. M., and D. M. Holtzman. 2019. Alzheimer disease: An update on pathobiology and treatment strategies. Cell 179 (2):312–39. doi:10.1016/j.cell.2019.09.001.
- Lu, X., M. A. Brennan, L. Serventi, J. Liu, W. Guan, and C. S. Brennan. 2018. Addition of mushroom powder to pasta enhances the antioxidant content and modulates the predictive glycaemic response of pasta. Food Chemistry 264:199–209. doi: 10.1016/j.foodchem.2018.04.130.
- Lu, Z., H. Zhang, X. Zhang, Y. Gao, and Z. Q. Yin. 2019. Lipoxin A4 delays the progression of retinal degeneration via the inhibition of microglial overactivation. Biochemical and Biophysical Research Communications 516 (3):900–6. doi: 10.1016/j.bbrc.2019.06.137.
- Luo, T., J. Qin, M. Liu, J. Luo, F. Ding, M. Wang, and L. Zheng. 2015. Astragalus polysaccharide attenuates lipopolysaccharide-induced inflammatory responses in microglial cells: Regulation of protein kinase B and nuclear factor-kappa B signaling. Inflammation Research : official Journal of the European Histamine Research Society. [et al.] 64 (3-4):205–12. doi: 10.1007/s00011-015-0798-9.
- Ma, Z. F., H. Zhang, S. S. Teh, C. W. Wang, Y. Zhang, F. Hayford, L. Wang, T. Ma, Z. Dong, Y. Zhang, et al. 2019. Goji berries as a potential natural antioxidant medicine: An insight into their molecular mechanisms of action. Oxidative Medicine and Cellular Longevity 2019:2437397. doi: 10.1155/2019/2437397.
- Medeiros, R., M. Kitazawa, G. F. Passos, D. Baglietto-Vargas, D. Cheng, D. H. Cribbs, and F. M. LaFerla. 2013. Aspirin-triggered lipoxin A4 stimulates alternative activation of microglia and reduces Alzheimer disease-like pathology in mice. The American Journal of Pathology 182 (5):1780–9. doi: 10.1016/j.ajpath.2013.01.051.
- Miller, M. G., D. A. Hamilton, J. A. Joseph, and B. Shukitt-Hale. 2018. Dietary blueberry improves cognition among older adults in a randomized, double-blind, placebo-controlled trial. European Journal of Nutrition 57 (3):1169–80. doi:10.1007/s00394-017-1400-8.
- Moayedi, A., L. Mora, M.-C. Aristoy, M. Hashemi, M. Safari, and F. Toldrá. 2017. ACE-inhibitory and antioxidant activities of peptide fragments obtained from tomato processing by-products fermented using Bacillus subtilis: Effect of amino acid composition and peptides molecular mass distribution. Applied Biochemistry and Biotechnology 181 (1):48–64. doi: 10.1007/s12010-016-2198-1.
- Morris, M. C., C. C. Tangney, Y. Wang, F. M. Sacks, L. L. Barnes, D. A. Bennett, and N. T. Aggarwal. 2015. MIND diet slows cognitive decline with aging. Alzheimer’s & Dementia 11 (9):1015–22. doi: 10.1016/j.jalz.2015.04.011.
- Moussa, C., M. Hebron, X. Huang, J. Ahn, R. A. Rissman, P. S. Aisen, and R. S. Turner. 2017. Resveratrol regulates neuro-inflammation and induces adaptive immunity in Alzheimer’s disease. Journal of Neuroinflammation 14 (1) doi: 10.1186/s12974-016-0779-0.
- Musi, C. A., G. Agrò, F. Santarella, E. Iervasi, and T. Borsello. 2020. JNK3 as therapeutic target and biomarker in neurodegenerative and neurodevelopmental brain diseases. Cells 9 (10):2190. doi: 10.3390/cells9102190.
- Muszyńska, B., A. Grzywacz-Kisielewska, K. Kała, and J. Gdula-Argasińska. 2018. Anti-inflammatory properties of edible mushrooms: A review. Food Chemistry 243:373–81. doi: 10.1016/j.foodchem.2017.09.149.
- Nillert, N., W. Pannangrong, J. U. Welbat, W. Chaijaroonkhanarak, K. Sripanidkulchai, and B. Sripanidkulchai. 2017. Neuroprotective effects of aged garlic extract on cognitive dysfunction and neuroinflammation induced by beta-Amyloid in rats. Nutrients 9 (1):24. doi: 10.3390/nu9010024.
- Olajide, O. A., and S. D. Sarker. 2020. Alzheimer’s disease: Natural products as inhibitors of neuroinflammation. Inflammopharmacology 28 (6):1439–55. doi:10.1007/s10787-020-00751-1.
- Pandita, D., S. Kumar, N. Poonia, and V. Lather. 2014. Solid lipid nanoparticles enhance oral bioavailability of resveratrol, a natural polyphenol. Food Research International 62:1165–74. doi: 10.1016/j.foodres.2014.05.059.
- Phan, M. A. T., J. Paterson, M. Bucknall, and J. Arcot. 2018. Interactions between phytochemicals from fruits and vegetables: Effects on bioactivities and bioavailability. Critical Reviews in Food Science and Nutrition 58 (8):1310–29. doi: 10.1080/10408398.2016.1254595.
- Plastira, I., E. Bernhart, L. Joshi, C. N. Koyani, H. Strohmaier, H. Reicher, E. Malle, and W. Sattler. 2020. MAPK signaling determines lysophosphatidic acid (LPA)-induced inflammation in microglia. Journal of Neuroinflammation 17 (1):127. doi: 10.1186/s12974-020-01809-1.
- Potì, F., D. Santi, G. Spaggiari, F. Zimetti, and I. Zanotti. 2019. Polyphenol health effects on cardiovascular and neurodegenerative disorders: A review and meta-analysis. International Journal of Molecular Sciences 20 (2):351. doi: 10.3390/ijms20020351.
- Qi, B., C. Shi, J. Meng, S. Xu, and J. Liu. 2018. Resveratrol alleviates ethanol-induced neuroinflammation in vivo and in vitro: Involvement of TLR2-MyD88-NF-κB pathway. The International Journal of Biochemistry & Cell Biology 103:56–64. doi: 10.1016/j.biocel.2018.07.007.
- Rodriguez-Vieitez, E., L. Saint-Aubert, S. F. Carter, O. Almkvist, K. Farid, M. Schöll, K. Chiotis, S. Thordardottir, C. Graff, A. Wall, et al. 2016. Diverging longitudinal changes in astrocytosis and amyloid PET in autosomal dominant Alzheimer’s disease. Brain 139 (Pt 3):922–36. doi: 10.1093/brain/awv404.
- Romano, M., E. Cianci, F. Simiele, and A. Recchiuti. 2015. Lipoxins and aspirin-triggered lipoxins in resolution of inflammation. European Journal of Pharmacology 760:49–63. doi: 10.1016/j.ejphar.2015.03.083.
- Russo, D. 2018. Flavonoids and the structure-antioxidant activity relationship. Journal of Pharmacognosy & Natural Products 4 (1):1000e109. doi: 10.4172/2472-0992.1000e109.
- Rutledge, G. A., D. R. Fisher, M. G. Miller, M. E. Kelly, D. F. Bielinski, and B. Shukitt-Hale. 2019. The effects of blueberry and strawberry serum metabolites on age-related oxidative and inflammatory signaling in vitro. Food & Function 10 (12):7707–13. doi: 10.1039/C9FO01913H.
- Sandhu, A. K., M. G. Miller, N. Thangthaeng, T. M. Scott, B. Shukitt-Hale, I. Edirisinghe, and B. Burton-Freeman. 2018. Metabolic fate of strawberry polyphenols after chronic intake in healthy older adults. Food & Function 9 (1):96–106. doi:10.1039/c7fo01843f.
- Sandhu, A., M. G. Miller, B. Shukitt-Hale, I. Edirisinghe, and B. Burton-Freeman. 2017. Metabolic fate of blueberry anthocyanins after chronic supplementation in healthy older adults. FASEB Journal 31:646.
- Sato, S., and Y. Mukai. 2020. Modulation of chronic inflammation by quercetin: The beneficial effects on obesity. Journal of Inflammation Research 13:421–31. doi:10.2147/JIR.S228361.
- Serhan, C. N., M. Hamberg, and B. Samuelsson. 1984. Lipoxins: Novel series of biologically active compounds formed from arachidonic acid in human leukocytes. Proceedings of the National Academy of Sciences of the United States of America 81 (17):5335–9. doi:10.1073/pnas.81.17.5335.
- Serhan, C. N., and K. A. Sheppard. 1990. Lipoxin formation during human neutrophil-platelet interactions. Evidence for the transformation of leukotriene A4 by platelet 12-lipoxygenase in vitro. Journal of Clinical Investigation 85 (3):772–80. doi: 10.1172/JCI114503.
- Serra, D., L. M. Almeida, and T. C. P. Dinis. 2018. Dietary polyphenols: A novel strategy to modulate microbiota-gut-brain axis. Trends in Food Science & Technology 78:224–33. doi: 10.1016/j.tifs.2018.06.007.
- Sharma, P., P. Srivastava, A. Seth, P. N. Tripathi, A. G. Banerjee, and S. K. Shrivastava. 2019. Comprehensive review of mechanisms of pathogenesis involved in Alzheimer’s disease and potential therapeutic strategies. Progress in Neurobiology 174:53–89. doi: 10.1016/j.pneurobio.2018.12.006.
- Shavandi, A., A. E. A. Bekhit, P. Saeedi, Z. Izadifar, A. A. Bekhit, and A. Khademhosseini. 2018. Polyphenol uses in biomaterials engineering. Biomaterials 167:91–106. doi: 10.1016/j.biomaterials.2018.03.018.
- Shi, J., M. N. Sabbagh, and B. Vellas. 2020. Alzheimer’s disease beyond amyloid: Strategies for future therapeutic interventions. BMJ (Clinical Research ed.) 371:m3684. doi: 10.1136/bmj.m3684.
- Shukitt-Hale, B., N. Thangthaeng, M. G. Miller, S. M. Poulose, A. N. Carey, and D. R. Fisher. 2019. Blueberries improve neuroinflammation and cognition differentially depending on individual cognitive baseline status. Journals of Gerontology Series A-Biological Sciences and Medical Sciences 74 (7):977–83. doi: 10.1093/gerona/glz048.
- Snyder, B., B. Shell, J. T. Cunningham, and R. L. Cunningham. 2017. Chronic intermittent hypoxia induces oxidative stress and inflammation in brain regions associated with early-stage neurodegeneration. Physiological Reports 5 (9):e13258. doi: 10.14814/phy2.13258.
- Solfrizzi, V., C. Custodero, M. Lozupone, B. P. Imbimbo, V. Valiani, P. Agosti, A. Schilardi, A. D’Introno, M. L. Montagna, M. Calvani, et al. 2017. Relationships of dietary patterns, foods, and micro- and macronutrients with Alzheimer’s disease and late-life cognitive disorders: A systematic review. Journal of Alzheimer’s Disease: JAD 59 (3):815–49. doi: 10.3233/JAD-170248.
- Song, H., J. Cui, V. V. Mossine, C. M. Greenlief, K. Fritsche, G. Y. Sun, and Z. Gu. 2020. Bioactive components from garlic on brain resiliency against neuroinflammation and neurodegeneration. Experimental and Therapeutic Medicine 19 (2):1554–9. doi: 10.3892/etm.2019.8389.
- Song, H., Y. Lu, Z. Qu, V. V. Mossine, M. B. Martin, J. Hou, J. Cui, B. A. Peculis, T. P. Mawhinney, J. Cheng, et al. 2016. Effects of aged garlic extract and FruArg on gene expression and signaling pathways in lipopolysaccharide-activated microglial cells. Scientific Reports 6:35323. doi: 10.1038/srep35323.
- Sripanidkulchai, B. 2020. Benefits of aged garlic extract on Alzheimer’s disease: Possible mechanisms of action. Experimental and Therapeutic Medicine 19 (2):1560–4. doi: 10.3892/etm.2019.8390.
- Su, A., W. Yang, L. Zhao, F. Pei, B. Yuan, L. Zhong, G. Ma, and Q. Hu. 2018. Flammulina velutipes polysaccharides improve scopolamine-induced learning and memory impairment in mice by modulating gut microbiota composition. Food & Function 9 (3):1424–32. doi: 10.1039/c7fo01991b.
- Sun, S. C. 2017. The non-canonical NF-kappaB pathway in immunity and inflammation. Nature Reviews. Immunology 17 (9):545–58. doi: 10.1038/nri.2017.52.
- Sun, X. Y., Q. X. Dong, J. Zhu, X. Sun, L. F. Zhang, M. Qiu, X. L. Yu, and R. T. Liu. 2019. Resveratrol rescues tau-induced cognitive deficits and neuropathology in a mouse model of tauopathy. Current Alzheimer Research 16 (8):710–22. doi: 10.2174/1567205016666190801153751.
- Sunny, A., K. Ramalingam, S. S. Das, B. Maliakel, I. M. Krishnakumar, and S. Ittiyavirah. 2019. Bioavailable curcumin alleviates lipopolysaccharide-induced neuroinflammation and improves cognition in experimental animals. Pharmacognosy Magazine 15:S111–S7. doi: 10.4103/pm.pm_307_18.
- Szczechowiak, K., B. S. Diniz, and J. Leszek. 2019. Diet and Alzheimer’s dementia—Nutritional approach to modulate inflammation. Pharmacology. Pharmacology, Biochemistry, and Behavior 184:172743. doi: 10.1016/j.pbb.2019.172743.
- Takeda, S. 2019. Progression of Alzheimer’s disease, tau propagation, and its modifiable risk factors. Neuroscience Research 141:36–42. doi:10.1016/j.neures.2018.08.005.
- Tanaka, M., S. Dohgu, G. Komabayashi, H. Kiyohara, F. Takata, Y. Kataoka, T. Nirasawa, M. Maebuchi, and T. Matsui. 2019. Brain-transportable dipeptides across the blood-brain barrier in mice. Scientific Reports 9 (1):5769. doi:10.1038/s41598-019-42099-9.
- Tang, K. S. 2019. The cellular and molecular processes associated with scopolamine-induced memory deficit: A model of Alzheimer’s biomarkers. Life Sciences 233:116695. doi: 10.1016/j.lfs.2019.116695.
- Teng, P., Y. Li, W. Cheng, L. Zhou, Y. Shen, and Y. Wang. 2013. Neuroprotective effects of Lycium barbarum polysaccharides in lipopolysaccharide-induced BV2 microglial cells. Molecular Medicine Reports 7 (6):1977–81. doi: 10.3892/mmr.2013.1442.
- Thorajak, P., W. Pannangrong, J. U. Welbat, W. Chaijaroonkhanarak, K. Sripanidkulchai, and B. Sripanidkulchai. 2017. Effects of aged garlic extract on cholinergic, glutamatergic and GABAergic systems with regard to cognitive impairment in Abeta-induced rats. Nutrients 9 (7):686. doi: 10.3390/nu9070686.
- Tiberi, M., and V. Chiurchiù. 2021. Specialized pro-resolving lipid mediators and glial cells: Emerging candidates for brain homeostasis and repair. Frontiers in Cellular Neuroscience 15:673549. doi: 10.3389/fncel.2021.673549.
- Trovato, A., R. Siracusa, R. Di Paola, M. Scuto, V. Fronte, G. Koverech, M. Luca, A. Serra, M. A. Toscano, A. Petralia, et al. 2016. Redox modulation of cellular stress response and lipoxin A4 expression by Coriolus versicolor in rat brain: Relevance to Alzheimer’s disease pathogenesis. NeuroToxicology 53:350–8. doi:10.1016/j.neuro.2015.09.012.
- Trovato, A., R. Siracusa, R. Di Paola, M. Scuto, M. L. Ontario, O. Bua, P. D. Mauro, M. A. Toscano, C. C. T. Petralia, L. Maiolino, et al. 2016. Redox modulation of cellular stress response and lipoxin A4 expression by Hericium Erinaceus in rat brain: Relevance to Alzheimer’s disease pathogenesis. Immunity & Ageing 13 (1):23. doi: 10.1186/s12979-016-0078-8.
- Trovato Salinaro, A., M. Pennisi, R. D. Paola, M. Scuto, R. Crupi, M. T. Cambria, M. L. Ontario, M. Tomasello, M. Uva, L. Maiolino, et al. 2018. Neuroinflammation and neurohormesis in the pathogenesis of Alzheimer’s disease and Alzheimer-linked pathologies: Modulation by nutritional mushrooms. Immunity & Ageing 15 (1):8. doi: 10.1186/s12979-017-0108-1.
- Tsai-Teng, T., C. Chin-Chu, L. Li-Ya, C. Wan-Ping, L. Chung-Kuang, S. Chien-Chang, H. F. Chi-Ying, C. Chien-Chih, and Y. J. Shiao. 2016. Erinacine A-enriched Hericium erinaceus mycelium ameliorates Alzheimer’s disease-related pathologies in APPswe/PS1dE9 transgenic mice. Journal of Biomedical Science 23 (1):49. doi: 10.1186/s12929-016-0266-z.
- Tyagi, E., R. Agrawal, C. Nath, and R. Shukla. 2008. Influence of LPS-induced neuroinflammation on acetylcholinesterase activity in rat brain. Journal of Neuroimmunology 205 (1-2):51–6. doi: 10.1016/j.jneuroim.2008.08.015.
- Vo, T.-S., BMi Ryu, and S.-K. Kim. 2013. Purification of novel anti-inflammatory peptides from enzymatic hydrolysate of the edible microalgal Spirulina maxima. Journal of Functional Foods 5 (3):1336–46. doi: 10.1016/j.jff.2013.05.001.
- Wang, M., W. K. Amakye, L. Guo, C. Gong, Y. Zhao, M. Yao, and J. Ren. 2019. Walnut-derived peptide PW5 ameliorates cognitive impairments and alters gut microbiota in APP/PS1 transgenic mice. Molecular Nutrition & Food Research 63 (18):e1900326. doi: 10.1002/mnfr.201900326.
- Wang, S., L. Zheng, T. Zhao, Q. Zhang, Y. Liu, B. Sun, G. Su, and M. Zhao. 2020. Inhibitory effects of walnut (Juglans regia) peptides on neuroinflammation and oxidative stress in lipopolysaccharide-induced cognitive impairment mice. Journal of Agricultural and Food Chemistry 68 (8):2381–92. doi: 10.1021/acs.jafc.9b07670.
- Wang, X. Y., D. D. Zhang, J. Y. Yin, S. P. Nie, and M. Y. Xie. 2019. Recent developments in Hericium erinaceus polysaccharides: Extraction, purification, structural characteristics and biological activities. Critical Reviews in Food Science and Nutrition 59 (sup1):S96–S115. doi: 10.1080/10408398.2018.1521370.
- Wang, Y., H. Xu, Q. Fu, R. Ma, and J. Xiang. 2011. Protective effect of resveratrol derived from Polygonum cuspidatum and its liposomal form on nigral cells in Parkinsonian rats. Journal of the Neurological Sciences 304 (1-2):29–34. doi: 10.1016/j.jns.2011.02.025.
- Welcome, M. O. 2020. Neuroinflammation in CNS diseases: Molecular mechanisms and the therapeutic potential of plant derived bioactive molecules. PharmaNutrition 11:100176. doi: 10.1016/j.phanu.2020.100176.
- Whyte, A. R., S. Rahman, L. Bell, I. Edirisinghe, R. Krikorian, C. M. Williams, and B. Burton-Freeman. 2021. Improved metabolic function and cognitive performance in middle-aged adults following a single dose of wild blueberry. European Journal of Nutrition 60 (3):1521–36. doi: 10.1007/s00394-020-02336-8.
- Wong, F.-C., J. Xiao, S. Wang, K.-Y. Ee, and T.-T. Chai. 2020. Advances on the antioxidant peptides from edible plant sources. Trends in Food Science & Technology 99:44–57. doi: 10.1016/j.tifs.2020.02.012.
- Wu, D. T., W. Liu, M. L. Xian, G. Du, X. Liu, J. J. He, P. Wang, W. Qin, and L. Zhao. 2020. Polyphenolic-protein-polysaccharide complexes from Hovenia dulcis: Insights into extraction methods on their physicochemical properties and in vitro bioactivities. Foods (Basel, Switzerland) 9 (4):456. doi: 10.3390/foods9040456.
- Wu, J., A. Wang, Z. Min, Y. Xiong, Q. Yan, J. Zhang, J. Xu, and S. Zhang. 2011. Lipoxin A4 inhibits the production of proinflammatory cytokines induced by beta-amyloid in vitro and in vivo. Biochemical and Biophysical Research Communications 408 (3):382–7. doi: 10.1016/j.bbrc.2011.04.013.
- Wu, J., D.-H. Ding, Q.-Q. Li, X.-Y. Wang, Y.-Y. Sun, and L.-J. Li. 2019. Lipoxin A4 regulates lipopolysaccharide-induced BV2 microglial activation and differentiation via the Notch signaling pathway. Frontiers in Cellular Neuroscience 13:19. doi: 10.3389/fncel.2019.00019.
- Wu, S., A. E.-D. A. Bekhit, Q. Wu, M. Chen, X. Liao, and J. Wang, Y. Ding. 2021. Bioactive peptides and gut microbiota: Candidates for a novel strategy for reduction and control of neurodegenerative diseases. Trends in Food Science & Technology 108:164–76. doi: 10.1016/j.tifs.2020.12.019.
- Xu, M. J., J. Y. Wang, X. Y. Zhang, T. X. Yan, B. Wu, K. S. Bi, and Y. Jia. 2020. Polysaccharide from Schisandra chinensis acts via LRP-1 to reverse microglia activation through suppression of the NF-kappa B and MAPK signaling. Journal of Ethnopharmacology 256 (13):112798. doi: 10.1016/j.jep.2020.112798.
- Xu, M., T. Yan, K. Fan, M. Wang, Y. Qi, F. Xiao, K. Bi, and Y. Jia. 2019. Polysaccharide of Schisandra Chinensis Fructus ameliorates cognitive decline in a mouse model of Alzheimer’s disease. Journal of Ethnopharmacology 237:354–65. doi: 10.1016/j.jep.2019.02.046.
- Xu, M., T. Yan, G. Gong, B. Wu, B. He, Y. Du, F. Xiao, and Y. Jia. 2020. Purification, structural characterization, and cognitive improvement activity of a polysaccharides from Schisandra chinensis. International Journal of Biological Macromolecules 163:497–507. doi: 10.1016/j.ijbiomac.2020.06.275.
- Xu, T., X. Shen, H. Yu, L. Sun, W. Lin, and C. Zhang. 2016. Water-soluble ginseng oligosaccharides protect against scopolamine-induced cognitive impairment by functioning as an antineuroinflammatory agent. Journal of Ginseng Research 40 (3):211–9. doi:10.1016/j.jgr.2015.07.007.
- Yang, W., F. Pei, Y. Shi, L. Zhao, Y. Fang, and Q. Hu. 2012. Purification, characterization and anti-proliferation activity of polysaccharides from Flammulina velutipes. Carbohydrate Polymers 88 (2):474–80. doi: 10.1016/j.carbpol.2011.12.018.
- Yang, Z., T. Kuboyama, and C. Tohda. 2019. Naringenin promotes microglial M2 polarization and Aβ degradation enzyme expression. Phytotherapy Research: PTR 33 (4):1114–21. doi: 10.1002/ptr.6305.
- Yilmaz, C., K. Karali, G. Fodelianaki, A. Gravanis, T. Chavakis, I. Charalampopoulos, and V. I. Alexaki. 2019. Neurosteroids as regulators of neuroinflammation. Frontiers in Neuroendocrinology 55:100788. doi: 10.1016/j.yfrne.2019.100788.
- Yu, H., L. Lin, Z. Zhang, H. Zhang, and H. Hu. 2020. Targeting NF-kappaB pathway for the therapy of diseases: Mechanism and clinical study. Signal Transduction and Target Therapy 5 (1):209. doi: 10.1038/s41392-020-00312-6.
- Yuan, B., L. Zhao, K. Rakariyatham, Y. Han, Z. Gao, B. Muinde Kimatu, Q. Hu, and H. Xiao. 2017. Isolation of a novel bioactive protein from an edible mushroom Pleurotus eryngii and its anti-inflammatory potential. Food & Function 8 (6):2175–83. doi: 10.1039/c7fo00244k.
- Yuliani, S., Mustofa, and G. Partadiredja. 2018. Turmeric (Curcuma longa L.) extract may prevent the deterioration of spatial memory and the deficit of estimated total number of hippocampal pyramidal cells of trimethyltin-exposed rats. Drug and Chemical Toxicology 41 (1):62–71. doi: 10.1080/01480545.2017.1293087.
- Zaky, A., A. Bassiouny, M. Farghaly, and B. M. El-Sabaa. 2017. A combination of resveratrol and curcumin is effective against aluminum chloride-induced neuroinflammation in rats. Journal of Alzheimer’s Disease: JAD 60 (s1):S221–S35. doi: 10.3233/JAD-161115.
- Zarezadeh, M., T. Baluchnejadmojarad, Z. Kiasalari, S. Afshin-Majd, and M. Roghani. 2017. Garlic active constituent s-allyl cysteine protects against lipopolysaccharide-induced cognitive deficits in the rat: Possible involved mechanisms. European Journal of Pharmacology 795:13–21. doi: 10.1016/j.ejphar.2016.11.051.
- Zhang, J., Y. Zheng, Y. Luo, Y. Du, X. Zhang, and J. Fu. 2019. Curcumin inhibits LPS-induced neuroinflammation by promoting microglial M2 polarization via TREM2/TLR4/NF-kappaB pathways in BV2 cells. Molecular Immunology 116:29–37. doi: 10.1016/j.molimm.2019.09.020.
- Zhang, Z., S. He, X. Cao, Y. Ye, L. Yang, J. Wang, H. Liu, and H. Sun. 2020. Potential prebiotic activities of soybean peptides Maillard reaction products on modulating gut microbiota to alleviate aging-related disorders in D-galactose-induced ICR mice. Journal of Functional Foods 65:103729. doi: 10.1016/j.jff.2019.103729.
- Zhao, H. F., N. Li, Q. Wang, X. J. Cheng, X. M. Li, and T. T. Liu. 2015. Resveratrol decreases the insoluble Aβ1-42 level in hippocampus and protects the integrity of the blood-brain barrier in AD rats. Neuroscience 310:641–9. doi: 10.1016/j.neuroscience.2015.10.006.
- Zheng, J., Q. Dai, K. Han, W. Hong, D. Jia, Y. Mo, Y. Lv, H. Tang, H. Fu, and W. Geng. 2020. JNK-IN-8, a c-Jun N-terminal kinase inhibitor, improves functional recovery through suppressing neuroinflammation in ischemic stroke. Journal of Cellular Physiology 235 (3):2792–9. doi: 10.1002/jcp.29183.
- Zhong, J., X. Qiu, Q. Zhang, H. Chen, and C. Yan. 2020. A novel polysaccharide from Acorus tatarinowii protects against LPS-induced neuroinflammation and neurotoxicity by inhibiting TLR4-mediated MyD88/NF-kappaB and PI3K/Akt signaling pathways. International Journal of Biological Macromolecules 163:464–75. doi: 10.1016/j.ijbiomac.2020.06.266.
- Zhou, Y., C. Cui, X. Ma, W. Luo, S. G. Zheng, and W. Qiu. 2020. Nuclear factor kappaB (NF-kappaB)-mediated inflammation in multiple sclerosis. Frontiers in Immunology 11:391. doi: 10.3389/fimmu.2020.00391.
- Zhu, Z., Y. Han, Y. Ding, B. Zhu, S. Song, and H. Xiao. 2021. Health effects of dietary sulfated polysaccharides from seafoods and their interaction with gut microbiota. Comprehensive Reviews in Food Science and Food Safety 20 (3):2882–913. doi: 10.1111/1541-4337.12754.
- Zou, J., P. S. Cai, C. M. Xiong, and J. L. Ruan. 2016. Neuroprotective effect of peptides extracted from walnut (Juglans Sigilata Dode) proteins on Abeta25-35-induced memory impairment in mice. Journal of Huazhong University of Science and Technology [Medical Sciences] 36 (1):21–30. doi: 10.1007/s11596-016-1536-4.