Abstract
Consumption of the Mediterranean dietary pattern (MedDiet) is associated with reduced risk of numerous non-communicable diseases. Modulation of the composition and metabolism of the gut microbiota represents a potential mechanism through which the MedDiet elicits these effects. We conducted a systematic literature search (Prospero registration: CRD42020168977) using PubMed, The Cochrane Library, MEDLINE, SPORTDiscuss, Scopus and CINAHL databases for randomized controlled trials (RCTs) and observational studies exploring the impact of a MedDiet on gut microbiota composition (i.e., relative abundance of bacteria or diversity metrics) and metabolites (e.g., short chain fatty acids). Seventeen RCTs and 17 observational studies were eligible for inclusion in this review. Risk of bias across the studies was mixed but mainly identified as low and unclear. Overall, RCTs and observational studies provided no clear evidence of a consistent effect of a MedDiet on composition or metabolism of the gut microbiota. These findings may be related to the diverse methods across studies (e.g., MedDiet classification and analytical techniques), cohort characteristics, and variable quality of studies. Further, well-designed studies are warranted to advance understanding of the potential effects of the MedDiet using more detailed examination of microbiota and microbial metabolites with reference to emerging characteristics of a healthy gut microbiome.
Graphical Abstract
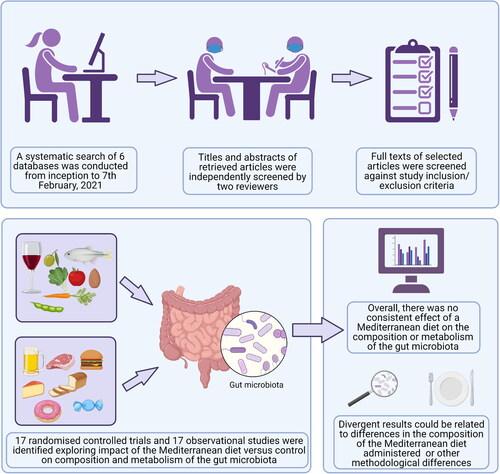
This systematic review explored effects of the Mediterranean diet on the composition and metabolism of the gut microbiota in both randomized controlled trials and observational studies. Although there is some evidence from a small number of studies indicated a positive impact of a Mediterranean diet on select microbiota, the findings of this systematic review suggest that this dietary pattern does not consistently alter microbiota composition or metabolism. The lack of a consistent effect could be related to methodological differences between studies, especially differences in the composition of the Mediterranean diet administered.
Introduction
Over the last few decades, the gut microbiome (a highly complex and diverse community of microbes living within gastrointestinal tract) has received considerable attention because of its fundamental role in human health and disease (Valdes et al. Citation2018). There is growing evidence that the gut microbiota plays a critical, symbiotic role in human health, participating in, amongst others, vitamin biosynthesis, the fermentation of undigested carbohydrates and proteins to produce short chain fatty acids (SCFAs; e.g., acetate, propionate and butyrate), the maintenance of gastrointestinal integrity, and regulation of immune function (Hooper and Gordon Citation2001; Rowland et al. Citation2018; Valdes et al. Citation2018). In addition, disturbances in the gut microbiota (i.e., dysbiosis) have been linked with a multitude of adverse health outcomes including inflammatory bowel disease, colorectal cancer, diabetes, obesity, cardiovascular disease, and dementia (Hills et al. Citation2019). The gut microbiota is modulated by diet, and also by other lifestyle factors including physical activity (PA), and smoking (Valdes et al. Citation2018), which have bigger effects than host genetic factors (Rothschild et al., Citation2018). Diet plays a significant role in shaping the microbiome by providing substrates that can differentially promote the growth and activities of specific microbes and communities (Singh et al. Citation2017) with, potentially, consequential beneficial effects on health (Hills et al. Citation2019; Valdes et al. Citation2018).
The Mediterranean dietary pattern (MedDiet), which reflects the traditional eating habits in countries situated around the Mediterranean Sea prior to the globalization of food systems, is a plant-based dietary pattern rich in fruit, vegetables, nuts, seeds, olive oil, and unrefined grains. Small-to-moderate amounts of animal products are typically consumed as part of the MedDiet, including moderate quantities of fish, small amount of poultry, and minimal consumption of red and processed meats. In addition, the MedDiet typically includes a low-to-moderate intake of alcohol, usually in the form of red wine, which is consumed socially at meal times (Bach-Faig et al. Citation2011; Shannon et al. Citation2019). Many of the characteristic components of the MedDiet including plant foods and red wine are rich in nutrients such as fiber and in phytochemicals (e.g. polyphenols) that have been independently associated with reduced risk of mortality (Kim & Je, Citation2017; Zamora-Ros et al., Citation2013) and morbidity from non-communicable diseases (Reis et al., Citation2016; Shishtar et al., Citation2020; Threapleton et al., Citation2013). Dietary fiber and polyphenols are known to have prebiotic actions which might mediate health-related outcomes associated with their intake (Gibson et al. Citation2017; Tuohy et al. Citation2012). Dietary fiber is fermented by bacteria in the colon to produce SCFA which are proposed to have systemic anti-inflammatory effects (van der Hee and Wells Citation2021). Polyphenols are metabolized initially by the gut microbiota, resulting in increased absorption, and the subsequent metabolism by host enzymes in the gut mucosa and liver can produce metabolites with multiple physiological effects (Istas et al., Citation2019). In addition, some polyphenols act as antimicrobials against pathogenic bacteria with consequential effects on host health (Ma & Chen, Citation2020).
While consumption of individual foods and food components may have metabolic and health consequences, they are not eaten in isolation and so to understand the overall effects of dietary choices it is important to characterize dietary patterns and to investigate their links with health (Schulze et al. Citation2018). The complex array of nutrients and other bioactive components within a dietary pattern, such as the MedDiet, will result in interactions and synergies that may influence the gut bacteria (Riaz Rajoka et al. Citation2017) in multiple ways. However, evidence from individual studies is often conflicting and paradoxical. For example, the diversity of the gut microbiota has been reported to increase in some studies (Cox et al. Citation2020; Maskarinec et al. Citation2019) and not change in others (Maldonado-Contreras et al. Citation2020; Meslier et al. Citation2020) with higher adherence to MedDiet. Similarly, the relative abundance of bacterial phyla (e.g., Bacteroidetes) has been shown to increase with greater MedDiet adherence, but decrease after MedDiet intervention (Di Iorio et al. Citation2019; Gutiérrez-Díaz et al. Citation2016). Therefore, we aimed to conduct the first systematic review exploring effects of the MedDiet on the composition and metabolism of the gut microbiota, drawing evidence from both observational studies and RCTs.
Methods
This systematic review was conducted according to the Preferred Reporting Items for Systematic Review and Meta-analyses (PRISMA) guidelines (Liberati et al. Citation2009) and was registered prospectively with the PROSPERO database (CRD42020168977).
Literature search
A systematic search of six databases (PubMed, The Cochrane Library, MEDLINE, SPORTDiscuss, Scopus and CINAHL) was conducted from inception through to 7th February 2021 to identify relevant articles for inclusion in this review. No publication date or language restrictions were applied. Searches were conducted using pre-defined search terms relating to the MedDiet and the composition and metabolism of the gut microbiota, with MeSH terms utilized where appropriate. The search strategy was tailored to the requirements of each database (Supplementary Material 1). The reference lists of eligible studies and relevant review articles were also searched to identify other potentially relevant studies. Gray literature was included in search results to minimize risk of publication bias.
Study selection
Inclusion criteria
The following criteria were used to identify appropriate articles for inclusion in this systematic review:
General criteria
RCTs and observational studies were included. No exclusion criteria were applied based around the study design for either RCTs (e.g. cross-over or parallel group design) or observational studies (e.g. cross-sectional, case-control, or prospective studies).
Studies with adult participants (aged ≥18 years) were included. Participants were not excluded based on health or smoking status.
Study-specific criteria
Observational studies
Studies exploring associations between MedDiet adherence and the composition and/or metabolism of the bacterial community were included, providing that MedDiet adherence was compared against a suitable reference (e.g., low MedDiet adherence) or control (e.g., intake of an alternative dietary pattern) group.
No exclusion criteria were made based on the method used to define MedDiet adherence.
RCTs
RCTs which explored the effects of a MedDiet intervention alone or in combination with other clinical, pharmacological or lifestyle interventions were included, as long as the study also included a suitable control group. For example, if the MedDiet was administered in combination with an exercise intervention, the study was within scope if the control group also underwent the same exercise intervention.
No exclusion criteria were made based on the specific composition of the MedDiet; we included articles where the dietary intervention was described as MedDiet by the authors of the article.
Exclusion criteria
We excluded studies that included non-adult subjects and studies including animals and in vitro studies. Studies were also excluded if the MedDiet was administered alongside another intervention (i.e., exercise, pharmacological agent, dietary supplement) and no suitable control or reference groups could be identified. For RCTs, use of antibiotics and/or laxatives before, or during, the intervention was an exclusion criterion, given their impact on the gut microbiota.
Screening
The titles and abstracts of retrieved articles were independently screened by two researchers (PG and RK) to evaluate their eligibility for inclusion in this review. The researchers met after completing the independent review of titles/abstracts to compare notes and reach a consensus. A third reviewer (OMS) was present to moderate the discussion and resolve disagreements about the eligibility of potential studies. Potentially eligible studies, that could not be excluded from an appraisal of their titles and abstracts, were carried over to the full-text stage of the review for further evaluation. Full-texts of the selected articles were evaluated against the study inclusion/exclusion criteria by two researchers (PG and RK) and a third researcher (OMS) helped resolve any disagreements.
Data extraction
Data were extracted independently by two reviewers (RK and PG) and were checked by a third reviewer (OMS). The following information was extracted: Surname of the first author, author contact details, publication year, country, information on the study design, study duration, study cohort, sample size, type of intervention (RCTs) and control group, method used to evaluate dietary intake and MedDiet adherence, age, gender, ethnicity, randomization procedure, blinding of measurements, compliance with the interventions, body mass index (BMI), medication use, smoking status, gut microbiota composition and times of measurements (e.g. baseline, post-intervention), markers of gut microbiota metabolism (e.g. SCFA concentrations) and the methods and techniques used to assess these measures.
Data synthesis
The extracted data were deemed unsuitable for meta-analysis due to the diversity in outcome measurements and the small number of studies reporting data on the same microbiota-related outcomes (Jackson and Turner Citation2017). Therefore, a narrative synthesis of the literature was conducted for the effect of MedDiet on microbiota diversity, bacterial abundance as well as microbial metabolites (Li et al. Citation2018; Tomas-Barberan, Selma, and Espín Citation2018; Vernocchi, Del Chierico, and Putignani Citation2016).
Assessment of study quality
Risk of bias of the included studies was assessed by one researcher (AG) and checked for accuracy by a second researcher (OMS). Study quality for RCTs was assessed using The Cochrane risk of bias tool (Higgins and Green Citation2011). Each RCT was classified as high, low or unclear (i.e. insufficient evidence) risk in relation to seven domains: sequence generation, allocation concealment, blinding of participants and personnel, blinding of outcome assessment, incomplete outcome data, selective reporting and other sources of bias. The Newcastle-Ottawa tool was used to assess risk of bias in observational studies. Studies were classified as high-quality (≥7 stars), medium-quality (4-6 stars) or low quality (0-3 stars) (Islam et al. Citation2016; Wells et al. Citation2000). An adapted version of this tool was used to assess risk of bias in cross-sectional studies (Modesti et al. Citation2016).
Results
Overview
A total of 1249 non-duplicated records were identified following electronic database searches. After screening the titles and abstracts, 42 of the records were deemed potentially eligible for inclusion and full-texts were retrieved for further evaluation. Two additional full-texts were identified via other sources and that were deemed potentially eligible for inclusion in this review. Following appraisal of 44 full-texts, a total of 34 articles (17 RCTs and 17 observational studies) were identified as eligible for inclusion in this review ().
Study characteristics
Observational studies
The characteristics of eligible observational studies are outlined in . Of the 17 observational studies, 14 were cross-sectional and 3 were prospective studies. The total number of participants across the observational studies was 4526. Based on the mean/median values provided in the studies, there was a median sample of 119 (range: 20-1735) and age of 60 (range: 22-95) years.
Table 1. Characteristics of observational studies investigating associations between MedDiet adherence and the gut microbiota.
In the majority of studies, the impact of the MedDiet was investigated in healthy participants (n = 10) (Barrea et al. Citation2019; Filippis et al. Citation2016; Gallè et al. Citation2020; Garcia-Mantrana et al. Citation2018; Gutiérrez-Díaz et al., Citation2017; Gutiérrez-Díaz et al. Citation2016; Maskarinec et al. Citation2019; Mitsou et al. Citation2017; Valeriani et al. Citation2020; Wang et al. Citation2021). Studies were also conducted in individuals with atrial fibrillation (n = 1) (Pastori et al. Citation2017), diabetes (n = 1)(Pignanelli et al. Citation2018), diabetes, hypertension and hypercholesterolemia (n = 1) (Almanza-Aguilera et al., Citation2017), cirrhosis (n = 1) (Cox et al. Citation2020), rheumatoid arthritis (n = 1) (Picchianti Diamanti et al. Citation2020), non-declared pathologies (n = 1)(Ruiz-Saavedra et al. Citation2020), and a high prevalence of chronic disease (n = 1) (Maldonado-Contreras et al. Citation2020). A range of instruments were used to characterize MedDiet adherence, which are summarized in and described in detail in Supplementary Table 4.
Table 4. Results summary of randomized controlled trials investigating effects of the MedDiet on the gut microbiota.
Randomized controlled trials
The characteristics of eligible RCTs are outlined in . Of the 17 RCTs, 12 used parallel group, and 5 used crossover, study designs. The total number of participants in the RCTs was 1882. Based on the mean/median values provided in the studies, there was a median sample size of 79 (range: 10-612), age of 60 (range: 22-79) years and intervention duration of 26 (range: <1–156) weeks. Participants included healthy individuals (n = 2) (Park et al. Citation2019; Zhu et al. Citation2020), healthy participants at increased risk of colon cancer (n = 3) (Djuric et al. Citation2018; Griffin et al. Citation2019; Umoh et al. Citation2016), individuals at increased risk of CVD (n = 1) (Vázquez-Fresno et al. Citation2015), individuals with overweight/obesity (n = 2) (Meslier et al. Citation2020; Pagliai et al. Citation2020), individuals with chronic kidney disease (n = 1) (Di Iorio et al. Citation2019), reactive hypoglycemia (n = 1) (Quercia et al. Citation2017), non-frail and pre-frail participants (n = 1) (Ghosh et al. Citation2020), individuals with and without mild cognitive impairment (n = 1) (Nagpal et al. Citation2019), and individuals with coronary heart disease (CHD) from the CORDIOPREV study including sub-sets with and without metabolic syndrome (MetS; n = 2) (Haro et al. Citation2016a; Santos-Marcos et al. Citation2019), with obesity (n = 1) (Haro et al. Citation2016b), and with and without obesity and MetS (n = 1) (Haro et al. Citation2017).
Table 2. Characteristics of randomized controlled trials investigating effects of the MedDiet on the gut microbiota.
A summary of the MedDiet interventions are shown in . In four studies (Meslier et al. Citation2020; Nagpal et al. Citation2019; Quercia et al. Citation2017; Zhu et al. Citation2020), the participants were provided with MedDiet ingredients and/or meals, and in 8 studies participants were given adjunct counseling or access to a dietician (Djuric et al. Citation2018; Ghosh et al. Citation2020; Griffin et al. Citation2019; Haro et al. Citation2017, Citation2016b, Citation2016a; Santos-Marcos et al. Citation2019; Vázquez-Fresno et al. Citation2015). The full details of interventions of included studies are outlined in Supplementary Table 5.
Study quality and risk of bias
Of the three prospective studies included in this systematic review, one study was deemed to have a moderate quality score of 6 stars (Pastori et al. Citation2017) and two studies a high score of ≥ 7 stars (Maskarinec et al. Citation2019; Wang et al. Citation2021) (). Of the included cross-sectional studies, two studies had a low quality score of 0-3 stars (Cox et al. Citation2020; Pignanelli et al. Citation2018), six studies had a moderate score of 4-6 stars (Almanza-Aguilera et al., Citation2017; Filippis et al. Citation2016; Gallè et al. Citation2020; Garcia-Mantrana et al. Citation2018; Picchianti Diamanti et al. Citation2020; Ruiz-Saavedra et al. Citation2020) and six studies had a high quality score of ≥ 7 stars (Barrea et al. Citation2019; Gutiérrez-Díaz et al., Citation2017; Gutiérrez-Díaz et al. Citation2016; Maldonado-Contreras et al. Citation2020; Mitsou et al. Citation2017; Valeriani et al. Citation2020) ().
The quality of RCTs included in this systematic review was mixed (, ). The risk of selection bias was considered to be low (43%) or unclear (57%; i.e., insufficient details provided to classify risk) across the identified studies. Performance bias was determined as low risk across all included studies, as blinding of participants and personnel to the nature of the intervention (which is difficult, if not impossible, in many dietary-pattern based interventions (Staudacher et al. Citation2017)) was deemed to have negligible effects on relevant outcome variables. The risk of detection and attrition bias was classified as low for ∼50% of included RCTs, and the remainder classified as unclear risk. Only 17% of included studies were classified as low risk in relation to reporting bias, with 28% classified as high risk, and for all remaining studies the risk was unclear. Other sources of bias were identified for four studies in relation to the management and analysis of data in which the MedDiet changes were reported relative to baseline rather than compared with the control (Djuric et al. Citation2018; Ghosh et al. Citation2020; Meslier et al. Citation2020; Rinott et al. Citation2021).The findings from the included studies are summarized in text below and in Supplementary Table 8, while individual study findings are described in more detail in Supplementary Text 1.
Table 3. Results summary of observational studies investigating associations between MedDiet adherence and the gut microbiota.
Findings from observational studies
Relationship between MedDiet and gut microbiota diversity
Alpha-diversity metrics summarize the structure of the bacterial community with regards to its richness (i.e., number of taxonomic groups) and/or evenness (i.e., distribution of abundances of the groups), whereas Beta-diversity metrics summarize the degree to which samples differ from one another (Willis Citation2019). The association between MedDiet adherence and gut microbiota diversity was reported in 8 studies (Cox et al. Citation2020; Filippis et al. Citation2016; Gallè et al. Citation2020; Garcia-Mantrana et al. Citation2018; Maldonado-Contreras et al. Citation2020; Maskarinec et al. Citation2019; Picchianti Diamanti et al. Citation2020; Wang et al. Citation2021). Multiple different indexes were used across studies, with several investigations reporting effects of the MedDiet on more than one diversity index. A summary of the findings can be found in . Because different measures of alpha and beta diversity may yield different results, we have detailed the measures used in each study in Supplementary Table 6 and specific results summarized in .
Figure 3. Summary of findings for the effect of MedDiet on microbiota diversity and phylum abundance from (A) observational studies and (B) randomized controlled trials.
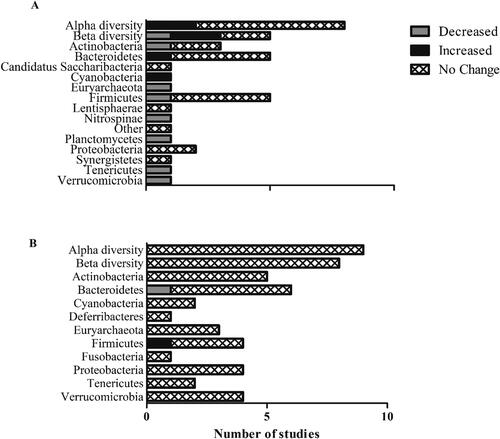
Two (of seven) studies reported a relationship between MedDiet and alpha diversity assessed via the Shannon index. Cox et al. (Citation2020) reported significantly higher Shannon index values in Turkish individuals with decompensated cirrhosis consuming a Med-style diet compared with a control group of American individuals consuming a Western diet. In a prospective study of healthy individuals from the USA, Maskarinec et al. (Citation2019) observed a significant linear trend for higher Shannon index values with increasing MedDiet adherence. Other measures of alpha diversity that were reported in individual studies, including Chao1 (Garcia-Mantrana et al. Citation2018), Faith’s Phylogenetic Diversity (Maldonado-Contreras et al. Citation2020), and an unspecified method (Filippis et al. Citation2016), were not significantly associated with the degree of MedDiet adherence.
In the two studies which measured beta-diversity via weighted and unweighted UniFrac distances, one reported no significant association with MedDiet score (Maldonado-Contreras et al. Citation2020), whilst the other (Maskarinec et al. Citation2019) reported significantly positively (unweighted axis 1 and weighted axis 2) or inversely (unweighted axis 2 and 3, weighted axis 1) associated weighted and unweighted UniFrac axes with MedDiet adherence level. Similarly, in the two studies measuring beta-diversity via Bray-Curtis dissimilarity, one reported no major differences in global structural variation of the gut microbiota (Wang et al. Citation2021), whilst the other reported significant dissimilarity in the bacterial profiles (Gallè et al. Citation2020).
Relationship between MedDiet and bacterial abundance
Similar to microbiota diversity, deployment of different processing and analysis methods could give rise to difference in abundance measures and the methods used in the included studies are reported in Supplementary Table 6. Overall 10 observational studies report the relationship between MedDiet and bacterial abundance at a minimum of one taxonomic level, as summarized in and (Gallè et al. Citation2020; Garcia-Mantrana et al. Citation2018; Gutiérrez-Díaz et al., Citation2017; Gutiérrez-Díaz et al. Citation2016; Maskarinec et al. Citation2019; Mitsou et al. Citation2017; Picchianti Diamanti et al. Citation2020; Ruiz-Saavedra et al. Citation2020; Valeriani et al. Citation2020; Wang et al. Citation2021). Half of the observational studies (3 of 6; Supplementary Table 8) reporting bacterial abundance at phyla level found a relationship with MedDiet. At lower taxonomic levels, relationships between MedDiet and bacterial abundance; (27 genera/species; ) were observed mainly for Faecalibacterium, Ruminococcus (phylum Firmicutes) and Bacteroides (Phlyum Bacteroidetes).
Relationships between MedDiet adherence and gut microbial metabolites
Eleven studies reported relationships between MedDiet adherence and microbial metabolites in feces, urine or plasma (Almanza-Aguilera et al., Citation2017; Barrea et al. Citation2019; Filippis et al. Citation2016; Garcia-Mantrana et al. Citation2018; Gutiérrez-Díaz et al., Citation2017; Gutiérrez-Díaz et al. Citation2016; Maldonado-Contreras et al. Citation2020; Mitsou et al. Citation2017; Pastori et al. Citation2017; Pignanelli et al. Citation2018; Ruiz-Saavedra et al. Citation2020).
Five studies reported associations between MedDiet adherence and the major SCFAs (Filippis et al. Citation2016; Gutiérrez-Díaz et al. Citation2016; Mitsou et al. Citation2017; Ruiz-Saavedra et al. Citation2020) (Maldonado-Contreras et al. Citation2020). Three out of 5 studies reported positive associations between MedDiet adherence and acetate (Filippis et al. Citation2016; Mitsou et al. Citation2017; Ruiz-Saavedra et al. Citation2020), propionate (Filippis et al. Citation2016; Gutiérrez-Díaz et al. Citation2016; Ruiz-Saavedra et al. Citation2020) and butyrate (Filippis et al. Citation2016; Gutiérrez-Díaz et al. Citation2016; Ruiz-Saavedra et al. Citation2020). Other microbial metabolites reported infrequently included other fatty acids, toxins and polyphenol metabolites ().
Findings from randomised controlled trials
Effects of MedDiet on gut microbiota diversity
The effect of MedDiet on microbial diversity metrics was reported in 11 RCTs (Di Iorio et al. Citation2019; Djuric et al. Citation2018; Ghosh et al. Citation2020; Haro et al. Citation2017, Citation2016b; Meslier et al. Citation2020; Nagpal et al. Citation2019; Pagliai et al. Citation2020; Quercia et al. Citation2017; Rinott et al. Citation2021; Zhu et al. Citation2020). No studies found an effect of MedDiet on alpha- or beta-diversity when compared with the control group (). However, in a pooled analysis of data from all participants in one study, in which participants were classified by their level of adherence to a MedDiet during the intervention, a significant decline in diversity was observed in individuals with low and medium MedDiet adherence (at baseline), whereas high MedDiet adherence attenuated the loss of diversity with time (Ghosh et al. Citation2020).
Effects of mediterranean diet on bacterial abundances
Fourteen RCTs reported the effect of MedDiet intervention on bacterial abundance at different taxonomic levels (Di Iorio et al. Citation2019; Djuric et al. Citation2018; Ghosh et al. Citation2020; Haro et al. Citation2017, Citation2016a, Citation2016b; Meslier et al. Citation2020; Nagpal et al. Citation2019; Pagliai et al. Citation2020; Quercia et al. Citation2017, Citation2017; Rinott et al. Citation2021; Santos-Marcos et al. Citation2019; Zhu et al. Citation2020). In contrast with the observational studies, the majority of included RCTs found that MedDiet intervention had no effect on bacterial abundance at phyla level (). One of six studies found a significant effect at phylum level. Di Iorio and colleagues (Citation2019) reported increased Firmicutes and decreased Bacteroidetes after MedDiet compared to control (habitual) diet in a crossover trial of individuals with chronic kidney disease. Across the included studies, MedDiet intervention was reported to have a significant effect on the abundance 37 genera of bacteria (direction of change in ), but, overall, there appeared to be no consistent pattern of change. The exception was for a few studies which found that, at species level, Collinsella aerofaciens (Phylum Actinobacteria) was reduced whereas Roseburia hominis (Phlyum Firmicutes) was increased with MedDiet. The most commonly investigated species was Faecalibacterium prausnitzii (Phlyum Firmicutes) which was increased by MedDiet in 4 out of six studies.
Effects of MedDiet intervention on microbial metabolites
Ten studies report the effect of MedDiet on microbial metabolites (Di Iorio et al. Citation2019; Griffin et al. Citation2019; Meslier et al. Citation2020; Nagpal et al. Citation2019; Pagliai et al. Citation2020; Park et al. Citation2019; Quercia et al. Citation2017; Umoh et al. Citation2016; Vázquez-Fresno et al. Citation2015; Zhu et al. Citation2020; ), with only four short-term interventions focusing on the main SCFA (Meslier et al. Citation2020; Nagpal et al. Citation2019; Pagliai et al. Citation2020; Quercia et al. Citation2017). There were no reported effects on fecal concentrations of acetate following MedDiet intervention in any study (Meslier et al. Citation2020; Nagpal et al. Citation2019; Pagliai et al. Citation2020; Quercia et al. Citation2017). In contrast, one of the four studies reported higher fecal butyrate concentrations (Nagpal et al. Citation2019) and another an increase in propionate (Pagliai et al. Citation2020) after 6 and 13 weeks of MedDiet, respectively.
Discussion
The current systematic review identified 17 RCTs and 17 observational studies which investigated the influence of MedDiet on the gut microbiota and microbial metabolites. Overall, the results suggest that there is little evidence that MedDiet affects microbiota diversity but some evidence that this dietary pattern may modulate taxonomic composition and SCFA production. However, the heterogeneity in both design and findings from the available studies precludes firm conclusions at this stage.
Investigation of the relationships between the MedDiet and the gut microbiome is hugely challenging. This is not only because of the inherent complexity of the MedDiet pattern, but also the diversity in a) methods used to assess adherence to this dietary pattern, b) the heterogeneity in the particular foods, and their relative amounts, that have been manipulated in the MedDiet interventions, c) whether participants were provided with supplementary ingredients/meals or simply recommendations to change their diet, and d) the duration of intervention (Hutchins-Wiese, Bales, and Porter Starr Citation2021). In addition, the human gut microbiome is a complex ecosystem including bacteria, archaea and fungi plus a vast array of viruses, most of which are bacteriophages that infect, and replicate in, bacteria and archaea. Although it is generally accepted that diet is a major factor influencing the gut microbiome, there is very limited understanding of causal relationships between what is eaten and the gut microbiota in humans (Leeming et al. Citation2021). The issue is compounded by lack of understanding of, and limited consensus on, the characteristics of a healthy microbiome (Lloyd-Price, Abu-Ali, and Huttenhower Citation2016; McBurney et al. Citation2019) so that differences in the microbiome associated with different diets, or dietary patterns, are difficult to interpret. While a number of studies have suggested associations between specific bacteria and some disease states, these are often disease-specific and the current literature is conflicting (Chen et al. Citation2021; Palmu et al. Citation2020). On the other hand, high microbiota diversity appears to be a consistent feature of a healthy gut microbiota (Lloyd-Price, Abu-Ali, and Huttenhower Citation2016), and loss of diversity has been associated with increased disease risk (He et al. Citation2018; Lloyd-Price, Abu-Ali, and Huttenhower Citation2016). Overall, there was no conclusive evidence that MedDiet affects gut microbiota diversity. However, the absence of conclusive evidence may reflect limitations in the studies to date and, in particular, may be due to the relatively small size of most of the prospective cohort studies included in this analysis. For example, in two prospective studies of healthy adults in the USA, only the larger study (n = 1735 vs 307) found an association between MedDiet and alpha-diversity (Maskarinec et al. Citation2019; Wang et al. Citation2021).
Furthermore, health status is likely an important factor in determining effects of the MedDiet on the gut microbiota. For example, Cox et al. (Citation2020) found a higher Shannon index in Turkish individuals with decompensated cirrhosis consuming a Med-style diet compared with a control group of American individuals consuming a Western diet, but not healthy controls or those with compensated cirrhosis. This is further supported by the CORDIOPREV RCT in which two years of MedDiet increased Bacteroides, Prevotella, Roseburia and Ruminococcus in males with MetS and obesity but not in obese males without MetS or non-obese males without MetS (Haro et al. Citation2017). These genera were also reduced in the participants with MetS and obesity compared with the non-obese individuals without MetS, suggesting that MedDiet may have potential to restore eubiosis, but that this is dependent on baseline abundance of bacteria. Indeed, individuals living with obesity and different disease states have different enterotypes, and may respond differently to dietary interventions (Arumugam et al. Citation2011). However, it is important to highlight that observed effects could also be confounded by medication use. Further research is needed to determine how different health conditions, and the medications used to treat those conditions, modulate the effects of diet on the gut microbiota.
Other characteristics of the human cohorts including age, sex, geography and ethnicity, as well as study durations may contribute to the heterogeneity in findings and make it difficult to draw clear and well-evidenced conclusions. In an attempt to identify potential differences between shorter and longer duration studies, we compared the effects of short-term and medium-long term MedDiet interventions on the gut microbiota (Supplementary Table 8). However, this did not reveal any clear differences associated with study duration. This finding is likely due to the considerable heterogeneity (as outlined above) in other methodological aspects between studies. As an example of the complexity in comparing the findings between different studies, Gutiérrez-Díaz et al. (Citation2016) found that higher adherence to MedDiet was associated with higher Bacteroidetes and lower Firmicutes in a study of healthy middle-aged Italians. However, the same authors report no such relationship in cohort of healthy older Spanish people (Gutierrez-Diaz et al., Citation2017). Both studies were cross-sectional and the authors used the same MedDiet scoring tool, which suggests that study design and the way in which the MedDiet was characterized are unlikely to explain key differences between the two investigations. The difference in finding could instead relate to the different age ranges in the two studies (Mariat et al. Citation2009), differences in the study setting, and other exposure or phenotypic differences between the two cohorts.
In light of the challenges described above, it is unsurprising that very few studies found an effect of MedDiet on bacterial abundance at phylum level, and none of the studies reported significant effects on Firmicutes/Bacteroidetes ratio. MedDiet appeared to affect bacterial composition at lower taxonomic levels but such effects were not consistent. There was some evidence, mainly from RCTs, that MedDiet reduced bacterial species that preferentially utilize oligosaccharides and simple sugars (e.g. C. aerofaciens and B. adolescentis) and increased those with an affinity for polysaccharides (e.g. R. hominis, B. thetaiotaomicron and E. eligans)(Clavel et al. Citation2016; De Angelis et al. Citation2015). Additionally, two studies found that higher MedDiet adherence was associated with greater abundance of A. equolifaciens which is involved in polyphenol metabolism (Picchianti Diamanti et al. Citation2020; Wang et al. Citation2021). Notably, both observational studies and RCTs found that MedDiet may increase F. prausnitzii, a keystone butyrate-producer associated with anti-inflammatory activities (Velasquez-Manoff Citation2015). However, MedDiet appeared to affect different F. prausnitzii strains in different studies (Ghosh et al. Citation2020; Meslier et al. Citation2020) which could have implications for SCFA metabolism and any consequential anti-inflammatory effects (Martín et al. Citation2017). Moreover, as is common in the complex gut microbial ecosystem, F. prausnitzii have been shown to cross-feed and to cooperate with, and to compete with, other bacterial species (Flint et al. Citation2007; Lindstad et al. Citation2021). A more MedDiet-like eating pattern will produce complex changes in the substrates flowing to the large bowel, each of which may have positive or negative effects on multiple members of this complex microbial ecosystem. Further variability is introduced by the different components and quantities of foods in the MedDiet administered (especially the quantity of prebiotics such as fiber and polyphenols (Gibson et al. Citation2017; Tuohy et al. Citation2012)). As a consequence, it may be naive to assume that the MedDiet would provoke simple, characteristic changes in the microbiota at any taxonomic level. It has been proposed that a more holistic approach that goes beyond characterization of bacterial composition and encompasses dynamic interactions between all components of the microbiota may help in investigation of links with health of the host (Clemente et al. Citation2012) and the same is likely to apply when attempting to understand changes in response to dietary patterns such as MedDiet. On that basis, dietary manipulation of the microbial ecosystem would be expected to result in complex (and, perhaps, difficult to predict) changes in the patterns of metabolites that are end-products of the multiple biochemical interactions between microbes and that can be measured in stool. Nevertheless. it is also likely that the pattern of these microbial metabolites (e.g. SCFA) will be critical in determining consequences for host physiology (Leeming et al. Citation2021). Despite this, few studies reported the effects of MedDiet on microbial metabolites, and we suggest that this should be a priority for future research.
Strengths and limitations
This is the first systematic review to explore effects of MedDiet on the composition and metabolism of the gut microbiota. A strength of this review is the inclusion of a large number of both RCTs and observational studies, which provide complementary insights into the impact of MedDiet on the gut microbiota. For example, inclusion of observational studies allowed us to explore the impact of the MedDiet in a ‘real-world’ setting, where cause-effect relationships cannot be established, but where larger sample sizes and longer follow ups are available compared with RCTs. This is an important factor, given that shifts in the microbiota change rapidly in response to dietary interventions (David et al. Citation2014) but whether such changes are sustained in the longer-term effects is unclear. Inclusion of RCTs allowed us to assess the evidence for causal effects of the MedDiet on gut microbiota in more controlled experimental settings, where the characteristics of the intervention may be well defined (albeit that the nature and duration of such interventions varied between studies). Several narrative reviews have explored the effects of the MedDiet on the gut microbiota (Del Chierico et al. Citation2014; García-Montero et al. Citation2021; Merra et al. Citation2020; Tsigalou et al. Citation2021). These usually include a small number of studies and report mainly positive findings and there is a significant inherent risk of selection bias in narrative reviews. Our systematic review includes data on gut microbiota metabolites, especially SCFAs, which are likely to be pivotal in mediating effects on human health (van der Hee and Wells Citation2021). Further strengths of this review include adherence to the PRISMA guidelines and prospectively registering our review protocol, which minimizes reviewer bias. In addition, our searches were conducted by an information specialist who is experienced with systematic review methodologies and used multiple databases whilst including gray literature to maximize our chance of identifying all relevant research. Nevertheless, despite our robust methods, it is possible that we did not identify all relevant studies in this area. A limitation of the evidence included in this systematic review was the mixed quality and heterogeneity of included studies, including some issues surrounding data analysis, which stresses the need for further well-controlled investigations in this area. A further limitation is that we were unable to quantitatively synthesize results from different studies via meta-analysis, because of substantial diversity in outcome measurements and the limited number of studies exploring effects of the MedDiet on consistent outcomes.
Conclusions and directions for future research
Although there is some evidence from a small number of studies indicated a positive impact of a MedDiet on specific gut microbiota, the findings of this systematic review suggest that this dietary pattern does not consistently alter microbiota composition or metabolism. This lack of a consistent effect is not surprising given the heterogeneity between study populations, analysis methods, duration and characterization of MedDiet and the limited approaches that have been used to characterize the complex ecosystem that constitutes the large bowel microbiome. This research area will benefit from improved conceptualization of, and compelling evidence for, the characteristics of a “healthy gut microbiome” (Goodrich et al. Citation2014; McBurney et al. Citation2019) that could be used as an external reference against which to judge the effects of any changes in the gut microbiome associated with the MedDiet or other dietary patterns/interventions. Further, given the differential effects of individual components of the MedDiet on the gut microbiota, this research area will also benefit from standardization of the composition of experimental MedDiet interventions and from standardization of the scoring methods used to assess MedDiet adherence in observational studies. Finally, the development, and adoption, of open access systems for data sharing would facilitate meta-analyses at individual participant level that may help to resolve some of the heterogeneity in reported findings.
Author contributions
This study was conceived by OMS and JCM. The study was planned and designed by RK, PG, AA, CS, KD, JM, AG, FCM, AJ, DH, ES, AMM, MS, OMS and JCM. JM designed the search strategy and performed the searches. RK and PG screened the titles, abstracts, and full-texts of retrieved papers, with any disputes resolved in consultation with OMS. RK and PG extracted data. AG conducted the risk of bias assessment, which was verified by OMS. RK, CS, FCM, AJ, DH, OMS and JCM interpreted the data. OMS and RK drafted the manuscript. PG, AA, CS, KD, JM, AG, FCM, AJ, DH, ES, AMM and MS further contributed toward the writing and critical revision of the manuscript. All authors have read and approved the final version of the manuscript.
Supplemental Material
Download MS Word (207.8 KB)Disclosure statement
No potential conflict of interest was reported by the authors.
Additional information
Funding
References
- Almanza-Aguilera, E., M. Urpi-Sarda, R. Llorach, R. Vázquez-Fresno, M. Garcia-Aloy, F. Carmona, A. Sanchez, F. Madrid-Gambin, R. Estruch, D. Corella, et al. 2017. Microbial metabolites are associated with a high adherence to a Mediterranean dietary pattern using a 1H-NMR-based untargeted metabolomics approach. The Journal of Nutritional Biochemistry 48:36–43. doi: 10.1016/j.jnutbio.2017.06.001.
- Arumugam, M., J. Raes, E. Pelletier, D. Le Paslier, T. Yamada, D. R. Mende, G. R. Fernandes, J. Tap, T. Bruls, J.-M. Batto, MetaHIT Consortium (additional members), et al. 2011. Enterotypes of the human gut microbiome. Nature 473 (7346):174–80. doi: 10.1038/nature09944.
- Bach-Faig, A., E. M. Berry, D. Lairon, J. Reguant, A. Trichopoulou, S. Dernini, F. X. Medina, M. Battino, R. Belahsen, G. Miranda, Mediterranean Diet Foundation Expert Group, et al. 2011. Mediterranean diet pyramid today. Science and cultural updates. Public Health Nutrition 14 (12A):2274–84. doi: 10.1017/S1368980011002515.
- Barrea, L., G. Annunziata, G. Muscogiuri, D. Laudisio, C. Di Somma, M. Maisto, G. C. Tenore, A. Colao, and S. Savastano. 2019. Trimethylamine N-oxide, Mediterranean diet, and nutrition in healthy, normal-weight adults: Also a matter of sex? Nutrition 62:7–17. doi: 10.1016/j.nut.2018.11.015.
- Chen, Z., J. Maqbool, F. Sajid, G. Hussain, and T. Sun. 2021. Human gut microbiota and its association with pathogenesis and treatments of neurodegenerative diseases. Microbial Pathogenesis 150:104675. doi: 10.1016/j.micpath.2020.104675.
- Clavel, T., I. Lagkouvardos, M. Blaut, and B. Stecher. 2016. The mouse gut microbiome revisited: From complex diversity to model ecosystems. International Journal of Medical Microbiology: IJMM 306 (5):316–27. doi: 10.1016/j.ijmm.2016.03.002.
- Clemente, J. C., L. K. Ursell, L. W. Parfrey, and R. Knight. 2012. The impact of the gut microbiota on human health: An integrative view. Cell 148 (6):1258–70. doi: 10.1016/j.cell.2012.01.035.
- Cox, I. J., R. Idilman, A. Fagan, D. Turan, L. Ajayi, A. D. L. Guennec, S. D. Taylor-Robinson, F. Karakaya, E. Gavis, R. A. Atkinson, et al. 2020. Metabolomics and microbial composition increase insight into the impact of dietary differences in cirrhosis. Liver International: Official Journal of the International Association for the Study of the Liver 40 (2):416–27. doi: 10.1111/liv.14256.
- David, L. A., C. F. Maurice, R. N. Carmody, D. B. Gootenberg, J. E. Button, B. E. Wolfe, A. V. Ling, A. S. Devlin, Y. Varma, M. A. Fischbach, et al. 2014. Diet rapidly and reproducibly alters the human gut microbiome. Nature 505 (7484):559–63. doi: 10.1038/nature12820.
- De Angelis, M., E. Montemurno, L. Vannini, C. Cosola, N. Cavallo, G. Gozzi, V. Maranzano, R. Di Cagno, M. Gobbetti, L. Gesualdo, et al. 2015. Effect of whole-grain barley on the human fecal microbiota and metabolome. Applied and Environmental Microbiology 81 (22):7945–56. doi: 10.1128/AEM.02507-15.
- Del Chierico, F., P. Vernocchi, B. Dallapiccola, and L. Putignani. 2014. Mediterranean diet and health: Food effects on gut microbiota and disease control. International Journal of Molecular Sciences 15 (7):11678–99. doi: 10.3390/ijms150711678.
- Di Iorio, B. R., M. T. Rocchetti, M. De Angelis, C. Cosola, S. Marzocco, L. Di Micco, I. di Bari, M. Accetturo, M. Vacca, M. Gobbetti, et al. 2019. Nutritional therapy modulates intestinal microbiota and reduces serum levels of total and free indoxyl sulfate and P-cresyl sulfate in chronic kidney disease (medika study). Journal of Clinical Medicine 8 (9):1424. doi: 10.3390/jcm8091424.
- Djuric, Z., C. M. Bassis, M. A. Plegue, J. Ren, R. Chan, E. Sidahmed, D. K. Turgeon, M. T. Ruffin, I. Kato, and A. Sen. 2018. Colonic mucosal bacteria are associated with inter-individual variability in serum carotenoid concentrations. Journal of the Academy of Nutrition and Dietetics 118 (4):606–16.e3. doi: 10.1016/j.jand.2017.09.013.
- Filippis, F. D., N. Pellegrini, L. Vannini, I. B. Jeffery, A. L. Storia, L. Laghi, D. I. Serrazanetti, R. D. Cagno, I. Ferrocino, C. Lazzi, et al. 2016. High-level adherence to a Mediterranean diet beneficially impacts the gut microbiota and associated metabolome. Gut 65 (11):1812–21. doi: 10.1136/gutjnl-2015-309957.
- Flint, H. J., S. H. Duncan, K. P. Scott, and P. Louis. 2007. Interactions and competition within the microbial community of the human colon: Links between diet and health. Environmental Microbiology 9 (5):1101–11. doi: 10.1111/j.1462-2920.2007.01281.x.
- Gallè, F., F. Valeriani, M. S. Cattaruzza, G. Gianfranceschi, R. Liguori, M. Antinozzi, B. Mederer, G. Liguori, and V. Romano Spica. 2020. Mediterranean diet, physical activity and gut microbiome composition: A cross-sectional study among healthy young Italian adults. Nutrients 12 (7):2164. doi: 10.3390/nu12072164.
- Garcia-Mantrana, I., M. Selma-Royo, C. Alcantara, and M. C. Collado. 2018. Shifts on gut microbiota associated to mediterranean diet adherence and specific dietary intakes on general adult population. Frontiers in Microbiology 9:890. doi: 10.3389/fmicb.2018.00890.
- García-Montero, C., O. Fraile-Martínez, A. M. Gómez-Lahoz, L. Pekarek, A. J. Castellanos, F. Noguerales-Fraguas, S. Coca, L. G. Guijarro, N. García-Honduvilla, A. Asúnsolo, et al. 2021. Nutritional components in western diet versus mediterranean diet at the gut microbiota–immune system interplay. Implications for health and disease. Nutrients 13 (2):699. doi: 10.3390/nu13020699.
- Ghosh, T. S., S. Rampelli, I. B. Jeffery, A. Santoro, M. Neto, M. Capri, E. Giampieri, A. Jennings, M. Candela, S. Turroni, et al. 2020. Mediterranean diet intervention alters the gut microbiome in older people reducing frailty and improving health status: The NU-AGE 1-year dietary intervention across five European countries. Gut 69 (7):1218–28. doi: 10.1136/gutjnl-2019-319654.
- Gibson, G. R., R. Hutkins, M. E. Sanders, S. L. Prescott, R. A. Reimer, S. J. Salminen, K. Scott, C. Stanton, K. S. Swanson, P. D. Cani, et al. 2017. Expert consensus document: The International Scientific Association for Probiotics and Prebiotics (ISAPP) consensus statement on the definition and scope of prebiotics. Nature Reviews. Gastroenterology & Hepatology 14 (8):491–502. doi: 10.1038/nrgastro.2017.75.
- Goodrich, J. K., S. C. Di Rienzi, A. C. Poole, O. Koren, W. A. Walters, J. G. Caporaso, R. Knight, and R. E. Ley. 2014. Conducting a microbiome study. Cell 158 (2):250–62. doi: 10.1016/j.cell.2014.06.037.
- Griffin, L. E., Z. Djuric, C. J. Angiletta, C. M. Mitchell, M. E. Baugh, K. P. Davy, and A. P. Neilson. 2019. A Mediterranean diet does not alter plasma trimethylamine N-oxide concentrations in healthy adults at risk for colon cancer. Food & Function 10 (4):2138–47. doi: 10.1039/c9fo00333a.
- Gutiérrez-Díaz, I., T. Fernández-Navarro, B. Sánchez, A. Margolles, and S. González. 2016. Mediterranean diet and faecal microbiota: A transversal study. Food & Function 7 (5):2347–56. doi: 10.1039/c6fo00105j.
- Gutiérrez-Díaz, I., T. Fernández-Navarro, N. Salazar, B. Bartolomé, M. V. Moreno-Arribas, E. J. de Andres-Galiana, J. L. Fernández-Martínez, C. G. de Los Reyes-Gavilán, M. Gueimonde, and S. González. 2017. Adherence to a mediterranean diet influences the fecal metabolic profile of microbial-derived phenolics in a Spanish cohort of middle-age and older people. Journal of Agricultural and Food Chemistry 65 (3):586–95. doi: 10.1021/acs.jafc.6b04408.
- Haro, C., M. Montes-Borrego, O. A. Rangel-Zúñiga, J. F. Alcalá-Díaz, F. Gómez-Delgado, P. Pérez-Martínez, J. Delgado-Lista, G. M. Quintana-Navarro, F. J. Tinahones, B. B. Landa, et al. 2016b. Two healthy diets modulate gut microbial community improving insulin sensitivity in a human obese population. The Journal of Clinical Endocrinology and Metabolism 101 (1):233–42. doi: 10.1210/jc.2015-3351.
- Haro, C., S. Garcia-Carpintero, J. F. Alcala-Diaz, F. Gomez-Delgado, J. Delgado-Lista, P. Perez-Martinez, O. A. Rangel Zuñiga, G. M. Quintana-Navarro, B. B. Landa, J. C. Clemente, et al. 2016a. The gut microbial community in metabolic syndrome patients is modified by diet. Journal of Nutritional Biochemistry 27:27–31. doi: 10.1016/j.jnutbio.2015.08.011.
- Haro, C., S. García-Carpintero, O. A. Rangel-Zúñiga, J. F. Alcalá-Díaz, B. B. Landa, J. C. Clemente, P. Pérez-Martínez, J. López-Miranda, F. Pérez-Jiménez, and A. Camargo. 2017. Consumption of two healthy dietary patterns restored microbiota dysbiosis in obese patients with metabolic dysfunction. Molecular Nutrition & Food Research 61 (12):1700300. doi: 10.1002/mnfr.201700300.
- He, Y., W. Wu, H.-M. Zheng, P. Li, D. McDonald, H.-F. Sheng, M.-X. Chen, Z.-H. Chen, G.-Y. Ji, Z.-D.-X. Zheng, et al. 2018. Regional variation limits applications of healthy gut microbiome reference ranges and disease models. Nature Medicine 24 (10):1532–5. doi: 10.1038/s41591-018-0164-x.
- Higgins, J. P. T, and S. E. Green. 2011. Cochrane handbook for systematic reviews of interventions version 5.1.0. London, UK: The Cochrane Collaboration.
- Hills, R. D., B. A. Pontefract, H. R. Mishcon, C. A. Black, S. C. Sutton, and C. R. Theberge. 2019. Gut microbiome: Profound implications for diet and disease. Nutrients 11 (7):1613. doi: 10.3390/nu11071613.
- Hooper, L. V., and J. I. Gordon. 2001. Commensal host-bacterial relationships in the gut. Science (New York, NY) 292 (5519):1115–8. doi: 10.1126/science.1058709.
- Hutchins-Wiese, H. L., C. W. Bales, and K. N. Porter Starr. 2021. Mediterranean diet scoring systems: Understanding the evolution and applications for Mediterranean and non-Mediterranean countries. British Journal of Nutrition:1–22. doi: 10.1017/S0007114521002476.
- Islam, M. M., U. Iqbal, B. Walther, S. Atique, N. K. Dubey, P.-A. Nguyen, T. N. Poly, J. H. B. Masud, Y.-C. Li, and S.-A. Shabbir. 2016. Benzodiazepine use and risk of dementia in the elderly population: A systematic review and meta-analysis. Neuroepidemiology 47 (3-4):181–91. doi: 10.1159/000454881.
- Istas, G., E. Wood, M. Le Sayec, C. Rawlings, J. Yoon, V. Dandavate, D. Cera, S. Rampelli, A. Costabile, E. Fromentin, and A. Rodriguez-Mateos. 2019. Effects of aronia berry (poly)phenols on vascular function and gut microbiota: a double-blind randomized controlled trial in adult men. The American Journal of Clinical Nutrition 110:316–329. doi: 10.1093/ajcn/nqz075.
- Jackson, D, and R. Turner. 2017. Power analysis for random-effects meta-analysis. Research Synthesis Methods 8 (3):290–302. doi: 10.1002/jrsm.1240.
- Kim, Y, and Y. Je. 2017. Flavonoid intake and mortality from cardiovascular disease and all causes: A meta-analysis of prospective cohort studies. Clinical Nutrition ESPEN 20:68–77. doi: 10.1016/j.clnesp.2017.03.004.
- Leeming, E. R., P. Louca, R. Gibson, C. Menni, T. D. Spector, and C. I. Le Roy. 2021. The complexities of the diet-microbiome relationship: Advances and perspectives. Genome Medicine 13 (1):10. doi: 10.1186/s13073-020-00813-7.
- Li, Z., G. Quan, X. Jiang, Y. Yang, X. Ding, D. Zhang, X. Wang, P. R. Hardwidge, W. Ren, and G. Zhu. 2018. Effects of metabolites derived from gut microbiota and hosts on pathogens. Frontiers in Cellular and Infection Microbiology 8:314. doi: 10.3389/fcimb.2018.00314.
- Liberati, A., D. G. Altman, J. Tetzlaff, C. Mulrow, P. C. Gøtzsche, J. P. A. Ioannidis, M. Clarke, P. J. Devereaux, J. Kleijnen, and D. Moher. 2009. The PRISMA statement for reporting systematic reviews and meta-analyses of studies that evaluate healthcare interventions: Explanation and elaboration. BMJ (Clinical Research ed.) 339:b2700. doi: 10.1136/bmj.b2700.
- Lindstad, L., G. Lo, S. Leivers, Z. Lu, L. Michalak, G. Pereira, Å. Røhr, E. Martens, L. McKee, P. Louis, et al. 2021. Human gut Faecalibacterium prausnitzii deploys a highly efficient conserved system to cross-feed on β-Mannan-derived oligosaccharides. mBio 12 (3):e03628–20. doi: 10.1128/mBio.03628-20.
- Lloyd-Price, J., G. Abu-Ali, and C. Huttenhower. 2016. The healthy human microbiome. Genome Medicine 8 (1):51. doi: 10.1186/s13073-016-0307-y.
- Ma, G. and Y. Chen. 2020. Polyphenol supplementation benefits human health via gut microbiota: A systematic review via meta-analysis. Journal of Functional Foods 66:103829.
- Maldonado-Contreras, A., S. E. Noel, D. V. Ward, M. Velez, and K. M. Mangano. 2020. Associations between diet, the gut microbiome, and short-chain fatty acid production among older Caribbean Latino adults. Journal of the Academy of Nutrition and Dietetics 120 (12):2047–60.e6. doi: 10.1016/j.jand.2020.04.018.
- Mariat, D., O. Firmesse, F. Levenez, V. Guimarăes, H. Sokol, J. Doré, G. Corthier, and J. Furet. 2009. The Firmicutes/Bacteroidetes ratio of the human microbiota changes with age. BMC Microbiology 9:123–6. doi: 10.1186/1471-2180-9-123.
- Martín, R., S. Miquel, L. Benevides, C. Bridonneau, V. Robert, S. Hudault, F. Chain, O. Berteau, V. Azevedo, J. M. Chatel, et al. 2017. Functional characterization of novel Faecalibacterium prausnitzii strains isolated from healthy volunteers: A step forward in the use of F. prausnitzii as a next-generation probiotic. Frontiers in Microbiology 8:1226. doi: 10.3389/fmicb.2017.01226.
- Maskarinec, G., M. A. J. Hullar, K. R. Monroe, J. A. Shepherd, J. Hunt, T. W. Randolph, L. R. Wilkens, C. J. Boushey, L. Le Marchand, U. Lim, et al. 2019. Fecal microbial diversity and structure are associated with diet quality in the multiethnic cohort adiposity phenotype study. The Journal of Nutrition 149 (9):1575–84. doi: 10.1093/jn/nxz065.
- McBurney, M. I., C. Davis, C. M. Fraser, B. O. Schneeman, C. Huttenhower, K. Verbeke, J. Walter, and M. E. Latulippe. 2019. Establishing what constitutes a healthy human gut microbiome: State of the science, regulatory considerations, and future directions. The Journal of Nutrition 149 (11):1882–95. doi: 10.1093/jn/nxz154.
- Merra, G., A. Noce, G. Marrone, M. Cintoni, M. G. Tarsitano, A. Capacci, and A. De Lorenzo. 2020. Influence of mediterranean diet on human gut microbiota. Nutrients 13 (1):7. doi: 10.3390/nu13010007.
- Meslier, V., M. Laiola, H. M. Roager, F. D. Filippis, H. Roume, B. Quinquis, R. Giacco, I. Mennella, R. Ferracane, N. Pons, et al. 2020. Mediterranean diet intervention in overweight and obese subjects lowers plasma cholesterol and causes changes in the gut microbiome and metabolome independently of energy intake. Gut 69 (7):1258–68. doi: 10.1136/gutjnl-2019-320438.
- Mitsou, E. K., A. Kakali, S. Antonopoulou, K. C. Mountzouris, M. Yannakoulia, D. B. Panagiotakos, and A. Kyriacou. 2017. Adherence to the Mediterranean diet is associated with the gut microbiota pattern and gastrointestinal characteristics in an adult population. The British Journal of Nutrition 117 (12):1645–55. doi: 10.1017/S0007114517001593.
- Modesti, P. A., G. Reboldi, F. P. Cappuccio, C. Agyemang, G. Remuzzi, S. Rapi, E. Perruolo, G. Parati, ESH Working Group on CV Risk in Low Resource Settings. 2016. Panethnic differences in blood pressure in Europe: A systematic review and meta-analysis. PLoS One 11 (1):e0147601. doi: 10.1371/journal.pone.0147601.
- Nagpal, R., B. J. Neth, S. Wang, S. Craft, and H. Yadav. 2019. Modified Mediterranean-ketogenic diet modulates gut microbiome and short-chain fatty acids in association with Alzheimer’s disease markers in subjects with mild cognitive impairment. EBioMedicine 47:529–42. doi: 10.1016/j.ebiom.2019.08.032.
- Pagliai, G., E. Russo, E. Niccolai, M. Dinu, V. Di Pilato, A. Magrini, G. Bartolucci, S. Baldi, M. Menicatti, B. Giusti, et al. 2020. Influence of a 3-month low-calorie Mediterranean diet compared to the vegetarian diet on human gut microbiota and SCFA: The CARDIVEG Study. European Journal of Nutrition 59 (5):2011–24. doi: 10.1007/s00394-019-02050-0.
- Palmu, J., A. Salosensaari, S. Havulinna Aki, S. Cheng, M. Inouye, M. Jain, A. Salido Rodolfo, K. Sanders, C. Brennan, C. Humphrey Gregory, et al. 2020. Association between the gut microbiota and blood pressure in a population cohort of 6953 individuals. Journal of the American Heart Association 9 (15):e016641. doi: 10.1161/JAHA.120.016641.
- Park, J. E., M. Miller, J. Rhyne, Z. Wang, and S. L. Hazen. 2019. Differential effect of short-term popular diets on TMAO and other cardio-metabolic risk markers. Nutrition, Metabolism, and Cardiovascular Diseases: NMCD 29 (5):513–7. doi: 10.1016/j.numecd.2019.02.003.
- Pastori, D., R. Carnevale, C. Nocella, M. Novo, M. Santulli, V. Cammisotto, D. Menichelli, P. Pignatelli, and F. Violi. 2017. Gut-derived serum lipopolysaccharide is associated with enhanced risk of major adverse cardiovascular events in atrial fibrillation: Effect of adherence to mediterranean diet. Journal of the American Heart Association 6 (6):e005784. doi: 10.1161/JAHA.117.005784.
- Picchianti Diamanti, A., C. Panebianco, G. Salerno, R. Di Rosa, S. Salemi, M. L. Sorgi, G. Meneguzzi, M. B. Mariani, A. Rai, D. Iacono, et al. 2020. Impact of mediterranean diet on disease activity and gut microbiota composition of rheumatoid arthritis patients. Microorganisms 8 (12):1989. doi: 10.3390/microorganisms8121989.
- Pignanelli, M., C. Just, C. Bogiatzi, V. Dinculescu, G. B. Gloor, E. Allen-Vercoe, G. Reid, B. L. Urquhart, K. N. Ruetz, T. J. Velenosi, et al. 2018. Mediterranean diet score: Associations with metabolic products of the intestinal microbiome, carotid plaque burden, and renal function. Nutrients 10 (6):779. doi: 10.3390/nu10060779.
- Quercia, S., S. Turroni, J. Fiori, M. Soverini, S. Rampelli, E. Biagi, A. Castagnetti, C. Consolandi, M. Severgnini, M. Pianesi, et al. 2017. Gut microbiome response to short-term dietary interventions in reactive hypoglycemia subjects. Diabetes/Metabolism Research and Reviews 33 (8):e2927. doi: 10.1002/dmrr.2927.
- Reis, J. F., V. V. S. Monteiro, R. de Souza Gomes, M. M. do Carmo, G. V. da Costa, P. C. Ribera, A. Monteiro, M. C. 2016. Action mechanism and cardiovascular effect of anthocyanins: a systematic review of animal and human studies. Journal of Translational Medicine 14:315. doi: 10.1186/s12967-016-1076-5.
- Riaz Rajoka, M. S., J. Shi, H. M. Mehwish, J. Zhu, Q. Li, D. Shao, Q. Huang, and H. Yang. 2017. Interaction between diet composition and gut microbiota and its impact on gastrointestinal tract health. Food Science and Human Wellness 6 (3):121–30. doi: 10.1016/j.fshw.2017.07.003.
- Rinott, E., I. Youngster, A. Y. Meir, G. Tsaban, H. Zelicha, A. Kaplan, D. Knights, K. Tuohy, F. Fava, M. U. Scholz, et al. 2021. Effects of diet-modulated autologous fecal microbiota transplantation on weight regain. Gastroenterology 160 (1):158–73.e10. doi: 10.1053/j.gastro.2020.08.041.
- Rothschild, D., O. Weissbrod, E. Barkan, A. Kurilshikov, T. Korem, D. Zeevi, P.I. Costea, A. Godneva, I.N. Kalka, N. Bar et al., 2018. Environment dominates over host genetics in shaping human gut microbiota. Nature 555:210–215. doi: 10.1038/nature25973.
- Rowland, I., G. Gibson, A. Heinken, K. Scott, J. Swann, I. Thiele, and K. Tuohy. 2018. Gut microbiota functions: Metabolism of nutrients and other food components. European Journal of Nutrition 57 (1):1–24. doi: 10.1007/s00394-017-1445-8.
- Ruiz-Saavedra, S., N. Salazar, A. Suárez, C. G. de Los Reyes-Gavilán, M. Gueimonde, and S. González. 2020. Comparison of different dietary indices as predictors of inflammation, oxidative stress and intestinal microbiota in middle-aged and elderly subjects. Nutrients 12 (12):3828. doi: 10.3390/nu12123828.
- Santos-Marcos, J. A., C. Haro, A. Vega-Rojas, J. F. Alcala-Diaz, H. Molina-Abril, A. Leon-Acuña, J. Lopez-Moreno, B. B. Landa, M. Tena-Sempere, P. Perez-Martinez, et al. 2019. Sex differences in the gut microbiota as potential determinants of gender predisposition to disease. Molecular Nutrition & Food Research 63 (7):e1800870. doi: 10.1002/mnfr.201800870.
- Schulze, M. B., M. A. Martínez-González, T. T. Fung, A. H. Lichtenstein, and N. G. Forouhi. 2018. Food based dietary patterns and chronic disease prevention. BMJ (Clinical Research ed.) 361:k2396. doi: 10.1136/bmj.k2396.
- Shannon, O. M., B. C. M. Stephan, A. Granic, M. Lentjes, S. Hayat, A. Mulligan, C. Brayne, K.-T. Khaw, R. Bundy, S. Aldred, et al. 2019. Mediterranean diet adherence and cognitive function in older UK adults: The European prospective investigation into cancer and nutrition-norfolk (EPIC-Norfolk) Study. American Journal of Clinical Nutrition. 110:938–948. doi: 10.1093/ajcn/nqz114.
- Shishtar, E., G. T. Rogers, J. B. Blumberg, R. Au, P. F. Jacques. 2020. Long-term dietary flavonoid intake and risk of Alzheimer disease and related dementias in the Framingham Offspring Cohort. American Journal of Clinical Nutrition. 112:343–353. doi: 10.1093/ajcn/nqaa079.
- Singh, R., H.-W. Chang, D. Yan, K. Lee, D. Ucmak, K. Wong, M. Abrouk, B. Farahnik, M. Nakamura, T. H. Zhu, et al. 2017. Influence of diet on the gut microbiome and implications for human health. Journal of Translational Medicine 15 (1):73. doi: 10.1186/s12967-017-1175-y.
- Staudacher, H. M., P. M. Irving, M. C. E. Lomer, and K. Whelan. 2017. The challenges of control groups, placebos and blinding in clinical trials of dietary interventions. The Proceedings of the Nutrition Society 76 (3):203–12. doi: 10.1017/S0029665117000350.
- Threapleton, D. E., D. C. Greenwood, C. E. L. Evans, C. L. Cleghorn, C. Nykjaer, C. Woodhead, J. E. Cade, C. P. Gale, V. J. Burley. 2013. Dietary fibre intake and risk of cardiovascular disease: systematic review and meta-analysis. BMJ 347:f6879. doi: 10.1136/bmj.f6879.
- Tomas-Barberan, F. A., M. V. Selma, and J. C. Espín. 2018. Polyphenols’ gut microbiota metabolites: Bioactives or biomarkers? Journal of Agricultural and Food Chemistry 66 (14):3593–4. doi: 10.1021/acs.jafc.8b00827.
- Tsigalou, C., A. Paraschaki, A. Karvelas, K. Kantartzi, K. Gagali, D. Tsairidis, and E. Bezirtzoglou. 2021. Gut microbiome and Mediterranean diet in the context of obesity. Current knowledge, perspectives and potential therapeutic targets. Metabolism Open 9:100081. doi: 10.1016/j.metop.2021.100081.
- Tuohy, K. M., L. Conterno, M. Gasperotti, and R. Viola. 2012. Up-regulating the human intestinal microbiome using whole plant foods, polyphenols, and/or fiber. Journal of Agricultural and Food Chemistry 60 (36):8776–82. doi: 10.1021/jf2053959.
- Umoh, F. I., I. Kato, J. Ren, P. L. Wachowiak, M. T. Ruffin, D. K. Turgeon, A. Sen, D. E. Brenner, and Z. Djuric. 2016. Markers of systemic exposures to products of intestinal bacteria in a dietary intervention study. European Journal of Nutrition 55 (2):793–8. doi: 10.1007/s00394-015-0900-7.
- Valdes, A. M., J. Walter, E. Segal, and T. D. Spector. 2018. Role of the gut microbiota in nutrition and health. BMJ (Clinical Research ed.) 361:k2179. doi: 10.1136/bmj.k2179.
- Valeriani, F., F. Gallè, M. S. Cattaruzza, M. Antinozzi, G. Gianfranceschi, N. Postiglione, V. Romano Spica, and G. Liguori. 2020. Are nutrition and physical activity associated with gut microbiota? A pilot study on a sample of healthy young adults. Annali di Igiene: Medicina Preventiva e di Comunita 32 (5):521–7. doi: 10.7416/ai.2020.2372.
- van der Hee, B, and J. M. Wells. 2021. Microbial regulation of host physiology by short-chain fatty acids. Trends in Microbiology 29 (8):700–12. doi: 10.1016/j.tim.2021.02.001.
- Vázquez-Fresno, R., R. Llorach, M. Urpi-Sarda, A. Lupianez-Barbero, R. Estruch, D. Corella, M. Fitó, F. Arós, M. Ruiz-Canela, J. Salas-Salvadó, et al. 2015. Metabolomic pattern analysis after mediterranean diet intervention in a nondiabetic population: A 1- and 3-year follow-up in the PREDIMED study. Journal of Proteome Research 14 (1):531–40. doi: 10.1021/pr5007894.
- Velasquez-Manoff, M. 2015. Gut microbiome: the peacekeepers. Nature 518 (7540):S3–S5. doi: 10.1038/518S3a.
- Vernocchi, P., F. Del Chierico, and L. Putignani. 2016. Gut microbiota profiling: Metabolomics based approach to unravel compounds affecting human health. Frontiers in Microbiology 7:1144. doi: 10.3389/fmicb.2016.01144.
- Wang, D. D., L. H. Nguyen, Y. Li, Y. Yan, W. Ma, E. Rinott, K. L. Ivey, I. Shai, W. C. Willett, F. B. Hu, et al. 2021. The gut microbiome modulates the protective association between a Mediterranean diet and cardiometabolic disease risk. Nature Medicine 27 (2):333–43. doi: 10.1038/s41591-020-01223-3.
- Wells, G. A. Shea, B. O’Connel, D. Peterson, J. Welch, V. Losos, and M. Tugwell. 2000. The Newcastle-Ottawa scale (NOS) for assessing the quailty of nonrandomised studies in meta-analyses. https://www.ohri.ca/programs/clinical_epidemiology/oxford.asp
- Willis, A. D. 2019. Rarefaction, alpha diversity, and statistics. Frontiers in Microbiology 10:2407. doi: 10.3389/fmicb.2019.02407.
- Zamora-Ros, R., M. Rabassa, A. Cherubini, M. Urpí-Sardà, S. Bandinelli, L. Ferrucci, C. Andres-Lacueva. 2013. High concentrations of a urinary biomarker of polyphenol intake are associated with decreased mortality in older adults. The Journal of nutrition 143:1445–1450. doi: 10.1136/gutjnl-2019-320438.
- Zhu, C., L. Sawrey-Kubicek, E. Beals, C. H. Rhodes, H. E. Houts, R. Sacchi, and A. M. Zivkovic. 2020. Human gut microbiome composition and tryptophan metabolites were changed differently by fast food and Mediterranean diet in 4 days: A pilot study. Nutrition Research (New York, NY) 77:62–72. doi: 10.1016/j.nutres.2020.03.005.