Abstract
The human brain grows rapidly in early life which requires adequate nutrition. Human milk provides optimal nutrition for the developing brain, and breastfeeding significantly improves the cognition development of infants. These benefits have been largely attributed to human milk oligosaccharides (HMOS), associated with sialic acid (Sia). Subsequently, sialylated HMOS present a vital source of exogenous Sia to infants. Sialic acid is a key molecule essential for proper development of gangliosides, and therefore critical in brain development and function. Recent pre-clinical studies suggest dietary supplementation with Sia or sialylated oligosaccharides enhances intelligence and cognition performance in early and later life. Furthermore, emerging evidence suggests the involvement of Sia in brain homeostasis and disbalance correlates with common pathologies such as Alzheimer’s disease (AD). Therefore, this review will discuss early brain health and development and the role of Sia in this process. Additionally, studies associating breastfeeding and specific HMOS to benefits in cognitive development are critically assessed. Furthermore, the review will assess studies implying the potential role of HMOS and microbiota in brain development via the gut-brain axis. Finally, the review will summarize recent advances regarding the role of Sia in neurodegenerative disease in later life and potential roles of dietary Sia sources.
Introduction
Globally, infants reserve the right to receive good and adequate nutrition (UNCRC Citation2009). Human milk is considered the optimal food for infants by the World Health Organization (WHO), which recommends mothers to exclusively breastfeed their infants for six months and continue to partially breastfeed up to at least two years. Breastfed infants have lower rates of autoimmune diseases, allergy, and gastrointestinal complaints in comparison to formula-fed infants (Matheson, Allen, and Tang Citation2012; Vieira Borba, Sharif, and Shoenfeld Citation2018). Likewise, breastfeeding has been associated with a reduced risk of becoming overweight and diabetic in adulthood (Eidelman et al. Citation2012; León-Cava et al. Citation2002; Mamun et al. Citation2015). Furthermore, prolonged breastfeeding has been associated with a higher intellectual quotient (IQ), school performance, and income in later life in contrast to formula-fed infants (C.G. Victora et al. Citation2015; Horta, De Mola, and Victora Citation2015; Isaacs et al. Citation2010; Kramer et al. Citation2008). Although breastfeeding is highly recommended, according to the “global breastfeeding scorecard, 2019,” only 41% of infants under six months of age are exclusively breastfed worldwide (UNICEF Citation2019), highlighting that globally the majority of infants do not receive the optimal nutrition.
Human milk is a complex mixture of macro- and micronutrients, with multiple bioactive factors (Ballard and Morrow Citation2013), which is currently impossible to mimic completely in infant formula. One group of bioactive components that are challenging to mimic is human milk oligosaccharides (HMOS). Human milk oligosaccharides are composed of six monosaccharides, i.e. glucose (Glc), galactose (Gal), N-acetyl-D-glucosamine (GlcNAc), N-acetyl-D-galactosamine (GalNAc), L-fucose (Fuc), and N-acetyl-D-neuraminic acid (Neu5Ac). The structural complexity of HMOS is large; i.e. 162 structures have been identified and over 240 compositional varieties have been observed, which is unique to human milk (Bode Citation2012; Urashima et al. Citation2018). To date, there has been ample evidence reported for the local and systemic beneficial effects of HMOS on infant development and health (B. Wang and Brand-Miller Citation2003; B. Wang Citation2009; Cheng et al. Citation2021; C. Kunz et al. Citation2000; Moossavi et al. Citation2019; Triantis, Bode, and van Neerven Citation2018). Human milk oligosaccharides can be regarded as “prebiotics” to shape a balanced and diverse infant intestinal microbiota (Borewicz et al. Citation2020; Liu et al. Citation2021; Moossavi et al. Citation2019). Human milk oligosaccharides serve as decoy receptors to prevent the adhesion of pathogens to host mucosal surfaces (C. Kunz et al. Citation2000). Furthermore, HMOS directly modulate intestinal epithelial cells and play a role in developing a balanced immune response (Triantis, Bode, and van Neerven Citation2018); Last but not least, human milk oligosaccharides have been implicated as a nutrient source for brain development (B. Wang and Brand-Miller Citation2003; B. Wang Citation2009).
Human milk oligosaccharides are present in various forms, with approximately 14–33% being cross-linked with Neu5Ac, also known as sialic acid (Sia) (Ninonuevo et al. Citation2006; Soyyilmaz et al. Citation2021; Thurl et al. Citation2017). Levels of Sia in bovine milk based infant formula are more limited (B. Wang et al. Citation2001a). A healthy brain contains high levels of Sia (Schnaar, Gerardy-Schahn, and Hildebrandt Citation2014) and Sia plays a fundamental role in brain development and health (B. Wang and Brand-Miller Citation2003; B. Wang Citation2009, Citation2012; Karim and Wang Citation2006; Schnaar, Gerardy-Schahn, and Hildebrandt Citation2014; Weinhold et al. Citation2005). The present review will discuss early brain development and the role of Sia in this process. Additionally, studies associating breastfeeding and specific HMOS to benefits in cognitive development are critically assessed. Furthermore, the review will assess studies implying the potential role of HMOS in brain development via the gut-brain axis. Finally, the review will summarize recent advances regarding the role of Sia in neurodegenerative disease in later life and the potential roles of external Sia sources.
Early human brain development
Human brain development begins after three weeks of gestation, and the fundamental structures are formed at the eighth gestational week, when also the blood-brain barrier is formed (Stiles and Jernigan Citation2010).
After birth, crucial processes such as myelination, synaptogenesis, and synapse pruning, peak during infancy () (Deoni Citation2018). Brain maturation is a complex and lasting process, which occurs in different stages and maturation is fully accomplished at approximately 25 years of age (Arain et al. Citation2013). In the first few years, the brain grows rapidly and during the first two years of life; the brain reaches nearly 80% of mature brain weight (Black Citation2018; B. Wang Citation2012). By the age of six, children have approximately 90% of adult brain mass (Brown and Jernigan Citation2012). Genetic and environmental factors are key influencers of brain development (Fusco et al. Citation2019; Gao et al. Citation2019; Stiles and Jernigan Citation2010). For example, exposure to a stimulating environment is a factor in increasing the infant’s IQ score. Other factors, such as prenatal drug exposures, parental engagement, mood, music, art, and quality of education also have clear influence on cognitive development (Gao et al. Citation2019; Horta, De Mola, and Victora 2015; Kramer et al. Citation2008). It has also been observed that dietary factors play a role, for example, Long-Chain Polyunsaturated Fatty Acids (LC-PUFA), in particular docosahexaenoic acid (DHA), are associated with cognitive maturation (Heird Citation2001; Lauritzen et al. Citation2016). Breastfeeding and milk composition have been associated with brain developmental outcomes (B. Wang Citation2009).
Figure 1. Overview of human brain development processes. Apart from Courchesne et al. Citation2019; Menassa and Gomez-Nicola (Citation2018). The total Sia concentration in maternal serum and saliva in first, second and third trimester of pregnancy (Alvi, Amer, and Sumerin Citation1988; Chhabra et al. Citation2015; Koç Öztürk et al. Citation2010; Salvolini et al. Citation1998). Abbreviations: BBB, blood-brain barrier; Sia, sialic acids.
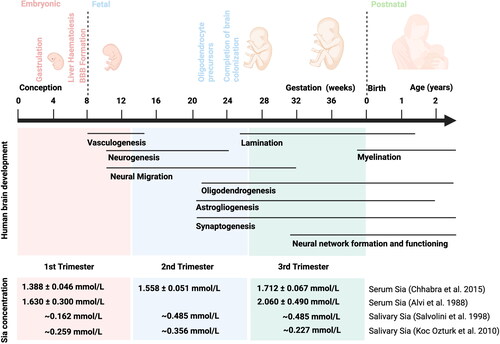
Early life nutrition and brain development
Nutrition is regarded as a critical factor in early brain growth (Black Citation2018; B. Wang Citation2012; Deoni Citation2018). Numerous studies have shown the beneficial effects of breastfeeding on increasing IQ test performance in comparison to formula-fed infants (Cai et al. Citation2015; Horta, De Mola, and Victora 2015; Isaacs et al. Citation2010; Kramer et al. Citation2008; Pang et al. Citation2020; Victora et al. Citation2015).
In 2015 Horta et al. carried out a systematic review and meta-analysis to assess the relationship between breastfeeding and performance in intelligence tests (Horta, De Mola, and Victora 2015). Two independent searches were performed by Medline, LILACS, SCIELO and Web of Science resulted in over 8000 hits, that were curated to 36 relevant papers that were curated to 17 papers that were included in the meta-analysis. Fixed- and random-effects models were used to pool the effect estimates, and a random-effects regression was used to assess potential sources of heterogeneity (Horta, De Mola, and Victora 2015).
Due to potential confounding influences, data collected from subjects was analyzed relative to maternal IQ to avoid the possible effects of differing parental intelligence on study outcomes. One of the major challenges is the variety in cognitive assessment tests that cannot always be readily compared. Also, timing of cognitive assessment varies between ages 1 to 15 years. Following data collection, the breastfeeding cohort still showed an elevated IQ compared to the non-breast-fed group (mean difference 2.62 points; 95% confidence: 1.25–3.98 points) following adjustment for maternal IQ differences (Horta, De Mola, and Victora 2015). When considering IQ differences at later ages (10–19 years) the effect of breastfeeding was reduced, but still significant (1.92 points; 95% confidence: 0.43–3.40 points). The studies included were carried out between 1988 and 2014, it should therefore be taken into account that formula milk composition has changed significantly in that timeframe. To note, a randomized trial also observed a positive association between breastfeeding and cognition (Horta, De Mola, and Victora 2015).
The Horta review excluded several papers that are still interesting to note. Several studies have highlighted a particular positive correlation of verbal or language IQ with breastfeeding (Cai et al. Citation2015; Isaacs et al. Citation2010; Kramer et al. Citation2008). Kramer et al. (Citation2008) describes a large cluster-randomized trial which was the Promotion of Breastfeeding Intervention Trial (PROBIT). Parents were stimulated to breastfeed in some maternity hospitals, while in others the regular practice was maintained. The study corrects for class, parental education and hereditary influences by randomizing the study population. The study included 17,046 healthy infants, randomized in two groups of whom 13,889 infants were followed up at age 6.5 years, to assess the effect of breastfeeding on children’s cognition (Kramer et al. Citation2008). In the intervention group, more mothers maintain breastfeeding longer than in the control group; i.e. 72.7% versus 60.0% at 3 months postpartum, to 19.7% versus 11.4% at 12 months. Also, the amounts of women exclusively breastfeeding was higher in the intervention group; i.e. 43.3% versus 6.4% at 3 months postpartum. In this study, the cognition test included subtest and IQ scores on the Wechsler Abbreviated Scales of Intelligence, teacher evaluations of academic performance in reading, writing, mathematics, and other subjects (Kramer et al. Citation2008). The results showed term infants who were randomized to the breastfeeding promotion intervention had higher verbal performance and full-scale IQ at age 6.5 years (Kramer et al. Citation2008).
Isaacs et al. (Citation2010) studied a relatively small group of preterm born infants (24 girls, 26 boys). The participants were divided into three groups; 28 infants were fed with a nutrient-enriched preterm formula (PTF), 13 infants were fed with a standard term formula (TF), and 9 infants received banked breast milk (BBM) (Isaacs et al. Citation2010). This study also performed Wechsler based IQ testing, appropriate for age at 15 y, 9 mo age. Beside the IQ testing, the volumes of total brain, white and gray matter were obtained by magnetic resonance imaging (MRI) scans at the mean age of 15 y 9 mo. Similar to Kramer et al. (Citation2008), this study observed a positive correlation between BBM feeding and verbal IQ. Moreover, the effects of breast milk were seen strongly on white matter in the brain in young males, whereas no significant relationships were found in girls (Isaacs et al. Citation2010). Moreover, excluding the BBM supplemented group showed that while the fortified or non-fortified supplements showed no predictive value for IQ, the percentage of expressed breastmilk received from the mothers did correlate significantly with verbal IQ and full-scale IQ. However, the study has limitations; firstly, there is no experimental design, which renders causation impossible to assess. Secondly, the BBM group consists of only 9 participants, and the total study only 50 participants limiting the power of the study.
Cai et al. (Citation2015) performed a detailed, blinded study to analyze cognitive development in relation to breastfeeding practice in a Singapore prospective cohort study, consisting of 408 participants (Cai et al. Citation2015). A diverse set of 15 cognitive tests were performed at ages of 6, 18 and 24 months. It should be noted that 212 mother-child pairs completed all tasks set at the three-time points. After correction for maternal education level and socio-economic status, the authors observed significant effects of breastfeeding practice in 4 out of 15 tasks, particularly in memory and language skills (Cai et al. Citation2015).
During the study, participants were stratified into high-, intermediate- and low breastfeeding groups. The definition of high breastfeeding (n = 54) was based on exclusive or predominant breastfeeding for at least four months, and continued partial breastfeeding up to six months of age. Low breastfeeding (n = 170) was defined as weaning off breastfeeding and exclusive formula feeding before three months of age. The intermediate breastfeeding group (n = 184) consisted of infants that were at least (predominantly) breastfed for three months, but did not meet high-breastfeeding criteria (Cai et al. Citation2015). It should be noted that the groups have broad definitions, and almost all infants received at least some breastfeeding. The researchers also have detailed information on time-of-weaning, allowing a semi-continuous scale to present dose-dependent correlations. Moreover, the high-breastfeeding group consisted of only 54 participants, of which most had diploma, technical education or university degree and none had only primary education (Cai et al. Citation2015). The low number of participants in some of the many stratified groups might result in lack of power to observe some of the potential effects. Another notable factor in the cohort is the high exposure of the infants to bilingual conditions ∼80%. Literature on bilingual advantage and early cognitive development is mixed in its findings, but an effect on early cognitive development may not be discounted either (D’Souza et al. Citation2020).
Subsequently, Victora et al. (Citation2015) performed a prospective, population-based birth cohort study to assess whether breastfeeding duration was associated with IQ test performance, educational attainment, and income at the age of 30 years in a Brazil cohort (Victora et al. Citation2015). In this study, information regarding IQ and breastfeeding was collected from 3493 participants. Furthermore, following delivery, information concerning breastfeeding behavior and food intake was recorded throughout the duration of the study (Victora et al. Citation2015). An intelligence test was performed, which included arithmetic, digit symbol, similarities, and picture completion subtests at a mean age of 30.2 years. At the same time, their educational attainment and income were recorded in the previous month (Victora et al. Citation2015).
It should be noted that the researchers analyzed the data with respect to the confounding variables, including maternal education, family income, birthweight and sex at delivery. The results presented that breastfeeding was associated with improved performance in intelligence tests in subjects at the age of thirty, and a strong association between IQ and family income at birth were found (Victora et al. Citation2015). To note, few Brazilians were aware of the benefits of breastfeeding, and no clear social patterning of breastfeeding existed for family income or parental education in Brazil. However, the study still has some potential limitations. Firstly, researchers only record the month preceding the interview. Additionally, findings did not take into account the effect of intelligence differences due to familial history as no IQ tests were performed on subjects’ biological parents. The study suggested that the beneficial effect of breastfeeding on cognition may continue to adulthood.
In order to detect whether the positive effect of breastfeeding on cognition was the result of breast milk composition or due to the mother-child contact during breast feeding, Pang et al. (Citation2020) explored the associations between type of milk feeding and mode of breast milk feeding in the Growing Up in Singapore Toward healthy Outcomes (GUSTO) cohort (Pang et al. Citation2020). A neurodevelopmental assessment was performed at the ages of 6, 18, 24, 41, 48 and 54 months. It should be noted all children were offered a neurodevelopmental assessment at 48 months, while a subset of children participated at 6, 18, 24, 41, and 54 months. Neurocognitive assessments included paper and pencil/computerized tasks requiring motor and/or verbal responses, behavioral observation and eye tracking (Pang et al. Citation2020).
During the study, participants characteristics were recorded by nursing (breast milk only) and nutrients (fed by bottle) at three months postpartum. For “nursing,” researchers included infants who were fully fed breast milk, comparing those fed directly at the breast (n = 59) versus those fed partially or completely by expressed milk via bottle (n = 63). For “nutrients,” researchers compared infants exclusively bottle-fed based on type of milk received: formula only (n = 296) versus some or all breast milk (n = 73) (Pang et al. Citation2020). The results showed that compared with bottle-fed formula only babies, bottle-fed breast milk babies performed better on the Bayley Scales of Infant and Toddler Development as well as gross motor skills at two years old, and on the Kaufman Brief Intelligence Test at 4.5 years old (Pang et al. Citation2020). Infants who fed directly at the breast had higher scores on several memory tasks compared to infants who were bottle-fed breast milk (Pang et al. Citation2020). However, this study only defined the type of nursing at three months of age. It is unable to detect whether the neurocognitive outcomes would be similar if the type of nursing was also compared at later ages. The small sizes for some cognitive measures conducted are another notable factor. To conclude, human milk itself is a key factor in brain development, although nursing-contact seems to play a role.
Taken together, there is clear evidence that breastfeeding is beneficial for brain development and cognition. Several studies have found a particular increase in linguistic ability (Cai et al. Citation2015; Horta, De Mola, and Victora 2015; Isaacs et al. Citation2010; Kramer et al. Citation2008). Although there is some influence of the nursing contact, the composition of breast milk, in contrast with formula also plays a role.
None of the above studies mentioned have specifically analyzed the breastmilk composition. To link specific nutrients, such as human milk oligosaccharides or DHA, the analysis of the milk composition should be taken into account as well. Human milk oligosaccharides are the third major component of human milk following lactose and lipids, amounting to approximately 24 g/L in colostrum, and 12 g/L in mature milk (Chen Citation2015; Soyyilmaz et al. Citation2021; Thurl et al. Citation2017; Van Leeuwen Citation2019). DHA is found in breast milk, and is known to play a role in neurodevelopment (Lauritzen et al. Citation2016). Currently, formula recommendations are to add between 0.2% and 0.5% DHA of total fatty acids, closely matched to milk from mothers consuming a Western diet (Koletzko et al. Citation2008). Since HMOS are one of the human milk components that are currently challenging to replicate in formula, it is a prime candidate to explain the difference between breast- and formula feeding. According to functional, structural elements, HMOS can be classified as fucosylated neutral, non-fucosylated neutral, and sialylated HMOS. Sialylated HMOS account for 14–33% of total HMOS in human milk (Ninonuevo et al. Citation2006; Soyyilmaz et al. Citation2021; Thurl et al. Citation2017) and sialic acid residues are attached to the core structures via α-2,3 and/or α-2,6 linkages. To date, about 30 sialylated HMOS have been detected, of which the dominant structures are 6′-Sialyllactose (6′-SL) and 3′-Sialyllactose (3′-SL) (Yan et al. Citation2018). The concentration of 6′-SL decreases with lactation, whereas 3′-SL remains stable (Austin et al. Citation2019; Ninonuevo et al. Citation2006; Samuel et al. Citation2020). Although mammals can synthesize sialic acid de novo, by a multi-step conversion of other monosaccharides, it has been suggested that this route provides insufficient sialic acid in infants to meet the high demand during the early, rapid development of the brain (B. Wang and Brand-Miller Citation2003; B. Wang et al. Citation2007c; Bode Citation2012). Therefore, sialylated HMOS may be considered a vital source of exogenous sialic acid needed for optimal brain growth and development during early life (Sprenger and Duncan Citation2012).
Sialic acid and the brain
Sialic acid (Sia) represents a family of acidic monosaccharides with a nine-carbon backbone, existing in a diversity of structures with more than 50 members identified to date (Kolter Citation2012). The most common sialic acid present in humans is Neu5Ac. The structure of Neu5Ac includes a strong carboxylic acid, hydrophilic groups, as well as a hydrophobic N-acetyl group (). Other Sia varieties include O-acetylations on different hydroxyls, deaminated nonulosonic acid (Kdn) and N-glycolyl-neuraminic acid (Neu5Gc) (). The latter occurs in the glycosylation of all known vertebrates, except for humans, where a species-wide point mutation in the CMP-N-acetylneuraminic acid hydroxylase (CMAH) occurs. The CMAH enzyme converts CMP-Neu5Ac into CMP-Neu5Gc, and also catalyzes the reverse reaction (Varki Citation2007). Dietary Neu5Gc can still be incorporated into human glycosylation and elevated levels have been implicated in auto-immune diseases and cancer, although causality has not yet been proven (Varki and Gagneux Citation2012).
Figure 2. (a) Major sialic acids structures: N-acetylneuraminic acid (Neu5Ac), N-glycolylneuraminic acid (Neu5Gc). The CMAH enzyme can convert CMP-Neu5Ac into CMP-Neu5Gc (B. Wang Citation2009). (b) Sialic acids on cell-surface and secreted molecules: glycosphingolipids, N-linked glycosphingolipids, polysialylated NCAM, free glycans. Adapted from Schnaar, Gerardy-Schahn, and Hildebrandt (Citation2014). Abbreviations: Galactose (Gal), glucose (Glc), fucose (Fuc), sialic acid (Sia), N-acetylgalacosamine (GalNAc), N-acetylglucosamine (GlcNAc), mannose (Man), asparagine (Asn), 3’-sialyllactose (3’SL), 6’-sialyllactose (6’SL), neural cell adhesion molecule (NCAM), fibronectin III (FnIII).
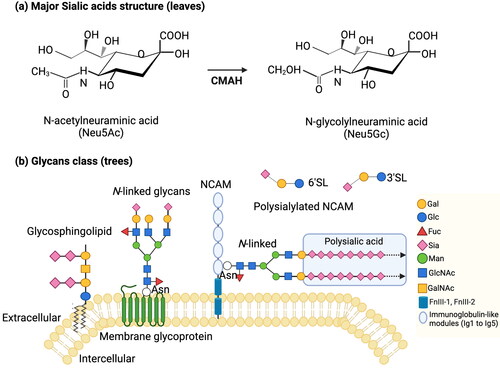
Sialic acid is present in all vertebrates and essentially exists in all tissues and can be synthesized de novo in the body. In mammalian bodies, Sia is rarely present as the free form but are widespread as Sia-glycoconjugates that are “tree-like” structures placed on (glyco)proteins and (glyco)lipids. depicts that the oligosaccharide chains functioning as the “trunk” and its “branches,” and the sialic residues at the end are the “leaves” of the tree (B. Wang and Brand-Miller Citation2003; B. Wang Citation2009; Cohen and Varki Citation2010). Many receptor interactions are mediated through sialic acid carrying glycosylation, due to accessibility of these “leaves,” e.g. siglecs (P. Crocker et al. Citation1998; P. R. Crocker and Varki Citation2001). It has been reported that Sia is a fundamental part of the central nervous system (CNS). The levels of sialic acid in the brain are higher than any other organ. Sialic acid is found on gangliosides (65%), glycoproteins (32%), and free Sia (3%) (Schnaar Citation2004). A study found that total Sia concentration in the human brain was 2–4 times higher than the other seven mammalian species, including chimpanzee (Pan troglodytes), rat (Rattus norvegicus), mouse (Mus musculus), rabbit (Oryctolagus cuniculus), sheep (Ovis aries), cow (Bos indicus) and pig (Sus scrofa) (B. Wang et al. Citation1998).
The monomers of Sia can be polymerized into polysialic acid (PSA). PSA is a linear, unique homopolymer consisting of more than 90 α-2,8 linked repeated Sia residues (). PSA is the main component of the neural cell adhesion molecule (NCAM) () (B. Wang Citation2012). NCAM is expressed on the surface of cells in the CNS, which plays a key role in synaptic formation, neuronal plasticity, and memory formation (B. Wang Citation2009; Colley, Kitajima, and Sato Citation2014; Hildebrandt et al. Citation2007; Regan and Fox Citation1995). Furthermore, polysialyltransferase II (ST8Sia II) and polysialyltransferase IV (ST8Sia IV) are the dominant enzymes to modulate functions of NCAM in a time-dependent and tissue-specific manner (B. Wang Citation2009). For example, in a mice model study, ST8Sia II was dominant in the embryonic and younger mice, whereas ST8Sia IV prevailed in the adult (Hildebrandt et al. Citation1998). Furthermore, ST8Sia IV deficient mice showed a decrease of PSA in most brain regions and impaired long-term potentiation (LTP), and long-term depression (LTD) in hippocampal CA1 synapses (Eckhardt et al. Citation2000). Different from ST8Sia IV deficient mice, ST8Sia II deficient mice resulted in the misguidance of infrapyramidal mossy fibers and the formation of ectopic synapses in the hippocampus (Angata et al. Citation2004). Notably, the polymorphisms of human genes responsible for the PSA biosynthesis are associated with psychiatric disorders (Schnaar, Gerardy-Schahn, and Hildebrandt Citation2014). It has been reported that the absence or severe reduction of sialic acid may lead to retardation and death (Schwarzkopf et al. Citation2002). In contrast, excessive sialylation with PSA in the myelin sheet leads to delamination and reduced functioning of the myelin sheet, which reduces neuronal isolation and sensitivity (Lazarov and Marr Citation2010; Massaro Citation2002; Wielgat and Braszko Citation2012). Therefore, there must be a delicate balance of PSA in the body.
Gangliosides are sialylated glycosphingolipids. Studies have shown that the protein-linked Sia in the brain and liver per gram of fresh weight are nearly identical, while the ganglioside-bound Sia in brain is 15 times higher than in the liver. Hence, ganglioside-bound Sia result in much higher total Sia concentrations in the brain than in any other organ (Alhadeff and Holzinger Citation1982; Tettamanti et al. Citation1973). Notably, the concentrations of sialic acid present in gangliosides vary dramatically with age. Whereas sialic acid seems to increase rapidly during early postnatal years, it appears to plateau until the age of approximately 50, before declining in later life. Furthermore, the structures of gangliosides are more complex in the brain than in the liver, there are currently more than 100 ganglioside structures identified in the brain (Schnaar Citation2004). Four related structures of gangliosides are dominated in adult mammalian brain including GM1, GD1a, GD1b and GT1b () (Tettamanti et al. Citation1973). Interestingly, HMOS and gangliosides contain the same monosaccharides and structural compositions, especially lacto and neolacto series (). Mouse genetic studies and human disorders of ganglioside metabolism have evidenced that gangliosides play a key role in nerve cell excitability modulation, axon-myelin interactions, axon stability and regeneration (B. Wang and Brand-Miller Citation2003; Schnaar, Gerardy-Schahn, and Hildebrandt Citation2014).
Figure 3. Carbohydrate core structures of glycosphingolipids, adapted from R. K. Yu et al. (Citation2011). Abbreviations: Galactose (Gal), glucose (Glc), N-acetylgalacosamine (GalNAc), N-acetylglucosamine (GlcNAc), mannose (Man).
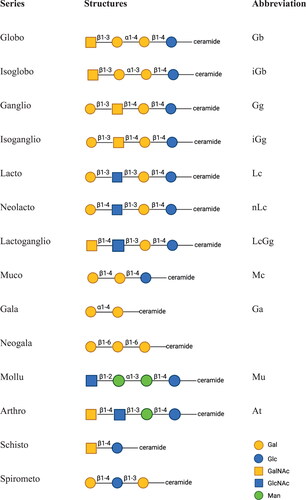
Sialic acid and pregnancy
Pregnancy increases the total sialic acid concentration in maternal serum and saliva (Alvi, Amer, and Sumerin Citation1988; B. Wang and Brand-Miller Citation2003; Chhabra et al. Citation2015; Koç Öztürk et al. Citation2010; Salvolini et al. Citation1998). Compared to thirty non-pregnant healthy women (the mean concentration of Sia is 1.191 ± 0.047 mmol/L), the concentration of serum total Sia is higher in thirty pregnant healthy women and significant elevation as the pregnancy advances (Chhabra et al. Citation2015). The mean concentrations of serum total Sia are 1.388 ± 0.046 mmol/L, 1.558 ± 0.051 mmol/L, 1.712 ± 0.067 mmol/L in first, second and third trimester of pregnancy respectively (Chhabra et al. Citation2015). In keeping with earlier study, Alvi, Amer, and Sumerin (Citation1988) measured serum total Sia concentration of 219 pregnant women (Alvi, Amer, and Sumerin Citation1988). Sia increases from 1.63 ± 0.3 mmol/L at five to eight weeks of gestation to 2.06 ± 0.49 mmol/L at term (Alvi, Amer, and Sumerin Citation1988). Salvolini et al. (Citation1998) performed a study to detect the concentration of total Sia in saliva (Salvolini et al. Citation1998). The study included forty-five healthy pregnant women and fifteen non-pregnant women (Salvolini et al. Citation1998). The concentration of salivary total Sia increases from 0.162 mmol/L at 10 weeks of gestation to around 0.485 mmol/L mg/l at 21 to 40 weeks (Salvolini et al. Citation1998). Subsequently, Koç Öztürk et al. (Citation2010) found similar results of salivary total Sia in the first and second trimester (Koç Öztürk et al. Citation2010). However, salivary total Sia decreases after second trimester (Koç Öztürk et al. Citation2010). The different results of two studies may due to the participants’ oral health during the late pregnancy (Koç Öztürk et al. Citation2010). Mothers synthesize more sialic acid during pregnancy. As shown in , the concentration of total Sia in serum and saliva increases as the pregnancy advances, corresponding to the period of fetal brain development ().
Recent studies have also shown that while non-pregnant women do not have HMOS in their bloodstream, pregnant women have incremental levels of HMOS in the blood serum from the first trimester (Jantscher-Krenn et al. Citation2019). The HMOS observed included sialylated species, i.e. 3′-sialyllactose (3’SL), 6′-sialyllactose (6’SL) and disialyllacto-N-tetraose (DSLNT) in low µM concentrations. Moreover, there has been evidence of transport of maternal serum HMOS across the placenta (Hirschmugl et al. Citation2019). We have not found any studies correlating maternal serum sialic acid levels with cognitive outcomes. Similarly, free sialic acids can cross the placenta (Briese et al. Citation1999) and may contribute to the fetal growth and brain development. The high concentration of Sia in human milk might provide a continuing need for infants postpartum.
Sialic acid in human milk versus infant formula and bovine milks
Sialic acids are recognized as important components of dairy products for infants and young children. Recently, human trials and animal experiments have indicated that 2′-fucosyllactose (2′-FL) and lacto-N-neotetraose (LNnT) are safe and well-tolerated, allowing the use of the two components in commercial infant formula (Hegar et al. Citation2019; Nowak-Wegrzyn et al. Citation2019; Vandenplas et al. Citation2018). However, the inclusion of sialic acid and sialylated HMOS in formula milk is limited due to challenges regarding the upscaling of production and stability (B. Wang et al. Citation2001a).
Compared with commercial formulas and bovine milk, human milk contains abundant and different forms of sialic acid. Human colostrum contains the highest concentrations of sialylated HMOS, which reach round 1500 mg/L (B. Wang et al. Citation2001a; Claumarchirant et al. Citation2016; Spichtig, Michaud, and Austin Citation2010), but decreases to around 200–300 mg/L at 10 weeks of lactation (B. Wang et al. Citation2001a; Claumarchirant et al. Citation2016; Spichtig, Michaud, and Austin Citation2010). The concentration of sialic acid is around 600 mg/L in bovine colostrum and 60–200 mg/L in mature milk (B. Wang et al. Citation2001a; Martín et al. Citation2001; Carlson Citation1985). However, the sialic acid levels reported for bovine milk in literature vary greatly, from 10 to 200 mg/L in mature milk (Martín et al. Citation2001; Spichtig, Michaud, and Austin Citation2010; Tang et al. Citation2008). Moreover, the sialic acid concentration reported for infant formula milk varies between 70–288 mg/L (B. Wang et al. Citation2001a; Claumarchirant et al. Citation2016), which is far less than early human milk. It should also be noted that the form of Sia is different between human milk and bovine milk. Sia in bovine milk is predominantly bound to glycoproteins, while 73% Sia in human milk is bound to free oligosaccharides (B. Wang et al. Citation2001a). Compared with oligosaccharides-bound Sia in human milk, the oligosaccharides-bound Sia is about 4.4 to 18 times lower in bovine milk and between 10 and 27 times lower in infant formulas (Martín-Sosa et al. Citation2003). In addition, bovine origin ingredients are usually employed in formula production containing both Neu5Gc and Neu5Ac, whereas human milk only contains Neu5Ac (B. Wang and Brand-Miller Citation2003; Claumarchirant et al. Citation2016).
As previously mentioned, although most vertebrates express significant amounts of Neu5Gc as well as Neu5Ac, humans only synthesize Neu5Ac. Antibodies against Neu5Gc can be used to detect the presence of Neu5Gc and showed evidence of Neu5Gc in some human cancer tissues and human fetal meconium (Hirabayashi et al. Citation1987). A study found that red meat (the richest source of Neu5Gc) is associated with diseases such as cancer and ischemic heart disease (Fraser Citation1999). Interestingly, Tangvoranuntakul et al. (Citation2003) performed an in vitro study which indicated that exogenous Neu5Gc could be absorbed by human cells and incorporated it into synthesized glycoproteins (Tangvoranuntakul et al. Citation2003). During the study, healthy human volunteers ingested Neu5Gc, and their saliva, hair, serum, and urine were collected to measure Sia and N-Acetylmannosamine levels (Tangvoranuntakul et al. Citation2003). The results showed that a significant portion was primarily excreted via the urine, while part potentially was incorporated into tissues—however, the absorption of Neu5Gc uptake into tissue was not further analyzed (Tangvoranuntakul et al. Citation2003). The study did, however, observe an increase in Neu5Gc in salivary glycoproteins, as well as in facial hair clippings of participants, compared to baseline levels prior to Neu5Gc ingestion.
Seppala et al. performed a mouse study, showing that incorporation of maternal dietary Neu5Gc into pups in utero did not show signs of increased Neu5Gc into brain tissue (Seppala, Lehto, and Gahl Citation1999). In addition, Neu5Gc is abundant in the liver, kidney, thymus, and spleen of mice, but is undetectable in the brain (Davies and Varki Citation2015; Schnaar, Gerardy-Schahn, and Hildebrandt Citation2014). To note, this study showed dietary stable isotope-labelled Neu5Ac was found in brain tissue, which is proof that dietary Neu5Ac can cross into the brain (Schnaar, Gerardy-Schahn, and Hildebrandt Citation2014). These studies suggest that dietary Neu5Gc cannot cross the mouse blood-brain barrier, while Neu5Ac can, or alternatively, that sialylation processes in the mouse brain selectively exclude Neu5Gc. It is known that formula milk contains of Neu5Gc; however, whether Neu5Gc enters brain glycosylation in formula-fed infants remains uncertain.
The potential role of sialic acid in brain development
Rodents studies
Rats and mice are extensively used in experimental models to study the role of sialic acid in brain development (Abdul Khalid et al. Citation2019; Docq et al. Citation2020; Oliveros et al. Citation2018; Sakai et al. Citation2006; Scholtz et al. Citation2013). Additionally, mounting evidence suggests that rat and mouse milk contains elevated 3′-SL and 6′-SL concentrations with approximately 80% of Sia bound to oligosaccharides such as sialyllactose, or sulfated sialyllactose (Li et al. Citation2021; Sprenger and Duncan Citation2012).
Several studies have shown that dietary supplementation with sialic acid results in increased sialic acid in the brain (Charbonneau et al. Citation2016; Sakai et al. Citation2006; Scholtz et al. Citation2013). Sakai et al. (Citation2006) performed a study supplementing the diet of rat pups with 1% Neu5Ac, 1% sialyllactose, or 1% galactosylated Neu5Ac, using 1% lactose as a control. The results showed a significant increase in GM3 ganglioside levels, indicating increased sialic acid levels in the brains of rats supplemented with sialyllactose or galactosylated Neu5Ac (Sakai et al. Citation2006). Another study showed that supplementation of the diet of rat pups with either cholesterol or sialic acid increased brain sialic acid levels independently (Scholtz et al. Citation2013). It should be noted that the intervention started at day 17 after delivery, while the rats were nursed for the first 17 days. The effect of maternal milk oligosaccharide levels could not be excluded in this study. Furthermore, the first two weeks are significant for brain ganglioside accumulation in rats (Vanier et al. Citation1971). Later, Charbonneau et al. (Citation2016) showed that supplementation of the diet with bovine milk oligosaccharides significantly increased brain sialic acid levels, compared with a control diet (Charbonneau et al. Citation2016). These studies clearly indicate that dietary supplementation of sialylated oligosaccharides in early life diet, increases levels of sialic acid in the brain.
There is also evidence that sialic acid supplementation results in cognitive ability (Abdul Khalid et al. Citation2019; Oliveros et al. Citation2018; Sakai et al. Citation2006). All three studies make use of T-maze or Y-maze tests to assess learning ability and long-term potentiation. Both Sakai et al. (Citation2006) and Abdul Khalid et al. (Citation2019) showed a significant increase in maze-performance when early diet is supplemented with sialic acid. Sakai et al. uses free sialic acid, sialyllactose and galactosylated Neu5Ac as sialic acid source, while Abdul Khalid et al. (Citation2019) uses edible bird nest (EBN) extract. EBN is a natural food product rich in sialic acid, due to the salivary mucins used by the birds to create the nest. During the study, the sialic acid was extracted from 14 raw unprocessed EBN samples.
Oliveros et al. (Citation2018) performed a study to assess the effects of sialic acid supplementation on memory and learning of pregnant rats and their pups from birth to 1 year old (Oliveros et al. Citation2018). During the study, Sprague-Dawley (SD) rats were administered with sialic acid in the form of 6′-SL incorporated into a standard chow diet for a year. Only male rats were selected for learning and memory tasks to assess LTP. Assessment of LTP was provided by the use of classical behavioral tests including the novel object recognition test, Y maze and IntelliCage protocol. The study’s findings suggested that LTP was elevated in rats that had previously been supplemented with 6′-SL (compared to control) by means of a two-way ANOVA (Oliveros et al. Citation2018). To note, only male rats were studied in this study. On the one side, same gender limits the confounders in the study; on the other side, it means that there is a gap in understanding for the female rats.
Beside studying cognitive ability and sialic acid levels, some studies have investigated brain structure and functioning in relation to dietary sialic acid (Abdul Khalid et al. Citation2019; Hauser et al. Citation2021). Abdul Khalid et al. (Citation2019) highlighted that sialic acid from Edible Bird Nest (EBN) increased the viability of pheochromocytoma and neuroblastoma cell lines (Abdul Khalid et al. Citation2019). The PC–12, SH–SY5Y, and SK–N–MC cell lines were treated with sialic acid extracts containing 20, 40, 60, 80, and 100 µg/ml sialic acid, and cell viability was determined using the MTT assay kit (Abdul Khalid et al. Citation2019). The results showed a relationship between increased sialic acid extract and increased neuron function associated with cognitive performance in mice (Abdul Khalid et al. Citation2019). This study suggested that sialic acid supplementation enhanced cognitive performance through improved neuronal development.
To identify the selective role and underlying mechanisms of sialylated HMOS in phenotypic programming, Hauser et al. (Citation2021) used a knock-out mouse model (B6.129-St6gal1tm2Jxm/J) lacking the gene responsible for the synthesis of 6′-SL to investigate the long-term consequences of a selective lactational deprivation of a specific sialylated HMO in mice (Hauser et al. Citation2021). It should be noted that the deleted gene is also responsible for sialylation of specific glycotopes on protein and ganglioside glycosylation. To correct for side-effects of this gene deletion in the pups, four experimental groups of male pups were formed, based on wild type (WT) and knockout (KO) mothers using both in- and cross-fostering strategy (WT-WT, WT-KO, KO-WT and KO-KO) with 37 mice per group (Hauser et al. Citation2021). The attention, perseverance, and memory were assessed in adult mice. The hippocampal electrophysiology, plasma metabolomics, and gut microbiota composition were investigated to detail the associated endophenotypes. The gene expression (at eye-opening and in adulthood) in two brain regions mediating executive functions and memory (hippocampus and prefrontal cortex, PFC) were analyzed to investigate the underlying molecular mechanisms (Hauser et al. Citation2021).
The results suggest that 6′-SL in maternal milk adjusts cognitive development through a short-term upregulation of genes modulating neuronal patterning in the PFC (Hauser et al. Citation2021). In addition, dietary supplements of Sia can modulate UDP-N-acetylglucosamine-2-epimerase/N-acetylmannosamine kinase (Gne) gene expression which is the key rate-limiting enzyme involved in the biosynthesis of sialic acid (B. Wang et al. Citation2007b). This study supports the hypothesis that sialylated HMOS in the nursing stage positively influences brain development, with further consequences observed in adulthood. Further clinical studies and efficacy data are desirable to understand the role of HMOS in human brain and cognitive development.
To conclude, the authors demonstrated the significance of increased exogenous sialic acid intake throughout development in murine models, suggesting that dietary sialic acid could markedly increase capacity for memory formation. Hence, results could indicate the potential involvement of sialic acid in pathologies resulting from disruption of memory processes, such as the occurrence of neurodegenerative disease. Many studies primarily focus on male pups; on the one hand there is a good reason for that, to limit the confounding experimental factors, since evidence suggests a marked difference in the male and female microbiome and brain developments (Jašarević, Morrison, and Bale Citation2016; Holingue et al. Citation2020). However, male-dominated experimental design and concerns regarding translatability of male-dominated research to female cohorts are problematic. Furthermore, concerns could be raised regarding the limited translatability of murine models to human brain development. For example, rats have significantly reduced gyri and sulci, indicating a reduction in complexity in their brain anatomical structure (Swanson Citation2018). Moreover, rodents use their tactile senses, and primarily olfaction as opposed to sight like humans (Arakawa and Iguchi Citation2018). In addition, the digestive system and intestinal microbiota differ between the rodents and humans (Nguyen et al. Citation2015). Thus, the differences in gut absorption and metabolism of sialic acids between murine models and human need to be considered. Therefore, the use of murine models may lead to unknown neurobiological mechanisms in memory, which may not necessarily be applicable to the process of human memory.
Piglets studies
The piglet is an appropriate model for studying human nutrition because the structural and physiological characteristics of piglets are closer to infants than murine models (B. Wang, Hu, and Yu Citation2006; Docq et al. Citation2020; Mudd and Dilger Citation2017; Odle et al. Citation2014). For example, the neonatal pig shares some similarities with the human infants with regard to nutrition and metabolism of lipids, the ontogenesis of neonatal carbohydrates, and the physiology and anatomical structure of digestive system. Notably, the oligosaccharide profiles in porcine milk appear more similar to human milk than bovine milk (Mudd et al. Citation2016). In porcine milk, the oligosaccharide profile did not contain Neu5Gc, suggesting that despite high Neu5Gc levels in porcine tissue and gut mucin (Banda et al. Citation2012), porcine milk more closely matches that of human milk than bovine milk in terms of the sialylated fraction (Mudd et al. Citation2016). Porcine milk contains approximately 16–29% sialylated oligosaccharides, and the concentration of free Sia significantly decreases, whereas total and bound-Sia increases during lactation. However, there are still limitations in the translatability exist in terms of morphological similarities. For example, most brain development in pigs occurs in the prenatal stage, whereas in humans, the brain undergoes rapid development in the neonatal years (Jacobi et al. Citation2016).
A study demonstrated dietary supplementation of sialic acid could modulate the gene expression of ST8Sia IV gene (ST8Sia IV encodes a key enzyme that is required for PSA synthesis) in the hippocampus of piglets (B. Wang, Hu, and Yu Citation2006). During the study, 6 three-day-old male piglets were fed with control diet or diet supplemented with sialic acid for 32 days. The amount of Sia was 140 mg/L in the control group with no added casein glycomacropeptide (cGMP, n = 3) and 830 mg/L in treatment group (n = 3). Tissues from brain frontal cortex, hippocampus and liver were collected in all piglets (B. Wang, Hu, and Yu Citation2006). The results showed that ST8Sia IV mRNA levels were increased around 4.5-fold in the hippocampus of piglets that underwent sialic acid supplementation, indicating that exogenous sialic acid is an essential nutrient for early brain development (B. Wang, Hu, and Yu Citation2006). However, the limitations of the study are the restricted sample size and only male piglets in this study; therefore, further study is required to validate the results with large cohorts.
Subsequently, B. Wang et al. (Citation2007c) performed another study to assess the effect of the sialic acid content of formula milk on early learning behaviors and gene expression of enzymes involved in sialic acid metabolism in piglets (B. Wang et al. Citation2007c). During the study, 54 three-day-old male domestic piglets were randomly allocated to 1 of 4 groups fed sow milk supplemented with sialic acid as cGMP for 35 days. Group 1 (n = 14) was the control group with no added cGMP (140 mg/L Sia); Group 2 (n = 13) was low dose group with 300 mg/L Sia; Group 3 (n = 14) was middle dose group with 635 mg/L Sia; Group 4 (n = 12) was the highest dose group with 830 mg/L Sia (B. Wang et al. Citation2007c). The experimental design consisted of learning performance and memory, which were assessed by easy and difficult visual cues in an 8-arm radial maze. Brain ganglioside and sialoprotein concentrations and mRNA expression of 2 learning-associated genes (ST8Sia IV and Gne) in the hippocampus, frontal cortex, and liver was measured (B. Wang et al. Citation2007c). The results showed supplementary sialic acid was associated with fewer trials needed for the acquisition of task with a dose-response correlation for the difficult task, higher concentrations of protein-bound sialic acid in the frontal cortex, and 2–3-fold higher mRNA levels of Gne and ST8Sia IV in a dose-dependent manner (B. Wang et al. Citation2007c). A previous study has shown that learning behavior itself could increase brain sialic acid incorporation (Savaki and Levis Citation1977). However, the present study cannot provide information whether dietary supplements of sialic acid increase neural tissue synthesis in the absence of learning activity.
Mudd, Fleming, et al. (Citation2017) performed a study using MRI technology for the first time to quantify the effect of dietary SL on the brain development of newborn piglets (Mudd, Fleming, et al. Citation2017). During the study, 38 two-day-old, male pigs were provided 1 of 4 diets containing SL for 30 days. Diets were formulated to contain: 0 mg SL/L, 130 mg SL/L, 380 mg SL/L, and 760 mg SL/L (Mudd, Fleming, et al. Citation2017). The concentration of sialic acids was measured in serum and tissue samples from the right hemisphere hippocampus, cerebellum and prefrontal cortex (Mudd, Fleming, et al. Citation2017). It should be noted that all pigs underwent structural MRI acquisition. Data indicated that pigs supplemented with SL showed an increased Sia concentration in the prefrontal cortex, and also influenced MRI measures in Corpus Callosum (Mudd, Fleming, et al. Citation2017). However, future work should seek to compare findings against a control diet lacking endogenous SL. Furthermore, dietary SL may influence the gut microbiota (A. L. Carlson et al. Citation2018). Recent evidence suggests that alterations in gut development may modulate brain development via gut-brain axis (Mudd, Berding, et al. Citation2017; Gao, Baumgartel, and Alexander Citation2020). Further studies would benefit by assessing the direct influence of dietary SL on brain development, possibly in a germ-free context.
Then, a study conducted by H. X. Wang et al. (Citation2021) used a Magnetic Resonance Spectroscopic (MRS) system to measure the effect of sialylated milk oligosaccharides on neurotransmitters and brain metabolites in neonatal piglets (H. X. Wang et al. Citation2021). The experiment consisted of three-day-old, male domestic piglets, which were randomly allocated to one of three groups and fed either sow milk (n = 15), supplemented with 9.5 g/kg 3′-SL and 6′-SL (SL, n = 16) or a combination of 9.5 g/kg SL and 6′-sialyl-lactosamine (SL/SLN, n = 15) for 35 days. Brain spectra were acquired using a 3 T MRS system, which provided more details on the influence of SL on neurodevelopment, gaining insights into cellular metabolites induced by nutrition intervention (H. X. Wang et al. Citation2021). The results showed that the concentrations of glutamate (Glu), myoinositol (mlns) and glutamate and glutamine pool (Glx) in the brain was significantly increased in SL/SLN group. Moreover, some brain metabolites [mlns, N-acetylaspartate (NAA), total NAA (TNAA), total choline (TCho), total creatine (TCr), scyllo-Inositol (SI) and glutathione (Glth)] positively correlated with the total white matter volume. Glu and SI were correlated with whole brain volume; and SI with whole brain weight, respectively. The authors concluded that sialylated milk oligosaccharide supplementation improves neuronal health and optimizes neurodevelopment in early postnatal life.
A study performed by Obelitz-Ryom et al. (Citation2019) showed supplementation with SL also improved cognition in a pre-term pig model (Obelitz-Ryom et al. Citation2019). During the study, a total of 46 (20 females and 26 males) preterm piglets were fed a diet based on raw bovine milk supplemented with either a bovine milk oligosaccharide-enriched whey with SL (n = 22) or lactose as control (n = 24) for 19 days. To note, control diet contains 120 ± 2 mg/L SL, while experimental diet contains 433 ± 5 mg/L SL (3′-SL: 6′-SL = 5:1) (Obelitz-Ryom et al. Citation2019). For reference, term piglets (9 females and 5 males) were delivered by cesarean section and fed the control diet. Another litter of 12 term piglets (6 females and 6 males) was born by vaginal delivery and received mother’s sow milk only (Obelitz-Ryom et al. Citation2019).
During the study, cognitive performance was tested in a spatial T-maze. Brains were collected for MRI, gene expression, and sialic acid measurements (Obelitz-Ryom et al. Citation2019). The results showed SL improved spatial cognition, with effects on hippocampal genes related to sialic acid metabolism, myelination, and ganglioside biosynthesis in preterm pigs (Obelitz-Ryom et al. Citation2019). Both sexes were included in data collection; however, no independent analysis based on gender was performed. Since gender was highlighted to have specific effects in rat models, which should be taken into consideration in piglet models (Shumake et al. Citation2014). Furthermore, findings do not provide information regarding long-term effects that persisted beyond the 19 days in this study. Therefore, further studies are required to establish the specific SL effects after administration as well as the optimal dose and timing of supplementation.
To investigate the role of SL dietary isomers, Jacobi et al. (Citation2016) supplemented piglets with human infant formula milk with both 3′-SL and 6′-SL and investigated if supplementation leads to the enrichment of sialic acid present in piglet brain tissue (Jacobi et al. Citation2016). The experimental design consisted of day-old piglets being randomly allocated into six diets (control, 2 or 4 g 3′-SL/L, 2 or 4 g 6′-SL/L, or 2 g polydextrose/L + 2 g galacto-oligosaccharides/L; n = 9) and fed three times/d for 21 days (Jacobi et al. Citation2016). The piglets were then exsanguinated, the brain tissue retrieved, and sialic acid concentration was determined in the cerebrum, cerebellum, corpus callosum, and hippocampal regions (Jacobi et al. Citation2016). The main results demonstrated that exogenous sialic acid intake has a dose-dependent relationship in the cerebellum and corpus callosum which are essential regions for cognitive aptitude (Jacobi et al. Citation2016). While the authors made essential insights into the effects of supplementation of formula milk with 3′-SL or 6′-SL in early brain development, future research could explore the long-term effects of dietary supplementation, such as memory consolidation. Furthermore, although porcine brains present favorable models to study human cognitive development, there are some limitations in translatability exist in terms of morphological similarities.
However, Fleming et al. (Citation2018) carried out a study presenting the opposite conclusion. The experimental design consisted of two-day-old piglets (n = 36) provided milk replacer with SL for 20 days (Fleming et al. Citation2018). The experimental diet was formulated using the control diet as the base and supplemented with bovine-derived modified whey enriched with SL to provide a final SL concentration of 190 mg SL/100 g milk replacer powder (Fleming et al. Citation2018). A novel object recognition task was used to assess piglets’ recognition memory. However, no evidence was found that supplementation with SL could alter recognition memory and daily performance in this study (Fleming et al. Citation2018). In this study, the contribution to the variation between study results may not have allowed sufficient time for SL to take effect. Furthermore, differences in piglet species used, task parameters, the dosage, and age of administration and testing differ dramatically in each study. The gender and sample size could also be a factor. Moreover, the researchers did not measure Sia levels in this study. In addition, the potential cognitive benefits from SL may have been masked by the inclusion of polydextrose (PDX) and galacto-oligosaccharides (GOS) in each diet.
Interestingly, a study that used 14C-labelled free sialic acid to detect its metabolic fate in piglets showed that an exogenous source of free sialic acid could cross the blood-brain barrier (BBB) and is absorbed by various tissues (B. Wang et al. Citation2007a). Moreover, mounting evidence suggests that mothers synthesize more Sia during pregnancy, which can cross the placenta to contribute toward fetal brain growth (B. Wang Citation2009; Ten Bruggencate et al. Citation2014). It would be interesting to compare the effect of Sia intake in a human study, since human infants are born with an earlier stage of brain development than piglets. An increasing part of the Sia-mediated brain development already takes place prenatally in piglets, while in humans, Sia is provided exogenously via milk. Another study indicated exogenous gangliosides could be taken up into the bloodstream and cross the BBB to be incorporated in neurons and enhance brain development (Schengrung and Mummert Citation1998). These findings further support the hypothesis that dietary sialic acid can end up in the brain and potentially be used in brain growth and development.
Clinical studies
Despite the crucial insights made during pre-clinical research, clinical studies provide more complete approaches to study the role of sialic acid in cognitive development. There are, to date, only few in vivo human studies investigating the link between breastfeeding, sialic acid levels, and brain development. B. Wang et al. carried out a study in 2001 to compare the saliva sialic acid level of preterm infants who were either fed human milk (n = 22) or were formula-fed (n = 14) (B. Wang et al. Citation2001b). The two study groups had similarity in gestational age, birth weight, and gender ratio. The saliva was collected at week 2, week 6, as well as at months 3, 4, and 5, and the total sialic acid concentration was analyzed. The saliva of preterm infants fed human milk contained twice the level of sialic acid compared to infants fed formula milk (B. Wang et al. Citation2001b). To conclude, breast milk seems to consistently supply more dietary sialic acid than formula milk does. This assumes that saliva could be a marker for sialic acid availability, with implications for the accretion of sialic acid in brain gangliosides. Moreover, a similar study was performed by Tram et al. (Citation1997), which found human milk-fed term infants had nearly 50% more sialic acid in saliva than formula-fed term infants (Tram et al. Citation1997). Both studies suggest that breastfeeding provides higher exposure to exogenous sialic acid.
As observed in animal models, feeding extra sialic acid in the infant stage, leads to higher levels of sialylated proteins and gangliosides in the brain. To study the influence of breastfeeding on brain development, B. Wang et al. (Citation2003) investigated the relationship between sufficient exogenous uptake of sialic acid in human breast milk with elevated brain ganglioside and glycoprotein sialic acid concentrations in brain tissue of human infants who died of sudden infant death syndrome (SIDS) (B. Wang et al. Citation2003). In this study, 25 frontal cortex samples were derived from SIDS infants, including 12 breastfed infants, 10 formula-fed infants, 1 mixed-fed infant (B. Wang et al. Citation2003). Ganglioside-bound and protein-bound sialic acid were determined by HPLC. Results showed the ganglioside Sia, and glycoprotein Sia were higher in breastfed infants compared to formula-fed infants (B. Wang et al. Citation2003). Findings indicate that not only are breastfed infants exposed to higher levels of sialic acid; there is also evidence that this sialic acid reaches the brain and influences brain development. These observations fit well with the observations in piglet studies, showing increased dietary Sia leads to higher Sia levels in parts of the brain (B. Wang et al. Citation2007c; Mudd, Fleming, et al. Citation2017; Jacobi et al. Citation2016). The number of participants is limited in this study. It is not clear whether there is a direct link to cognitive development, since that was not assessed in this study. It is also arguably unclear what caused SIDS and whether the SIDS influences (in some cases) the outcomes of the study associated with sialic acid in the brain.
Gut-brain axis
Human milk oligosaccharides not only play a direct role in neurocognitive development, but also can be considered as prebiotics to enhance the growth of beneficial bacteria and indirectly affect brain development via the gut-brain axis. The gut microbiome has been identified as a link in the gut-brain axis moderating cognitive development and health (Gao, Baumgartel, and Alexander Citation2020). A prospective study showed that infants with the Bacteroides-predominant enterotype had better cognitive performance in later life compared to other enterotypes (A. L. Carlson et al. Citation2018).
Tarr et al. (Citation2015) performed a study to investigate whether 3′-SL or 6′-SL would prevent stressor-induced alterations in gut microbial community composition and attenuate stressor-induced anxiety-like behavior in a mouse study (Tarr et al. Citation2015). During the study, male C57/BL6 mice were fed a control AIN-93G diet, or a diet supplemented with 3′-SL or 6′-SL for 2 weeks before stressor exposure with a continuation of diet under stressor exposure for one week (Tarr et al. Citation2015). Open field and light/dark preference apparatus were used to determine the anxiety-like behavior. Colonic microbiota was analyzed by Illumina MiSeq. The study showed that 3′-SL and 6′-SL impact microbial community structure in the absence of stress, and both have the capacity to support microbial community structure in the colon and influence normal behavior if fed prior to exposure to the stressor (Tarr et al. Citation2015). However, the results of the study have limited extrapolation to females. Moreover, the gut microbiota composition in mice is significantly different from human or infant microbiota, providing limited translatability. Further studies are needed to determine how changes in the gut microbiota are related to the number of immature neurons in the brain of stressor-exposed mice. The present study gives the possibility in the future that HMOS may prevent stressor-induced anxiety-like behavior mediated through the commensal microbiota.
In vitro studies have shown that supplementation with most fucosylated HMOS, 3′-SL, and 6′-SL promoted the growth of Bifidobacterium longum (Z. T. Yu, Chen, and Newburg Citation2013). B. longum has strong effects on the brain-gut axis (Bercik et al. Citation2011; Khoshdel et al. Citation2013; Tillisch et al. Citation2013; Zhou and Foster Citation2015). In a mice study, perfusion of an in-situ preparation of mouse myenteric plexus neurons with B. longum–fermented medium decreased the excitability of the enteric nervous system neurons (Bercik et al. Citation2011; Khoshdel et al. Citation2013). In human trials, healthy female volunteers were intervened with a mixture of probiotics for 4 weeks, including B. animalis subsp Lactis, Streptococcus thermophiles, Lactobacillus bulgaricus, and Lactococcus lactis subsp Lactis. The results showed that probiotic consumption influenced brain activity in the emotional centers in healthy individuals (Tillisch et al. Citation2013). Huiying Wang et al. (Citation2021) performed a randomized, double-blinded, placebo-controlled trial to detect the effects of B. longum 1714™ on neural responses to social stress (H. Wang et al. Citation2019). In this study, 40 healthy volunteers received either B. longum 1714™ or placebo for 4 weeks at a dose of 1 × 109 cfu/d. Social stress was induced by the “Cyberball game,” which is a standardized social stress paradigm. Brain activity was measured by magnetoencephalography, and health status was measured based on the 36-item short-form health survey (H. Wang et al. Citation2019). The results showed that neural oscillation was altered after the social stress in B. longum 1714™ group, with increased theta and alpha band power in the frontal and cingulate cortex and supramarginal gyrus (H. Wang et al. Citation2021). It may suggest that B. longum 1714™ is associated with the activation of brain coping centers to counter-regulate negative emotions. It is not known, however, how this effect is mediated and which microbial metabolites are responsible for the brain effects. It should be noted that the microbiota-gut-brain axis research is still in its infancy and increasing evidence is needed to understand the level and complexity of the interaction of microbial metabolites with brain activity and vice-versa.
The potential role of sialic acid in neurodegenerative disease
Sialic acids not only provide beneficial effects on CNS development, but recent studies also suggested that sialic acid-dependent events were intricately involved with the pathology of neurodegenerative diseases (B. Wang Citation2009; Magistretti et al. Citation2019). Alzheimer’s Disease (AD) is the most common neurodegenerative disease worldwide and remains a significant challenge in proper therapy.
Abnormal ganglioside metabolism is known to occur in neurodegenerative brain tissue. The role of gangliosides has been documented extensively since the 1940s; however, their potential neuroprotective mechanisms remain unclear. Studies show that profiles of ganglioside change in degenerative CNS diseases such as AD, Parkinson disease (PD), and Huntington’s disease (HD) (Magistretti et al. Citation2019). These alterations are seen progressively in GM1 (a downstream derivative of GM3) (), the dominant brain gangliosides that play a functional role in the nervous system that is markedly affected when the CNS is injured (Newburn et al. Citation2014; Rubovitch et al. Citation2017). For example, a study performed by Fukami et al. (Citation2017) indicated that altered ganglioside metabolism could associate with AD’s pathogenesis (Fukami et al. Citation2017). In this study, five AD patients (one male and four females) with mean age of 82 years old, and three control subjects (one male and two females) at the mean age of 63 years old were included. All of participants died at hospital and frontal poles were obtained from their brains to analyze gangliosides (Fukami et al. Citation2017). Results showed that the total sialic acid content, particularly in a-series gangliosides including GM1 and GD1a, were dramatically reduced on brain gangliosides in patients with Alzheimer’s disease compared with control subjects (Fukami et al. Citation2017). However, this case study only incorporated five participating AD patients and three healthy control subjects; therefore, solid conclusions regarding findings are somewhat limited.
Figure 4. (a) One of the brain gangliosides: monosialotetrahexosylganglioside (GM1), adapted from Schnaar, Gerardy-Schahn, and Hildebrandt (Citation2014). (b) The major brain gangliosides adapted from Schnaar, Gerardy-Schahn, and Hildebrandt (Citation2014). Abbreviations: Glucose (Glc), galactose (Gal), N-acetylgalacosamine (GalNAc), sialic acid (Sia), ceramide (Cer).
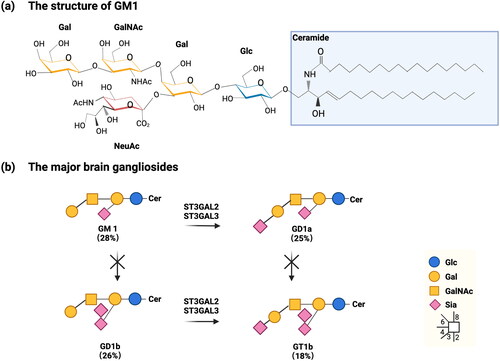
Although there is no current effective therapy for neurodegenerative disorders, GM1 showed therapeutic potential in 1994 and was administered as a therapeutic agent in an attempt to reduce neurological symptoms (Svennerholm Citation1994). In a double-blind, randomized study, patients with spinal cord injury were treated with 100 mg GM1 every day starting within 72 h post-injury. The results showed that patients who underwent treatment with GM1 showed enhanced neurologic recovery compared with the placebo group (Geisler, Dorsey, and Coleman Citation1991). Furthermore, Lenzi et al. (Citation1994) performed a randomized, double-blind, placebo-controlled trial including 792 patients (Lenzi et al. Citation1994). During the study, patients were intravenously injected with 200 mg GM1 or placebo within 5 hours after the onset of stroke, followed by a second intravenous dose of 100 mg 12 hours later; subsequently, patients received a daily intravenous dose of 100 mg from day 2 through day 10 and an intramuscular dose of 100 mg from day 11 through day 21. Participants were followed up for four months (Lenzi et al. Citation1994). The results showed that the dose of GM1 used is safe. Furthermore, patients treated with GM1 showed significant improvement in neurological status in contrast to placebo-treated patients (Lenzi et al. Citation1994).
Moreover, Huntington’s disease (HD) is a neurodegenerative disease with motor, cognitive and psychiatric disorder (Alpaugh et al. Citation2017). In a mouse experiment, HD mice under different genetic background (R6/2 mice, Q140 mice, YAC128 mice) were treated by intraventricular infusion of GM1 (Alpaugh et al. Citation2017). Authors provided evidence that GM1 administration improved motor functions, psychiatric-like and cognitive dysfunctions in HD mice (Alpaugh et al. Citation2017). Consequently, clinical studies may provide great insight into the potential role of sialic acid supplementation and brain homeostasis in later life.
Previous research has highlighted the relationship between increased CD33 mRNA expression in microglia and the onset of neurodegenerative disorders. The study showed that CD33 (Myeloid cell surface antigen; sialic acid-binding immunoglobulin-like lectin 3), present on the surface of microglial cells has been implicated as a risk factor for AD. Alzheimer’s disease is characterized by the increased self-assembly of amyloid-β (Aβ) toxic species, leading to progressive neurodegeneration (Griciuc et al. Citation2013). Consequently, polymorphisms have linked decreased CD33 expression with the late-onset Alzheimer’s disease, and interestingly with the increased uptake of toxic AD peptide Aβ into microglial cells via phagocytosis (Hooli and Tanzi Citation2016). Studies from Genome-Wide Association Studies (GWAS) have shown that AD phagocytosis is inhibited in microglial cells that overexpress CD33 in some human polymorphisms (Hooli and Tanzi Citation2016). Further studies showed that using a quantitative real-time PCR, performed on frozen cortical tissue from AD patients, revealed a 5-fold increase in CD33 levels in AD cases relative to controls (p < 0.01, student’s t-test) (Anderson, Johnstone, and Remley Citation1999). The reduced amount of sialylated structures in the brain in later life and during neurodegeneration may be explained by the decreased capacity of endogenous synthetic capacity of sialic acid (Sprenger and Duncan Citation2012). Therefore, increased dietary sialic acid uptake may provide a potential avenue for treatment prior to, or during neurodegenerative onset; however, the safety and effectiveness of dietary sialic acid supplementation should be further investigated before it can be applied (Sprenger and Duncan Citation2012).
Additionally, a study illustrated the neuroprotective role of a sialic acid mimetic known as P22 with its interaction with binding domain CD33, leading to the facilitated uptake of toxic AD peptide amyloid-β (Aβ) into microglial cells via phagocytosis, which is one of the routes of amyloid A peptide clearance (Miles et al. Citation2019). Findings were obtained by the collection of X-ray diffraction data from P22-CD33 complex crystals. Results illustrated the high affinity of P22 and CD33 interactions by the use of a flow cytometry assay (Miles et al. Citation2019). Consequently, the sialic acid-binding site present on the CD33 could serve as a potential receptor for target drugs to promote the clearance of toxic Aβ peptides and therefore attenuate the development of AD. Although the findings have shown a direct translatable link to sialic acid and decreased amyloid plaque formation, it remains unclear if P22 microparticles act as an agonist or as antagonists in their interaction with CD33 (Miles et al. Citation2019). Findings lack evidence implicating the possible effects of exogenous supplementation of sialic acid due to the ambiguous interactions with CD33 ligand. Furthermore, the increased activation of phagocytosis linked to P22 was not investigated in CD33-mediated microglial uptake; only the interaction between the domains of P22 and CD33 was investigated, limiting the study’s findings, as interaction with the host environment is not accounted for.
Yin et al. (Citation2015) performed an in vitro study showing that sialic acid-modified selenium nanoparticles conjugated with an alternative peptide-B6 (B6-SA-SeNPs, a synthetic selenoprotein analogue), has high permeability across the BBB and presents the potential to serve as a novel nanomedicine for disease modification in AD (Yin et al. Citation2015). The results showed B6-SA-SeNPs could not only effectively inhibit Aβ aggregation but could disaggregate preformed amyloid Aβ fibrils into nontoxic amorphous oligomers (Yin et al. Citation2015). The findings suggested that B6-SA-SeNPs could be a valuable tool for treating AD because of its potential anti-amyloid, anti-oxidant properties, and high brain delivery efficiency.
The disarrangement of PSA-NCAM has been associated with psychiatric disorders (Vawter Citation2000). Schizophrenia is a complex mental disorder that is clinically characterized by delusions, conceptual disorganization, hallucinations, cognitive dysfunction, and psychosocial impairments, which emerge during adolescence or early adulthood (Rapoport, Giedd, and Gogtay Citation2012). It has been reported that the schizophrenic phenotype depended on the polymorphisms of ST8SIA II in a human trial (Tao et al. Citation2007; Vawter Citation2000). In mouse experiments, the ST8Sia II-knockout mice developed a schizophrenia-like phenotype (Kröcher et al. Citation2015). It should be noted that the ST8Sia II gene encodes one of the sialyltransferase enzymes central to polysialic acid synthesis. According to the recent evidence, stimulation of synthesis of PSA may improve brain repair (Pekcec et al. Citation2007, Citation2008).
Furthermore, a study carried out by Demirci et al. (Citation2019) assessed whether Sia levels are significantly associated with the autism spectrum disorder (ASD) (Demirci et al. Citation2019). During the study, 46 ASD children and 30 typically developing children (3 to 10 years old) as a control were included in the study (Demirci et al. Citation2019). Behavioral symptoms were assessed by the Autism Behavior Checklist, the Childhood Autism Rating Scale, and the Aberrant Behavior Checklist. Saliva samples were collected for Sia analysis (Demirci et al. Citation2019). The results showed that Sia level was significantly lower in the ASD children compared to healthy controls. Sia levels might behave as a potential marker for autism-like behaviors, in particular, stereotypes and hyperactivity. However, there are some limitations to the current study. The researchers could not assess the oral intake levels/per day of Sia. Furthermore, an increased number of samples from children are required in a follow-up study. Additionally, future studies should take measures to control for dietary intake; or perform a double-blind placebo-controlled intervention with additional Sia in both groups to detect if dietary Sia is processed differently in ASD children compared with typically developing children.
It has been reported that increased lysosomal storage of free sialic acid leads to a spectrum of neurodegenerative disorders known as storage disease disorders (FSASDs) (Huizing et al. Citation2021). FSASD was divided into separate allelic disorders, including Salla disease, intermediate severe Salla disease, and infantile free sialic acid storage disease (ISSD) by the least functionally changed phenotype. Subsequent studies demonstrated that FSASDs have implicated sialic acid transport malfunction with neurological dysfunction and death (Wreden, Wlizla, and Reimer Citation2005). The effects of FSASDs range from severe, global development delays to mild cognitive impairment and spasticity (Barmherzig et al. Citation2017). The study highlighted the significance of functional sialic acid uptake in normal brain development and brain homeostasis in later life.
N-acetylneuraminic acid synthase (NANS) mediated synthesis of sialic acid is required for brain and skeletal development (Van Karnebeek et al. Citation2016). It is known that biallelic mutations in the NANS gene impair the endogenous synthesis of sialic acid, leading to the accumulation of the precursor, N-acetylmannosamine (ManNAc), and to a multisystemic disorder with intellectual disability (Tran et al. Citation2021). Tran et al. (Citation2021) performed a study to detect whether sialic acid supplementation might be a therapeutic avenue for NANS-deficient patients (Tran et al. Citation2021). During the study, four adults and two children with NANS deficiency and four healthy adults received oral Neu5Ac acid (150 mg/kg/d) over three days. Total Neu5Ac, free Neu5Ac, and ManNAc were analyzed in plasma and urine at different time points (Tran et al. Citation2021). The result showed that oral Neu5Ac was safe and well-tolerated. However, alterations in ManNAc levels were not seen in patients or controls. Orally administered free Neu5Ac was rapidly absorbed but also rapidly excreted in the urine, fitting previous results (Tangvoranuntakul et al. Citation2003). Although these results do not support the potential for oral free Neu5Ac in the treatment of NANS deficiency, it may highlight the importance of further studies investigating the effect in larger cohort sizes and more extended periods of observation to find potential therapy avenues.
Conclusion
To conclude, a plethora of studies highlight the links between breastfeeding and cognitive development. Although many confounding variables are not always controlled for, there is still a significant confidence support for the hypothesis that breastfeeding is beneficial for cognitive development. Additionally, preclinical studies investigating the role of exogenous sialic acid intake and sialic acid uptake in the brain showed significant data suggesting that dietary sialic acid reaches the brain. Furthermore, early-life exposure to sialic acid leads to elevated levels of sialic acid in the brain and alterations in gut microbiota, potentially affecting brain function via the gut-brain axis, although the latter field is still young and needs to develop further. Also, both murine and piglet models have shown that increased dietary intake of sialic acid, results in increased learning and memory ability.
There are multiple studies showing that in humans, sialic acid concentrations increase during pregnancy. Moreover, breastfeeding provides higher levels of sialic acid nutrition than formula feeding. Further systematic studies of sialic acid metabolism in infants would be beneficial, although there is a clear medical ethical limitation to in vivo human studies. Finally, there are links between neurodegenerative diseases and sialic acid levels in the brain. The mechanisms of sialic acid potential neuroprotective mechanisms are not yet fully understood, and there is limited evidence for sialic acid-based treatments for some neurodegenerative diseases; while for others, there is no clear evidence showing that exogenous sialic acid provides benefit in the present studies. However, there is still potential to investigate the effects of exogenous sialic acid for the prevention and treatment of brain-associated diseases.
Acknowledgements
Fan Liu, Anna Bella Simpson, Esmée D’Costa, and Fanny Sophia Bunn wrote the paper and conducted data collection; Sander S. van Leeuwen critically assessed and revised the manuscript.
Disclosure statement
The authors have declared no conflict of interest.
Additional information
Funding
References
- Abdul Khalid, S. K., A. A. Rashed, S. A. Aziz, and H. Ahmad. 2019. Effects of sialic acid from edible bird nest on cell viability associated with brain cognitive performance in mice. World Journal of Traditional Chinese Medicine 5 (4):214–9. doi: 10.4103/wjtcm.wjtcm_22_19.
- Alhadeff, J. A., and R. T. Holzinger. 1982. Sialyltransferase, sialic acid and sialoglycoconjugates in metastatic tumor and human liver tissue. International Journal of Biochemistry 14 (2):119–26. doi: 10.1016/0020-711X(82)90151-3.
- Alpaugh, M., D. Galleguillos, J. Forero, L. C. Morales, S. W. Lackey, P. Kar, A. D. Pardo, A. Holt, B. J. Kerr, K. G. Todd, et al. 2017. Disease-modifying effects of ganglioside GM1 in Huntington’s disease models. EMBO Molecular Medicine 9 (11):1537–57. doi: 10.15252/emmm.201707763.
- Alvi, M. u. H., N. A. Amer, and I. Sumerin. 1988. Serum 5-nucleotidase and serum sialic acid in pregnancy. Obstetrics and Gynecology 72 (2):171–4.
- Anderson, J. W., B. M. Johnstone, and D. T. Remley. 1999. Breast-feeding and cognitive development: A meta-analysis. The American Journal of Clinical Nutrition 70 (4):525–35. doi:10.1080/08035320310010400.
- Angata, K., J. M. Long, O. Bukalo, W. J. Lee, A. Dityatev, A. Wynshaw-Boris, M. Schachner, M. Fukuda, and J. D. Marth. 2004. Sialyltransferase ST8Sia-II assembles a subset of polysialic acid that directs hippocampal axonal targeting and promotes fear behavior. The Journal of Biological Chemistry 279 (31):32603–13. doi: 10.1074/jbc.M403429200.
- Arain, M., M. Haque, L. Johal, P. Mathur, W. Nel, A. Rais, R. Sandhu, and S. Sharma. 2013. Maturation of the adolescent brain. Neuropsychiatric Disease and Treatment 9:449–61. doi: 10.2147/NDT.S39776.
- Arakawa, H., and Y. Iguchi. 2018. Ethological and multi-behavioral analysis of learning and memory performance in laboratory rodent models. Neuroscience Research 135:1–12. doi: 10.1016/j.neures.2018.02.001.
- Austin, S., C. A. De Castro, N. Sprenger, A. Binia, M. Affolter, C. L. Garcia-Rodenas, L. Beauport, J. F. Tolsa, and C. J. F. Fumeaux. 2019. Human milk oligosaccharides in the milk of mothers delivering term versus preterm infants. Nutrients 11 (6):1282. doi: 10.3390/nu11061282.
- Ballard, O., and A. L. Morrow. 2013. Human milk composition: Nutrients and bioactive factors. Pediatric Clinics of North America 60 (1):49–74. doi: 10.1016/j.pcl.2012.10.002.
- Banda, K., C. J. Gregg, R. Chow, N. M. Varki, and A. Varki. 2012. Metabolism of vertebrate amino sugars with N-glycolyl groups: Mechanisms underlying gastrointestinal incorporation of the non-human sialic acid xeno-autoantigen N-glycolylneuraminic acid. The Journal of Biological Chemistry 287 (34):28852–64. doi: 10.1074/jbc.M112.364182.
- Barmherzig, R., G. Bullivant, D. Cordeiro, D. S. Sinasac, S. Blaser, and S. Mercimek-Mahmutoglu. 2017. A new patient with intermediate severe salla disease with hypomyelination: A literature review for salla disease. Pediatric Neurology 74:87–91.e2. doi: 10.1016/j.pediatrneurol.2017.05.022.
- Bercik, P., A. J. Park, D. Sinclair, A. Khoshdel, J. Lu, X. Huang, P. Blennerhassett, M. Fahnestock, D. Moine, B. Berger, et al. 2011. The anxiolytic effect of Bifidobacterium Longum NCC3001 involves vagal pathways for gut-brain communication. Neurogastroenterology and Motility 23 (12):1132–9. doi: 10.1111/j.1365-2982.2011.01796.x.
- Black, M. M. 2018. Impact of nutrition on growth, brain, and cognition. Nestle Nutrition Institute Workshop Series 89:185–95. doi: 10.1159/000486502.
- Bode, L. 2012. Human milk oligosaccharides: Every baby needs a sugar mama. Glycobiology 22 (9):1147–62. doi: 10.1093/glycob/cws074.
- Borewicz, K., F. J. Gu, E. Saccenti, C. Hechler, R. Beijers, C. de Weerth, S. S. van Leeuwen, H. A. Schols, and H. Smidt. 2020. The association between breastmilk oligosaccharides and faecal microbiota in healthy breastfed infants at two, six, and twelve weeks of age. Scientific Reports 10 (1):4270. doi: 10.1038/s41598-020-61024-z.
- Briese, V., S. Kunkel, C. Plath, K. Wutzke, and R. Plesse. 1999. Sialic acid, steroids and proteohormones in maternal, cord and retroplacental blood. Zeitschrift für Geburtshilfe und Neonatologie 203:63–8.
- Brown, T. T., and T. L. Jernigan. 2012. Brain development during the preschool years. Neuropsychology Review 22 (4):313–33. doi: 10.1007/s11065-012-9214-1.
- Cai, S., W. W. Pang, Y. L. Low, L. W. Sim, S. C. Sam, M. B. Bruntraeger, E. Q. Wong, D. Fok, B. F. Broekman, L. Singh, on behalf of the GUSTO Study Group, et al. 2015. Infant feeding effects on early neurocognitive development in Asian children. The American Journal of Clinical Nutrition 101 (2):326–36. doi: 10.3945/ajcn.114.095414.
- Carlson, S. E. 1985. N-Acetylneuraminic acid concentrations in human milk oligosaccharides and glycoproteins during lactation. The American Journal of Clinical Nutrition 41 (4):720–6. doi:10.1093/ajcn/41.4.720.
- Carlson, A. L., K. Xia, M. A. Azcarate-Peril, B. D. Goldman, M. Ahn, M. A. Styner, A. L. Thompson, X. J. Geng, J. H. Gilmore, and R. C. Knickmeyer. 2018. Infant gut microbiome associated with cognitive development. Biological Psychiatry 83 (2):148–59. doi: 10.1016/j.biopsych.2017.06.021.
- Charbonneau, M. R., D. O’Donnell, L. V. Blanton, S. M. Totten, J. C. C. Davis, M. J. Barratt, J. Cheng, J. Guruge, M. Talcott, J. R. Bain, et al. 2016. Sialylated milk oligosaccharides promote microbiota-dependent growth in models of infant undernutrition. Cell 164 (5):859–71. doi: 10.1016/j.cell.2016.01.024.
- Chen, X. 2015. Human Milk Oligosaccharides (HMOS): Structure, function, and enzyme-catalyzed synthesis. In Advances in carbohydrate chemistry and biochemistry, eds. D. C. Baker and D. Horton, 1st ed, Vol. 72. Cambridge, MA: Academic Press. doi: 10.1016/bs.accb.2015.08.002.
- Cheng, L. H., R. Akkerman, C. L. Kong, M. T. Walvoort, and P. de Vos. 2021. More than sugar in the milk: Human milk oligosaccharides as essential bioactive molecules in breast milk and current insight in beneficial effects. Critical Reviews in Food Science and Nutrition 61 (7):1184–200. doi: 10.1080/10408398.2020.1754756.
- Chhabra, R. J., S. Singh, K. Mangukiya, and J. Gorasiya. 2015. A study of circulating sialic acid levels in pregnancy. The Journal of Medical Research 1 (4):126–8. doi: 10.31254/jmr.2015.1407.
- Claumarchirant, L., L. M. Sanchez-Siles, E. Matencio, A. Alegría, and M. J. Lagarda. 2016. Evaluation of sialic acid in infant feeding: Contents and bioavailability. Journal of Agricultural and Food Chemistry 64 (44):8333–42. doi: 10.1021/acs.jafc.6b03273.
- Cohen, M., and A. Varki. 2010. The sialome-far more than the sum of its parts. Omics 14 (4):455–64. doi: 10.1089/omi.2009.0148.
- Colley, K. J., K. Kitajima, and C. Sato. 2014. Polysialic acid: Biosynthesis, novel functions and applications. Critical Reviews in Biochemistry and Molecular Biology 49 (6):498–532. doi: 10.3109/10409238.2014.976606.
- Courchesne, E., T. Pramparo, V. H. Gazestani, M. V. Lombardo, K. Pierce, and N. E. Lewis. 2019. The ASD living biology: From cell proliferation to clinical phenotype. Molecular Psychiatry 24 (1):88–107. doi: 10.1038/s41380-018-0056-y.
- Crocker, P. R., E. A. Clark, M. Filbin, S. Gordon, Y. Jones, J. H. Kehrl, S. Kelm, N. Le Douarin, L. Powell, J. Roder, et al. 1998. Siglecs: A family of sialic-acid binding lectins. Glycobiology 8 (2):v–vi. doi: 10.1093/glycob/8.1.0.
- Crocker, P. R., and A. Varki. 2001. Siglecs in the immune system. Immunology 103 (2):137–45. doi: 10.1046/j.0019-2805.2001.01241.x.
- D’Souza, D., D. Brady, J. X. Haensel, and H. D’Souza. 2020. Is mere exposure enough? The effects of bilingual environments on infant cognitive development. Royal Society Open Science 7 (2):180191. doi: 10.1098/rsos.180191.
- Davies, L. R. L., and A. Varki. 2015. Why is N-glycolylneuraminic acid rare in the vertebrate brain? Topics in Current Chemistry 366:31–54. doi: 10.1007/128_2013_419.
- Demirci, E., Y. Guler, S. Ozmen, M. Canpolat, and S. Kumandas. 2019. Levels of salivary sialic acid in children with autism spectrum disorder; could it be related to stereotypes and hyperactivity? Clinical Psychopharmacology and Neuroscience 17 (3):415–22. doi: 10.9758/cpn.2019.17.3.415.
- Deoni, S. C. 2018. Neuroimaging of the developing brain and impact of nutrition. Nestle Nutrition Institute Workshop Series 89:155–74. doi: 10.1159/000486500.
- Docq, S., M. Spoelder, W. Wang, and J. R. Homberg. 2020. The protective and long-lasting effects of human milk oligosaccharides on cognition in mammals. Nutrients 12 (11):3572. doi: 10.3390/nu12113572.
- Eckhardt, M., O. Bukalo, G. Chazal, L. H. Wang, C. Goridis, M. Schachner, R. Gerardy-Schahn, H. Cremer, and A. Dityatev. 2000. Mice deficient in the polysialyltransferase ST8SiaIV/PST-1 allow discrimination of the roles of neural cell adhesion molecule protein and polysialic acid in neural development and synaptic plasticity. The Journal of Neuroscience 20 (14):5234–44. doi: 10.1523/JNEUROSCI.20-14-05234.2000.
- Eidelman, A., R. J. Schanler, M. Johnston, S. Landers, L. Noble, K. Szucs, and L. Viehmann, Section on Breastfeeding. 2012. Breastfeeding and the use of human milk. Pediatrics 129 (3):e827–e841. doi: 10.1542/peds.2011-3552.
- Fleming, S. A., M. Chichlowski, B. M. Berg, S. M. Donovan, and R. N. Dilger. 2018. Dietary sialyllactose does not influence measures of recognition memory or diurnal activity in the young pig. Nutrients 10 (4):395. doi: 10.3390/nu10040395.
- Fraser, G. E. 1999. Associations between diet and cancer, ischemic heart disease, and all-cause mortality in non-Hispanic White California Seventh-day Adventists. The American Journal of Clinical Nutrition 70 (3):532S–8S. doi: 10.1093/ajcn/70.3.532s.
- Fukami, Y., T. Ariga, M. Yamada, and N. Yuki. 2017. Brain gangliosides in Alzheimer’s disease: Increased expression of cholinergic neuron-specific gangliosides. Current Alzheimer Research 14 (6):586–91. doi: 10.2174/1567205014666170117094038.
- Fusco, S., M. Spinelli, S. Cocco, C. Ripoli, A. Mastrodonato, F. Natale, M. Rinaudo, G. Livrizzi, and C. Grassi. 2019. Maternal insulin resistance multigenerationally impairs synaptic plasticity and memory via gametic mechanisms. Nature Communications 10 (1):4799. doi: 10.1038/s41467-019-12793-3.
- Gao, W., K. L. Baumgartel, and S. A. Alexander. 2020. The gut microbiome as a component of the gut-brain axis in cognitive health. Biological Research for Nursing 22 (4):485–94. doi: 10.1177/1099800420941923.
- Gao, W., K. Grewen, R. C. Knickmeyer, A. Q. Qiu, A. Salzwedel, W. L. Lin, and J. H. Gilmore. 2019. A review on neuroimaging studies of genetic and environmental influences on early brain development. NeuroImage 185:802–12. doi: 10.1016/j.neuroimage.2018.04.032.
- Geisler, F. H., F. C. Dorsey, and W. P. Coleman. 1991. Recovery of motor function after spinal-cord injury-a randomized, placebo-controlled trial with GM-1 ganglioside. The New England Journal of Medicine 324 (26):1829–38. doi: 10.1056/NEJM199106273242601.
- Griciuc, A., A. Serrano-Pozo, A. R. Parrado, A. N. Lesinski, C. N. Asselin, K. Mullin, B. Hooli, S. H. Choi, B. T. Hyman, and R. E. Tanzi. 2013. Alzheimer’s disease risk gene CD33 inhibits microglial uptake of amyloid beta. Neuron 78 (4):631–43. doi: 10.1016/j.neuron.2013.04.014.
- Hauser, J., E. Pisa, A. Arias Vásquez, F. Tomasi, A. Traversa, V. Chiodi, F.-P. Martin, N. Sprenger, O. Lukjancenko, A. Zollinger, et al. 2021. Sialylated human milk oligosaccharides program cognitive development through a non-genomic transmission mode. Molecular Psychiatry 26 (7):2854–71. doi: 10.1038/s41380-021-01054-9.
- Hegar, B., Y. Wibowo, R. W. Basrowi, R. G. Ranuh, S. M. Sudarmo, Z. Munasir, A. F. Atthiyah, A D. Widodo, M. K. Supriatmo, et al. 2019. The role of two human milk oligosaccharides, 2’-fucosyllactose and lacto-N-neotetraose, in infant nutrition. Pediatric Gastroenterology Hepatology and Nutrition 22 (4):330–40. doi: 10.5223/pghn.2019.22.4.330.
- Heird, W. C. 2001. The role of polyunsaturated fatty acids in term and preterm infants and breastfeeding mothers. Pediatric Clinics of North America 48 (1):173–88. doi: 10.1016/S0031-3955(05)70292-3.
- Hildebrandt, H., C. Becker, M. Mürau, R. Gerardy-Schahn, and H. Rahmann. 1998. Heterogeneous expression of the polysialyltransferases ST8Sia II and ST8Sia IV during postnatal rat brain development. Journal of Neurochemistry 71 (6):2339–48. doi: 10.1046/j.1471-4159.1998.71062339.x.
- Hildebrandt, H., M. Mühlenhoff, B. Weinhold, and R. Gerardy-Schahn. 2007. Dissecting polysialic acid and NCAM functions in brain development. Journal of Neurochemistry 103 (s1):56–64. doi: 10.1111/j.1471-4159.2007.04716.x.
- Hirabayashi, Y., H. Kasakura, M. Matsumoto, H. Higashi, S. Kato, N. Kasai, and M. Naiki. 1987. Specific expression of unusual GM2 ganglioside with hanganutziu-deicher antigen activity on human colon cancers. Japanese Journal of Cancer Research: Gann 78 (3):251–60. doi: 10.20772/cancersci1985.78.3_251.
- Hirschmugl, B., W. Brandl, B. Csapo, M. van Poppel, H. Köfeler, G. Desoye, C. Wadsack, and E. Jantscher-Krenn. 2019. Evidence of human milk oligosaccharides in cord blood and maternal-to-fetal transport across the placenta. Nutrients 11 (11):2640. doi: 10.3390/nu11112640.
- Holingue, C., A. C. Budavari, K. M. Rodriguez, C. R. Zisman, G. Windheim, and M. D. Fallin. 2020. Sex differences in the gut-brain axis: Implications for mental health. Current Psychiatry Reports 22 (12):83. doi: 10.1007/s11920-020-01202-y.
- Hooli, B., and R. E. Tanzi. 2016. The genetic basis of Alzheimer’s disease: Findings from genome-wide studies. Genomics, Circuits, and Pathways in Clinical Neuropsychiatry 34:547–71. doi: 10.1016/B978-0-12-800105-9.00034-2.
- Horta, B. L., C. L. De Mola, and C. G. Victora. 2015. Breastfeeding and intelligence: A systematic review and meta-analysis. Acta Paediatrica (Oslo, Norway: 1992) 104 (467):14–9. doi: 10.1111/apa.13139.
- Huizing, M., M. E. Hackbarth, D. R. Adams, M. Wasserstein, M. C. Patterson, S. U. Walkley, and W. A. Gahl, FSASD Consortium. 2021. Free sialic acid storage disorder: Progress and promise. Neuroscience Letters 755:135896. doi: 10.1016/j.neulet.2021.135896.
- Isaacs, E. B., B. R. Fischl, B. T. Quinn, W. K. Chong, D. G. Gadian, and A. Lucas. 2010. Impact of breast milk on intelligence quotient, brain size, and white matter development. Pediatric Research 67 (4):357–62. doi: 10.1203/PDR.0b013e3181d026da.
- Jacobi, S. K., T. Yatsunenko, D. P. Li, S. Dasgupta, R. K. Yu, B. M. Berg, M. Chichlowski, and J. Odle. 2016. Dietary isomers of sialyllactose increase ganglioside sialic acid concentrations in the corpus callosum and cerebellum and modulate the colonic microbiota of formula-fed piglets. The Journal of Nutrition 146 (2):200–8. doi: 10.3945/jn.115.220152.
- Jantscher-Krenn, E., J. Aigner, B. Reiter, H. Köfeler, B. Csapo, G. Desoye, L. Bode, and M. N. M. van Poppel. 2019. Evidence of human milk oligosaccharides in maternal circulation already during pregnancy: A pilot study. American Journal of Physiology. Endocrinology and Metabolism 316 (3):E347–E357. doi: 10.1152/ajpendo.00320.2018.
- Jašarević, E., K. E. Morrison, and T. L. Bale. 2016. Sex differences in the Gut microbiome-brain axis across the lifespan. Philosophical Transactions of the Royal Society of London, Series B, Biological Sciences 371 (1688):20150122. doi: 10.1098/rstb.2015.0122.
- Karim, M., and B. Wang. 2006. Is sialic acid in milk food for the brain? CAB Reviews: Perspectives in Agriculture, Veterinary Science, Nutrition and Natural Resources 1 (018):1–11. doi: 10.1079/PAVSNNR20061018.
- Khoshdel, A., E. F. Verdu, W. Kunze, P. Mclean, G. Bergonzelli, and J. D. Huizinga. 2013. Bifidobacterium Longum NCC3001 inhibits AH neuron excitability. Neurogastroenterology and Motility 25 (7):e478–e484. doi: 10.1111/nmo.12147.
- Koç Öztürk, L., S. Akyüz, A. Yarat, S. Koç, N. Gül, and B. N. Doğan. 2010. Salivary lipid peroxidation and total sialic acid levels during healthy gestation and postpartum: A longitudinal study. Clinical Biochemistry 43 (4–5):430–4. doi: 10.1016/j.clinbiochem.2009.10.053.
- Koletzko, B., E. Lien, C. Agostoni, H. Böhles, C. Campoy, I. Cetin, T. Decsi, W. Dudenhausen, C. Dupont, S. Forsyth, World Association of Perinatal Medicine Dietary Guidelines Working Group, et al. 2008. The roles of long-chain polyunsaturated fatty acids in pregnancy, lactation and infancy: Review of current knowledge and consensus recommendations. Journal of Perinatal Medicine 36 (1):5–14. doi: 10.1515/JPM.2008.001.
- Kolter, T. 2012. Ganglioside biochemistry. ISRN Biochemistry 2012:506160–36. doi: 10.5402/2012/506160.
- Kramer, M. S., F. Aboud, E. Mironova, I. Vanilovich, R. W. Platt, L. Matush, S. Igumnov, E. Fombonne, N. Bogdanovich, T. Ducruet, et al. 2008. Breastfeeding and child cognitive development. Archives of General Psychiatry 65 (5):578–84. doi: 10.1111/j.1365-2214.2009.01070.x.
- Kröcher, T., K. Malinovskaja, M. Jürgenson, A. Aonurm-Helm, T. Zharkovskaya, A. Kalda, I. Röckle, M. Schiff, B. Weinhold, R. Gerardy-Schahn, et al. 2015. Schizophrenia-like phenotype of polysialyltransferase ST8SIA2-deficient mice. Brain Structure & Function 220 (1):71–83. doi: 10.1007/s00429-013-0638-z.
- Kunz, C., S. Rudloff, W. Baier, N. Klein, and S. Strobel. 2000. Oligosaccharides in human milk: Structural, functional, and metabolic aspects. Annual Review of Nutrition 20:699–722. doi: 10.1146/annurev.nutr.20.1.699.
- Lauritzen, L., P. Brambilla, A. Mazzocchi, L. B. Harsløf, V. Ciappolino, and C. Agostoni. 2016. DHA effects in brain development and function. Nutrients 8 (1):6. doi: 10.3390/nu8010006.
- Lazarov, O., and R. A. Marr. 2010. Neurogenesis and Alzheimer’s disease: At the crossroads. Experimental Neurology 223 (2):267–81. doi: 10.1016/j.expneurol.2009.08.009.
- Lenzi, G. L., F. Grigoletto, M. Gent, R. S. Roberts, M. D. Walker, J. D. Easton, A. Carolei, C. Dorsey, W. A. Rocca, and R. Bruno. 1994. Early treatment of stroke with monosialoganglioside GM-1. Efficacy and safety results of the Early Stroke Trial. Stroke 25 (8):1552–8. doi: 10.1161/01.STR.25.8.1552.
- León-Cava, C. Lutter, J. Ross, and L. Martin. 2002. Quantifying the benefits of breastfeeding: A summary of the evidence. Washington, DC: PAHO.
- Li, J. Q., M. R. Jiang, J. R. Zhou, J. J. Ding, Z. M. Guo, M. Li, F. Ding, W. G. Chai, J. Y. Yan, and X. M. Liang. 2021. Characterization of rat and mouse acidic milk oligosaccharides based on hydrophilic interaction chromatography coupled with electrospray tandem mass spectrometry. Carbohydrate Polymers 259:117734. doi: 10.1016/j.carbpol.2021.117734.
- Liu, F., J. Y. Yan, X. F. Wang, C. Y. Wang, L. L. Chen, Y. R. Li, J. L. Chen, and H. Y. Guo. 2021. Maternal fucosyltransferase 2 status associates with the profiles of human milk oligosaccharides and the fecal microbiota composition of breastfed infants. Journal of Agricultural and Food Chemistry 69 (10):3032–43. doi: 10.1021/acs.jafc.0c04575.
- Magistretti, P. J., F. H. Geisler, J. S. Schneider, P. A. Li, H. Fiumelli, and S. Sipione. 2019. Gangliosides: Treatment avenues in neurodegenerative disease. Frontiers in Neurology 10:859. doi: 10.3389/fneur.2019.00859.
- Mamun, A. A., M. J. O’Callaghan, G. M. Williams, J. M. Najman, L. Callaway, and H. D. McIntyre. 2015. Breastfeeding is protective to diabetes risk in young adults: A longitudinal study. Acta Diabetologica 52 (5):837–44. doi: 10.1007/s00592-014-0690-z.
- Martín, M. J., S. Martín-Sosa, L. A. García-Pardo, and P. Hueso. 2001. Distribution of bovine milk sialoglycoconjugates during lactation. Journal of Dairy Science 84 (5):995–1000. doi: 10.3168/jds.S0022-0302(01)74558-4.
- Martín-Sosa, S., M. J. Martín, L. A. García-Pardo, and P. Hueso. 2003. Sialyloligosaccharides in human and bovine milk and in infant formulas: Variations with the progression of lactation. Journal of Dairy Science 86 (1):52–9. doi: 10.3168/jds.S0022-0302(03)73583-8.
- Massaro, A. R. 2002. The role of NCAM in remyelination. Neurological Sciences 22 (6):429–35. doi: 10.1007/s100720200001.
- Matheson, M. C., K. J. Allen, and M. L. Tang. 2012. Understanding the evidence for and against the role of breastfeeding in allergy prevention. Clinical and Experimental Allergy 42 (6):827–51. doi: 10.1111/j.1365-2222.2011.03925.x.
- Menassa, D. A., and D. Gomez-Nicola. 2018. Microglial dynamics during human brain development. Frontiers in Immunology 9:1014. doi: 10.3389/fimmu.2018.01014.
- Miles, L. A., S. J. Hermans, G. A. Crespi, J. H. Gooi, L. Doughty, T. L. Nero, J. Markulić, A. Ebneth, B. Wroblowski, D. Oehlrich, et al. 2019. Small molecule binding to Alzheimer risk factor CD33 promotes Aβ phagocytosis. iScience 19:110–8. doi: 10.1016/j.isci.2019.07.023.
- Moossavi, S., F. Atakora, K. Miliku, S. Sepehri, B. Robertson, Q. L. Duan, A. B. Becker, J. Mandhane, S. E. Turvey, T. J. Moraes, et al. 2019. Integrated analysis of human milk microbiota with oligosaccharides and fatty acids in the child cohort. Frontiers in Nutrition 6:58. doi: 10.3389/fnut.2019.00058.
- Mudd, A. T., K. Berding, M. Wang, S. M. Donovan, and R. N. Dilger. 2017. Serum cortisol mediates the relationship between fecal Ruminococcus and brain N-acetylaspartate in the young pig. Gut Microbes 8 (6):589–600. doi: 10.1080/19490976.2017.1353849.
- Mudd, A. T., S. A. Fleming, B. Labhart, M. Chichlowski, B. M. Berg, S. M. Donovan, and R. N. Dilger. 2017. Dietary sialyllactose influences sialic acid concentrations in the prefrontal cortex and magnetic resonance imaging measures in corpus callosum of young pigs. Nutrients 9 (12):1297. doi: 10.3390/nu9121297.
- Mudd, A. T., J. Salcedo, L. S. Alexander, S. K. Johnson, C. M. Getty, M. Chichlowski, B. M. Berg, D. Barile, and R. N. Dilger. 2016. Porcine milk oligosaccharides and sialic acid concentrations vary throughout lactation. Frontiers in Nutrition 3:39. doi: 10.3389/fnut.2016.00039.
- Mudd, A. T., and R. N. Dilger. 2017. Early-life nutrition and neurodevelopment: Use of the piglet as a translational model. Advances in Nutrition (Bethesda, Md.) 8 (1):92–104. doi: 10.3945/an.116.013243.
- Newburn, E. N., A. Duchemin, N. H. Neff, and M. Hadjiconstantinou. 2014. GM1 ganglioside enhances ret signaling in striatum. Journal of Neurochemistry 130 (4):541–54. doi: 10.1111/jnc.12760.
- Nguyen, T. L. A., S. Vieira-Silva, A. Liston, and J. Raes. 2015. How informative is the mouse for human gut microbiota research? DMM. Disease Models & Mechanisms 8 (1):1–16. doi: 10.1242/dmm.017400.
- Ninonuevo, M. R., Y. Park, H. F. Yin, J. H. Zhang, R. E. Ward, B. H. Clowers, J. B. German, L. Freeman, K. Killeen, R. Grimm, et al. 2006. A strategy for annotating the human milk glycome. Journal of Agricultural and Food Chemistry 54 (20):7471–80. doi: 10.1021/jf0615810.
- Nowak-Wegrzyn, A., L. Czerkies, K. Reyes, B. Collins, and R. G. Heine. 2019. Confirmed hypoallergenicity of a novel whey-based extensively hydrolyzed infant formula containing two human milk oligosaccharides. Nutrients 11 (7):1447. doi: 10.3390/nu11071447.
- Obelitz-Ryom, K., S. B. Bering, S. H. Overgaard, S. F. Eskildsen, S. Ringgaard, J. L. Olesen, K. Skovgaard, S. Pankratova, B. Wang, A. Brunse, et al. 2019. Bovine milk oligosaccharides with sialyllactose improves cognition in preterm pigs. Nutrients 11 (6):1335. doi: 10.3390/nu11061335.
- Odle, J., X. Lin, S. K. Jacobi, S. W. Kim, and C. H. Stahl. 2014. The suckling piglet as an agrimedical model for the study of pediatric nutrition and metabolism. Annual Review of Animal Biosciences 2 (1):419–44. doi: 10.1146/annurev-animal-022513-114158.
- Oliveros, E., E. Vázquez, A. Barranco, M. Ramírez, A. Gruart, J. M. Delgado-García, R. Buck, R. Rueda, and M. J. Martín. 2018. Sialic acid and sialylated oligosaccharide supplementation during lactation improves learning and memory in rats. Nutrients 10 (10):1519. doi: 10.3390/nu10101519.
- Pang, W. W., P. T. Tan, S. Cai, D. Fok, M. C. Chua, S. B. Lim, L. P. Shek, Y. Chan, K. H. Tan, F. Yap, et al. 2020. Nutrients or nursing? Understanding how breast milk feeding affects child cognition. European Journal of Nutrition 59 (2):609–19. doi: 10.1007/s00394-019-01929-2.
- Pekcec, A., M. Mühlenhoff, R. Gerardy-Schahn, and H. Potschka. 2007. Impact of the PSA-NCAM system on pathophysiology in a chronic rodent model of temporal lobe epilepsy. Neurobiology of Disease 27 (1):54–66. doi: 10.1016/j.nbd.2007.04.002.
- Pekcec, A., C. Fuest, M. Mühlenhoff, R. Gerardy-Schahn, and H. Potschka. 2008. Targeting epileptogenesis-associated induction of neurogenesis by enzymatic depolysialylation of NCAM counteracts spatial learning dysfunction but fails to impact epilepsy development. Journal of Neurochemistry 105 (2):389–400. doi: 10.1111/j.1471-4159.2007.05172.x.
- Rapoport, J. L., J. N. Giedd, and N. Gogtay. 2012. Neurodevelopmental model of schizophrenia: Update 2012. Molecular Psychiatry 17 (12):1228–38. doi: 10.1038/mp.2012.23.
- Regan, C. M., and G. B. Fox. 1995. Polysialylation as a regulator of neural plasticity in rodent learning and aging. Neurochemical Research 20 (5):593–8. doi: 10.1007/BF01694541.
- Rubovitch, V., Y. Zilberstein, J. Chapman, S. Schreiber, and C. G. Pick. 2017. Restoring GM1 ganglioside expression ameliorates axonal outgrowth inhibition and cognitive impairments induced by blast traumatic brain injury. Scientific Reports 7:41269. doi: 10.1038/srep41269.
- Sakai, F., Y. Ikeuchi, T. Urashima, M. Fujihara, K. Ohtsuki, and S. Yanahira. 2006. Effects of feeding sialyllactose and galactosylated N-acetylneuraminic acid on swimming learning ability and brain lipid composition in adult rats. Journal of Applied Glycoscience 53 (4):249–54. doi: 10.5458/jag.53.249.
- Samuel, T. M., Q. L. Zhou, F. Giuffrida, D. Munblit, V. Verhasselt, and S. K. Thakkar. 2020. Nutritional and non-nutritional composition of human milk is modulated by maternal, infant, and methodological factors. Frontiers in Nutrition 7:576133. doi: 10.3389/fnut.2020.576133.
- Salvolini, E., R. Di Giorgio, A. Curatola, L. Mazzanti, and G. Fratto. 1998. Biochemical modifications of human whole saliva induced by pregnancy. British Journal of Obstetrics and Gynaecology 105 (6):656–60. doi: 10.1111/j.14710528.1998.tb10181.x.
- Savaki, H. E., and G. M. Levis. 1977. Changes in rat brain gangliosides following active avoidance conditioning. Pharmacology, Biochemistry, and Behavior 7 (1):7–12. doi: 10.1016/0091-3057(77)90004-1.
- Schengrund, C. L., and C. M. Mummert. 1998. Exogenous gangliosides. How do they cross the blood-brain barrier and how do they inhibit cell proliferation Annals of the New York Academy of Sciences 845 (845):278–84. doi: 10.1111/j.1749-6632.1998.tb09681.x.
- Schnaar, R. L. 2004. Glycolipid-mediated cell-cell recognition in inflammation and nerve regeneration. Archives of Biochemistry and Biophysics 426 (2):163–72. doi: 10.1016/j.abb.2004.02.019.
- Schnaar, R. L., R. Gerardy-Schahn, and H. Hildebrandt. 2014. Sialic acids in the brain: Gangliosides and polysialic acid in nervous system development, stability, disease, and regeneration. Physiological Reviews 94 (2):461–518. doi: 10.1152/physrev.00033.2013.
- Scholtz, S. A., S. Gottipati, B. J. Gajewski, and S. E. Carlson. 2013. Dietary sialic acid and cholesterol influence cortical composition in developing rats. The Journal of Nutrition 143 (2):132–5. doi: 10.3945/jn.112.169508.
- Schwarzkopf, M., K. P. Knobeloch, E. Rohde, S. Hinderlich, N. Wiechens, L. Lucka, I. Horak, W. Reutter, and R. Horstkorte. 2002. Sialylation is essential for early development in mice. Proceedings of the National Academy of Sciences of the United States of America 99 (8):5267–70. doi: 10.1073/pnas.072066199.
- Seppala, R., V. P. Lehto, and W. A. Gahl. 1999. Mutations in the human UDP-N-acetylglucosamine 2-epimerase gene define the disease sialuria and the allosteric site of the enzyme. American Journal of Human Genetics 64 (6):1563–9. doi: 10.1086/302411.
- Shumake, J., D. W. Barrett, M. A. Lane, and A. J. Wittke. 2014. Behavioral effects of bovine lactoferrin administration during postnatal development of rats. Biometals 27 (5):1039–55. doi: 10.1007/s10534-014-9735-6.
- Soyyilmaz, B., M. H. Mikš, C. H. Röhrig, M. Matwiejuk, A. Meszaros‐matwiejuk, and L. K. Vigsnaes. 2021. The mean of milk: A review of human milk oligosaccharide concentrations throughout lactation. Nutrients 13 (8):2737. doi: 10.3390/nu13082737.
- Spichtig, V., J. Michaud, and S. Austin. 2010. Determination of sialic acids in milks and milk-based products. Analytical Biochemistry 405 (1):28–40. doi: 10.1016/j.ab.2010.06.010.
- Sprenger, N., and P. I. Duncan. 2012. Sialic acid utilization. Advances in Nutrition 3 (3):392S–75. doi: 10.3945/an.111.001479.
- Stiles, J., and T. L. Jernigan. 2010. The basics of brain development. Neuropsychology Review 20 (4):327–48. doi: 10.1007/s11065-010-9148-4.
- Svennerholm, L. 1994. Gangliosides - A new therapeutic agent against stroke and Alzheimer’s disease. Life Sciences 55 (25–26):2125–34. doi: 10.1016/0024-3205(94)00393-9.
- Swanson, L. W. 2018. Brain maps 4.0-structure of the rat brain: An open access atlas with global nervous system nomenclature ontology and flatmaps. The Journal of Comparative Neurology 526 (6):935–43. doi: 10.1002/cne.24381.
- Tang, K. T., L. Li-Na, C. Ya-Qi, and M. Shi-Fen. 2008. Determination of sialic acid in milk and products using high performance anion-exchange chromatography coupled with pulsed amperometric detection. Chinese Journal of Analytical Chemistry 36 (11):1535–8. doi: 10.1016/S1872-2040(09)60005-0.
- Tangvoranuntakul, P., P. Gagneux, S. Diaz, M. Bardor, N. Varki, A. Varki, and E. Muchmore. 2003. Human uptake and incorporation of an immunogenic nonhuman dietary sialic acid. Proceedings of the National Academy of Sciences of the United States of America 100 (21):12045–50. doi: 10.1073/pnas.2131556100.
- Tao, R., C. Li, Y. L. Zheng, W. Qin, J. Zhang, X. W. Li, Y. F. Xu, Y. Y. Shi, G. Y. Feng, and L. He. 2007. Positive association between SIAT8B and schizophrenia in the Chinese Han population. Schizophrenia Research 90 (1–3):108–14. doi: 10.1016/j.schres.2006.09.029.
- Tarr, A. J., J. D. Galley, S. E. Fisher, M. Chichlowski, B. M. Berg, and M. T. Bailey. 2015. The Prebiotics 3’Sialyllactose and 6’Sialyllactose diminish stressor-induced anxiety-like behavior and colonic microbiota alterations: Evidence for effects on the gut-brain axis. Brain, Behavior, and Immunity 50:166–77. doi: 10.1016/j.bbi.2015.06.025.
- Ten Bruggencate, S. J., I. M. Bovee-Oudenhoven, A. L. Feitsma, E. van Hoffen, and M. H. Schoterman. 2014. Functional role and mechanisms of sialyllactose and other sialylated milk oligosaccharides. Nutrition Reviews 72 (6):377–89. doi: 10.1111/nure.12106.
- Tettamanti, G., F. Bonali, S. Marchesini, and V. Zambotti. 1973. A new procedure for the extraction, purification and fractionation of brain gangliosides. Biochimica et Biophysica Acta 296 (1):160–70. doi: 10.1016/0005-2760(73)90055-6.
- Thurl, S., M. Munzert, G. Boehm, C. Matthews, and B. Stahl. 2017. Systematic review of the concentrations of oligosaccharides in human milk. Nutrition Reviews 75 (11):920–33. doi: 10.1093/nutrit/nux044.
- Tillisch, K., J. Labus, L. Kilpatrick, Z. G. Jiang, J. Stains, B. Ebrat, D. Guyonnet, S. Legrain-Raspaud, B. Trotin, B. Naliboff, et al. 2013. Consumption of fermented milk product with probiotic modulates brain activity. Gastroenterology 144 (7):1394–15. doi: 10.1053/j.gastro.2013.02.043.
- Tram, T. H., J. C. B. Miller, Y. McNeil, and P. McVeagh. 1997. Sialic acid content of infant saliva: Comparison of breast fed with formula fed infants. Archives of Disease in Childhood 77 (4):315–8. doi: 10.1136/adc.77.4.315.
- Tran, C., L. Turolla, D. Ballhausen, S. C. Buros, T. Teav, H. Gallart-Ayala, J. Ivanisevic, M. Faouzi, D. J. Lefeber, I. Ivanovski, et al. 2021. The fate of orally administered sialic acid: First insights from patients with N-acetylneuraminic acid synthase deficiency and control subjects. Molecular Genetics and Metabolism Reports 28:100777. doi: 10.1016/j.ymgmr.2021.100777.
- Triantis, V., L. Bode, and J. R. van Neerven. 2018. Immunological effects of human milk oligosaccharides. Frontiers in Pediatrics 6:190–14. doi: 10.3389/fped.2018.00190.
- UNCRC. 2009. The UN convention on the rights of the child. Unicef 12 (12).
- UNICEF. 2019. Global breastfeeding scorecard 2019. Global Breastfeeding Scorecard (3):4.
- Urashima, T., J. Hirabayashi, S. Sato, and A. Kobata. 2018. Human milk oligosaccharides as essential tools for basic and application studies on galectins. Trends in Glycoscience and Glycotechnology 30 (172):SE51–65. doi: 10.4052/tigg.1734.1SE.
- Vandenplas, Y., B. Berger, V. P. Carnielli, J. Ksiazyk, H. Lagström, M. S. Luna, N. Migacheva, J. Mosselmans, J. Picaud, M. Possner, et al. 2018. Human milk oligosaccharides: 2’-fucosyllactose (2’-FL) and lacto-n-neotetraose (LNnT) in infant formula. Nutrients 10 (9):1161. doi: 10.3390/nu10091161.
- Vanier, M. T., M. Holm, R. Öhman, and L. Svennerholm. 1971. Developmental profiles of gangliosides in human and rat brain. Journal of Neurochemistry 18 (4):581–92. doi: 10.1111/j.1471-4159.1971.tb11988.x.
- Van Karnebeek, C. D. M., L. Bonafé, X. Y. Wen, M. Tarailo-Graovac, S. Balzano, B. Royer-Bertrand, A. Ashikov, L. Garavelli, I. Mammi, L. Turolla, et al. 2016. NANS-mediated synthesis of sialic acid is required for brain and skeletal development. Nature Genetics 48 (7):777–84. doi: 10.1038/ng.3578.
- Van Leeuwen, S. S. 2019. Challenges and pitfalls in human milk oligosaccharide analysis. Nutrients 11 (11):2684. doi: 10.3390/nu11112684.
- Varki, A. 2007. Glycan-based interactions involving vertebrate sialic-acid-recognizing proteins. Nature 446 (7139):1023–9. doi: 10.1038/nature05816.
- Varki, A., and P. Gagneux. 2012. Multifarious roles of sialic acids in immunity. Annals of the New York Academy of Sciences 1253 (1):16–36. doi: 10.1111/j.1749-6632.2012.06517.x.
- Vawter, M. P. 2000. Dysregulation of the neural cell adhesion molecule and neuropsychiatric disorders. European Journal of Pharmacology 405 (1–3):385–95. doi: 10.1016/S0014-2999(00)00568-9.
- Victora, C. G., B. L. Horta, C. L. de Mola, L. Quevedo, R. T. Pinheiro, D. P. Gigante, H. Gonçalves, and F. C. Barros. 2015. Association between breastfeeding and intelligence, educational attainment, and income at 30 years of age: A prospective birth cohort study from Brazil. The Lancet. Global Health 3 (4):e199–205–e205. doi: 10.1016/S2214-109X(15)70002-1.
- Vieira Borba, V., K. Sharif, and Y. Shoenfeld. 2018. Breastfeeding and autoimmunity: Programing health from the beginning. American Journal of Reproductive Immunology 79 (1):e12778. doi: 10.1111/aji.12778.
- Wang, B. 2009. Sialic acid is an essential nutrient for brain development and cognition. Annual Review of Nutrition 29 (1):177–222. doi: 10.1146/annurev.nutr.28.061807.155515.
- Wang, B. 2012. Molecular mechanism underlying sialic acid as an essential nutrient for brain development and cognition. Advances in Nutrition (Bethesda, MD) 3 (3):465S–72S. doi: 10.3945/an.112.001875.
- Wang, B., and J. Brand-Miller. 2003. The role and potential of sialic acid in human nutrition. European Journal of Clinical Nutrition 57 (11):1351–69. doi: 10.1038/sj.ejcn.1601704.
- Wang, B., J. Brand-Miller, P. McVeagh, and P. Petocz. 2001a. Concentration and distribution of sialic acid in human milk and infant formulas. The American Journal of Clinical Nutrition 74 (4):510–5. doi: 10.1093/ajcn/74.4.510.
- Wang, B., J. A. Downing, P. Petocz, J. Brand-Miller, and W. L. Bryden. 2007a. Metabolic fate of intravenously administered N-acetylneuraminic Acid-6-14C in newborn piglets. Asia Pacific Journal of Clinical Nutrition 16 (1):110–5.
- Wang, B., H. H. Hu, and B. Yu. 2006. Molecular characterization of pig ST8Sia IV-a critical gene for the formation of neural cell adhesion molecule and its response to sialic acid supplement in piglets. Nutritional Neuroscience 9 (3–4):147–54. doi: 10.1080/10284150600903594.
- Wang, B., H. H. Hu, B. Yu, and F. A. Troy. 2007b. Molecular characterization of pig UDP-N-acetylglucosamine-2-epimerase/N-acetylmannosamine kinase (Gne) Gene: Effect of dietary sialic acid supplementation on gene expression in piglets. Current Topics in Nutraceutical Research 5 (4):165–75.
- Wang, B., P. McVeagh, P. Petocz, and J. Brand-Miller. 2003. Brain ganglioside and glycoprotein sialic acid in breastfed compared with formula-fed infants. The American Journal of Clinical Nutrition 78 (5):1024–9. doi: 10.1093/ajcn/78.5.1024.
- Wang, B., J. Brand-Miller, Y. McNeil, and P. McVeagh. 1998. Sialic acid concentration of brain gangliosides: Variation among eight mammalian species. Comparative Biochemistry and Physiology Part A: Molecular & Integrative Physiology 119 (1):435–9. doi: 10.1016/S1095-6433(97)00445-5.
- Wang, B., J. Brand-Miller, Y. Sun, Z. Ahmad, P. McVeagh, and P. Petocz. 2001b. A longitudinal study of salivary sialic acid in preterm infants: Comparison of human milk-fed versus formula-fed infants. The Journal of Pediatrics 138 (6):914–6. doi: 10.1067/mpd.2001.113044.
- Wang, B., B. Yu, M. Karim, H. H. Hu, Y. Sun, P. McGreevy, P. Petocz, S. Held, and J. Brand-Miller. 2007c. Dietary sialic acid supplementation improves learning and memory in piglets. The American Journal of Clinical Nutrition 85 (2):561–9. doi: 10.1093/ajcn/85.2.561.
- Wang, H. X., Y. Chen, Z. Haque, M. de Veer, G. Egan, and B. Wang. 2021. Sialylated milk oligosaccharides alter neurotransmitters and brain metabolites in piglets: An in vivo magnetic resonance spectroscopic (MRS) study. Nutritional Neuroscience 24 (11):885–95. doi: 10.1080/1028415X.2019.1691856.
- Wang, H. Y., C. Braun, E. F. Murphy, and P. Enck. 2019. Bifidobacterium longum 1714TM strain modulates brain activity of healthy volunteers during social stress. American Journal of Gastroenterology 114 (7):1152–62. doi: 10.14309/ajg.0000000000000203.
- Weinhold, B., R. Seidenfaden, I. Röckle, M. Mühlenhoff, F. Schertzinger, S. Conzelmann, J. D. Marth, R. Gerardy-Schahn, and H. Hildebrandt. 2005. Genetic ablation of polysialic acid causes severe neurodevelopmental defects rescued by deletion of the neural cell adhesion molecule. The Journal of Biological Chemistry 280 (52):42971–7. doi: 10.1074/jbc.M511097200.
- Wielgat, P., and J. J. Braszko. 2012. Significance of the cell adhesion molecules and sialic acid in neurodegeneration. Advances in Medical Sciences 57 (1):23–30. doi: 10.2478/v10039-012-0011-0.
- Wreden, C. C., M. Wlizla, and R. J. Reimer. 2005. Varied mechanisms underlie the free sialic acid storage disorders. The Journal of Biological Chemistry 280 (2):1408–16. doi: 10.1074/jbc.M411295200.
- Yan, J. Y., J. J. Ding, G. W. Jin, D. P. Yu, L. Yu, Z. Long, Z. M. Guo, W. G. Chai, and X. M. Liang. 2018. Profiling of sialylated oligosaccharides in mammalian milk using online solid phase extraction-hydrophilic interaction chromatography coupled with negative-ion electrospray mass spectrometry. Analytical Chemistry 90 (5):3174–82. doi: 10.1021/acs.analchem.7b04468.
- Yin, T. T., L. C. Yang, Y. N. Liu, X. B. Zhou, J. Sun, and J. Liu. 2015. Sialic acid (SA)-modified selenium nanoparticles coated with a high blood-brain barrier permeability peptide-B6 peptide for potential use in Alzheimer’s disease. Acta Biomaterialia 25:172–83. doi: 10.1016/j.actbio.2015.06.035.
- Yu, R. K., Y. Tsai, T. Ariga, and M. Yanagisawa. 2011. Structures, biosynthesis, and functions of gangliosides - An overview. Journal of Oleo Science 60 (10):537–44. doi: 10.5650/jos.60.537.
- Yu, Z. T., C. Chen, and D. S. Newburg. 2013. Utilization of major fucosylated and sialylated human milk oligosaccharides by isolated human gut microbes. Glycobiology 23 (11):1281–92. doi: 10.1093/glycob/cwt065.
- Zhou, L. H., and J. A. Foster. 2015. Psychobiotics and the gut-brain axis: In the pursuit of happiness. Neuropsychiatric Disease and Treatment 11:715–23. doi: 10.2147/NDT.S61997.