Abstract
Undernutrition and inflammatory processes are predictors of early mortality in the elderly and require a rapid and accurate diagnosis. Currently, there are laboratory markers for assessing nutritional status, but new markers are still being sought. Recent studies suggest that sirtuin 1 (SIRT1) has the potential to be a marker for undernutrition. This article summarizes available studies on the association of SIRT1 and undernutrition in older people. Possible associations between SIRT1 and the aging process, inflammation, and undernutrition in the elderly have been described. The literature suggests that low SIRT1 levels in the blood of older people may not be associated with physiological aging processes, but with an increased risk of severe undernutrition associated with inflammation and systemic metabolic changes.
Introduction
According to global demographics, by 2050, there will be more people over 60 years of age than those under 15, and a total population of 2.1 billion compared to 901 million in 2015 (United Nations Department of Economic and Social Affairs, Population Division Citation2022). The aging of the population is associated with rising social costs, including the provision of adequate health services. Aging is a main risk factor for many serious diseases, including type 2 diabetes, cancer, cardiovascular, metabolic, neurodegenerative diseases, and malnutrition.
According to the European Society for Clinical Nutrition and Metabolism (ESPEN), the definition of malnutrition is unambiguous, open-ended and often used to describe undernutrition (Cederholm et al. Citation2015). “Malnutrition” or “undernutrition” are equally used both in the literature and in clinical practice, with a slight preponderance for malnutrition to describe the inadequate supply of energy and nutrients. In this article, the above terms are used equivalently. The risk of malnutrition increases with age (Russell and Elia Citation2011) and occurs in 27% of the population and 50% of people living in health facilities (Cereda et al. Citation2016). In the elderly, malnutrition may be associated with inadequate nutrient intake, leading to changes in body composition, which reduce physical and mental function and deteriorate clinical outcomes (Morley Citation2001). Malnutrition is accompanied by inflammation, which increases the need for energy and protein (Rondanelli et al. Citation2016).
Various causes of malnutrition in the elderly include nutritional disorders, gastrointestinal diseases, and impaired chewing due to poor or absent dentition. Aging is associated with a loss of organoleptic qualities, such as reduced taste and smell sensation, as well as reduced physical activity, which can significantly affect nutrient intake and lead to malnutrition with potentially serious health consequences (Hickson Citation2006).
The incidence of malnutrition is estimated to be 5.8% (Robberecht et al. Citation2019), while the risk of malnutrition may affect one third of elderly people (Kaiser et al. Citation2010). This risk increases when patients have difficulty performing basic tasks of daily life (Tamura et al. Citation2013). In addition, it carries an increased long-term risk, and individual nutritional support can reduce the risk of malnutrition (Hickson Citation2006).
Elderly people may experience frailty owing to chronic inflammation which may lead to the development of eating disorders. Symptoms of frailty are mainly sarcopenia, including loss of skeletal muscle mass, dysfunction of the neuroendocrine and immune systems, cognitive and motor disorders, and disturbances in energy regulation (Clegg et al. Citation2013). In the elderly, sarcopenia and inflammation frequently co-occur with malnutrition (Morley Citation2010; Volkert et al. Citation2019). In many studies, oxidative stress, central obesity, serum albumin (Wu et al. Citation2009), inflammation (Leng et al. Citation2002, Citation2004, Citation2007, Citation2009), 25-hydroxy vitamin D (Hirani et al. Citation2013), and vitamin E (Ble et al. Citation2006) have been used as parameters to identify malnutrition, sarcopenia, and frailty. Recent studies suggest a link between malnutrition in the elderly and Sirtuin 1 (SIRT1) (El Assar et al. Citation2018). This literature review aimed to investigate whether SIRT1 is associated with malnutrition and whether it can be used as a marker of malnutrition in the elderly, a population heavily affected.
Undernutrition
Criteria for diagnosing the risk of malnutrition
There are several criteria for diagnosing malnutrition. ESPEN distinguishes the following nutritional disorders: malnutrition, micronutrient abnormalities, and overnutrition (Cederholm et al. Citation2015). Malnutrition includes starvation-related weight loss, disease-related cachexia, frailty, and sarcopenia. The definition of malnutrition according to ESPEN is based on a decrease in body mass index (BMI) <18.5 kg/m2 or unintentional weight loss >10% indefinitely or >5% in the past three months combined with a BMI < 20 kg/m2 for those aged 70 years or <22 kg/m2 for those aged ≥ 70 years or fat-free mass index < 15 and 17 kg/m2 in women and men (Cederholm et al. Citation2015), respectively. The Global Leadership Initiative on Malnutrition (GLIM) developed in 2020 new diagnostic criteria for undernutrition, including three phenotypic and two etiological criteria (de van der Schueren et al. Citation2020). According to GLIM, the diagnosis of undernutrition requires at least one phenotypic and etiological criterion (Cederholm et al. Citation2019; Jensen et al. Citation2019).
If malnutrition is suspected, screening tools should be used to quickly assess its risk. There are several screening tools including the Mini-Nutritional Assessment (MNA) (Burns, Brayne, and Folstein Citation1998; Rubenstein et al. Citation2001; Vellas et al. Citation2006), the Nutrition Risk Screening (NRS-2002) (Liu, Zhang, et al. Citation2012), the Subjective Global Assessment Short Form (SGA) (Allard et al. Citation2020), and the Malnutrition Universal Screening Tool (MUST) The MNA is currently the most widely used screening tool for assessing malnutrition in older people. Donini et al. (Citation2016) evaluated several screening tools, such as MNA, NRS-2002, and MUST, and revealed that MNA was the best tool for predicting survival in well-nourished older people in nursing homes. The procedures used for detecting malnutrition are presented in .
Table 1. Instruments to identify the risk of malnutrition.
Laboratory indicators of nutritional status
The most important and common blood indicators used in the diagnosis of malnutrition include visceral proteins (Volpi et al. Citation2001), such as albumin, prealbumin, and retinol-binding protein, which are primarily synthesized in the liver. Low protein and energy intake, impaired liver synthesis, and inflammation in the body contribute to a decrease in visceral protein levels. Acute inflammation increases protein production, whereby the liver alters protein synthesis to reduce the synthesis of visceral proteins. In turn, the level of visceral protein correlates with the severity of the damage.
Although malnutrition is often associated with inflammation, the role of inflammatory biomarkers in malnutrition remains unclear. Above all, the biomarkers have low specificity and the presence of inflammation has a strong effect on visceral proteins in the serum. The uncertainty of the role of biomarkers is confirmed by the fact that their role has not been investigated in large randomized controlled studies (Cholewa et al. Citation2017).
Zhang et al. discussed the role of biomarkers in assessing the severity of malnutrition in the elderly and considered validated tools for assessing nutritional status (Zhang et al. Citation2017). They analyzed 111 observational and cohort studies with 52,911 participants from a variety of clinical settings. Biomarkers such as BMI and concentrations of albumin, hemoglobin, total cholesterol, prealbumin, and total protein among those at high risk of malnutrition assessed by MNA were significantly lower than those not at risk (p < .001). In the case of malnutrition, as determined by SGA and NRS 2002 in patients with acute illnesses, the predictive value of albumin and prealbumin was markedly reduced, leading to the conclusion that they are markers of inflammation rather than malnutrition. According to the authors, BMI, hemoglobin, and total cholesterol are useful markers of malnutrition in older adults.
The role of inflammation in the pathology of malnutrition
Inflammation plays a critical role in malnutrition pathophysiology. Weight loss is considered one of the most important criteria for malnutrition but disregarding other criteria may lead to an inappropriate diagnosis (Kirkland et al. Citation2013).
Inflammatory cytokines such as tumor necrosis factor-alpha (TNFα), Interleukin-1 (IL1), Interleukin-6 (IL6), serotonin, and gamma interferon play a significant role in the development of cachexia, as they stimulate the release of acute phase proteins, protein breakdown in muscle, and fat breakdown. The increase in pro-inflammatory cytokines is influenced by the progressive increase in glucocorticoids and catecholamines as well as by the decrease in growth and sex hormones. Studies suggest that systemic inflammation associated with TNFα production is involved in the mechanism of muscle mass loss and anorexia. In addition, this mechanism may occur during aging (Godoy et al. Citation1996).
Researchers have suggested that aging causes a pro-inflammatory response, which may be associated with a gradual decline in physical activity and initially manifests as chronic, low-grade inflammation (Crossland et al. Citation2010). In the development of inflammation, the source of inflammatory molecules appears to be fatty tissue (especially visceral fatty tissue), which contributes to the increased activation and infiltration of immune cells, especially macrophages and neutrophils. Activated macrophages can stimulate lipolysis in visceral adipose tissue and increase the concentration of circulating free fatty acids (FFA) by signaling TNF-α/Toll-like receptor-4. While increased levels of FFA amplify macrophages to increase secretion of important anti-inflammatory molecules (such as TNF-α, IL-1, and IL-6), these molecules also influence skeletal muscles, especially in those with a sedentary lifestyle. Increased levels of TNF-α, IL-6, and FFA appear to contribute to the activation of skeletal muscle proteolysis and insulin resistance, which are two major factors involved in different types of muscle atrophy. Degradation and reduction of the body proteins needed for the proper functioning of the organs, in favor of visceral proteins as an inflammatory reaction, gradually deteriorates the health of older people (Brocca et al. Citation2012). The occurrence of other comorbidities such as cancer or heart disease may contribute to the release of catabolic hormones such as cortisol, inflammatory cytokines, and increased reactive oxygen species (ROS). This process may be associated with the risk of malnutrition and concomitant sarcopenia (Crossland et al. Citation2008).
Chronic systemic inflammation is an important factor in both sarcopenia and malnutrition. If inflammation can be regulated by pro-inflammatory mediators, such as TNF-α, IL-1, and IL-6, malnutrition may be influenced. The pathway mediated by forkhead box 03 (FOX03), which activates the ubiquitin-proteasome system, is also involved in the inflammatory process, which primarily involves TNF-α and IL-6 (Sente et al. Citation2016). These cytokines may not only promote sarcopenia (Budui, Rossi, and Zamboni Citation2015) but also inflammatory malnutrition due to the interaction between inflammatory cells and organs, leading to reduced protein synthesis, increased protein breakdown, impairment of organ function, and the manifestation of clinical diseases associated with malnutrition (Jensen et al. Citation2010).
Inflammatory response in malnutrition
Malnutrition has been shown to be associated with inflammation (Bourke, Berkley, and Prendergast Citation2016). The proper development of the immune system is altered by malnutrition during critical periods in humans, including pregnancy, newborn puberty, and weaning (Keusch Citation2003; McDade et al. Citation2001). Chronic malnutrition and infection in children have been shown to weaken the immune response, leading to changes in immune cells and an increase in inflammatory mediators (Dülger et al. Citation2002). In addition, malnutrition in the early years of life increases the risk of chronic inflammation (Khoruts Citation2018; Latini et al. Citation2004; Martin-Gronert and Ozanne Citation2012), obesity (WHO 2004) (Swinburn et al. Citation2019), adverse metabolic alterations (Crispi, Miranda, and Gratacós Citation2018; Sommer et al. Citation2017), and intestinal dysfunction (Heijmans et al. Citation2008; Winick and Noble Citation1966) later in life.
The sirtuin family
Division and functions of sirtuins
Sirtuin proteins are involved in cellular processes, such as mitochondrial biosynthesis, lipid metabolism, apoptosis, cellular stress response, fatty acid oxidation, insulin secretion, and aging (Tanno et al. Citation2007). Eighteen different histone deacetylases (HDACs) have been identified in humans. HDACs are grouped into four classes (class I, II, III, and IV) and two families: classical and sirtuins (Gregoretti, Lee, and Goodson Citation2004). Sirtuins are a heterogeneous group of class III histone-nicotinamide adenine dinucleotide (NAD)-deacetylase-dependent enzymes that are not sequentially similar to the classic HDAC family but have a high sequence similarity to silent information regulator 2 (SIR2), originally discovered in the yeast Saccharomyces cerevisiae (Asher and Schibler Citation2011; Finkel, Deng, and Mostoslavsky Citation2009). Homologs of the yeast SIR2 gene have been detected in most organisms, including mammals (Haigis and Sinclair Citation2010). Seven isoforms of sirtuin, SIRT1, SIRT2, SIRT3, SIRT4, SIRT5, SIRT6, and SIRT7, have been identified in humans, which contain a conserved catalytic core domain composed of approximately 275 amino acids, except SIRT1 which is expressed. The sirtuins show different tissue specificities, target substrates, and cell localization (Price et al. Citation2012). A summary of all sirtuins, including the general distribution, the enzymes involved, and their locations, is given in .
Table 2. Division of sirtuins based on Manjula, Anuja, and Alcain (Citation2020), Yan et al. (Citation2018), Wu et al. (Citation2018), Gao et al. (Citation2018).
SIRT1
SIRT1 is a nuclear protein that deacetylates transcription factors and cofactors that regulate metabolic pathways in the circadian rhythm and energy metabolism (Nakahata et al. Citation2008). SIRT1 plays key roles in aging, inflammation, gene silencing, apoptosis, and stress resistance (Chung et al. Citation2010; Rahman et al. Citation2012; Yao and Rahman Citation2012). These functions are mainly performed by the deacetylation of histones, transcription factors, or coactivators such as p53, forkhead box 0 (FOX0), nuclear factor αB (NF-αB), and coactivator 1α of the peroxisome proliferator-activated γ receptor (PGC-1α) and heterodimer Ku70 (Yao et al. Citation2012). The system-related biological functions of Sirtuin 1 are shown in .
Table 3. Biological functions of sirtuin 1 in the human body (authors’ own study).
Modulatory function of sirtuins
Studies have shown that molecules that activate sirtuins in humans can offer broad health benefits with strong anti-inflammatory, cardioprotective, neuroprotective, and anti-cancer effects (Kugel et al. Citation2016).
The therapeutic potential of increased SIRT1 activity in mammals has been demonstrated in animal models and in early clinical studies, making this enzyme an attractive drug target (Bonkowski and Sinclair Citation2016; Hubbard and Sinclair Citation2014). Therefore, the modulation of sirtuin activity may play an important role in the development of new therapies for age-related and metabolic diseases. Most sirtuin activators described concern SIRT1, inhibitors of SIRT1 and SIRT2, and of the least concern are SIRT3 and SIRT5 (Alcaín et al. Citation2010). The most effective substance, activating SIRT1 by more than 10 times, is resveratrol (3,5,4′-trihydroxystilben), a natural product of grapes and red wine (Gertz et al. Citation2012). In addition, a variety of synthetic resveratrol derivatives with changes at the B ring 4′ position may improve naturally occurring sirtuin activators and reduce toxicity to human cells, as well as increase the strength of SIRT1 activation and longer life in budding yeasts (Yang et al. Citation2007).
Reports have suggested that SIRT1 inhibitors and other sirtuin isoforms may also be useful (Chalkiadaki and Guarente Citation2015). Increasing advances in research and a growing number of structures of the sirtuin/inhibitor complex have aimed to develop potent and specific inhibitors targeting sirtuin (Schutkowski et al. Citation2014). Some pathophysiologies and sirtuin isoforms require inhibition rather than activation, for example, SIRT2 in neurodegenerative diseases (Donmez and Outeiro Citation2013). Studies have shown that inhibitors have the potential to be used as therapeutic drugs, including salermide, which inhibits the apoptosis of cancer cells, and suramine, an antiprotozoic agent. Sirtuin inhibitors are listed in .
Table 4. Sirtuin inhibitor compounds.
Nutritional regulation of SIRT1 expression
The activity of sirtuins depends on the availability of NAD + and its precursors, such as niacin and nicotinamide, which stimulate sirtuins (Bleeker and Houtkooper Citation2016). To explain the mechanism regulating SIRT1 expression, a reference should be made to the complex reactions catalyzed by sirtuins, in which NAD + is degraded to nicotinamide and adenine. The effects of these enzymes require NAD + resynthesis, which occurs in two reactions. In the first reaction, catalyzed by nicotinamide phosphoribosyl transferase (NAMPT), phosphoribose binds to nicotinamide, resulting in nicotinamide mononucleotide. Subsequently, adenine binding catalyzes nicotinic acid mononucleotide adenyltransferase. Studies in mice have shown that the increase in NAD + levels is due to an increase in NAMPT activity (Revollo, Grimm, and Imai Citation2004). In other studies, the administration of NAMPT to young and older mice also increased the expression and activity of SIRT1 (de Picciotto et al. Citation2016).
Calorie restriction
Today, one of the best-described and proven factors to increase the sirtuin activity is the limitation of energy intake. However, the effects of the level of nutrient deficiency, duration, and type of organism studied on sirtuin levels remain unclear.
The natural and synthetic activators of sirtuins, their possible health effects, and their sources in food are summarized in and .
Table 5. Natural activators of sirtuins and their sources.
Table 6. Synthetic activators of sirtuins.
SIRT1 expression increases in response to hunger or food restriction. During short-term famine, the cyclic AMP-activated factor (CREB) migrates through glucagon into the cell nucleus, binds to the promoter of the SIRT1 gene, and induces its expression. Simultaneously, high protein kinase A activity inhibits the translocation of the transcription factor that binds the carbohydrate response sequence (ChREBP) from the cytoplasm to the nucleus, thereby reversing its inhibitory effect on SIRT1 expression.
This process occurs during starvation for more than 24 h and is likely associated with deacetylation, and consequently, the inactivation of CREBP by SIRT1. When food is available, the glucose level in the cells increases, leading to rapid activation of ChREBP in the nucleus, where it suppresses the expression of the SIRT1 gene (Noriega et al. Citation2011).
In another study, a low-calorie diet was preferable to the Sirtuin-1 activator resveratrol. In patients with nonalcoholic fatty liver disease, the calorie-based diet reduced weight, BMI, waist circumference, waist-to-hip ratio, alanine aminotransferase, aspartate aminotransferase, and lipid profiles compared to resveratrol with placebo (Asghari et al. Citation2018).
Effect of nutrients on inhibition of SIRT1 expression
An excessive supply of energy leads to inhibition of the expression and activity of sirtuins. A high-fat diet (HFD) has been shown to reduce hepatic function (Valdecantos et al. Citation2012, Citation2017), and skeletal muscle (Haohao et al. Citation2015) expression of SIRT1 and SIRT3. This may be related to studies in which short or long-term HFDs and high ethanol consumption synergistically induce acute liver injury in mice (Chang et al. Citation2015).
The long-term use of a high-carbohydrate diet reduces SIRT1 expression more than an HFD with the same energy value. A high intake of carbohydrates in the diet, without excessive energy, leads to fatty liver and inhibition of NAMPT activity. Decreased SIRT1 levels under a high-carbohydrate diet are associated with increased miR-34 expression, which directly inhibits NAMPT in obesity (Li et al. Citation2015).
High levels of simple sugars in the body inhibit sirtuin activity. In vitro studies on tubular endothelial cells have shown that glucose inhibited SIRT1 expression (Zhou et al. Citation2015). In in vivo studies, a decrease in all SIRT1-SIRT7 sirtuins was observed in the hearts of mice with type 1 diabetes. Animals with type 2 diabetes have lower SIRT1 and SIRT2 levels and increased SIRT3 levels in the heart muscle (Bagul, Dinda, and Banerjee Citation2015).
Excess fructose may also contribute to a disturbance of sirtuin expression. The muscles of mice fed a fructose-rich diet exhibited increased levels of advanced glycation products (AGE), which inhibited the activation of SREBP-1c, thereby increasing the intensity of hepatic lipogenesis. The decrease in SIRT1 activity induced by AGE was also associated with a change in muscle fiber composition and a decrease in muscle strength. These studies indicate the possible negative effects of excessive dietary fructose on the risk of malnutrition (Mastrocola et al. Citation2016).
Effect of nutrients on the induction of SIRT1 expression
Adequate dietary intake of branched-chain amino acids is important for regulating sirtuin activity. Higher SIRT1 expression in the heart and diaphragm was observed in mice fed 1.5 mg branched-chain amino acids/kg/day compared to animals without supplementation. Additionally, physical activity amplifies this effect. Leucine increased the expression of SIRT1 in the liver, brown fat, and skeletal muscle of mice fed high-fat and low-fat diets (Li et al. Citation2012).
Studies have shown that certain substances can induce SIRT1 activity. Bogacka et al. showed that in non-diabetics, SIRT1 levels in adipose tissue were increased when caffeine, ephedrine, and the oral antidiabetic drug pioglitazone were administered concomitantly (Bogacka et al. Citation2007).
The intake of bioactive lipids in the diet appears to have a positive effect. Studies have shown that polyunsaturated fatty acids stimulate SIRT1 by kinase A-dependent activation of the SIRT1-PGC1α complex, thereby increasing the oxidation rate of fatty acids and preventing aging-related lipid dysregulation (Das Citation2021).
Other studies have shown that components of dairy products can act as SIRT1 activators. The consumption of dairy products leads to systemic changes that can promote the biogenesis of mitochondria in important target tissues, such as muscle and fatty tissue, both by direct activation of SIRT1 and by SIRT1-independent pathways (Bruckbauer and Zemel Citation2011). Leucine administration stimulates mitochondrial biogenesis and fat metabolism in both adipocytes and muscle cells. These effects were partly mediated by SIRT1 and were significantly attenuated by SIRT1 (Sun and Zemel Citation2009).
Materials and methods
Search strategy
PubMed databases were used to identify studies with the combined search terms such as sirt 1 AND inflammation, sirt 1 AND malnutrition, sirt 1 AND undernutrition, and with single search terms such as malnutrition, undernutrition, elderly, sirtuin 1, inflammation, oxidative stress, sarcopenia, frailty, weakness syndrome, biomarkers of inflammation, biomarkers of undernutrition, biomarkers of malnutrition, caloric restriction, aging, and nutritional status. In order to increase the accuracy of the literature search on the subject of the work, the year of publication was not definitively limited. The inclusion criteria were publications in English, such as randomized controlled trials, clinical trials, meta-analyses, and systematic reviews. Publications involving animal experiments were also included. To better identify relevant studies and journals, review and original articles were searched manually using the ISNN journal databases. The search terms are shown in . Duplicates and publications based only on abstracts were excluded ().
Effects of malnutrition on SIRT1 activity
Reduction of body reserves after level 1—insufficient nutrient intake, including metabolic disorders, increased demand, undernourishment, or excessive weight loss leads to depletion of body reserves and mild undernourishment (step 2). Minor signs of malnutrition lead to simple, uncomplicated dietary hunger (Level 3). During prolonged fasting, hunger, or extreme diets, NAD + levels increase as both NAD + biosynthesis and oxidation to NAD + increase (Cantó, Menzies, and Auwerx Citation2015). This leads to an increase in SIRT1 as a result of NAD + expression (step 3). The last stage of the pathomechanism of malnutrition is the occurrence of organ lesions and adverse changes in the body, such as increased catabolism and reduced protein, fat, and energy requirements within 24 h, which contribute to a decrease in SIRT1 levels in the body. According to previous studies, SIRT1 decreases in the fasting state of the pancreas, and during this time the mitochondrial uncoupling protein 2 increases, which may explain the lower insulin secretion due to calorie reduction (Bordone et al. Citation2006). A decline in SIRT1 is caused by long-term starvation, which leads to a physiological reduction in insulin and fasting glucose levels. A decrease in SITR1 in the pancreas may also be associated with malnutrition, as described in the three types of inflammatory disease-related malnutrition (DRM), non-inflammatory DRM, and disease-free malnutrition (Cederholm et al. Citation2017). However, the exact role of fasting in the activity of SIRT1 is not yet fully understood and appears to vary from tissue to tissue (Chen et al. Citation2008).
The pathomechanisms of malnutrition and possible SIRT1 relationship are shown in (in-house development based on the ESPEN guidelines).
Figure 3. The pathomechanism of malnutrition and the effects of malnutrition on blood SIRT1 levels. Inadequate supply of food constituents contributes to the depletion of body reserves, which in turn leads to disruptions of physiological processes and disruptions of biochemical processes. These changes lead to minor symptoms of malnutrition, which lead to starvation of the uncomplicated simple depending on the diet. The uncomplicated simple hunger increases the expression of NAD+, which in turn increases the expression of SIRT1. However, the persistent depletion of the body’s reserves leads to more severe organ damage, which manifests itself in catabolism, protein loss in the body, fat loss, energy demand and glycogen loss in the body within 24 h. This leads to a decrease in the level of SIRT1 in the body. Unfavorable organ changes contribute to malnutrition, which can affect DRM + I, DRM − I, Malnutrition without disease, depending on the severity (DRM disease related malnutrition—ESPEN). Authors own study based on Bordone et al. (Citation2006); Cantó, Menzies, and Auwerx (Citation2015); Cederholm et al. (Citation2017); Chen et al. (Citation2008); McClain, Kirpich, and Smart (Citation2017); Nemoto, Fergusson, and Finkel (Citation2004).
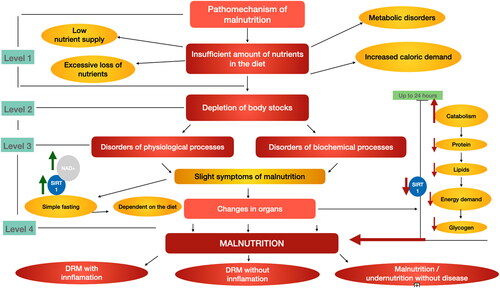
SIRT1 is involved in glycolipid metabolism in the liver. In short-term hunger, SIRT1 may inhibit target of rapamycin C2, which in turn inhibits gluconeogenesis and lowers blood sugar levels. Short-term fasting increases the expression and concentration of SIRT1 (Nemoto, Fergusson, and Finkel Citation2004). During prolonged starvation, SIRT1 deacetylates and activates the substrates peroxisome proliferator-activated receptor alpha (PPARα) and PPAR-γ co-activator-1α (PGC-1α), which contribute to the oxidation of fatty acids and improvement of glucose homeostasis. Second, SIRT1 deacetylates forkhead box 01 (FOX01) and signal transducers and activators of transcription 3 (STAT3) by promoting gluconeogenesis and inhibiting glycolysis, respectively. SIRT1 deacetylates FOX01 in hepatocytes, thereby releasing glucose. Long-term starvation contributes to a reduction in SIRT1 levels. The dietary status in liver disease may depend on factors such as overeating, malnutrition, or altered metabolism (McClain, Kirpich, and Smart Citation2017). Patients with complications such as liver disease, ascites, and hepatic encephalopathy have been shown to have a higher incidence of sarcopenia and malnutrition (Knudsen et al. Citation2016). The role of SIRT1 in hepatic glycolipid metabolism appears to be promising, and activation of SIRT1 may be beneficial not only in metabolic disorders and aging retardation, but also in malnutrition (Bonda et al. Citation2011; Mouchiroud et al. Citation2013). The fasting hepatic glycolipid metabolism by sirtuin 1 is shown in .
Figure 4. Effect of SIRT1 in hepatic glycolipid metabolism under starvation. Authors’ own study based on Hallows, Yu, and Denu (Citation2012); Horton, Goldstein, and Brown (Citation2002); Purushotham et al. (Citation2009).
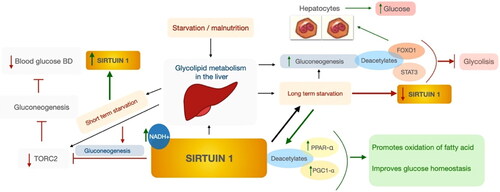
The human defense system consists of different enzymatic and non-enzymatic components (Harman Citation1956). These enzymes include glutathione peroxidase (GSH-Px), glutathione reductase (GSH-Rd), superoxide dismutase (SOD), and catalase. Non-enzymatic substances include endogenous antioxidants, such as reduced glutathione (GSH), ubiquinone (coenzyme Q10), uric acid, alpha lipoic acid, metallothionein, albumin, transferrin, and ceruloplasmin, as well as exogenous antioxidants, such as vitamin E (especially a-tocopherol), carotenoids (β-carotene, α-carotene, lycopene, lutein, zeaxanthin, astaxanthin, and canthaxanthin), vitamin C, flavonoids, mannitol, aminoguanidine and pyridoxine (Ferreira et al. Citation2011; Vasconcelos et al. Citation2007).
Elderly people with poor nutrition and malnutrition have low body weight, which in turn predisposes them to a variety of complications, such as reduced immunity (Lesourd and Mazari Citation1997), functional impairment (Moreira Citation2011), frailty (Wu et al. Citation2009) (with involuntary weight loss, fatigue, weakness, reduced walking speed, and physical activity) (Macedo, Gazzola, and Najas Citation2008), and an increase in morbidity and mortality (Vetta et al. Citation1999).
Chronic malnutrition has been shown to increase oxidative stress as albumin concentration in the body decreases, which in turn can act as an antioxidant (Fechner et al. Citation2001). Malnutrition followed by hypoalbuminemia increases oxidative stress in the plasma and liver (Fechner et al. Citation2001; Manary, Leeuwenburgh, and Heinecke Citation2000; van Zutphen et al. Citation2016).
Excessive oxidative stress impairs mitochondrial function due to mitochondrial DNA failure (Nissanka and Moraes Citation2018). Malnutrition may increase oxidative stress in skeletal muscles, which are rich in mitochondria. It has been shown that cellular oxidation can occur during the aging process itself and that these factors carry the risk of chronic diseases in older people (Halliwell and Gutteridge Citation2015). Oxidative stress causes cell aging, increasing the role of SIRT1 in protecting cells from aging by regulating FOX0, p53, and p21, as well as molecules involved in DNA damage and repair (Furukawa et al. Citation2007; Yao and Rahman Citation2012).
There is an association between plasma levels of oxidative stress markers (8-iso-PGF2α and 8-oxo-dGuo), nutritional status indicators (BMI), and cardiovascular risk (waist-hip ratio) (Tôrres et al. Citation2013).
Studies show that SIRT1 regulates oxidative stress and cellular toxicity (Chua et al. Citation2005; Kitamura et al. Citation2005). Causes may include SIRT1-mediated induction of manganese peroxide dismutase (MnSOD) by deacetylation and activation of the transcription factor FOX03. Research shows that the induction enhancer is resveratrol, which increases resistance to oxidative stress in myoblasts (Tanno et al. Citation2010). In other studies, FOX03 activation has also been shown to protect against oxidative stress caused by MnSOD (Kops et al. Citation2002; Li et al. Citation2006). SIRT1 expression and FOX03 deacetylation, which results in increased catalase expression, also protects the heart from oxidative stress in mouse models (Alcendor et al. Citation2007). Liver SIRT1 deficiency increases ROS production, impairing TOR/Akt signaling in other insulin-sensitive organs, resulting in insulin resistance (Kume et al. Citation2006).
SIRT1 inhibits IKB kinase, which in turn inhibits IKB degradation and, thus, the inflammatory response. Activation of SIRT1 leads to deacetylation and activation of the transcription factor FOX03, resulting in the production of catalase and MnSOD. Both compounds reduce oxidative stress. The mechanism for regulating oxidative stress and inflammation by SIRT1 is shown in .
These studies suggest that oxidative stress, inflammation, and malnutrition are closely associated (Hirabayashi et al. Citation2021). Inflammation associated with disease is often observed in undernourished individuals. Inflammation affects the body’s need for nutrients as well as food intake, including appetite. It contributes to changes in metabolism, including increased resting energy consumption and increased muscle catabolism (Jensen Citation2015). Furthermore, malnutrition impairs the metabolic capacity of both fast and free muscles owing to AMP-activated protein kinase (AMPK)-independent inhibition of SIRT1 through increased oxidative stress (Hirabayashi et al. Citation2021).
Long-term moderate calorie restriction without undernutrition induces significant and sustained inhibition of inflammation without worsening key indicators of cellular immunity in vivo (Meydani et al. Citation2016).
Oxidative stress contributes to inflammation and malnutrition. Malnutrition can manifest as inflammation and increased oxidative stress; together, all these factors may lead to a decrease in SIRT1 expression. Decreased SIRT1 expression is associated with inflammation, oxidative stress, and malnutrition and is associated with the acetylation of NF-κB, an important redox-sensitive transcription factor responsible for the transcription of pro-inflammatory genes (Toledano and Leonard Citation1991). Inflammatory cytokines, such as interleukin-8 (IL-8), IL-6, and TNF-α are involved in the activation of immune cells and an inflammatory reaction. One of the main sources of these pro-inflammatory mediators are macrophages at the site of injury. In these cells, the transcription of these cytokines is regulated by NF-κB which undergoes various post-translational modifications, including phosphorylation and acetylation (Chen et al. Citation2005). Acetylation, together with phosphorylation, plays an important role in regulating the nuclear activity of NF-κB. Both p300 and CBP transferases have been reported to acetylate the RelA/p65 subunit NF-αB at Lys218, Lys221, and Lys310 (Chen, Mu, and Greene Citation2002). Acetylation of separate lysine residues in RelA/p65 modulates various functions of NF-κB, including transcription activation, DNA binding, and folding with its Iα inhibitor (Lanzillotta et al. Citation2010). After reduced NADH+, SIRT1 is degraded with simultaneous acetylation of NFKB and ReIA/p65 and the release of inflammatory mediators, which in turn may lead to malnutrition associated with inflammation and oxidative stress. The relationship between the factors that may stimulate reduced SIRT1 expression is shown in .
Figure 6. Possible factors that stimulate a decrease in SIRT1 expression in the body Degradation of SIRT1 by NAD + waste with simultaneous acetylation of NF-KB and ReIA/p65 and release of inflammatory mediators. Authors’ own study based on literature Abdelmohsen et al. (Citation2007).
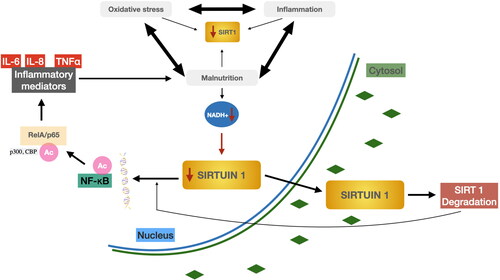
SIRT1 in frailty
Kumar et al. revealed that blood sirtuin levels are associated with geriatric fragility syndrome (Kumar et al. Citation2014). SIRT1, SIRT2, and SIRT3 were significantly lower in subjects with frailty syndrome than in those without, persisting after correction for independent factors such as number of concomitant diseases, sex, age, cognitive impairment, diabetes mellitus, and hypertension. In another study, circulating SIRT1 was higher in weak older adults, including those with weight loss (Ma et al. Citation2019).
While the evaluation of inflammatory biomarkers is useful in assessing the risk of undernutrition in relation to severe diseases in which they play a role, the association of SIRT1 with frailty is quite promising, as it may indicate significant sensitivity and specificity, without an ongoing inflammatory process in malnutrition.
SIRT1 as potential malnutrition biomarker
Many studies point to an association between DRM and inflammation, oxidative stress, and organ dysfunction, including endothelial dysfunction in patients with inflammatory DRM (Bañuls et al. Citation2019; Solmi et al. Citation2015; Victor et al. Citation2009). The role of oxidative stress regulation of SIRT1 in the potential inflammatory mechanism and aging has been described in the literature (Hwang et al. Citation2013), but the role of SIRT1 as a biomarker for malnutrition has not yet been described.
While calorie restriction without malnutrition promotes longevity and delays aging in the SIRT1 regulatory mechanism (Fontana, Partridge, and Longo Citation2010), the effects of prolonged calorie restriction and hunger on the severity of undernourishment are not well described.
In one study, SIRT1 levels were measured in three age groups (children, adults, and the elderly) to understand how SIRT1 levels change with age. The relationship between the polymorphisms of the single nucleotide SIRT1 (rs7 895 833 A > G in the promoter region, rs7 069 102 C > G in intron 4, and rs2 273 773 C > T in Exon 5—silent mutation) and the expression levels of SIRT1, endothelial nitric oxide synthase, and paraoxonase-1 were investigated. Paraoxonase 1 (PON-1), cholesterol levels, total antioxidant status (TAS), total oxidation status, and oxidation stress index (OSI) increased with increasing age in a Turkish population. A significant increase in SIRT1 levels was observed in elderly patients and a significant positive correlation between SIRT1 levels and age was observed in the overall study population. The oldest individuals with the AG genotype for rs7 895 833 had the highest SIRT1 value, suggesting a relationship between rs7 895 833 SNP and longevity. Elderly patients had a higher OSI and lower PON-1 levels than adults and children, which may lead to high SIRT1 levels as a compensatory mechanism for oxidative stress in the elderly. In addition, a decrease in low-density lipoprotein (LDL) levels has been observed in elderly patients and a positive correlation between high-density lipoprotein (HDL) cholesterol and SIRT1 levels has been observed in elderly patients (Kilic et al. Citation2015). The aging process itself contributes to the oxidative damage of DNA, which in turn leads to an increase in ROS, a higher rate of oxidative stress, and is an important factor in the occurrence of age-related diseases (Bohr Citation2002). Oxidative stress induces a reduction in NAD + by activation of PPAR, which in turn results in reduced expression of SIRT1. In vivo studies in rats have shown that this mechanism plays an important role in aging. In other studies, TAS was higher in elderly patients than in adults, but did not differ significantly from TAS levels in children. This may be related to the high SIRT1 levels in older people and the role of SIRT1 in the induction of antioxidants (Olmos et al. Citation2013).
Many studies have shown that the oxidative stress caused by TAS and total, LDL, and HDL cholesterol is associated with SIRT1 levels in the elderly. The correlation between cholesterol and SIRT1 levels may be explained by changes in hepatic metabolism associated not only with age, but also with malnutrition associated with metabolic disorders (Morena et al. Citation2000). Older people have lower HDL cholesterol and higher LDL cholesterol levels than younger people. Some studies have shown that higher LDL-C levels in elderly patients are associated with increased mortality mainly due to oxidative stress and inflammatory reactions (Benn, Tybjærg-Hansen, and Nordestgaard Citation2019; Yeboah Citation2018).
A Chinese study found an association between the LDL-C/HDL-C ratio and overall mortality in elderly patients with hypertension. Both low and high LDL-C/HDL-C ratios resulted in higher overall mortality, and patients with an LDL-C/HDL-C 1.67–2.10 had a significantly higher chance of survival (Yu et al. Citation2020).
El Assar et al. have shown that the improvement in nutritional status, as measured by MNA in older people, regardless of frailty syndrome or adherence to the Mediterranean diet, is positively associated with SIRT1 gene expression. The increased risk of malnutrition (based on MNA scores) was significantly associated with lower SIRT1 expression, confirming that older people with good nutrition have higher SIRT1 expression levels. These findings suggest that Sirtuin 1 is a potential biomarker for health interventions in the elderly (El Assar et al. Citation2018).
This work underlines the importance of sirtuins in the context of malnutrition in older people. Malnutrition, with or without inflammation or DRM caused by calorie restriction, may be associated with changes in SIRT1 expression in the cell nucleus. Calorie reduction has been shown to have a positive effect on longevity and aging and calorie restriction alone should not be equated with malnutrition (Michan Citation2014).
However, it should be noted that sirtuin activity is influenced by nutrient availability. SIRT1 levels increase during fasting or calorie reduction with negative energy balance (Rodgers and Puigserver Citation2007). Nutrient values (e.g., glucose, lipids, and amino acids) were measured with AMPK, SIRT1, or mTOR energy sensors (Escobar et al. Citation2019; Finkel Citation2015; Nemoto, Fergusson, and Finkel Citation2004). Malnutrition without disease may be associated with a short-term calorie reduction, which in turn activates AMPK or SIRT1 but inhibits mammalian target of rapamycin (mTOR), which is reduced in nutrient deficiency. Long-term calorie restriction and malnutrition with or without inflammation, decreases NADH+, which reduces the expression of SIRT1. Simultaneous deacetylation of STAT3 and FOX01 in hepatocytes, results in the transfer of SIRT1 from the nucleus to the cytosol and degradation of SIRT1. shows SIRT1 as a potential biomarker of nutritional status in the mechanism of decreasing and increasing activity in the cell nucleus.
Conclusions
The key to successful treatment of the elderly is early detection of undernutrition and its risk factors, and the earliest possible application of therapy. Animal studies point to potential molecular mechanisms regulating sirtuin 1 expression. In this review, we proposed the pathomechanism and pathway of sirtuin 1 activation and attempted to explain how malnutrition affects sirtuin 1 expression. Studies suggest that short-term fasting can increase SIRT1 expression, while long-term fasting can have the opposite effect. Some studies suggest a potential link between sirtuin 1 and nutritional status in older adults. However, these data, with the current state of knowledge are limited to a small number of human and animal studies, and therefore require further research to confirm the potential of sirtuin 1 as a biomarker of age-related undernutrition. When evaluating the usefulness of sirtuin 1 as a biomarker of undernutrition in humans, it is important to consider other medical conditions that may themselves cause changes in sirtuin 1 concentration and, on the other hand, chronic diseases may lead to malnutrition. Taking all these factors into account, further studies are desirable to confirm the role of sirtuin 1 in malnutrition and to investigate the predictive value, specificity and sensitivity of sirtuin 1 as a biomarker.
Author contributions
Writing–original draft preparation, K.K.; writing—review and editing, K.K. and A.W.
Availability of data and material
The data used to support the findings of this study are available from the corresponding author upon request.
Abbreviations | ||
AGE | = | Advanced glycation products |
AMPK | = | AMP-activated protein kinase |
BMI | = | Body Mass Index |
ChREBP | = | Carbohydrate response sequence |
CREB | = | AMP-activated factor |
DRM | = | Disease-related malnutrition |
FFA | = | Free fatty acids |
FOX0 | = | Forkhead box 0 |
FOX01 | = | Forkhead box 01 |
FOX03 | = | Forkhead box 03 |
GSH | = | Reduced glutathione |
GSH-Px | = | Glutathione peroxidase |
GSH-Rd | = | Glutathione reductase |
HDACs | = | Histone deacetylases |
HDL | = | High-density lipoprotein |
HFD | = | High-fat diet |
IL1 | = | Interleukin-1 |
IL6 | = | Interleukin-6 |
IL-8 | = | Interleukin-8 |
LDL | = | Low-density lipoprotein |
mTOR | = | Mammalian target of rapamycin |
MNA | = | Mini-Nutritional Assessment |
MnSOD | = | Manganese peroxide dismutase |
MUST | = | Malnutrition Universal Screening Tool |
NAD | = | Histone-nicotinamide adenine dinucleotide |
NAMPT | = | Nicotinamide phosphoribosyltransferase |
NF-αB | = | Nuclear factor αB |
NRS-2002 | = | Nutrition Risk Screening |
OSI | = | Oxidation stress index |
PGC-1α | = | Peroxisome proliferator-activated receptor gamma coactivator 1-alpha |
PON-1 | = | Paraoxonase 1 |
PPARα | = | Peroxisome proliferator-activated receptor alpha |
ROS | = | Reactive oxygen species |
SIR2 | = | Silent information regulator 2 |
SIRT1 | = | Sirtuin 1 |
SOD | = | Superoxide dismutase |
SGA | = | Subjective Global Assessment Short Form |
STAT3 | = | Signal transducers and activators of transcription 3 |
TAS | = | Total antioxidant status |
TNFα | = | Tumor necrosis factor-alpha |
Disclosure statement
The authors declare no conflict of interest.
Additional information
Funding
References
- Abdelmohsen, K., R. Pullmann, A. Lal, H. H. Kim, S. Galban, X. Yang, J. D. Blethrow, M. Walker, J. Shubert, D. A. Gillespie, et al. 2007. Phosphorylation of HuR by Chk2 regulates SIRT1 expression. Molecular Cell 25 (4):543–57. doi: 10.1016/j.molcel.2007.01.011.
- Alcaín, F. J., R. K. Minor, J. M. Villalba, and R. de Cabo. 2010. Small molecule modulators of sirtuin activity. In The future of aging: pathways to human life extension, ed. G. M. Fahy, M. D. West, L. S. Coles, and S. B. Harris, 331–56. Dordrecht: Springer Netherlands.
- Alcendor, R. R., S. Gao, P. Zhai, D. Zablocki, E. Holle, X. Yu, B. Tian, T. Wagner, S. F. Vatner, and J. Sadoshima. 2007. Sirt1 regulates aging and resistance to oxidative stress in the heart. Circulation Research 100 (10):1512–21. doi: 10.1161/01.RES.0000267723.65696.4a.
- Allard, J. P., H. Keller, L. Gramlich, K. N. Jeejeebhoy, M. Laporte, and D. R. Duerksen. 2020. GLIM criteria has fair sensitivity and specificity for diagnosing malnutrition when using SGA as comparator. Clinical Nutrition (Edinburgh, Scotland) 39 (9):2771–77. doi: 10.1016/j.clnu.2019.12.004.
- Amirchaghmaghi, M., Z. Delavarian, M. Iranshahi, M. T. Shakeri, P. Mosannen Mozafari, A. H. Mohammadpour, F. Farazi, and M. Iranshahy. 2015. A randomized placebo-controlled double blind clinical trial of Quercetin for treatment of oral lichen planus. Journal of Dental Research, Dental Clinics, Dental Prospects 9 (1):23–8. doi: 10.15171/joddd.2015.005.
- An, F., S. Wang, Q. Tian, and D. Zhu. 2015. Effects of orientin and vitexin from Trollius chinensis on the growth and apoptosis of esophageal cancer EC-109 cells. Oncology Letters 10 (4):2627–33. doi: 10.3892/ol.2015.3618.
- Asghari, S., M. Asghari-Jafarabadi, M. H. Somi, S. M. Ghavami, and M. Rafraf. 2018. Comparison of calorie-restricted diet and resveratrol supplementation on anthropometric indices, metabolic parameters, and serum sirtuin-1 levels in patients with nonalcoholic fatty liver disease: A randomized controlled clinical trial. Journal of the American College of Nutrition 37 (3):223–33. doi: 10.1080/07315724.2017.1392264.
- Asher, G., and U. Schibler. 2011. Crosstalk between components of circadian and metabolic cycles in mammals. Cell Metabolism 13 (2):125–37. doi: 10.1016/j.cmet.2011.01.006.
- Ashikawa, K., S. Majumdar, S. Banerjee, A. C. Bharti, S. Shishodia, and B. B. Aggarwal. 2002. Piceatannol inhibits TNF-induced NF-kappaB activation and NF-kappaB-mediated gene expression through suppression of IkappaBalpha kinase and p65 phosphorylation. Journal of Immunology 169 (11):6490–97. doi: 10.4049/jimmunol.169.11.6490.
- Bae, J. S. 2015. Inhibitory effect of orientin on secretory group IIA phospholipase A2. Inflammation 38 (4):1631–8. doi: 10.1007/s10753-015-0139-8.
- Bagul, P. K., A. K. Dinda, and S. K. Banerjee. 2015. Effect of resveratrol on sirtuins expression and cardiac complications in diabetes. Biochemical and Biophysical Research Communications 468 (1–2):221–227. doi: 10.1016/j.bbrc.2015.10.126.
- Bartosch, C., S. Monteiro-Reis, D. Almeida-Rios, R. Vieira, A. Castro, M. Moutinho, M. Rodrigues, I. Graça, J. M. Lopes, and C. Jerónimo. 2016. Assessing sirtuin expression in endometrial carcinoma and non-neoplastic endometrium. Oncotarget 7 (2):1144–54. doi: 10.18632/oncotarget.6691.
- Bañuls, C., A. M. de Marañon, S. Veses, I. Castro-Vega, S. López-Domènech, C. Salom-Vendrell, S. Orden, Á. Álvarez, M. Rocha, V. M. Víctor, et al. 2019. Malnutrition impairs mitochondrial function and leukocyte activation. Nutrition Journal 18 (1):89. doi: 10.1186/s12937-019-0514-7.
- Benn, M., A. Tybjærg-Hansen, and B. G. Nordestgaard. 2019. Low LDL Cholesterol by PCSK9 Variation Reduces Cardiovascular Mortality. Journal of the American College of Cardiology 73 (24):3102–14. doi: 10.1016/j.jacc.2019.03.517.
- Bhardwaj, M., S. Paul, R. Jakhar, and S. C. Kang. 2015. Potential role of vitexin in alleviating heat stress-induced cytotoxicity: Regulatory effect of Hsp90 on ER stress-mediated autophagy. Life Sciences 142:36–48. doi: 10.1016/j.lfs.2015.10.012.
- Ble, A., A. Cherubini, S. Volpato, B. Bartali, J. D. Walston, B. G. Windham, S. Bandinelli, F. Lauretani, J. M. Guralnik, and L. Ferrucci. 2006. Lower plasma vitamin E levels are associated with the frailty syndrome: The InCHIANTI study. The Journals of Gerontology. Series A, Biological Sciences and Medical Sciences 61 (3):278–83. doi: 10.1093/gerona/61.3.278.
- Bleeker, J. C., and R. H. Houtkooper. 2016. Sirtuin activation as a therapeutic approach against inborn errors of metabolism. Journal of Inherited Metabolic Disease 39 (4):565–72. doi: 10.1007/s10545-016-9939-8.
- Bogacka, I., T. W. Gettys, L. de Jonge, T. Nguyen, J. M. Smith, H. Xie, F. Greenway, and S. R. Smith. 2007. The effect of beta-adrenergic and peroxisome proliferator-activated receptor-gamma stimulation on target genes related to lipid metabolism in human subcutaneous adipose tissue. Diabetes Care 30 (5):1179–86. doi: 10.2337/dc06-1962.
- Bohr, V. A. 2002. Repair of oxidative DNA damage in nuclear and mitochondrial DNA, and some changes with aging in mammalian cells. Free Radical Biology & Medicine 32 (9):804–12. doi: 10.1016/s0891-5849(02)00787-6.
- Bonda, D. J., H. G. Lee, A. Camins, M. Pallàs, G. Casadesus, M. A. Smith, and X. Zhu. 2011. The sirtuin pathway in ageing and Alzheimer disease: Mechanistic and therapeutic considerations. The Lancet. Neurology 10 (3):275–9. doi: 10.1016/s1474-4422(11)70013-8.
- Bonkowski, M. S., and D. A. Sinclair. 2016. Slowing ageing by design: The rise of NAD+ and sirtuin-activating compounds. Nature Reviews. Molecular Cell Biology 17 (11):679–90. doi: 10.1038/nrm.2016.93.
- Boominathan, S., G. Sarangan, S. Srikakulapu, S. Rajesh, C. Duraipandian, P. Srikanth, and L. Jo. 2014. Antiviral activity of bioassay guided fractionation of Plumbago zeylanica roots against herpes simplex virus type 2. World Journal of Pharmacy Pharmaceutical Sciences, 3 (12):1003–17.
- Bordone, L., M. C. Motta, F. Picard, A. Robinson, U. S. Jhala, J. Apfeld, T. McDonagh, M. Lemieux, M. McBurney, A. Szilvasi, et al. 2006. Sirt1 regulates insulin secretion by repressing UCP2 in pancreatic beta cells. PLoS Biology 4 (2):e31. doi: 10.1371/journal.pbio.0040031.
- Borghi, S. M., T. T. Carvalho, L. Staurengo-Ferrari, M. S. Hohmann, P. Pinge-Filho, R. Casagrande, and W. A. Verri. Jr 2013. Vitexin inhibits inflammatory pain in mice by targeting TRPV1, oxidative stress, and cytokines. Journal of Natural Products 76 (6):1141–9. doi: 10.1021/np400222v.
- Bourke, C. D., J. A. Berkley, and A. J. Prendergast. 2016. Immune dysfunction as a cause and consequence of malnutrition. Trends in Immunology 37 (6):386–98. doi: 10.1016/j.it.2016.04.003.
- Brocca, L., J. Cannavino, L. Coletto, G. Biolo, M. Sandri, R. Bottinelli, and M. A. Pellegrino. 2012. The time course of the adaptations of human muscle proteome to bed rest and the underlying mechanisms. The Journal of Physiology 590 (20):5211–30. doi: 10.1113/jphysiol.2012.240267.
- Bruckbauer, A., and M. B. Zemel. 2011. Effects of dairy consumption on SIRT1 and mitochondrial biogenesis in adipocytes and muscle cells. Nutrition & Metabolism 8:91. doi: 10.1186/1743-7075-8-91.
- Budui, S. L., A. P. Rossi, and M. Zamboni. 2015. The pathogenetic bases of sarcopenia. Clinical Cases in Mineral and Bone Metabolism 12 (1):22–6. doi: 10.11138/ccmbm/2015.12.1.022.
- Burns, A., C. Brayne, and M. Folstein. 1998. Key papers in geriatric psychiatry: mini-mental state: A practical method for grading the cognitive state of patients for the clinician. M. Folstein, S. Folstein and P. McHugh. 1975. Journal of Psychiatric Research 12 189–198. International Journal of Geriatric Psychiatry 13 (5):285–94. doi: 10.1002/(SICI)1099-1166(199805)13:5 < 285::AID-GPS753 > 3.0.CO;2-V.
- Bódis, J., E. Sulyok, T. Kőszegi, K. Gödöny, V. Prémusz, and Á. Várnagy. 2019. Serum and follicular fluid levels of sirtuin 1, sirtuin 6, and resveratrol in women undergoing in vitro fertilization: An observational, clinical study. The Journal of International Medical Research 47 (2):772–82. doi: 10.1177/0300060518811228.
- Calgarotto, A. K., V. Maso, G. C. F. Junior, A. E. Nowill, P. L. Filho, J. Vassallo, and S. T. O. Saad. 2018. Antitumor activities of Quercetin and Green Tea in xenografts of human leukemia HL60 cells. Scientific Reports 8 (1):3459. doi: 10.1038/s41598-018-21516-5.
- Cantó, C., K. J. Menzies, and J. Auwerx. 2015. NAD+ metabolism and the control of energy homeostasis: A balancing act between mitochondria and the nucleus. Cell Metabolism 22 (1):31–53. doi: 10.1016/j.cmet.2015.05.023.
- Cea, M., D. Soncini, F. Fruscione, L. Raffaghello, A. Garuti, L. Emionite, E. Moran, M. Magnone, G. Zoppoli, D. Reverberi, et al. 2011. Synergistic interactions between HDAC and sirtuin inhibitors in human leukemia cells. PloS One 6 (7):e22739. doi: 10.1371/journal.pone.0022739.
- Cederholm, T., R. Barazzoni, P. Austin, P. Ballmer, G. Biolo, S. C. Bischoff, C. Compher, I. Correia, T. Higashiguchi, M. Holst, et al. 2017. ESPEN guidelines on definitions and terminology of clinical nutrition. Clinical Nutrition (Edinburgh, Scotland) 36 (1):49–64. doi: 10.1016/j.clnu.2016.09.004.
- Cederholm, T., I. Bosaeus, R. Barazzoni, J. Bauer, A. Van Gossum, S. Klek, M. Muscaritoli, I. Nyulasi, J. Ockenga, S. M. Schneider, et al. 2015. Diagnostic criteria for malnutrition – An ESPEN Consensus Statement. Clinical Nutrition (Edinburgh, Scotland) 34 (3):335–340. doi: 10.1016/j.clnu.2015.03.001.
- Cederholm, T., G. L. Jensen, M. I. T. D. Correia, M. C. Gonzalez, R. Fukushima, T. Higashiguchi, G. Baptista, R. Barazzoni, R. Blaauw, A. Coats, GLIM Working Group, et al. 2019. GLIM criteria for the diagnosis of malnutrition – A consensus report from the global clinical nutrition community. Clinical Nutrition (Edinburgh, Scotland) 38 (1):1–9. doi: 10.1016/j.clnu.2018.08.002.
- Cereda, E., C. Pedrolli, C. Klersy, C. Bonardi, L. Quarleri, S. Cappello, A. Turri, M. Rondanelli, and R. Caccialanza. 2016. Nutritional status in older persons according to healthcare setting: A systematic review and meta-analysis of prevalence data using MNA®. Clinical Nutrition (Edinburgh, Scotland) 35 (6):1282–90. doi: 10.1016/j.clnu.2016.03.008.
- Chahinian, A. P., J. P. Mandeli, H. Gluck, H. Naim, A. S. Teirstein, and J. F. Holland. 1998. Effectiveness of cisplatin, paclitaxel, and suramin against human malignant mesothelioma xenografts in athymic nude mice. Journal of Surgical Oncology 67 (2):104–11. doi: 10.1002/(sici)1096-9098(199802)67:2 < 104::aid-jso6 > 3.0.co;2-e.
- Chalkiadaki, A., and L. Guarente. 2015. The multifaceted functions of sirtuins in cancer. Nature Reviews. Cancer 15 (10):608–24. doi: 10.1038/nrc3985.
- Chandramowlishwaran, P., A. Vijay, D. Abraham, G. Li, S. M. Mwangi, and S. Srinivasan. 2020. Role of sirtuins in modulating neurodegeneration of the enteric nervous system and central nervous system. Frontiers in Neuroscience 14:614331. doi: 10.3389/fnins.2020.614331.
- Chang, B., M.-J. Xu, Z. Zhou, Y. Cai, M. Li, W. Wang, D. Feng, A. Bertola, H. Wang, G. Kunos, et al. 2015. Short‐or long‐term high‐fat diet feeding plus acute ethanol binge synergistically induce acute liver injury in mice: An important role for CXCL1. Hepatology (Baltimore, Md.) 62 (4):1070–85. doi: 10.1002/hep.27921.
- Chen, D., J. Bruno, E. Easlon, S. J. Lin, H. L. Cheng, F. W. Alt, and L. Guarente. 2008. Tissue-specific regulation of SIRT1 by calorie restriction. Genes & Development 22 (13):1753–57. doi: 10.1101/gad.1650608.
- Chen, L. F., Y. Mu, and W. C. Greene. 2002. Acetylation of RelA at discrete sites regulates distinct nuclear functions of NF-kappaB. The EMBO Journal 21 (23):6539–48. doi: 10.1093/emboj/cdf660.
- Chen, L. F., S. A. Williams, Y. Mu, H. Nakano, J. M. Duerr, L. Buckbinder, and W. C. Greene. 2005. NF-kappaB RelA phosphorylation regulates RelA acetylation. Molecular and Cellular Biology 25 (18):7966–75. doi: 10.1128/mcb.25.18.7966-7975.2005.
- Cheng, H.-L., R. Mostoslavsky, S. Saito, J. P. Manis, Y. Gu, P. Patel, R. Bronson, E. Appella, F. W. Alt, and K. F. Chua. 2003. Developmental defects and p53 hyperacetylation in Sir2 homolog (SIRT1)-deficient mice. Proceedings of the National Academy of Sciences of the United States of America 100 (19):10794–99. doi: 10.1073/pnas.1934713100.
- Cholewa, J. M., D. Dardevet, F. Lima-Soares, K. de Araújo Pessôa, P. H. Oliveira, J. R. Dos Santos Pinho, H. Nicastro, Z. Xia, C. E. T. Cabido, and N. E. Zanchi. 2017. Dietary proteins and amino acids in the control of the muscle mass during immobilization and aging: Role of the MPS response. Amino Acids 49 (5):811–20. doi: 10.1007/s00726-017-2390-9.
- Choo, C. Y., N. Y. Sulong, F. Man, and T. W. Wong. 2012. Vitexin and isovitexin from the Leaves of Ficus deltoidea with in-vivo α-glucosidase inhibition. Journal of Ethnopharmacology 142 (3):776–81. doi: 10.1016/j.jep.2012.05.062.
- Chua, K. F., R. Mostoslavsky, D. B. Lombard, W. W. Pang, S. Saito, S. Franco, D. Kaushal, H.-L. Cheng, M. R. Fischer, N. Stokes, et al. 2005. Mammalian SIRT1 limits replicative life span in response to chronic genotoxic stress. Cell Metabolism 2 (1):67–76. doi: 10.1016/j.cmet.2005.06.007.
- Chung, S., H. Yao, S. Caito, J. W. Hwang, G. Arunachalam, and I. Rahman. 2010. Regulation of SIRT1 in cellular functions: Role of polyphenols. Archives of Biochemistry and Biophysics 501 (1):79–90. doi: 10.1016/j.abb.2010.05.003.
- Clegg, A., J. Young, S. Iliffe, M. O. Rikkert, and K. Rockwood. 2013. Frailty in elderly people. Lancet (London, England) 381 (9868):752–62. doi: 10.1016/s0140-6736(12)62167-9.
- Crispi, F., J. Miranda, and E. Gratacós. 2018. Long-term cardiovascular consequences of fetal growth restriction: Biology, clinical implications, and opportunities for prevention of adult disease. American Journal of Obstetrics and Gynecology 218 (2s):S869–S879. doi: 10.1016/j.ajog.2017.12.012.
- Crossland, H., D. Constantin-Teodosiu, S. M. Gardiner, D. Constantin, and P. L. Greenhaff. 2008. A potential role for Akt/FOXO signalling in both protein loss and the impairment of muscle carbohydrate oxidation during sepsis in rodent skeletal muscle. The Journal of Physiology 586 (22):5589–600. doi: 10.1113/jphysiol.2008.160150.
- Crossland, H., D. Constantin-Teodosiu, P. L. Greenhaff, and S. M. Gardiner. 2010. Low-dose dexamethasone prevents endotoxaemia-induced muscle protein loss and impairment of carbohydrate oxidation in rat skeletal muscle. The Journal of Physiology 588 (Pt 8):1333–47. doi: 10.1113/jphysiol.2009.183699.
- Currais, A., M. Prior, R. Dargusch, A. Armando, J. Ehren, D. Schubert, O. Quehenberger, and P. Maher. 2014. Modulation of p25 and inflammatory pathways by fisetin maintains cognitive function in Alzheimer’s disease transgenic mice. Aging Cell 13 (2):379–90. doi: 10.1111/acel.12185.
- Das, U. N. 2021. Cell membrane theory of senescence and the role of bioactive lipids in aging, and aging associated diseases and their therapeutic implications. Biomolecules 11 (2):241. doi: 10.3390/biom11020241.
- Davis, J. M., E. A. Murphy, M. D. Carmichael, and B. Davis. 2009. Quercetin increases brain and muscle mitochondrial biogenesis and exercise tolerance. American Journal of Physiology. Regulatory, Integrative and Comparative Physiology 296 (4):R1071–R11077. doi: 10.1152/ajpregu.90925.2008.
- de Picciotto, N. E., L. B. Gano, L. C. Johnson, C. R. Martens, A. L. Sindler, K. F. Mills, S.-I. Imai, and D. R. Seals. 2016. Nicotinamide mononucleotide supplementation reverses vascular dysfunction and oxidative stress with aging in mice. Aging Cell 15 (3):522–530. doi: 10.1111/acel.12461.
- Donini, L. M., E. Poggiogalle, A. Molfino, A. Rosano, A. Lenzi, F. Rossi Fanelli, and M. Muscaritoli. 2016. Mini-nutritional assessment, malnutrition universal screening tool, and nutrition risk screening tool for the nutritional evaluation of older nursing home residents. Journal of the American Medical Directors Association 17 (10):959.e911–959.e918. doi: 10.1016/j.jamda.2016.06.028.
- Donmez, G., and T. F. Outeiro. 2013. SIRT1 and SIRT2: Emerging targets in neurodegeneration. EMBO Molecular Medicine 5 (3):344–52. doi: 10.1002/emmm.201302451.
- Du, J., Y. Zhou, X. Su, J. J. Yu, S. Khan, H. Jiang, J. Kim, J. Woo, J. H. Kim, B. H. Choi, et al. 2011. Sirt5 is a NAD-dependent protein lysine demalonylase and desuccinylase. Science (New York, N.Y.) 334 (6057):806–9. doi: 10.1126/science.1207861.
- Duke, J., and M. J. Bogenschutz. 1994. Dr. Duke’s phytochemical and ethnobotanical databases: Washington, DC: USDA, Agricultural Research Service.
- Dykes, L., and L. W. Rooney. 2006. Sorghum and millet phenols and antioxidants. Journal of Cereal Science 44 (3):236–51. doi: 10.1016/j.jcs.2006.06.007.
- Dülger, H., M. Arik, M. R. Sekeroğlu, M. Tarakçioğlu, T. Noyan, Y. Cesur, and R. Balahoroğlu. 2002. Pro-inflammatory cytokines in Turkish children with protein-energy malnutrition. Mediators of Inflammation 11 (6):363–35. doi: 10.1080/0962935021000051566.
- El Assar, M., J. Angulo, S. Walter, J. A. Carnicero, F. J. García-García, J.-M. Sánchez-Puelles, C. Sánchez-Puelles, and L. Rodríguez-Mañas. 2018. Better nutritional status is positively associated with mRNA expression of SIRT1 in community-dwelling older adults in the Toledo Study for Healthy Aging. The Journal of Nutrition 148 (9):1408–14. doi: 10.1093/jn/nxy149.
- Elliott, P. J., and M. Jirousek. 2008. Sirtuins: Novel targets for metabolic disease. Curr Opin Investig Drugs 9 (4):371–8.
- Escobar, K. A., N. H. Cole, C. M. Mermier, and T. A. VanDusseldorp. 2019. Autophagy and aging: Maintaining the proteome through exercise and caloric restriction. Aging Cell 18 (1):e12876. doi: 10.1111/acel.12876.
- Fechner, A., C. C. Böhme, S. Gromer, M. Funk, R. H. Schirmer, and K. Becker. 2001. Antioxidant Status and Nitric Oxide in the Malnutrition Syndrome Kwashiorkor. Pediatric Research 49 (2):237–43. doi: 10.1203/00006450-200102000-00018.
- Ferreira, A., C. Renatacorrea, C. Freire, P. Moreira, C. Berchieri-Ronchi, R. Reis, and C. Nogueira. 2011. Metabolic syndrome: Updated diagnostic criteria and impact of oxidative stress on metabolic syndrome pathogenesis. International Journal of Molecular Sciences 23 (2):786
- Ferry, D. R., A. Smith, J. Malkhandi, D. W. Fyfe, P. G. deTakats, D. Anderson, J. Baker, and D. J. Kerr. 1996. Phase I clinical trial of the flavonoid quercetin: Pharmacokinetics and evidence for in vivo tyrosine kinase inhibition. Clin Cancer Res 2 (4):659–68.
- Finkel, T. 2015. The metabolic regulation of aging. Nature Medicine 21 (12):1416–23. doi: 10.1038/nm.3998.
- Finkel, T., C. X. Deng, and R. Mostoslavsky. 2009. Recent progress in the biology and physiology of sirtuins. Nature 460 (7255):587–91. doi: 10.1038/nature08197.
- Flores, G., K. Dastmalchi, A. J. Dabo, K. Whalen, P. Pedraza-Peñalosa, R. F. Foronjy, J. M. D’Armiento, and E. J. Kennelly. 2012. Antioxidants of therapeutic relevance in COPD from the neotropical blueberry Anthopterus wardii. Food Chemistry 131 (1):119–25. doi: 10.1016/j.foodchem.2011.08.044.
- Fontana, L., L. Partridge, and V. D. Longo. 2010. Extending healthy life span–from yeast to humans. Science (New York, N.Y.) 328 (5976):321–6. doi: 10.1126/science.1172539.
- Furukawa, A., S. Tada-Oikawa, S. Kawanishi, and S. Oikawa. 2007. H2O2 accelerates cellular senescence by accumulation of acetylated p53 via decrease in the function of SIRT1 by NAD+ depletion. Cellular Physiology and Biochemistry 20 (1–4):45–54. doi: 10.1159/000104152.
- Galleano, M., V. Calabro, P. D. Prince, M. C. Litterio, B. Piotrkowski, M. A. Vazquez-Prieto, R. M. Miatello, P. I. Oteiza, and C. G. Fraga. 2012. Flavonoids and metabolic syndrome. Annals of the New York Academy of Sciences 1259:87–94. doi: 10.1111/j.1749-6632.2012.06511.x.
- Gao, J., Z. Feng, X. Wang, M. Zeng, J. Liu, S. Han, J. Xu, L. Chen, K. Cao, J. Long, et al. 2018. SIRT3/SOD2 maintains osteoblast differentiation and bone formation by regulating mitochondrial stress. Cell Death and Differentiation 25 (2):229–40. doi: 10.1038/cdd.2017.144.
- Gertz, M., G. T. T. Nguyen, F. Fischer, B. Suenkel, C. Schlicker, B. Fränzel, J. Tomaschewski, F. Aladini, C. Becker, D. Wolters, et al. 2012. A molecular mechanism for direct sirtuin activation by resveratrol. PloS One 7 (11):e49761. doi: 10.1371/journal.pone.0049761.
- Godoy, I. D., M. Donahoe, W. J. Calhoun, J. Mancino, and R. M. Rogers. 1996. Elevated TNF-alpha production by peripheral blood monocytes of weight-losing COPD patients. American Journal of Respiratory and Critical Care Medicine 153 (2):633–7. doi: 10.1164/ajrccm.153.2.8564110.
- Gregoretti, I. V., Y. M. Lee, and H. V. Goodson. 2004. Molecular evolution of the histone deacetylase family: Functional implications of phylogenetic analysis. Journal of Molecular Biology 338 (1):17–31. doi: 10.1016/j.jmb.2004.02.006.
- Grozinger, C. M., E. D. Chao, H. E. Blackwell, D. Moazed, and S. L. Schreiber. 2001. Identification of a class of small molecule inhibitors of the sirtuin family of NAD-dependent deacetylases by phenotypic screening. The Journal of Biological Chemistry 276 (42):38837–43. doi: 10.1074/jbc.M106779200.
- Haigis, M. C., and D. A. Sinclair. 2010. Mammalian sirtuins: Biological insights and disease relevance. Annual Review of Pathology 5:253–95. doi: 10.1146/annurev.pathol.4.110807.092250.
- Halliwell, B., and J. M. Gutteridge. 2015. Free radicals in biology and medicine: Oxford: Oxford University Press.
- Hallows, W. C., W. Yu, and J. M. Denu. 2012. Regulation of glycolytic enzyme phosphoglycerate mutase-1 by Sirt1 protein-mediated deacetylation. The Journal of Biological Chemistry 287 (6):3850–8. doi: 10.1074/jbc.M111.317404.
- Hamdy, A. A., and M. A. Ibrahem. 2010. Management of aphthous ulceration with topical quercetin: A randomized clinical trial. The Journal of Contemporary Dental Practice 11 (4):9–16. doi: 10.5005/jcdp-11-4-9.
- Haohao, Z., Q. Guijun, Z. Juan, K. Wen, and C. Lulu. 2015. Resveratrol improves high-fat diet induced insulin resistance by rebalancing subsarcolemmal mitochondrial oxidation and antioxidantion. Journal of Physiology and Biochemistry 71 (1):121–31. doi: 10.1007/s13105-015-0392-1.
- Harman, D. 1956. Aging: A theory based on free radical and radiation chemistry. Journal of Gerontology 11 (3):298–300. doi: 10.1093/geronj/11.3.298.
- Harris, M. B., R. M. Hoffman, and M. Olesiak. 2021. Chronic exercise mitigates the effects of sirtuin inhibition by salermide on endothelium-dependent vasodilation. Cardiovascular Toxicology 21 (10):790–9. doi: 10.1007/s12012-021-09669-8.
- He, M., J. W. Min, W. L. Kong, X. H. He, J. X. Li, and B. W. Peng. 2016. A review on the pharmacological effects of vitexin and isovitexin. Fitoterapia 115:74–85. doi: 10.1016/j.fitote.2016.09.011.
- Heijmans, B. T., E. W. Tobi, A. D. Stein, H. Putter, G. J. Blauw, E. S. Susser, P. E. Slagboom, and L. H. Lumey. 2008. Persistent epigenetic differences associated with prenatal exposure to famine in humans. Proceedings of the National Academy of Sciences of the United States of America 105 (44):17046–9. doi: 10.1073/pnas.0806560105.
- Heinz, S. A., D. A. Henson, M. D. Austin, F. Jin, and D. C. Nieman. 2010. Quercetin supplementation and upper respiratory tract infection: A randomized community clinical trial. Pharmacological Research 62 (3):237–42. doi: 10.1016/j.phrs.2010.05.001.
- Herranz, D., M. Muñoz-Martin, M. Cañamero, F. Mulero, B. Martinez-Pastor, O. Fernandez-Capetillo, and M. Serrano. 2010. Sirt1 improves healthy ageing and protects from metabolic syndrome-associated cancer. Nature Communications 1:3. doi: 10.1038/ncomms1001.
- Hickson, M. 2006. Malnutrition and ageing. Postgraduate Medical Journal 82 (963):2–8. doi: 10.1136/pgmj.2005.037564.
- Hirabayashi, T., R. Nakanishi, M. Tanaka, B. U. Nisa, N. Maeshige, H. Kondo, and H. Fujino. 2021. Reduced metabolic capacity in fast and slow skeletal muscle via oxidative stress and the energy-sensing of AMPK/SIRT1 in malnutrition. Physiological Reports 9 (5):e14763. doi: 10.14814/phy2.14763.
- Hirani, V., V. Naganathan, R. G. Cumming, F. Blyth, D. G. Le Couteur, D. J. Handelsman, L. M. Waite, and M. J. Seibel. 2013. Associations between frailty and serum 25-hydroxyvitamin D and 1,25-dihydroxyvitamin D concentrations in older Australian men: The Concord Health and Ageing in Men Project. The Journals of Gerontology. Series A, Biological Sciences and Medical Sciences 68 (9):1112–21. doi: 10.1093/gerona/glt059.
- Hirao, M., J. Posakony, M. Nelson, H. Hruby, M. Jung, J. A. Simon, and A. Bedalov. 2003. Identification of selective inhibitors of NAD+-dependent deacetylases using phenotypic screens in yeast. The Journal of Biological Chemistry 278 (52):52773–82. doi: 10.1074/jbc.M308966200.
- Horton, J. D., J. L. Goldstein, and M. S. Brown. 2002. SREBPs: Activators of the complete program of cholesterol and fatty acid synthesis in the liver. The Journal of Clinical Investigation 109 (9):1125–31. doi: 10.1172/jci15593.
- Hubbard, B. P., and D. A. Sinclair. 2014. Small molecule SIRT1 activators for the treatment of aging and age-related diseases. Trends in Pharmacological Sciences 35 (3):146–54. doi: 10.1016/j.tips.2013.12.004.
- Hwang, J. W., H. Yao, S. Caito, I. K. Sundar, and I. Rahman. 2013. Redox regulation of SIRT1 in inflammation and cellular senescence. Free Radical Biology & Medicine 61:95–110. doi: 10.1016/j.freeradbiomed.2013.03.015.
- Ishibashi, Y., M. Ito, and Y. Hirabayashi. 2020. The sirtuin inhibitor cambinol reduces intracellular glucosylceramide with ceramide accumulation by inhibiting glucosylceramide synthase. Bioscience, Biotechnology, and Biochemistry 84 (11):2264–72. doi: 10.1080/09168451.2020.1794785.
- Jang, M., L. Cai, G. O. Udeani, K. V. Slowing, C. F. Thomas, C. W. Beecher, H. H. Fong, N. R. Farnsworth, A. D. Kinghorn, R. G. Mehta, et al. 1997. Cancer chemopreventive activity of resveratrol, a natural product derived from grapes. Science (New York, N.Y.) 275 (5297):218–20. doi: 10.1126/science.275.5297.218.
- Jensen, G. L. 2015. Malnutrition and inflammation-“burning down the house”: Inflammation as an adaptive physiologic response versus self-destruction? Journal of Parenteral and Enteral Nutrition 39 (1):56–62. doi: 10.1177/0148607114529597.
- Jensen, G. L., T. Cederholm, M. I. T. D. Correia, M. C. Gonzalez, R. Fukushima, T. Higashiguchi, G. A. de Baptista, R. Barazzoni, R. Blaauw, A. J. S. Coats, et al. 2019. GLIM criteria for the diagnosis of malnutrition: A consensus report from the Global Clinical Nutrition Community. Journal of Parenteral and Enteral Nutrition 43 (1):32–40. doi: 10.1002/jpen.1440.
- Jensen, G. L., J. Mirtallo, C. Compher, R. Dhaliwal, A. Forbes, R. F. Grijalba, G. Hardy, J. Kondrup, D. Labadarios, I. Nyulasi, International Consensus Guideline Committee, et al. 2010. Adult starvation and disease-related malnutrition: A proposal for etiology-based diagnosis in the clinical practice setting from the International Consensus Guideline Committee. Journal of Parenteral and Enteral Nutrition 34 (2):156–9. doi: 10.1177/0148607110361910.
- Kaiser, M. J., J. M. Bauer, C. Rämsch, W. Uter, Y. Guigoz, T. Cederholm, D. R. Thomas, P. S. Anthony, K. E. Charlton, M. Maggio, Mini Nutritional Assessment International Group, et al. 2010. Frequency of malnutrition in older adults: A multinational perspective using the mini nutritional assessment. Journal of the American Geriatrics Society 58 (9):1734–8. doi: 10.1111/j.1532-5415.2010.03016.x.
- Kemelo, M. K., A. Horinek, N. K. Canová, and H. Farghali. 2016. Comparative effects of Quercetin and SRT1720 against D-galactosamine/lipopolysaccharide-induced hepatotoxicity in rats: Biochemical and molecular biological investigations. European Review for Medical and Pharmacological Sciences. 20 (2):363–71.
- Kershaw, J., and K. H. Kim. 2017. The therapeutic potential of piceatannol, a natural stilbene, in metabolic diseases: A review. Journal of Medicinal Food 20 (5):427–38. doi: 10.1089/jmf.2017.3916.
- Keusch, G. T. 2003. The history of nutrition: Malnutrition, infection and immunity. The Journal of Nutrition 133 (1):336s–40s. doi: 10.1093/jn/133.1.336S.
- Khan, N., D. N. Syed, N. Ahmad, and H. Mukhtar. 2013. Fisetin: A dietary antioxidant for health promotion. Antioxidants & Redox Signaling 19 (2):151–62. doi: 10.1089/ars.2012.4901.
- Khanra, S., S. K. Juin, J. J. Jawed, S. Ghosh, S. Dutta, S. A. Nabi, J. Dash, D. Dasgupta, S. Majumdar, and R. Banerjee. 2020. In vivo experiments demonstrate the potent antileishmanial efficacy of repurposed suramin in visceral leishmaniasis. PLoS Neglected Tropical Diseases 14 (8):e0008575. doi: 10.1371/journal.pntd.0008575.
- Khazaei, M. R., M. H. Nasr-Esfahani, F. Chobsaz, and M. Khazaei. 2019. Noscapine inhibiting the growth and angiogenesis of human eutopic endometrium of endometriosis patients through expression of apoptotic genes and nitric oxide reduction in three-dimensional culture model. Iranian Journal of Pharmaceutical Research 18 (2):836–45. doi: 10.22037/ijpr.2019.1100642.
- Khoruts, A. 2018. Targeting the microbiome: From probiotics to fecal microbiota transplantation. Genome Medicine 10 (1):80. doi: 10.1186/s13073-018-0592-8.
- Kilic, U., O. Gok, U. Erenberk, M. R. Dundaroz, E. Torun, Y. Kucukardali, B. Elibol-Can, O. Uysal, and T. Dundar. 2015. A remarkable age-related increase in SIRT1 protein expression against oxidative stress in elderly: SIRT1 gene variants and longevity in human. PloS One 10 (3):e0117954. doi: 10.1371/journal.pone.0117954.
- Kim, J. H., B. C. Lee, J. H. Kim, G. S. Sim, D. H. Lee, K. E. Lee, Y. P. Yun, and H. B. Pyo. 2005. The isolation and antioxidative effects of vitexin from Acer palmatum. Archives of Pharmacal Research 28 (2):195–202. doi: 10.1007/bf02977715.
- Kim, S., P. A. Thiessen, E. E. Bolton, J. Chen, G. Fu, A. Gindulyte, L. Han, J. He, S. He, B. A. Shoemaker, et al. 2016. PubChem Substance and Compound databases. Nucleic Acids Research 44 (D1):D1202–1213. doi: 10.1093/nar/gkv951.
- Kim, S.-J., I. S. M. Zaidul, T. Suzuki, Y. Mukasa, N. Hashimoto, S. Takigawa, T. Noda, C. Matsuura-Endo, and H. Yamauchi. 2008. Comparison of phenolic compositions between common and tartary buckwheat (Fagopyrum) sprouts. Food Chemistry 110 (4):814–20. doi: 10.1016/j.foodchem.2008.02.050.
- Kirkland, L. L., D. T. Kashiwagi, S. Brantley, D. Scheurer, and P. Varkey. 2013. Nutrition in the hospitalized patient. Journal of Hospital Medicine 8 (1):52–8. doi: 10.1002/jhm.1969.
- Kitamura, Y. I., T. Kitamura, J. P. Kruse, J. C. Raum, R. Stein, W. Gu, and D. Accili. 2005. FoxO1 protects against pancreatic beta cell failure through NeuroD and MafA induction. Cell Metabolism 2 (3):153–63. doi: 10.1016/j.cmet.2005.08.004.
- Kiyak Caglayan, E., Y. Engin-Ustun, A. Y. Gocmen, M. F. Polat, and A. Aktulay. 2015. Serum sirtuin 1 levels in patients with polycystic ovary syndrome. Journal of Obstetrics and Gynaecology 35 (6):608–11. doi: 10.3109/01443615.2014.990428.
- Knudsen, A. W., A. Krag, I. Nordgaard-Lassen, E. Frandsen, F. Tofteng, C. Mortensen, and U. Becker. 2016. Effect of paracentesis on metabolic activity in patients with advanced cirrhosis and ascites. Scandinavian Journal of Gastroenterology 51 (5):601–9. doi: 10.3109/00365521.2015.1124282.
- Kops, G. J. P. L., T. B. Dansen, P. E. Polderman, I. Saarloos, K. W. A. Wirtz, P. J. Coffer, T.-T. Huang, J. L. Bos, R. H. Medema, and B. M. T. Burgering. 2002. Forkhead transcription factor FOXO3a protects quiescent cells from oxidative stress. Nature 419 (6904):316–21. doi: 10.1038/nature01036.
- Kratz, E. M., I. Kokot, V. Dymicka-Piekarska, and A. Piwowar. 2021. Sirtuins-The New Important Players in Women’s Gynecological Health. Antioxidants 10 (1):84. doi: 10.3390/antiox10010084.
- Krcatović, E., G. Rusak, N. Bezić, and M. Krajacić. 2008. Inhibition of tobacco mosaic virus infection by quercetin and vitexin. Acta Virologica 52 (2):119–24.
- Kugel, S., C. Sebastián, J. Fitamant, K. N. Ross, S. K. Saha, E. Jain, A. Gladden, K. S. Arora, Y. Kato, M. N. Rivera, et al. 2016. SIRT6 suppresses pancreatic cancer through control of Lin28b. Cell 165 (6):1401–15. doi: 10.1016/j.cell.2016.04.033.
- Kumar, R., N. Mohan, A. D. Upadhyay, A. P. Singh, V. Sahu, S. Dwivedi, A. B. Dey, and S. Dey. 2014. Identification of serum sirtuins as novel noninvasive protein markers for frailty. Aging Cell 13 (6):975–80. doi: 10.1111/acel.12260.
- Kume, S., M. Haneda, K. Kanasaki, T. Sugimoto, S-i Araki, M. Isono, K. Isshiki, T. Uzu, A. Kashiwagi, and D. Koya. 2006. Silent information regulator 2 (SIRT1) attenuates oxidative stress-induced mesangial cell apoptosis via p53 deacetylation. Free Radical Biology & Medicine 40 (12):2175–82. doi: 10.1016/j.freeradbiomed.2006.02.014.
- Kwon, G. T., J. I. Jung, H. R. Song, E. Y. Woo, J.-G. Jun, J.-K. Kim, S. Her, and J. H. Y. Park. 2012. Piceatannol inhibits migration and invasion of prostate cancer cells: Possible mediation by decreased interleukin-6 signaling. The Journal of Nutritional Biochemistry 23 (3):228–38. doi: 10.1016/j.jnutbio.2010.11.019.
- Lain, S., J. J. Hollick, J. Campbell, O. D. Staples, M. Higgins, M. Aoubala, A. McCarthy, V. Appleyard, K. E. Murray, L. Baker, et al. 2008. Discovery, in vivo activity, and mechanism of action of a small-molecule p53 activator. Cancer Cell 13 (5):454–63. doi: 10.1016/j.ccr.2008.03.004.
- Lall, R. K., V. M. Adhami, and H. Mukhtar. 2016. Dietary flavonoid fisetin for cancer prevention and treatment. Molecular Nutrition & Food Research 60 (6):1396–405. doi: 10.1002/mnfr.201600025.
- Lam, K. Y., A. P. Ling, R. Y. Koh, Y. P. Wong, and Y. H. Say. 2016. A review on medicinal properties of orientin. Advances in Pharmacological Sciences 2016:4104595. doi: 10.1155/2016/4104595.
- Lanzillotta, A., I. Sarnico, R. Ingrassia, F. Boroni, C. Branca, M. Benarese, G. Faraco, F. Blasi, A. Chiarugi, P. Spano, et al. 2010. The acetylation of RelA in Lys310 dictates the NF-κB-dependent response in post-ischemic injury. Cell Death & Disease 1 (11):e96. doi: 10.1038/cddis.2010.76.
- Lara, E., A. Mai, V. Calvanese, L. Altucci, P. Lopez-Nieva, M. L. Martinez-Chantar, M. Varela-Rey, D. Rotili, A. Nebbioso, S. Ropero, et al. 2009. Salermide, a Sirtuin inhibitor with a strong cancer-specific proapoptotic effect. Oncogene 28 (6):781–91. doi: 10.1038/onc.2008.436.
- Latini, G., B. De Mitri, A. Del Vecchio, G. Chitano, C. De Felice, and R. Zetterström. 2004. Foetal growth of kidneys, liver and spleen in intrauterine growth restriction: “Programming” causing “metabolic syndrome” in adult age. Acta Paediatrica 93 (12):1635–9. doi: 10.1080/08035250410023106.
- Lee, E. B., J. H. Kim, Y.-S. Cha, M. Kim, S. B. Song, D. S. Cha, H. Jeon, J. S. Eun, S. Han, and D. K. Kim. 2015. Lifespan Extending and stress resistant properties of vitexin from Vigna angularis in Caenorhabditis elegans. Biomolecules & Therapeutics 23 (6):582–9. doi: 10.4062/biomolther.2015.128.
- Lee, W., S. K. Ku, and J. S. Bae. 2014. Vascular barrier protective effects of orientin and isoorientin in LPS-induced inflammation in vitro and in vivo. Vascular Pharmacology 62 (1):3–14. doi: 10.1016/j.vph.2014.04.006.
- Lee, Y., S.-O. Ka, H.-N. Cha, Y.-N. Chae, M.-K. Kim, S.-Y. Park, E. J. Bae, and B.-H. Park. 2017. Myeloid sirtuin 6 deficiency causes insulin resistance in high-fat diet-fed mice by eliciting macrophage polarization toward an M1 phenotype. Diabetes 66 (10):2659–68. doi: 10.2337/db16-1446.
- Lemieux, M. E., X. Yang, K. Jardine, X. He, K. X. Jacobsen, W. A. Staines, M. E. Harper, and M. W. McBurney. 2005. The Sirt1 deacetylase modulates the insulin-like growth factor signaling pathway in mammals. Mechanisms of Ageing and Development 126 (10):1097–105. doi: 10.1016/j.mad.2005.04.006.
- Leng, S., P. Chaves, K. Koenig, and J. Walston. 2002. Serum interleukin-6 and hemoglobin as physiological correlates in the geriatric syndrome of frailty: A pilot study. Journal of the American Geriatrics Society 50 (7):1268–71. doi: 10.1046/j.1532-5415.2002.50315.x.
- Leng, S. X., A. R. Cappola, R. E. Andersen, M. R. Blackman, K. Koenig, M. Blair, and J. D. Walston. 2004. Serum levels of insulin-like growth factor-I (IGF-I) and dehydroepiandrosterone sulfate (DHEA-S), and their relationships with serum interleukin-6, in the geriatric syndrome of frailty. Aging Clinical and Experimental Research 16 (2):153–7. doi: 10.1007/bf03324545.
- Leng, S. X., Q. L. Xue, J. Tian, Y. Huang, S. H. Yeh, and L. P. Fried. 2009. Associations of neutrophil and monocyte counts with frailty in community-dwelling disabled older women: Results from the Women’s Health and Aging Studies I. Experimental Gerontology 44 (8):511–6. doi: 10.1016/j.exger.2009.05.005.
- Leng, S. X., Q. L. Xue, J. Tian, J. D. Walston, and L. P. Fried. 2007. Inflammation and frailty in older women. Journal of the American Geriatrics Society 55 (6):864–71. doi: 10.1111/j.1532-5415.2007.01186.x.
- Lesourd, B. M., and L. Mazari. 1997. Immune responses during recovery from protein-energy malnutrition. Clinical Nutrition (Edinburgh, Scotland) 16(Suppl 1):37–46. doi: 10.1016/s0261-5614(97)80047-7.
- Li, H., M. Xu, J. Lee, C. He, and Z. Xie. 2012. Leucine supplementation increases SIRT1 expression and prevents mitochondrial dysfunction and metabolic disorders in high-fat diet-induced obese mice. American Journal of Physiology-Endocrinology and Metabolism 303 (10):E1234–1244. doi: 10.1152/ajpendo.00198.2012.
- Li, M., J. F. Chiu, B. T. Mossman, and N. K. Fukagawa. 2006. Down-regulation of manganese-superoxide dismutase through phosphorylation of FOXO3a by Akt in explanted vascular smooth muscle cells from old rats. The Journal of Biological Chemistry 281 (52):40429–39. doi: 10.1074/jbc.M606596200.
- Li, X., F. Lian, C. Liu, K. Q. Hu, and X. D. Wang. 2015. Isocaloric pair-fed high-carbohydrate diet induced more hepatic steatosis and inflammation than high-fat diet mediated by miR-34a/SIRT1 axis in mice. Scientific Reports 5:16774. doi: 10.1038/srep16774.
- Lin, Q., S. Feng, Y. Cen, Y. Yang, and L. Wang. 2004. Study on the antibacterial and antiviral activity compositions of Trollium chinensis Bunge. Journal of Zhejiang University (Science Edition) 31 (4):412–5.
- Liu, G., L. Su, X. Hao, N. Zhong, D. Zhong, S. Singhal, and X. Liu. 2012. Salermide up-regulates death receptor 5 expression through the ATF4-ATF3-CHOP axis and leads to apoptosis in human cancer cells. Journal of Cellular and Molecular Medicine 16 (7):1618–28. doi: 10.1111/j.1582-4934.2011.01401.x.
- Liu, P., Z. F. Zhang, J. J. Cai, B. S. Wang, and X. Yan. 2012. NRS2002 assesses nutritional status of leukemia patients undergoing hematopoietic stem cell transplantation. Chinese Journal of Cancer Research 24 (4):299–303. doi: 10.3978/j.issn.1000-9604.2012.09.01.
- Lo Sasso, G., K. J. Menzies, A. Mottis, A. Piersigilli, A. Perino, H. Yamamoto, K. Schoonjans, and J. Auwerx. 2014. SIRT2 deficiency modulates macrophage polarization and susceptibility to experimental colitis. PloS One 9 (7):e103573. doi: 10.1371/journal.pone.0103573.
- Lu, C-C., Y-Q Xu, J-C Wu, P-Z Hang, Y. Wang, C. Wang, J-W Wu, J-C Qi, Y. Zhang, and Z-M Du. 2013. Vitexin protects against cardiac hypertrophy via inhibiting calcineurin and CaMKII signaling pathways. Naunyn-Schmiedeberg’s Archives of Pharmacology 386 (8):747–55. doi: 10.1007/s00210-013-0873-0.
- Ma, L., H. Niu, G. Sha, Y. Zhang, P. Liu, and Y. Li. 2019. Serum SIRT1 is associated with frailty and adipokines in older adults. The Journal of Nutrition, Health & Aging 23 (3):246–50. doi: 10.1007/s12603-018-1149-7.
- Macedo, C., J. M. Gazzola, and M. Najas. 2008. Frailty syndrome in elderly patients: The importance of physiotherapy. Arquivos Brasileiros de Ciências da Saúde 33 (3):177–84. doi: 10.7322/abcs.v33i3.154.
- Magyar, K., R. Halmosi, A. Palfi, G. Feher, L. Czopf, A. Fulop, I. Battyany, B. Sumegi, K. Toth, and E. Szabados. 2012. Cardioprotection by resveratrol: A human clinical trial in patients with stable coronary artery disease. Clinical Hemorheology and Microcirculation 50 (3):179–87. doi: 10.3233/ch-2011-1424.
- Maher, P., T. Akaishi, and K. Abe. 2006. Flavonoid fisetin promotes ERK-dependent long-term potentiation and enhances memory. Proceedings of the National Academy of Sciences of the United States of America 103 (44):16568–73. doi: 10.1073/pnas.0607822103.
- Maher, P., R. Dargusch, J. L. Ehren, S. Okada, K. Sharma, and D. Schubert. 2011. Fisetin lowers methylglyoxal dependent protein glycation and limits the complications of diabetes. PloS One 6 (6):e21226. doi: 10.1371/journal.pone.0021226.
- Manary, M. J., C. Leeuwenburgh, and J. W. Heinecke. 2000. Increased oxidative stress in kwashiorkor. The Journal of Pediatrics 137 (3):421–4. doi: 10.1067/mpd.2000.107512.
- Manjula, R., K. Anuja, and F. J. Alcain. 2020. SIRT1 and SIRT2 activity control in neurodegenerative diseases. Frontiers in Pharmacology 11:585821. doi: 10.3389/fphar.2020.585821.
- Manna, D., R. Bhuyan, and R. Ghosh. 2018. Probing the mechanism of SIRT1 activation by a 1,4-dihydropyridine. Journal of Molecular Modeling 24 (12):340. doi: 10.1007/s00894-018-3877-3.
- Martin-Gronert, M. S., and S. E. Ozanne. 2012. Metabolic programming of insulin action and secretion. Diabetes, Obesity & Metabolism 14(Suppl 3):29–39. doi: 10.1111/j.1463-1326.2012.01653.x.
- Mastrocola, R., D. Nigro, F. Chiazza, C. Medana, F. Dal Bello, G. Boccuzzi, M. Collino, and M. Aragno. 2016. Fructose-derived advanced glycation end-products drive lipogenesis and skeletal muscle reprogramming via SREBP-1c dysregulation in mice. Free Radical Biology & Medicine 91:224–35. doi: 10.1016/j.freeradbiomed.2015.12.022.
- Mazloom, Z., S. M. Abdollahzadeh, M. H. Dabbaghmanesh, and A. Rezaianzadeh. 2014. The effect of quercetin supplementation on oxidative stress, glycemic control, lipid profile and insulin resistance in type 2 diabetes: A randomized clinical trial. Journal of Health Sciences & Surveillance System 2 (1):8–14.
- McBurney, M. W., X. Yang, K. Jardine, M. Hixon, K. Boekelheide, J. R. Webb, P. M. Lansdorp, and M. Lemieux. 2003. The mammalian SIR2alpha protein has a role in embryogenesis and gametogenesis. Molecular and Cellular Biology 23 (1):38–54. doi: 10.1128/mcb.23.1.38-54.2003.
- McClain, C., I. Kirpich, and L. Smart. 2017. Malnutrition and liver disease. In Schiff’s diseases of the liver, 460–87. New York: John Wiley and Sons.
- McDade, T. W., M. A. Beck, C. W. Kuzawa, and L. S. Adair. 2001. Prenatal undernutrition and postnatal growth are associated with adolescent thymic function. The Journal of Nutrition 131 (4):1225–31. doi: 10.1093/jn/131.4.1225.
- Meydani, S. N., S. K. Das, C. F. Pieper, M. R. Lewis, S. Klein, V. D. Dixit, A. K. Gupta, D. T. Villareal, M. Bhapkar, M. Huang, et al. 2016. Long-term moderate calorie restriction inhibits inflammation without impairing cell-mediated immunity: A randomized controlled trial in non-obese humans. Aging 8 (7):1416–31. doi: 10.18632/aging.100994.
- Michan, S. 2014. Calorie restriction and NAD+/sirtuin counteract the hallmarks of aging. Frontiers in Bioscience (Landmark Edition) 19 (8):1300–19. doi: 10.2741/4283.
- Milne, J. C., P. D. Lambert, S. Schenk, D. P. Carney, J. J. Smith, D. J. Gagne, L. Jin, O. Boss, R. B. Perni, C. B. Vu, et al. 2007. Small molecule activators of SIRT1 as therapeutics for the treatment of type 2 diabetes. Nature 450 (7170):712–6. doi: 10.1038/nature06261.
- Min, J.-W., J.-J. Hu, M. He, R. M. Sanchez, W.-X. Huang, Y.-Q. Liu, N. B. Bsoul, S. Han, J. Yin, W.-H. Liu, et al. 2015. Vitexin reduces hypoxia-ischemia neonatal brain injury by the inhibition of HIF-1alpha in a rat pup model. Neuropharmacology 99:38–50. doi: 10.1016/j.neuropharm.2015.07.007.
- Mishra, A. P., A. Bajpai, and A. K. Rai. 2019. 1,4-Dihydropyridine: A dependable heterocyclic ring with the promising and the most anticipable therapeutic effects. Mini Reviews in Medicinal Chemistry 19 (15):1219–54. doi: 10.2174/1389557519666190425184749.
- Moreira, P. L. 2011. Nutritional status and functional capacity of institutionalized elderly in Botucatu/SP. Geriatrics, Gerontology and Aging 5 (1).
- Morena, M., J. P. Cristol, T. Dantoine, M. A. Carbonneau, B. Descomps, and B. Canaud. 2000. Protective effects of high-density lipoprotein against oxidative stress are impaired in haemodialysis patients. Nephrology, Dialysis, Transplantation 15 (3):389–95. doi: 10.1093/ndt/15.3.389.
- Morley, J. E. 2001. Decreased food intake with aging. The Journals of Gerontology. Series A, Biological Sciences and Medical Sciences 56:81–8. doi: 10.1093/gerona/56.suppl_2.81.
- Morley, J. E. 2010. Anorexia, weight loss, and frailty. Journal of the American Medical Directors Association 11 (4):225–8. doi: 10.1016/j.jamda.2010.02.005.
- Mouchiroud, L., R. H. Houtkooper, N. Moullan, E. Katsyuba, D. Ryu, C. Cantó, A. Mottis, Y.-S. Jo, M. Viswanathan, K. Schoonjans, et al. 2013. The NAD+/sirtuin pathway modulates longevity through activation of mitochondrial UPR and FOXO signaling. Cell 154 (2):430–41. doi: 10.1016/j.cell.2013.06.016.
- Nagai, S., C. Matsumoto, M. Shibano, and K. Fujimori. 2018. Suppression of fatty acid and triglyceride synthesis by the flavonoid orientin through decrease of C/EBPδ expression and inhibition of PI3K/Akt-FOXO1 signaling in adipocytes. Nutrients 10 (2):130. doi: 10.3390/nu10020130.
- Nakahata, Y., M. Kaluzova, B. Grimaldi, S. Sahar, J. Hirayama, D. Chen, L. P. Guarente, and P. Sassone-Corsi. 2008. The NAD+-dependent deacetylase SIRT1 modulates CLOCK-mediated chromatin remodeling and circadian control. Cell 134 (2):329–40. doi: 10.1016/j.cell.2008.07.002.
- Nayak, V., and P. U. Devi. 2005. Protection of mouse bone marrow against radiation-induced chromosome damage and stem cell death by the ocimum flavonoids orientin and vicenin. Radiation Research 163 (2):165–71. doi: 10.1667/rr3263.
- Nemoto, S., M. M. Fergusson, and T. Finkel. 2004. Nutrient availability regulates SIRT1 through a forkhead-dependent pathway. Science (New York, N.Y.) 306 (5704):2105–8. doi: 10.1126/science.1101731.
- Nguyen, A. V., M. Martinez, M. J. Stamos, M. P. Moyer, K. Planutis, C. Hope, and R. F. Holcombe. 2009. Results of a phase I pilot clinical trial examining the effect of plant-derived resveratrol and grape powder on Wnt pathway target gene expression in colonic mucosa and colon cancer. Cancer Management and Research 1:25–37.
- Nissanka, N., and C. T. Moraes. 2018. Mitochondrial DNA damage and reactive oxygen species in neurodegenerative disease. FEBS Letters 592 (5):728–42. doi: 10.1002/1873-3468.12956.
- Noriega, L. G., J. N. Feige, C. Canto, H. Yamamoto, J. Yu, M. A. Herman, C. Mataki, B. B. Kahn, and J. Auwerx. 2011. CREB and ChREBP oppositely regulate SIRT1 expression in response to energy availability. EMBO Reports 12 (10):1069–76. doi: 10.1038/embor.2011.151.
- Ohara, K., K. Kusano, S. Kitao, T. Yanai, R. Takata, and O. Kanauchi. 2015. ε-Viniferin, a resveratrol dimer, prevents diet-induced obesity in mice. Biochemical and Biophysical Research Communications 468 (4):877–82. doi: 10.1016/j.bbrc.2015.11.047.
- Olmos, Y., F. J. Sánchez-Gómez, B. Wild, N. García-Quintans, S. Cabezudo, S. Lamas, and M. Monsalve. 2013. SirT1 regulation of antioxidant genes is dependent on the formation of a FoxO3a/PGC-1α complex. Antioxidants & Redox Signaling 19 (13):1507–21. doi: 10.1089/ars.2012.4713.
- Orecchia, A., C. Scarponi, F. Di Felice, E. Cesarini, S. Avitabile, A. Mai, M. L. Mauro, V. Sirri, G. Zambruno, C. Albanesi, et al. 2011. Sirtinol treatment reduces inflammation in human dermal microvascular endothelial cells. PloS One 6 (9):e24307. doi: 10.1371/journal.pone.0024307.
- Ota, H., E. Tokunaga, K. Chang, M. Hikasa, K. Iijima, M. Eto, K. Kozaki, M. Akishita, Y. Ouchi, and M. Kaneki. 2006. Sirt1 inhibitor, Sirtinol, induces senescence-like growth arrest with attenuated Ras-MAPK signaling in human cancer cells. Oncogene 25 (2):176–85. doi: 10.1038/sj.onc.1209049.
- Outeiro, T. F., E. Kontopoulos, S. M. Altmann, I. Kufareva, K. E. Strathearn, A. M. Amore, C. B. Volk, M. M. Maxwell, J.-C. Rochet, P. J. McLean, et al. 2007. Sirtuin 2 inhibitors rescue alpha-synuclein-mediated toxicity in models of Parkinson’s disease. Science (New York, N.Y.) 317 (5837):516–9. doi: 10.1126/science.1143780.
- Pais, T. F., É. M. Szegő, O. Marques, L. Miller-Fleming, P. Antas, P. Guerreiro, R. M. de Oliveira, B. Kasapoglu, and T. F. Outeiro. 2013. The NAD-dependent deacetylase sirtuin 2 is a suppressor of microglial activation and brain inflammation. The EMBO Journal 32 (19):2603–16. doi: 10.1038/emboj.2013.200.
- Park, H.-H., S. Lee, H.-Y. Son, S.-B. Park, M.-S. Kim, E.-J. Choi, T. S. K. Singh, J.-H. Ha, M.-G. Lee, J.-E. Kim, et al. 2008. Flavonoids inhibit histamine release and expression of proinflammatory cytokines in mast cells. Archives of Pharmacal Research 31 (10):1303–11. doi: 10.1007/s12272-001-2110-5.
- Piccinelli, A. L., M. García Mesa, D. M. Armenteros, M. A. Alfonso, A. C. Arevalo, L. Campone, and L. Rastrelli. 2008. HPLC-PDA-MS and NMR characterization of C-glycosyl flavones in a hydroalcoholic extract of Citrus aurantifolia leaves with antiplatelet activity. Journal of Agricultural and Food Chemistry 56 (5):1574–81. doi: 10.1021/jf073485k.
- Posakony, J., M. Hirao, S. Stevens, J. A. Simon, and A. Bedalov. 2004. Inhibitors of Sir2: Evaluation of splitomicin analogues. Journal of Medicinal Chemistry 47 (10):2635–44. doi: 10.1021/jm030473r.
- Potter, G. A., L. H. Patterson, E. Wanogho, P. J. Perry, P. C. Butler, T. Ijaz, K. C. Ruparelia, J. H. Lamb, P. B. Farmer, L. A. Stanley, et al. 2002. The cancer preventative agent resveratrol is converted to the anticancer agent piceatannol by the cytochrome P450 enzyme CYP1B1. British Journal of Cancer 86 (5):774–8. doi: 10.1038/sj.bjc.6600197.
- Poulsen, M. M., P. F. Vestergaard, B. F. Clasen, Y. Radko, L. P. Christensen, H. Stødkilde-Jørgensen, N. Møller, N. Jessen, S. B. Pedersen, and J. O. L. Jørgensen. 2013. High-dose resveratrol supplementation in obese men: An investigator-initiated, randomized, placebo-controlled clinical trial of substrate metabolism, insulin sensitivity, and body composition. Diabetes 62 (4):1186–95. doi: 10.2337/db12-0975.
- Price, N. L., A. P. Gomes, A. J. Y. Ling, F. V. Duarte, A. Martin-Montalvo, B. J. North, B. Agarwal, L. Ye, G. Ramadori, J. S. Teodoro, et al. 2012. SIRT1 is required for AMPK activation and the beneficial effects of resveratrol on mitochondrial function. Cell Metabolism 15 (5):675–90. doi: 10.1016/j.cmet.2012.04.003.
- Purushotham, A., T. T. Schug, Q. Xu, S. Surapureddi, X. Guo, and X. Li. 2009. Hepatocyte-specific deletion of SIRT1 alters fatty acid metabolism and results in hepatic steatosis and inflammation. Cell Metabolism 9 (4):327–38. doi: 10.1016/j.cmet.2009.02.006.
- Qin, J., Y. Liu, Y. Lu, M. Liu, M. Li, J. Li, and L. Wu. 2017. Hypoxia-inducible factor 1 alpha promotes cancer stem cells-like properties in human ovarian cancer cells by upregulating SIRT1 expression. Scientific Reports 7 (1):10592. doi: 10.1038/s41598-017-09244-8.
- Quílez, A., B. Berenguer, G. Gilardoni, C. Souccar, S. de Mendonça, L. F. S. Oliveira, M. J. Martín-Calero, and G. Vidari. 2010. Anti-secretory, anti-inflammatory and anti-Helicobacter pylori activities of several fractions isolated from Piper carpunya Ruiz & Pav. Journal of Ethnopharmacology 128 (3):583–9. doi: 10.1016/j.jep.2010.01.060.
- Rack, J. G. M., R. Morra, E. Barkauskaite, R. Kraehenbuehl, A. Ariza, Y. Qu, M. Ortmayer, O. Leidecker, D. R. Cameron, I. Matic, et al. 2015. Identification of a class of protein ADP-ribosylating sirtuins in microbial pathogens. Molecular Cell 59 (2):309–20. doi: 10.1016/j.molcel.2015.06.013.
- Rahman, I., V. L. Kinnula, V. Gorbunova, and H. Yao. 2012. SIRT1 as a therapeutic target in inflammaging of the pulmonary disease. Preventive Medicine 54 (Suppl):S20–S28. doi: 10.1016/j.ypmed.2011.11.014.
- Revollo, J. R., A. A. Grimm, and S. Imai. 2004. The NAD biosynthesis pathway mediated by nicotinamide phosphoribosyltransferase regulates Sir2 activity in mammalian cells. The Journal of Biological Chemistry 279 (49):50754–63. doi: 10.1074/jbc.M408388200.
- Rezvan, N., A. Moini, L. Janani, K. Mohammad, A. Saedisomeolia, M. Nourbakhsh, S. Gorgani-Firuzjaee, M. Mazaherioun, and M. J. Hosseinzadeh-Attar. 2017. Effects of quercetin on adiponectin-mediated insulin sensitivity in polycystic ovary syndrome: A randomized placebo-controlled double-blind clinical trial. Hormone and Metabolic Research 49 (2):115–21. doi: 10.1055/s-0042-118705.
- Rimando, A. M., R. Nagmani, D. R. Feller, and W. Yokoyama. 2005. Pterostilbene, a new agonist for the peroxisome proliferator-activated receptor alpha-isoform, lowers plasma lipoproteins and cholesterol in hypercholesterolemic hamsters. Journal of Agricultural and Food Chemistry 53 (9):3403–7. doi: 10.1021/jf0580364.
- Robberecht, H., T. De Bruyne, E. Davioud-Charvet, J. Mackrill, and N. Hermans. 2019. Selenium status in elderly people: Longevity and age-related diseases. Current Pharmaceutical Design 25 (15):1694–706. doi: 10.2174/1381612825666190701144709.
- Rodgers, J. T., and P. Puigserver. 2007. Fasting-dependent glucose and lipid metabolic response through hepatic sirtuin 1. Proceedings of the National Academy of Sciences of the United States of America 104 (31):12861–6. doi: 10.1073/pnas.0702509104.
- Rondanelli, M., C. Klersy, G. Terracol, J. Talluri, R. Maugeri, D. Guido, M. A. Faliva, B. S. Solerte, M. Fioravanti, H. Lukaski, et al. 2016. Whey protein, amino acids, and vitamin D supplementation with physical activity increases fat-free mass and strength, functionality, and quality of life and decreases inflammation in sarcopenic elderly. The American Journal of Clinical Nutrition 103 (3):830–40. doi: 10.3945/ajcn.115.113357.
- Rosa, S. I., F. Rios-Santos, S. O. Balogun, and D. T. Martins. 2016. Vitexin reduces neutrophil migration to inflammatory focus by down-regulating pro-inflammatory mediators via inhibition of p38, ERK1/2 and JNK pathway. Phytomedicine: International Journal of Phytotherapy and Phytopharmacology 23 (1):9–17. doi: 10.1016/j.phymed.2015.11.003.
- Rubenstein, L. Z., J. O. Harker, A. Salvà, Y. Guigoz, and B. Vellas. 2001. Screening for undernutrition in geriatric practice: Developing the short-form mini-nutritional assessment (MNA-SF). The Journals of Gerontology. Series A, Biological Sciences and Medical Sciences 56 (6):M366–372. doi: 10.1093/gerona/56.6.m366.
- Russell, C. A., and M. Elia. 2011. Nutrition screening survey in the UK and Republic of Ireland in 2001. www.bapen.org.uk:
- Santolla, M. F., S. Avino, M. Pellegrino, E. M. De Francesco, P. De Marco, R. Lappano, A. Vivacqua, F. Cirillo, D. C. Rigiracciolo, A. Scarpelli, et al. 2015. SIRT1 is involved in oncogenic signaling mediated by GPER in breast cancer. Cell Death & Disease 6 (7):e1834. doi: 10.1038/cddis.2015.201.
- Schloms, L., and A. C. Swart. 2014. Rooibos flavonoids inhibit the activity of key adrenal steroidogenic enzymes, modulating steroid hormone levels in H295R cells. Molecules (Basel, Switzerland) 19 (3):3681–95. doi: 10.3390/molecules19033681.
- Schug, T. T., Q. Xu, H. Gao, A. Peres-da-Silva, D. W. Draper, M. B. Fessler, A. Purushotham, and X. Li. 2010. Myeloid deletion of SIRT1 induces inflammatory signaling in response to environmental stress. Molecular and Cellular Biology 30 (19):4712–21. doi: 10.1128/mcb.00657-10.
- de van der Schueren, M. A. E., H. Keller, T. Cederholm, R. Barazzoni, C. Compher, M. I. T. D. Correia, M. C. Gonzalez, H. Jager-Wittenaar, M. Pirlich, A. Steiber, GLIM Consortium, et al. 2020. Global Leadership Initiative on Malnutrition (GLIM): Guidance on validation of the operational criteria for the diagnosis of protein-energy malnutrition in adults. Clinical Nutrition (Edinburgh, Scotland) 39 (9):2872–2880. doi: 10.1016/j.clnu.2019.12.022.
- Schutkowski, M., F. Fischer, C. Roessler, and C. Steegborn. 2014. New assays and approaches for discovery and design of sirtuin modulators. Expert Opinion on Drug Discovery 9 (2):183–99. doi: 10.1517/17460441.2014.875526.
- Sente, T., A. M. Van Berendoncks, E. Fransen, C. J. Vrints, and V. Y. Hoymans. 2016. Tumor necrosis factor-α impairs adiponectin signalling, mitochondrial biogenesis, and myogenesis in primary human myotubes cultures. American Journal of Physiology. Heart and Circulatory Physiology 310 (9):H1164–1175. doi: 10.1152/ajpheart.00831.2015.
- Serban, M., A. Sahebkar, A. Zanchetti, D. P. Mikhailidis, G. Howard, D. Antal, F. Andrica, A. Ahmed, W. S. Aronow, P. Muntner, for the Lipid and Blood Pressure Meta‐analysis Collaboration (LBPMC) Group, et al. 2016. Effects of quercetin on blood pressure: A systematic review and meta-analysis of randomized controlled trials. Journal of the American Heart Association 5 (7):e002713. doi: 10.1161/JAHA.115.002713.
- Seyed, M. A., I. Jantan, S. N. Bukhari, and K. Vijayaraghavan. 2016. A comprehensive review on the chemotherapeutic potential of piceatannol for cancer treatment, with mechanistic insights. Journal of Agricultural and Food Chemistry 64 (4):725–37. doi: 10.1021/acs.jafc.5b05993.
- Shinbo, Y., Y. Nakamura, M. Altaf-Ul-Amin, H. Asahi, K. Kurokawa, M. Arita, … S. Kanaya. 2006. KNApSAcK: A Comprehensive Species-Metabolite Relationship Database. In Plant metabolomics, ed. K. Saito, R. A. Dixon, and L. Willmitzer, 165–81. Berlin, Heidelberg: Springer Berlin Heidelberg.
- Shirane, A., O. Wada-Hiraike, M. Tanikawa, T. Seiki, H. Hiraike, Y. Miyamoto, K. Sone, M. Hirano, H. Oishi, K. Oda, et al. 2012. Regulation of SIRT1 determines initial step of endometrial receptivity by controlling E-cadherin expression. Biochemical and Biophysical Research Communications 424 (3):604–10. doi: 10.1016/j.bbrc.2012.06.160.
- Smith, J. S., C. B. Brachmann, I. Celic, M. A. Kenna, S. Muhammad, V. J. Starai, J. L. Avalos, J. C. Escalante-Semerena, C. Grubmeyer, C. Wolberger, et al. 2000. A phylogenetically conserved NAD+-dependent protein deacetylase activity in the Sir2 protein family. Proceedings of the National Academy of Sciences of the United States of America 97 (12):6658–63. doi: 10.1073/pnas.97.12.6658.
- Solmi, M., N. Veronese, A. Favaro, P. Santonastaso, E. Manzato, G. Sergi, and C. U. Correll. 2015. Inflammatory cytokines and anorexia nervosa: A meta-analysis of cross-sectional and longitudinal studies. Psychoneuroendocrinology 51:237–52. doi: 10.1016/j.psyneuen.2014.09.031.
- Sommer, F., J. M. Anderson, R. Bharti, J. Raes, and P. Rosenstiel. 2017. The resilience of the intestinal microbiota influences health and disease. Nature Reviews. Microbiology 15 (10):630–8. doi: 10.1038/nrmicro.2017.58.
- Song, S., S.-Y. Kim, Y.-M. Hong, D.-G. Jo, J.-Y. Lee, S. M. Shim, C.-W. Chung, S. J. Seo, Y. J. Yoo, J.-Y. Koh, et al. 2003. Essential role of E2-25K/Hip-2 in mediating amyloid-beta neurotoxicity. Molecular Cell 12 (3):553–63. doi: 10.1016/j.molcel.2003.08.005.
- Sun, A., G. Ren, C. Deng, J. Zhang, X. Luo, X. Wu, S. Mani, W. Dou, and Z. Wang. 2016. C-glycosyl flavonoid orientin improves chemically induced inflammatory bowel disease in mice. Journal of Functional Foods 21:418–30. doi: 10.1016/j.jff.2015.12.029.
- Sun, X., and M. B. Zemel. 2009. Leucine modulation of mitochondrial mass and oxygen consumption in skeletal muscle cells and adipocytes. Nutrition & Metabolism 6:26. doi: 10.1186/1743-7075-6-26.
- Swinburn, B. A., V. I. Kraak, S. Allender, V. J. Atkins, P. I. Baker, J. R. Bogard, H. Brinsden, A. Calvillo, O. De Schutter, R. Devarajan, et al. 2019. The global syndemic of obesity, undernutrition, and climate change: The Lancet Commission report. Lancet (London, England) 393 (10173):791–846. doi: 10.1016/s0140-6736(18)32822-8.
- Syed, D. N., V. M. Adhami, M. I. Khan, and H. Mukhtar. 2013. Inhibition of Akt/mTOR signaling by the dietary flavonoid fisetin. Anti-Cancer Agents in Medicinal Chemistry 13 (7):995–1001. doi: 10.2174/18715206113139990129.
- Tamura, B. K., C. L. Bell, K. H. Masaki, and E. J. Amella. 2013. Factors associated with weight loss, low BMI, and malnutrition among nursing home patients: A systematic review of the literature. Journal of the American Medical Directors Association 14 (9):649–55. doi: 10.1016/j.jamda.2013.02.022.
- Tan, C. W., I. C. Sam, W. L. Chong, V. S. Lee, and Y. F. Chan. 2017. Polysulfonate suramin inhibits Zika virus infection. Antiviral Research 143:186–94. doi: 10.1016/j.antiviral.2017.04.017.
- Tang, Y. L., and S. W. Chan. 2014. A review of the pharmacological effects of piceatannol on cardiovascular diseases. Phytotherapy Research : PTR 28 (11):1581–8. doi: 10.1002/ptr.5185.
- Tanno, M., A. Kuno, T. Yano, T. Miura, S. Hisahara, S. Ishikawa, K. Shimamoto, and Y. Horio. 2010. Induction of manganese superoxide dismutase by nuclear translocation and activation of SIRT1 promotes cell survival in chronic heart failure. The Journal of Biological Chemistry 285 (11):8375–82. doi: 10.1074/jbc.M109.090266.
- Tanno, M., J. Sakamoto, T. Miura, K. Shimamoto, and Y. Horio. 2007. Nucleocytoplasmic shuttling of the NAD+-dependent histone deacetylase SIRT1. The Journal of Biological Chemistry 282 (9):6823–32. doi: 10.1074/jbc.M609554200.
- Tantray, M. A., I. Khan, H. Hamid, M. S. Alam, A. Dhulap, and A. A. Ganai. 2017. Oxazolo[4,5-b]pyridine-based piperazinamides as GSK-3β inhibitors with potential for attenuating inflammation and suppression of pro-inflammatory mediators. Archiv Der Pharmazie 350 (8):1700022. doi: 10.1002/ardp.201700022.
- Toledano, M. B., and W. J. Leonard. 1991. Modulation of transcription factor NF-kappa B binding activity by oxidation-reduction in vitro. Proceedings of the National Academy of Sciences of the United States of America 88 (10):4328–32. doi: 10.1073/pnas.88.10.4328.
- Tong, X., X. Wang, C. Wang, and L. Li. 2018. Elevated levels of serum MiR-152 and miR-24 in uterine sarcoma: Potential for inducing autophagy via SIRT1 and deacetylated LC3. British Journal of Biomedical Science 75 (1):7–12. doi: 10.1080/09674845.2017.1340225.
- Tyagi, A., C. U. Nguyen, T. Chong, C. R. Michel, K. S. Fritz, N. Reisdorph, L. Knaub, J. E. B. Reusch, and S. Pugazhenthi. 2018. SIRT3 deficiency-induced mitochondrial dysfunction and inflammasome formation in the brain. Scientific Reports 8 (1):17547. doi: 10.1038/s41598-018-35890-7.
- Tôrres, L. H., D. D. da Silva, A. L. Neri, J. B. Hilgert, F. N. Hugo, and M. L. Sousa. 2013. Association between underweight and overweight/obesity with oral health among independently living Brazilian elderly. Nutrition (Burbank, Los Angeles County, Calif.) 29 (1):152–7. doi: 10.1016/j.nut.2012.05.011.
- Uma Devi, P., A. Ganasoundari, B. S. Rao, and K. K. Srinivasan. 1999. In vivo radioprotection by ocimum flavonoids: Survival of mice. Radiation Research 151 (1):74–8. doi: 10.2307/3579750.
- United Nations Department of Economic and Social Affairs, Population Division. 2022. World population prospects 2022: Summary of results, vol. UN DESA/POP/2022/TR/NO.3. New York: United Nations, Population Division.
- Valdecantos, M. P., V. Pardo, L. Ruiz, L. Castro-Sánchez, B. Lanzón, E. Fernández-Millán, C. García-Monzón, A. I. Arroba, Á. González-Rodríguez, F. Escrivá, et al. 2017. A novel glucagon‐like peptide 1/glucagon receptor dual agonist improves steatohepatitis and liver regeneration in mice. Hepatology (Baltimore, Md.) 65 (3):950–68. doi: 10.1002/hep.28962.
- Valdecantos, M. P., P. Pérez-Matute, P. González-Muniesa, P. L. Prieto-Hontoria, M. J. Moreno-Aliaga, and J. A. Martínez. 2012. Lipoic acid administration prevents nonalcoholic steatosis linked to long-term high-fat feeding by modulating mitochondrial function. The Journal of Nutritional Biochemistry 23 (12):1676–84. doi: 10.1016/j.jnutbio.2011.11.011.
- Valente, S., P. Mellini, F. Spallotta, V. Carafa, A. Nebbioso, L. Polletta, I. Carnevale, S. Saladini, D. Trisciuoglio, C. Gabellini, et al. 2016. 1,4-Dihydropyridines active on the SIRT1/AMPK pathway ameliorate skin repair and mitochondrial function and exhibit inhibition of proliferation in cancer cells. Journal of Medicinal Chemistry 59 (4):1471–91. doi: 10.1021/acs.jmedchem.5b01117.
- van Zutphen, T., J. Ciapaite, V. W. Bloks, C. Ackereley, A. Gerding, A. Jurdzinski, R. A. de Moraes, L. Zhang, J. C. Wolters, R. Bischoff, et al. 2016. Malnutrition-associated liver steatosis and ATP depletion is caused by peroxisomal and mitochondrial dysfunction. Journal of Hepatology 65 (6):1198–208. doi: 10.1016/j.jhep.2016.05.046.
- Vasconcelos, S. M. L., M. O. F. Goulart, J. B. D. F. Moura, V. Manfredini, M. D. S. Benfato, and L. T. Kubota. 2007. Espécies reativas de oxigênio e de nitrogênio, antioxidantes e marcadores de dano oxidativo em sangue humano: Principais métodos analíticos para sua determinação. Química Nova 30 (5):1323–38. doi: 10.1590/S0100-40422007000500046.
- Vellas, B., H. Villars, G. Abellan, M. E. Soto, Y. Rolland, Y. Guigoz, … P. Garry. 2006. Overview of the MNA–Its history and challenges. Journal of Nutrition, Health and Aging 10 (6):456–63. discussion 463–455.
- Vetta, F., S. Ronzoni, G. Taglieri, and M. R. Bollea. 1999. The impact of malnutrition on the quality of life in the elderly. Clinical Nutrition (Edinburgh, Scotland) 18 (5):259–67. doi: 10.1016/s0261-5614(98)80022-8.
- Victor, V. M., M. Rocha, C. Bañuls, M. Sanchez-Serrano, E. Sola, M. Gomez, and A. Hernandez-Mijares. 2009. Mitochondrial complex I impairment in leukocytes from polycystic ovary syndrome patients with insulin resistance. The Journal of Clinical Endocrinology and Metabolism 94 (9):3505–12. doi: 10.1210/jc.2009-0466.
- Villalba, J. M., and F. J. Alcaín. 2012. Sirtuin activators and inhibitors. BioFactors (Oxford, England) 38 (5):349–59. doi: 10.1002/biof.1032.
- Vion, E., G. Page, E. Bourdeaud, M. Paccalin, J. Guillard, and A. Rioux Bilan. 2018. Trans ε-viniferin is an amyloid-β disaggregating and anti-inflammatory drug in a mouse primary cellular model of Alzheimer’s disease. Molecular and Cellular Neurosciences 88:1–6. doi: 10.1016/j.mcn.2017.12.003.
- Vitrac, X., A. Bornet, R. Vanderlinde, J. Valls, T. Richard, J.-C. Delaunay, J.-M. Mérillon, and P.-L. Teissédre. 2005. Determination of stilbenes (delta-viniferin, trans-astringin, trans-piceid, cis- and trans-resveratrol, epsilon-viniferin) in Brazilian wines. Journal of Agricultural and Food Chemistry 53 (14):5664–9. doi: 10.1021/jf050122g.
- Volkert, D., A. M. Beck, T. Cederholm, A. Cruz-Jentoft, S. Goisser, L. Hooper, E. Kiesswetter, M. Maggio, A. Raynaud-Simon, C. C. Sieber, et al. 2019. ESPEN guideline on clinical nutrition and hydration in geriatrics. Clinical Nutrition (Edinburgh, Scotland) 38 (1):10–47. doi: 10.1016/j.clnu.2018.05.024.
- Volpi, E., M. Sheffield-Moore, B. B. Rasmussen, and R. R. Wolfe. 2001. Basal muscle amino acid kinetics and protein synthesis in healthy young and older men. JAMA 286 (10):1206–12. doi: 10.1001/jama.286.10.1206.
- Vu, C. B., J. E. Bemis, J. S. Disch, P. Y. Ng, J. J. Nunes, J. C. Milne, D. P. Carney, A. V. Lynch, J. J. Smith, S. Lavu, et al. 2009. Discovery of imidazo[1,2-b]thiazole derivatives as novel SIRT1 activators. Journal of Medicinal Chemistry 52 (5):1275–83. doi: 10.1021/jm8012954.
- Wang, L., Y. C. Tu, T. W. Lian, J. T. Hung, J. H. Yen, and M. J. Wu. 2006. Distinctive antioxidant and antiinflammatory effects of flavonols. Journal of Agricultural and Food Chemistry 54 (26):9798–804. doi: 10.1021/jf0620719.
- Wang, Y., Y. Zhen, X. Wu, Q. Jiang, X. Li, Z. Chen, G. Zhang, and L. Dong. 2015. Vitexin protects brain against ischemia/reperfusion injury via modulating mitogen-activated protein kinase and apoptosis signaling in mice. Phytomedicine 22 (3):379–84. doi: 10.1016/j.phymed.2015.01.009.
- Weinberger, L. S., and T. Shenk. 2007. An HIV feedback resistor: Auto-regulatory circuit deactivator and noise buffer. PLoS Biology 5 (1):e9. doi: 10.1371/journal.pbio.0050009.
- WHO. 2004. Appropriate body-mass index for Asian populations and its implications for policy and intervention strategies. Lancet 363 (9403):157–63. doi: 10.1016/s0140-6736(03)15268-3.
- Wiedemar, N., D. A. Hauser, and P. Mäser. 2020. 100 Years of suramin. Antimicrobial Agents and Chemotherapy 64 (3):e01168–19. doi: 10.1128/AAC.01168-19.
- Winick, M., and A. Noble. 1966. Cellular response in rats during malnutrition at various ages. The Journal of Nutrition 89 (3):300–6. doi: 10.1093/jn/89.3.300.
- Wu, D., Y. Li, K. S. Zhu, H. Wang, and W. G. Zhu. 2018. Advances in cellular characterization of the sirtuin isoform, SIRT7. Frontiers in Endocrinology 9:652. doi: 10.3389/fendo.2018.00652.
- Wu, I. C., S. C. Shiesh, P. H. Kuo, and X. Z. Lin. 2009. High oxidative stress is correlated with frailty in elderly chinese. Journal of the American Geriatrics Society 57 (9):1666–71. doi: 10.1111/j.1532-5415.2009.02392.x.
- Yan, W., Y. Liang, Q. Zhang, D. Wang, M. Lei, J. Qu, X. He, Q. Lei, and Y. Wang. 2018. Arginine methylation of SIRT7 couples glucose sensing with mitochondria biogenesis. EMBO Reports 19 (12):e46377. doi: 10.15252/embr.201846377.
- Yang, H., J. A. Baur, A. Chen, C. Miller, J. K. Adams, A. Kisielewski, K. T. Howitz, R. E. Zipkin, and D. A. Sinclair. 2007. Design and synthesis of compounds that extend yeast replicative lifespan. Aging Cell 6 (1):35–43. doi: 10.1111/j.1474-9726.2006.00259.x.
- Yao, H., S. Chung, J-w Hwang, S. Rajendrasozhan, I. K. Sundar, D. A. Dean, M. W. McBurney, L. Guarente, W. Gu, M. Rönty, et al. 2012. SIRT1 protects against emphysema via FOXO3-mediated reduction of premature senescence in mice. The Journal of Clinical Investigation 122 (6):2032–45. doi: 10.1172/jci60132.
- Yao, H., and I. Rahman. 2012. Perspectives on translational and therapeutic aspects of SIRT1 in inflammaging and senescence. Biochemical Pharmacology 84 (10):1332–9. doi: 10.1016/j.bcp.2012.06.031.
- Yeboah, J. 2018. Review: More-intensive vs less-intensive LDL-cholesterol lowering reduces mortality. Annals of Internal Medicine 169 (2):Jc6. doi: 10.7326/acpjc-2018-169-2-006.
- Yoo, H., S. K. Ku, T. Lee, and J. S. Bae. 2014. Orientin inhibits HMGB1-induced inflammatory responses in HUVECs and in murine polymicrobial sepsis. Inflammation 37 (5):1705–17. doi: 10.1007/s10753-014-9899-9.
- Yoo, J.-Y., T. H. Kim, A. T. Fazleabas, W. A. Palomino, S. H. Ahn, C. Tayade, D. P. Schammel, S. L. Young, J.-W. Jeong, and B. A. Lessey. 2017. KRAS Activation and over-expression of SIRT1/BCL6 Contributes to the Pathogenesis of Endometriosis and Progesterone Resistance. Scientific Reports 7 (1):6765. doi: 10.1038/s41598-017-04577-w.
- Yu, B., Y. Jiang, B. Zhang, H. Yang, and T. Ma. 2018. Resveratrol dimer trans-ε-viniferin prevents rotaviral diarrhea in mice by inhibition of the intestinal calcium-activated chloride channel. Pharmacological Research 129:453–61. doi: 10.1016/j.phrs.2017.11.016.
- Yu, Y., M. Li, X. Huang, W. Zhou, T. Wang, L. Zhu, C. Ding, Y. Tao, H. Bao, and X. Cheng. 2020. A U-shaped association between the LDL-cholesterol to HDL-cholesterol ratio and all-cause mortality in elderly hypertensive patients: A prospective cohort study. Lipids in Health and Disease 19 (1):238. doi: 10.1186/s12944-020-01413-5.
- Yuan, Y., S. Ma, Y. Qi, X. Wei, H. Cai, L. Dong, Y. Lu, Y. Zhang, and Q. Guo. 2016. Quercetin inhibited cadmium-induced autophagy in the mouse kidney via inhibition of oxidative stress. Journal of Toxicologic Pathology 29 (4):247–52. doi: 10.1293/tox.2016-0026.
- Yuan, Z-p., L-j Chen, L-y Fan, M-h Tang, G-l Yang, H-s Yang, X-b Du, G-q Wang, W-x Yao, Q-m Zhao, et al. 2006. Liposomal quercetin efficiently suppresses growth of solid tumors in murine models. Clinical Cancer Research 12 (10):3193–9. doi: 10.1158/1078-0432.Ccr-05-2365.
- Yáñez, M., N. Fraiz, E. Cano, and F. Orallo. 2006. (−)-Trans-epsilon-viniferin, a polyphenol present in wines, is an inhibitor of noradrenaline and 5-hydroxytryptamine uptake and of monoamine oxidase activity. European Journal of Pharmacology 542 (1-3):54–60. doi: 10.1016/j.ejphar.2006.06.005.
- Zhang, Z., S. L. Pereira, M. Luo, and E. M. Matheson. 2017. Evaluation of blood biomarkers associated with risk of malnutrition in older adults: A systematic review and meta-analysis. Nutrients 9 (8):829. doi: 10.3390/nu9080829.
- Zhao, H., T. Ma, B. Fan, L. Yang, C. Han, J. Luo, and L. Kong. 2016. Protective effect of trans-δ-viniferin against high glucose-induced oxidative stress in human umbilical vein endothelial cells through the SIRT1 pathway. Free Radical Research 50 (1):68–83. doi: 10.3109/10715762.2015.1108412.
- Zhou, L., D. Y. Xu, W. G. Sha, L. Shen, G. Y. Lu, X. Yin, and M. J. Wang. 2015. High glucose induces renal tubular epithelial injury via Sirt1/NF-kappaB/microR-29/Keap1 signal pathway. Journal of Translational Medicine 13:352. doi: 10.1186/s12967-015-0710-y.
- Zhou, S., X. Tang, and H. Z. Chen. 2018. Sirtuins and insulin resistance. Frontiers in Endocrinology 9:748. doi: 10.3389/fendo.2018.00748.
- Zhu, Y., E. J. Doornebal, T. Pirtskhalava, N. Giorgadze, M. Wentworth, H. Fuhrmann-Stroissnigg, L. J. Niedernhofer, P. D. Robbins, T. Tchkonia, and J. L. Kirkland. 2017. New agents that target senescent cells: The flavone, fisetin, and the BCL-X(L) inhibitors, A1331852 and A1155463. Aging 9 (3):955–63. doi: 10.18632/aging.101202.