Abstract
Reducing salt in food without compromising its quality is a huge challenge. Some review articles have been recently published on saltiness perception in some colloidal systems such as emulsions. However, no published reviews are available on saltiness perceptions of gel-based matrices, even though salt release and perception in these systems have been extensively studied. This article reviews the recent advances in salt perception in gel-based systems and provides a detailed analysis of the main factors affecting salt release. Strategies to enhance saltiness perception in gels and emulsion-filled gels are also reviewed. Saltiness perception can be improved through addition of biopolymers (proteins and polysaccharides) due to their ability to modulate texture and/or to adhere to or penetrate through the mucosal membrane on the tongue to prolong sodium retention. The composition of the product and the distribution of salt within the matrix are the two main factors affecting the perception of salty taste. Food structure re-design can lead to control the level of interaction between the salt and other components and change the structure, which in turn affects the mobility and release of the salt. The change of ingredients/matrix can affect the texture of the product, highlighting the importance of sensory evaluation.
Introduction
Worldwide, elevated blood pressure causes 7.6 million deaths (49% of heart disease and 69% of strokes) annually (Lawes, Vander Hoorn, and Rodgers Citation2008). The World Health Organization (WHO) action plan globally for 2013–2020 suggested a 30% reduction in population’s intake of sodium (WHO, Citation2013). The production of healthier foods with reduced salt is a major challenge within the food industry. Preference is one of the major hurdles to salt reduction, reducing sodium content results in less favorable foods, people habituated to such a diet rapidly notice the difference. The function of sodium in foods is not only to provide nutrition and to deliver the salty taste but also to act as a processing aid, control water activity, enhance food flavor and help in texture creation (Busch, Yong, and Goh Citation2013). To achieve factual sodium reduction and viable healthier options for consumers, these complex multidimensional functions need to be achieved together. The United Kingdom was successful in lowering salt intake by 1 g/day between 2003 and 2008 via a combination of initiatives including education, legislation, labeling, and product reformulation (Webster et al. Citation2011). However, such methods are always not efficacious.
Even though numerous approaches have been investigated as strategies for salt reduction in foods, further reduction is still needed, as the mean salt consumption globally continues to be above the quantities recommended by the WHO (5 g/day). summarizes promising investigated strategies to reduce salt and some of their limitations.
Table 1. Summary of some of the investigated strategies (A) and their limitations (B) to reduce salt (NaCl) in food products.
An outstanding fact about sodium perception is that some foods such as cheese, condiments, and sauces may contain as much sodium as sea water however, those products are undoubtedly not tasting as salty. This might be a result of taste–taste interactions and the food matrix complexity. Product reformulation to reduce salt might not be an easy task as it involves many factors, such as the interactions between food components (fat, protein, and carbohydrate), sensory attributes (viscosity, texture, and taste/flavor), and how they affect saltiness perception which might be synergistic or antagonistic by increasing or decreasing sodium mobility. Increasing sodium mobility could boost its release in saliva throughout consumption, which leads to an enhanced salty perception.
The food industry is making decent development to reduce salt in bakery products (the largest supplier of sodium) (Ferrari et al. Citation2022); however, significant challenges continue in liquid and semisolid savory products such as sauces and soups (Agarwal et al. Citation2015). Simply because sodium is soluble in water. To the extent that sodium is concerned, the key food products for sodium intake are dairy, bakery, meat, and ready-to-eat foods, which represent around 50% of the salt consumption in industrial countries while the use of table salt contributes to 10–20% of the total salt consumed. Solid lipoproteic colloid (SLC) foods, such as sausage and cheese, consist of an oil‐in‐water emulsion of lipid and protein and contribute a substantial amount of salt to modern diets.
There are three main principles for sodium reduction in foods: (1) chemical stimulation to increase the saltiness perception peripherally, (2) cognitively increasing consumer awareness or shifting the saltiness preference, and (3) structural food design to optimize the salt delivery to the taste buds (Busch, Yong, and Goh Citation2013).
Modification of physical properties in liquid and semisolid products may enhance saltiness perception. These include viscosity, salt distribution, and the use of inert fillers that concentrate sodium in the aqueous phase such as in the case of emulsion systems (Busch, Yong, and Goh Citation2013). Engineering the food structure to enhance sodium release has been wished for as one key strategy for sodium reduction in processed foods (Stieger and van de Velde Citation2013). Although these synergistic/antagonistic effects have been investigated individually in-depth, few studies tested sodium reduction in a complex food product as affected by multiple antagonistic/synergistic factors.
A recent review article has been published on saltiness perception in some colloidal systems such as emulsions (Wang et al. Citation2021). However, no published reviews are available on saltiness perception of gel-based matrices, including liquid and semisolid foods. This article reviews the recent advances in salt perception in gel-based systems (i.e., gels and emulsion gels), the main factors affecting salt release, and strategies to enhance saltiness perception in food systems using gels and emulsion-filled gels (EFGs).
Saltiness perception
Saltiness perception is influenced by the mobility of sodium salt and possible release during mastication (Kuo and Lee Citation2014a; Okada and Lee Citation2017). Understanding food structure design (formation) and rheological/textural response to applied stress (deformation) may help designing food products with enhanced salt release and saltiness perception. Altering food structural design can affect sodium distribution and release by altering intermolecular interactions. As an example, emulsion gel microstructure was possibly modified by altering the formulation and processing parameters for enhanced sodium release during large deformation, affecting saltiness perception (Kuo and Lee Citation2014a). Protein matrix which is highly compact/ordered may adversely impact the saltiness perception by enhanced protein–salt interaction which results in sodium being unavailable (Okada and Lee Citation2017).
It is known that saltiness perception is very complex, involving aspects related to the traveling of sodium in the oral cavity, the influx of sodium from the tongue surface into the taste receptor cells (TRCs) and subsequent cognitive transduction of signal responsible for saltiness generation (Kuo and Lee Citation2014b). is a graphical illustration for taste signal transmission between tongue and brain. However, all the discussions presented here highlight the importance of investigations regarding the first stage of this entire process (i.e., migration of sodium from the solid foods to surroundings) and the enormous number of ways through which the gelled matrices may be altered to improve sodium release, and, consequently, to allow salt reduction in such important products.
Figure 1. Taste signal transmission from the tongue to the brain depicted in a schematic diagram (adapted from Ahmad and Dalziel Citation2020). Taste receptor cells (TRC) are found in taste buds located in various papillae of the tongue and mouth. TRCs receive the signal, and then, send it to the brain through the nucleus solitarius in medulla, which then transfer it via thalamus to gustatory cortex. The right side illustrates the tongue with the taste buds distributed on its surface. The taste bud on the left side displays taste TRCs and a simplified taste receptor signal transduction pathway.
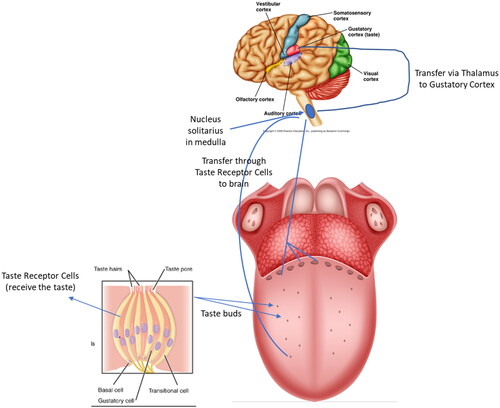
Gels and emulsion gels: an overview
Gels and EFGs have been arousing the interest of researchers and industry, as they are an alternative to reduce the lipid content in food (fat replacers) without significantly compromising product quality (Oliver, Scholten, and van Aken Citation2015). Such a fact is because these matrices may be produced using a wide range of biopolymers (especially proteins and polysaccharides), surfactants and processing conditions, and present, therefore, different properties (structural, rheological, and textural) and different responsiveness to environmental stresses (e.g., pH, temperature, enzyme, salt) (Mao et al. Citation2020).
Gels can be defined as semisolid systems consisting of a liquid phase entrapped in a three-dimensional network, which are known for their high nutritional value, excellent biocompatibility and good stability (Mao et al. Citation2020). Due to their unique combination of rigidity and elasticity, these systems are important for providing desirable textural and rheological properties to several food products. Among the several types of gelled food products, there are gelatins, dairy desserts, puddings, yoghurts, fresh cheese and sausages. In fact, some of these products are referred to as emulsion-filled gels (EFGs) (Mao et al. Citation2020).
Proteins can form three-dimensional networks which result in a gelled structure, intermediate phase between solid–liquid phases, which can retain low molecular weight molecules (Han et al. Citation2009). Gels can be formed by means of different mechanisms that underlie gelation (Ben-Harb et al. Citation2018). First, protein thermal treatment unfolds globular native compact structure, which exposes the buried hydrophobic residues in the structure and results in the subsequent aggregation of denatured proteins. Second, cold gelation can be performed through direct acidification using agents such as glucono-delta-lactone (GDL) or by using lactic starter cultures (Lucey and Singh Citation2003). Finally, enzymes are also used to produce protein gel networks through different mechanisms (Horne and Lucey Citation2017).
Emulsion gels (EGs) are soft solid-like materials generated from a stable liquid-like emulsion by gelation of the continuous aqueous phase. EGs can provide the advantages of both emulsions and gels and show improved emulsion stability against coalescence and creaming (Torres, Murray, and Sarkar Citation2016). EGs are also known as gelled emulsions, and according to the state of the oil droplets in the matrix, they can be classified in two categories: emulsion-droplet gels (also known as oemulsion-filled gels (EFGs)) and emulsion droplet-aggregated gels (also known as emulsion particulate gels) (Lin, Kelly, and Miao Citation2020). The focus of this review is on EFGs. EFGs contain both hydrophobic material (dispersed oil phase) and hydrophilic material (gel protein network) which allows them to be used for co-encapsulation of both hydrophilic and hydrophobic molecules with different solubilities. EFGs have the potential to deliver both hydrophobic and hydrophilic ingredients simultaneously (Mao et al. Citation2020). EFGs are increasingly used in pharmaceutics, cosmetics, and food industries (Farjami and Madadlou Citation2019). EFGs are a very complex biopolymer system, which consist of emulsion droplets or lipid particles entrapped in a gelled matrix (). Their characteristics are defined by the properties of the gelling agents, dispersed fillers, and the interactions among them (Sala et al. Citation2009; Geremias-Andrade et al. Citation2016).
Figure 2. Schematic representation of the two types of emulsion gels: (A) emulsion droplet-filled gels, and (B) emulsion droplet-aggregated gels (adapted from Lin, Kelly, and Miao Citation2020).
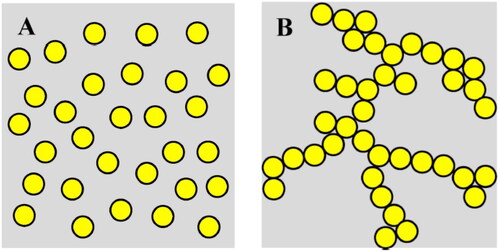
Factors that affect saltiness perceptions in gel systems
Effect of chemical composition on saltiness perception
During mastication, salt needs to be released from the matrix of the product into saliva before saltiness can be perceived. The composition of the food matrix has a significant role on sodium release and saltiness perception (Kuo and Lee Citation2014b). Most food systems are vastly complex and heterogeneous: they contain a combination of numerous elements such as lipids, proteins, carbohydrates, water, salts, and other micronutrients (Summarized in ). The complexity of the food matrix and possible interactions with sodium might create enormous diversity of probable final sensory effects (Kuo and Lee Citation2014b).
Table 2. Effect of food composition on saltiness perception.
In their study, Lauverjat et al. (Citation2009) found that in model cheese, higher diffusion coefficients of NaCl were obtained when cheese had higher NaCl and fat and lower dry matter and firmness. Besides cheeses, sausages are also important EFGs for the food industry, which represent significant sources of sodium. For this reason, different strategies have been addressed to increase sodium release in such products, including the incorporation of polysaccharides (Stieger Citation2011) and variations of compositions (Ruusunen, Simolin, and Puolanne Citation2001; Chabanet et al. Citation2013). For example, Chabanet et al. (Citation2013) evaluated the influence of varying protein, lipid, water, and salt contents on temporal sodium release in chicken sausages. The authors verified that the rheological properties of the products were especially affected by their water and fat contents, which influenced the salt release. According to their data, the higher the fat content, the lower the level of sodium release, probably due to a slowing of mass transfer of sodium in the matrix. Water content, conversely, had little or no effect on sodium release in saliva. As expected, the effect of the salt level on sodium release was positive and highly significant (Chabanet et al. Citation2013).
Effect of salt content
The amount of the added sodium salt is important to determine the saltiness perception in food systems. When sodium salt is added to a high quantity, sodium ions will bind to the food matrix until it reaches an equilibrium. In their study, Boisard et al. (Citation2013) using 23Na nuclear magnetic resonance (NMR) spectroscopy, reported that adding low salt level (0.5% NaCl) resulted in lower sodium mobility supporting the hypothesis that below 1.5% of NaCl, sodium binds to the matrix until it reaches a “binding” or saturation. However, higher concentrations of NaCl (1.5 − 3.5%) had no further effect on the overall sodium mobility. This was attributed by the authors to the system possibly being in a highly mobile state, beyond “binding” saturation. This is consistent with previous literature findings that reported increased sodium mobility as salt concentration increased, then leveled off in relaxation times with increasing added sodium (Gobet et al. Citation2009). Similarly, in a chicken soup broth containing xanthan gum (0.3% w/v), sodium mobility was constant at sodium concentration between 75 and 100 mg total Na per serving (240 mL), then increased until around 150 mg, then, remained fairly constant until up to 500 mg (Rosett et al. Citation1996).
It is also worth mentioning that the concentration of sodium salt affects food functionality. Zhang et al. (Citation2017) investigated the swelling power and rheological properties of waxy, normal, and high amylose maize starch at various salt concentrations. The swelling power decreased with increasing salt concentration from 0% to 2.0% among all starch samples. In addition, the gelatinization temperature of waxy starch and normal starch increased. It was found that high amylose starch had the most initial salinity and in-mouth saltiness intensity, as well as the largest thickness, lubrication, and stickiness.
Effect of fat/oil content
Although fats/oils appear to be tasteless by themselves, they contribute to food texture, mouthfeel, and chemosensory attributes through interaction with other food components. There are different mechanisms through which fat/oil concentration in emulsion systems can influence saltiness perception, either by enhancing or suppressing it.
Oil can suppress saltiness by acting as a barrier between sodium and taste receptors/buds through mouth coating and limiting sodium mobility/migration decreasing the perceived saltiness (Hughes, Cofrades, and Troy Citation1997). Oil can also increase the viscosity of foods, affecting the diffusion coefficients and retention times of sodium in the mouth (Malone, Appelqvist, and Norton Citation2003). Le Révérend, Norton, and Bakalis (Citation2013) using mathematical models attributed incomplete boosting of sodium perception at high fat contents to the high viscosity of the matrix which slows sodium transport to the taste receptors.
High fat/oil content was also reported to increase the sodium content in the aqueous phase enhancing saltiness perception (Koriyama et al. Citation2002; Malone, Appelqvist, and Norton Citation2003; Kuo and Lee Citation2014a). The increase in the perceived saltiness was reported by most of the studies to be due to increase the effective sodium content by increasing fat content because there was less water to dissolve the same amount of salt (Malone, Appelqvist, and Norton Citation2003), which is known as the “filler effect.” Koriyama et al. (Citation2002) assumed that oils and fats occupy volume in emulsions (no NaCl or KCl molecules), and thus, a 100% of sodium gets partitioned in the aqueous phase. Mattes (Citation2009) suggested that fat/oil have certain fatty acids which can enhance saltiness through sensitizing the sodium TRCs. In canola O/W emulsions with up to 40% oil content, saltiness was more intense and perceived using electronic‐tongue in emulsions than solutions, representing a saltiness‐enhancing effect caused by oil (Torrico and Prinyawiwatkul Citation2015). Suzuki et al. (Citation2014) reported that oil may contribute to coating the mouth tongue and retard the “washing out,” especially with liquid and semisolid foods, causing an increased saltiness perception.
It is worth mentioning that the effect of fat/oil content on saltiness perception is concentration dependent. Studies reported that fat content can improve saltiness through the filler effect up to a certain level of fat in the emulsion. However, above this concentration, saltiness might be suppressed through other mechanisms such as viscosity and texture. In their study to incorporate sodium caseinate O/W emulsions with 20–30% oil concentrations in soup, Lima et al. (Citation2018) found that the greatest enhancement of the saltiness perception was achieved with the addition of low/moderate fat concentrations (1–10%), what would be expected of the filler effect, and that above 20% the increase in saltiness was around half what is predictable from the filler effect.
Effect of carbohydrates
A surprising remark made by Lima et al. (Citation2018) in the study mentioned in the previous section was that lactose/glucose syrup existing in the marketable creamer suppresses saltiness perception with a strong correlation when applied in soup, with lactose having greater suppression. However, maltodextrin had no suppression effect on saltiness. A plausible rationale for the diminished perception of salt in the context of lactose/glucose could be the interaction between sweetness and saltiness (taste–taste interaction), resulting from a significant sweetness imparted by both lactose and glucose syrup. The authors also claimed that the joint effect of sweetness and viscosity might be the reason why thick salsas are moderately salty even though they have salt content similar as sea water. A suggestion made by the authors to improve saltiness perception and to have the right amount of salt within the WHO dietary recommended daily intake would be balancing sweetness and product texture.
In another study by Frankowski, Miracle, and Drake (Citation2014), an investigation was carried-out to ascertain the sensory and compositional characteristics of whey permeates and ascertain whether components other than sodium are responsible for the saline flavor of permeate. 18 whey (n = 14) and 4 reduced lactose (n = 4) permeates were procured from commercial facilities in duplicate. Lactose-free permeates had a very noticeable saltiness. Sensory analyses using sodium chloride solutions verified that the presence of sodium was not the only reason why reduced lactose permeates tasted salty. Other compounds including NaCl, KCl, lactic acid, citric acid, hippuric acid, uric acid, orotic acid, and urea were used to make permeate models and also contributed to the salty taste in addition to NaCl.
Effect of moisture content
Saltiness is formed by electrophysiological signaling from the tongue to the brain triggered by the sodium ions absorbed by ion channels on the tongue (Gilbertson, Damak, and Margolskee Citation2000). Therefore, a mobile aqueous environment is needed for sodium ions to be transported to the ion channels. High moisture content resulted in enhanced saltiness in lipoprotein matrices (Lawrence et al. Citation2012) which has been attributed by Chabanet et al. (Citation2013) to the facilitated sodium extraction from the food matrix which in turn results in high salivation. As previously mentioned, most of the sodium ions are washed away in liquid foods without reaching ion channels on the tongue surface, and therefore, do not contribute to saltiness. In case of low moisture foods such as food powders, salt is normally entrapped in the food matrix and for this reason powdered foods need to be rehydrated first to liberate sodium ions to the aqueous phase before being transported to the ion channels on the tongue to start the signaling (Yucel and Peterson Citation2015).
It is noteworthy that formulation of food products with salt gradients using the compartmentalized approach, as discussed here before, is less challenging for low moisture foods, such as bread, which has been successfully applied (Noort et al. Citation2010). The reason is that diffusion/migration of salt from the inner phase to the external phase by osmotic pressure is restricted. Another composition feature important for sodium release in solid lipoproteic colloids (SLCs) is the water content. According to Lawrence et al. (Citation2012), higher water content represents higher solvating capacity of sodium and, therefore, higher release rates.
Sodium location in gel system and its effect on saltiness perception
Inhomogeneous sodium distribution
There are certain solid and semisolid gelled foods that can be reduced in salt content by inhomogeneous spatial distribution of salt within the matrix of the food, such as breads, cheese, and sausage (Sinesio et al. Citation2019). These could be made by sheeting alternating layers with different salt concentrations and layer thicknesses. Fahmy et al. (Citation2021) investigated the effects of inhomogeneous NaCl distribution by 3D printing utilizing hydrated wheat starch-egg white powder combinations in alternating layers with various configurations. The results showed that if the sensory contrast was great enough and the overall NaCl concentration was low, the inhomogeneous spatial sodium chloride distribution led to an increase in saltiness. Unfortunately, implementing this technology on a broad scale to produce low-salt foods is a tough task. is an illustration of the effect of sodium distribution on saltiness perception.
Figure 3. Schematic representation of the impact of sodium distribution within the gel matrix on saltiness perception.
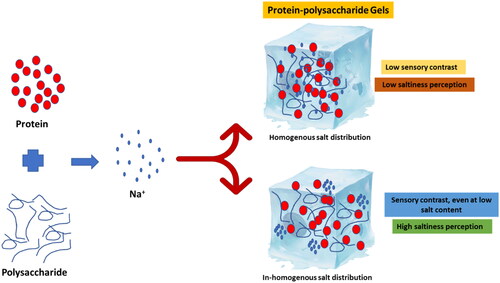
Previous research found that the salt enriched soy protein isolates/gum Arabic coacervate could be utilized to prepare food gels with inhomogeneous salt distributions, allowing for a 30% reduction in salt content while maintaining flavor and textural properties (Li et al. Citation2020). It is worth noting that despite the inhomogeneous salt distribution in a food matrix, preparation, subsequent cooking, storage, and last-minute reheating contributed to sodium migration and homogeneity loss (Emorine et al. Citation2013).
Sodium–biopolymers interactions
Protein interactions, explicitly the ionic form, with sodium were reported to influence saltiness perception and to give a basis to create higher flavor quality low-sodium foods. In complex food systems, charged segments of biopolymers can interact electrostatically with sodium, although is preferred for processing, it affects sodium release and its transport to the taste buds on the tongue (Kuo and Lee Citation2014b). For example, casein in cheese structure can bind sodium in the matrix limiting its migration and availability in the mouth (Lauverjat et al. Citation2009). Ruusunen, Simolin, and Puolanne (Citation2001) produced Bologna type sausages with 71% fat reduction and replacement with lean meat and water and reported that saltiness was lowered when lean meat was used. They attributed this to the ionic interaction between lean meat protein and sodium which limited sodium availability and its perception.
Boisard et al. (Citation2013) used 23Na NMR spectroscopy to study the sodium ions mobility in cheese as affected by protein and NaCl content. It was concluded that higher mobility of sodium was declared in cheeses with a lower protein content. Okada and Lee (Citation2017) explored the impact of different levels of NaCl, protein, and lipid and high-pressure homogenization (HPH) on sodium availability in a model heat-set EFGs as an approach for sodium reduction. Conversely, salt content and HPH treatment had no significant impact on sodium mobility. A mechanism was suggested by the authors for the negative impact of higher protein concentrations on saltiness perception, that is, the reduced water availability in a dense protein network as transportation medium for aqueous sodium. In a study by Kuo and Lee (Citation2014a) the saltiness perception in SLCs, as a model emulsion system, with 8% protein was more perceived than SLCs with 16% protein.
Saltiness perception will differ if sodium salt binds to a pure protein solution or if fat is added and the protein is on the oil interface. In their study, Yucel and Peterson (Citation2015) explored the impact of interaction between sodium and protein (sodium caseinate), used either separately or to emulsify oil. It was reported that increasing the protein content in the aqueous protein solutions resulted in significantly lower saltiness and a higher salty aftertaste; however, when casein was used to formulate an emulsion, the saltiness perception was improved. This was attributed by the authors to the increase in the interfacial area of the emulsion, exposing more hydrophilic segments of the protein toward the aqueous phase, resulting in enhanced saltiness. They concluded that such protein structure in these emulsions, when in a powder form, would enhance the powder rehydration and control the perception of saltiness. Casein on the oil interface forms as a hydrophilic protein layer, which can diffuse up to 10 nm from the particle surface (Leermakers et al. Citation1996) which is anticipated to enhance the sodium availability. Similarly, Lawrence et al. (Citation2012) reported that sodium ions bind to anionic liposome matrixes, enhancing salt release and consequently the salty taste in cheese products.
Regarding mixed biopolymeric gels, the degree of coarseness and microphase separation have shown to influence serum release. According to some authors, heterogeneous, bi-continuous, or coarse stranded gels produced with mixtures of proteins and polysaccharides tend to present higher serum release, and, consequently, resulting in salt release and saltiness perception, in comparison to homogeneous or protein-continuous microstructures (Stieger Citation2011; Stieger and van de Velde Citation2013; Kuo and Lee Citation2014a).
Porosity, microstructural organization and, therefore, sodium release, may be altered through variations of protein concentration in gelled systems. However, the impacts of such variations over salt release are not only related to the porosity/permeability, but also to sodium–protein interactions (Kuo and Lee Citation2014a). According to the literature, increases of protein concentration in SLC gels reduces the amount of free sodium and, therefore, may reduce the salt release (Ruusunen, Simolin, and Puolanne Citation2001; Lauverjat et al. Citation2009; Boisard et al. Citation2013; Kuo and Lee Citation2014a). In their study, Okada and Lee (Citation2017), for example, verified that the higher the protein and fat contents, the lower the total mobility of sodium inside SLC gels (measured by single quantum – SQ – experiments). According to these authors, such phenomenon occurs because such alterations increased the structure surrounding bounded sodium as well as increased the amount of sodium in a “bounded state” (measured by double quantum-filtered – DQF – experiments), due to increased interfacial protein interactions.
According to the literature, the release of salt from gelled matrices depends on many factors including the breakdown characteristics, the amount of water in the systems and the interactions among salt and other molecules in the structures (Phan et al. Citation2008; Kuo and Lee Citation2014b). In general, dry and hard gels require more mastication for the breakdown and adequate salt release (Phan et al. Citation2008). Besides, sodium–polymer interaction may reduce the sodium availability and affect the concentration gradient, which is determinant for sodium liberation (Kuo and Lee Citation2014b).
The high impact of high protein concentrations on the saltiness perception has been related by several studies to the following reasons: (1) The ionic interaction between sodium and protein could lower sodium release; as reported in cheese systems (Lauverjat et al. Citation2009; Boisard et al. Citation2014) and processed meat products (Ruusunen, Simolin, and Puolanne Citation2001); (2) increased protein content slows down sodium diffusion, due to the increased tortuosity of the protein network within the gel, which makes the texture more rubbery and less brittle (Lauverjat et al. Citation2009); (3) high protein concentration lowers water content which could decrease serum release during the mastication, decreasing the convective transfer rate for sodium release (Kuo and Lee Citation2014a, Citation2014b). From the discussions presented here, it is clear that exploring molecular interactions and microstructural aspects is a valuable tool to increase salt release and decrease the sodium in such food products, however, it is also known that the saltiness perception is more complex and involves many other aspects.
Environment around sodium in gel matrix
The sodium environment in food matrix is one of the most essential properties that confer flavor, saltiness, and sweetness release and can be reflected by food structure and rheology. Modifying the food structure can enhance saltiness perception, increase the sodium diffusion coefficient, and result in a higher degree of fragmentation (Kuo and Lee Citation2014b).
Rheology of a polymer solution may adversely impact saltiness perception due to the slowdown of mixing/mass transportation of sodium ions across the boundary coatings on the tongue (Le Révérend, Norton, and Bakalis Citation2013; Aubert, Lima, and Le Révérend Citation2016). For example, in a solution of a biopolymer with a concentration above the entanglement concentration (c*), aroma or taste perception was greatly lowered (Baines and Morris Citation1987). The viscosity and the efficiency of mixing of the product with saliva, would explain this decrease in perception (Koliandris et al. Citation2008). Tarrega et al. (Citation2011), in an initiative to study the panellists’ mastication activities as related to model cheese texture (low fat vs. full fat), low fat samples were harder and needed higher amount of incorporated saliva, higher chewing work per cycle, and number of chewing strokes.
Food thickness was found to have a negative effect on sweetness in semisolid dairy desserts (Tournier et al. Citation2009), but the researchers were not able to decide whether this was solely caused by physicochemical interactions or also perceptual interactions have taken part. It was previously mentioned that sodium mobility and sodium binding to the matrix are important factors affecting saltiness perception. A recent study investigated the impact of protein matrices (milk protein, gelatin, and soy protein) at concentrations of (2.5 and 9% w/w), and pH levels of (5.5, 6.2, and 6.8). The authors suggested that rheological behavior had a larger effect on saltiness perception than ionic binding and sodium mobility (Mosca et al. Citation2015; Defnet, Zhu, and Schmidt Citation2016).
Rheological properties, especially rupture/fracture parameters, are commonly associated to the behavior of gelled systems during mastication and they are essential for the comprehension of sodium release. Koliandris et al. (Citation2008), for example, investigated the relation between sodium release and microstructural organizations of low-/high-acetyl gellan gels and κ-carrageenan/locust bean gum gels, verified that systems with more of smaller fragments at rupture exhibited significantly higher release of sodium. According to the authors this was due to the increased surface area following fracture during chewing. Therefore, an important conclusion was that the intensity of flavor perception in gels was dominated by the release of salt, which was favored by a low strain at break.
It is known the microstructural organization of mixed biopolymeric gels are a result of the balance between the gelation and the phase separation processes, which depend on the properties of the ingredients and the processing conditions (de Jong, Klok, and van de Velde Citation2009). Therefore, the sodium release of these systems may be adjusted through the adequate selection of ingredients, and the control of the gelling conditions by the application of microscopy techniques and rheology tests (Stieger Citation2011; Stieger and van de Velde Citation2013; Kuo and Lee Citation2014a).
Strategies to enhance saltiness perception in food systems using gels and emulsion-filled gels
Many approaches have been investigated for sodium reduction in gelled matrices, including the use of salt replacers (Carmi and Benjamin Citation2017) and application of aroma–taste interaction strategy (Thomas-Danguin et al. Citation2015). However, one of the most promising alternatives for the reduction of salt in food products consists in promoting the efficient sodium release from a gelled structure, considering that 70% to 95% of sodium could still remain within the matrix right before being swallowed, not contributing for saltiness generation (Phan et al. Citation2008; Busch, Yong, and Goh Citation2013; Kuo and Lee Citation2014a, Citation2014b). Important studies regarding SLC gels, including the ones shown in , revealed that the major mechanisms of sodium release during the breakdown of such systems are via serum release (governed by the gel porosity) and sodium diffusion (governed by particle size of fat), which may be modulated especially by composition and process variations (Kuo and Lee Citation2014b; Mosca et al. Citation2015; Kuo and Lee Citation2017; Okada and Lee Citation2017). Most of the authors state that higher serum releases (i.e., sodium releases) are obtained from more porous and coarse stranded gels, especially due to the hydraulic permeability, which, according to the literature, describes the ease of fluid transport through pore spaces and is directly proportional to the square of the pore radius (Stieger and van de Velde Citation2013; Kuo and Lee Citation2014a). Even though increasing sodium release does not necessarily result in increases of saltiness perceived, as many investigations have shown great interindividual differences in flavor release and perception (Taylor Citation2002; Phan et al. Citation2008).
Table 3. Some studies investigating aspects related to sodium release from model gels and solid lipoproteic colloid (SLC) gels.
In most of these studies, protein and lipid contents of the samples were also altered and their effects over salt release were observed. Regarding protein content, the conclusions obtained were similar to the ones obtained from SLCs, therefore, increases in protein concentration generally decreased the availability of sodium, due to higher ionic interactions (Lauverjat et al. Citation2009; Boisard et al. Citation2014), as well as due to the increased tortuosity of the protein matrices (Kuo and Lee Citation2017).
In addition, alterations in fat content or particle size of fat also revealed to exert important effects over the extent of gel breakdown, and therefore, sodium release (Phan et al. Citation2008; Kuo and Lee Citation2014b). Previous studies verified that lower size of fat globules on model cheese made of rennet casein led to lower fracture stress and fracture energy and, higher concentration of sodium release (de Loubens et al. Citation2011a, Citation2011b; Phan et al. Citation2008; Boisard et al. Citation2013, Citation2014). Similarly, studies on the effects of fat/protein ratio in model cheeses verified that an increase in this ratio led to an increase in in vitro sodium release rate, in-mouth sodium release, and saltiness perception (Boisard et al. Citation2013, Citation2014). According to the authors, such results were related to the weaker structure formed and the higher sodium mobility, which were evidenced by lower stress at maximal deformation and higher NMR relaxation times of sodium.
Conversely, according to Kuo and Lee (Citation2014b) the process of saltiness perception in solid/gelled systems starts with the migration of sodium from the food matrix to surroundings (i.e., sodium release), which is mostly influenced by the initial availability of the sodium, its spontaneous diffusion and facilitated migration under matrix deformation. Due to this reason, several studies have investigating the relationship between sodium release and structural aspects of two kinds of “model foods”: (I) mixed gels of proteins and/or polysaccharides (Stieger Citation2011; Kuo and Lee Citation2014a), and (II) EFGs (Ruusunen, Simolin, and Puolanne Citation2001; Phan et al. Citation2008; Lauverjat et al. Citation2009; de Loubens et al. Citation2011a; Chabanet et al. Citation2013; Boisard et al. Citation2014; Kuo and Lee Citation2014a; Kuo and Lee Citation2017; Okada and Lee Citation2017). According to Stieger and van de Velde (Citation2013) these two food models represent the four major constituents of foods (i.e., protein, carbohydrate, fat, and water), so, they are extremely important and their behaviors are generally significant for the food industry.
Even though biopolymeric gels are very important for the food industry, the conclusions regarding these systems, referred by Kuo and Lee (Citation2017) as “nonfat model gel system,” may have limited implications for the development of lipoproteic products. For this reason, many studies found in the literature have been investigating the effects of variating the formulation and processing parameters on the microstructures of EFGs, and its consequence over the serum and sodium release (Kuo and Lee Citation2014a; Kuo and Lee Citation2017; Okada and Lee Citation2017). The EFGs investigated are mainly SLC gels (i.e., product prototypes) or model products, including cheeses and sausages, which are known as by their high sodium content.
Several strategies in the literature have been suggested in gel systems to reduce salt which are summarized in , and graphically presented in and detailed here below.
Figure 4. A graphical presentation of the strategies for maximization of saltiness perception through control of chemical composition and careful structure design in gel systems.
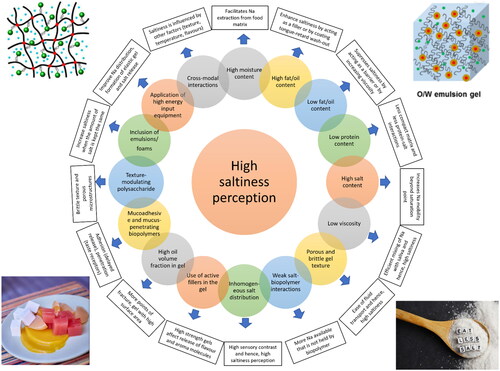
Table 4. Strategies for salt reduction using gels and emulsion-filled gels.
Using active fillers
Emulsion droplets can be classified according to the interactions they have with the biopolymer network, as active or inactive fillers. Active fillers can be defined as the droplets which interact with the gel structure due to covalent and/or noncovalent bonds. Conversely, inactive fillers are supposed to have none or very low level of interaction with the biopolymer network. The type of the filler in the EFGs is usually identified by uniaxial compression data. According to the literature, whereas the addition of inactive particles leads to a reduction in the value of Eg (elasticity modulus), an increase or decrease in the rupture strain (εH) and a decrease in the rupture stress (σH) of the gels, the addition of active particles can lead to an increase or decrease in the value of Eg, a decrease in εH, and an increase in σH (Oliver, Scholten, and van Aken Citation2015). Although this generalization exists and is widely accepted, the interpretation of the results of uniaxial compression tests of loaded gels is very complex, since the intensity of each of these effects depends on specific characteristics of the different loaded gels. Using octenyl succinic anhydride (OSA) starch-stabilized droplets as active fillers in starch gels, Torres et al. (Citation2017) obtained higher stabilized and high strength emulsion gels, which can affect the release of lipophilic flavor and aroma molecules.
Emulsion volume fraction in the gel
Salt is primarily dispersed in the water phase and/or at the interface of emulsion gels, but the oil phase can enhance or weaken the gel network, modifying salt diffusion, and release during food oral processing. In a larger volume emulsion system (higher gel strength), after a certain amount of salt is added, the release of salt will be significantly reduced (Zhang et al. Citation2021). Other authors (Koliandris et al. Citation2008; Kuo and Lee Citation2014a) affirmed that the increase of lipid dispersed throughout the protein networks results in more points of fracture upon compression, leading to gels with greater surface area after fracture and, therefore, with greater sodium release. Such different conclusions emphasize the complexity associated to the impact of fat concentration over salt release of EFGs/SLC gels, especially because it depends on the sizes of the particles/droplets dispersed throughout the matrices.
According to Chabanet et al. (Citation2013), fat, being a hydrophobic material, may form a barrier between NaCl and taste receptors, preventing sodium migration and resulting in a decrease in perception taste intensity. Conversely, salt was released at lower rates in the gels with higher volume of emulsions. An emulsion with a higher oil fraction displayed a more vigorous salty intensity than an emulsion with a lower oil fraction. This could be explained by that the oil droplets in emulsions could work as fillers and reduce the amount of water that salt can be dissolved in, resulting in an increase in the local concentration of NaCl (Malone, Appelqvist, and Norton Citation2003).
Addition of biopolymers
Addition of polysaccharides to modulate texture
More recently, another strategy to improve saltiness perception was addressed by Benjamin et al. (Citation2018). The authors investigated the influence of adding polysaccharides (kappa carrageenan (κ-CG), iota carrageenan, locust bean gum (LBG), and sodium alginate (SA) or low acyl gellan (LAG)) on texture and salt release of model fresh cheese. The amount of salt released by the cheese depends on both the ion mobility through the pores and the strength of the protein network. Salt reduction in cheese could be achieved by enhancing the salt release rate by modifying cheese structure with polysaccharides. According to the authors, such research provided an important step toward the utilization of polysaccharides to reduce salt content in dairy products (Benjamin et al. Citation2018). In additional, Wang, Feng, and Xia (Citation2021) investigated the effect of four common polysaccharides on the salt release and saltiness perception in surimi emulsified sausages. The κ-CG based sausage had the highest salt release and saltiness perception, due to the ability of κ-CG to form porous and interpenetrating network and promote hydrophobic interactions and formation of disulfide bonds. Interactions between polysaccharides and protein molecules result in differences in microstructure. An increase in the rate of salt release was observed with brittle texture and porous microstructures, and interpenetrating structure.
By understanding and controlling the microstructure of gels and EFGs it is possible to design and develop food products to support healthier lifestyles (Stieger and van de Velde Citation2013). According to Stieger and van de Velde (Citation2013), the best model foods to investigate the influence of the microstructure on the oral processing and texture perception of food products are the ones in which the microstructure and texture can be independently manipulated. In their comprehensive review about this subject, these authors state that two types of structures are especially suitable for such an investigation: EFGs and phase separated mixed gels of proteins and polysaccharides. Therefore, if they are adequate systems for the study of the influence of the microstructure on the taste and texture perceptions, they are also suitable for the conception of food structures which can be mimetic to fat or control salt release. de Souza Paglarini et al. (Citation2021) reported the use of inulin-based emulsion filled gel as a fat substitute in low-fat, low-salt meat products with a higher fiber content and a healthier fatty acid profile. However, the samples with salt and fat reduction simultaneously scored lowest in sensory evaluation. It is still necessary to improve those properties in the further research.
Due to these microstructure characteristics, the application of EFGs may also allow the controlled release and adsorption of different ingredients, including bioactive compounds and sodium (Brito‐Oliveira et al. Citation2017; Mao et al. Citation2020). The control of salt release and, consequently, saltiness perception, is a very promising alternative for the reduction of sodium in food formulations, which, as previously mentioned, is very desirable for the development of new systems in the food industry (Kuo and Lee Citation2014b). Salt reduction can make nonfilled gels softer and less elastic by altering their texture and structure. A softer gel may dissolve more quickly, resulting in a faster release of salt and a stronger perception of salt. In EFGs, oil droplets and water are held together by a protein matrix. The salt level of EFGs may impact emulsion stability, causing oil droplets to separate from the protein matrix. Oil droplets may interfere with the feeling of salt on taste buds, affecting the salt sensation. Furthermore, emulsion texture might alter salt perception, as smoother emulsions may breakdown faster and release salt more quickly.
Mucoadhesive and mucus-penetrative proteins and polysaccharides
Mucosal membranes cover several body regions which contain a mucus gel layer that represents a potential barrier for delivering active molecules (such as drugs) to the underlying epithelium (Lai, Wang, and Hanes Citation2009). Mucoadhesive and mucus-penetrative proteins and polysaccharides can work to enhance the taste of low-salt foods, either by adhering to mucosal surfaces and prolonging contact time (mucoadhesive biopolymers) or by penetrating the mucus layer and improving the interaction between the food and taste receptors (mucus-penetrative biopolymers). This can ultimately lead to a reduction in salt content in processed foods while maintaining the desired taste. For adhesion to occur, biopolymer molecules must bond to the tongue mucosal surface with the help of different chemical bonds with mucin (mucosal layer protein) such as ionic, covalent, hydrogen, van der Walls or hydrophobic bonds (Andrews, Laverty, and Jones Citation2009), thus, improving the retention time of the incorporated active substance and promoting a site-specific delivery (Sosnik, das Neves, and Sarmento Citation2014). summarizes the different mechanisms by which biopolymers can interact with the oral mucosa to help delivering sodium.
Figure 5. The different mechanisms by which biopolymers can interact with the oral mucosa to help delivering sodium.
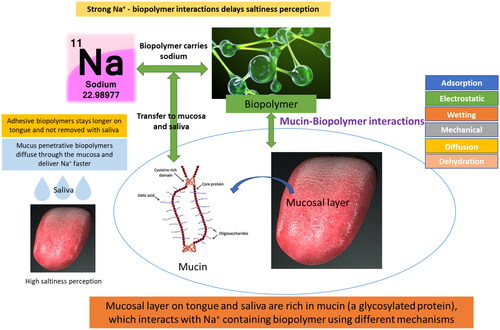
Various food grade polysaccharides are considered as mucoadhesives. Examples of mucoadhesive food grade polysaccharides include chitosan (cationic), [sodium carboxymethyl cellulose (CMC), gum arabic (GA), sodium alginate (SA), and pectin (PE)] (anionic) and [hydroxypropylmethylcellulose (HPMC), pullulan (PU), and dextran (DE)] (nonionic) (Patel et al. Citation2003; Richardson et al. Citation2004; Thirawong et al. Citation2007; Sogias, Williams, and Khutoryanskiy Citation2008; Yehia, El-Gazayerly, and Basalious Citation2009). The design of sodium or sugar containing biopolymer systems with high mucoadhesive ability can prolong the time these tastants are in contact with taste receptors on the tongue, thus, increasing saltiness perception.
In pharmaceutics, mucoadhesives can be incorporated into various formulations such as tablets, patches, films, sprays and viscous liquids containing an active pharmaceutical ingredient (API). However, mucoadhesives has not been fully exploited by the food industry as a mean of retaining small molecules/tastants, such as sodium or sugar as a strategy for improving saltiness, sweetness or flavor perception. CMC as a mucoadhesive polysaccharides, has been investigated as salt substitutes in various food products. Cook et al. (Citation2018) reported that CMC can prolong the adherence of the matrix to the mucosa; it also retains the model tastant, sodium, for a longer period than starch and water matrices. The release of flavor molecules from the matrix is influenced by the polysaccharide’s viscosity and swelling capacity. Chitin nanomaterials have the ability to adsorb negative ions, increasing the volume of free Na+ ions in the system (Taylor et al. Citation2019), and as a consequence, salty perceptions increases. Tsai et al. (Citation2019) discovered a beneficial connection between saltiness perceptions and the quantity, surface area, and degree of deacetylation value of chitin nanoparticles.
Relatively little is known about the mucoadhesive ability of amphoteric polymers such as proteins. It is believed that amphoteric polymers have poor ability to adhere to mucosal surfaces due to the self-neutralisation between cationic and anionic groups present within their macromolecules (Khutoryanskiy Citation2011). Mucoadhesion of milk proteins has been reported in a study (Withers et al. Citation2013) which investigated the binding of different milk proteins to distinct areas of the tongue in an attempt to explain negative sensory attributes such as drying. However, there is still a lot to explore in this area.
Mucus penetration (MP) shows a high spreading ability of the biopolymer over the mucosa and can penetrate deeper mucus regions, eventually reaching the underlying epithelium (Dünnhaupt et al. Citation2015; Yamazoe, Fang, and Tahara 2021). MP has emerged as a technique for engineering nanocarriers to overcome the mucus layer barrier in the gastrointestinal tract, thus significantly improving the bioavailability of therapeutics. Importantly, this finding can be used for food applications to improve saltiness and sweetness perception. It was reported that high amounts of GA enable a 30% reduction in the salt content of liquid and semisolid foods (yogurt drink and mayonnaise) through its mucus-penetrating ability without altering the saltiness perception (Li, Wan, and Yang Citation2019). The findings suggested that formulations containing GA could enhance the saltiness perception based on their MP property.
The use of mucoadhesive and mucus-penetrative proteins/polysaccarides as a strategy for reducing salt intake is still in the early stages, and further research is needed to fully understand their potential. This can enhance the taste perception of low-salt foods by improving the interaction between the food and the taste receptors, similar to how mucoadhesive proteins and polysaccharides work.
Inclusion of emulsions/foams in the dispersion
The presence of suspended colloids (emulsions/foams) within a liquid dispersion can either increase or decrease the perceived saltiness of a liquid food product (Malone, Appelqvist, and Norton Citation2003). The inclusion of emulsions/foams into a liquid dispersion is regularly found to increase saltiness perception when the total amount of salt in the product is kept the same. This was specially highlighted through the investigation developed by Chiu et al. (Citation2015), who evaluated the incorporation of air as a filler particle within hydrogels, produced with type B bovine gelatin and whey protein isolate, and its impact on the temporal delivery of salt and congruent aroma delivery in-nose, and its resulting impact on saltiness and overall flavor perception. The authors verified that the addition of air within hydrogels enhanced the delivery of sodium and perception of saltiness and allowed an 80% reduction in total sodium with no loss of saltiness perception. The conclusions revealed that air inclusions may be used as an alternative to increase both the delivery and perception of salt and aroma, and also increase overall flavor perception.
Application of high energy input equipment
Even though optimizing salt release is important for sodium reduction in meat preparations such as sausages, alterations in formulation for this type of product demand more effort, considering that salt is necessary during the processing to induce structural changes through electrostatic interactions between muscle proteins and sodium and chloride ions (Marchetti et al. Citation2015; Yang et al. Citation2015). In processed meat products, such as meat batters, salt is normally added at concentrations between 1.5% and 2.5% to improve solubility of myofibrillar proteins, increase water binding capacity, gelation, fat retention, and decrease the cooking loss (Ruusunen and Puolanne Citation2005; Desmond Citation2006). A major concern is that the reduction of salt concentrations in sausages may lead to decreases in extracted and solubilized myofibrillar proteins, affecting the functionality of the meat system (Marchetti et al. Citation2015).
In addition to above strategies, salt reduction can also be achieved through different processing methods. There are successful strategies to reduce salt in food products by high energy input equipment, such as ultrasound and high-pressure, and pulsed electric field (PEF) among others. In this context, the most recent studies involving low-sodium sausages focus on investigating the application of different techniques to increase the functional properties of such products, including, for example, high pressure processing (Bolumar et al. Citation2013; Tokifuji et al. Citation2013; Yang et al. Citation2015), and not necessarily finding alternatives to increase serum/sodium release, which is the focus of this section of the present review.
Yang et al. (Citation2015) studied the use of high-pressure processing to improve the functional qualities of reduced-salt (1%) sausage. At certain condition of high-pressure, the highest scores for sensory evaluation were observed even the salt content level was lower than normal. According to a previous study, high pressure accelerates the creation of salt bridges between myofibrillar proteins (Tokifuji et al. Citation2013), which may be due to high-pressure treatment’s capacity to induce and increase in myofibrillar protein solubilization as a result of partial protein depolymerization, aggregation, and unfolding, which results in the production of a more stable gel matrix (Sikes, Tobin, and Tume Citation2009).
Ultrasound has the potential to deliver higher salt perception even with lower overall salt content by improving salt distribution. Li et al. (Citation2015) reported that, compared with the control chicken meat batter (2% salt), ultrasound treatment for 20 min with reduced salt (1.5%) had not significant effect (p > 0.05) on texture, cooking loss or water holding capacity of chicken meat batter. Conversely, ultrasound technology significantly changed the gel network formation and gel elasticity to achieve salt reduction.
PEF is a novel technology in the food processing industry, PEF treatment improved saltiness by regulating salt diffusion and sodium delivery without affecting sensory quality, lipid oxidation and microbiological stability of the products, according to Bhat et al. (Citation2020). The PEF treatment has shown the potential to make changes to protein structure and function, modify product microstructure, and enhanced membrane permeability and mass transfer processes. The type of protein, product composition, and the relationship between instrument processing parameters and product properties are all important factors for salt release and saltiness perception, additional work needs to be performed.
Cross-modal interactions
Cross-modal interaction refers to the way in which different sensory modalities, such as sight, sound, taste, touch, and smell, interact and influence each other. In the context of salt release, cross-modal interaction refers to the way in which sensory information from multiple modalities is integrated to create a perception of the taste of salt. It plays an important role in the perception of saltiness and other flavors in food, and understanding these interactions can be important for creating foods that are both delicious and nutritious.
The perception of saltiness is not solely determined by the chemical composition of salt but is also influenced by factors such as texture, temperature, and the presence of other flavors. The texture and temperature of food can affect how readily salt dissolves and how it is distributed in the mouth, which can in turn affect the intensity and duration of the perception of saltiness. In addition, the presence of other flavors can interact with the perception of saltiness through a phenomenon called "flavor enhancement" which can be used as one of the strategies for reducing salt. Cook et al. (Citation2018) presented that aroma intensity is dependent on the perception of a congruent tastant. Texture-induced cross-modal interactions between taste and somatosensory cues appear to play a key role in reducing perceived taste impact, especially at lower polysaccharide concentrations or in the presence of solid particles (Scherf et al. Citation2015).
There are barriers to reducing salt in food through cross-modal interaction, particularly in gels. Salt helps to regulate the balance between attractive and repulsive forces between protein molecules, which affects the gelation process and the resulting texture and stability of the gel. However, there are also ways to overcome these barriers and reduce salt in gels while still maintaining their texture, stability, and flavor. For example, replacing some of the salt with other flavor enhancers, such as glutamates or nucleotides, can help to maintain the perception of saltiness and enhance other flavors in the gel. Alternatively, other techniques, such as adjusting the pH or incorporating different functional ingredients, can also be employed (Jimenez-Colmenero et al. Citation2010).
Conclusions and prospects
In gel systems, salt release is influenced by many factors including the chemical composition of the product matrix (e.g., the content of salt, fat/oil, carbohydrate and moisture) and the environment where the salt is located (e.g., Inhomogeneous distribution, salt–biopolymers interactions, rheological behavior, texture, and product matrix). In complex food systems, charged segments of biopolymers can interact electrostatically with sodium. While this interaction is preferred for processing, it affects the release of sodium and its transportation to the taste buds on the tongue. Saltiness perception is influenced by the mobility of sodium salt and possible release during mastication. The first stage of this entire oral processing (i.e., migration of sodium from the solid foods to surroundings) is crucial and there are enormous number of ways through which the gelled matrices may be altered to improve sodium release, and, consequently, to allow salt reduction in such important products. Many approaches have been investigated for sodium reduction in gelled matrices, including the use of salt replacers and application of aroma–taste interaction strategy. However, one of the most promising alternatives for the reduction of salt in such systems consists in promoting the efficient sodium release, considering that 70% to 95% of sodium could still remain within the matrix right before being swallowed, not contributing for saltiness generation. Understanding food structure design (formation) and rheological/textural response to applied stress (deformation) may help designing food products with enhanced salt release and saltiness perception. Altering food structural design can affect sodium distribution and release by altering gel porosity, microstructural organization, grade of fragmentation and intermolecular interactions. Several strategies have been applied to enhance saltiness perception in biopolymer gel systems through redesigning food structure. These include the use of different active fillers, controlling the oil volume fraction of EFGs, the use of biopolymers such as polysaccharides to modulate gel microstructure or as mucoadhesive or mucus penetrating to help prolong saltiness perception (mucoadhesion) or accelerate salt travel to taste buds (mucus penetration), the inclusion of emulsions/foams in the dispersion, the application of high-energy methods and cross-modal interactions. Research in these areas is still at initial stages and further input is still needed.
Authors’ contributions
Essam Hebishy: Conceptualization, Investigation, Writing - original draft, Writing – review & editing, Visualization, Project administration, Supervision. Han Du: Writing - original draft, review & editing, Visualization. Thais C. Brito-Oliveira and Samantha C. Pinho: Writing-Review and editing, and Song Miao: Conceptualization, supervision, writing-review & editing, Funding acquisition, Project administration.
Disclosure statement
The authors declare that they have no known competing financial interests or personal relationships that could have appeared to influence the work reported in this article.
Data availability statement
No data was used for the research described in the article.
Additional information
Funding
References
- World Health Organization. 2013. NCD Global Monitoring Framework., http://www.who.int/nmh/global_monitoring_framework/en/.
- Agarwal, S., V. L. Fulgoni, L. Spence, and P. Samuel. 2015. Sodium intake status in United States and potential reduction modeling: An NHANES 2007-2010 analysis. Food Science & Nutrition 3 (6):577–85 doi: 10.1002/fsn3.248.
- Ahmad, R., and J. E. Dalziel. 2020. G protein-coupled receptors in taste physiology and pharmacology. Frontiers in Pharmacology 11:587664 doi: 10.3389/fphar.2020.587664.
- Andrews, G. P., T. P. Laverty, and D. S. Jones. 2009. Mucoadhesive polymeric platforms for controlled drug delivery. European Journal of Pharmaceutics and Biopharmaceutics 71 (3):505–18 doi: 10.1016/j.ejpb.2008.09.028.
- Aubert, B., A. Lima, and B. Le Révérend. 2016. Biophysical basis of taste modulation by viscous solutions in humans. Food Hydrocolloids 60:494–9 doi: 10.1016/j.foodhyd.2016.04.018.
- Baines, Z. V., and E. R. Morris. 1987. Flavour/taste perception in thickened systems: The effect of guar gum above and below c. Food Hydrocolloids 1 (3):197–205 doi: 10.1016/S0268-005X(87)80003-6.
- Ben-Harb, S., M. Panouillé, D. Huc-Mathis, G. Moulin, A. Saint-Eve, F. Irlinger, P. Bonnarme, C. Michon, and I. Souchon. 2018. The rheological and microstructural properties of pea, milk, mixed pea/milk gels and gelled emulsions designed by thermal, acid, and enzyme treatments. Food Hydrocolloids 77:75–84 doi: 10.1016/j.foodhyd.2017.09.022.
- Benjamin, O., M. Davidovich-Pinhas, A. Shpigelman, and G. Rytwo. 2018. Utilization of polysaccharides to modify salt release and texture of a fresh semi hard model cheese. Food Hydrocolloids 75:95–106.
- Bhat, Z. F., J. D. Morton, S. L. Mason, and A. E. A. Bekhit. 2020. The application of pulsed electric field as a sodium reducing strategy for meat products. Food Chemistry 306:125622 doi: 10.1016/j.foodchem.2019.125622.
- Boisard, L., I. Andriot, C. Arnould, C. Achilleos, C. Salles, and E. Guichard. 2013. Structure and composition of model cheeses influence sodium NMR mobility, kinetics of sodium release and sodium partition coefficients. Food Chemistry 136 (2):1070–7 doi: 10.1016/j.foodchem.2012.09.035.
- Boisard, L., I. Andriot, C. Martin, C. Septier, V. Boissard, C. Salles, and E. Guichard. 2014. The salt and lipid composition of model cheeses modifies in-mouth flavour release and perception related to the free sodium ion content. Food Chemistry 145:437–44 doi: 10.1016/j.foodchem.2013.08.049.
- Bolumar, T., M. Enneking, S. Toepfl, and V. Heinz. 2013. New developments in shockwave technology intended for meat tenderization: Opportunities and challenges. A review. Meat Science 95 (4):931–9 doi: 10.1016/j.meatsci.2013.04.039.
- Brito-Oliveira, T. C., C. V. Molina, F. M. Netto, and S. C. Pinho. 2017. Encapsulation of beta‐carotene in lipid microparticles stabilized with hydrolyzed soy protein isolate: Production parameters, alpha‐tocopherol coencapsulation and stability under stress conditions. Journal of Food Science 82 (3):659–69 doi: 10.1111/1750-3841.13642.
- Busch, J. L. H. C., F. Y. S. Yong, and S. M. Goh. 2013. Sodium reduction: Optimizing product composition and structure towards increasing saltiness perception. Trends in Food Science & Technology 29 (1):21–34 doi: 10.1016/j.tifs.2012.08.005.
- Carmi, I. K., and O. Benjamin. 2017. Reduction in sodium content of fresh, semihard Tzfat cheese using salt replacer mixtures: Taste, texture and shelf life evaluation. International Journal of Dairy Technology 70 (3):354–64 doi: 10.1111/1471-0307.12369.
- Chabanet, C., A. Tarrega, C. Septier, F. Siret, and C. Salles. 2013. Fat and salt contents affect the in-mouth temporal sodium release and saltiness perception of chicken sausages. Meat Science 94 (2):253–61 doi: 10.1016/j.meatsci.2012.09.023.
- Chiu, N., L. Hewson, N. Yang, R. Linforth, and I. Fisk. 2015. Controlling salt and aroma perception through the inclusion of air fillers. LWT - Food Science and Technology 63 (1):65–70 doi: 10.1016/j.lwt.2015.03.098.
- Cook, S. L., S. Woods, L. Methven, J. K. Parker, and V. V. Khutoryanskiy. 2018. Mucoadhesive polysaccharides modulate sodium retention, release and taste perception. Food Chemistry 240:482–9 doi: 10.1016/j.foodchem.2017.07.134.
- de Jong, S., H. J. Klok, and F. van de Velde. 2009. The mechanism behind microstructure formation in mixed whey protein–polysaccharide cold-set gels. Food Hydrocolloids 23 (3):755–64 doi: 10.1016/j.foodhyd.2008.03.017.
- de Loubens, C., A. Saint-Eve, I. Déléris, M. Panouillé, M. Doyennette, I. C. Tréléa, and I. Souchon. 2011a. Mechanistic model to understand in vivo salt release and perception during the consumption of dairy gels. Journal of Agricultural and Food Chemistry 59 (6):2534–42 doi: 10.1021/jf103792b.
- de Loubens, C., M. Panouillé, A. Saint-Eve, I. Déléris, I. C. Tréléa, and I. Souchon. 2011b. Mechanistic model of in vitro salt release from model dairy gels based on standardized breakdown test simulating mastication. Journal of Food Engineering 105 (1):161–8 doi: 10.1016/j.jfoodeng.2011.02.020.
- de Souza Paglarini, C., V. A. Vidal, W. Ribeiro, A. P. Badan Ribeiro, O. D. Bernardinelli, A. M. Herrero, C. Ruiz-Capillas, E. Sabadini, and M. A. Rodrigues Pollonio. 2021. Using inulin-based emulsion gels as fat substitute in salt reduced Bologna sausage. Journal of the Science of Food and Agriculture 101 (2):505–17 doi: 10.1002/jsfa.10659.
- Defnet, E., L. Zhu, and S. J. Schmidt. 2016. Characterization of sodium mobility, binding, and apparent viscosity in full-fat and reduced-fat model emulsion systems. Journal of Food Measurement and Characterization 10 (3):444–52 doi: 10.1007/s11694-016-9323-2.
- Desmond, E. 2006. Reducing salt: A challenge for the meat industry. Meat Science 74 (1):188–96 doi: 10.1016/j.meatsci.2006.04.014.
- dos Santos, B. A., P. C. B. Campagnol, M. A. Morgano, and M. A. R. Pollonio. 2014. Monosodium glutamate, disodium inosinate, disodium guanylate, lysine and taurine improve the sensory quality of fermented cooked sausages with 50% and 75% replacement of NaCl with KCl. Meat Science 96 (1):509–13 doi: 10.1016/j.meatsci.2013.08.024.
- Dötsch, M., J. Busch, M. Batenburg, G. Liem, E. Tareilus, R. Mueller, and G. Meijer. 2009. Strategies to reduce sodium consumption: A food industry perspective. Critical Reviews in Food Science and Nutrition 49 (10):841–51 doi: 10.1080/10408390903044297.
- Dünnhaupt, S., O. Kammona, C. Waldner, C. Kiparissides, and A. Bernkop-Schnürch. 2015. Nano-carrier systems: Strategies to overcome the mucus gel barrier. European Journal of Pharmaceutics and Biopharmaceutics 96:447–53 doi: 10.1016/j.ejpb.2015.01.022.
- Emorine, M., C. Septier, T. Thomas-Danguin, and C. Salles. 2013. Heterogeneous salt distribution in hot snacks enhances saltiness without loss of acceptability. Food Research International 51 (2):641–7 doi: 10.1016/j.foodres.2013.01.006.
- Emorine, M., C. Septier, T. Thomas-Danguin, and C. Salles. 2014. Ham particle size influences saltiness perception in flans. Journal of Food Science 79 (4):S693–S696 doi: 10.1111/1750-3841.12399.
- Fahmy, A. R., L. S. Amann, A. Dunkel, O. Frank, C. Dawid, T. Hofmann, T. Becker, and M. Jekle. 2021. Sensory design in food 3D printing – Structuring, texture modulation, taste localization, and thermal stabilization. Innovative Food Science & Emerging Technologies 72:102743 doi: 10.1016/j.ifset.2021.102743.
- Farjami, T., and A. Madadlou. 2019. An overview on preparation of emulsion-filled gels and emulsion particulate gels. Trends in Food Science & Technology 86:85–94 doi: 10.1016/j.tifs.2019.02.043.
- Fellendorf, S., M. G. O’Sullivan, and J. P. Kerry. 2016. Impact of ingredient replacers on the physicochemical properties and sensory quality of reduced salt and fat black puddings. Meat Science 113:17–25 doi: 10.1016/j.meatsci.2015.11.006.
- Ferrari, G. T., Proserpio, C., Stragliotto, L. K., Boff, J. M., Pagliarini, E., and Oliveira V. R. d. 2022. Salt reduction in bakery products: A critical review on the worldwide scenario, its impacts and different strategies. Trends in Food Science & Technology 129:440–8 doi: 10.1016/j.tifs.2022.10.013.
- Frankowski, K. M., R. E. Miracle, and M. A. Drake. 2014. The role of sodium in the salty taste of permeate. Journal of Dairy Science 97 (9):5356–70 doi: 10.3168/jds.2014-8057.
- Geremias-Andrade, I. M., N. Souki, I. C. F. Moraes, and S. C. Pinho. 2016. Rheology of emulsion-filled gels applied to the development of food materials. Gels (Basel, Switzerland) 2 (3):22 doi: 10.3390/gels2030022.
- Gilbertson, T. A., L. Liu, I. Kim, C. A. Burks, and D. R. Hansen. 2005. Fatty acid responses in taste cells from obesity-prone and -resistant rats. Physiology & Behavior 86 (5):681–90 doi: 10.1016/j.physbeh.2005.08.057.
- Gilbertson, T. A., S. Damak, and R. F. Margolskee. 2000. The molecular physiology of taste transduction. Current opinion in neurobiology, 10(4), 519–527.
- Gobet, M., M. Mouaddab, N. Cayot, J. M. Bonny, E. Guichard, J. L. Le Quere, C. Moreau, and L. Foucat. 2009. The effect of salt content on the structure of iota-carrageenan systems: (23)Na DQF NMR and rheological studies. Magnetic Resonance in Chemistry 47 (4):307–12 doi: 10.1002/mrc.2389.
- Guerrero, A., S. S.-Y. Kwon, and D. V. Vadehra. (1995). Compositions to enhance taste of salt used in reduced amounts. US Patent No. US5, 711.
- Han, M., Y. Zhang, Y. Fei, X. Xu, and G. Zhou. 2009. Effect of microbial transglutaminase on NMR relaxometry and microstructure of pork myofibrillar protein gel. European Food Research and Technology 228 (4):665–70 doi: 10.1007/s00217-008-0976-x.
- Heidolph, B., Ray, D., Roller, S., Koehler, P., Weber, J., Slocum, S., and Noort, M. (2011). Looking for my lost shaker of salt… replacer: Flavor, function, future. Cereal Foods World 56(1), 5 doi: 10.1094/CFW-56-1-0005.
- Horne, D. S., and J. A. Lucey. 2017. Rennet-induced coagulation of milk. In L. H. Paul, McSweeney, P. F. Fox, P. D. Cotter, & D. W. Everett (Eds.), Cheese: Chemistry, physics and microbiology (4th ed., Vol. 1, pp. 115–143). Academic Press.
- Hughes, E., S. Cofrades, and D. J. Troy. 1997. Effects of fat level, oat fibre and carrageenan on frankfurters formulated with 5, 12 and 30% fat. Meat Science 45 (3):273–81 doi: 10.1016/s0309-1740(96)00109-x.
- Jimenez-Colmenero, F., S. Cofrades, I. Lopez-Lopez, C. Ruiz-Capillas, T. Pintado, and M. T. Solas. 2010. Technological and sensory characteristics of reduced/low-fat, low-salt frankfurters as affected by the addition of konjac and seaweed. Meat Science 84 (3):356–63 doi: 10.1016/j.meatsci.2009.09.002.
- Khutoryanskiy, V. V. 2011. Advances in mucoadhesion and mucoadhesive polymers. Macromolecular Bioscience 11 (6):748–64 doi: 10.1002/mabi.201000388.
- Koliandris, A., A. Lee, A.-L. Ferry, S. Hill, and J. Mitchell. 2008. Relationship between structure of hydrocolloid gels and solutions and flavour release. Food Hydrocolloids. 22 (4):623–30 doi: 10.1016/j.foodhyd.2007.02.009.
- Koriyama, T., S. Wongso, K. Watanabe, and H. Abe. 2002. Fatty acid compositions of oil species affect the 5 basic taste perceptions. Journal of Food Science 67 (2):868–73 doi: 10.1111/j.1365-2621.2002.tb10691.x.
- Kremer, S., J. Mojet, and R. Shimojo. 2009. Salt reduction in foods using naturally brewed soy sauce. Journal of Food Science 74 (6):S255–S262 doi: 10.1111/j.1750-3841.2009.01232.x.
- Kuo, W.-Y, and Y. Lee. 2014. Effect of Food Matrix on Saltiness Perception—Implications for Sodium Reduction. Comprehensive Reviews in Food Science and Food Safety 13:906–23.
- Kuo, W. Y., and Y. Lee. 2014a. Effect of food matrix on saltiness perception—Implications for sodium reduction. Comprehensive Reviews in Food Science and Food Safety 13 (5):906–23 doi: 10.1111/1541-4337.12094.
- Kuo, W. Y., and Y. Lee. 2014b. Temporal sodium release related to gel microstructural properties-implications for sodium reduction. Journal of Food Science 79 (11):E2245–2252doi: 10.1111/1750-3841.12669.
- Kuo, W.-Y., and Y. Lee. 2017. Correlating structural properties to sodium release of model solid lipoproteic colloids. Journal of Food Engineering 203:16–24 doi: 10.1016/j.jfoodeng.2017.01.025.
- Lai, S. K., Y. Y. Wang, and J. Hanes. 2009. Mucus-penetrating nanoparticles for drug and gene delivery to mucosal tissues. Advanced Drug Delivery Reviews 61 (2):158–71 doi: 10.1016/j.addr.2008.11.002.
- Lauverjat, C., I. Deleris, I. C. Trelea, C. Salles, and I. Souchon. 2009. Salt and aroma compound release in model cheeses in relation to their mobility. Journal of Agricultural and Food Chemistry 57 (21):9878–87 doi: 10.1021/jf901446w.
- Lawes, C. M., S. Vander Hoorn, and A. Rodgers. 2008. Global burden of blood-pressure-related disease, 2001. Lancet (London, England) 371 (9623):1513–8 doi: 10.1016/S0140-6736(08)60655-8.
- Lawrence, G., C. Salles, O. Palicki, C. Septier, J. Busch, and T. Thomas-Danguin. 2011. Using cross-modal interactions to counterbalance salt reduction in solid foods. International Dairy Journal 21 (2):103–10 doi: 10.1016/j.idairyj.2010.09.005.
- Lawrence, G., S. Buchin, C. Achilleos, F. Berodier, C. Septier, P. Courcoux, and C. Salles. 2012. In vivo sodium release and saltiness perception in solid lipoprotein matrices. 1. Effect of composition and texture. Journal of Agricultural and Food Chemistry 60 (21):5287–98 doi: 10.1021/jf204434t.
- Le Révérend, B. J., I. T. Norton, and S. Bakalis. 2013. Modelling the human response to saltiness. Food & Function 4 (6):880–8 doi: 10.1039/c3fo30106k.
- Leermakers, F. A., P. J. Atkinson, E. Dickinson, and D. S. Horne. 1996. Self-consistent-field modeling of adsorbed β-casein: Effects of pH and ionic strength on surface coverage and density profile. Journal of Colloid and Interface Science 178 (2):681–93 doi: 10.1006/jcis.1996.0166.
- Li, K., Z. L. Kang, Y. F. Zou, X. L. Xu, and G. H. Zhou. 2015. Effect of ultrasound treatment on functional properties of reduced-salt chicken breast meat batter. Journal of Food Science and Technology 52 (5):2622–33 doi: 10.1007/s13197-014-1356-0.
- Li, Y., K. Han, Z. Wan, and X. Yang. 2020. Salt reduction in semi-solid food gel via inhomogeneous distribution of sodium-containing coacervate: Effect of gum arabic. Food Hydrocolloids 109:106102 doi: 10.1016/j.foodhyd.2020.106102.
- Li, Y., Z. Wan, and X. Yang. 2019. Salt reduction in liquid/semi-solid foods based on the mucopenetration ability of gum Arabic. Food & Function 10 (7):4090–101 doi: 10.1039/c8fo02593b.
- Liem, D. G., F. Miremadi, and R. S. Keast. 2011. Reducing sodium in foods: The effect on flavor. Nutrients 3 (6):694–711 doi: 10.3390/nu3060694.
- Lima, A., M. Dufauret, B. Le Révérend, and T. J. Wooster. 2018. Deconstructing how the various components of emulsion creamers impact salt perception. Food Hydrocolloids 79:310–8 doi: 10.1016/j.foodhyd.2018.01.005.
- Lin, D., A. L. Kelly, and S. Miao. 2020. Preparation, structure-property relationships and applications of different emulsion gels: Bulk emulsion gels, emulsion gel particles, and fluid emulsion gels. Trends in Food Science & Technology 102:123–37 doi: 10.1016/j.tifs.2020.05.024.
- Lucey, J., and H. Singh. 2003. Acid coagulation of milk. Advanced Dairy Chemistry—1 Proteins: Part A/Part B 1001–25.
- Malone, M. E., I. A. M. Appelqvist, and I. T. Norton. 2003. Oral behaviour of food hydrocolloids and emulsions. Part 2. Taste and aroma release. Food Hydrocolloids 17 (6):775–84 doi: 10.1016/s0268-005x(03)00098-5.
- Mao, L., Y. Lu, M. Cui, S. Miao, and Y. Gao. 2020. Design of gel structures in water and oil phases for improved delivery of bioactive food ingredients. Critical Reviews in Food Science and Nutrition 60 (10):1651–66 doi: 10.1080/10408398.2019.1587737.
- Marchetti, L., N. Argel, S. C. Andres, and A. N. Califano. 2015. Sodium-reduced lean sausages with fish oil optimized by a mixture design approach. Meat Science 104:67–77 doi: 10.1016/j.meatsci.2015.02.005.
- Mattes, R. D. 2009. Is there a fatty acid taste? Annual Review of Nutrition 29 (1):305–27doi: 10.1146/annurev-nutr-080508-141108.
- McDonnell, C., P. Allen, C. Morin, and J. Lyng. 2014. The effect of ultrasonic salting on protein and water–protein interactions in meat. Food Chemistry 147:245–51 doi: 10.1016/j.foodchem.2013.09.125.
- Metcalf, K. L., and Z. M. Vickers. 2002. Taste intensities of oil-in-water emulsions with varying fat content. Journal of Sensory Studies 17 (5):379–90 doi: 10.1111/j.1745-459X.2002.tb00354.x.
- Mosca, A. C., I. Andriot, E. Guichard, and C. Salles. 2015. Binding of Na + ions to proteins: Effect on taste perception. Food Hydrocolloids. 51:33–40 doi: 10.1016/j.foodhyd.2015.05.003.
- Noort, M. W. J., J. H. F. Bult, M. Stieger, and R. J. Hamer. 2010. Saltiness enhancement in bread by inhomogeneous spatial distribution of sodium chloride. Journal of Cereal Science 52 (3):378–86 doi: 10.1016/j.jcs.2010.06.018.
- Ojha, K. S., D. F. Keenan, A. Bright, J. P. Kerry, and B. K. Tiwari. 2016. Ultrasound assisted diffusion of sodium salt replacer and effect on physicochemical properties of pork meat. International Journal of Food Science & Technology 51 (1):37–45 doi: 10.1111/ijfs.13001.
- Okada, K. S., and Y. Lee. 2017. Characterization of sodium mobility and binding by (23) Na NMR spectroscopy in a model lipoproteic emulsion gel for sodium reduction. Journal of Food Science 82 (7):1563–8 doi: 10.1111/1750-3841.13750.
- Oliver, L., E. Scholten, and G. A. van Aken. 2015. Effect of fat hardness on large deformation rheology of emulsion-filled gels. Food Hydrocolloids 43:299–310 doi: 10.1016/j.foodhyd.2014.05.031.
- Patel, M. M., J. D. Smart, T. G. Nevell, R. J. Ewen, P. J. Eaton, and J. Tsibouklis. 2003. Mucin/poly (acrylic acid) interactions: A spectroscopic investigation of mucoadhesion. Biomacromolecules 4 (5):1184–90 doi: 10.1021/bm034028p.
- Paulsen, M. T., A. Nys, R. Kvarberg, and M. Hersleth. 2014. Effects of NaCl substitution on the sensory properties of sausages: Temporal aspects. Meat Science 98 (2):164–70 doi: 10.1016/j.meatsci.2014.05.020.
- Phan, V. A., C. Yven, G. Lawrence, C. Chabanet, J. M. Reparet, and C. Salles. 2008. In vivo sodium release related to salty perception during eating model cheeses of different textures. International Dairy Journal 18 (9):956–63 doi: 10.1016/j.idairyj.2008.03.015.
- Philps, T., F. Angus, S. Clegg, D. Kilcast, C. Narain, and C. Den Ridder. 2006. Sensory isss in salt reduction. Food Quality and Preference 17 (7-8):633–4.
- Rama, R., N. Chiu, M. Carvalho Da Silva, L. Hewson, J. Hort, and I. D. Fisk. 2013. Impact of salt crystal size on in-mouth delivery of sodium and saltiness perception from snack foods. Journal of Texture Studies 44 (5):338–45 doi: 10.1111/jtxs.12017.
- Richardson, J. C., P. W. Dettmar, F. C. Hampson, and C. D. Melia. 2004. Oesophageal bioadhesion of sodium alginate suspensions: Particle swelling and mucosal retention. European Journal of Pharmaceutical Sciences 23 (1):49–56 doi: 10.1016/j.ejps.2004.05.001.
- Rosett, T. R., S. L. Kendregan, Y. Gao, S. J. Schmidt, and B. P. Klein. 1996. Thickening agents effects on sodium binding and other taste qualities of soup systems. Journal of Food Science 61 (5):1099–104 doi: 10.1111/j.1365-2621.1996.tb10939.x.
- Ruusunen, M., and E. Puolanne. 2005. Reducing sodium intake from meat products. Meat Science 70 (3):531–41 doi: 10.1016/j.meatsci.2004.07.016.
- Ruusunen, M., M. Simolin, and E. Puolanne. 2001. The effect of fat content and flavor enhancers on the perceived saltiness of cooked? Bologna-type? Sausages. Journal of Muscle Foods 12 (2):107–20 doi: 10.1111/j.1745-4573.2001.tb00303.x.
- Sala, G., T. Van Vliet, M. A. C. Stuart, G. A. Van Aken, and F. Van de Velde. 2009. Deformation and fracture of emulsion-filled gels: Effect of oil content and deformation speed. Food Hydrocolloids 23 (5):1381–93 doi: 10.1016/j.foodhyd.2008.11.016.
- Scherf, K. A., T. Pflaum, P. Koehler, and T. Hofmann. 2015. Salt taste perception in hydrocolloid systems is affected by sodium ion release and mechanosensory–gustatory cross-modal interactions. Food Hydrocolloids 51:486–94 doi: 10.1016/j.foodhyd.2015.05.043.
- Sikes, A. L., A. B. Tobin, and R. K. Tume. 2009. Use of high pressure to reduce cook loss and improve texture of low-salt beef sausage batters. Innovative Food Science & Emerging Technologies 10 (4):405–12 doi: 10.1016/j.ifset.2009.02.007.
- Sinesio, F., A. Raffo, M. Peparaio, E. Moneta, E. Saggia Civitelli, V. Narducci, V. Turfani, S. Ferrari Nicoli, and M. Carcea. 2019. Impact of sodium reduction strategies on volatile compounds, sensory properties and consumer perception in commercial wheat bread. Food Chemistry 301:125252 doi: 10.1016/j.foodchem.2019.125252.
- Sogias, I. A., A. C. Williams, and V. V. Khutoryanskiy. 2008. Why is chitosan mucoadhesive? Biomacromolecules 9 (7):1837–42 doi: 10.1021/bm800276d.
- Sosnik, A., J. das Neves, and B. Sarmento. 2014. Mucoadhesive polymers in the design of nano-drug delivery systems for administration by non-parenteral routes: A review. Progress in Polymer Science 39 (12):2030–75 doi: 10.1016/j.progpolymsci.2014.07.010.
- Stieger, M. 2011. Texture-taste interactions: Enhancement of taste intensity by structural modifications of the food matrix. Procedia Food Science 1:521–7 doi: 10.1016/j.profoo.2011.09.079.
- Stieger, M., and F. van de Velde. 2013. Microstructure, texture and oral processing: New ways to reduce sugar and salt in foods. Current Opinion in Colloid & Interface Science 18 (4):334–48 doi: 10.1016/j.cocis.2013.04.007.
- Suzuki, A., H. Zhong, J. Lee, and S. Martini. 2014. Effect of lipid content on saltiness perception: A psychophysical study. Journal of Sensory Studies 29 (6):404–12 doi: 10.1111/joss.12121.
- Tarrega, A., C. Yven, E. Sémon, and C. Salles. 2011. In-mouth aroma compound release during cheese consumption: Relationship with food bolus formation. International Dairy Journal 21 (5):358–64 doi: 10.1016/j.idairyj.2010.12.010.
- Taylor, A. J. 2002. Release and transport of flavors in vivo: Physicochemical, physiological, and perceptual considerations. Comprehensive Reviews in Food Science and Food Safety 1 (2):45–57 doi: 10.1111/j.1541-4337.2002.tb00006.x.
- Taylor, T., A. Chouljenko, F. Bonilla, R. Scott, F. Bueno, V. Reyes, C. Lanclos, K. F. Calumba, and S. Sathivel. 2019. A pectin-gelatin gel containing oral rehydration solution and the release of sodium chloride under simulated gastric conditions. International Journal of Biological Macromolecules 136:1112–8 doi: 10.1016/j.ijbiomac.2019.06.146.
- Thirawong, N., J. Nunthanid, S. Puttipipatkhachorn, and P. Sriamornsak. 2007. Mucoadhesive properties of various pectins on gastrointestinal mucosa: An in vitro evaluation using texture analyzer. European Journal of Pharmaceutics and Biopharmaceutics 67 (1):132–40 doi: 10.1016/j.ejpb.2007.01.010.
- Thomas-Danguin, T., G. Lawrence, M. Emorine, N. Nasri, L. Boisard, E. Guichard, and C. Salles. 2015. Strategies to enhance saltiness in food involving cross modal interactions. In The chemical sensory informatics of food: measurement, analysis, integration, Washington DC: Oxford University Press, 27–40. ACS Symposium Series, ACS Publications.
- Tokifuji, A., Y. Matsushima, K. Hachisuka, and K. Yoshioka. 2013. Texture, sensory and swallowing characteristics of high-pressure-heat-treated pork meat gel as a dysphagia diet. Meat Science 93 (4):843–8 doi: 10.1016/j.meatsci.2012.11.050.
- Torres, O., B. Murray, and A. Sarkar. 2016. Emulsion microgel particles: Novel encapsulation strategy for lipophilic molecules. Trends in Food Science & Technology 55:98–108 doi: 10.1016/j.tifs.2016.07.006.
- Torres, O., N. M. Tena, B. Murray, and A. Sarkar. 2017. Novel starch based emulsion gels and emulsion microgel particles: Design, structure and rheology. Carbohydrate Polymers 178:86–94 doi: 10.1016/j.carbpol.2017.09.027.
- Torrico, D. D., and W. Prinyawiwatkul. 2015. Psychophysical effects of increasing oil concentrations on saltiness and bitterness perception of oil‐in‐water emulsions. Journal of Food Science 80 (8):S1885–S1892 doi: 10.1111/1750-3841.12945.
- Tournier, C., C. Sulmont-Rossé, E. Sémon, A. Vignon, S. Issanchou, and E. Guichard. 2009. A study on texture–taste–aroma interactions: Physico-chemical and cognitive mechanisms. International Dairy Journal 19 (8):450–8doi: 10.1016/j.idairyj.2009.01.003.
- Tsai, W. C., S. T. Wang, K. B. Chang, and M. L. Tsai. 2019. Enhancing saltiness perception using chitin nanomaterials. Polymers 11 (4):719 doi: 10.3390/polym11040719.
- Wang, X., N. Ullah, Y. Shen, Z. Sun, X. Wang, T. Feng, X. Zhang, Q. Huang, and S. Xia. 2021. Emulsion delivery of sodium chloride: A promising approach for modulating saltiness perception and sodium reduction. Trends in Food Science & Technology 110:525–38 doi: 10.1016/j.tifs.2021.02.020.
- Wang, X., T. Feng, and S. Xia. 2021. Saltiness perception related to salt release of surimi emulsified sausages: Modulation in texture and microstructure by polysaccharides. International Journal of Food Science & Technology 56 (8):3893–902doi: 10.1111/ijfs.15006.
- Webster, J. L., E. K. Dunford, C. Hawkes, and B. C. Neal. 2011. Salt reduction initiatives around the world. Journal of Hypertension 29 (6):1043–50 doi: 10.1097/HJH.0b013e328345ed83.
- Withers, C. A., M. T. Cook, L. Methven, M. A. Gosney, and V. V. Khutoryanskiy. 2013. Investigation of milk proteins binding to the oral mucosa. Food & Function 4 (11):1668–74 doi: 10.1039/c3fo60291e.
- Yamamoto, Y., and M. Nakabayashi. 2000. Effects of an oil phase on the salt taste of oil/water emulsions (Hydrocolloids (451–6). Elsevier.
- Yamazoe, E., J.-Y. Fang, and K. Tahara. 2021. Oral mucus-penetrating PEGylated liposomes to improve drug absorption: Differences in the interaction mechanisms of a mucoadhesive liposome. International Journal of Pharmaceutics 593:120148 doi: 10.1016/j.ijpharm.2020.120148.
- Yang, H., M. Han, X. Wang, Y. Han, J. Wu, X. Xu, and G. Zhou. 2015. Effect of high pressure on cooking losses and functional properties of reduced-fat and reduced-salt pork sausage emulsions. Innovative Food Science & Emerging Technologies 29:125–33doi: 10.1016/j.ifset.2015.02.013.
- Yehia, S. A., O. N. El-Gazayerly, and E. B. Basalious. 2009. Fluconazole mucoadhesive buccal films: In vitro/in vivo performance. Current Drug Delivery 6 (1):17–27 doi: 10.2174/156720109787048195.
- Yucel, U., and D. G. Peterson. 2015. Effect of protein-lipid-salt interactions on sodium availability in the mouth and consequent perception of saltiness: In solutions. Journal of Agricultural and Food Chemistry 63 (34):7487–93 doi: 10.1021/acs.jafc.5b02311.
- Zhang, X., D. Guo, J. Xue, S. Yanniotis, and I. Mandala. 2017. The effect of salt concentration on swelling power, rheological properties and saltiness perception of waxy, normal and high amylose maize starch. Food & Function 8 (10):3792–802 doi: 10.1039/c7fo01041a.
- Zhang, Y., H. Yang, H. Zheng, D. Yuan, and L. Mao. 2021. Physical properties and salt release of potato starch-based emulsion gels with OSA starch-stabilized oil droplets. LWT 141:110929 doi: 10.1016/j.lwt.2021.110929.