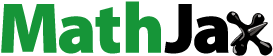
Abstract
The global aging population has brought about a pressing health concern: dysphagia. To effectively address this issue, we must develop specialized diets, such as thickened fluids made with polysaccharide–dextrin (e.g., water, milk, juices, and soups), which are crucial for managing swallowing-related problems like aspiration and choking for people with dysphagia. Understanding the flow behaviors of these thickened fluids is paramount, and it enables us to establish methods for evaluating their suitability for individuals with dysphagia. This review focuses on the shear and extensional flow properties (e.g., viscosity, yield stress, and viscoelasticity) and tribology (e.g., coefficient of friction) of polysaccharide–dextrin-based thickened fluids and highlights how dextrin inclusion influences fluid flow behaviors considering molecular interactions and chain dynamics. The flow behaviors can be integrated into the development of diverse evaluation methods that assess aspects such as flow velocity, risk of aspiration, and remaining fluid volume. In this context, the key in-vivo (e.g., clinical examination and animal model), in-vitro (e.g., the Cambridge Throat), and in-silico (e.g., Hamiltonian moving particles semi-implicit) evaluation methods are summarized. In addition, we explore the potential for establishing realistic assessment methods to evaluate the swallowing performance of thickened fluids, offering promising prospects for the future.
1. Introduction
In the current landscape, the global population is grappling with the challenges posted by the aging demographic. According to the United Nations’ World Population Prospects: The 2019 Revision, it is projected that the elderly population will comprise approximately 16% of the world’s total by the year 2050 (source: https://www.un.org/zh/global-issues/aging). Among the issues faced by older individuals, dysphagia stands out, representing the difficulty in smoothly moving food from the mouth to the stomach (Abu-Ghanem, Chen, and Amin Citation2020). Dysphagia also affects specific groups dealing with various medical conditions, such as sarcopenia, neurological disorders, cancer, and inflammation-related ailments (e.g., stroke, Parkinson’s, and dementia) (Smukalla et al. Citation2017). It is noteworthy that over 50% of patients with acute illnesses and nursing home residents aged 65 or older may experience symptoms of dysphagia (Forster et al. Citation2011). In particular, older individuals may exhibit reduced chewing ability, decreased saliva production, and delayed closure of the epiglottis, leading to dysphagia symptoms like aspiration and choking during food swallowing (Rodd, Tas, and Taylor Citation2022; Vivanti et al. Citation2009; Pae, Meydani, and Wu Citation2012). Additionally, dysphagia can cause issues like dehydration, malnutrition, pneumonia, and other respiratory ailments, elevating hospitalization rates and mortality among affected patients (Ebihara et al. Citation2016).
To enhance the quality of life for individuals grappling with dysphagia, a range of specialized diets has been developed, including thickened fluids (e.g., water, milk, juices, and soups) and texture-modified foods (TMFs) (e.g., vegetables, fruits, pork, and chicken), have been developed. Among these options, thickened fluids are favored by people with dysphagia, as they require less chewing effort during swallowing compared with TMFs (Hadde and Chen Citation2021). It has been observed that the presence of a thickening agent in fluids can elevate viscosity and cohesiveness, thereby reducing flow velocity. This, in turn, affords more time for muscle regulation and the closure of the epiglottis in individuals with dysphagia, mitigating the risks associated with dysphagia, such as aspiration and choking (Methacanon et al. Citation2021; Kongjaroen, Methacanon, and Gamonpilas Citation2022; Hadde and Chen Citation2019; Marconati and Ramaioli Citation2020).
Dysphagia fluids are typically thickened using various polysaccharides, such as xanthan gum (XG), guar gum (GG), konjac glucomannan (KGM), locust bean gum (LBG), κ-carrageenan (KC), methylcellulose (MC), sodium carboxymethylcellulose (CMC), and modified starch (Ross et al. Citation2019). These polysaccharide chains exhibit entanglement and interactions (e.g., hydrogen bonding) within the fluid matrix, resulting in remarkable thickening effects that play a crucial role in the swallowing performance of these fluids, affecting aspects like aspiration risk and residual amount (Evageliou Citation2020). To ensure the dissolution, dispersion, and thickening properties of polysaccharides at room temperature, binder components, typically dextrins, are commonly incorporated. The addition of dextrins, such as maltodextrin and cyclodextrin, enhances the surface adhesion of polysaccharide particles within the fluid matrix. This, in turn, facilitates the collision and binding of wetted particles, ensuring instant dispersion at room temperature (Lee and Yoo Citation2021; Jeong, Bak, and Yoo Citation2019). Consequently, this leads to alterations in the chain interactions and thickening characteristics of the resultant polysaccharide–dextrin fluids. Furthermore, dextrin serves as a functional component with a significant role in enhancing immune system function, stabilizing blood sugar and lipid levels, and providing nutritional supplementation (Farhangi et al. Citation2018; Gentilcore et al. Citation2011; Slavin et al. Citation2009). Over time, extensive efforts have been dedicated to crafting polysaccharide–dextrin-thickened fluids tailored to exhibit specific flow behaviors and optimized swallowing performance (Kim, Kim, and Yoo Citation2017; Aguilera and Park Citation2016). Also, these dysphagia fluid diets can be easily prepared using a variety of commercial thickeners that combine polysaccharides and dextrin, such as Nestlé ThickenUp Clear (TUC), Nutricia Nutilis Powder, and Hormel Thick & Easy (Kongjaroen, Methacanon, and Gamonpilas Citation2022).
In recent years, significant attention has been devoted to understanding and optimizing the flow properties (e.g., shear viscosity, yield stress and extensional modulus) of polysaccharide–dextrin-thickened fluids. This focus extends to the development of evaluation methods that incorporate these flow characteristics to ensure the safety of fluid swallowing, including in-vivo methods (e.g., videofluoroscopy method and animal models), in-vitro techniques (e.g., the Cambridge Throat and the Gothenburg Throat), and in-silico simulations (e.g., the semi-implicit motion particle method) ones, as illustrated in .
Figure 1. Flow behaviors and swallowing features of thickened fluids by polysaccharides with dextrin.
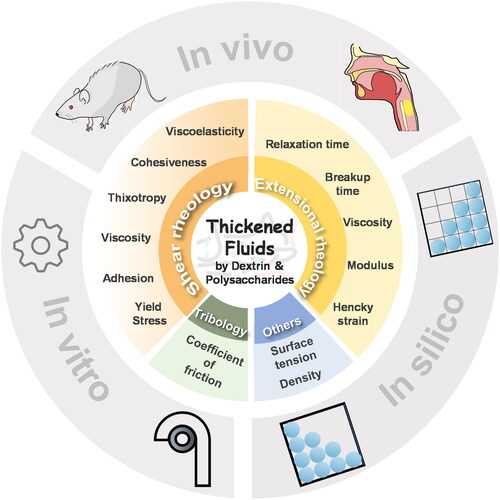
Given that polysaccharide–dextrin-thickened fluids undergo pronounced shear and extensional deformations throughout the oral cavity, pharynx, and esophagus during swallowing, the flow properties, both shear and extensional, as well as tribological properties, assume pivotal roles in determining their safety and performance during ingestion (Waqas et al. Citation2017). A systematic overview of the flow behaviors and associated swallowing evaluation methods for polysaccharide–dextrin-thickened fluids hold great significance in the development of special fluid diets tailored to the preferences and needs of individuals coping with dysphagia.
To date, some reviews have explored the impact of polysaccharides on shear rheological properties and swallowing behaviors. For instance, Schmidt et al. (Citation2021) showed the influences of polysaccharides (XG, GG, and KGM) on the viscosity, viscoelasticity, and sensory attributes of special dysphagia diets. Also, researchers reviewed the importance of rheology and tribology in specific polysaccharide fluids (e.g., starch and XG) in the context of oropharyngeal dysphagia, emphasizing the need for further research into the nutritional implications arising from interactions between thickeners and food ingredients (Methacanon et al. Citation2021). However, a comprehensive review of the flow characteristics of polysaccharide–dextrin-thickened fluids and the concurrent advancements in swallowing assessment methods remains outstanding. This knowledge is essential for the development and verification of thickened fluid formulations tailored to individuals experiencing dysphagia symptoms.
This present review delves into the influence of dextrin supplementation on the shear and extensional rheological properties, along with tribological properties, of polysaccharide-based fluids, intimately connected with their swallowing performance and safety. Additionally, it explores various in-vivo, in-vitro and in-silico models that incorporate flow characteristics to assess crucial aspects like oropharyngeal flow velocity, risk of aspiration, and remaining fluid volume in polysaccharide–dextrin-thickened fluids. This understanding is of paramount importance in the design of fluid-based diets that enhance the quality of life for individuals afflicted by dysphagia.
2. Basic aspects of dysphagia and thickened fluids
2.1. Overview of fluid food swallowing and dysphagia
Swallowing food boluses represents a complex physiological process within the human body (Clavé and Shaker Citation2015), divided into three distinct stages: the oral stage, the pharynx stage, and the esophagus stage. The swallowing process for fluid foods is illustrated in .
Figure 2. Human swallowing process. (a) The tongue lift pushes the bolus into the oropharynx. (b) The raised soft palate closes the opening of the nasal cavity as the bolus enters the pharynx. (c) The bolus is advanced through the pharynx through the closed epiglottis and the upper esophageal sphincter into the esophagus. (d) The bolus travels through the esophagus to the stomach via peristaltic waves.
Reprinted from Ref. (Hennessy and Goldenberg Citation2016) with permission from Elsevier, Copyright 2016.

During the oral stage (), unlike the case of solid foods, fluid foods do not require chewing and can be mixed directly with saliva to form a bolus (Rommel and Hamdy Citation2016; Smukalla et al. Citation2017). A food bolus is positioned at the front part of the tongue under the influence of the soft palate. With the muscles of the base of the tongue and the floor of the mouth in action, the tongue is pushed upwards against the hard palate and then moved backward to propel the bolus.
In the pharyngeal stage (), the entry of the food bolus into the pharynx and the base of the tongue initiates a swallowing reflex, which includes the closure of the airway by the soft palate and the epiglottis cartilage near the airway, the peristalsis of the pharynx, and the opening of the upper esophageal sphincter (UES) to allow passage of the ingested food. As the pharynx contracts, the esophagus begins to move in a primary peristalsis wave, and the bolus enters the esophagus (). This action stretches the esophageal wall, triggering the secondary peristalsis of the esophagus.
In the final esophageal stage (), the striated and smooth muscles in the esophagus generate peristaltic waves under the control of central control mechanisms and local reflexes, respectively. These waves propel the fluid bolus into the stomach. The soft palate and tongue relax, and the epiglottis opens, allowing resumption of resume breathing (Rommel and Hamdy Citation2016; Smukalla et al. Citation2017).
The elderly and patients, particularly those afflicted by neurological diseases, often experience muscle weakness, reduced saliva production, impaired tongue-jaw coordination, and incomplete or slow epiglottis closure. These factors can result in boluses entering the trachea or nasal cavity, leading to symptoms such as choking, aspiration, esophageal reflux, chest pain, and more. This condition is known as dysphagia, which can be categorized into oropharyngeal dysphagia and esophageal dysphagia (Jean Citation2001).
Compared to esophageal dysphagia, oropharyngeal dysphagia exhibits a higher prevalence, more severe symptoms, an increased risk of complications, and greater associated mortality (Clavé and Shaker Citation2015). Oropharyngeal dysphagia can stem from a variety of aging and disease factors. Symptoms of oropharynx dysphagia, including decreased saliva production, delayed epiglottis closure, and neurological impairments, can affect bolus formation and result in reflux into the nasal cavity or trachea (Sasegbon and Hamdy Citation2017). Oropharyngeal dysphagia commonly causes choking, aspiration, malnutrition, dehydration, and pneumonia.
On the other hand, esophageal dysphagia is often related to reflex-based causes and can be triggered by intrinsic and extrinsic mechanical causes, primary or secondary neuromuscular diseases, or inflammatory processes affecting the lower esophageal sphincter (LES) and the esophagus (Clavé and Shaker Citation2015; Nikaki et al. Citation2019). Esophageal dysphagia typically manifests as symptoms like slow food passage and temporary food entrapment.
2.2. Overview of features of thickened fluids for individuals with dysphagia
Developing special diets has been proven effective at managing dysphagia symptoms and enhancing the quality of life for individuals afflicted with dysphagia. To prevent severe complications, individuals with dysphagic are often recommended to include TMFs (semi-solid and gel forms) and thickened fluids in their dietary plans (Steele et al. Citation2015). Within these specialized dietary options, thickened fluids, such as water, milk, juices, and soups, have gained widespread utilization for addressing dysphagia-related challenges such as choking and aspiration. In long-term-care facilities, TMF accounts for 15–30% of use, while thickened fluids are more extensively used (Cichero et al. Citation2013). The inclusion of thickeners, primarily polysaccharides, serves to decelerate the flow of the fluid bolus during swallowing, providing the epiglottis with ample time to maneuver and safeguard against food entering the trachea, ultimately alleviating the symptoms of dysphagia (Poursani, Razavi, and Norouzi Citation2021). However, the reduced flowability in the mouth due to excessive viscosity may result in the accumulation of food residues during swallowing, thereby heightening the risk of choking and aspiration (Steele et al. Citation2015; Ullrich and Crichton Citation2015).
Polysaccharides such as XG, GG, KGM, and LBG are commonly employed in the thickening of fluid diets for individuals with dysphagia (Jo, Bak, and Yoo Citation2018). However, pure polysaccharides have a propensity to form clumps when dissolved at room temperature due to their small particle size, which can impede their instantaneous and complete dissolution in fluids (Lee and Yoo Citation2021). Meet the demands of fluid thickening typically necessitates the inclusion of binder components to improve the dispersion, dissolution, and thickening properties of the polysaccharide components. Dextrins are the most commonly used binders, and they have minimal impact on the nutritional values and functions of foods. They play a pivotal role in enhancing the surface adhesion of polysaccharide particles, facilitating the collision and adhesion of wetted polysaccharide particles. This process results in the formation of larger agglomerates, ensuring their instant dispersion (Lee and Yoo Citation2021; Jeong, Bak, and Yoo Citation2019). In addition, the inclusion of dextrins can reduce the cost of production, and alter the rheological properties (e.g., viscosity) of fluids (Jeong, Bak, and Yoo Citation2019). Dextrins are already integral components of various commercial thickeners, such as TUC, Nutricia Nutilis Powder, and Hormel Thick & Easy. Consequently, substantial endeavors have been dedicated to creating thickened fluids by combining polysaccharide with dextrins to facilitate dysphagia management. Representative polysaccharides and dextrin structures used for fluid thickening are illustrated in .
Table 1. Polysaccharides and dextrins for fluid thickening.
Dysphagia fluid diets, including those thickened by polysaccharide–dextrin, can be classified into different grades in different countries (). Wood (Citation1968) initially proposed that the assessment of fluid thickness in the oral context takes place at a shear rate of approximately 50 s−1. Subsequently, this criterion for viscosity at this specific shear rate was adopted by the United States and other countries as a standard for grading dysphagia-friendly foods. Line-spread test (LST) and Bostwick concentration meters are also commonly employed for this purpose. Furthermore, the International Dysphagia Diet Standardization Initiative (IDDSI) introduced a comprehensive framework for the identification and categorization of food consistencies, employing user-friendly methods to promote the global circulation of food commodities () (Cichero et al. Citation2017). To facilitate clinical treatment, researchers have attempted to correlate IDDSI ratings with dysphagia assessments, such as the IDDSI Functional Diet Scale () and dietary recommendations based on the Water Drinking Test (Steele et al. Citation2018; Su et al. Citation2018). And, the Ball-Back Extrusion (BBE) cell coupled with a texture analyzer has been introduced as a means to quantitatively characterize the IDDSI thickened fluid framework using apparent stress (Hadde et al. Citation2022). This method offers an approach to grading the swallowing properties of fluid based on their flow behavior in industrial production. The use of fluid flow for precise, objective correlation with dysphagia and clinical standards holds significant importance in the realm of industrial production.
Figure 3. (a) The international dysphagia Diet standardisation initiative (IDDSI) 2019 classification and (b) IDDSI Functional Diet Scale. IDDSI Functional Diet Scale is a scoring chart for a patient to determine the IDDSI-FDS score (the numbers in intersecting cells of columns show the patient’s food texture recommendation, and rows indicate the patient’s drink consistency recommendation). (a) is reprinted from https://iddsi.org/framework/ Licensed under the CreativeCommons Attribution ShareAlike 4.0 License. (b) is reprinted from Ref. (Steele et al. Citation2018) with permission from Elsevier, Copyright 2018.
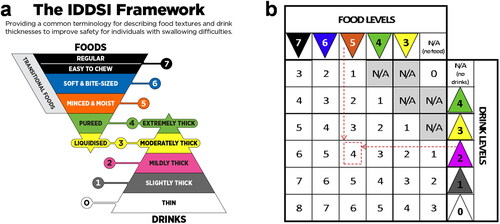
Table 2. Fluid grading standards of various countries, regions, and organizations.
Considering this, several methods have been devised to encompass a broader range of flow parameters for fluids, including those thickened by polysaccharide–dextrin, in order to enhance the evaluation of their swallowing performance.
3. Flow behaviors of polysaccharide–dextrin thickened fluids for individuals with dysphagia
Throughout the process of swallowing, a fluid thickened with polysaccharide–dextrin experiences deformation and flow phenomena within the oral cavity, pharynx, and esophagus. Comprehending the flow characteristics of these thickened fluids holds immense significance in the development of corresponding swallowing assessment techniques and, consequently, the creation of fluid diets tailored for individuals with dysphagia. This section summarizes the recent advances in the shear and extensional flow characteristics of polysaccharide–dextrin-thickened fluids, including viscosity, modulus, loss tangent (tan δ), yield stress (τ0), thixotropy, adhesion, cohesion, and other parameters. Additionally examined are the tribological properties of these composite fluids.
3.1. Rheology
Rheology is the science about the intricate dynamics of matter deformation and flow. A fluid’s rheological equation, as elucidated by the relationship between stress (force per unit area), strain (alteration in position along the flow direction), and strain rate (change in strain over time), is pivotal in understanding its behavior (Alghooneh, Razavi, and Kasapis Citation2020).
Swallowing, a complex process, subjects the fluid to both shear and extensional deformations. Shear deformation prompts layers of molecules to move relative to each other, a phenomenon explored through rheometers. These devices are instrumental in examining parameters like viscosity, the ratio of shear stress to shear rate. The choice of geometry for rheological measurements, be it concentric cylinders, parallel plate-plate, or cone-plate, depends on the viscosity of the thickened fluid. It is noteworthy that most thickened fluids used in dysphagia management exhibit non-Newtonian, shear-thinning behavior. As shear rate increases during swallowing, the viscosity of thickened fluids decreases due to the disentanglement and orientation of polysaccharide chains (Xie Citation2023).
Furthermore, certain thickened fluids exhibit solid-like behavior under low stresses and transform into flowing states under high stresses, and this critical point is known as yield stress (τ0). The yield stress is believed to be linked to the tongue pressure required for effective swallowing. Considering the viscoelastic or thixotropic time factor, the fluid’s stress response evolves during flows (Li et al. Citation2023). These two time-dependent characteristics are distinguished from the relaxation of stress after the end of flow and elasticity. Thixotropy is typically associated with rapid relaxation and viscosity, while viscoelasticity manifests as slow and elastic behavior (Larson and Wei Citation2019; Bui and Ho Citation2020). Viscoelasticity, based on the type of response, branching into small-amplitude oscillatory shear (SAOS) and large-amplitude oscillatory shear (LAOS). A nuanced understanding of these time-dependent characteristics proves invaluable in assessing the swallowing efficacy of thickened fluids (Alghooneh, Razavi, and Kasapis Citation2020; Li et al. Citation2023). In contrast to shear deformation, extensional deformation involves the removal of molecules within the fluid. Extensional viscosity, filament break-up time, and cohesiveness serve as representative indices of filament deformation with time. The elasticity of thickened fluids is considered to correlate with extensional viscosity and the first normal stress difference (N1, the difference between the normal stress in the direction of flow (σ11) and the normal stress perpendicular to the flow (σ22) (Xie, Halley, and Avérous Citation2012). While the measurement of extensional viscosity is challenging due to fluid breakage before stabilization, current instruments, like CaBER (HAAKE CaBER1), typically gauge apparent extensional viscosity (Gallegos et al. Citation2021).
3.1.1. Viscosity
Viscosity is a key factor in assessing fluid foods for individuals with dysphagia as it characterize the material’s resistance to flow. This parameter has been adopted by standards such as the National Dysphagia Diet (NDD) of the American Dietetic Association and the Japanese Diet for Dysphagia (JDD) of the Japanese Society for Dysphagia Rehabilitation (JSDR) (Newman et al. Citation2016; Seo and Yoo Citation2013; Ross et al. Citation2019). While the steady-state viscosity is typically measured using Brookfield viscometers, contemporary rheometers offer a broader range of insights into fluid behavior (Zargaraan et al. Citation2013). It is widely acknowledged that elevating the shear viscosity of thickened fluids, such as those with nectar-like and honey-like consistencies, enhances their cohesiveness and decelerates their flow. This deliberate slowing effort affords the epiglottis ample time to function, safeguarding against the entry of fluid food into the trachea, ultimately diminishing the likelihood of aspiration and choking incidents.
The inclusion of dextrin into polysaccharide-thickened fluids has the capacity to modulate their steady-state viscosities. It is revealed that the presence of dextrin influences the hydrogen bonding and chain entanglement of polysaccharides in thickened fluids, thereby altering their rheological properties under shear conditions (Pourmohammadi et al. Citation2018; Witczak et al. Citation2010; Kim, Kim, and Yoo Citation2017; Habibi and Khosravi-Darani Citation2017; Rao, Ramadevi, and Sirisha Citation2014). For most polysaccharides (e.g., starch, XG, and GG), the addition of dextrin elevates shear viscosity (Hadde, Nicholson, et al. Citation2020; Newman et al. Citation2016) and can contribute to improving swallowing safety. However, in certain instances, the introduction of dextrin can lead to a reduction in shear viscosity, as observed in fluids thickened by KGM (Wei et al. Citation2021). The reasons will be mentioned later in this section.
More specifically, the thickening effect of starch depends on the hydrogen bonding between amylopectin and amylose or hydrate bridging (Dewar and Joyce Citation2006). In the case of starch-thickened fluids, different dextrins can yield different effects by vying for water and altering the interactions between starch chains. Typically, the inclusion of branched limit dextrin (BLD), a low-molecular-mass dextrin with an amylopectin core, competes for water with maize starch, reducing the mobility of starch chains and subsequently increasing viscosity (Wang et al. Citation2018). In contrast, adding maltodextrins (MDs) limits starch’s accessibility to water molecules, resulting in reduced viscosity within the system due to limited gelatinization (Pourmohammadi et al. Citation2018; Witczak et al. Citation2010).
In addition, the shear viscosities of thickened fluids with non-starch polysaccharides and dextrin have been investigated. For instance, the thickening effect of XG is intricately tied to the formation of a physical network through the combination of O-acetyl groups and pyruvate residues, which is upheld by chain hydrogen bonding and chain entanglements (Kumar, Madhusudana Rao, and Han Citation2018). While XG has the ability to transition from an ordered helical structure to a coiled (disordered) structure, thereby augmenting viscosity (Habibi and Khosravi-Darani Citation2017), MD inclusion enhanced the disordering and entanglement of XG molecule chains, resulting in increased fluid viscosity (Kim, Kim, and Yoo Citation2017).
Furthermore, viscosity reductions were observed in fluids thickened with non-starch polysaccharide–dextrin. Notably, the inclusion of MD with a low dextrose equivalent (DE) (especially DE ≤ 6) significantly reduced the viscosity of galactomannan systems (e.g., KGM, GG, and LBG), and a high MD concentration (10% w/w) made the flow behaviors of galactomannan matrices (1% w/w) resemble that of an MD system (Tha Goh, Sui Mei Wee, and Hemar Citation2013). This effect can be attributed to the phase separation between two polysaccharides (as summarized in ). Compared to the high mixing entropy observed when anionic polysaccharides (e.g., XG) are mixed with MD, the mixing entropy is lower for two neutral polysaccharides, making phase separation more likely and resulting in reduced system viscosity (Boyd et al. Citation2009). It was disclosed that MD could function as a plasticizer within a polysaccharide matrix (e.g., KGM), hindering chain associations and the formation of entangled networks due to steric hindrance, ultimately leading to a significant reduction in viscosity (Wei et al. Citation2021). Additionally, as the hydrophobic cavity of CD, which can accommodate the hydrophobic tails of polysaccharides and partially deactivate hydrophobic associations, resulted in reduced apparent viscosity in polysaccharide systems like methylcellulose (MC) and sodium carboxymethylcellulose (CMC) (Rao, Ramadevi, and Sirisha Citation2014; Tha Goh, Sui Mei Wee, and Hemar Citation2013; Beheshti et al. Citation2007) when β-cyclodextrin (β-CD) was introduced. Likewise, the presence of CD decreased the viscosity of a fluid thickened with KC when compared to the counterpart with KC alone (Yuan, Ritzoulis, et al. Citation2018).
Other than shear viscosities, the extensional rheological behaviors of polysaccharide–dextrin-thickened fluids have been assessed. At present, CaBER and hyperbolic contraction flow equipment can be applied to the measurement of extensional viscosity, with the former holding greater popularity. It is noteworthy that thickened fluids with identical shear viscosities can exhibit significantly different extensional characteristics (Kongjaroen, Methacanon, and Gamonpilas Citation2022; Hadde and Chen Citation2019; Marconati and Ramaioli Citation2020). This was substantiated by the alterations observed in commercial polysaccharide thickeners containing dextrin (). Using an extensional rheometer, an apparent extensional viscosity (ηE) and a Hencky strain (ε) can be calculated based on Equationequations (1)(1)
(1) and Equation(2)
(2)
(2) ().
(1)
(1)
(2)
(2)
Figure 4. Rheological characteristic of IDDSI grade 3 fluids thickened by different commercial thickeners containing maltodextrin (MD). (a) shear viscosity; (b) variation in filament diameter during extension; (c) storage modulus G′ and loss modulus Gʺ; (d) schematic diagram of extensional rheology. (a), (b), and (c) are adapted from Ref. (Kongjaroen, Methacanon, and Gamonpilas Citation2022) with permission from Elsevier, Copyright 2022.
Notes: TUC represents is Resource Thickenup clear by Nestlé, composed of xanthan gum (33%), MD (66.4%), and potassium chloride (0.6%); TIC represents Thick-It Clear Advantage by Kent Precision Foods Group, composed of xanthan gum, MD, and ascorbic acid; QT represents QuikThik by Dr. MacLeod’s Medical food, composed of xanthan gum, MD, dextrose, and tricalcium phosphate (anticaking agent); SP represents Supercol by Supercol Australia, composed of guar gum (100%); PT represents Purathick by Parapharma Tech, composed of Tara gum, MD, and calcium carbonate.
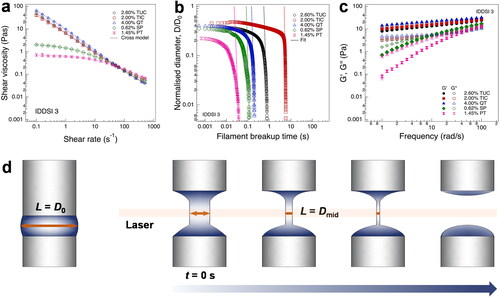
In these equations, σ is surface tension, D0 is the initial sample diameter (before stretch), and Dmid(t) is the mid-filament diameter at any given time. X is the geometry coefficient that accounts for the deviation of the filament shape from a uniform cylinder due to both inertia and gravity effects (Kongjaroen, Methacanon, and Gamonpilas Citation2022).
The extensional-rheological study has been corroborated using different polysaccharide–dextrin thickeners with the same viscosity in 50 s−1 and grade of IDDSI () (Kongjaroen, Methacanon, and Gamonpilas Citation2022). The filaments break-up time showed variations attributable to the assortment of polysaccharides and other components introduced. It is noted that the influence of additional components (e.g., MD, ascorbic acid, potassium) on XG as a natural anionic polyelectrolyte (TUC, Thick-It Clear Advantage (TIC), and QuikThik (QT)) was more evident than neutral polysaccharides like talar gum and guar gum (Supercol (SP) and Purathick (PT)) (Kongjaroen, Methacanon, and Gamonpilas Citation2022). Thickened fluids with a high extensional viscosity usually exhibit strong cohesion, which refers to the internal bonds that hold the product together, and these fluids tend to resist breaking into small droplets during swallowing, thereby improving swallowing behaviors (Hadde and Chen Citation2019; Hadde, Chen, et al. Citation2020). However, excessive extensional viscosity can result in heightened resistance to extensional deformation, especially for individuals with dysphagia who may have low tongue pressure (Hadde and Chen Citation2019).
3.1.2. Modulus
Modulus is yet another vital parameter that mirrors the flow characteristics of fluid matrices thickened with polysaccharide–dextrin, and it holds a significant connection to the safety of swallowing these fluids. Typically, the thickened fluids display viscoelastic behavior, demonstrating both elastic characteristics (indicated by storage modulus G′) and viscous features (reflected by loss modulus G′′). The ratio of G′′ to G′ gives rise to the loss factor, tan δ. An increase in G′ signifies that the fluid possesses a more extended deformation range, as manifested by increased extensional viscosity and cohesion (Schmidt et al. Citation2021). The tan δ value in the range of 0.1 to 1 is employed to indicate weak gel properties in foods and has been proposed as a criterion for foods that can be safely swallowed by individuals with dysphagia (Sharma et al. Citation2017). As depicted in , the G′′ values of the majority of polysaccharide–dextrin-thickened fluids, especially those primarily composed of xanthan gum, were lower than the G′ value (i.e., tan δ < 1), indicating the presence of weak gel properties (Kongjaroen, Methacanon, and Gamonpilas Citation2022).
Polysaccharide–dextrin-thickened fluids can exhibit different moduli compared to those thickened solely with non-dextrin polysaccharides. Kim, Yoo, and Yoo (Citation2014) and Yoon and Yoo (Citation2017) found that for pudding-like fluids thickened using two different thickeners containing XG and dextrin, G′ consistently exceeded G′′. In contrast, when MD was introduced into carboxymethylated curdlan (CMCD) and XG solutions, both G′ and G′′ increased (Wei et al. Citation2021), a trend also observed for GG- and LBG-thickened fluids in the presence of MD (Lee and Yoo Citation2021). This was attributed to MD enhancing the polysaccharide network, thereby boosting G′ (Wei et al. Citation2021; Kim, Kim, and Yoo Citation2017). Likewise, the use of indigestible dextrin (IDD), BLD, or CD heightened the viscoelasticity of KC-thickened fluid, linked to the enhanced helical structures of KC and the arrangement of random coils (Yuan et al. Citation2020; Yuan, Ritzoulis, et al. Citation2018). Compared to IDD, BLD, with its higher molar mass and branched structure, more effectively promoted the rearrangement of polysaccharide chains’ random coils, resulting in a more rapid increase in viscoelasticity (Yuan et al. Citation2020).
The aforementioned studies collectively demonstrate that dextrin inclusion enhances the viscoelasticity of polysaccharide-thickened fluids, thereby promoting ease of swallowing. Conversely, some instances have shown a reduction in G′ following the addition of dextrin to polysaccharide-thickened fluids. This effect was observed when a modified high-molar-mass dextrin (MWS-1000) (from waxy maize) was introduced, which suppressed starch retrogradation by increasing steric hindrance between starch chains. This, in turn, led to a reduction in G′ (and an increase in tan δ), rendering the system softer and more liquid-like (Sumida et al. Citation2021). The fluids containing KGM and MD exhibited lower G' values compared to the pure KGM-thickened fluid, as the steric hindrance induced by MD reduced the physical entanglement of KGM, consequently diminishing the mechanical resistance to strain (Wei et al. Citation2021). In addition, the excessive addition of dextrin tended to sterically hinder the aggregation of polysaccharide chains and reduce G′. Research showed that the G′ value of a KC-thickened fluid decreased when too much IDD and BLD (3% w/w and 5% w/w, respectively) were included (Yuan et al. Citation2020).
Extensional modulus (G), categorized as an elastic modulus, assume a critical role in preserving the structural integrity of thickened fluids during the act of swallowing. Utilizing the fitting Equationequations (1)(1)
(1) and Equation(2)
(2)
(2) for tensile viscosity, the tensile modulus and relaxation time can be derived through the following equation fitting (Yuan, Ritzoulis, et al. Citation2018):
(3)
(3)
In this equation, G is the extensional modulus, D0 is the initial filament diameter, and λ is the relaxation time.
Surface tension serves as an independent variable for fitting the extensional modulus and relaxation time. The parameter λ signifies the initiation of chain stretching in thickened fluids (Kongjaroen, Methacanon, and Gamonpilas Citation2022). Significantly, there has been a concerted effort to comprehend the extensional modulus in various fluid contexts, including those thickened with polysaccharides. For example, Yuan, Ritzoulis, et al. (Citation2018) determined the tensile modulus of okra mucilage by fitting the surface tension and filament diameter data. Further research is required to establish the suitability of the tensile modulus as a characterization parameter for polysaccharide–dextrin-thickened fluids for individuals with dysphagia. This is crucial for the evaluation and enhancement of dysphagia diets with improved swallowing performance.
3.1.3. Large amplitude oscillatory shear (LAOS) features
The modulus mentioned above is acquired through SAOS testing. SAOS is conducted within the linear viscoelastic region (LVR), representing the undisturbed structure of a fluid, describing its initial structural properties. On the other hand, LAOS testing assesses the viscoelastic modulus of a fluid beyond the LVR, simulating the disruption of the fluid’s structure, which closely resembles the conditions during oral food processing (Sukkar et al. Citation2018). The LAOS analysis protocol developed by Ewoldt, Hosoi, and McKinley (Citation2008) determines the nonlinear viscoelastic properties during LAOS measurements by utilizing the Fourier transform of higher-order harmonics. In addition, Ewoldt, Hosoi, and McKinley (Citation2008) defined two new viscoelastic moduli (minimum strain elastic modulus, , and maximum strain elastic modulus,
) and two new instantaneous viscosity at minimum shear rate (instantaneous viscosity at minimum shear rate,
, and instantaneous viscosity at maximum shear rate,
). These parameters can be viewed in the Lissajous-Bowditch diagram (). The relevant content is introduced in detail elsewhere (Melito, Daubert, and Foegeding Citation2013). Joyner (Citation2021) and Wang and Selomulya (Citation2022) have summarized the recent application of LAOS in the food industry, with a specific focus on the analysis techniques and findings obtained from LAOS studies conducted on various food products. It is anticipated that LAOS will find increasing application in the study of the relationships between food structure, function, texture, and sensory attributes. Research in the food industry has started to recognize the value of LAOS measurements.
Figure 5. (a) Example plots of Lissajous-Bowditch curves showing the determination of ,
, and
under SAOS (I, II) and LAOS (III, IV) testing. (b) Lissajous plots for A. homolocarpum seed gum aqueous dispersions treated at (I) different concentrations (at 25 °C) and (II) temperatures (at 1% w/v). Line colors indicate different strains: red- 0.1%, green- 10%, blue- 50%, and purple- 100%. (c) Correlation between droplet aspect ratio and maximum extensional viscosity.(a), (b) and (c) are adapted with permission from Ewoldt, Hosoi, and McKinley (Citation2008) with permission from The Society of Rheology, Copyright 2008, Anvari, Tabarsa, and Joyner (Citation2018) with permission from Elsevier, Copyright 2018 and Hadde, Chen, et al. (Citation2020) with permission from Elsevier, Copyright 2020, respectively.
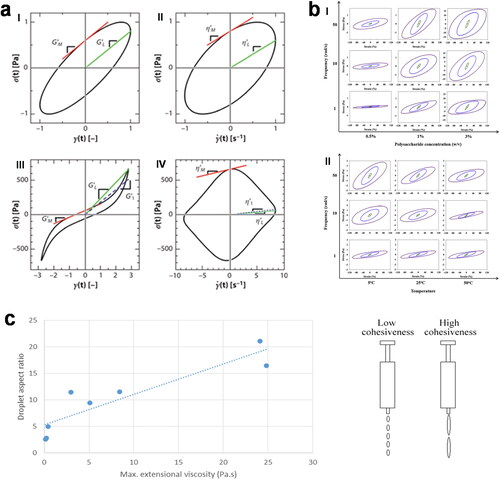
On the basis of Ewoldt, Hosoi, and McKinley (Citation2008), Anvari, Tabarsa, and Joyner (Citation2018) used a Lissajous-Bowditch plot and phase angle to characterize the effect of temperature and concentration on A. holocarpum seeds gum influence LAOS behavior (). In the LVR, the Lissajous-Bowditch plot turns out to be a perfect ellipse. Under large deformation, the ellipse morphs into a parallelogram. This suggests that the sample transitions from a predominantly elastic behavior to a predominantly viscous behavior. This is also confirmed by the increase of the phase angle at high strain and the change in the ratio between the third-order harmonic data (/
), the elastic modulus (
/
), and the instantaneous viscosity (
) from the output stress wave (refer to the paper of Melito, Daubert, and Foegeding (Citation2013) for analysis). These behavioral changes are related to permanent deformation and flow caused by gel network failure at high strain. High concentration and high temperature caused greater stability or smaller permanent deformation of polysaccharides under LAOS, respectively (Anvari, Tabarsa, and Joyner Citation2018). In addition, there was no significant difference in the XG’s flow behavior within the LVR with the presence of NaCl, but differences became apparent outside the LVR (Carmona et al. Citation2014). While the significance of LAOS in the oral processing of thickened fluids for dysphagia has been underscored, there is limited research on LAOS in the oral processing of polysaccharide-dextrin fluids.
3.1.4. Yield stress
Yield stress (τ0) represents the minimum stress required for a fluid to flow. In an ideal situation, a simple yield stress fluid is considered to be non-thixotropic and inelastic, i.e., the shear stress depends only on the applied shear rate. Therefore, Bingham, Herschel-Bulkley, Casson and other models are often used to calculate the yield stress of thickened fluids (Frigaard Citation2019). Dinkgreve et al. (Citation2016) used steady shear measurements method (Herschel–Bulkley model), oscillatory measurement, stress growth experiment, and creep experiment to measure the yield stress of simple yield stress fluid and thixotropic fluid. Among them, the yield stress obtained by Herschel–Bulkley model is considered to be reliable.
There remains limited research on the yield stress of dysphagia fluids. Hadde, Ann Yvette Cichero, and Michael Nicholson (Citation2016) posited that yield stress was associated with the tongue pressure during the swallowing reflex, suggesting that fluids with higher yield stress demanded greater tongue pressure. However, this view was refuted by Ross et al. (Citation2019), who uncovered that, despite significant variations in the perceived propulsion force among samples, there were no noteworthy differences in yield stress. Observations indicated that the addition of dextrin altered the yield stress of polysaccharide-thickened fluids. Specifically, the presence of MD increased the yield stress of XG and CMCD-containing fluids but decreased it in the case of KGM-thickened fluids (Wei et al. Citation2021). Yuan et al. (Citation2020) applied the Herschel-Bulkley model to fit the stress of a KC-thickened fluid. They found that the yield stress of the KC-thickened fluid containing IDD or BLD was higher than that of the KC-thickened counterpart when the dextrin concentration was below 5% and 7% w/w, respectively. The appropriate addition of dextrin can significantly reduce the yield stress (IDD and BLD content 5%) with less variation in consistency coefficient (K, namely zero-shear-rate viscosity). This suggests that a lower tongue pressure was required for swallowing, making it more suitable for older adults.
3.1.5. Thixotropy
Polysaccharide–dextrin-thickened fluids typically exhibit time-dependent characteristics. Thixotropy serves as an indicator of how time affects the viscosity of fluid foods, with methods such as hysteresis loops and three interval thixotropy tests (3ITT) being employed for assessment (Li et al. Citation2023). The primary approach is 3ITT, encompassing static, shear and structural recovery stages (i.e., low shear rate, high shear rate, and then low shear rate again). This method allows for both rotational (viscosity) and oscillatory tests.
Thixotropy imparts distinct viscosity behavior to thickened fluids at rest compared to when subjected to shear, a factor closely tied to the fluid’s safety for swallow (Dewar and Joyce Citation2006). Individuals managing dysphagia should consider the timing effect when consuming thickened fluids. Dewar and Joyce (Citation2006) conducted tests examining the thixotropic behavior of corn starch and MD systems in dysphagia treatment. Their findings revealed the thixotropic nature of the corn starch system, while the MD system was not thixotropic, and dextrin-based thickened matrices exhibited a Newtonian flow regime (Dewar and Joyce Citation2006). Further research is essential to comprehend whether including dextrin in polysaccharide-based fluids influences hydrogen bonding and chain entanglements, leading to variations in thixotropic behavior.
3.1.6. Cohesiveness and adhesiveness
The significance of cohesiveness and adhesiveness in the context of swallowing thickened fluids cannot be overlooked, although there is currently no universally accepted quantification standard (Nishinari et al. Citation2019). Cohesiveness signified the degree of the intermolecular attraction within a food and the cohesion of its particle components. Increasing cohesiveness contributes to the formation of a well-bound, lubricated bolus, which in turn aids in averting aspiration. The swallowing perception test confirmed a robust positive correlation between the cohesiveness of food during swallowing and the extensional viscosity (Nishinari et al. Citation2019). At the shear rate of 10 s−1, the perceived cohesion had the strongest correlation with the viscosity of the thickened fluid (r = 0.97) (Ross et al. Citation2019). Hadde, Chen, et al. (Citation2020) proposed a strong relationship between the maximum extensional viscosity of a fluid and the filament breaking time, both of which are closely linked to the cohesiveness of visual perception during swallowing (). In addition, the droplet aspect ratio, determined using the syringe extrusion flow behavior technique, serves as a quantifiable measure of cohesiveness. High cohesiveness materials exhibit greater elongation before breaking down into droplets compared to materials with low cohesiveness (Hadde, Chen, et al. Citation2020). Adhesiveness pertains to a sticky food’s capacity to adhere to the lining of the mouth. Elevated adhesiveness typically demands increased tongue pressure, thereby heightening the risk of suffocation. Vickers et al. (Citation2015) showed that fluids exhibiting a higher degree of shear thinning (low n and high K) are typically associated with reduced adhesiveness. Swallowing is observed when the act of chewing produces an optimal food particle size, and the cohesiveness and adhesiveness between these particles in the subsequent bolus formation reach their maximum levels (Sun-Waterhouse et al. Citation2021). Quantification of cohesiveness and adhesiveness will help to investigate the behavior of thickening fluid during swallowing.
3.2. Tribology
Tribology is the science of friction, wear, and lubrication of surfaces in relative motion, finds significance in oral processing, where it is termed oral tribology. This specific term refers to the study of the interaction between food and the internal surface of the mouth during food consumption (Methacanon et al. Citation2021). As a burgeoning technology, oral tribology addresses the limitations of sensory testing in assessing swallowing rheology and safe swallowing. For example, the coefficient of friction plays a role in the astringency of food (Pradal and Stokes Citation2016), and the easy of swallowing may be related to the lubricating properties of food (Araiza-Calahorra et al. Citation2023).
In the journey of swallowing, the perception of food transitions from rheology to tribology (You, Murray, and Sarkar Citation2021). The Stribeck curve categorizes the lubrication states of fluids in the oral environment into boundary lubrication, mixed lubrication, and fluid lubrication. In the process of fluid lubrication, rheology is dominant, and the thickness of the fluid is enough to separate the friction contact surface. Fluid lubrication corresponds to the beginning of food oral processing. The oral lubrication film formed by food and saliva separates the tongue from the palate, with the texture perception primarily influenced by the rheological properties of the food, such as viscosity. Moving into mixed lubrication, tribological factors progressively assume a more significant role. As oral processing advances, the contact between the tongue and the palate’s surface intensifies, impacting oral friction, which, in turn, shapes the texture perception of food (You, Murray, and Sarkar Citation2021).
The measurement of bolus friction behavior stands out as a pivotal challenge in tribology. To simulate the soft properties of the tongue, deformable material like polydimethylsiloxane (PDMS) are often used, mimicking the tongue’s elasticity (Sarkar et al. Citation2021). Frosted glass, with appropriate surface roughness, emulates a hard palate (Funami and Nakauma Citation2021). Several devices, including friction tester, mounted tribological device, mini-traction machine, have been employed to measure food tribology (Sethupathy, Moses, and Anandharamakrishnan Citation2020). Sarkar et al. (Citation2021) suggested techniques such as small angle X-ray scattering (SAXS), quartz crystal microbalance with dissipation monitoring (QCM-D), and atomic force microscopy (AFM) to complement the friction properties of foods, enhancing the study of food tribology.
The “lubricating” property of the fluid, an opposite factor to the fluid’s friction coefficient, plays a pivotal role in bolus formation and swallowing (Laguna and Sarkar Citation2017). The lubrication properties of fluids are affected by particles. For example, Ji et al. (Citation2022) demonstrated that the lubrication properties of starch suspensions are affected by particle size and swelling. Better swelling capacity correlates with larger, round starch particles having a lower friction coefficient. The presence of starch particles provides sufficient dynamic force to eliminate boundary lubrication, enhancing the overall lubricating effect (You, Murray, and Sarkar Citation2021). Notably, the presence of saliva mitigates friction differences caused by particles (Ji et al. Citation2022). Additionally, viscosity affects the lubrication properties of fluids. In the presence of artificial saliva, the coefficient of friction decreased at high slip speeds compared to pure starch-based thickeners (ambiguous and refined commercial thickeners). The XG thickeners mixed with starch consistently exhibited the highest coefficient of friction, which was considered to be influenced by viscosity (Baixauli et al. Citation2023). Gum-based thickeners (e.g., flaxseed gum and XG) were found to possess superior lubricating properties compared to starch with equivalent viscosity (Vieira et al. Citation2021; Gamonpilas, Kongjaroen, and Methacanon Citation2023). Baixauli et al. (Citation2023) observed that consumers preferred fluids with smooth texture (low coefficient of friction), tasteless, and refreshing properties, endorsing gum-based thickeners and dispersed-starch thickeners over starch-containing particles. Furthermore, differences in the adsorption properties of polysaccharides on soft elastomer surface (e.g., PDMS) suggested varied ways in which polysaccharides are adsorbed in the oral cavity, impacting fluid film maintenance between surfaces, achieving ultra-low friction (Rodrigues et al. Citation2021).
In recent years, tribology has emerged as a crucial tool in uncovering sensory aspects that influence food swallowing. The confirmation of bolus swallowability hinges on reaching threshold values for particle size, cohesiveness, and lubrication (Malone, Appelqvist, and Norton Citation2003). The preliminary judgment of the swallowing characteristics of thickened fluids through the measurement of the coefficient of friction holds significant implications for the development of thickeners for dysphagia.
3.3. Other characteristics
Additional flow characteristics associated with polysaccharide–dextrin-thickened fluids include density and surface tension, both of which are linked to the swallowing dynamics of these fluids.
Density is intricately linked to the fluid quality and exhibit a positive correlation with swallowing velocity (Steele and Cichero Citation2008; Mizunuma, Sonomura, and Shimokasa Citation2020). Following Newton’s second law, the acceleration of the bolus, indicative of the rate of velocity change, can be influenced by mass, a factor linked to density. Therefore, with a fixed volume and force applied to the bolus, density consistently influences the bolus’s entry into the oral cavity and its acceleration into the pharynx. As viscosity increases, the flow tends to simplify into a theoretical type of lubrication, with density playing a diminished role. Fluid dynamics in oral processes are primarily governed by fluid density, viscosity, and tongue pressure. In the case of low-viscosity boluses (< ∼100 cP), density significantly dictates fluid dynamics, while for high-viscosity boluses (> ∼1000 cP), system viscosity takes prevalence (Nicosia and Robbins Citation2001).
Moreover, the effects of surface tension on the extensional rheological parameters of thickened fluids have attracted significant attention (Nishinari et al. Citation2019; Gallegos et al. Citation2021; Hadde, Nicholson, et al. Citation2020). The formation of a fluid bolus is related to its surface tension, and a higher surface tension makes the bolus more cohesive (related to extensional rheology), which causes a reduced flow rate in the mouth and pharynx (being friendly to people with dysphagia) (Lucas et al. Citation2002). It was reported that XG- and carrageenan-thickened fluids at high concentrations showed an increased surface tension, presumably due to increases in viscosity and gelation (Poursani, Razavi, and Norouzi Citation2021; Huang, Kakuda, and Cui Citation2001). However, a lower surface tension does not mean a lower viscosity.
Higher thickener concentration can lead to a reduction in surface tension. For instance, elevating the concentration of a polysaccharide–dextrin-based thickener (such as TUC and TIC) was found to reduce the surface tension of the thickened fluid by mitigating the cohesion resulting from the buildup of the biopolymers on the surface. This effect could be counteracted by increasing the extensional viscosity (Hadde, Nicholson, et al. Citation2020; Kongjaroen, Methacanon, and Gamonpilas Citation2022).
In addition, the choice of measurement method can impact the relationship between concentration and surface tension. As reported by Lee et al. (Citation2012), for thickened fluids by sodium alginate, carboxymethyl cellulose (CMC), XG, and pectin, the surface tension from the DuNouy ring method increased as the solid concentration rose, but the drop weight method showed an opposite trend. This discrepancy arises because the du Nouy ring is affected by the viscous force of the thickened fluid during surface tension measurement, causing variations in the force required for the ring to detach from the liquid surface of the sample or the maximum weight it can support, leading to significant errors. Despite the need for some corrections, the drop weight method is considered more appropriate for measuring the surface tension of thickened fluids due to its consistency with surface thermodynamic theorems (Lee et al. Citation2012). This finding hints to us that for establishing assessment criteria for dysphagia fluid diets, both the surface tension and the flow characteristics should be considered to precisely regulate the swallowing performance of thickened fluids.
Therefore, significant efforts have been devoted to understanding the flow characteristics of fluids thickened with polysaccharides and dextrin. This research is invaluable for the assessment and enhancement of dysphagia-friendly fluid foods. While some flow parameters like shear viscosity and modulus have received substantial attention, others, such as extensional rheological behaviors, tribology and the interactions between polysaccharide and dextrin, remain insufficiently explored. To enhance the precise control of flow behavior during swallowing, further research is imperative to establish correlations between the polysaccharide–dextrin interplay and the flow properties of polysaccharide–dextrin-thickened fluids. This aims to enable more accurate regulation of fluid behavior at the molecular level. Furthermore, extending this technique to the investigation of polysaccharides and other substances (e.g., polysaccharides–polysaccharides, polysaccharides–proteins, polysaccharides–lipids) is crucial. This extension will contribute to the development of more effective evaluation methods for assessing the swallowability of dysphagia diets.
3.4. Influences of environmental factors
Thickeners commonly encounter a range of complex challenges. Environmental factors (pH, dispersion medium, temperature and ionic strength) and saliva can affect the rheological properties of polysaccharide–dextrin-thickened fluid, consequently altering swallowing behavior.pH. Thickeners encounter varying pH environments. Normally, food tends to be acidic (pH = 3–7). This means that testing only in water (approximately neutral) is not appropriate when considering the effect of dysphagia thickeners. Yoon and Yoo (Citation2016) examined the flow behavior of thickened fluids using a commercial thickener based on XG–GG–dextrin and another one based on starch–LBG–dextrin at different pH values (pH = 3, 4, 5, 6, and 7). At pH 3, both thickeners exhibited a low K value and a high n value at pH 3. As the pH increased, the XG–GG–dextrin thickener exhibited an escalating K value while n, G′, and G″ decreased. While XG is typically recognized for its ability to maintain high viscosity across a broad pH spectrum, it is important to note that XG molecules can degrade, and experience suppressed electrostatic action at extreme pH levels. In such conditions, the molecular structure becomes more compact, leading to a reduction in viscosity. The flow behavior of starch-based thickeners remained relatively stable within the pH range of 4–7, and K decreased significantly at pH 3. In contrast, Hadde, Nicholson, and Cichero (Citation2015) found that the viscosity, yield stress, and thickening kinetics of an XG-based thickener did not change significantly in carbonated beverage (pH 2.75) and herbal tea (pH 9.50). The difference between these studies may arise from the different sources of XG. When utilizing polysaccharide–dextrin thickeners, it is crucial to identify the pH range for stable thickening effects and ensure that the thickened fluid reaches the appropriate swallowing grade.
Dispersing media. Dispersing media include protein, fat, pigment, salt and other substances, which can impact the thickening effect of polysaccharides. These different dispersion media were studied. Kongjaroen, Methacanon, and Gamonpilas (Citation2022) investigated the shear and stretching behaviors of XG (with dextrin and KCl) and GG in water, milk, and apple juice. In all dispersion media, XG and GG exhibited higher zero-shear viscosity than water, but at high shear rates (especially 50 s−1), their viscosity equalized. Furthermore, XG dissolved in apple juice and milk, as well as GG in milk, displayed more pronounced viscoelastic solid-like behavior than water. Particularly noteworthy are the differences in extensional characteristics, with XG showing a lower filament break time and extensional viscosity than water in both milk and apple juice. The extensional properties of GG were enhanced in milk and decreased in apple juice. Sopade et al. (Citation2007, Citation2008a, Citation2008b) studied the flow properties of different thickeners across a range of liquids, including water, raspberry cordial, homogenized and pasteurized full cream, skim milk, ultra-high-temperature or ultra-heat-treated full cream, and different fruit juices (apple, orange and pineapple). Notably, the viscosity, density, and yield stress of skimmed milk after thickening were found to be significantly higher than those of whole milk, contrary to the findings of Hadde, Nicholson, and Cichero (Citation2015), which suggested a direct correlation between milk fat content and viscosity. However, the juices showed no significant changes in density, yield stress, and viscosity after thickening. Karataş and Aydoğmuş (Citation2023) compared the flow behavior of XG and pectin when dispersed in lemon juice. Lemon juice, in comparison to water, displayed reduced surface tension after thickening and expedited dissolution of the polysaccharides, resulting in higher viscosity. These findings underscore the importance for consumers to have clear guidelines regarding the dosage and application range of polysaccharide-dextrin-based thickeners in various dispersion media.
Temperature. Temperature plays a crucial role in influencing the rheological properties of fluids, especially thickened fluids. Lower temperatures are associated with increased viscosity in the thickened fluid (Adeleye and Rachal Citation2007). Hadde, Nicholson, and Cichero (Citation2015) showed that the viscosity and yield stress of high-concentration Resource ThickenUp Clear (which is XG-based) remained stable, whereas the thickener’s viscosity and yield stress decreased as the temperature increased at a low concentration. Hong et al. (Citation2012) assessed the flow characteristics of an XG-based thickener at temperatures of 5, 25, 35, and 50 °C. The results indicate that the flow characteristics of the XG-based thickener remained relatively stable within the range of 5–35 °C, but there was a notable decrease in η50 (viscosity at 50 s−1) and K at 50 °C. Furthermore, it is important to note that both temperature and taste are significant factors influencing swallowing. By employing cold and acidic simulation, it is possible to reduce the pharyngeal passage time in patients with oropharyngeal dysphagia, thus serving as an adjuvant therapeutic approach (Cola et al. Citation2012).
Ionic strength. Adding minerals to thickeners can not only enhance their nutritional value but also alter their flow characteristics. However, in the realm of commercial thickener research, ionic strength remains a relatively understudied factor when compared to other influential variables. Cho, Yoo, and Yoo (Citation2015) found that different thickeners based on XG and other polysaccharides exhibited varying flow behaviors with NaCl concentrations (0.3%, 0.6%, 0.9%, and 1.2%). Low NaCl concentrations (0.3%) showed synergy with XG, while high NaCl concentrations (>0.3%) reduced K, yield stress, and η50. This phenomenon may arise from Na+ ions shielding the side chain groups of XG, leading to viscosity reduction. Moreover, the addition of 0.6–1.2% NaCl reduced shear thinning and increased n, improving taste. Notably, when 0.6% NaCl was added, the combination of XG, GG, and dextrin consistently exhibited a synergistic effect on G′ within the tested Na+ concentration range. In contrast, the complex of XG, CMC, GG, and dextrin showed lower G’ at high NaCl concentrations. Other thickeners also experienced inhibition at high concentrations but higher G′ values than without NaCl. Kongjaroen, Methacanon, and Gamonpilas (Citation2022) suggested that the presence of cations like K or Na in milk (Na 41.7 mg/100g) and apple juice (Na 12.5 mg/100g) could mitigate repulsive interactions between polyelectrolyte chains. These chains then interact and form hydrogen bonds, thereby increasing their viscosity, especially at low shear rates. In essence, a low concentration of ions in combination with polysaccharides demonstrates a synergistic effect, and an appropriate increase of ionic strength is conducive to achieving better thickening effects.
Saliva. Saliva plays a vital role in oral hygiene, oral wear and lubrication, and the processing of food in the mouth (including the formation and movement of boluses and the transmission of odors) (Mu and Chen Citation2023). It accounts for a significant difference between simulated swallowing and actual mouth conditions. When swallowing occurs, saliva fills the gaps between particles, enhancing cohesion and lubrication, ultimately reaching the threshold for triggering the swallowing reflex (Mosca and Chen Citation2017). This process facilitates bolus formation and movement. Furthermore, the interaction between saliva and the bolus contributes to food digestion, as saliva contains salivary amylase. The presence of starch mixed with saliva results in the decomposition of macromolecules and a notable reduction in viscosity. Vallons et al. (Citation2015) subjected a starch-thickened fluid (1315 ± 364 mPa·s) and a gum-based thickened fluid (1,376 ± 252 mPa·s) to various durations of oral treatment to assess changes in fluid viscosity. Following oral treatment for 10 s, the viscosity of the starch-thickened fluid decreased to 383.6 mPa·s, while the gum-base thickened fluid decreased to 901.1 mPa·s. After 20 s, the starch-thickened fluid transitioned from a honey-like viscosity to a nectar-like viscosity, while the viscosity of the gum-based thickened fluid remained unchanged. Turcanu et al. (Citation2018) showed that salivary amylase significantly reduced the axial force of a starch-thickened fluid (by about 15 mN) but did not significantly impact a gum-based thickener. The effect of saliva on starch can be reduced by combinations of different polysaccharides or within an acidic environment (pH < 3.6) (Hanson, Cox, et al. Citation2012). Hanson, O’Leary, et al. (Citation2012) compared the viscosity between a starch-based thickener and that added with gum under saliva. Their study revealed that saliva reduced the viscosity of both thickened fluids. While the starch-based thickened fluid underwent complete decomposition, the compound thickener retained a certain level of consistency. In contrast, no measurable reduction in viscosity was observed for thickened orange juice (pH 3.8).
Hence, examining the flow characteristics of polysaccharide–dextrin systems in diverse environments expands the dietary options for dysphagia patients, aiding in reducing resistance to eating and addressing malnutrition. The thickening effect of polysaccharide–dextrin thickeners varied with pH, dispersion medium, temperature, and ionic strength. Sourness, coldness, and appropriate temperature play a beneficial role in encouraging patient swallowing. Within the pH range of 4–7, at low temperatures and reduced ionic strength, most polysaccharide–dextrin-thickened fluids demonstrate stable and effective thickening. When saliva is present, gum-based thickeners exhibit more stability compared to starch. However, it is worth noting that the influence of low pH (<3.6) on saliva decomposition should not be overlooked.
Subsequent studies should delve into the interconnections among shear and extensional behaviors, tribology, and inherent fluid properties (e.g., density and surface tension), and their correlation with swallowing. Building on this foundation, there should be a concerted effort to simulate the clinical conditions of dysphagia diets. This involves a meticulous examination of the rheological changes of the fluid under substantial large deformation, considering both extensional and frictional properties. Additionally, it is essential to select temperature, pH, and salt concentrations that align with the specific requirements of a patient’s diet. Further investigations ought to concentrate on unraveling the link between molecular interactions, facilitating a systematic evaluation of the characteristics of polysaccharide–dextrin mixtures (or polysaccharides mixed with other substances) in the context of swallowing.
4. Methods to evaluate swallowing of polysaccharide–dextrin-thickened fluids for individuals with dysphagia
By incorporating the flow characteristics discussed above, a range of evaluation methods have been developed to realistically simulate the swallowing attributes (e.g., flow velocity, risk of aspiration, and residual amount) of polysaccharide–dextrin-thickened fluids. These advancements are fundamental in crafting specialized fluid diets for individuals with dysphagia. This section provides an overview of various swallowing assessment methods (in-vivo, in-vitro, and in-silico) developed in recent years for thickened fluid systems.
4.1. In-vivo evaluation methods for fluid swallowing behaviors
4.1.1. Clinical examination
Different clinical methods, such as videofluoroscopy swallowing study (VFSS), endoscopy, swallowing acoustics, surface electromyography (sEMG), and computed tomography (CT)., have been employed to examine the swallowing characteristics (e.g., flow velocity and aspiration risk) of fluids thickened with polysaccharide-based thickeners (Koyama et al. Citation2021).
VFSS is considered a standard method to judge the swallowing status (Pavithran et al. Citation2020). This technique based on X-ray enables us to visualize the swallowing behaviors of thickened fluid boluses in the oral phase (e.g., food spillage), pharyngeal phase (e.g., fluid residue and aspiration), and esophagus phase (e.g., food stagnation and reflux) (Palmer et al. Citation1993). Utilizing VFSS images, it becomes possible to ascertain how the flow characteristics (e.g., shear viscosity) of polysaccharide–dextrin-thickened fluids impact their swallowing safety (Steele et al. Citation2019; Bolivar-Prados et al. Citation2019; Vilardell et al. Citation2016). Bolivar-Prados et al. (Citation2019) employed VFSS to evaluate Nutilis Clear (containing MD, XG, and GG)-thickened fluids with varying shear viscosities (ranging from 150 to 2000 mPa·s). It was noted that the swallowing safety of these fluids predominantly hinged on their viscosity, while the roles of other rheological attributes (e.g., elasticity, adhesion, cohesion, and tensile viscoelastic characteristics) warrant further scrutiny (Bolivar-Prados et al. Citation2019). Similar findings have been reported in other investigations (Steele et al. Citation2019; Vilardell et al. Citation2016).
However, there are certain limitations associated with VFSS examinations. Typically, a contrast medium (e.g., barium) is introduced to enhance the visualization, resulting in alterations to the rheological properties of fluid boluses, rendering them more viscous (Park, Yoo, and Yoo Citation2019; Barbon and Steele Citation2019; Park and Yoo Citation2021). Furthermore, the presence of barium leaves a coating on the mucous membranes of the pharynx and esophagus, thereby obscuring the inherent nature of the fluid (Steele et al. Citation2013). Additionally, to accurately capture muscle forces acting on the fluid bolus, VFSS necessitates coordination with invasive endoscopic examinations, such as fiberoptic endoscopic evaluation of swallowing (FEES) and high-resolution manometry (HRM). These endoscopic methods entail catheter insertion into the body, which can impede and disrupt the flow of the fluid bolus flow during swallowing (Koyama et al. Citation2021).
In light of this, a noninvasive clinical approach, sEMG, has been employed to examine the swallowing characteristics of thickened fluids. This method gauges the electrical signals emanating from muscle activities associated with fluid ingestion (Zhu et al. Citation2017), as different fluids elicit varied muscle responses during swallowing (Vaiman and Eviatar Citation2009). Koyama et al. (Citation2021) reported that thickened fluids containing 2% dextrin exhibited longer muscle activity durations compared with water, indicating a reduced fluid flow velocity (and, consequently, diminished aspiration risk) during swallowing. Additionally, it was observed that augmenting the shear viscosity of sesame paste could prolong the swallowing cycle (Zhu et al. Citation2017). Another noninvasive method for assessing fluid bolus swallowing is swallowing acoustics, which involves analyzing the sounds generated within the organs (Enz et al. Citation2021; Nakauma et al. Citation2011). Nakauma et al. (Citation2011) disclosed that XG (0.3% to 0.9%)-thickened fluids exhibited a more coherent flow pattern (as opposed to a scattered flow) when compared to thickened counterparts with LBG (ranging from 0.5 to 0.8%). Despite these advancements, it is imperative to integrate these noninvasive methods with VFSS to gain a comprehensive understanding of how the remaining attributes of polysaccharide–dextrin-thickened fluids affect their swallowing behaviors.
CT, as another new evaluation mode, addresses the limitations of VF through dynamic three-dimensional observation. Typically, the patient ingest a fluid containing a contrast agent, facilitating a multi-phase scan of the 3D image to capture a dynamic swallowing image. This approach enables detailed measurements and a broader quantification of swallowing dynamics. Notably, CT introduced a groundbreaking visualization of vocal cord closure (the last line of defense during swallowing), allowing, for the first time, the laryngeal closure (glottis closure, laryngeal vestibular closure, and epiglottic inversion) processes safeguarding the airway to be independently observed and measured. The movement of the upper esophageal sphincter (UES) was also distinctly observable in this technique (Inamoto, González-Fernández, and Saitoh Citation2022; Inamoto, Ueha, and Gonzalez-Fernandez Citation2023). Besides, 3D-CT excels in accurate structural measurement and time recording. As a result, CT gained widespread adoption in the study of swallowing since 2011. For example, Inamoto et al. employed 3D-CT to scrutinize the movement of 10 mL of honey concentrated barium (5% v/w) and thin barium (5% v/w) in a 45° inclined position. The findings revealed that for thin fluids, the bolus reached the lower pharynx earlier and remained longer in the lower pharynx compared to thickened fluids (Inamoto et al. Citation2013). The use of CT scanning of the human body will be more beneficial to construct a model of the swallowing process and better simulate the flow of thickened fluids.
Clinical examinations tend to prioritize the assessment of physiological processes during the ingestion of polysaccharide-thickened fluids. While the methods offer insights into the correlations among flow parameters of polysaccharide–dextrin-thickened fluids and their swallowing traits, conducting such investigations within the human body presents ethical and economic challenges. To aid the development of dysphagia diets, there is a need to establish animal models, as well as in-vitro and in-silico approaches, to authentically evaluate the swallowing dynamics of polysaccharide–dextrin-thickened fluids with diverse flow characteristics.
4.1.2. Animal model
Animal models (e.g., rodent, pig, and dog) can serve as valuable tools for assessing the swallowing characteristics of thickened fluids, including those employing polysaccharide–dextrin systems. These models offer the advantage of fewer ethical issues and reduced individual variabilities. Utilizing these animal models for swallowing assessments of thickened fluids provide a means to validate their efficacy in dysphagia management (Au-Lever et al. Citation2015; German et al. Citation2017; Lammers et al. Citation2020; Harris et al. Citation2017; DeLozier et al. Citation2018). To date, a range of animal models with dysphagia have been established, such as elderly, nerve injury, Parkinson’s disease, stroke, amyotrophic lateral sclerosis, and Down syndrome (Russell et al. Citation2013; Cullen et al. Citation2018; Cullins and Connor Citation2019; Sugiyama et al. Citation2014; Osman et al. Citation2020; Glass, Twadell, et al. Citation2019; Glass, Valmadrid, et al. Citation2019; Wang et al. Citation2017; Welby et al. Citation2020; Gould et al. Citation2018). Kletzien, Cullins and Connor (Citation2019) conducted VFSS examinations on aging rodents and disclosed that the aging process altered the swallowing biomechanics of peanut butter (IDDSI level 4) and influenced the bolus flow behavior (Kletzien, Cullins and Connor Citation2019). In an aging dysphagia dog model, Harris et al. (Citation2017) showed that thin fluids were ingested more rapidly than purees matrices, aligning with VFSS findings in humans. Additionally, research indicated that higher viscosity in XG-thickened fluids resulted in prolonged retention in the lungs, potentially leading to more severe lung injuries (Araie et al. Citation2020; Nativ-Zeltzer et al. Citation2018).
Nonetheless, to date, there is a lack of sufficient understanding of whether animal models can provide a comprehensive swallowing assessment of the swallowing properties of polysaccharide–dextrin-thickened fluids, taking into account the influence of various flow parameters. Further research is required to validate the efficacy of thickened fluids utilizing polysaccharide-based thickeners through animal models, particularly with regard to their relevance to the human body.
4.2. In-vitro evaluation methods for fluid swallowing behaviors
To address ethical concerns and enhance data reproducibility, in-vitro simulations have been developed to visually and realistically assess the swallowing performance of polysaccharide–dextrin-thickened fluids under varying fluid flow parameters (Marconati, Engmann, et al. Citation2019).
Using in-vitro modeling methods could capture the swallowing performance of diverse thickened fluid matrices (), with several models discussed below. The Cambridge Throat model is the most commonly used in-vitro model in laboratory settings () (Mackley et al. Citation2013). This model combines tongue pressure and fluid flow parameters (e.g., viscosity) to illustrate the oropharynx phase of swallowing thickened fluids (Patel et al. Citation2020). Using the Cambridge Throat, various polysaccharide-based thickeners, such as Nutilis (including XG, GG and MD), TUC (containing MD and XG), THU (containing modified starch), XG, and CMC, have been assessed. It allows for the collection of data on parameters, such as swallowing time, stretch length, and bolus position. Increasing the thickener concentration has been linked to longer swallowing times, indicative of enhanced swallowing safety. Also, this model reveals the role of extensional behaviors of thickened fluids during swallowing. It is noteworthy that this model simulates a scenario where the epiglottis is impaired, and the bolus, driven by gravity, traverses the esophagus, which differs from peristaltic esophageal transport (Marconati, Lopez, et al. Citation2019; Mowlavi et al. Citation2016; Mackley et al. Citation2013; Patel et al. Citation2020).
Figure 6. In-vitro simulation equipment: (a) the cambridge throat; (b) sketch of the gothenburg pharynx model; (c) Overview and side view of the Swall-E swallowing robot.(a) is adapted from Ref. (Mowlavi et al. Citation2016) with permission from Elsevier, Copyright 2016. (b) is adapted from Ref. (Qazi et al. Citation2019), and (c) is adapted from Ref. (Fujiso et al. Citation2018).
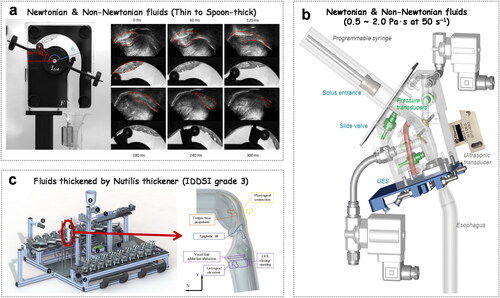
Table 3. In-vitro methods assessing fluid swallowing behaviors.
On the other hand, the Gothenburg Throat model has the capability to incorporate shear rate and pressure, which are significant factors in assessing the dynamic swallowing of various flowing thickened fluids () (Qazi et al. Citation2019). The model can replicate the velocity and shape of a fluid bolus and its movement into the pharynx, UES, and nasopharynx (Stading et al. Citation2019). It was employed to analyze rapeseed oil and a thickened fluid using Fresubin Clear Thickener (containing modified starch, MD, XG, and modified cellulose). Results indicated that the rapeseed oil fluid showed a parabolic curve in terms of shear rate, while the velocity profile of the Fresubin Clear fluid exhibited a plug flow curve due to the presence of surfactants. However, when it came to pressure measurement, rapeseed oil showed no difference from Fresubin Clear fluid (Stading et al. Citation2019). Furthermore, for honey-like (1 Pa·s) and pudding-like (2 Pa·s) fluids thickened with Nutricia Nordic AB (Stockholm, Sweden), the thicker fluids exhibited slower flow rates, lower shear rates and higher pharyngeal pressures than the thinner fluids.
In addition, Fujiso et al. (Citation2018) developed a robotic simulator called Swall-E () to replicate the pharyngeal phase of human swallowing. This innovative model was used to assess a fluid (IDDSI grade 3) thickened with a Nutilis thickener containing MD, XG, and GG. During simulation, high-speed camera observations captured the velocity and motion of the fluid within the model, and the residue of the fluid bolus was recorded. The accuracy of the model was validated by comparing its results with VFSS images (Fujiso et al. Citation2018).
4.3. In-silico evaluation methods for fluid swallowing behaviors
In-silico simulation methods have been established for the noninvasive and visual assessment of the swallowing performance of thickened fluids, including those with polysaccharide–dextrin formulations (). These simulations involve modeling the swallowing process of thickened fluids using mesh-based methods, such as Euler, Lagrangian, and arbitrary Lagrangian-Euler (ALE), and organ representations are created using CT and VF images, along with other methods like finite element model (FEM) and finite volume model (FVM) (Sonomura et al. Citation2011; Ho et al. Citation2017; Michiwaki et al. Citation2020). To perform these simulations, physical and flow parameters (e.g., viscosity, density, and bolus volume) of thickened fluids are utilized as input parameters. In essence, in-silico models can depict the relationship between the input flow parameters of various thickened fluids and their resulting swallowing characteristics.
Table 4. In-silico methods assessing fluid swallowing behaviors.
Mizunuma et al. (Citation2009, Mizunuma, Sonomura, and Shimokasa Citation2020) and Sonomura et al. (Citation2011) employed the Euler mesh method to simulate water and non-Newtonian fluids with varying viscosities in assessing oropharyngeal swallowing dynamics (). Their simulations involved modeling the movement of different fluid volumes within a healthy model and two dysphagia models characterized by epiglottis dysfunctions. Their findings indicated that higher viscosity led to reduced fluid flow velocity, and the aspiration pattern was influenced by bolus size. In cases of dysphagia marked by premature termination of the swallowing process, excessively viscous fluids moved slowly, and residual fluid entered the trachea after epiglottis closure, resulting in aspiration (Sonomura et al. Citation2011; Mizunuma et al. Citation2009; Mizunuma, Sonomura, and Shimokasa Citation2020).
Figure 7. In-silico simulation equipment: (a) finite element model (FEM) of fluid simulation with euler meshes; (b) smoothed particle hydrodynamics (SPH) with a dynamic mesh as the fluid boundary condition. The bolus is grey and streaky, and the air is dark; (c) Hamiltonian moving particle semi-implicit (HMPS) method. The simulation of swallowed non-Newtonian fluids in patients with mild dysphagia appeared to be penetrating and corresponded well to the VFSS images.(a) is adapted from Ref. (Sonomura et al. Citation2011) with permission from John Wiley & Sons, Copyright 2011. (b) is reprinted from Ref. (Ho et al. Citation2017) with permission from Elsevier, Copyright 2017. (c) is adapted from Ref. (Michiwaki et al. Citation2020) with permission from Elsevier, Copyright 2020.
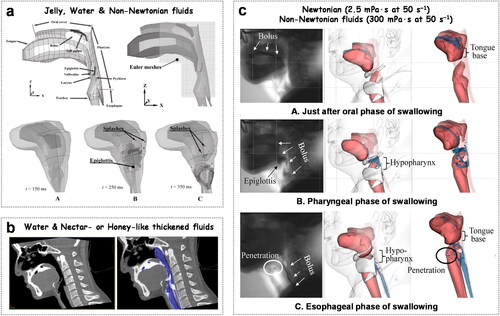
Furthermore, mesh-based methods like ALE were employed to simulate and monitor the changes in the fluid boundary (Hirt, Amsden, and Cook Citation1974). Nicosia (Citation2007) utilized the ALE mesh method to simulate thickened fluids with varying viscosities (1000 and 10 cP). The data revealed that high-viscosity fluids could be better retained in the oral cavity compared to low-viscosity fluids. However, it is important to note that the meshes methods can exhibit significant distortion when the fluid exceeds its boundaries, which is undesirable for accurately assessing the swallowing behaviors of thickened fluids (Nicosia Citation2007).
To address this challenge, mesh-free methods have been proposed, such as smoothed particle hydrodynamics (SPH) and the moving particles semi-implicit (MPS) method. These approaches offer advantages in simulating two-phase flows, fluid splashes, and intricate 3D geometries (Kamiya et al. Citation2013). Ho et al. (Citation2017) applied the SPH method to simulate throat fluid flow in the presence of saliva lubrication, using both thin and nectar- and honey-like fluids for testing (). The results indicated that fluid flow closely resembled reality when lubrication was absent, although residual levels were significantly higher than actual values.
While SPH is suitable for simulating compressible fluids, the MPS method is employed for simulating incompressible fluids. Michiwaki et al. (Citation2020) introduced the Hamiltonian moving particles semi-implicit (HMPS) method, derived from MPS, to simulate Newtonian and non-Newtonian liquids. They utilized this approach to construct a 3D swallowing simulator known as “Swallow Vision” (). With this model, it becomes possible to realistically simulate various swallowing characteristics, such as flow velocity, aspiration probability, shear rate, and residual amount, for fluids thickened with polysaccharide–dextrin. The viscosity of non-Newtonian fluids was defined using a power-law function, and input parameters like density, viscosity, surface tension, and contact angle could be readily adjusted based on fluid properties and formula calculations. Importantly, the Swallow Vision simulation of polysaccharide-thickened fluids aligns with the VFSS images (Kikuchi et al. Citation2015; Kikuchi et al. Citation2017; Michiwaki et al. Citation2020).
Hence, considering fluid flow behaviors, both in-vitro and in-silico models demonstrate significant promise in evaluating and designing thickened fluid foods tailored to the desired characteristics of individuals with dysphagia. Present models facilitate numerical analyses of the swallowing process of developed fluids, which can be cross-referenced with actual VFSS and CT images. However, it is important to acknowledge that swallowing is a multifaceted process, and current models somewhat simplify the incorporation of fluid flow attributes and organ movements. In the future, for a more accurate assessment of the swallowing performance of thickened fluid matrices, it will be crucial to integrate more comprehensive features like yield stress, extensional viscosity, and the presence of saliva into the development of various in-vitro and in-silico methods.
5. Conclusions and outlook
Polysaccharide–dextrin-thickened fluids play a vital role in meeting the nutrition needs of individuals with dysphagia. In the pursuit of tailored dysphagia diets, extensive efforts have been dedicated to comprehending the flow characteristics of these thickened fluids and subsequently devising methods to assess their swallowing behavior. Previous research has illuminated the impact of dextrin inclusion on chain entanglement and hydrogen bonding within polysaccharide-thickened fluids, resulting in alterations to key flow characteristics, including viscosity (both shear and extensional), modulus, cohesiveness, adhesiveness, yield stress, thixotropic properties, and coefficient of friction. These flow characteristics are intimately linked to the swallowing performance of thickened fluids. For instance, elevating the viscosity of a polysaccharide–dextrin-thickened fluid can decelerate its flow during swallowing, affording ample time for epiglottis closure, thereby reducing the risks of choking and aspiration. Lowering the coefficient of friction makes it easier to swallow and provide a refreshing sensation. Taking into account and incorporating flow characteristics, a range of methods has emerged for assessing the swallowing attributes of polysaccharide-thickened fluids. While in-vivo approaches (e.g., VFSS, swallowing acoustics, sEMG, CT, and animal models) have been documented, considerable emphasis has been placed on the development of in-vitro models (e.g., the Cambridge Throat and the Gothenburg Throat) and in-silico modeling techniques (e.g., HMPS, Lagrangian, and ALE). In comparison to in-vivo methods, in-vitro and in-silico modeling offer ethical advantages, enhance data reproducibility, and prove more cost-effective. These models empower us to scrutinize various swallowing characteristics (e.g., flow velocity, choking, aspiration risk, and residual amount) of thickened fluids.
Nonetheless, existing in-vitro and in-silico modeling approaches tend to oversimplify fluid dynamics, often focusing on only partial flow parameters like shear viscosity. For future model development, it becomes imperative to incorporate more comprehensive flow characteristics (e.g., extensional rheological characteristics) of polysaccharide–dextrin-thickened fluids, as these are pivotal for an accurate assessment of thickened fluid swallowing behaviors. Furthermore, our understanding of the intricate interplay among polysaccharide–dextrin interactions, flow characteristics, and the swallowing performance of related thickened fluids remains incomplete. This knowledge is of utmost importance in the evaluation and design of dysphagia-friendly foods that meet safety, nutritional and sensory requirements.
Author contributions
All the authors contributed to the writing of this review.
Nomenclature | ||
AFM | = | atomic force microscopy |
ALE | = | arbitrary Lagrangian and Eulerian |
BBE | = | ball-back extrusion |
BLD | = | branched limit dextrin |
CD | = | cyclodextrin |
CMC | = | carboxymethylcellulose |
CMCD | = | carboxymethylated curdlan |
CT | = | computed tomography |
DE | = | dextrose equivalent |
FEM | = | finite element model |
FVM | = | finite volume model |
G′ | = | storage modulus |
G″ | = | loss modulus |
GA | = | gum Arabic |
GG | = | Guar gum |
HMPS | = | Hamiltonian moving particles semi-implicit |
IDD | = | indigestible dextrin |
IDDSI | = | international Dysphagia diet standardization initiative |
JDD | = | the Japanese Diet for Dysphagia |
JSDR | = | the Japanese Society for Dysphagia Rehabilitation |
K | = | consistency coefficient |
KC | = | κ-Carrageenan |
KGM | = | Konjac glucomannan |
LAOS | = | large amplitude oscillatory shear |
LBG | = | Locust bean gum |
LES | = | lower esophageal sphincter |
LST | = | line-spread test |
LVR | = | linear viscoelastic region |
MD | = | maltodextrin |
MC | = | methylcellulose |
MPS | = | moving particles semi-implicit |
n | = | flow index |
N1 | = | first normal stress difference |
NDD | = | the National Dysphagia Diet |
PDMS | = | polydimethylsiloxane |
PT | = | Purathick |
QCM-D | = | quartz crystal microbalance with dissipation monitoring |
QT | = | QuikThik |
SAOS | = | small-amplitude oscillatory shear |
SAXS | = | small-angle X-ray scattering |
sEMG | = | surface electromyography |
SP | = | supercol |
SPH | = | smoothed particle hydrodynamics |
THU | = | Nestlé ThickenUp |
TIC | = | thick-it clear advantage |
TMFs | = | texture-modified foods |
TUC | = | Nestlé ThickenUp Clear |
UES | = | upper esophageal sphincter |
VFSS | = | Videofluoroscopy swallowing study |
XG | = | Xanthan gum |
τ0 | = | yield stress |
σ | = | stress |
tan δ | = | loss factor |
= | minimum strain elastic modulus, | |
= | maximum strain elastic modulus | |
= | instantaneous viscosity at minimum shear rate | |
= | instantaneous viscosity at maximum shear rate, |
Acknowledgments
B. Zhang and D. Qiao would like to acknowledge the National Natural Science Foundation of China (32372275 and 32172240), and the Key R & D Project of Hubei Province (2022BBA004).
Disclosure statement
No potential conflict of interest was reported by the authors.
References
- Abu-Ghanem, S., S. Chen, and M. R. Amin. 2020. Oropharyngeal dysphagia in the elderly: evaluation and prevalence. Current Otorhinolaryngology Reports 8 (1):34–42. doi: 10.1007/s40136-020-00258-x.
- Adeleye, B., and C. Rachal. 2007. Comparison of the rheological properties of ready-to-serve and powdered instant food–thickened beverages at different temperatures for dysphagic patients. Journal of the American Dietetic Association 107 (7):1176–82. doi: 10.1016/j.jada.2007.04.011.
- Aguilera, J. M., and D. J. Park. 2016. Texture-modified foods for the elderly: Status, technology and opportunities. Trends in Food Science & Technology 57:156–64. doi: 10.1016/j.tifs.2016.10.001.
- Alghooneh, A., S. M. A. Razavi, and S. Kasapis. 2020. A new approach to distinguish thixotropic and viscoelastic phenomena. Food Biophysics 15 (1):72–84. doi: 10.1007/s11483-019-09607-w.
- Anvari, M., M. Tabarsa, and H. S. Joyner. 2018. Large amplitude oscillatory shear behavior and tribological properties of gum extracted from Alyssum homolocarpum seed. Food Hydrocolloids. 77:669–76. doi: 10.1016/j.foodhyd.2017.11.008.
- Araie, T., H. Ono Minagi, Y. Usami, K. Ikai, M. Sakai, N. Gojo, K. Nohara, and T. Sakai. 2020. Effect of xanthan gum-thickened liquid aspiration on the lungs in a mouse model. Oral Science International 17 (2):78–85. doi: 10.1002/osi2.1047.
- Araiza-Calahorra, A., A. R. Mackie, G. Feron, and A. Sarkar. 2023. Can tribology be a tool to help tailor food for elderly population? Current Opinion in Food Science 49:100968. doi: 10.1016/j.cofs.2022.100968.
- Au-Lever, T. E., S. M. Au- Braun, R. T. Au- Brooks, R. A. Au- Harris, L. L. Au- Littrell, R. M. Au- Neff, C. J. Au- Hinkel, M. J. Au Allen, and M. A. Au- Ulsas. 2015. Adapting human videofluoroscopic swallow study methods to detect and characterize dysphagia in murine disease models. Journal of Visualized Experiments: JoVE (97):e52319. doi: 10.3791/52319.
- Baixauli, R., A. Dobiašová, A. Tarrega, and L. Laguna. 2023. Pairing physical and sensory properties of dysphagia thickeners to understand disliking. Food Hydrocolloids for Health 3:100140. doi: 10.1016/j.fhfh.2023.100140.
- Barbon, C. E. A., and C. M. Steele. 2019. Characterizing the flow of thickened barium and non-barium liquid recipes using the IDDSI flow test. Dysphagia 34 (1):73–9. doi: 10.1007/s00455-018-9915-6.
- Beheshti, N., K. Zhu, A.-L. Kjøniksen, and B. Nyström. 2007. Effects of β-cyclodextrin and β-cyclodextrin polymer addition and temperature on the modulation of hydrophobic interactions in aqueous solutions of two hydrophobically modified biopolymers. Journal of Non-Crystalline Solids 353 (41-43):3906–14. doi: 10.1016/j.jnoncrysol.2007.03.028.
- Bolivar-Prados, M., L. Rofes, V. Arreola, S. Guida, W. V. Nascimento, A. Martin, N. Vilardell, O. Ortega Fernández, D. Ripken, M. Lansink, et al. 2019. Effect of a gum-based thickener on the safety of swallowing in patients with poststroke oropharyngeal dysphagia. Neurogastroenterology and Motility 31 (11):e13695. doi: 10.1111/nmo.13695.
- Boyd, M. J., F. C. Hampson, I. G. Jolliffe, P. W. Dettmar, J. R. Mitchell, and C. D. Melia. 2009. Strand-like phase separation in mixtures of xanthan gum with anionic polyelectrolytes. Food Hydrocolloids 23 (8):2458–67. doi: 10.1016/j.foodhyd.2009.07.008.
- Bui, C. M., and T. X. Ho. 2020. Influence of thixotropic parameters on a non-Newtonian fluid flow. AIP Advances 10 (3):035303. doi: 10.1063/1.5143620.
- Carmona, J. A., P. Ramírez, N. Calero, and J. Muñoz. 2014. Large amplitude oscillatory shear of xanthan gum solutions. Effect of sodium chloride (NaCl) concentration. Journal of Food Engineering 126:165–72. doi: 10.1016/j.jfoodeng.2013.11.009.
- Cho, H.-M., W. Yoo, and B. Yoo. 2015. Effect of NaCl addition on rheological behaviors of commercial gum-based food thickener used for dysphagia diets. Preventive Nutrition and Food Science 20 (2):137–42. doi: 10.3746/pnf.2015.20.2.137.
- Cichero, J. A. Y., P. Lam, C. M. Steele, B. Hanson, J. Chen, R. O. Dantas, J. Duivestein, J. Kayashita, C. Lecko, J. Murray, et al. 2017. Development of international terminology and definitions for texture-modified foods and thickened fluids used in dysphagia management: The IDDSI framework. Dysphagia 32 (2):293–314. doi: 10.1007/s00455-016-9758-y.
- Cichero, J. A. Y., C. Steele, J. Duivestein, P. Clavé, J. Chen, J. Kayashita, R. Dantas, C. Lecko, R. Speyer, P. Lam, et al. 2013. The need for international terminology and definitions for texture-modified foods and thickened liquids used in Dysphagia management: Foundations of a global initiative. Current Physical Medicine and Rehabilitation Reports 1 (4):280–91. doi: 10.1007/s40141-013-0024-z.
- Clavé, P., and R. Shaker. 2015. Dysphagia: Current reality and scope of the problem. Nature Reviews. Gastroenterology & Hepatology 12 (5):259–70. doi: 10.1038/nrgastro.2015.49.
- Cola, P. C., A. R. Gatto, R. G. da Silva, A. A. Spadotto, P. W. Ribeiro, A. O. Schelp, L. R. Carvalho, and M. A. C. A. Henry. 2012. Taste and temperature in swallowing transit time after Stroke. Cerebrovascular Diseases Extra 2 (1):45–51. doi: 10.1159/000339888.
- Cullen, K. P., L. M. Grant, C. A. Kelm-Nelson, A. F. L. Brauer, L. B. Bickelhaupt, J. A. Russell, and M. R. Ciucci. 2018. Pink1 −/− rats show early-onset swallowing deficits and correlative brainstem pathology. Dysphagia 33 (6):749–58. doi: 10.1007/s00455-018-9896-5.
- Cullins, M. J., and N. P. Connor. 2019. Reduced tongue force and functional swallowing changes in a rat model of post stroke dysphagia. Brain Research 1717:160–6. doi: 10.1016/j.brainres.2019.04.023.
- DeLozier, K. R., F. D. H. Gould, J. Ohlemacher, A. J. Thexton, and R. Z. German. 2018. Impact of recurrent laryngeal nerve lesion on oropharyngeal muscle activity and sensorimotor integration in an infant pig model. Journal of Applied Physiology (Bethesda, Md.: 1985) 125 (1):159–66. doi: 10.1152/japplphysiol.00963.2017.
- Dewar, R. J., and M. J. Joyce. 2006. The thixotropic and rheopectic behaviour of maize starch and maltodextrin thickeners used in dysphagia therapy. Carbohydrate Polymers 65 (3):296–305. doi: 10.1016/j.carbpol.2006.01.018.
- Dinkgreve, M., J. Paredes, M. M. Denn, and D. Bonn. 2016. On different ways of measuring “the” yield stress. Journal of Non-Newtonian Fluid Mechanics 238:233–41. doi: 10.1016/j.jnnfm.2016.11.001.
- Ebihara, S., H. Sekiya, M. Miyagi, T. Ebihara, and T. Okazaki. 2016. Dysphagia, dystussia, and aspiration pneumonia in elderly people. Journal of Thoracic Disease 8 (3):632–9. doi: 10.21037/jtd.2016.02.60.
- Enz, V. C. Q., A. R. C. Vaz, M. C. d A. Nunes, M. d O. Rosa, J. d A. Nunes, J. M. Marques, and R. S. Santos. 2021. Accuracy of acoustic evaluation of swallowing as a diagnostic method of dysphagia in individuals affected by stroke: Preliminary analysis. Dysphagia 37 (4):724–35. doi: 10.1007/s00455-021-10358-5.
- Evageliou, V. 2020. Shear and extensional rheology of selected polysaccharides. International Journal of Food Science & Technology 55 (5):1853–61. doi: 10.1111/ijfs.14545.
- Ewoldt, R. H., A. E. Hosoi, and G. H. McKinley. 2008. New measures for characterizing nonlinear viscoelasticity in large amplitude oscillatory shear. Journal of Rheology 52 (6):1427–58. doi: 10.1122/1.2970095.
- Farhangi, M. A., A. Z. Javid, B. Sarmadi, P. Karimi, and P. Dehghan. 2018. A randomized controlled trial on the efficacy of resistant dextrin, as functional food, in women with type 2 diabetes: Targeting the hypothalamic–pituitary–adrenal axis and immune system. Clinical Nutrition (Edinburgh, Scotland) 37 (4):1216–23. doi: 10.1016/j.clnu.2017.06.005.
- Forster, A., N. Samaras, G. Gold, and D. Samaras. 2011. Oropharyngeal dysphagia in older adults: A review. European Geriatric Medicine 2 (6):356–62. doi: 10.1016/j.eurger.2011.08.007.
- Frigaard, I. 2019. Simple yield stress fluids. Current Opinion in Colloid & Interface Science 43:80–93. doi: 10.1016/j.cocis.2019.03.002.
- Fujiso, Y., N. Perrin, J. van der Giessen, N. E. Vrana, F. Neveu, and V. Woisard. 2018. Swall-E: A robotic in-vitro simulation of human swallowing. PloS One 13 (12):e0208193-e0208193. doi: 10.1371/journal.pone.0208193.
- Funami, T., and M. Nakauma. 2021. Instrumental characteristics from extensional rheology and tribology of polysaccharide solutions. Journal of Texture Studies 52 (5-6):567–77. doi: 10.1111/jtxs.12638.
- Gallegos, C., M. Turcanu, G. Assegehegn, and E. Brito-de la Fuente. 2021. Rheological Issues on Oropharyngeal Dysphagia. Dysphagia 38 (2):558–85. doi: 10.1007/s00455-021-10337-w.
- Gamonpilas, C., A. Kongjaroen, and P. Methacanon. 2023. The importance of shear and extensional rheology and tribology as the design tools for developing food thickeners for dysphagia management. Food Hydrocolloids. 140:108603. doi: 10.1016/j.foodhyd.2023.108603.
- Gentilcore, D., L. Vanis, J. C. Teng, J. M. Wishart, J. D. Buckley, C. K. Rayner, M. Horowitz, and K. L. Jones. 2011. The oligosaccharide α-cyclodextrin has modest effects to slow gastric emptying and modify the glycaemic response to sucrose in healthy older adults. The British Journal of Nutrition 106 (4):583–7. doi: 10.1017/S0007114511000444.
- German, R. Z., A. W. Crompton, F. D. H. Gould, and A. J. Thexton. 2017. Animal models for dysphagia studies: what have we learnt so far. Dysphagia 32 (1):73–7. doi: 10.1007/s00455-016-9778-7.
- Glass, T. J., S. L. Twadell, L. C. Valmadrid, and N. P. Connor. 2019. Early impacts of modified food consistency on oromotor outcomes in mouse models of Down syndrome. Physiology & Behavior 199:273–81. doi: 10.1016/j.physbeh.2018.11.031.
- Glass, T. J., L. C. V. Valmadrid, and N. P. Connor. 2019. The adult Ts65Dn mouse model of down syndrome shows altered swallow function. Frontiers in Neuroscience 13:906. doi: 10.3389/fnins.2019.00906.
- Gould, F. D. H., A. Gross, R. Z. German, and J. R. Richardson. 2018. Evidence of oropharyngeal dysfunction in feeding in the rat rotenone model of Parkinson’s disease. Parkinson’s Disease 2018:6537072. doi: 10.1155/2018/6537072.
- Habibi, H., and K. Khosravi-Darani. 2017. Effective variables on production and structure of xanthan gum and its food applications: A review. Biocatalysis and Agricultural Biotechnology 10:130–40. doi: 10.1016/j.bcab.2017.02.013.
- Hadde, E. K., J. Ann Yvette Cichero, and T. Michael Nicholson. 2016. Viscosity of thickened fluids that relate to the Australian National Standards. International Journal of Speech-Language Pathology 18 (4):402–10. doi: 10.3109/17549507.2015.1081289.
- Hadde, E. K., and J. Chen. 2019. Shear and extensional rheological characterization of thickened fluid for dysphagia management. Journal of Food Engineering 245:18–23. doi: 10.1016/j.jfoodeng.2018.10.007.
- Hadde, E. K., and J. Chen. 2021. Texture and texture assessment of thickened fluids and texture-modified food for dysphagia management. Journal of Texture Studies 52 (1):4–15. doi: 10.1111/jtxs.12567.
- Hadde, E. K., W. Chen, and J. Chen. 2020. Cohesiveness visual evaluation of thickened fluids. Food Hydrocolloids. 101:105522. doi: 10.1016/j.foodhyd.2019.105522.
- Hadde, E. K., T. M. Nicholson, and J. A. Y. Cichero. 2015. Rheological characterisation of thickened fluids under different temperature, pH and fat contents. Nutrition & Food Science 45 (2):270–85. doi: 10.1108/NFS-06-2014-0053.
- Hadde, E. K., T. M. Nicholson, and J. A. Y. Cichero. 2020. Evaluation of thickened fluids used in dysphagia management using extensional rheology. Dysphagia 35 (2):242–52. doi: 10.1007/s00455-019-10012-1.
- Hadde, E. K., S. Prakash, W. Chen, and J. Chen. 2022. Instrumental texture assessment of IDDSI texture levels for dysphagia management. Part 1: Thickened fluids. Journal of Texture Studies 53 (5):609–16. doi: 10.1111/jtxs.12707.
- Hanson, B., B. Cox, E. Kaliviotis, and C. H. Smith. 2012. Effects of saliva on starch-thickened drinks with acidic and neutral pH. Dysphagia 27 (3):427–35. doi: 10.1007/s00455-011-9386-5.
- Hanson, B., M. T. O’Leary, and C. H. Smith. 2012. The Effect of saliva on the viscosity of thickened drinks. Dysphagia 27 (1):10–9. doi: 10.1007/s00455-011-9330-8.
- Harris, R. A., M. E. Grobman, M. J. Allen, J. Schachtel, N. E. Rawson, B. Bennett, J. Ledyayev, B. Hopewell, J. R. Coates, C. R. Reinero, et al. 2017. Standardization of a videofluoroscopic swallow study protocol to investigate dysphagia in dogs. Journal of Veterinary Internal Medicine 31 (2):383–93. doi: 10.1111/jvim.14676.
- Hayoun, P., J. Engmann, S. Mowlavi, B. Le Reverend, A. Burbidge, and M. Ramaioli. 2015. A model experiment to understand the oral phase of swallowing of Newtonian liquids. Journal of Biomechanics 48 (14):3922–8. doi: 10.1016/j.jbiomech.2015.09.022.
- Hennessy, M., and D. Goldenberg. 2016. Surgical anatomy and physiology of swallowing. Operative Techniques in Otolaryngology-Head and Neck Surgery 27 (2):60–6. doi: 10.1016/j.otot.2016.04.002.
- Hirt, C. W., A. A. Amsden, and J. L. Cook. 1974. An arbitrary Lagrangian-Eulerian computing method for all flow speeds. Journal of Computational Physics 14 (3):227–53. doi: 10.1016/0021-9991(74)90051-5.
- Ho, A., R. Affoo, N. Rogus-Pulia, M. Nicosia, Y. Inamoto, E. Saitoh, S. Green, and S. Fels. 2017. Inferring the effects of saliva on liquid bolus flow using computer simulation. Computers in Biology and Medicine 89:304–13. doi: 10.1016/j.compbiomed.2017.07.014.
- Hong, S.-R., D.-S. Sun, W. Yoo, and B. Yoo. 2012. Flow behaviors of commercial food thickeners used for the management of dysphagia: Effect of temperature. International Journal of Food Engineering 8 (2). doi: 10.1515/1556-3758.2215.
- Huang, X., Y. Kakuda, and W. Cui. 2001. Hydrocolloids in emulsions: Particle size distribution and interfacial activity. Food Hydrocolloids. 15 (4-6):533–42. doi: 10.1016/S0268-005X(01)00091-1.
- Inamoto, Y., M. González-Fernández, and E. Saitoh. 2022. 3D-CT evaluation of swallowing: metrics of the swallowing response using swallowing CT. Dysphagia 37 (2):237–49. doi: 10.1007/s00455-021-10288-2.
- Inamoto, Y., E. Saitoh, S. Okada, H. Kagaya, S. Shibata, K. Ota, M. Baba, N. Fujii, K. Katada, P. Wattanapan, et al. 2013. The effect of bolus viscosity on laryngeal closure in swallowing: kinematic analysis using 320-row area detector CT. Dysphagia 28 (1):33–42. doi: 10.1007/s00455-012-9410-4.
- Inamoto, Y., R. Ueha, and M. Gonzalez-Fernandez. 2023. Emerging dysphagia technologies: swallowing CT. Current Otorhinolaryngology Reports 11 (2):154–60. doi: 10.1007/s40136-023-00453-6.
- Islam, A. M., G. O. Phillips, A. Sljivo, M. J. Snowden, and P. A. Williams. 1997. A review of recent developments on the regulatory, structural and functional aspects of gum Arabic. Food Hydrocolloids. 11 (4):493–505. doi: 10.1016/S0268-005X(97)80048-3.
- Jean, A. 2001. Brain stem control of swallowing: neuronal network and cellular mechanisms. Physiological Reviews 81 (2):929–69. doi: 10.1152/physrev.2001.81.2.929.
- Jeong, G. Y., J. H. Bak, and B. Yoo. 2019. Physical and rheological properties of xanthan gum agglomerated in fluidized bed: Effect of HPMC as a binder. International Journal of Biological Macromolecules 121:424–8. doi: 10.1016/j.ijbiomac.2018.10.048.
- Ji, L., H. Zhang, L. Cornacchia, G. Sala, and E. Scholten. 2022. Effect of gelatinization and swelling degree on the lubrication behavior of starch suspensions. Carbohydrate Polymers 291:119523. doi: 10.1016/j.carbpol.2022.119523.
- Jo, W., J. H. Bak, and B. Yoo. 2018. Rheological characterizations of concentrated binary gum mixtures with xanthan gum and galactomannans. International Journal of Biological Macromolecules 114:263–9. doi: 10.1016/j.ijbiomac.2018.03.105.
- Joyner, H. S. 2021. Nonlinear (large-amplitude oscillatory shear) rheological properties and their impact on food processing and quality. Annual Review of Food Science and Technology 12 (1):591–609. doi: 10.1146/annurev-food-061220-100714.
- Kamiya, T., Y. Toyama, Y. Michiwaki, and T. Kikuchi. 2013. Development of a numerical simulator of human swallowing using a particle method (Part 1. Preliminary evaluation of the possibility of numerical simulation using the MPS method). Paper Presented at the 2013 35th Annual International Conference of the IEEE Engineering in Medicine and Biology Society (EMBC), 3–7 July 2013.
- Karataş, M., and E. Aydoğmuş. 2023. Effects of dispersion medium on surface tension and rheological behavior of pectin and guar gum based thickeners used in treatment of dysphagia. International Journal of Advanced Natural Sciences and Engineering Researches 7 (4):452–7. doi: 10.59287/ijanser.790.
- Kikuchi, T., Y. Michiwaki, T. Kamiya, Y. Toyama, T. Tamai, and S. Koshizuka. 2015. Human swallowing simulation based on videofluorography images using Hamiltonian MPS method. Computational Particle Mechanics 2 (3):247–60. doi: 10.1007/s40571-015-0049-4.
- Kikuchi, T., Y. Michiwaki, S. Koshizuka, T. Kamiya, and Y. Toyama. 2017. Numerical simulation of interaction between organs and food bolus during swallowing and aspiration. Computers in Biology and Medicine 80:114–23. doi: 10.1016/j.compbiomed.2016.11.017.
- Kim, Y.-H., S.-G. Kim, and B. Yoo. 2017. Effect of maltodextrin on physical properties of granulated xanthan gum prepared by fluidized-bed granulator. International Journal of Food Engineering 13 (8). doi: 10.1515/ijfe-2017-0069.
- Kim, S.-G., W. Yoo, and B. Yoo. 2014. Effect of thickener type on the rheological properties of hot thickened soups suitable for elderly people with swallowing difficulty. Preventive Nutrition and Food Science 19 (4):358–62. doi: 10.3746/pnf.2014.19.4.358.
- Kletzien, H., M. J. Cullins, and N. P. Connor. 2019. Age-related alterations in swallowing biomechanics. Experimental Gerontology 118:45–50. doi: 10.1016/j.exger.2019.01.006.
- Kongjaroen, A., P. Methacanon, and C. Gamonpilas. 2022. On the assessment of shear and extensional rheology of thickened liquids from commercial gum-based thickeners used in dysphagia management. Journal of Food Engineering 316:110820. doi: 10.1016/j.jfoodeng.2021.110820.
- Kongjaroen, A., P. Methacanon, N. Seetapan, A. Fuongfuchat, C. Gamonpilas, and K. Nishinari. 2022. Effects of dispersing media on the shear and extensional rheology of xanthan gum and guar gum-based thickeners used for dysphagia management. Food Hydrocolloids. 132:107857. doi: 10.1016/j.foodhyd.2022.107857.
- Koyama, Y., N. Ohmori, H. Momose, E. Kondo, S-i Yamada, and H. Kurita. 2021. Detection of swallowing disorders using a multiple channel surface electromyography sheet: A preliminary study. Journal of Dental Sciences 16 (1):160–7. doi: 10.1016/j.jds.2020.06.009.
- Kumar, A., K. Madhusudana Rao, and S. S. Han. 2018. Application of xanthan gum as polysaccharide in tissue engineering: A review. Carbohydrate Polymers 180:128–44. doi: 10.1016/j.carbpol.2017.10.009.
- Laguna, L., and A. Sarkar. 2017. Oral tribology: Update on the relevance to study astringency in wines. Tribology - Materials, Surfaces & Interfaces 11 (2):116–23. doi: 10.1080/17515831.2017.1347736.
- Lammers, A. R., S. Abid, P. Ding, and R. Z. German. 2020. Effects of superior laryngeal nerve lesion on kinematics of swallowing and airway protection in an infant pig model. Dysphagia 35 (6):907–17. doi: 10.1007/s00455-020-10100-7.
- Larson, R. G., and Y. Wei. 2019. A review of thixotropy and its rheological modeling. Journal of Rheology 63 (3):477–501. doi: 10.1122/1.5055031.
- Lavoisier, A., S. Shreeram, M. Jedwab, and M. Ramaioli. and Marco %J Journal of Texture Studies Ramaioli. 2021. Effect of the rheological properties of the liquid carrier on the in vitro swallowing of Solid Oral Dosage Forms. Journal of Texture Studies 52 (5-6):623–37. doi: 10.1111/jtxs.12618.
- Lee, B.-B., E.-S. Chan, P. Ravindra, and T. A. Khan. 2012. Surface tension of viscous biopolymer solutions measured using the du Nouy ring method and the drop weight methods. Polymer Bulletin 69 (4):471–89. doi: 10.1007/s00289-012-0782-2.
- Lee, H., and B. Yoo. 2021. Agglomeration of galactomannan gum powders: Physical, rheological, and structural characterizations. Carbohydrate Polymers 256:117599. doi: 10.1016/j.carbpol.2020.117599.
- Li, R., P. Wang, A. Ma, and H. Zhang. 2023. Time-dependent thixotropy of dysphagia management-oriented polysaccharide solutions. Food Hydrocolloids. 144:109018. doi: 10.1016/j.foodhyd.2023.109018.
- Lucas, P. W., J. F. Prinz, K. R. Agrawal, and I. C. Bruce. 2002. Food physics and oral physiology. Food Quality and Preference 13 (4):203–13. doi: 10.1016/S0950-3293(00)00036-7.
- Mackley, M. R., C. Tock, R. Anthony, S. A. Butler, G. Chapman, and D. C. Vadillo. 2013. The rheology and processing behavior of starch and gum-based dysphagia thickeners. Journal of Rheology 57 (6):1533–53. doi: 10.1122/1.4820494.
- Malone, M. E., I. A. M. Appelqvist, and I. T. Norton. 2003. Oral behaviour of food hydrocolloids and emulsions. Part 1. Lubrication and deposition considerations. Food Hydrocolloids. 17 (6):763–73. doi: 10.1016/S0268-005X(03)00097-3.
- Marconati, M., J. Engmann, A. S. Burbidge, V. Mathieu, I. Souchon, and M. Ramaioli. 2019. A review of the approaches to predict the ease of swallowing and post-swallow residues. Trends in Food Science & Technology 86:281–97. doi: 10.1016/j.tifs.2019.02.045.
- Marconati, M., F. Lopez, C. Tuleu, M. Orlu, and M. Ramaioli. 2019. In vitro and sensory tests to design easy-to-swallow multi-particulate formulations. European Journal of Pharmaceutical Sciences 132:157–62. doi: 10.1016/j.ejps.2019.02.026.
- Marconati, M., and M. Ramaioli. 2020. The role of extensional rheology in the oral phase of swallowing: An in vitro study. Food & Function 11 (5):4363–75. doi: 10.1039/C9FO02327E.
- Melito, H. S., C. R. Daubert, and E. A. Foegeding. 2013. Relating large amplitude oscillatory shear and food behavior: correlation of nonlinear viscoelastic, rheological, sensory and oral processing behavior of whey protein isolate/κ-carrageenan gels. Journal of Food Process Engineering 36 (4):521–34. doi: 10.1111/jfpe.12015.
- Methacanon, P., C. Gamonpilas, A. Kongjaroen, and C. Buathongjan. 2021. Food polysaccharides and roles of rheology and tribology in rational design of thickened liquids for oropharyngeal dysphagia: A review. Comprehensive Reviews in Food Science and Food Safety 20 (4):4101–19. doi: 10.1111/1541-4337.12791.
- Michiwaki, Y., T. Kamiya, T. Kikuchi, Y. Toyama, M. Takai, K. Hanyu, M. Inoue, N. Yahiro, and S. Koshizuka. 2020. Realistic computer simulation of bolus flow during swallowing. Food Hydrocolloids. 108:106040. doi: 10.1016/j.foodhyd.2020.106040.
- Mizunuma, H., M. Sonomura, and K. Shimokasa. 2020. Numerical simulation of pharyngeal bolus flow influenced by bolus viscosity and apparent slip. Journal of Texture Studies 51 (5):742–54. doi: 10.1111/jtxs.12527.
- Mizunuma, H., M. Sonomura, K. Shimokasa, H. Ogoshi, S. Nakamura, and N. Tayama. 2009. Numerical modeling and simulation on the swallowing of jelly. Journal of Texture Studies 40 (4):406–26. doi: 10.1111/j.1745-4603.2009.00189.x.
- Mosca, A. C., and J. Chen. 2017. Food-saliva interactions: Mechanisms and implications. Trends in Food Science & Technology 66:125–34. doi: 10.1016/j.tifs.2017.06.005.
- Mowlavi, S., J. Engmann, A. Burbidge, R. Lloyd, P. Hayoun, B. Le Reverend, and M. Ramaioli. 2016. In vivo observations and in vitro experiments on the oral phase of swallowing of Newtonian and shear-thinning liquids. Journal of Biomechanics 49 (16):3788–95. doi: 10.1016/j.jbiomech.2016.10.011.
- Mu, R., and J. Chen. 2023. Oral bio-interfaces: Properties and functional roles of salivary multilayer in food oral processing. Trends in Food Science & Technology 132:121–31. doi: 10.1016/j.tifs.2023.01.003.
- Nakauma, M., S. Ishihara, T. Funami, and K. Nishinari. 2011. Swallowing profiles of food polysaccharide solutions with different flow behaviors. Food Hydrocolloids. 25 (5):1165–73. doi: 10.1016/j.foodhyd.2010.11.003.
- Nativ-Zeltzer, N., M. A. Kuhn, D. M. Imai, R. P. Traslavina, A. S. Domer, J. K. Litts, B. Adams, and P. C. Belafsky. 2018. The effects of aspirated thickened water on survival and pulmonary injury in a rabbit model. The Laryngoscope 128 (2):327–31. doi: 10.1002/lary.26698.
- Newman, R., N. Vilardell, P. Clavé, and R. Speyer. 2016. Effect of bolus viscosity on the safety and efficacy of swallowing and the kinematics of the swallow response in patients with oropharyngeal dysphagia: White paper by the European Society for Swallowing Disorders (ESSD). Dysphagia 31 (2):232–49. doi: 10.1007/s00455-016-9696-8.
- Ng, G. C. F., E. M. Gray-Stuart, M. P. Morgenstern, J. R. Jones, N. P. Grigg, and J. E. Bronlund. 2017. The slip extrusion test: A novel method to characterise bolus properties. Journal of Texture Studies 48 (4):294–301. doi: 10.1111/jtxs.12254.
- Nicosia, M. A. 2007. A planar finite element model of bolus containment in the oral cavity. Computers in Biology and Medicine 37 (10):1472–8. doi: 10.1016/j.compbiomed.2007.01.007.
- Nicosia, M. A. 2013. Theoretical estimation of shear rate during the oral phase of swallowing: effect of partial slip. Journal of Texture Studies 44 (2):132–9. doi: 10.1111/jtxs.12005.
- Nicosia, M. A., and J. Robbins. 2001. The fluid mechanics of bolus ejection from the oral cavity. Journal of Biomechanics 34 (12):1537–44. doi: 10.1016/S0021-9290(01)00147-6.
- Nikaki, K., A. Sawada, A. Ustaoglu, and D. Sifrim. 2019. Neuronal control of esophageal peristalsis and its role in esophageal disease. Current Gastroenterology Reports 21 (11):59. doi: 10.1007/s11894-019-0728-z.
- Nishinari, K., M. Turcanu, M. Nakauma, and Y. Fang. 2019. Role of fluid cohesiveness in safe swallowing. Npj Science of Food 3 (1):5. doi: 10.1038/s41538-019-0038-8.
- Noh, Y., M. Segawa, K. Sato, W. Chunbao, H. Ishii, J. Solis, A. Takanishi, A. Katsumata, and Y. Iida. 2011. Development of a robot which can simulate swallowing of food boluses with various properties for the study of rehabilitation of swallowing disorders. Paper presented at the 2011 IEEE International Conference on Robotics and Automation, 9–13 May 2011.
- Osada, T., T. Kamiya, Y. Toyama, N. Jinno, T. Kikuchi, and Y. Michiwaki. 2014. Numerical analyses of food bolus velocity and force on epiglottis during swallowing using 3d swallowing simulator “swallow vision” R. Simulation 40 (60):20–40.
- Osman, K. L., S. Kohlberg, A. Mok, R. Brooks, L. A. Lind, K. McCormack, A. Ferreira, M. Kadosh, M. K. Fagan, E. Bearce, et al. 2020. Optimizing the translational value of mouse models of ALS for dysphagia therapeutic discovery. Dysphagia 35 (2):343–59. doi: 10.1007/s00455-019-10034-9.
- Pae, M., S. N. Meydani, and D. Wu. 2012. The role of nutrition in enhancing immunity in aging. Aging and Disease 3 (1):91–129. doi: PMC3320807.
- Palmer, J. B., K. V. Kuhlemeier, D. C. Tippett, and C. Lynch. 1993. A protocol for the videofluorographic swallowing study. Dysphagia 8 (3):209–14. doi: 10.1007/BF01354540.
- Park, J., and B. Yoo. 2021. Rheological properties of thickened barium liquids prepared with xanthan gum-based thickener and barium powder used in the diagnosis of dysphagia: Effect of thickener concentration and serving temperature. Journal of Food Processing and Preservation 45 (4):e15234. doi: 10.1111/jfpp.15234.
- Park, J., W. Yoo, and B. Yoo. 2019. Standard recipes for the preparation of thickened barium liquids used in the diagnosis of dysphagia. Clinical Nutrition Research 8 (4):265–71. doi: 10.7762/cnr.2019.8.4.265.
- Patel, S., W. J. McAuley, M. T. Cook, Y. Sun, S. Hamdy, and F. Liu. 2020. The swallowing characteristics of thickeners, jellies and yoghurt observed using an in vitro model. Dysphagia 35 (4):685–95. doi: 10.1007/s00455-019-10074-1.
- Pavithran, J., I. Vadakke Puthiyottil, M. Kumar, A. Viswambharan Nikitha, S. Vidyadharan, R. Bhaskaran, A. Chandrababu Jaya, K. Thankappan, I. Subramania, and K. R. Sundaram. 2020. Exploring the utility of fibreoptic endoscopic evaluation of swallowing in young children- A comparison with videofluoroscopy. International Journal of Pediatric Otorhinolaryngology 138:110339. doi: 10.1016/j.ijporl.2020.110339.
- Pourmohammadi, K., E. Abedi, S. M. B. Hashemi, and L. Torri. 2018. Effects of sucrose, isomalt and maltodextrin on microstructural, thermal, pasting and textural properties of wheat and cassava starch gel. International Journal of Biological Macromolecules 120 (Pt B):1935–43. doi: 10.1016/j.ijbiomac.2018.09.172.
- Poursani, P., S. M. A. Razavi, and A. Norouzi. 2021. Numerical investigation of a two-phase system on swallowing behavior in dysphagia: A case study on cress seed gum–xanthan gum thickened liquids. Food and Bioproducts Processing 128:186–201. doi: 10.1016/j.fbp.2021.05.004.
- Pradal, C., and J. R. Stokes. 2016. Oral tribology: Bridging the gap between physical measurements and sensory experience. Current Opinion in Food Science 9:34–41. doi: 10.1016/j.cofs.2016.04.008.
- Qazi, W. M., O. Ekberg, J. Wiklund, R. Kotze, and M. Stading. 2019. Assessment of the food-swallowing process using bolus visualisation and manometry simultaneously in a device that models human swallowing. Dysphagia 34 (6):821–33. doi: 10.1007/s00455-019-09995-8.
- Rao, G. C. S., K. Ramadevi, and K. Sirisha. 2014. Effect of β-cyclodextrin on rheological properties of some viscosity modifiers. Indian Journal of Pharmaceutical Sciences 76 (6):545–8. doi: PMC4293687.
- Redfearn, A., and B. Hanson. 2018. A mechanical simulator of tongue–palate compression to investigate the oral flow of non-newtonian fluids. IEEE/ASME Transactions on Mechatronics 23 (2):958–65. doi: 10.1109/TMECH.2018.2808704.
- Rodd, B. G., A. A. Tas, and K. D. A. Taylor. 2022. Dysphagia, texture modification, the elderly and micronutrient deficiency: A review. Critical Reviews in Food Science and Nutrition 62 (26):7354–69. doi: 10.1080/10408398.2021.1913571.
- Rodrigues, S. A., C. Pradal, L. Yu, K. J. Steadman, J. R. Stokes, and G. E. Yakubov. 2021. Creating polysaccharide-protein complexes to control aqueous lubrication. Food Hydrocolloids. 119:106826. doi: 10.1016/j.foodhyd.2021.106826.
- Rommel, N., and S. Hamdy. 2016. Oropharyngeal dysphagia: Manifestations and diagnosis. Nature Reviews. Gastroenterology & Hepatology 13 (1):49–59. doi: 10.1038/nrgastro.2015.199.
- Ross, A. I. V., P. Tyler, M. G. Borgognone, and B. M. Eriksen. 2019. Relationships between shear rheology and sensory attributes of hydrocolloid-thickened fluids designed to compensate for impairments in oral manipulation and swallowing. Journal of Food Engineering 263:123–31. doi: 10.1016/j.jfoodeng.2019.05.040.
- Russell, J. A., M. R. Ciucci, M. J. Hammer, and N. P. Connor. 2013. Videofluorographic assessment of deglutitive behaviors in a rat model of aging and Parkinson disease. Dysphagia 28 (1):95–104. doi: 10.1007/s00455-012-9417-x.
- Sarkar, A., S. Soltanahmadi, J. Chen, and J. R. Stokes. 2021. Oral tribology: Providing insight into oral processing of food colloids. Food Hydrocolloids. 117:106635. doi: 10.1016/j.foodhyd.2021.106635.
- Sasegbon, A., and S. Hamdy. 2017. The anatomy and physiology of normal and abnormal swallowing in oropharyngeal dysphagia. Neurogastroenterology and Motility 29 (11):e13100. doi: 10.1111/nmo.13100.
- Schmidt, H. d O. S., M. R. Komeroski, T. Steemburgo, and V. R. de Oliveira. 2021. Influence of thickening agents on rheological properties and sensory attributes of dysphagic diet. Journal of Texture Studies 52 (5-6):587–602. doi: 10.1111/jtxs.12596.
- Seo, C.-W., and B. Yoo. 2013. Steady and dynamic shear rheological properties of gum-based food thickeners used for diet modification of patients with dysphagia: Effect of concentration. Dysphagia 28 (2):205–11. doi: 10.1007/s00455-012-9433-x.
- Sethupathy, P., J. A. Moses, and C. Anandharamakrishnan. 2020. Food oral processing and tribology: instrumental approaches and emerging applications. Food Reviews International 37 (5):538–71. doi: 10.1080/87559129.2019.1710749.
- Sharma, M., E. Kristo, M. Corredig, and L. Duizer. 2017. Effect of hydrocolloid type on texture of pureed carrots: Rheological and sensory measures. Food Hydrocolloids. 63:478–87. doi: 10.1016/j.foodhyd.2016.09.040.
- Slavin, J. L., V. Savarino, A. Paredes-Diaz, and G. Fotopoulos. 2009. A review of the role of soluble fiber in health with specific reference to wheat dextrin. The Journal of International Medical Research 37 (1):1–17. doi: 10.1177/147323000903700101.
- Smukalla, S. M., I. Dimitrova, J. M. Feintuch, and A. Khan. 2017. Dysphagia in the elderly. Current Treatment Options in Gastroenterology 15 (3):382–96. doi: 10.1007/s11938-017-0144-0.
- Sonomura, M., H. Mizunuma, T. Numamori, H. Michiwaki, and K. Nishinari. 2011. Numerical simulation of the swallowing of liquid bolus. Journal of Texture Studies 42 (3):203–11. doi: 10.1111/j.1745-4603.2011.00287.x.
- Sopade, P. A., P. J. Halley, J. A. Y. Cichero, and L. C. Ward. 2007. Rheological characterisation of food thickeners marketed in Australia in various media for the management of dysphagia. I: Water and cordial. Journal of Food Engineering 79 (1):69–82. doi: 10.1016/j.jfoodeng.2006.01.045.
- Sopade, P. A., P. J. Halley, J. A. Y. Cichero, L. C. Ward, L. S. Hui, and K. H. Teo. 2008a. Rheological characterisation of food thickeners marketed in Australia in various media for the management of dysphagia. II. Milk as a dispersing medium. Journal of Food Engineering 84 (4):553–62. doi: 10.1016/j.jfoodeng.2007.06.024.
- Sopade, P. A., P. J. Halley, J. A. Y. Cichero, L. C. Ward, J. Liu, and S. Varliveli. 2008b. Rheological characterization of food thickeners marketed in Australia in various media for the management of dysphagia. III. Fruit juice as a dispersing medium. Journal of Food Engineering 86 (4):604–15. doi: 10.1016/j.jfoodeng.2007.11.013.
- Stading, M., M. Q. Waqas, F. Holmberg, J. Wiklund, R. Kotze, and O. Ekberg. 2019. A device that models human swallowing. Dysphagia 34 (5):615–26. doi: 10.1007/s00455-018-09969-2.
- Steele, C. M., W. A. Alsanei, S. Ayanikalath, C. E. A. Barbon, J. Chen, J. A. Y. Cichero, K. Coutts, R. O. Dantas, J. Duivestein, L. Giosa, et al. 2015. The influence of food texture and liquid consistency modification on swallowing physiology and function: A systematic review. Dysphagia 30 (1):2–26. doi: 10.1007/s00455-014-9578-x.
- Steele, C. M., and J. A. Y. Cichero. 2008. A question of rheological control. Dysphagia 23 (2):199–201. doi: 10.1007/s00455-007-9105-4.
- Steele, C. M., S. M. Molfenter, M. Péladeau-Pigeon, and S. Stokely. 2013. Challenges in preparing contrast media for videofluoroscopy. Dysphagia 28 (3):464–7. doi: 10.1007/s00455-013-9476-7.
- Steele, C. M., A. M. Namasivayam-MacDonald, B. T. Guida, J. A. Cichero, J. Duivestein, B. Hanson, P. Lam, and L. F. Riquelme. 2018. Creation and initial validation of the international dysphagia diet standardisation initiative functional diet scale. Archives of Physical Medicine and Rehabilitation 99 (5):934–44. doi: 10.1016/j.apmr.2018.01.012.
- Steele, C. M., M. Peladeau-Pigeon, C. A. E. Barbon, B. T. Guida, A. M. Namasivayam-MacDonald, W. V. Nascimento, S. Smaoui, M. S. Tapson, T. J. Valenzano, A. A. Waito, et al. 2019. Reference values for healthy swallowing across the range from thin to extremely thick liquids. Journal of Speech, Language, and Hearing Research: JSLHR 62 (5):1338–63. doi: 10.1044/2019_JSLHR-S-18-0448.
- Su, M., G. Zheng, Y. Chen, H. Xie, W. Han, Q. Yang, J. Sun, Z. Lv, and J. Chen. 2018. Clinical applications of IDDSI framework for texture recommendation for dysphagia patients. Journal of Texture Studies 49 (1):2–10. doi: 10.1111/jtxs.12306.
- Sugiyama, N., E. Nishiyama, Y. Nishikawa, T. Sasamura, S. Nakade, K. Okawa, T. Nagasawa, and A. Yuki. 2014. A novel animal model of dysphagia following stroke. Dysphagia 29 (1):61–7. doi: 10.1007/s00455-013-9481-x.
- Sukkar, S. G., N. Maggi, B. Travalca Cupillo, and C. Ruggiero. 2018. Optimizing texture modified foods for oro-pharyngeal dysphagia: a difficult but possible target? Frontiers in Nutrition 5 (68):68. doi: 10.3389/fnut.2018.00068.
- Sumida, R., S. Kishishita, A. Yasuda, M. Miyata, A. Mizote, T. Yamamoto, H. Mitsuzumi, H. Aga, K. Yamamoto, and K. Kawai. 2021. A novel dextrin produced by the enzymatic reaction of 6-α-glucosyltransferase. II. Practical advantages of the novel dextrin as a food modifier. Bioscience, Biotechnology, and Biochemistry 85 (7):1746–52. doi: 10.1093/bbb/zbab076.
- Sun-Waterhouse, D., W. Kang, C. Ma, and G. I. N. Waterhouse. 2021. Towards human well-being through proper chewing and safe swallowing: Multidisciplinary empowerment of food design. Journal of Future Foods 1 (1):1–24. doi: 10.1016/j.jfutfo.2021.09.001.
- Tha Goh, K. K., M. Sui Mei Wee, and Y. Hemar. 2013. Phase stability-induced complex rheological behaviour of galactomannan and maltodextrin mixtures. Food & Function 4 (4):627–34. doi: 10.1039/C2FO30176H.
- Turcanu, M., N. Siegert, S. Secouard, E. Brito-de la Fuente, C. Balan, and C. Gallegos. 2018. An alternative elongational method to study the effect of saliva on thickened fluids for dysphagia nutritional support. Journal of Food Engineering 228:79–83. doi: 10.1016/j.jfoodeng.2018.02.015.
- Ullrich, S., and J. Crichton. 2015. Older people with dysphagia: Transitioning to texture-modified food. British Journal of Nursing (Mark Allen Publishing) 24 (13):686, 688–92. doi: 10.12968/bjon.2015.24.13.686.
- Vaiman, M., and E. Eviatar. 2009. Surface electromyography as a screening method for evaluation of dysphagia and odynophagia. Head & Face Medicine 5 (1):9. doi: 10.1186/1746-160X-5-9.
- Vallons, K. J. R., L. A. A. C. M. Oudhuis, H. J. Helmens, and C. Kistemaker. 2015. The effect of oral processing on the viscosity of thickened drinks for patients with dysphagia. Annals of Rehabilitation Medicine 39 (5):772–7. doi: 10.5535/arm.2015.39.5.772.
- Vickers, Z., H. Damodhar, C. Grummer, H. Mendenhall, K. Banaszynski, R. Hartel, J. Hind, A. Joyce, A. Kaufman, and J. Robbins. 2015. Relationships among rheological, sensory texture, and swallowing pressure measurements of hydrocolloid-thickened fluids. Dysphagia 30 (6):702–13. doi: 10.1007/s00455-015-9647-9.
- Vieira, J. M., C. C. P. Andrade, T. P. Santos, P. K. Okuro, S. T. Garcia, M. I. Rodrigues, A. A. Vicente, and R. L. Cunha. 2021. Flaxseed gum-biopolymers interactions driving rheological behaviour of oropharyngeal dysphagia-oriented products. Food Hydrocolloids. 111:106257. doi: 10.1016/j.foodhyd.2020.106257.
- Vilardell, N., L. Rofes, V. Arreola, R. Speyer, and P. Clavé. 2016. A comparative study between modified starch and xanthan gum thickeners in post-stroke oropharyngeal dysphagia. Dysphagia 31 (2):169–79. doi: 10.1007/s00455-015-9672-8.
- Vivanti, A. P., K. L. Campbell, M. S. Suter, M. T. Hannan-Jones, and J. A. Hulcombe. 2009. Contribution of thickened drinks, food and enteral and parenteral fluids to fluid intake in hospitalised patients with dysphagia. Journal of Human Nutrition and Dietetics 22 (2):148–55. doi: 10.1111/j.1365-277X.2009.00944.x.
- Wang, X., C. Bryan, A.-S. LaMantia, and D. Mendelowitz. 2017. Altered neurobiological function of brainstem hypoglossal neurons in DiGeorge/22q11.2 Deletion Syndrome. Neuroscience 359:1–7. doi: 10.1016/j.neuroscience.2017.06.057.
- Wang, Y., and C. Selomulya. 2022. Food rheology applications of large amplitude oscillation shear (LAOS). Trends in Food Science & Technology 127:221–44. doi: 10.1016/j.tifs.2022.05.018.
- Wang, L., J. Xu, X. Fan, Q. Wang, P. Wang, J. Yuan, Y. Yu, Y. Zhang, and L. Cui. 2018. Characterization of branched limit dextrin and impact on corn starch pasting properties. Food Hydrocolloids. 79:55–62. doi: 10.1016/j.foodhyd.2017.12.005.
- Waqas, M. Q., J. Wiklund, A. Altskär, O. Ekberg, and M. Stading. 2017. Shear and extensional rheology of commercial thickeners used for dysphagia management. Journal of Texture Studies 48 (6):507–17. doi: 10.1111/jtxs.12264.
- Wei, Y., Y. Guo, R. Li, A. Ma, and H. Zhang. 2021. Rheological characterization of polysaccharide thickeners oriented for dysphagia management: Carboxymethylated curdlan, konjac glucomannan and their mixtures compared to xanthan gum. Food Hydrocolloids. 110:106198. doi: 10.1016/j.foodhyd.2020.106198.
- Welby, L., H. Caudill, G. Yitsege, A. Hamad, F. Bunyak, I. E. Zohn, T. Maynard, A.-S. LaMantia, D. Mendelowitz, and T. E. Lever. 2020. Persistent feeding and swallowing deficits in a mouse model of 22q11.2 deletion syndrome. Frontiers in Neurology 11:4. doi: 10.3389/fneur.2020.00004.
- Witczak, M., J. Korus, R. Ziobro, and L. Juszczak. 2010. The effects of maltodextrins on gluten-free dough and quality of bread. Journal of Food Engineering 96 (2):258–65. doi: 10.1016/j.jfoodeng.2009.07.022.
- Wood, F. W. 1968. Psychophysical studies on the consistency of liquid foods. Rheology and Texture of Foodstuffs 27:40–9.
- Xie, F. 2023. Chapter 4 - 3D printing of biopolymer-based hydrogels 3D printing of biopolymer-based hydrogels. In Additive Manufacturing of Biopolymers, edited by Mehrshad Mehrpouya and Henri Vahabi, 65–100. Amsterdam, Netherlands: Elsevier.
- Xie, F., P. J. Halley, and L. Avérous. 2012. Rheology to understand and optimize processibility, structures and properties of starch polymeric materials. Progress in Polymer Science 37 (4):595–623. doi: 10.1016/j.progpolymsci.2011.07.002.
- Yoon, S. N., and B. Yoo. 2016. Effect of pH on rheological properties of dysphagia-oriented thickened water. Preventive Nutrition and Food Science 21 (1):73–7. doi: 10.3746/pnf.2016.21.1.73.
- Yoon, S-n, and B. Yoo. 2017. Rheological behaviors of thickened infant formula prepared with xanthan gum-based food thickeners for dysphagic infants. Dysphagia 32 (3):454–62. doi: 10.1007/s00455-017-9786-2.
- You, K.-M., B. S. Murray, and A. Sarkar. 2021. Rheology and tribology of starch + κ-carrageenan mixtures Rheology and tribology of starch + κ-carrageenan mixtures. Journal of Texture Studies 52 (1):16–24. doi: 10.1111/jtxs.12570.
- Yuan, B., C. Ritzoulis, and J. Chen. 2018. Extensional and shear rheology of okra polysaccharides in the presence of artificial saliva. Npj Science of Food 2 (1):20. doi: 10.1038/s41538-018-0029-1.
- Yuan, C., L. Sang, Y. Wang, and B. Cui. 2018. Influence of cyclodextrins on the gel properties of kappa-carrageenan. Food Chemistry 266:545–50. doi: 10.1016/j.foodchem.2018.06.060.
- Yuan, C., W. Zhan, B. Cui, B. Yu, P. Liu, and Z. Wu. 2020. Influence of two functional dextrins on the gel properties of kappa-carrageenan. Food Research International (Ottawa, Ont.) 138 (Pt A):109666. doi: 10.1016/j.foodres.2020.109666.
- Zargaraan, A., R. Rastmanesh, G. Fadavi, F. Zayeri, and M. A. Mohammadifar. 2013. Rheological aspects of dysphagia-oriented food products: A mini review. Food Science and Human Wellness 2 (3-4):173–8. doi: 10.1016/j.fshw.2013.11.002.
- Zhu, M., B. Yu, W. Yang, Y. Jiang, L. Lu, Z. Huang, S. Chen, and G. Li. 2017. Evaluation of normal swallowing functions by using dynamic high-density surface electromyography maps. BioMedical Engineering OnLine 16 (1):133. doi: 10.1186/s12938-017-0424-x.