Abstract
The human gut microbiome has emerged as a key influencer of human health and disease, particularly through interactions with dietary fiber. However, national dietary guidelines worldwide are only beginning to capitalize on the potential of microbiome research, which has established the vital role of host-microbe interactions in mediating the physiological effects of diet on overall health and disease. β-glucans have been demonstrated to modulate the composition of the gut microbiota, leading to improved outcomes in cardiovascular disease (CVD). Raised serum cholesterol and blood pressure are important modifiable risk factors in the development of CVD and emerging evidence highlights the role of the gut microbiota in ameliorating such biomarkers and clinical characteristics of the disease. The proposed mechanism of action of β-glucans on the pathophysiological mechanisms of disease have yet to be elucidated. Validating gaps in the literature may substantiate β-glucans as a potential novel dietary therapy against modifiable risk factors for CVD and would further support the public health significance of including a habitual fiber-rich diet.
Introduction
The human gut microbiome has recently emerged as a pivotal player in modulating human health and disease, with dietary fiber at the forefront of diet-microbe-host interactions (O’Grady, O’Connor, and Shanahan Citation2019). National dietary guidelines worldwide are only just starting to harness the potential of the scientific advancements in the field of microbiome research, informed by the mechanistic evidence to date, establishing that host-microbe interactions play a crucial role in mediating the physiological effects of diet on health and disease (Armet et al. Citation2022).
The existing body of research comprises a lack of substantiation regarding the molecular diversity and characteristics of different types of fiber, as well as the significance of certain fibers as a substrate for gut microbiota metabolism. β-glucans have been shown to modulate the gut microbiota composition leading to improved cardiovascular disease (CVD) outcomes (Chen et al. Citation2002). These interactions between β-glucans and the microbiome highlight the potential role of these dietary fibers in mitigating cardiovascular risk factors such as raised serum cholesterol and blood pressure.
By presenting a comprehensive and critical assessment of the current scientific evidence, this narrative review seeks to provide a deeper understanding of the effects of cereal β-glucans on cardiovascular risk factors and the potential involvement of the gut microbiome. In our approach we explored in a systematic way existing published literature on this topic, appraised previous studies and summarized their key findings, in order to identify knowledge gaps. We explored three databases (Medline, Embase and Cochrane library) to identify English articles using human subjects only, using the terms cereals, β-glucans and cardiovascular disease risk. We included all studies published in English language, which looked into dietary interventions, as well as systematic reviews and meta-analyses of dietary interventions with no restriction on publication dates.
Cardiovascular disease and dietary fiber
Raised serum cholesterol and high blood pressure (BP) are important modifiable risk factors in the development of CVD. Current therapies for CVD include pharmacotherapy interventions targeting serum cholesterol with statins, which can induce various side effects such as myalgia affecting 1–10% of patients (Ramkumar, Raghunath, and Raghunath Citation2016). Antihypertensive drug therapies include diuretics, angiotensin-converting enzyme (ACE) inhibitors, angiotensin-2 receptor blockers (ARBs), beta‐blockers, calcium‐channel blockers, alpha‐blockers, direct vasodilators or peripheral adrenergic antagonists, among others (Musini et al. Citation2017), which can also exhibit side effects that can contribute to noncompliance and therefore uncontrolled hypertension (Morgado et al. Citation2010).
In search for effective complementary preventative and treatment measures to manage CVD risk, novel dietary approaches have been investigated, including dietary fibers (Mathews, Kamil, and Chu Citation2020). Dietary fibers comprise a complex group of carbohydrates and lignin that are not digested or absorbed in the human body as they cannot be hydrolyzed by human enzymes. They are classified according to their water solubility as either soluble or insoluble. Soluble fibers can be found in some fruits, vegetables, oats, and barley and previous research has pointed toward their cholesterol lowering effects via various mechanisms. Insoluble fibers are present in whole-grain foods, bran, nuts, and seeds and can decrease the intestinal transit time and increase fecal bulk, leading to digestive regularity (Soliman Citation2019).
The cardioprotective effects of dietary fiber through the reduction of serum lipids was initially demonstrated as part of the Seven Countries Study in the 1960s (Keys, Anderson, and Grande Citation1960). When diet types and blood lipids in men (n = 28) aged 41–63 yr were compared in controlled experiments during a 6-wk period, they found that foods high in dietary fiber resulted in lower levels of serum cholesterol. Several studies have confirmed these findings since (Threapleton et al. Citation2013). A systematic review of 23 randomized controlled trials (RCTs) (n = 1513) found that in 17 of the included trials (n = 1067) there was a beneficial effect of increased dietary fiber on levels of total cholesterol (TC) (Mean Difference (MD) −0.23 mmol/L) and low-density lipoprotein cholesterol (LDL-C) (MD: −0.14 mmol/L) but not on triglyceride (TG) levels, and there was a very small but statistically significant decrease in HDL levels with increased fiber intake (MD −0.03 mmol/L) (Hartley et al. Citation2016). Fewer studies, 10 of the 23 trials (n = 661), reported a significant effect on diastolic blood pressure (DBP) (MD: −1.77 mmHg) with increased fiber intake and a slight reduction in systolic blood pressure (SBP) (MD: −1.92 mmHg), however these findings were not statistically significant. Studies had small sample sizes and were therefore inadequately powered to detect a causal effect, the interventions were short in duration looking at a range of fiber sources, which added to the heterogeneity observed in the findings.
Although the significant cardioprotective role of overall dietary fiber is well reported, specific fiber types have differing effects and associations with CVD risk parameters. In a network meta-analysis of 55 intervention trials (n = 3900) comparing the effects and rank of various whole grains and bran on blood lipids, it was found that whole oat grain and oat bran ranked highest as the most effective intervention for reduced cholesterol, compared with barley, brown rice and wheat (Hui et al. Citation2019). Reductions in TC (−0.35 mmol/L (95% CI: −0.47, − 0.23 mmol/L) and LDL-C (−0.32 mmol/L (95% CI: −0.44, −0.19 mmol/L) were observed after oat bran consumption for a median duration of 6 wk. Reductions of −0.26 mmol/L (95% CI: −0.36, −0.15 mmol/L) in TC and −0.17 mmol/L (95% CI: −0.28, − 0.07 mmol/L) in LDL-C were demonstrated after oat consumption during the same time frame. However, it is difficult to elucidate the exact association between dietary fiber types and reductions in TC and LDL-C demonstrated in this and similar studies with regard to CVD risk reduction due to their short duration, the intake amounts not being well controlled to allow for a dose response analysis, and the data for relative risk evaluation being unavailable in the studies included.
A recent umbrella meta-analysis considered 52 meta-analyses involving 47197 participants and reported that compared to the control group, dietary fiber intake induced a positive outcome on glucose metabolism, lipid profiles (TC and LDL-C) and blood pressure, while ameliorating inflammation factors (TNF-α), indicating a strong protection effect against cardiovascular-related diseases (Fu et al. Citation2022). Interestingly again β-glucan possessed a greater efficacy in lowering serum TC and LDL-C concentration than inulin-type fructans, but this analysis overall did not provide evidence for a dose–response relationship between dietary fiber intake and improvements in cardiovascular risk factors. Even though well-controlled randomized trials with larger sample sizes are required to define any causal effect, the findings of the above studies confirm the important role of oats in cardiovascular health and have thus been extensively examined.
β-glucans: structure, sources and properties
The dietary fibers present in oats and other cereals, which are proposed to be responsible for their cardioprotective properties, are a viscous type of soluble fiber known as beta (β)-glucans. β-glucans are naturally occurring non-starch polysaccharides found primarily in sources such as oats, barley and wheat (Bashir and Choi Citation2017) and can be consumed as part of the daily diet or supplemented. They are also present in yeast and fungus cells and in some microorganisms. β-glucans are made up of D-glucose monomers linked together by β-glycosidic linkages (Barsanti et al. Citation2011). They can be insoluble or soluble fibers within soluble β-glucans being composed of β-(1,3/1,4)-D-linked glucose units, and soluble β-glucans being composed of β-(1,3/1,6)-D-linked glucose units (Golisch et al. Citation2021).
The structure of β-glucans can either be branched or unbranched and this is determined by the β-glucan source. Sources of β-glucans include both cereal and non-cereal groups; β-glucans from cereal such as oats and barley generally have 1,3 or 1,4 glycosidic linkages and no branching (Borchani et al. Citation2015) as seen in . The proportion of β-glucan varies between cereal sources, as there is more β-glucan content in barley (7%) than in oats (5%) (Rondanelli, Opizzi, and Monteferrario Citation2009).
Figure 1. (a) β-Glucan structure from cereals sources (oats, barley), linear β-1,3 or β-1,4 backbone with no branching, (b) β-glucan structure from fungus or yeast; β-1,3 backbone with β-1,6 branches (Kaur et al. Citation2019).
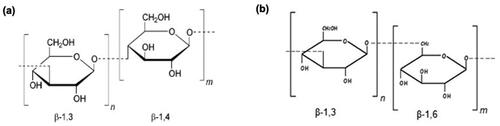
Non-cereal sources of β-glucans include yeast, fungi, bacteria, and algae and have varying degrees of 1,6 glycosidic linkages with branching (Mejía, De Francisco, and Bohrer Citation2020) as shown in . The structure and properties determine the effect and mode of action of β-glucans and variances can occur between β-glucan sources such as molecular weight (Mw), glycosidic linkages, presence of branching, degree of polymerization and solubility (Kaur et al. Citation2019). This level of variance underlies the wide scope of heterogeneous applications of β-glucans continually investigated in clinical trials. It seems that the high Mw and high viscosity of β-glucans are proposed to be responsible for their hypocholesterolaemic and hypoglycemic properties (Du et al. Citation2019). Indeed, β-glucans have been found to play a significant role in a diverse range of health and pathological conditions such as in insulin resistance, dyslipidaemia, hypertension, immune modulation, metabolic syndrome, and obesity (Yang, Zhou, and Zhang Citation2019).
The potential of β-glucans to decrease blood cholesterol levels was known since the 1960s, where a study in 21 participants substituted white bread for bread with rolled oats for 3 wk and found a lowering effect (11%) on blood cholesterol levels (de Groot, Luyken, and Pikaar Citation1963). Several studies have confirmed these findings since. A systematic review and meta-analysis of RCTs assessed 14 studies (n = 615) with a duration of more than 3 wk examining the effects of barley β-glucan (6.5–6.9 g/d) in the diet on CVD risk factors (Ho et al. Citation2016). Results showed significant LDL-C lowering effects (MD: −0.25 mmol/L, 95% CI: [−0.30, −0.20]) as well as reduced non-high-density lipoprotein cholesterol (HDL-C) (MD: −0.31 mmol/L, 95% CI: −0.39, −0.23), with no significant changes to Apolipoprotein B (ApoB) levels, when compared with control diets.
In light of these beneficial effects on cardiovascular health, the European Food Safety Authority (EFSA, Citation2010) approved a health claim underpinning the role of oat β-glucans in blood cholesterol reduction following the consumption of 3 g/day. This supports the consideration of β-glucans as a promising potential dietary treatment for people with increased risk for CVD, as they are naturally occurring and do not cause any significant adverse effects, such as liver or renal disorders. Continually emerging evidence highlights their potential cardioprotective mechanisms (Sima, Vannucci, and Vetvicka Citation2018). Additionally, it has more recently emerged that β-glucans exhibit prebiotic effects that modulate the gut microbiome leading to beneficial cardiovascular health effects (Wouk et al. Citation2021).
Several mechanisms have been described to explain the hypocholesterolaemic effect of β-glucans, however these are not yet thoroughly understood (Tosh and Bordenave Citation2020). An older study with an interesting study design investigated excretion patterns in ileostomy patients (n = 9) given oat-bran bread with and without a β-glucan-degrading enzyme (β-Glucanase) found that among participants consuming intact oat-derived β-glucans (12.5 g/d), a 53% higher excretion rate of bile acids over 24 hrs occurred when compared to the β-Glucanase group (p < 0.05) (Lia et al. Citation1995). This suggested a potential mechanism of action for the serum lipid-reducing properties of β-glucans and led to further investigations. We now know that among the potential mechanisms of action are the increased viscosity at the small intestinal level and subsequently slowed gastric emptying rate and the absorption of sugar, as well as the binding and reduction of bile acids in the intestine which leads to increased use of cholesterol for bile acid synthesis, improved insulin sensitivity, and inhibited cholesterol synthesis by short chain fatty acids (SCFAs), acetate and propionate, which are produced by fermentation of β-glucans in the colon (De Angelis et al. Citation2015). Wang et al. (Citation2017) suggested that β-glucans possibly interrupt the enterohepatic circulation of bile acids rather than inhibiting cholesterol absorption and synthesis but their study design did not allow to confirm this as the main mechanism responsible for their cholesterol-lowering effect.
Despite the growing body of evidence supporting the cardioprotective effects of β-glucans, the effects of β-glucans on lipid regulation in patients with hypercholesterolemia have been inconsistent, leading to heterogeneity in the findings. Additionally, significant challenges exist regarding long term clinical studies and extrapolation of studies validating β-glucans as a successful management intervention. The insufficient understanding of the various mechanisms of action of β-glucans remains a key knowledge gap along with the poorly understood significance of different sources and effective dose concentrations of β-glucans (Murphy et al. Citation2020).
Mechanisms of action – the effect of β-glucans on CVD risk factors
The mode of action of β-glucans on CVD risk factors such as cholesterol and BP is not yet fully understood, however it is strongly linked with the process of digestion and the interactions in the gut microbiome, as depicted in . The hypocholesterolaemic and anti-hypertensive effects associated with consumption of β-glucans have been extensively examined in clinical intervention trials and a few animal-based in-vivo studies, though infrequently reported through in-vitro studies (Hartley et al. Citation2016) ().
Figure 2. The proposed mechanisms of action of β-glucans on CVD risk parameters; elevated BP and cholesterol with the interaction of the microbiome and potentially other metabolic factors (adapted and modified by Schmidt Citation2022).
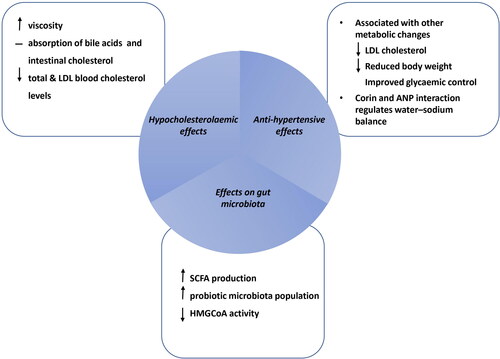
Table 1. A summary and timeline of key studies to date investigating serum lipids and cholesterol metabolism outcomes as reported in: (a) systematic reviews and meta-analyses, (b) human studies and (c) animal studies.
Table 2. A Summary and timeline of key studies to date investigating the effects of cereal β-glucans on blood pressure as reported in: (a) systematic reviews and meta-analyses, (b) human studies and (c) animal studies.
Effect of β -glucans on serum cholesterol
The anti-hypercholesterolaemic effects of β-glucans are proposed to be attributable to their gel-forming properties, through regulating bile acid and cholesterol metabolism, and potentially removing intestinal cholesterol for excretion (Joyce et al. Citation2019). B-glucans increase viscosity, interact and bind with components of the small intestine resulting in the absorption of cholesterol and bile acids (Silva et al. Citation2021). The production of bile acids is predominantly regulated by the cytochrome P450 enzyme 7-α hydroxylase (CYP7A1) which controls cholesterol synthesis and excretion from the liver (Chiang and Ferrell Citation2020).
It has been well established that blood cholesterol levels have an inverse relationship with dietary consumption of β‑glucans in individuals with hyperlipidemia (). A large meta-analysis of 126 studies that investigated the effect of β-glucan intake of 3 g/d on measures of blood cholesterol found a significant reduction of TC (−0.85 to −0.34 mmol/L), LDL-C (−0.96 to −0.36 mmol/L), and TG (−0.15 to 0.07 mmol/L) following consumption of oat and barley β-glucans (Tiwari and Cummins Citation2011). Following this, a meta-analysis of 17 RCTs (n = 916) examined the effects of β-glucan consumption in participants with hypercholesterolemia and found that β-glucan consumption significantly lowered TC (MD: −0.26 mmol/L; 95% CI, −0.33 to −0.18; p < 0.00001) and LDL-C (MD: −0.21 mmol/L; 95% CI, −0.27 to −0.14; p < 0.00001) in participants with elevated serum cholesterol (Zhu et al. Citation2015).
This supports previous findings of a dose-response relationship between oat derived β-glucans and reduced LDL-C in participants (n = 156) with elevated cholesterol, first reported by Davidson et al. (Davidson et al. Citation1991) who discovered significantly reduced TC and LDL-C concentrations in adults (30–65 yr) with LDL-C levels >3.37 mmol/L consuming oat derived β-glucans for 6 wk. A 15.9% reduction in LDL-C was seen in those consuming 56 g/d oat bran. This dose-response was later confirmed in a meta-analysis of 20 RCTs that reported reduced TC levels of −0.13 mmol/L at the 95% CI with β-glucan concentrations of 3 g/d (Ripsin et al. Citation1992). These findings led to the Food and Drug Administration (U.S.) registering oat bran as the first dietary therapy for cholesterol reduction at concentrations of 3 g β-glucans per day (FDA, Citation1996).
A more recent systematic literature review conducted by Thies and colleagues (Thies et al. Citation2014) supported the findings of observational studies consistent with the older meta-analysis by Ripsin and colleagues (Ripsin et al. Citation1992). They also aimed to investigate further the effects in longer term intervention studies, comparing results between trial durations of 2 wk − 6 months. A total of 64 studies were examined to find a significantly reduced TC and LDL-C mostly in participants with elevated cholesterol (Thies et al. Citation2014). However, 59% of studies had <30 participants and lacked statistical power to detect a significant effect of oat β-glucans on CVD risk factors. Out of 64 studies, 37 (58%) and 34 (49%) demonstrated a significant reduction in TC (2–19% reduction) and LDL-C (4–23% reduction) respectively. Fewer studies reported significant effects on HDL-C and triglyceride (TG) concentrations. Long-term dietary intake of oat derived β-glucans exhibited a beneficial effect on blood cholesterol. However, the heterogeneity among studies regarding the amount and type of oats consumed, as well as the duration of trials and initial blood cholesterol concentration of participants resulted in significant variation and confounding variables. Further assessment of larger, appropriately controlled interventions is required, in order to address this and determine a significant effect.
The various effects of different sources and concentrations of β-glucans have also been investigated. Behall and colleagues (Behall, Scholfield, and Hallfrisch Citation2004) investigated whether the consumption of barley β-glucans would reduce cardiovascular disease risk factors comparably with that of other sources of soluble fiber on participants with mild hypercholesterolemia (9 postmenopausal women, 9 premenopausal women, and 7 men). The participants consumed controlled American Heart Association Step 1 diets for 17 wk with whole-grain foods containing 0, 3, or 6 g β-glucan/d from barley and the researchers found a significantly improved lipid profile depicted by a reduction of LDL-C by 13.8% and 17.4% with higher intakes of barley β-glucans consumed (3 g and 6 g, respectively) (Behall, Scholfield, and Hallfrisch Citation2004). These results indicated that dietary changes involving greater consumption of whole grains including barley derived β-glucans can reduce risk factors associated with CVD. Further meta‑analyses verified the positive effect of increased intake of barley β‑glucans on serum cholesterol levels. A meta-analysis investigating the effects of barley derived soluble fiber on serum lipids in adults both healthy and with hypercholesterolemia (n = 391), found that barley intake significantly lowered TC (MD: −13.38 mg/dL; 95% CI, −18.46 to −8.31 mg/dL), LDL-C, (MD: −10.02 mg/dL; 95% CI, −14.03 to −6.00 mg/dL) and TG (MD: −11.83 mg/dL; 95% CI, −20.12 to −3.55 mg/dL) but did not significantly alter HDL-C (p = 0.07) (38). Additionally, a meta-analysis of 11 RCTs examining the lipid-lowering capacity of barley derived β-glucans demonstrated reduced TC and LDL-C concentrations by 0.30 mmol/L (95% CI: −0.39 to −0.21, p < 0.00001) and 0.27 mmol/L (95% CI: −0.34 to −0.20, p < 0.00001), respectively, compared with control group (AbuMweis, Jew, and Ames Citation2020).
Furthermore, it has been reported that there is a greater β-glucan content in barley than in oats, however barley has an inferior taste and is consumed less often (Poppitt Citation2007), and oats are an easier dietary component to target. An RCT of 75 men and women with raised cholesterol supplemented with 6 g/d dietary oat β-glucans or control for 6 wk observed significant reductions in TC (−0.3 ± 0.1 mmol/L) and LDL-C (−0.3 ± 0.1 mmol/L). Fermentability of concentrated oat β-glucan with inulin and guar gum was compared through a model of intestinal fermentation system and showed that oat β-glucan was fermentable, producing highest amounts of butyrate (Queenan et al. Citation2007). Following this, different β-glucan concentrations were examined and have shown varying degrees of effectiveness on cholesterol reduction. A meta-analysis of 28 RCTs was conducted comparing oat β-glucan recommended dose of 3 g/d with doses of >3 g/d and found that increasing the recommended dose resulted in further reductions of LDL-C and TC (Whitehead et al. Citation2014).
β-glucan solubility in foods varies depending on the source and processing conditions (Wang et al. Citation2016). Additionally, it has been shown that enzymatic hydrolysis of oat β-glucans affects cholesterol lowering activity, attributed to higher fecal excretion of cholesterol and bile acids, as well as the varying Mw affecting bile acid binding capacity. A study investigating two groups of rats fed high-cholesterol diets comparing β-glucan supplements with the addition of β-glucan hydrolysate demonstrated an improved lipid profile in both groups, with significantly reduced levels of LDL (25–31%) and VLDL (0.2–23%) cholesterol (Bae et al. Citation2010). A significantly lower triglyceride level was seen in the β-glucan hydrolysate group (p < 0.05), as well as greater fecal bile acid excretion. In addition, increased excretion of fecal cholesterol and triglyceride was seen in the hydrolysate group, further demonstrating its effectiveness in improving lipid profile. The results of this study suggested that factors other than viscosity may influence the bile acid binding capacities of soluble dietary fiber, indicating the need for more in vivo experiments to further elucidate these findings. Interestingly, oat fermentation has been shown to significantly increase the antioxidant capacity due to the changes and release of multiple nutrients during fermentation, and a study examining the effects of probiotic-enriched fermented oat or fermented oat with honey extracts on streptozotocin-induced diabetes in rats showed lipid lowering and antidiabetic effects (Alharbi, Algonaiman, and Barakat Citation2022).
Following these observations, an RCT with a crossover design in 30 adults with mild hypercholesterolemia consuming 3 g/d or 5 g/d of intact or partially hydrolyzed barley β-glucans compared with a control diet, found that the diet with intact barley β-glucans significantly reduced TC (−0.12 mmol/L), whereas no significant reduction was seen with partially hydrolyzed β-glucans (p = 0.0046) (Wang et al. Citation2016). This study demonstrated the effect of β-glucan solubility and Mw in meal bolus viscosity development and the cholesterol lowering effect of β-glucans. Additionally, a randomized, double-blind parallel clinical trial investigated the ability of physicochemical properties of oat β-glucan to reduce serum LDL-C among 367 participants given 3 g/d or 4 g/d oat β-glucans in extruded cereals with controlled Mw ranges (in order to achieve high-low meal bolus viscosity during digestion) for 28 days (Wolever et al. Citation2010). It was found that LDL-C significantly reduced with 3 g high-Mw, 4 g medium-Mw, and 3 g medium-Mw oat β-Glucan cereals by 0.21 mmol/L (MD: 5.5%; 95% CI: [−0.11, −0.30]; p = 0.002), 0.26 mmol/L (MD: 6.5%; 95% CI: [−0.14, −0.37]; p = 0.0007), and 0.19 mmol/L (MD: 4.7%; 95% CI: [−0.08, −0.30]; p = 0.01), respectively, when compared with control. This indicated that various degrees of viscosity may be contribute as a mechanism of action for serum cholesterol levels. Further studies are required to validate these findings with more in vivo experiments investigating whether viscosity is the determining factor for the lipid lowering effect of β-glucans.
A systematic review and meta-analysis of 28 RCTs with parallel-arm or crossover blinded interventions, ≥ 2 wk in duration, in men and women ≥18 yr of age (n = 1494) with or without hyperlipidemia compared oat or isolated β-glucan with a placebo/control group and found that both oat and isolated β-glucan interventions improved lipid profiles (de Morais Junior et al. Citation2023). Oat interventions decreased TC (−0.61, 95% CI: −0.84; −0.39, p < 0.00001, and −0.70, 95% CI: −1.07; −0.34, p = 0.0002, respectively) and LDL (−0.51, 95% CI: −0.71; −0.31, p < 0.00001, and −0.38, 95% CI: −0.60; −0.15, p = 0.001, respectively). Additionally, isolated β-glucan interventions from parallel-arm studies decreased TC (−0.73, 95% CI: −1.01; −0.45, p < 0.00001), LDL (−0.58, 95% CI: −0.85; −0.32, p < 0.0001) and triglycerides (−0.30, 95% CI: −0.49; −0.12, p = 0.001). HDL was not altered by either oat or isolated β-glucan (p > 0.05). This supports that oat consumption or isolated β-glucan supplements are effective in countering dyslipidaemia and should be considered in CVD prevention advice.
Involvement of the microbiome
It has been well established that the diverse commensal bacteria that comprise the microbiota present in the gastrointestinal tract are associated with various positive health outcomes such as in cardiovascular health, diabetes, and immune function, among others, and alterations to the microbial composition, known as gut dysbiosis can have adverse health effects (Rinninella et al. Citation2019). This review focuses on the involvement of β‐glucans and gut microbiota dysbiosis on cholesterol reduction.
Gut bacteria play an important metabolic role involving many nutrients and metabolites such as bile acids, lipids, amino acids, vitamins and SCFAs. Subtypes of SCFAs induce positive gastrointestinal, metabolic and immune effects in the host. Acetate is used as an energy source for liver and peripheral tissues and acts as a signaling molecule in gluconeogenesis and lipogenesis (Zambell, Fitch, and Fleming Citation2003). Propionate acts as a precursor for gluconeogenesis and inhibits the synthesis of cholesterol in the liver (Bull and Plummer Citation2014) and butyric acid serves as the main energy source for colonocytes and protects against inflammation (Canani et al. Citation2011).
It has also been shown that different β-glucans act as fermentable substrates for Bacteroidetes and Bifidobacterium as glycan degraders (Fernandez-Julia, Munoz-Munoz, and van Sinderen Citation2021). Bacteroides is one of the most abundant bacterial components of the human gut microbiota, while Bifidobacteria has been established as a probiotic agent. The precise mechanisms underlying these effects remain uncertain, and their influence on the gut microbiota requires revision.
β‐Glucans have more recently been found to have a positive effect on microbiota regulation and blood cholesterol reduction through involvement of the microbiome. This was demonstrated in an animal study that examined the effects of oat based dietary fiber on serum lipids, microbiota and SCFA production in rats fed 500 g oat-based products/kg for 6 wk (Drzikova, Dongowski, and Gebhardt Citation2005). It was observed that serum TC decreased (MD: −0.236 ± 0.057) in the dietary fiber fed group compared with the control group (p = 0.05). Bifidobacteria were higher in the test group (p = 0.001) and coliforms were lower (p = 0.05) compared with a control diet of no oat product intake. Bifidobacterium are a genus of beneficial bacteria that colonize the intestine and exhibit a role in immune function, metabolic processes and SCFA production (Zambell, Fitch, and Fleming Citation2003), whereas bacterial overgrowth by coliform bacteria in the intestine is an indicator of dysbiosis.
A randomized cross-over dietary intervention trial examined the effect of daily consumption of oat β-glucans (45 g/d) for 12 wk on the microbiota and cardiometabolic risk factors on individuals (n = 32) at risk of developing cardio-metabolic disease, owing to mild hypercholesterolemia or glucose intolerance (Connolly et al. Citation2016). This study found a prebiotic effect on the gut microbiota composition, depicted by a significant elevation in fecal bifidobacteria (p = 0.0001), lactobacilli (p = 0.001) and total bacterial count (p = 0.008), as well as significantly improved lipid profile by virtue of reduced TC (p = 0.0001) and LDL-C (p = 0.02), after oat intervention compared with control group.
The proposed mechanism of action of this interaction is yet undetermined. However, one of the hypotheses is that the β-linkages make β-glucan indigestible, therefore they are fermented in the colon by gut microbiota resulting in the production of SCFAs such as acetate, propionate, and butyrate, which are readily absorbed by epithelial cells (Jenkins et al. Citation2000). The gut microbiota metabolizes the fiber and yields propionate to the host, leading to an increased ratio of propionate to acetic acid, which is the main substrate for cholesterol biosynthesis, thus resulting in decreased cholesterol production (Theuwissen and Mensink Citation2008). SCFAs also limit the activity of 3-hydroxy-3-methylglutaryl coenzyme A (HMG-CoA), the rate limiting enzyme of cholesterol biosynthesis, thus further inhibiting cholesterol synthesis (Jayachandran et al. Citation2018). This was demonstrated in an experimental in vitro study that found SCFAs (propionate and butyrate) reduced the mRNA levels of HMG-Co-A, in Caco-2/TC-7 enterocytes (Alvaro et al. Citation2008), indicating the distinct effect of SCFAs on intestinal lipid metabolism and suppression of cholesterol biosynthesis.
Several findings have supported the observation that SCFAs may regulate lipid metabolism at the intestinal level. It was first seen in animal experiments that a mixture of SCFAs suppressed cholesterol synthesis (Hara et al. Citation1999). Experimental studies in rats investigated the effects of dietary consumption of SCFAs (acetic, propionic, and butyric acids) on hepatic and intestinal cholesterol synthesis. In vivo cholesterol synthesis rates in both the liver and intestine were significantly decreased (−3 mmol/L and −0.5 mmol/L respectively) in the SCFA group compared to the control group. This indicated that decreased hepatic cholesterol synthesis is mainly associated with reduced plasma cholesterol levels in rats fed SCFAs. It has also been established that propionate may decrease hepatic cholesterol biosynthesis by inhibiting HMGCR (Alvaro et al. Citation2008). It cannot be concluded however from these findings, the mechanism responsible for the cholesterol-reducing effect of soluble fibre β-glucans.
A controlled, single‐blinded, randomized crossover study investigated individuals with mild hypercholesterolemia (n = 22) given either a treatment diet containing 3 g/d high Mw, 3 g/d low Mw, 5 g/d low Mw barley β-glucan, or a control diet for 5 wk (Wang et al. Citation2014). Microbiota diversity was affected in treatment groups (p = 0.02) with the most significant difference seen between 3 g/d high Mw β-Glucan ingestion. Consumption of 3 g/d high Mw β-Glucan resulted in decreased levels of Firmicutes (77.7% vs. 88.8%) and increased Bacteroidetes (18.2% vs. 10.0%), compared to control diet (p < 0.001). Such findings indicate that consumption of 3 g/d high Mw barley β-glucan encourages certain bacterial growth, beneficial to gut health and that β-glucan induces shifts in gut microbiota in a Mw-dependent manner.
It has been established that colonic fermentation is dependent on the microbiota composition and fermentable substrate availability (Bernalier-Donadille Citation2012). A study that examined the fecal microbiota of 26 healthy individuals before and after consuming barley β-glucans (3 g/d) for two months, found altered microbial composition (Firmicutes and Bacteroidetes phyla constituting the most abundant bacterial strains) and a marked increase in SCFAs, such as 2-methyl-propanoic, acetic, butyric, and propionic acids (De Angelis et al. Citation2015). Consumption of barley β-glucans for 2 months appeared to regulate the composition and metabolic pathways of the intestinal microbiota, leading to increased SCFAs. A pilot study later found that healthy volunteers (n = 26) given 3 g/100g barley β-glucan rich pasta daily for 2 months, showed significant changes when compared with a control diet (Cosola et al. Citation2017). An increase in SCFAs associated with a saccharolytic shift in the gut microbiota metabolism was found, along with improved endothelial vascular reactivity and significantly reduced serum cholesterol including TC (183.8 ± 30.3 vs 173.3 ± 27.4 mg/dl; 95% CI: [171.0–196.6] vs [161.7–184.8]; p < 0.001) and LDL-C (107.4 ± 25.2 vs 93.8 ± 24.5 mg/dl; 95% CI [96.7–118.0] vs [83.4–104.1]; p = 0.003). However, the mechanism of action and role of the gut microbiota is still not clear and larger more controlled clinical trials are needed to confirm these findings.
Investigations into novel therapeutic interventions for cardiometabolic disease have incorporated studies investigating the effect of common pharmaceutical interventions such as statins on the gut microbiome and comparing these effects with nutritional interventions. This was examined in an animal study involving two groups of Apo-E deficient mice given a high-fat and high-cholesterol diet with one group receiving a dietary intervention of oat β‐glucan and the other receiving simvastatin (Ryan et al. Citation2017). Simvastatin is an example of a CVD therapeutic drug intervention which modulates gut microbiota composition (Catry et al. Citation2015). The oat β‐glucan intervention group compared with simvastatin, showed significant positive changes in gut microbiota composition, with the oat β‐glucan intervention exhibiting prebiotic effects on the cecal microbiota through enrichment of Verrucomicrobia population, including Akkermansia, which has been shown to have major metabolic implications (Everard et al. Citation2013). Reduced triglyceride levels were reported in the oat β‐glucan treated animals (p < 0.05) as well as a reduced extent of plaque build-up and atherogenesis. The dietary intervention was thereby deemed the preferred novel dietary intervention for long term safe use for CVD risk reduction (Ryan et al. Citation2017), however extrapolating these findings for human application is difficult.
A systematic assessment of how oat β-glucan is broken down during in vitro digestion and fermentation by mice fecal microbiota found that β-glucan could be partially degraded by in vitro digestion and fermentation (Bai, Zhao, et al. Citation2021). The researchers simulated gastrointestinal digestion and used acid hydrolysis to study the metabolism of β-glucan. They also conducted in vitro fermentation experiments and used untargeted metabolomics and 16S rRNA sequencing to analyze the fermentation process in the large intestine. The study’s findings revealed that β-glucan, when subjected to simulated gastric acidity, experienced partial degradation, resulting in a slight reduction in its molecular weight. When fermented by the microbiota found in mice feces, β-glucan activated changes in both the microbiome and metabolic pathways. The most prevalent genus involved in β-glucan utilization was Lactobacillus, while the metabolism of β-glucan in the intestine had the greatest impact on amino acids. Additionally, the breakdown of β-glucan coincided with the biosynthesis of linoleic acid. These results provide valuable insights into the metabolism of non-starch polysaccharides and the interactions between diet and gut microbiota.
In future studies, SCFAs obtained from the in vitro fermentation of soluble fibre in a human intestinal fermentation model system could be studied in order to help determine the mechanism of action. In vitro fermentation is a noninvasive and time-efficient method used to assess fibre fermentability in vivo, and can be extrapolated to human studies. Additionally, the integration of microbiome and metabolome approach to intestinal fermentation should be investigated further as this would possibly allow β-glucans to be incorporated in dietary recommendations and functional foods as a novel approach to CVD management.
Effect of β-glucans on blood pressure
High BP or hypertension is defined as systolic over diastolic blood pressure of >140/90 mmHg (NICE. Citation2019) and is also a major modifiable risk factor for CVD (Kjeldsen Citation2018). Far fewer studies have demonstrated the anti-hypertensive effects of β-glucans than its cholesterol-lowering effects. Results from animal studies have shown the possible antihypertensive effects of oat derived β-glucans either alone or in combination with commonly used medications for hypertension management such as hydrochlorothiazide (Raj et al. Citation2023). Further human intervention trials are necessary in order to confirm the mechanisms underlying the protective effects of β-glucans against hypertension ().
Research to date highlights the potential anti-hypertensive effects of dietary fiber, as seen in a meta-analysis of 24 randomized placebo-controlled trials investigating the effect of dietary fiber on BP in adults (n = 1404, median age of 42 yr) (Streppel et al. Citation2005). It was found that fiber supplementation (average dose 11.5 g/d) reduced SBP by −1.13 mmHg (95% CI: −2.49 to 0.23) and DBP by −1.26 mmHg (–2.04 to −0.48). Reductions in BP were greater in adults >40 yr old with hypertension than in younger, normotensive populations. A randomized crossover study on adults with hyperlipidemia (n = 68) reported small reductions in BP following intake of a high-fiber diet containing β-glucan or psyllium (8 g/d over 4 wk) (Jenkins et al. Citation2002), however this was observational data and required further exploration. In a randomized parallel-group study on adults with hypertension and hyperinsulinaemia, it was found that the group consuming oat cereal (5.52 g/d of β-glucan) exhibited a significant reduction in both SBP and DBP in comparison to the control group (<1 g/d of total fiber) over 6 wk (Keenan et al. Citation2002). Similarly, in a randomized double-blind placebo-controlled trial on participants (n = 110) with untreated elevated BP or stage 1 hypertension, the consumption of 8 g/d of supplemented soluble fiber from oat bran over 12 wk significantly reduced both SBP [MD: −1.8 mmHg (95% CI: −4.3 to 0.8, p = 0.17)] and DBP [MD: 1.2 mmHg (95% CI: −3.0 to 0.5, p = 0.17)] in comparison to the control group (He et al. Citation2004).
Additionally, a meta-analysis of 25 RCTs by Whelton et al. (Citation2005) found that dietary fiber intake was associated with a significant reduction in both SBP and DBP in trials conducted among patients with hypertension (SBP MD: −5.95 mmHg, 95% CI, −9.50 to −2.40; DBP MD: −4.20 mmHg, 95% CI, −6.55 to −1.85) and in trials with a duration of intervention ≥8 wk (SBP MD: −3.12 mmHg, 95% CI, −5.68 to −0.56; DBP MD: −2.57 mmHg, 95% CI, −4.01 to −1.14). Results indicated a strong potential association between increased, habitual dietary fiber consumption and reduced BP, however the mechanism of action of antihypertensive effects of dietary fiber is yet to be confirmed.
Further investigations demonstrated the potential effects of β-glucans on BP reduction, as seen in a systematic review and meta-analysis of 28 RCTs investigating the effects of dietary fiber type on BP, which found that higher consumption of β-glucans is linked with lower SBP and DBP (Evans et al. Citation2015). Results showed that diets rich in β-glucans (4 g/d) reduced SBP by 2.9 mmHg (95% CI 0.9 to 4.9 mmHg) and DBP by 1.5 mmHg (95% CI 0.2 to 2.7 mmHg).
Furthermore, in an extensive review previously discussed (Thies et al. Citation2014), it was also found that 3 of the 25 studies included showed a substantial (4–6%) decline in SBP with oat derived β-glucan consumption (Keenan et al. Citation2002; Pins et al. Citation2002; Saltzman et al. Citation2001). Two of the studies (Keenan et al. Citation2002; Saltzman et al. Citation2001) had < 30 participants in the oat intervention group, therefore were insufficiently powered to precisely gauge the effect of oats on BP outcomes. Pins et al. (Citation2002) reported reduced requirement for anti-hypertensive medication in 73% of participants after 12 wk of oat consumption compared with 42% in the control group (p = 0.05). Additionally, greater BP control with a 7 mmHg decrease in SBP and a 4 mmHg reduction in DBP was observed in participants consuming oats whose medication was not reduced. The remaining 22 studies reviewed found no significant effect of oat consumption on SBP. A significant challenge in the adequate interpretation of these studies was the methodological heterogeneity used to measure the BP of participants, thus leading to heterogeneity in the findings. Results were mostly averaged from only two or three consecutive measurements.
This was addressed in an RCT where BP was measured using more consecutive readings than conventionally carried out (until the last three measurements varied by <8%), and a significant reduction would not have been determined otherwise (Tighe et al. Citation2010). This study investigated the effect of increased consumption of whole-grain foods (3 portions of 120 g/d) on cardiovascular risk markers including BP in healthy middle-aged adults (n = 206). A significant reduction in SBP (5–6 mmHg) was reported after 12 wk in the whole-grain (wheat or oats) intervention group compared with a refined cereals group (p = 0.01). This could be translated to potentially result in a decrease in the incidence of CVD events by ≥15% when compared to similar results seen in 27 pharmacological trials (Law, Morris, and Wald Citation2009). The requirement for various intervention trials including pharmaceutical, supplementary, dietary, lifestyle, among others, where BP is an outcome incorporating accurate and consistent methodology for measuring BP is highlighted.
More recent research reaffirmed previous findings, as seen in a systematic review and meta-analysis of RCTs which demonstrated that consumption of β-glucans for ≥4 wk may result in BP reduction (Khan et al. Citation2018). Twenty-two studies (n = 1430) examined the effect of viscous soluble fiber including β-glucans and psyllium on SBP and twenty-one RCTs (n = 1343) investigated DBP. Results showed reduced SBP (MD: −1.59 mmHg [95% CI: −2.72 − 0.46]) and DBP (MD: −0.39 mmHg [95% CI: −0.76 − 0.01]) at a median dose of 8.7 g/d after a median duration of 7 wk. Substantial heterogeneity in the analysis of both SBP and DBP occurred (I2 = 72%, p < 0.01; I2 = 67%, p < 0.01, respectively), therefore a causal effect could not be confirmed. Additionally, reductions in SBP were only observed using psyllium fiber (MD: −2.39 mmHg [95% CI: −4.62 − 0.17]).
Various mechanisms underlying the antihypertensive effects of β-glucans have been hypothesized and are proposed to be strongly associated with other metabolic changes such as reduced LDL-C, body weight and improved glycaemic control (Cloetens et al. Citation2012). Insulin resistance is a major underlying mechanism contributing to the development of hypertension (Ferrannini et al. Citation1987). Soluble fibers such as β-glucans may affect BP by modulating insulin metabolism (Ferri et al. Citation1999). Reductions in serum cholesterol following the ingestion of soluble fibers have also been associated with improvements in BP (Anderson et al. Citation1995; Vogel, Corretti, and Plotnick Citation1996; Fu et al. Citation2022). β-Glucans have been shown to be effective in ameliorating blood glucose concentrations and hyperlipidemia, and thus consequently hypertension, as seen in findings from the Action to Control Cardiovascular Risk in Diabetes (ACCORD) trial (Goff et al. Citation2007), which investigated whether CVD event rates could be reduced in patients with diabetes (n = 10,251) by targeting CVD risk factors using a randomized, multicenter, double blind, 2 × 2 factorial design.
Lastly, the mechanism for BP reduction has also been proposed to be associated with weight loss related to β-glucan consumption, which is reviewed in greater detail elsewhere (Khoury et al. Citation2012). Body weight has an inverse association with hypertension. A meta-analysis of 25 RCTs published between 1996 and 2002 (n = 4874) reported that ∼5 kg body weight loss through lifestyle changes resulted in −4.4/3.6 mmHg BP reduction (Neter et al. Citation2003). Furthermore, a randomized, double-blind, controlled clinical trial investigated the effects of oat β-glucan consumption for 12 wk on BP outcomes in adults with elevated BP (n = 97) (Maki et al. Citation2007). Results showed reduced SBP (8.3 mmHg, p = 0.008) and DBP (3.9 mmHg, p = 0.018) in the β-glucan intervention group among participants with BMI above the median (31.5 kg/m2), compared to the control group.
It is therefore difficult to discern whether there is a direct beneficial causal effect of β-glucan intake on BP or this is a result of overall improved cardiovascular health due to increased β-glucan intake (Aleixandre and Miguel Citation2016). More robust, adequately controlled, longer-term clinical trials with larger cohorts and various β-glucan extracts need to be conducted to confirm which components are effective and how significantly.
An animal study investigating a potential mechanism of action of β-glucans exhibiting antihypertensive properties in mice reported a novel hypothesis involving Corin and ANP (Lee, Lee, and Kim Citation2020). Corin is a cardiac protease (ANP-converting enzyme) that activates natriuretic peptides. Activated corin cleaves pro-ANP to ANP, which regulates water-sodium balance and lowers BP. It was shown that oral consumption of β-glucans (100 mg/d) induced the expression of corin and ANP, and increased natriuresis in mice. Further research is required to confirm the mechanism by which β-glucans induce corin gene expression and extrapolation of findings to human studies for clinical translation is necessary.
What is the current gap in research?
Despite the growing body of evidence demonstrating a strong association between β-glucan intake and reduced CVD risk factors, the incomplete understanding of the diverse mechanisms of action of β-glucans remains to be a key knowledge gap in the research. Another poorly understood issue is the variation that exists in the effectiveness of various β-glucan types and sources. Evidence from both animal studies (Hara et al. Citation1999; Drzikova, Dongowski, and Gebhardt Citation2005; Bae et al. Citation2010; Ryan et al. Citation2017) and observational studies has supported the positive impact of β-glucans on various health conditions such as CVD, as a result of cholesterol and blood pressure lowering effects but there is difficulty in extrapolating from such studies, due to study outcome heterogeneity. There is an abundance of studies confirming the overall role of β-glucans in cardiovascular health, however further validation is needed regarding the optimal sources and concentrations of β-glucans for the best outcome, the varying effectiveness for different cohorts, the long-term benefits, any potential contraindications, and the degree of effectiveness for various populations. The mechanisms of action need to be fully validated particularly regarding the interaction between β-glucans and the microbiome in cardiovascular health, which is also poorly understood but an extremely promising development.
A thorough analysis of well-conducted, longer-term human based clinical intervention studies with strict inclusion and exclusion criteria, larger study cohorts and pure β-glucan extracts is necessary to fill in these research gaps, along with in-vitro studies investigating the mechanisms of action of β-glucans and the involvement of the microbiome on CVD risk factors.
Public health significance
Considering the continually emerging evidence and despite the gaps in research, there is strong evidence of a distinct relationship between β-glucan consumption and reduction in both cholesterol and BP concentrations, in order to validate recommended dietary intake for individuals with CVD. However public health policy and the food industry lags behind the findings. There is more of a focus on free sugars as they are perceived as a more achievable target for the food industry and policy makers as the body of evidence is strong and it is easier to impose limitations and restrictions than to implement increased β-glucan intakes. This is portrayed by the inadequate intakes of dietary fiber among the UK population (Evans Citation2020). A significant proportion of the UK population does not meet the current dietary recommendations for overall fiber intake of 30 g per day, the average intake is 17.2 g/d for women and 20.1 g/d for men (SACN, Citation2015), despite the robust evidence available supporting the significant role of fiber in gut health. This indicates the strength of evidence required for policy to be introduced regarding a recommended intake of β-glucans, emphasizing their benefit to cardiovascular health and also consumer marketing, labeling and availability of high-fiber foods.
Conclusion and future directions
Previous research has shown that the possible mechanisms through which β-glucans exert their hypocholesterolaemic effects are their gel-forming properties, and inhibition of the absorption of bile acid and intestinal cholesterol, whereas their anti-hypertensive effects involve corin and ANP interaction regulating sodium-water balance, and also other metabolic changes. Although the exact mechanisms by which β-glucans decrease serum cholesterol and BP concentrations still remain under investigation, findings to date have important public health implications and provide a sound scientific basis to support current recommendations for dietary fiber intake as well as potentially to contribute to the consumption of β-glucans as a novel non-pharmacological treatment. In light of the global prevalence of chronic diseases associated with diet, the significance of evidence-based dietary recommendations cannot be overstated in promoting health.
Further research is required to validate the proposed role of β-glucans in protecting against CVD. A critical analysis of the degree of effectiveness of β-glucan sources and concentrations, their extraction and administration methods and proposed mechanisms of action on CVD risk parameters need to be corroborated to identify food sources and composition data to be adapted to dietary intake recommendations for both the general adult population or targeted subpopulations with CVD. Longer term human clinical intervention trials and in vitro studies are necessary to substantiate the prolonged effect of dietary inclusion on cholesterol and BP and the interaction of the microbiome. The most commonly reported potential mechanisms of action need to be validated, particularly regarding the involvement of the gut microbiota in order to clarify the associations reported between β-glucan intake and various cardiometabolic health parameters. Validating gaps in the literature has the potential to substantiate β-glucans as a potential novel dietary therapy against modifiable risk factors for CVD and would further push the public health significance of including a habitual fiber rich diet.
At present, there is a lack of data from RCTs that establish the impact of β-glucan on clinical events related to CVD or prospective studies that used risk prediction algorithms to estimate future risk of cardiovascular disease in individuals who have high intake of β-glucans. Therefore, in our approach we focused on studies that looked at individual cardiovascular biomarkers. Furthermore, due to the challenges with adherence in a dietary intervention and the nature of nutritional research protocols, the majority of studies reported have been of short duration to report CVD clinical events, which questions whether the observed effects on biomarkers are sustained over time. Hence, as in most nutrition studies, there is a requirement for more extended, carefully executed RCTs with standardized intervention protocols, in people from a wide range of ethnicities and with a wide range of dietary habits, to assess the impact and delivery methods of β-glucan (supplements versus dietary sources) on both CVD events and CVD risk for primary prevention. The data we have are convincing enough though to emphasize the importance of increased intake of dietary fiber from a variety of plant sources in our dietary recommendations for public health nutrition purposes.
Authors’ contributions
AZK and CM were involved in the conception, study design, literature search; CM drafted the article; All authors were involved in the discussion, analysis and interpretation of studies; AZK and EP substantially revised and critically reviewed the submitted version; All authors agreed on the journal to which the article will be submitted and on the version of the article before submission.
Abbreviations | ||
ACCORD | = | action to control cardiovascular risk in diabetes |
ARBs | = | angiotensin-2 receptor blockers |
BMI | = | Body Mass Index |
BP | = | blood pressure |
CI | = | confidence interval |
CVD | = | CARDIOVASCULAR DISEASE |
CYP7A1 | = | cytochrome P450 enzyme 7-α hydroxylase |
DBP | = | diastolic blood pressure |
EFSA | = | European Food Safety Authority |
FDA | = | Food and Drug Administration |
HDL | = | high density lipoprotein |
HDL-C | = | high density lipoprotein cholesterol |
HMGCoA | = | 3-hydroxy-3-methylglutaryl coenzyme A |
HMGCoA-R | = | 3-hydroxy-3-methylglutaryl coenzyme A reductase |
LDL | = | low-density lipoprotein |
LDL-C | = | low-density lipoprotein cholesterol |
LDL-R | = | low-density lipoprotein receptor |
MD | = | mean difference |
Mw | = | molecular weight |
RCT | = | randomized controlled trial |
SBP | = | systolic blood pressure |
SCFA | = | short chain fatty acid |
TC | = | total cholesterol |
TG | = | triglycerides |
UKPDS | = | United Kingdom Prospective Diabetes Study |
Disclosure statement
The authors report there are no competing interests to declare.
Additional information
Funding
References
- AbuMweis, S. S., S. Jew, and N. P. Ames. 2020. β-glucan from barley and its lipid-lowering capacity: A meta-analysis of randomized, controlled trials. European Journal of Clinical Nutrition 64 (12):1472–80. doi: 10.1038/ejcn.2010.178.
- Aleixandre, A., and M. Miguel. 2016. Dietary fibre and blood pressure control. Food & Function 7 (4):1864–71. doi: 10.1039/c5fo00950b.
- Alharbi, H. F., R. Algonaiman, and H. Barakat. 2022. Ameliorative and antioxidative potential of Lactobacillus plantarum-fermented oat (Avena sativa) and fermented oat supplemented with Sidr honey against streptozotocin-induced type 2 diabetes in rats. Antioxidants 11 (6):1122. doi: 10.3390/antiox11061122.
- Alvaro, A., R. Solà, R. Rosales, J. Ribalta, A. Anguera, L. Masana, and J. C. Vallvé. 2008. Gene expression analysis of a human enterocyte cell line reveals downregulation of cholesterol biosynthesis in response to short-chain fatty acids. IUBMB life 60 (11):757–64. doi: 10.1002/iub.110.
- Anderson, T. J., I. T. Meredith, A. C. Yeung, B. Frei, A. P. Selwyn, and P. Ganz. 1995. The effect of cholesterol-lowering and antioxidant therapy on endothelium-dependent coronary vasomotion. The New England Journal of Medicine 332 (8):488–93. doi: 10.1056/NEJM199502233320802.
- Armet, A. M., E. C. Deehan, A. F. O’Sullivan, J. F. Mota, C. J. Field, C. M. Prado, A. J. Lucey, and J. Walter. 2022. Rethinking healthy eating in light of the gut microbiome. Cell Host & Microbe 30 (6):764–85. doi: 10.1016/j.chom.2022.04.016.
- Bae, I. Y., S. M. Kim, S. Lee, and H. G. Lee. 2010. Effect of enzymatic hydrolysis on cholesterol lowering activity of oat beta-glucan. New Biotechnology 27 (1):85–8. doi: 10.1016/j.nbt.2009.11.003.
- Bai, J., J. Zhao, W. Al-Ansi, J. Wang, L. Xue, J. Liu, Y. Wang, M. Fan, H. Qian, Y. Li, et al. 2021. Oat β-glucan alleviates DSS-induced colitis via regulating gut microbiota metabolism in mice. Food & Function 12 (19):8976–93. doi: 10.1039/d1fo01446c.
- Bai, J., T. Li, W. Zhang, M. Fan, H. Qian, Y. Li, and L. Wang. 2021. Systematic assessment of oat β-glucan catabolism during in vitro digestion and fermentation. Food Chemistry 348:129116. 30 doi: 10.1016/j.foodchem.2021.129116.
- Barsanti, L., V. Passarelli, V. Evangelista, A. M. Frassanito, and P. Gualtieri. 2011. Chemistry, physico-chemistry and applications linked to biological activities of β-glucans. Natural Product Reports 28 (3):457–66. doi: 10.1039/c0np00018c.
- Bashir, K., and J. S. Choi. 2017. Clinical and physiological perspectives of β-glucans: The past, present, and future. International Journal of Molecular Sciences 18 (9):1906. doi: 10.3390/ijms18091906.
- Behall, K. M., D. J. Scholfield, and J. Hallfrisch. 2004. Diets containing barley significantly reduce lipids in mildly hypercholesterolemic men and women. The American Journal of Clinical Nutrition 80 (5):1185–93. doi: 10.1093/ajcn/80.5.1185.
- Bernalier-Donadille, A. 2012. Fermentative metabolism by the human gut microbiota. Gastroenterologie Clinique et Biologique 34(Suppl 1):S16–S22. doi: 10.1016/S0399-8320(10)70016-6.
- Borchani, C., F. Fonteyn, G. Jamin, J. Destain, L. Willems, M. Paquot, C. Blecker, P. Thonart, B. Chema, F. Fabienne, et al. 2015. Structural characterization, technological functionality and physiological aspects of fungal β-D-glucans: A review. Critical Reviews in Food Science and Nutrition 56 (10):1746–52. doi: 10.1080/10408398.2013.854733.
- Bull, M. J., and N. T. Plummer. 2014. Part 1: The human gut microbiome in health and disease. Integrative medicine (Encinitas, Calif) 13(6), 17–22.
- Canani, R. B., M. D. Costanzo, L. Leone, M. Pedata, R. Meli, and A. Calignano. 2011. Potential beneficial effects of butyrate in intestinal and extraintestinal diseases. World Journal of Gastroenterology 17 (12):1519–28. doi: 10.3748/wjg.v17.i12.1519.
- Catry, E., B. D. Pachikian, N. Salazar, A. M. Neyrinck, P. D. Cani, and N. M. Delzenne. 2015. Ezetimibe and simvastatin modulate gut microbiota and expression of genes related to cholesterol metabolism. Life Sciences 132:77–84. doi: 10.1016/j.lfs.2015.04.004.
- Chen, C., X. Huang, H. Wang, F. Geng, and S. Nie. 2002. Effect of β-glucan on metabolic diseases: A review from the gut microbiota perspective. Current Opinion in Food Science. 47:100907. doi: 10.1016/j.cofs.2022.100907.
- Chiang, J. Y. L., and J. M. Ferrell. 2020. Up to date on cholesterol 7 alpha-hydroxylase (CYP7A1) in bile acid synthesis. Liver Research 4 (2):47–63. doi: 10.1016/j.livres.2020.05.001.
- Cloetens, L., M. Ulmius, A. Johansson-Persson, B. Akesson, and G. Onning. 2012. Role of dietary beta-glucans in the prevention of the metabolic syndrome. Nutrition Reviews 70 (8):444–58. doi: 10.1111/j.1753-4887.2012.00494.x.
- Connolly, M. L., X. Tzounis, K. M. Tuohy, and J. A. Lovegrove. 2016. Hypocholesterolemic and prebiotic effects of a whole-grain oat-based granola breakfast cereal in a cardio-metabolic "at risk" population. Frontiers in Microbiology 7:1675. doi: 10.3389/fmicb.2016.01675.
- Cosola, C., M. De Angelis, M. Rocchetti, E. Montemurno, V. Maranzano, G. Dalfino, C. Manno, A. Zito, M. Gesualdo, M. Ciccone, et al. 2017. Beta-glucans supplementation associates with reduction in P-cresyl sulfate levels and improved endothelial vascular reactivity in healthy individuals. PLoS One 12 (1):0169635. 20
- Davidson, M. H., L. D. Dugan, J. H. Burns, J. Bova, K. Story, and K. B. Drennan. 1991. The hypocholesterolemic effects of b-glucan in oatmeal and oat bran: A dose-controlled study. JAMA 265 (14):1833–9. doi: 10.1001/jama.1991.03460140061027.
- De Angelis, M., E. Montemurno, L. Vannini, C. Cosola, N. Cavallo, G. Gozzi, V. Maranzano, R. Di Cagno, M. Gobbetti, L. Gesualdo, et al. 2015. Effect of whole-grain barley on the human fecal microbiota and metabolome. Applied and Environmental Microbiology 81 (22):7945–56. doi: 10.1128/AEM.02507-15.
- de Groot, A., R. Luyken, and N. A. Pikaar. 1963. Cholesterol lowering effect of rolled oats. Lancet (London, England) 2 (7302):303–4. doi: 10.1016/s0140-6736(63)90210-1.
- de Morais Junior, A. C., R. M. Schincaglia, R. B. Viana, A. M. Armet, C. M. Prado, J. Walter, and J. F. Mota. 2023. The separate effects of whole oats and isolated beta-glucan on lipid profile: A systematic review and meta-analysis of randomized controlled trials. Clinical Nutrition ESPEN 53:224–37. doi: 10.1016/j.clnesp.2022.12.019.
- Drzikova, B., G. Dongowski, and E. Gebhardt. 2005. Dietary fibre-rich oat-based products affect serum lipids, microbiota, formation of short-chain fatty acids and steroids in rats. The British Journal of Nutrition 94 (6):1012–25. doi: 10.1079/bjn20051577.
- Du, B., M. Meenu, H. Liu, and B. Xu. 2019. A concise review on the molecular structure and function relationship of β-glucan. International Journal of Molecular Sciences 20 (16):4032. doi: 10.3390/ijms20164032.
- European Food Safety Authority 2010. Outcome of the public consultation on the draft opinion of the scientific panel on dietetic products, nutrition and allergies (NDA) on dietary reference values for carbohydrates and dietary fibre. EFSA Journal 8 (5):1508.
- Evans, C., D. Greenwood, D. Threapleton, C. Cleghorn, C. Nykjaer, C. Woodhead, C. Gale, and V. Burley. 2015. Effects of dietary fibre type on blood pressure. Journal of Hypertension 33 (5):897–911. doi: 10.1097/HJH.0000000000000515.
- Evans, C. 2020. Dietary fibre and cardiovascular health: A review of current evidence and policy. The Proceedings of the Nutrition Society 79 (1):61–7. doi: 10.1017/S0029665119000673.
- Everard, A., C. Belzer, L. Geurts, J. P. Ouwerkerk, C. Druart, L. B. Bindels, Y. Guiot, M. Derrien, G. G. Muccioli, N. M. Delzenne, et al. 2013. Cross-talk between Akkermansia muciniphila and intestinal epithelium controls diet-induced obesity. Proceedings of the National Academy of Sciences of the United States of America 110 (22):9066–71. doi: 10.1073/pnas.1219451110.
- Fernandez-Julia, P. J., J. Munoz-Munoz, and D. van Sinderen. 2021. A comprehensive review on the impact of β-glucan metabolism by Bacteroides and Bifidobacterium species as members of the gut microbiota. International Journal of Biological Macromolecules 181:877–89. doi: 10.1016/j.ijbiomac.2021.04.069.
- Ferrannini, E., G. Buzzigoli, R. Bonadonna, M. A. Giorico, M. Oleggini, L. Graziadei, R. Pedrinelli, L. Brandi, and S. Bevilacqua. 1987. Insulin resistance in essential hypertension. The New England Journal of Medicine 317 (6):350–7. doi: 10.1056/NEJM198708063170605.
- Ferri, C., C. Bellini, G. Desideri, M. Valenti, G. De Mattia, A. Santucci, N. K. Hollenberg, and G. H. Williams. 1999. Relationship between insulin resistance and nonmodulating hypertension: Linkage of metabolic abnormalities and cardiovascular risk. Diabetes 48 (8):1623–30. doi: 10.2337/diabetes.48.8.1623.
- Food and Drug Administration 1996. Food labelling: Health claims: Oats and coronary heart disease. Federal Register. 61:296–313.
- Fu, L., G. Zhang, S. Qian, Q. Zhang, and M. Tan. 2022. Associations between dietary fiber intake and cardiovascular risk factors: An umbrella review of meta-analyses of randomized controlled trials. Frontiers in Nutrition 9:972399. doi: 10.3389/fnut.2022.972399.
- Goff, D. C., Jr., H. C. Gerstein, H. N. Ginsberg, W. C. Cushman, K. L. Margolis, R. P. Byington, J. B. Buse, S. Genuth, J. L. Probstfield, and D. G. Simons-Morton. 2007. 2007. Prevention of cardiovascular disease in persons with type 2 diabetes mellitus: Current knowledge and rationale for the Action to Control Cardiovascular Risk in Diabetes (ACCORD) trial. The American Journal of Cardiology 99 (12A):4i–20i. doi: 10.1016/j.amjcard.2007.03.002.
- Golisch, B., Z. Lei, K. Tamura, and H. Brumer. 2021. Configured for the human gut microbiota: molecular mechanisms of dietary β-glucan utilization. ACS Chemical Biology 16 (11):2087–102. doi: 10.1021/acschembio.1c00563.
- Hara, H., S. Haga, Y. Aoyama, and S. Kiriyama. 1999. Short-chain fatty acids suppress cholesterol synthesis in rat liver and intestine. The Journal of Nutrition 129 (5):942–8. doi: 10.1093/jn/129.5.942.
- Hartley, L., M. D. May, E. Loveman, J. L. Colquitt, and K. Rees. 2016. Dietary fibre for the primary prevention of cardiovascular disease. The Cochrane Database of Systematic Reviews 2016 (1):CD011472. Jan 7
- He, J., R. H. Streiffer, P. Muntner, M. A. Krousel-Wood, and P. K. Whelton. 2004. Effect of dietary fiber intake on blood pressure: A randomized, double-blind, placebo-controlled trial. Journal of Hypertension 22 (1):73–80. doi: 10.1097/00004872-200401000-00015.
- Ho, H. V., J. L. Sievenpiper, A. Zurbau, M. S. Blanco, E. Jovanovski, F. Au-Yeung, A. L. Jenkins, and V. Vuksan. 2016. A systematic review and meta-analysis of randomized controlled trials of the effect of barley β-glucan on LDL-C, non-HDL-C and apoB for cardiovascular disease risk reduction. European Journal of Clinical Nutrition 70 (11):1239–45. doi: 10.1038/ejcn.2016.89.
- Hui, S., K. Liu, H. Lang, Y. Liu, X. Wang, X. Zhu, S. Doucette, L. Yi, and M. Mi. 2019. Comparative effects of different whole grains and brans on blood lipid: A network meta-analysis. European Journal of Nutrition 58 (7):2779–87. doi: 10.1007/s00394-018-1827-6.
- Jayachandran, M., J. Chen, S. Sum Man Chung, and B. Xu. 2018. A critical review on the impacts of β-glucans on gut microbiota and human health. The Journal of Nutritional Biochemistry 61:101–10. doi: 10.1016/j.jnutbio.2018.06.010.
- Jenkins, D. J., C. W. Kendall, M. Axelsen, L. S. Augustin, and V. Vuk-San. 2000. Viscous and nonviscous fibres, nonabsorbable and low-glycaemic index carbohydrates, blood lipids and coronary heart disease. Current Opinion in Lipidology 11 (1):49–56. doi: 10.1097/00041433-200002000-00008.
- Jenkins, D. J. A., C. W. C. Kendall, V. Vuksan, E. Vidgen, T. Parker, D. Faulkner, C. C. Mehling, M. Garsetti, G. Testolin, S. C. Cunnane, et al. 2002. Soluble fiber intake at a dose approved by the US Food and Drug Administration for a claim of health benefits: Serum lipid risk factors for cardiovascular disease assessed in a randomized controlled crossover trial. The American Journal of Clinical Nutrition 75 (5):834–9. doi: 10.1093/ajcn/75.5.834.
- Joyce, S. A., A. Kamil, L. Fleige, and C. G. M. Gahan. 2019. The cholesterol-lowering effect of oats and oat beta glucan: modes of action and potential role of bile acids and the microbiome. Frontiers in Nutrition 6:171. doi: 10.3389/fnut.2019.00171.
- Kaur, R., M. Sharma, D. Ji, M. Xu, and D. Agyei. 2019. Structural features, modification, and functionalities of beta-glucan. Fibers 8 (1):1. doi: 10.3390/fib8010001.
- Keenan, J. M., J. J. Pins, C. Frazel, A. Moran, and L. Turnquist. 2002. Oat ingestion reduces systolic and diastolic blood pressure in patients with mild or borderline hypertension: A pilot trial. The Journal of Family Practice 51 (4):369.
- Keys, A., J. T. Anderson, and F. Grande. 1960. Diet-type (fats constant) and blood lipids in man. The Journal of Nutrition 70 (2):257–66. doi: 10.1093/jn/70.2.257.
- Khan, K., E. Jovanovski, H. V. T. Ho, A. C. R. Marques, A. Zurbau, S. B. Mejia, J. L. Sievenpiper, and V. Vuksan. 2018. The effect of viscous soluble fibre on blood pressure: A systematic review and meta-analysis of randomized controlled trials. Nutrition, Metabolism, and Cardiovascular Diseases: NMCD 28 (1):3–13. doi: 10.1016/j.numecd.2017.09.007.
- Khoury, D. E., C. Cuda, B. L. Luhovyy, and G. H. Anderson. 2012. Beta glucan: health benefits in obesity and metabolic syndrome. Journal of Nutrition and Metabolism 2012:851362–28. doi: 10.1155/2012/851362.
- Kjeldsen, S. E. 2018. Hypertension and cardiovascular risk: General aspects. Pharmacological Research 129:95–9. doi: 10.1016/j.phrs.2017.11.003.
- Law, M. R., J. K. Morris, and N. J. Wald. 2009. Use of blood pressure lowering drugs in the prevention of cardiovascular disease: Meta-analysis of 147 randomised trials in the context of expectations from prospective epidemiological studies. BMJ (Clinical Research ed.) 338 (may19 1):b1665–b1665. doi: 10.1136/bmj.b1665.
- Lee, S. J., D. H. Lee, and H. W. Kim. 2020. Novel antihypertension mechanism of β-glucan by corin and ANP-mediated natriuresis in mice. Mycobiology 48 (5):399–409. doi: 10.1080/12298093.2020.1812150.
- Lia, A., G. Hallmans, A. S. Sandberg, B. Sundberg, P. Aman, and H. Andersson. 1995. Oat beta-glucan increases bile excretion and a fibre rich barley fracton increases cholesterol excretion in ileostomy subjects. The American Journal of Clinical Nutrition 62 (6):1245–51. doi: 10.1093/ajcn/62.6.1245.
- Maki, K. C., R. Galant, P. Samuel, J. Tesser, M. S. Witchger, J. D. Ribaya-Mercado, J. B. Blumberg, and J. Geohas. 2007. Effects of consuming foods containing oat beta-glucan on blood pressure, carbohydrate metabolism and biomarkers of oxidative stress in men and women with elevated blood pressure. European Journal of Clinical Nutrition 61 (6):786–95. doi: 10.1038/sj.ejcn.1602562.
- Mathews, R., A. Kamil, and Y. Chu. 2020. Global review of heart health claims for oat beta-glucan products. Nutrition Reviews 78 (Suppl 1):78–97. doi: 10.1093/nutrit/nuz069.
- Mejía, S. M. V., A. De Francisco, and B. Bohrer. 2020. A comprehensive review on cereal beta-glucan: Extraction, characterization, causes of degradation, and food application. Critical Reviews in Food Science and Nutrition 60 (21):3693–704. doi: 10.1080/10408398.2019.1706444.
- Morgado, M., S. Rolo, A. F. Macedo, L. Pereira, and M. Castelo-Branco. 2010. Predictors of uncontrolled hypertension and antihypertensive medication nonadherence. Journal of Cardiovascular Disease Research 1 (4):196–202. doi: 10.4103/0975-3583.74263.
- Murphy, E. J., E. Rezoagli, I. Major, N. J. Rowan, and J. G. Laffey. 2020. β-glucan metabolic and immunomodulatory properties and potential for clinical application. Journal of Fungi 6 (4):356. 10doi: 10.3390/jof6040356.
- Musini, V. M., F. Gueyffier, L. Puil, D. M. Salzwedel, and J. M. Wright. 2017. Pharmacotherapy for hypertension in adults aged 18 to 59 years. The Cochrane Database of Systematic Reviews 8 (8):CD008276. doi: 10.1002/14651858.CD008276.pub2.
- Neter, J. E., B. E. Stam, F. J. Kok, D. E. Grobbee, and J. M. Geleijnse. 2003. Influence of weight reduction on blood pressure: A meta-analysis of randomized controlled trials. Hypertension (Dallas, Tex.: 1979) 42 (5):878–84. doi: 10.1161/01.HYP.0000094221.86888.AE.
- NICE. 2019. Hypertension in adults: Diagnosis and management; Accessed July 2021; Available at: www.nice.org.uk/guidance/cg127/ chapter/1-Guidance
- O’Grady, J., E. M. O’Connor, and F. Shanahan. 2019. Review article: Dietary fibre in the era of microbiome science. Alimentary Pharmacology & Therapeutics 49 (5):506–15. doi: 10.1111/apt.15129.
- Pins, J. J., D. Geleva, J. M. Keenan, C. Frazel, P. J. O’Connor, and L. M. Cherney. 2002. Do whole-grain oat cereals reduce the need for antihypertensive medications and improve blood pressure control? The Journal of Family Practice 51 (4):353–9.
- Poppitt, S. D. 2007. Soluble fibre oat and barley beta-glucan enriched products: Can we predict cholesterol-lowering effects? The British Journal of Nutrition 97 (6):1049–50. doi: 10.1017/S0007114507690023.
- Queenan, K. M., M. L. Stewart, K. N. Smith, W. Thomas, R. G. Fulcher, and J. L. Slavin. 2007. Concentrated oat beta-glucan, a fermentable fiber, lowers serum cholesterol in hypercholesterolemic adults in a randomized controlled trial. Nutrition Journal 6 (1):6. doi: 10.1186/1475-2891-6-6.
- Raj, P., K. Sayfee, L. Yu, A. Sabra, C. Wijekoon, L. Malunga, S. J. Thandapilly, and T. Netticadan. 2023. Oat beta-glucan alone and in combination with hydrochlorothiazide lowers high blood pressure in male but not female spontaneously hypertensive rats. Nutrients 15 (14):3180. doi: 10.3390/nu15143180.
- Ramkumar, S., A. Raghunath, and S. Raghunath. 2016. Statin therapy: review of safety and potential side effects. Acta Cardiologica Sinica 32 (6):631–9.
- Rinninella, E., P. Raoul, M. Cintoni, F. Franceschi, G. Miggiano, A. Gasbarrini, and M. C. Mele. 2019. What is the healthy gut microbiota composition? A changing ecosystem across age, environment, diet, and diseases. Microorganisms 7 (1):14. doi: 10.3390/microorganisms7010014.
- Ripsin, C. M., J. M. Keenan, D. R. Jacobs, P. J. Elmer, R. R. Welch, L. Van Horn, K. Liu, W. H. Turnbull, F. W. Thye, and M. Kestin. 1992. Oat products and lipid lowering: A meta-analysis. JAMA 267 (24):3317–25.
- Rondanelli, M., A. Opizzi, and F. Monteferrario. 2009. The biological activity of beta-glucans. Minerva Medica 100 (3):237–45.
- Ryan, P. M., L. E. E. London, T. C. Bjorndahl, R. Mandal, K. Murphy, G. F. Fitzgerald, F. Shanahan, R. P. Ross, D. S. Wishart, N. M. Caplice, et al. 2017. Microbiome and metabolome modifying effects of several cardiovascular disease interventions in apo-E−/− mice. Microbiome. Microbiome 5 (1):30. doi: 10.1186/s40168-017-0246-x.
- Scientific Advisory Committee on Nutrition 2015. – GOV.UK.2021. Public Health England. Accessed Online June 2021: https://www.gov.uk/government/publications/sacn-carbohydrates-and-health-report.
- Saltzman, E., S. K. Das, A. H. Lichtenstein, G. E. Dallal, A. Corrales, E. J. Schaefer, A. S. Greenberg, and S. B. Roberts. 2001. An oat-containing hypocaloric diet reduces systolic blood pressure and improves lipid profile beyond effects of weight loss in men and women. The Journal of Nutrition 131 (5):1465–70. doi: 10.1093/jn/131.5.1465.
- Schmidt, M. 2022. Cereal beta-glucans: An underutilized health endorsing food ingredient. Critical Reviews in Food Science and Nutrition 62 (12):3281–300. doi: 10.1080/10408398.2020.1864619.
- Sima, P., L. Vannucci, and V. Vetvicka. 2018. β-glucans and cholesterol. International Journal of Molecular Medicine 41 (4):1799–808.
- Silva, I. M. V., F. Machado, M. J. Moreno, C. Nunes, M. A. Coimbra, and F. Coreta-Gomes. 2021. Polysaccharide structures and their hypocholesterolemic potential. Molecules (Basel, Switzerland) 26 (15):4559. doi: 10.3390/molecules26154559.
- Soliman, G. A. 2019. Dietary fiber, atherosclerosis, and cardiovascular disease. Nutrients 11 (5):1155. doi: 10.3390/nu11051155.
- Streppel, M. T., L. R. Arends, P. van ‘t Veer, D. E. Grobbee, and J. M. Geleijnse. 2005. Dietary fiber and blood pressure: A meta-analysis of randomized placebo-controlled trials. Archives of Internal Medicine 165 (2):150–6. doi: 10.1001/archinte.165.2.150.
- Talati, R., W. L. Baker, M. S. Pabilonia, C. M. White, and C. Coleman. 2009. The effects of barley-derived soluble fibre on serum lipids. Annals of Family Medicine 7 (2):157–63. doi: 10.1370/afm.917.
- Theuwissen, E., and R. P. Mensink. 2008. Water-soluble dietary fibers and cardiovascular disease. Physiology & Behavior 94 (2):285–92. doi: 10.1016/j.physbeh.2008.01.001.
- Thies, F., L. F. Masson, P. Boffetta, and P. Kris-Etherton. 2014. Oats and CVD risk markers: A systematic literature review. The British Journal of Nutrition 112 Suppl 2:S19–S30. doi: 10.1017/S0007114514002281.
- Threapleton, D. E., D. C. Greenwood, C. E. L. Evans, C. L. Cleghorn, C. Nykjaer, C. Woodhead, J. E. Cade, C. P. Gale, and V. J. Burley. 2013. Dietary fibre intake and risk of cardiovascular disease: Systematic review and meta-analysis. BMJ (Clinical Research ed.) 347 (dec19 2):f6879–f6879. doi: 10.1136/bmj.f6879.
- Tighe, P., G. Duthie, N. Vaughan, J. Brittenden, W. G. Simpson, S. Duthie, W. Mutch, K. Wahle, G. Horgan, and F. Thies. 2010. Effect of increased consumption of whole-grain foods on blood pressure and other cardiovascular risk markers in healthy middle-aged persons: A randomized controlled trial. The American Journal of Clinical Nutrition 92 (4):733–40. doi: 10.3945/ajcn.2010.29417.
- Tiwari, U., and E. Cummins. 2011. Meta-analysis of the effect of b-glucan intake on blood cholesterol and glucose levels. Nutrition (Burbank, Los Angeles County, Calif.) 27 (10):1008–16. doi: 10.1016/j.nut.2010.11.006.
- Tosh, S. M., and N. Bordenave. 2020. Emerging science on benefits of whole grain oat and barley and their soluble dietary fibres for heart health, glycemic response, and gut microbiota. Nutrition Reviews 78 (Suppl 1):13–20. doi: 10.1093/nutrit/nuz085.
- Vogel, R. A., M. C. Corretti, and G. D. Plotnick. 1996. Changes in flow-mediated brachial artery vasoactivity with lowering of desirable cholesterol levels in healthy middle-aged men. The American Journal of Cardiology 77 (1):37–40. doi: 10.1016/s0002-9149(97)89131-x.
- Wang, Y., N. Ames, S. Li, P. Jones, and E. Khafipour. 2014. High molecular weight barley β-glucan supports bacterial populations beneficial for gut health (647.45). FASEB Journal. 28 (1).
- Wang, Y., N. P. Ames, H. M. Tun, S. M. Tosh, P. J. Jones, and E. Khafipour. 2016. High molecular weight barley b-glucan alters gut microbiota toward reduced cardiovascular disease risk. Frontiers in Microbiology 7:129. doi: 10.3389/fmicb.2016.00129.
- Wang, Y., S. Harding, S. Thandapilly, S. Tosh, P. Jones, and N. Ames. 2017. Barley β-glucan reduces blood cholesterol levels via interrupting bile acid metabolism. The British Journal of Nutrition 118 (10):822–9. doi: 10.1017/S0007114517002835.
- Whelton, S. P., A. D. Hyre, B. Pedersen, Y. Yi, P. K. Whelton, and J. He. 2005. Effect of dietary fiber intake on blood pressure: A meta-analysis of randomized, controlled clinical trials. Journal of Hypertension 23 (3):475–81. doi: 10.1097/01.hjh.0000160199.51158.cf.
- Whitehead, A., E. J. Beck, S. Tosh, and T. M. S. Wolever. 2014. Cholesterol-lowering effects of oat b-glucan: A meta-analysis of randomized controlled trials. The American Journal of Clinical Nutrition 100 (6):1413–21. doi: 10.3945/ajcn.114.086108.
- Wolever, T. M., S. M. Tosh, A. L. Gibbs, J. Brand-Miller, A. M. Duncan, V. Hart, B. Lamarche, B. A. Thomson, R. Duss, and P. J. Wood. 2010. Physicochemical properties of oat β-glucan influence its ability to reduce serum LDL cholesterol in humans: A randomized clinical trial. The American Journal of Clinical Nutrition 92 (4):723–32. doi: 10.3945/ajcn.2010.29174.
- Wouk, J., R. F. H. Dekker, E. A. I. F. Queiroz, and A. M. Barbosa-Dekker. 2021. β-Glucans as a panacea for a healthy heart? Their roles in preventing and treating cardiovascular diseases. International Journal of Biological Macromolecules 177:176–203. doi: 10.1016/j.ijbiomac.2021.02.087.
- Yang, D., Z. Zhou, and L. Zhang. 2019. An overview of fungal glycan-based therapeutics. Progress in Molecular Biology and Translational Science 163:135–63. doi: 10.1016/bs.pmbts.2019.02.001.
- Zambell, K. L., M. D. Fitch, and S. E. Fleming. 2003. Acetate and butyrate are the major substrates for de novo lipogenesis in rat colonic epithelial cells. The Journal of Nutrition 133 (11):3509–15. doi: 10.1093/jn/133.11.3509.
- Zhu, X., X. Sun, M. Wang, C. Zhang, Y. Cao, G. Mo, J. Liang, and S. Zhu. 2015. Quantitative assessment of the effects of beta-glucan consumption on serum lipid profile and glucose level in hypercholesterolemic subjects. Nutrition, Metabolism, and Cardiovascular Diseases: NMCD 25 (8):714–23. Aug doi: 10.1016/j.numecd.2015.04.008.