Abstract
This review systematically explores the pivotal role of food science and technology as a support for Phenylketonuria (PKU) dietary management. It delves into the genetic and metabolic underpinnings of PKU, highlighting the crucial need for stringent dietary regulation to manage phenylalanine levels and mitigate neurological complications. Through bibliometric analysis and current product evaluations, it identifies trends in PKU food research, emphasizing recent innovations in food formulations such as glycomacropeptide (GMP) supplements and higher appealing low-phenylalanine food products. Furthermore, it accentuates the sensory and consumer aspects of PKU dietary solutions, underscoring the importance of palatability for adherence. Notably, the review introduces 3D food printing as an emerging technology for creating personalized, nutrient-optimized, and sensory-appealing foods for PKU patients, offering a new horizon in dietary management. This comprehensive assessment underscores the dynamic interplay between nutritional science, food technology, and sensory evaluation in improving the quality of life for individuals with PKU.
Introduction
Phenylketonuria (PKU) is a genetic disorder characterized by an inability to metabolize the amino acid phenylalanine due to a deficiency of the enzyme phenylalanine hydroxylase (PAH). This enzyme is crucial for converting phenylalanine into tyrosine, another amino acid essential for various bodily functions (Delsoglio et al. Citation2023; Soltanizadeh and Mirmoghtadaie Citation2014). When PAH is deficient or absent, phenylalanine accumulates in the blood and brain, leading to a range of metabolic and neurological complications.
PKU is inherited in an autosomal recessive pattern, which means that an individual must receive a defective gene from both parents to be affected (Mitchell, Trakadis, and Scriver Citation2011). The gene responsible for PKU is located on chromosome 12 and involves various mutations in the PAH gene. These mutations result in reduced activity or a complete lack of the PAH enzyme. Over 600 different mutations of the PAH gene have been identified, contributing to the variability in the severity and presentation of PKU among affected individuals (Groselj et al. Citation2012).
The accumulation of phenylalanine in individuals with PKU can have severe metabolic and neurological implications if not managed effectively. High levels of phenylalanine can interfere with brain development and function, leading to intellectual disability, behavioral problems, seizures, developmental delays, psychiatric disorders, and skin conditions, such as eczema (Pilotto et al. Citation2021).
The primary management strategy for PKU involves a strict diet that limits phenylalanine intake, preventing its accumulation (van Calcar and Ney Citation2012; Newby Citation2023). This is a cornerstone for PKU management as individuals with PKU must avoid foods high in protein, such as meat, fish, eggs, nuts, dairy, and legumes, as well as foods containing aspartame, a sweetener that is metabolized into phenylalanine (Maler et al. Citation2023). Adhering to such a restrictive diet can be challenging, especially over the long term. This diet is typically low in high-protein foods and may include specially formulated medical foods and protein substitutes that are phenylalanine-free or low in phenylalanine (Newby Citation2023; Lim et al. Citation2007). It is recommended that the diet be well-planned to include a variety of fruits and vegetables to prevent micronutrient deficiencies commonly associated with restrictive diets (van Wegberg et al. Citation2017). The European guidelines suggest that metabolic dietitians should closely monitor and adjust these diets to cater to the individual needs of patients, ensuring they receive a balanced intake of vitamins, minerals, and other nutrients.
Early and consistent dietary management can prevent most of the adverse effects of PKU, allowing individuals with this condition to lead healthy lives. Recent advances have also introduced enzyme therapy as a treatment option for adults with PKU, which can help reduce blood phenylalanine levels (Mahan, Gandhi, and Anand Citation2019). Recent developments in pharmacological interventions for PKU management have seen the utilization of sapropterin dihydrochloride (Kuvan®), an orally-administered synthetic form of tetrahydrobiopterin (BH4), and pegylated phenylalanine ammonia-lyase (pegvaliase), which have been effectively used alongside a phenylalanine-restricted diet to enhance metabolic control and augment natural protein tolerance in the majority of non-classical PKU patients, with a smaller subset of classical PKU patients also exhibiting responsiveness to the treatment (Upadia et al. Citation2024). Additionally, research continues into gene therapy and other innovative treatments to address the underlying genetic cause of PKU. New protein-based ingredients with reduced phenylalanine intake have been introduced into the diets of subjects suffering from PKU. In particular, the glycomacropeptide (GMP) stands out, in recent years has been introduced into protein supplements as it contains very low content of Phe (van Calcar and Ney Citation2012; C. Proserpio et al. Citation2019; Delsoglio et al. Citation2023).
The dietary restrictions can have significant social and psychological impacts. Individuals with PKU may feel isolated during social events centered around food, such as parties or family gatherings (Delsoglio et al. Citation2023). Children, in particular, may feel different from their peers and experience difficulties in school or social settings (Teruya, Remor, and Schwartz Citation2020). Maintaining strict dietary control is a lifelong commitment and can be particularly challenging as children with PKU grow into adolescence and adulthood. The desire for independence and peer influence can lead to dietary non-adherence, which can have immediate and long-term health consequences. Low-phenylalanine medical foods and protein substitutes often have different taste and texture if compared to their high-protein counterparts, which can be less appealing and may affect adherence to the diet (Delsoglio et al. Citation2023; Newby Citation2023).
In response to these challenges, research in food science and technology aims to develop new food products and dietary strategies that can help mitigate some of the limitations of the traditional low-phenylalanine diet. Innovations include the development of more palatable and nutritionally adequate protein substitutes, as well as enzyme therapies that offer alternative or complementary approaches to managing PKU (Mahan, Gandhi, and Anand Citation2019). Improving the palatability and variety of PKU-friendly foods is crucial for dietary adherence. Advances in food technology allow for the development of low-phenylalanine products that closely mimic the taste and texture of their higher-phenylalanine counterparts (Escalante‐Aburto et al. Citation2021). This includes protein substitutes, low-protein versions of staple foods, and phenylalanine-free snacks and treats. Greater variety and improved taste can help alleviate diet fatigue and make it easier for individuals with PKU to maintain their dietary regimen over the long term (Soltanizadeh and Mirmoghtadaie Citation2014).
Food design also focuses on making PKU dietary management more convenient and accessible. This includes creating ready-to-eat products, easy-to-prepare meal kits, and portable snacks that fit seamlessly into busy lifestyles (Poole et al. Citation2022). Increasing the availability of PKU-friendly foods in supermarkets and online platforms is also a key objective, making it easier for individuals and families to obtain the products they need.
This review aims to assess the impact of food science and technology in the development of low phenylalanine protein substitutes for PKU subjects. To evaluate this, the review is divided into three parts: the first part involves a bibliometric analysis of scientific databases related to the "PKU-food" topic to study scientific trends; the second section focuses on currently existing products for PKU, including protein supplements and alternative foods, followed by a third section focusing on insights and innovations in food science for PKU management. This latter section places particular emphasis on sensory aspects and consumer science, highlighting how advancements in these areas can contribute to more effective and appealing food solutions for PKU patients.
Method of review
Bibliometric analysis
The investigation chose a bibliometric approach over a systematic literature review (SLR) due to its advantageous attributes. In fact, unlike SLRs which often depend on qualitative evaluations and could potentially introduce bias, the bibliometric approach is fundamentally quantitative. This quantitative emphasis enhances the objectivity of the research findings by minimizing the likelihood of bias (Donthu et al. Citation2021).
The investigation took place in February 2024. The aim was to evaluate the research trends based on literature in the Web of Science and Elsevier Scopus databases, over the last 23 years (from 2000 to February 2024), by retrieving and selecting those papers that investigate the relevance of food science and technology in the formulation of new PKU food for diet management, protein substitutes and low phenylalanine food. Keywords, headings, and abstracts were inspected to identify relevant research. To ensure the quality of the research, only peer-reviewed articles were selected. In order to be scientifically reliable, preprints, non-English language articles, or other non-peer-reviewed material were excluded. The query used for the research of papers and keywords is the following: (TITLE-ABS-KEY (phenylketonuria) AND TITLE-ABS-KEY (food) OR TITLE-ABS-KEY (food AND design) OR TITLE-ABS-KEY (food AND science) OR TITLE-ABS-KEY (low AND phenylalanine AND food) OR TITLE-ABS-KEY (meal)) AND PUBYEAR > 1999 AND PUBYEAR < 2025 AND (EXCLUDE (DOCTYPE, “dp”) OR EXCLUDE (DOCTYPE, “er”) OR EXCLUDE (DOCTYPE, “bk”) OR EXCLUDE (DOCTYPE, “sh”) OR EXCLUDE (DOCTYPE, “ed”) OR EXCLUDE (DOCTYPE, “le”) OR EXCLUDE (DOCTYPE, “no”)) AND (LIMIT-TO (LANGUAGE, “English”))
Software used and procedures
Measurements of the quality and quantity of the scientific production were accomplished using the VOS Viewer science mapping software tool (Eck and Waltman Citation2020). Previously, selected data for VOS Viewer supported file types (Web of Science and Elsevier Scopus) were exported to Microsoft 365 Excel and then elaborated. From the database, 2580 keywords were extrapolated, but for the analysis, the occurrence threshold was set at a minimum of 10 between all of the documents (Russo et al. Citation2024). Along with the VOS viewer, the R package bibliometrix was used to clean, analyze, and visualize the literature data. Bibliometrix was created by Aria M. and Cuccurullo C. and built in R, a programming language for statistical computing and graphics (Aria and Cuccurullo Citation2017).
The methodology for this research incorporated procedures recommended by Echchakoui (Citation2020) for combining data from the WoS and Scopus databases, executed in the following sequence:
Transformation of WoS and Scopus database records into bibliographic files via Endnote software.
Conversion of the bibliographic files from both databases into BibTeX format utilizing the Bibliometrix package (Aria and Cuccurullo Citation2017).
Standardization of field tags across both databases by Microsoft Excel.
Integration of the two databases into a cohesive set with the aid of the Bibliometrix package.
Elimination of any redundant entries resulting from the database merger by employing Microsoft Excel.
The output of these procedures was: a performance analysis, which includes the evaluation of scientific publications; and science mapping, which includes the collaboration structure between countries, the co-occurrence citation network, a keywords factorial analysis (through multiple correspondence analysis) and thematic maps.
Thematic mapping is a method for analyzing and visualizing academic research topics, originally introduced by Callon, Courtial, and Laville (Citation1991). It utilizes a coordinate system based on two metrics: centrality (x-axis) and density (y-axis). Centrality reflects the extent of a cluster’s connections with others, indicating its importance to the scientific or technological community. Density measures the cohesion within a cluster, showing how integrated and coherent the research problems are. This approach allows for the classification of research subjects into four quadrants, each representing a distinct thematic area, based on the position of relevant keywords from bibliographic data. Over the past decade, interpretations of these quadrants have evolved to categorize them into motor themes (central and developed), basic and transversal themes (central and undeveloped), highly developed and isolated themes (peripheral and developed), and emerging or declining themes (peripheral and undeveloped), as per Cobo et al.’s interpretation (Yu and Muñoz-Justicia Citation2020). This method provides a structured way to understand the distribution and significance of research topics within a field.
PKU-food bibliometric analysis results
A total of 505 documents were detected, of which 361 research papers, 104 reviews, 24 book chapters and 16 conference papers. The number of documents produced each year, published since 2000 on the selected topic is reported in .
Figure 1. Annual scientific production obtained from Scopus-Web of Science database on PKU-food related search.
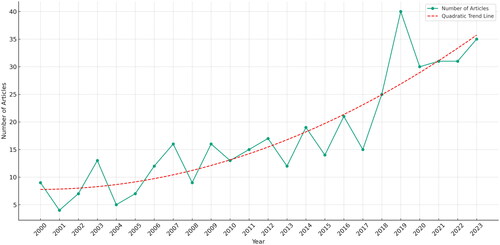
presents the trend in annual scientific production related to PKU and its association with food products, protein substitutes, and food design/technology, as indexed by Scopus and WoS databases from 2000 to February 2024. The time series graph indicates a fluctuating yet overall upward trajectory in the number of articles published over the two decades. Notably, after a period of relatively lower and stable output from 2000 to 2014, there is a noticeable increase in publications from 2015 onwards, reaching a peak around 2019. The increasing publications in recent years (2019–2023) could indicate a surge in innovative research focused on developing new food products and dietary strategies for PKU management.
This pattern reflects a growing recognition of the importance of dietary management in PKU and the development of novel food products and protein substitutes. The peak in 2019 could correspond to breakthrough research, significant funding, or a surge in academic and clinical interest, while the fluctuations may indicate varying research priorities, funding availability, or changes in publication practices over the years. The graph underscores the dynamic nature of research activity in this field, active engagement with the challenges of managing PKU through dietary innovations. This upward trend suggests growing interest and advancements in PKU research, particularly in the last decade.
To understand the most relevant countries identified in the bibliometric analysis, reports the distribution of corresponding authors’ countries.
Figure 2. Distribution of corresponding authors’ countries in PKU-related research, categorized by single country publications (SCP) and multiple country publications (MCP).
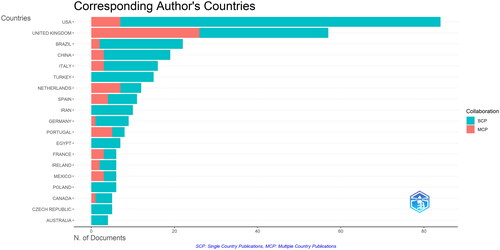
illustrates the geographical distribution of corresponding authors’ countries in publications related to PKU-food topic, highlighting the global research efforts and collaborative dynamics in this field. The conducted analysis distinguishes between single-country publications (SCP) and multiple-country publications (MCP), providing insights into collaborative trends. The prevalence of SCPs across all countries underscores a strong national focus on PKU research. However, the presence of MCPs, particularly in the United Kingdom and Netherlands, points to international research collaborations, likely driven by the need for diverse genetic and dietary studies across populations, which can lead to more generalizable findings.
The United States leads in the total number of articles published, with 84 articles, which suggests a strong research interest and investment in the topics of PKU-food science within the country. However, it is notable that the majority of these publications are SCP, with only a small portion (8.3%) being MCP, indicating a lower rate of international collaboration. The United Kingdom, despite having fewer total articles (57) compared to the USA, has a significantly higher rate of international collaboration, with 45.6% of its articles being MCP. This demonstrates the UK’s strong engagement in international research networks and a propensity to collaborate beyond its borders.
Interestingly, some countries with fewer total publications exhibit very high rates of international collaboration. For instance, Portugal, with 8 articles, has the highest MCP ratio (62.5%), followed by the Netherlands (58.3%), which indicates that these countries, while producing less research overall, are highly collaborative internationally.
Brazil, China and Italy also show substantial contributions, suggesting a high level of engagement with PKU research, which may reflect the prevalence of the condition or the focus of medical research in these countries.
The contributions from countries like Turkey, Iran, and Italy, although smaller in number, are significant as they indicate growing research interest and potential advancements in PKU research within these regions. The collaboration patterns suggest that while PKU research is concentrated in certain countries, there is a global effort to address the challenges posed by this genetic condition. Such collaborations are essential for advancing the understanding of PKU management, improving dietary interventions, and developing innovative treatment strategies that can be adapted across different healthcare systems and dietary habits worldwide.
Key research focus areas and keywords
A keyword cloud related to searched keywords is represented in . The font size and dimensions of clouds of the given keywords is proportional to the number of times they appeared in literature records.
Figure 3. Database keywords co-occurrence network based on the search of PKU-food topic from Scopus and Web of Science database (a, left) and overlay visualization based on temporal dimension from 2000 to 2023 (b, right).
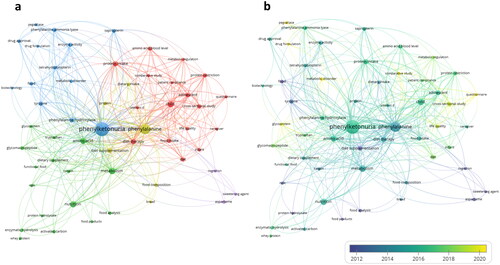
The network’s complexity, with multiple intersecting nodes and connections, highlights the interdisciplinary nature of PKU research, encompassing nutrition, biochemistry, and food science. It also points to an increase in collaborative efforts, as proven by the interlinked keywords across various study areas. The keywords co-occurrence network analysis obtained 5 different clusters depicted in different colors ().
As expected, “phenylketonuria” is the core topic, being the focus of the research. The high frequency indicates ongoing research efforts in understanding and managing PKU.
Within this context, the prominence of “glycomacropeptide”, “dietary supplement”, and “functional food” suggests a focus on the development of specialized food products tailored to the nutritional needs of individuals with PKU. The presence of specific treatment-related keywords (e.g., tetrahydrobiopterin, pegvaliase) points to an expanding interest in therapeutic interventions beyond traditional dietary management.
The appearance of “protein hydrolysate”, “enzyme hydrolysis”, and “activated carbon” within close proximity indicates that technological processes are pivotal in creating low-Phe food products, employing methods such as enzymatic hydrolysis to break down protein structures and reduce Phe content. The integration of “food analysis”, “nutrition”, and “metabolism” within the network emphasizes the importance of rigorous food product testing and nutritional analysis to ensure these foods meet metabolic requirements without compromising on taste and quality. The increase in publications over time and the diversity of focus areas reflect a dynamic research landscape, with ongoing efforts to improve PKU management through both dietary and pharmacological means.
The temporal dimension of keywords, as indicated by the color gradient (), reveals a progression of research focus over time. The most recent terms, shown in yellow, include “glycoprotein”, “comparative study” and “questionnaire”, reflecting a growing interest in PKU consumer acceptance. This suggests that the field is moving toward a more holistic approach, considering not only the metabolic but also the developmental and social aspects of PKU.
In , the Principal Component Analysis (PCA) of keywords found in the bibliometric database is illustrated.
Figure 4. Principal component analysis of keywords from bibliometric database. Each point represents a keyword, color-coded by clusters that signify thematic concentrations in the research field. The axes intersect at zero, highlighting the mean-centered scaling of PCA dimensions.
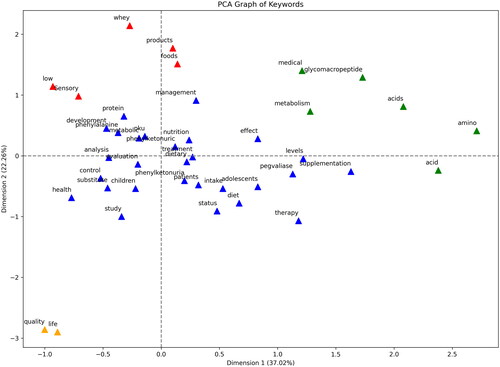
The PCA of keywords from the PKU-food bibliometric database reveals distinct clusters that represent the focal areas of the research within the field. Cluster 1, colored blue, includes keywords such as “phenylketonuria”, “patients”, “dietary”, and “management”, indicating a concentration of studies focused on the management of PKU through dietary interventions. This cluster suggests a research trend aimed at understanding the disease’s management in affected individuals.
Cluster 2, depicted in green, includes “amino acid” and “glycomacropeptide”, signaling a scientific emphasis on the biochemical aspects of PKU and the potential therapeutic implications of amino acids and specific proteins. This may reflect ongoing research into the metabolic pathways affected by PKU and the search for novel treatment modalities.
Cluster 3, marked in red, contains “foods”, “products”, and “sensory”, which could be indicative of research into the sensory characteristics and acceptance of low-phenylalanine foods and supplements, essential for patient adherence to a PKU diet. This acknowledges the importance of the product sensory profile in dietary compliance.
Notably, cluster 4 (orange) includes ‘life’ and ‘quality’, suggesting a broader scope of research focused on the quality of life of patients with PKU. This indicates a holistic research approach, considering not just the biochemical and nutritional aspects, but also the overall well-being of individuals living with this condition.
The distribution of keywords across the two principal dimensions highlights the multidisciplinary nature of PKU research, encompassing clinical management, biochemical treatment strategies, nutritional aspects, and the psychosocial impact on patients. The PCA graph serves as a visual synthesis of the complex research landscape, pinpointing the thematic concentrations and potential interconnections between various research domains in PKU and food science.
To delve deeper into the scientific trends on PKU-related foods, a thematic map was elaborated and reported in .
showcases a thematic map to elucidate the scientific landscape surrounding PKU-related food research. The map is segmented into four quadrants, each representing a different thematic focus: Basic Themes, Motor Themes, Emerging or Declining Themes, and Niche Themes. At the core, basic themes such as ‘phenylketonuria’ and ‘phenylalanine’ establish the fundamental research focus, indicating a sustained and substantial body of research. These terms are well-developed and central to the field, underpinning most of the studies analyzed. Motor Themes, located in the upper right quadrant, include “metabolism”, “body composition”, and “activated carbon”. These represent mature and well-developed areas of research that drive the field forward. The prominence of GMP as basic theme suggests that research is heavily geared toward exploring alternative protein sources, which are crucial for PKU management. Emerging or Declining Themes, situated in the lower left quadrant, contain terms like ‘sensory evaluation’ and ‘special low protein foods’. These themes are either gaining momentum as new areas of interest or losing prominence as the field evolves. The presence of ‘sensory evaluation’ indicates a nascent interest in the palatability and acceptance of PKU dietary interventions, which could have implications for adherence to dietary regimens. In fact, the sensory aspect of PKU management represents a burgeoning interest within the scientific community, particularly underscored by recent publications (Çoşkun and Yildiz Citation2022; Delsoglio et al. Citation2023; Li et al. Citation2024; C. Proserpio et al. Citation2019).
Lastly, Niche Themes in the upper left quadrant, such as ‘health-related quality of life’ and ‘parents’, reflect specialized, yet less developed areas of research within the field. These themes suggest that while there is an acknowledgment of the broader impacts of PKU on lifestyle and family dynamics, these areas are not yet as extensively researched as the biological or dietary aspects. In particular, it is interesting the presence of Check-all-that-apply (CATA) in this area, indicating that consumer science for PKU patients is an area still to be explored.
This thematic map highlights a multifaceted research domain, with a strong central focus on the biochemical understanding of PKU, evolving interest in the dietary and sensory aspects of food products for PKU, and a growing acknowledgement of the psychosocial elements of the disease. It signals a research community that is both grounded in established knowledge and actively seeking new horizons to improve the lives of individuals with PKU.
Food production for PKU subjects
Innovative dietary alternatives are essential to enhance adherence to a low-phenylalanine regimen and subsequent metabolic management in individuals diagnosed with PKU (van Calcar and Ney Citation2012). In PKU patients, the individual dietary tolerance to Phe is influenced by the genotype, phenotype, catabolic vs anabolic status, energy intake, dosage and distribution of Phe-free L-amino acid supplements, and target blood Phe concentration that, according to the European guidelines, varies with age (120–360 micromol/L before 12 years of age; 120–600 micromol/L > 12 years). Phe tolerance per kg of body weight is generally highest in early infancy (0–3 months of age) until 12 months (van Wegberg et al. Citation2017). After the age of 1 year, there is a slow and steady decline in tolerance per kg of body weight. Usually, Phe tolerance is <20 mg/kg/day (250–300 mg/day) in classic PKU, 20–25 mg/kg/day (350–400 mg/day) in moderate PKU and 25–50 mg/kg/day (400–600 mg/day) in mild PKU. However, in clinical practice, Phe tolerance of all patients should be reevaluated periodically, particularly at the times of rapid growth, changes in body composition or use of different treatment modalities.
Typically, fruits and vegetables with a phenylalanine content of under 50 mg per 100 grams are considered safe for unrestricted inclusion in the diets of patients (Weetch and Macdonald Citation2006; MacDonald et al. Citation2020). Therefore, the formulation of new food for PKU patients must take into account these restrictions.
Manufacturing food products that incorporate protein hydrolysates or alternatives involves the creation of items that supply a mix of carbohydrates, fats, vitamins, minerals, and proteins in various quantities. An overview of companies producing PKU food items for different developmental phases, including for infants and adults, is detailed in . In the compilation of the previously mentioned table, comprehensive web-based research was conducted, focusing on products recommended by various national agencies dedicated to the management of PKU, such as the National Society for Phenylketonuria (NSPKU, UK). It is important to note that during this research process, powdered protein supplements were deliberately excluded from consideration. This exclusion stems from a deliberate focus on exploring advancements within the realm of food technology, rather than the broader spectrum of nutritional supplements.
Table 1. PKU specified food supplements list obtained after web research.
The data provided showcases a broad spectrum of PKU-specific food products developed by leading companies in the field, highlighting significant advancements in food science and technology tailored for PKU management.
Relief Therapeutics (Geneva, Switzerland) offers PKU GOLIKE®, a medical food bar and granules with minimal phenylalanine content, utilizing a blend of fruit and prolonged-release amino acid mixtures, showcasing an innovative approach to combining taste with medical necessity. Danone Nutricia (Hoofddorp, The Netherlands)’s range, including Lophlex®, PhenylAde®, Easiphen, and GMPro LQ, spans juices, jams, powdered formulas, and drinks, all designed to be phenylalanine-free or low in phenylalanine, utilizing ingredients such as orange juice concentrate and whey protein isolate. These products are tailored for ease of use and palatability, ensuring PKU patients can adhere to their dietary restrictions without compromising on flavor or convenience.
Notably, the phenylalanine content varies significantly across these products, from zero to levels carefully calibrated to meet the dietary restrictions of PKU patients. The main ingredients underscore the innovation in food formulation, incorporating a variety of phenylalanine-free amino acid mixtures, specialized fats, and natural flavorings to enhance the sensory appeal. The production areas span globally, indicating a wide-reaching effort to address the nutritional needs of PKU patients worldwide. This diversity in product type, formulation, and geographical availability emphasizes the role of food science and technology in expanding the dietary options for PKU patients, moving beyond traditional dietary management to offer foods that cater to both the nutritional requirements and the sensory preferences of individuals with PKU, thereby potentially improving dietary adherence and quality of life (Soltanizadeh and Mirmoghtadaie Citation2014).
We could suppose that the especially designed food items for PKU individuals are developed through two main approaches:
Certain food and drink items are formulated to include all necessary nutritional supplements, excluding phenylalanine. These products come in various forms, such as ready-to-eat items, snack bars, and powder mixes intended for combination with liquids like water or juice.
Other items are specifically modified to reduce their phenylalanine levels either by breaking down the proteins they contain and separating the resulting fractions or by substituting them with proteins that have a low phenylalanine content (van Calcar and Ney Citation2012).
These modified food products provide a viable alternative to traditional foods that are either prohibited or severely restricted for PKU patients.
It is necessary to specify that PKU food products are intended for the specific dietary management of patients with PKU. They belong to a category of food products that are specially processed or formulated and presented for patients’ dietary management and used only under medical supervision. Such products are known as “food for medical special purposes” (FSMPs) in EU or “medical foods” (MF) in some non-EU countries (e.g., US and Argentina) and are subject to special regulations in addition to the general food legislation (Ruthsatz et al. Citation2022). Medical foods include metabolic formulas, also called medical formulas (liquids), as well as solid foods that are specially formulated and processed. The formulas are typically either powdered and reconstituted with water or juice, or they may be liquid products that are ready to consume (Stroup et al. Citation2017).
Glycomacropeptide (GMP) in PKU food production
Foods containing proteins and peptides free from phenylalanine or with low levels of it are crucial not only for providing essential amino acids (AAs) but also for offering the functional characteristics necessary to diversify food options (Pena et al. Citation2021). GMP, a peptide derived from whey protein that makes up 15% to 20% of its protein content and is composed of 64 AAs, is particularly effective for crafting phenylalanine-free AA-based formulas (Lammi et al. Citation2023). GMP is noteworthy for its very low content of aromatic amino acids like tyrosine, tryptophan, and Phe while containing higher amounts of threonine and isoleucine (Delsoglio et al. Citation2023; van Calcar and Ney Citation2012; Lammi et al. Citation2023)
The consistent research into GMP and other protein substitutes highlights a trend toward exploring alternative protein sources that cater to the unique dietary needs of individuals with PKU.
The study by Delsoglio et al. (Citation2023) investigated a new formulation of protein substitute based on GMP for individuals with PKU and tyrosinemia (TYR). The new GMP formulation was generally well-tolerated. Most participants did not report new or worsening gastrointestinal symptoms while using the product. Most significantly, the introduction of the new GMP-based protein substitute did not negatively impact the metabolic control of participants. Blood Phe levels remained stable or improved in most patients during the study period. This stability is critical as maintaining Phe levels within the target range is essential for preventing cognitive impairment in individuals with PKU and TYR (Pena et al. Citation2018).
The study by Lim et al. (Citation2007) explored the development of a range of GMP-based food items, such as sports drinks, chocolate beverages, puddings, fruit leathers, and snack crackers, showcasing the possibility of crafting savory products with minimal phenylalanine content appropriate for a PKU diet. The inherent properties of GMP, including its solubility in acidic environments, its capacity to generate foams or gels, and its heat stability, make it particularly well-suited for creating semi-solid food items and drinks. For example, the use of GMP in chocolate beverages not only amplifies the chocolate flavor but also effectively conceals the dairy-like taste of GMP, illustrating its versatility (Lim et al. Citation2007; van Calcar and Ney Citation2012; Rondanelli et al. Citation2023). Further applications of GMP have led to the production of a diverse array of food products, such as puddings, snack bars, puffed cereals, beverages, crackers, and salad dressings, highlighting its broad utility in dietary management for PKU (van Calcar and Ney Citation2012). Moreover, there is clear evidence that GMP formulations are more acceptable than traditional amino acid mixtures (Proserpio et al. Citation2018).
Over the years it has been hypothesized that GMP can significantly influence and enhance satiety (Chun Lam et al. Citation2009) thereby reducing energy intake and moderating weight gain. However, despite the initial hypothesis, many studies found no observable difference in satiety effects or weight management between the AA and GMP-AA groups (Daly et al. Citation2020; Chungchunlam et al. Citation2014). Daly et al. (Citation2020) research underscores the complexity of dietary management in PKU, pointing out that while all macronutrients possess unique physiological properties that influence metabolic pathways, the specific role of GMP in controlling appetite and preventing overweight and obesity in PKU requires further investigation.
To create GMP-based dietary products for specialized medical foods, it is essential to utilize a molecule that is highly purified, with phenylalanine content of less than 2.0 mg per gram of protein, and enhanced with additional amino acids such as arginine, histidine, leucine, tyrosine, and tryptophan (Ney, Blank, and Hansen Citation2014).
Ajinomoto Cambrooke manufactures a variety of medical food formulas containing a patented GMP formulation called Glytactin designed to help meet the specialized nutritional needs of patients with therapeutic or chronic medical needs (Sizer et al. Citation2015). Glytactin is the world’s first PKU protein formula that is made from a natural whole protein derived from GMP (Stroup et al. Citation2017). The inherent lack of Phe in this protein renders it perfectly suited for the PKU diet. Introduced as Glytactin, this innovative formula merges GMP with essential amino acids such as arginine and tyrosine, which GMP does not naturally contain. Before Glytactin’s market launch in 2010, PKU nutritional solutions exclusively comprised synthetic, artificially created free amino acid proteins. Research indicates that natural intact proteins like GMP are absorbed more slowly by the body and utilized more efficiently compared to single amino acids, offering a more natural simulation of whole protein intake (Stroup et al. Citation2017). The ability to mix the new amino acid substitute into various foods (e.g., pasta sauce, stir fry, smoothies) could allow for a more flexible approach to meeting dietary requirements (Newby Citation2023). This adaptability is particularly beneficial for addressing food neophobia, as it allows the protein substitute to be incorporated into familiar and favored foods.
Despite the potential benefits of GMP, evidence suggests that the Phe content in GMP can still influence blood Phe levels, particularly when it is used as the sole source of protein substitute. This impact is significant because even small amounts of Phe in the diet of PKU patients can lead to fluctuations in blood Phe levels, which must be carefully monitored and controlled (A. Daly et al. Citation2019).
Innovative PKU food ingredients procedures
The prevalent method for creating PKU-friendly foods involves the hydrolysis and subsequent separation of the obtained products or removal of proteins and the addition of artificial nutrients. The Fischer ratio, which is the ratio of branched-chain amino acids (BCAA) to aromatic amino acids (AAA), plays a pivotal role in determining a hydrolysate’s suitability for PKU food formulations (Wang et al. Citation2024). Hydrolysates with a high Fischer ratio are often selected for PKU management due to their superior absorption in the gastrointestinal tract compared to free amino acids and their relatively minor impact on osmotic pressure. These protein hydrolysates, known for their optimal nutritional and functional properties, are produced through a process that includes the selective enzymatic release of AAA followed by the removal of these free AAAs through techniques such as gel filtration and adsorption onto resins or activated carbon (Su, Wang, McClements, et al. Citation2021; Zhang et al. Citation2024).
However, this method is fraught with several drawbacks, such as escalated costs, deterioration in the quality of food due to the hydrolysis process, limitations on the variety of foods that can be used, a decrease in shelf life, increased susceptibility to spoilage, and, notably, deterioration in taste (Soltanizadeh and Mirmoghtadaie Citation2014). To improve taste, producers add large quantities of sweeteners, complicating dietary management for PKU patients (Chattopadhyay, Raychaudhuri, and Chakraborty Citation2014).
In the evolving landscape of low Phe procedures, recent advancements underscore the significance of innovative adsorption techniques in the refinement of low Phe ingredients. Among these, activated carbon adsorption emerges as a particularly promising method, offering a nuanced balance between efficacy and nutrient preservation (Lima, Oliveira, and Franca Citation2022). This technique capitalizes on the molecular interactions between the benzene rings of Phe and the graphene structures of activated carbon, alongside hydrogen bonding with oxygen-containing groups on the carbon surface (Zhang et al. Citation2024). Such interactions not only facilitate high rates of Phe removal, ranging from 91.71% to 97.78% as demonstrated by Su, Wang, Lu, et al. (Citation2021a), but also ensure the retention of essential low molecular weight nutrients, crucial for the nutritional integrity of the processed food products. The optimization of this process, influenced by variables such as particle size, pH, and adsorption duration, suggests a targeted approach that can enhance the adsorptive capacity, thereby refining the quality of low Phe ingredients (Lima, Oliveira, and Franca Citation2022). Furthermore, the application of whey protein concentrate (WPC) within this context, recognized for its comprehensive nutritional profile, including an abundance of essential amino acids like cysteine, points toward a holistic approach in the development of semi-medicated dietary solutions (Zhang et al. Citation2024). Such innovations are not merely technical achievements but represent a stride toward improving the quality of life for individuals requiring stringent dietary restrictions, by offering more palatable and nutritionally balanced low Phe options. Very recently a study by Zhang et al. (Citation2024) reported the development and efficacy of a novel two-step enzymatic hydrolysis process followed by activated carbon adsorption for the removal of Phe from WPC, aimed at producing low Phe protein hydrolysates (LPHs). This innovative approach achieved a substantial reduction in Phe content, with enzymatic hydrolysis resulting in a 90.8% free rate of Phe, and subsequent activated carbon adsorption further reducing Phe levels to 89.79% at a concentration of 2.19 mg/g protein equivalent. The resultant LPHs are characterized by their low Phe content while retaining essential amino acids, making them suitable for inclusion in dietary plans for individuals with PKU. Importantly, the study also notes that the flavor profile of the LPHs remained unaffected, with no significant flavor alterations aside from the type of acid, ensuring the palatability of foods prepared using these hydrolysates.
The research of Bu et al. (Citation2020) studied a two-step enzymatic hydrolysis of whey protein followed by adsorption with macroporous resin. The enzymatic combination of thermolysin or pancreatin with Protease A2SD achieved hydrolysis degrees between 42.62% and 45.43%, releasing over 90% of Phe. The D101 macroporous resin column proved significantly more effective than activated carbon in Phe removal, eliminating 98.38% of Phe from the hydrolysate, resulting in an LPH with a protein content of 60.41 g/100 g hydrolysate and a Phe level of 1.38 ± 0.11 mg/g protein equivalent.
Another innovation is reported by Ghalamboran, Kohnavard, and Hassani (Citation2023). The authors demonstrated the effectiveness of chitosan nanoparticles in reducing phenylalanine while maximizing phenylalanine ammonia-lyase (PAL) activity in rice kernels. Spraying rice kernels with chitosan nanoparticles at various concentrations (0, 500, 1000, 1500 µl L−1) effectively decreased the phenylalanine and total protein levels. The potential of chitosan nanoparticles to provide a dietary solution for PKU patients by reducing phenylalanine levels in food crops is promising, yet additional research is required to fully understand and optimize this innovative approach.
Impact of food science on PKU management
A therapeutic approach for managing PKU involves dietary modification, incorporating processed, semi-medicated foods designed to mitigate the elevation and accumulation of Phe in bodily fluids. This method aims to regulate the metabolic imbalance characteristic of PKU by controlling the intake of specific amino acids, thereby preventing the harmful build-up of Phe, which is pivotal for the health and well-being of individuals afflicted with this condition (Okano et al. Citation2016). There’s a clear indication that the PKU-research field is moving toward more specialized and user-friendly dietary solutions for PKU, with a focus on both the nutritional content and the sensory attributes of food products (Cassidy et al. Citation2023).
At present, the predominant approach employed by food producers for individuals diagnosed with PKU involves the hydrolysis of food substances, subsequently amalgamating these hydrolyzed components with artificially synthesized nutrients, including various vitamins and essential nutrients (Soltanizadeh and Mirmoghtadaie Citation2014; Newby Citation2023). This methodology, however, is not without its drawbacks. It is characterized by several limitations, including elevated costs of the final products, a decrease in food quality due to the hydrolysis procedure, constraints concerning the diversity of food types that can be utilized, a decreased shelf life, increased susceptibility to spoilage, and, most notably, a degradation in taste quality of the food items. As a corrective measure to counteract the adverse taste profiles of these processed food items, manufacturers are compelled to incorporate substantial quantities of sweeteners (Ghalamboran, Kohnavard, and Hassani Citation2023). This practice, however, introduces additional complications for PKU patients, who must navigate these dietary challenges in conjunction with their condition.
This study of Çoşkun and Yildiz (Citation2022) focused on developing a yoghurt substitute for PKU patients, utilizing a commercial low-protein milk substitute, xanthan gum, commercial yoghurt gelling agent, starch, and pectin. The fermentation of these yoghurt substitutes was completed within 5 h. The study found that the yoghurt substitutes with added corn starch and pectin had higher total solid matter and negligible syneresis values, indicating less whey separation. The study concluded that the yoghurt substitutes developed could be a valuable addition to the dietary options for PKU patients, with protein and phenylalanine levels within the safe consumption limits for this group.
Goldar, Givianrad, and Shams (Citation2016) provide insights into the utilization of a fermentation process for the production of a new yoghurt low in Phe suitable for individuals with PKU. This low Phe yoghurt could serve as a model for creating other fermented dairy or nondairy products that meet the dietary restrictions of PKU without compromising on taste or nutritional value. The utilization of transglutaminase (TGase) for cross-linking milk proteins in yoghurt production suggests a method to enhance the textural properties of PKU-friendly foods.
The focus on acceptability and amino acids suggests an interdisciplinary approach, combining nutritional science, food technology, and sensory analysis, to develop effective and appealing dietary interventions for PKU
Applications of 3D food printing for PKU food
In the topic of personalized nutrition and dietary management, 3D food printing (3DFP) emerges as a groundbreaking technology with the potential to revolutionize diet formulation for various health conditions, including PKU (Escalante‐Aburto et al. Citation2021). 3D printing, also known as additive manufacturing, is a type of digital fabrication technique for the manufacture of physical objects from a geometrical model through the addition of materials. In the food sector, the common 3D printing techniques include extrusion-based printing, binder jetting, inkjet printing and selective laser sintering printing (Agunbiade et al. Citation2022). Extrusion-based 3D printing is considered as the most widely applied 3D printing technology for food. It involves the deposition of the food material, commonly referred to as “food ink”, from a nozzle layer-by-layer. As illustrated in , the primary components of an extrusion-based 3D printer include a cartridge equipped with a piston to push the food ink through an extrusion tube and out of a nozzle. Typically three extrusion mechanism can be applied: syringe-type extrusion, screw-type extrusion and air pressure-driven extrusion. The nozzle moves along multiple axes, controlled by the machine’s frame structure to accurately dispense the food substrate onto the printer bed. The process is similar to traditional 3D printing but adapted for food-safe materials. Each layer is added to build up the final product. Intricate designs and patterns can be sent to the printer through a PC connection port or an SD card.
The technology’s success in creating customized foods for individuals with dysphagia (Hemsley et al. Citation2015; Dong et al. Citation2019), and digestive disorders (Liu, Bhandari, and Zhang Citation2020) by tailoring textures and consistencies, parallels the precision required in managing PKU diets, where control over phenylalanine levels is critical. Furthermore, 3DFP’s application in producing nutrient-dense foods for children and incorporating pre- and probiotics for digestive health underscores its capability to enhance the nutritional profile of diets tailored for pediatric populations (Carou-Senra et al. Citation2023). By enabling the incorporation of PKU-friendly nutrients and the exploration of alternative protein sources with minimal phenylalanine content, 3DFP holds promise in diversifying and enriching the PKU diet beyond traditional limitations. This technology not only addresses the need for strict dietary adherence but also opens avenues for improving the palatability and nutritional adequacy of foods designed for PKU management, illustrating a significant step forward in the application of personalized nutrition solutions for specialized dietary needs.
The study by Ko et al. (Citation2021) presents an innovative method utilizing a coaxial nozzle-assisted 3D food printer to enhance the texture of meat analogues through the insertion of artificial muscle fibers made from cross-linkable hydrocolloids. This technology, leveraging the properties of kappa and iota carrageenan (CG), sodium alginate (SA), and glucomannan (GM), achieved to simulate the elasticity and hardness of meat, offering a new pathway for creating sustainable, plant-based protein sources. In the context of PKU dietary management, this 3DFP technology opens up new possibilities. By adjusting the hydrocolloid formulations, PKU-specific food products with tailored textures and nutritional profiles could be developed. For example, protein sources for PKU patients often lack variety and sensory appeal (Proserpio et al. Citation2018); utilizing this method, meat analogues could be designed to not only meet dietary restrictions but also enhance the eating experience through improved taste and texture.
Another emerging technology linked to 3DFP is the Selective Sintering Extrusion (SSE). SSE technology has been used to create personalized pharmaceutical forms for pediatric patients, addressing challenges such as accurate dosing, palatability, and ease of administration (Carou-Senra et al. Citation2023). SSE can be applied for the development of orodispersible films and chewable tablets for pediatric patients (Eduardo, Ana, and José B Citation2021). This indicates a path for creating PKU foods that are easier for children to consume, who may find traditional dietary management challenging or unappealing. To give more insights and a critical point of view of 3DFP, reports the advantages and disadvantages of this technology for PKU food production.
Table 2. Advantages and disadvantages of 3D food printing for PKU food production and formulations.
This technology could facilitate the creation of a wide array of appealing, low-phenylalanine foods, from meat analogues to snacks and desserts, thereby improving dietary adherence and quality of life for individuals with PKU.
A prospective study could explore the implementation of 3DFP in PKU management, where a group of PKU patients receives customized 3D-printed meals tailored to their individual nutritional needs and preferences. This study could assess the impact of these meals on dietary adherence, nutritional status, phenylalanine levels, and overall patient satisfaction compared to a control group receiving traditional PKU diets. Such a study would provide valuable data on the feasibility, benefits, and challenges of integrating 3DFP into dietary management for PKU, offering insights into future applications and developments in this area.
Sensory evaluation and consumer acceptance of PKU subjects
The study by Delsoglio et al. (Citation2023) highlighted that the protein substitute’s palatability plays a significant role in adherence, particularly as patients age and become more responsible for their own dietary choices. With adolescence known as a period where adherence to dietary restrictions tends to wane, the introduction of more palatable treatment options could have a substantial impact on long-term health outcomes for individuals with PKU. The research suggested that the protein substitutes with improved sensory attributes could contribute to better adherence to dietary management, reflecting the potential for such innovations to ease the dietary challenges and improve the quality of life for patients with PKU and other metabolic disease.
Li et al. (Citation2024) conducted a sensory analysis of biscuits made with whey protein hydrolysates (W-LPHs), creating a suitable low Phe option for PKU management. The study determined that the optimal addition of W-LPHs in the biscuit formulation was 15%, which resulted in biscuits containing 0.25 g/100 g of Phe and a protein content of 9.10 g/100 g. This higher acceptance of 15% W-LPH biscuits is attributed to the beneficial effects of W-LPHs on dough fermentation, resulting in an enhanced color and overall appeal of the biscuits.
The research of Proserpio et al. (Citation2019) aimed to assess the acceptability of protein substitutes for individuals with PKU in different regions of Italy, comparing different formulas, both the participants from Milan and Naples showed a higher liking for GMP samples over the traditional amino acid formulas. This preference was consistent across the regions, despite noted dietary pattern differences in Italy. Moreover, the preference for GMP flavored beverages was consistent with previous studies and confirmed the importance of flavor in the acceptance of protein substitutes (Lim et al. Citation2007).
Additionally, another significant contribution on GMP alternative food was reported by the same authors (Proserpio et al. Citation2018). In that study, the CATA assessment revealed significant differences in the sensory attributes associated with each sample, demonstrating that participants could discern distinct sensory characteristics between the samples. GMP beverages were associated with more favorable sensory attributes, such as sweet taste and natural odor, while L-amino acid formulas were described with less preferred attributes like bitter taste and artificial odor.
In the study of Goldar, Givianrad, and Shams (Citation2016), the sensory evaluation of the developed yoghurts, particularly those with higher acceptability scores, underscores the importance of taste, texture, and overall enjoyment in dietary management. Another recent study evaluated the formulation of yoghurt substitutes developed for PKU subjects (Çoşkun and Yildiz Citation2022). The authors found that the samples containing vegetable-based gelling agent (carob powder and calcium lactate) received the highest overall acceptance in terms of sensory attributes such as color, appearance, taste, odor, texture, consistency in mouth, consistency on spoon, and general acceptability. This suggests that the commercial gelling agent significantly improves the sensory properties of low-protein yoghurt substitutes, making it the most preferred option among the alternatives tested. The study also noted the potential improvement in taste and general acceptability with the addition of sweeteners to the yoghurt substitutes. This suggests a direction for future product development, where the inclusion of PKU-friendly sweeteners could enhance the palatability of food substitutes, making them more appealing, especially to children with PKU who tend to have a preference for sweeter foods (Ghalamboran, Kohnavard, and Hassani Citation2023).
Bu et al. (Citation2020) studied the sensory aspects of an LPH. The authors found that after adsorption, there was a notable increase in peptides below 600 Da which led to heightened bitterness and astringency as measured by the electronic tongue. The process also reduced aroma-active compounds associated with the Millard reaction and lipid oxidation, altering the flavor profile of the LPH to have fewer off-odors. Despite these sensory changes, the study suggests that LPH could serve as a nutritional substitute for PKU patients. However, further sensory evaluation by trained panels on the LPH is required.
The sensory results underscore the importance of optimizing the formulation of food substitutes for PKU patients to ensure they are not only safe and nutritious but also enjoyable to consume, thereby encouraging adherence to dietary restrictions necessary for managing the condition.
Conclusion
The integration of food science and technology in managing PKU has marked a significant leap forward, offering more than just dietary restrictions to patients. By focusing on the development of phenylalanine-free and low-phenylalanine foods that do not compromise on sensory qualities, this review highlights the increasing importance of palatability and consumer preferences in dietary adherence. Innovations like 3D food printing promise personalized nutrition, ensuring that PKU management is not only effective but also enjoyable. This convergence of technology and sensory science paves the way for a future where dietary management aligns with quality-of-life improvements, signaling a transformative period in PKU care.
Disclosure statement
No potential conflict of interest was reported by the author(s).
Data availability statement
The data presented in this study are available on request from the corresponding author.
Additional information
Funding
References
- Agunbiade, A. O., L. Song, O. J. Agunbiade, C. E. Ofoedu, J. S. Chacha, H. T. Duguma, S. M. Hossaini, W. A. Rasaq, I. Shorstkii, C. M. Osuji, et al. 2022. Potentials of 3D extrusion-based printing in resolving food processing challenges: A perspective review. Journal of Food Process Engineering 45 (4):e13996. doi: 10.1111/jfpe.13996.
- Aria, M., and C. Cuccurullo. 2017. Bibliometrix: An R-tool for comprehensive science mapping analysis. Journal of Informetrics 11 (4):959–75. doi: 10.1016/j.joi.2017.08.007.
- Bu, T., M. Zhou, J. Zheng, P. Yang, H. Song, S. Li, and J. Wu. 2020. Preparation and characterization of a low-phenylalanine whey hydrolysate using two-step enzymatic hydrolysis and macroporous resin adsorption. LWT 132:109753. doi: 10.1016/j.lwt.2020.109753.
- Callon, M., J. P. Courtial, and F. Laville. 1991. Co-word analysis as a tool for describing the network of interactions between basic and technological research: The case of polymer chemsitry. Scientometrics 22 (1):155–205. doi: 10.1007/BF02019280.
- Carou-Senra, P., L. Rodríguez-Pombo, E. Monteagudo-Vilavedra, A. Awad, C. Alvarez-Lorenzo, A. W. Basit, A. Goyanes, and M. L. Couce. 2023. 3D printing of dietary products for the management of inborn errors of intermediary metabolism in pediatric populations. Nutrients 16 (1):61. doi: 10.3390/nu16010061.
- Cassidy, S., S. Evans, A. Pinto, A. Daly, C. Ashmore, S. Ford, S. Buckley, and A. MacDonald. 2023. Parent’s perception of the types of support given to families with an infant with phenylketonuria. Nutrients 15 (10):2328. doi: 10.3390/nu15102328.
- Chattopadhyay, S., U. Raychaudhuri, and R. Chakraborty. 2014. Artificial sweeteners - A review. Journal of Food Science and Technology 51 (4):611–21. doi: 10.1007/s13197-011-0571-1.
- Chun Lam, C., S. P. J. Moughan, A. Awati, and H. R. Morton. 2009. The influence of whey protein and glycomacropeptide on satiety in adult humans. Physiology & Behavior 96 (1):162–8. doi: 10.1016/j.physbeh.2008.09.022.
- Chungchunlam, S. M. S., S. J. Henare, S. Ganesh, and P. J. Moughan. 2014. Effect of whey protein and glycomacropeptide on measures of satiety in normal-weight adult women. Appetite 78:172–8. doi: 10.1016/j.appet.2014.03.027.
- Çoşkun, F., and G. Yildiz. 2022. Determination of physicochemical, rheological, microbiological and sensory properties of low protein yoghurt substitutes produced for PKU (Phenylketonuria) patients. Tarım Bilimleri Dergisi 29 (4):973–82. doi: 10.15832/ankutbd.892513.
- Daly, A., S. Evans, A. Pinto, R. Jackson, C. Ashmore, J. C. Rocha, and A. Macdonald. 2020. The impact of the use of glycomacropeptide on satiety and dietary intake in phenylketonuria. Nutrients 12 (9):2704. doi: 10.3390/nu12092704.
- Daly, A., S. Evans, S. Chahal, S. Santra, A. Pinto, R. Jackson, C. Gingell, J. Rocha, F. J. Van Spronsen, and A. MacDonald. 2019. Glycomacropeptide: Long-term use and impact on blood phenylalanine, growth and nutritional status in children with PKU. Orphanet Journal of Rare Diseases 14 (1):44. doi: 10.1186/s13023-019-1011-y.
- Delsoglio, M., R. Capener, A. MacDonald, A. Daly, C. Ashmore, S. Donald, L. Gaff, L. VanDorp, R. Skeath, C. Ellerton, et al. 2023. Evaluation of a new ‘mix-in’ style glycomacropeptide-based protein substitute for food and drinks in patients with phenylketonuria and tyrosinemia. Nutrients 15 (16):3598. doi: 10.3390/nu15163598.
- Dong, X., Y. Huang, Y. Pan, K. Wang, S. Prakash, and B. Zhu. 2019. Investigation of sweet potato starch as a structural enhancer for three-dimensional printing of scomberomorus Niphonius Surimi. Journal of Texture Studies 50 (4):316–24. doi: 10.1111/jtxs.12398.
- Donthu, N., S. Kumar, D. Mukherjee, N. Pandey, and W. M. Lim. 2021. How to conduct a bibliometric analysis: An overview and guidelines. Journal of Business Research 133:285–96. doi: 10.1016/j.jbusres.2021.04.070.
- Echchakoui, S. 2020. Why and how to merge scopus and web of science during bibliometric analysis: The case of sales force literature from 1912 to 2019. Journal of Marketing Analytics 8 (3):165–84. doi: 10.1057/s41270-020-00081-9.
- Eck, N. J. v., and L. Waltman. 2020. VOSviewer manual version 1.6.16. Univeristeit Leiden (The Netherlands).
- Eduardo, D. T., S. E. Ana, and F. José B. 2021. A micro-extrusion 3D printing platform for fabrication of orodispersible printlets for pediatric use. International Journal of Pharmaceutics 605:120854. doi: 10.1016/j.ijpharm.2021.120854.
- Escalante‐Aburto, A., G. Trujillo‐de Santiago, M. M. Álvarez, and C. Chuck‐Hernández. 2021. Advances and prospective applications of 3D food printing for health improvement and personalized nutrition. Comprehensive Reviews in Food Science and Food Safety 20 (6):5722–41. doi: 10.1111/1541-4337.12849.
- Ghalamboran, M. R., A. Kohnavard, and S. B. Hassani. 2023. Phenylalanine response in rice kernel under chitosan nanoparticles spraying. Acta Physiologiae Plantarum 45 (4):61. doi: 10.1007/s11738-023-03538-3.
- Goldar, P., M. H. Givianrad, and A. Shams. 2016. Effect of ultrafiltered milk permeate and non-dairy creamer powder concentration on low phenylalanine yoghurt’s physicochemical properties during storage. Journal of Food Science and Technology 53 (7):3053–9. doi: 10.1007/s13197-016-2278-9.
- Groselj, U., M. Z. Tansek, J. Kovac, T. Hovnik, K. T. Podkrajsek, and T. Battelino. 2012. Five novel mutations and two large deletions in a population analysis of the phenylalanine hydroxylase gene. Molecular Genetics and Metabolism 106 (2):142–8. doi: 10.1016/j.ymgme.2012.03.015.
- Hemsley, B., S. Balandin, J. J. Sheppard, A. Georgiou, and S. Hill. 2015. A call for dysphagia-related safety incident research in people with developmental disabilities. Journal of Intellectual and Developmental Disability 40 (1):99–103. doi: 10.3109/13668250.2014.994172.
- Ko, H. J., Y. Wen, J. H. Choi, B. R. Park, H. W. Kim, and H. J. Park. 2021. Meat analog production through artificial muscle fiber insertion using coaxial nozzle-assisted three-dimensional food printing. Food Hydrocolloids 120:106898. doi: 10.1016/j.foodhyd.2021.106898.
- Lammi, C., C. Bollati, L. Fiori, J. Li, M. Fanzaga, L. d’Adduzio, M. Tosi, A. Burlina, G. Zuccotti, and E. Verduci. 2023. Glycomacropeptide (GMP) rescued the oxidative and inflammatory activity of free L-AAs in human Caco-2 cells: New insights that support GMP as a valid and health-promoting product for the dietary management of phenylketonuria (PKU) patients. Food Research International (Ottawa, ON) 173 (Pt 1):113258. doi: 10.1016/j.foodres.2023.113258.
- Li, H., S. Zhang, H. Chen, L. Yang, J. Li, X. Liu, Z. Luo, H. Li, and J. Yu. 2024. Preparation and characterisation of low phenylalanine biscuits with whey protein hydrolysates. International Journal of Dairy Technology 77 (1):262–8. doi: 10.1111/1471-0307.13028.
- Lim, K., S. C. van Calcar, K. L. Nelson, S. T. Gleason, and D. M. Ney. 2007. Acceptable low-phenylalanine foods and beverages can be made with glycomacropeptide from cheese whey for individuals with PKU. Molecular Genetics and Metabolism 92 (1–2):176–8. doi: 10.1016/j.ymgme.2007.06.004.
- Lima, W. C., L. S. Oliveira, and A. S. Franca. 2022. Adsorption of phenylalanine from aqueous solutions using activated carbon from sunflower meal functionalized with sulfonic groups. Foods (Basel, Switzerland) 11 (21):3427. doi: 10.3390/foods11213427.
- Liu, Z., B. Bhandari, and M. Zhang. 2020. Incorporation of probiotics (Bifidobacterium Animalis Subsp. Lactis) into 3D printed mashed potatoes: Effects of variables on the viability. Food Research International (Ottawa, ON) 128:108795. doi: 10.1016/j.foodres.2019.108795.
- MacDonald, A., A. M. J. van Wegberg, K. Ahring, S. Beblo, A. Bélanger-Quintana, A. Burlina, J. Campistol, T. Coşkun, F. Feillet, M. Giżewska, et al. 2020. PKU dietary handbook to accompany PKU guidelines. Orphanet Journal of Rare Diseases 15 (1):230. doi: 10.1186/s13023-020-01391-y.
- Mahan, K. C., M. A. Gandhi, and S. Anand. 2019. Pegvaliase: A novel treatment option for adults with phenylketonuria. Current Medical Research and Opinion 35 (4):647–51. doi: 10.1080/03007995.2018.1528215.
- Maler, V., V. Goetz, M. Tardieu, A. El Khalil, J. M. Alili, P. Meunier, F. Maillot, and F. Labarthe. 2023. Aspartame and phenylketonuria: An analysis of the daily phenylalanine intake of aspartame-containing drugs marketed in France. Orphanet Journal of Rare Diseases 18 (1):142. doi: 10.1186/s13023-023-02770-x.
- Mitchell, J. J., Y. J. Trakadis, and C. R. Scriver. 2011. Phenylalanine hydroxylase deficiency. Genetics in Medicine: Official Journal of the American College of Medical Genetics 13 (8):697–707. doi: 10.1097/GIM.0b013e3182141b48.
- Newby, C. 2023. Introducing a granule based protein substitute to the diet of a child with phenylketonuria to address reluctance to ingest phenylalanine-free protein substitute: A case report. Nutrition and Health 30 (1):35–8. doi: 10.1177/02601060231184934.
- Ney, D. M., R. D. Blank, and K. E. Hansen. 2014. Advances in the nutritional and pharmacological management of phenylketonuria. Current Opinion in Clinical Nutrition and Metabolic Care 17 (1):61–8. doi: 10.1097/MCO.0000000000000002.
- Okano, Y., T. Hattori, H. Fujimoto, K. Noi, M. Okamoto, T. Watanabe, R. Watanabe, R. Fujii, and T. Tamaoki. 2016. Nutritional status of patients with phenylketonuria in Japan. Molecular Genetics and Metabolism Reports 8:103–10. doi: 10.1016/j.ymgmr.2016.08.005.
- Pena, M. J., A. Pinto, A. Daly, A. Macdonald, L. Azevedo, J. C. Rocha, and N. Borges. 2018. The use of glycomacropeptide in patients with phenylketonuria: A systematic review and meta-analysis. Nutrients 10 (11):1794. doi: 10.3390/nu10111794.
- Pena, M. J., A. Pinto, M. F. de Almeida, C. de Sousa Barbosa, P. C. Ramos, S. Rocha, A. Guimas, R. Ribeiro, E. Martins, A. Bandeira, et al. 2021. Continuous use of glycomacropeptide in the nutritional management of patients with phenylketonuria: A clinical perspective. Orphanet Journal of Rare Diseases 16 (1):84. doi: 10.1186/s13023-021-01721-8.
- Pilotto, A., C. M. Zipser, E. Leks, D. Haas, G. Gramer, P. Freisinger, E. Schaeffer, I. Liepelt-Scarfone, K. Brockmann, W. Maetzler, et al. 2021. Phenylalanine effects on brain function in adult phenylketonuria. Neurology 96 (3):e399–e411. doi: 10.1212/WNL.0000000000011088.
- Poole, G., A. Pinto, S. Evans, S. Ford, M. O’Driscoll, S. Buckley, C. Ashmore, A. Daly, and A. MacDonald. 2022. Hungry for change: The experiences of people with PKU, and their caregivers, when eating out. Nutrients 14 (3):626. doi: 10.3390/nu14030626.
- Proserpio, C., E. Pagliarini, J. Zuvadelli, S. Paci, A. Re Dionigi, G. Banderali, C. Cattaneo, and E. Verduci. 2018. Exploring drivers of liking of low-phenylalanine products in subjects with phenyilketonuria using check-all-that-apply method. Nutrients 10 (9):1179. doi: 10.3390/nu10091179.
- Proserpio, C., E. Verduci, I. Scala, P. Strisciuglio, J. Zuvadelli, and E. Pagliarini. 2019. Liking and sensory description of protein substitutes in phenylketonuria subjects: A case-study in Northern and Southern Italy. Italian Journal of Food Science 31 (4):705–15. doi: 10.14674/IJFS-1519.
- Rondanelli, M., F. Porta, C. Gasparri, G. C. Barrile, A. Cavioni, F. Mansueto, G. Mazzola, Z. Patelli, G. Peroni, M. Pirola, et al. 2023. A food pyramid for adult patients with phenylketonuria and a systematic review on the current evidences regarding the optimal dietary treatment of adult patients with PKU. Clinical Nutrition (Edinburgh, Scotland) 42 (5):732–63. doi: 10.1016/j.clnu.2023.03.007.
- Russo, G. L., A. L. Langellotti, E. Torrieri, and P. Masi. 2024. Emerging technologies in seafood processing: An overview of innovations reshaping the aquatic food industry. Comprehensive Reviews in Food Science and Food Safety 23 (1):e13281. doi: 10.1111/1541-4337.13281.
- Ruthsatz, M., J. Chen, C. Wu, and T. Morck. 2022. Foods for special medical purposes/medical foods: A global regulatory synopsis. Regulatory Focus. https://www.raps.org/news-and-articles/news-articles/2022/8/foods-for-special-medical-purposesmedical-foods-a.
- Sizer, C., Alea, L., and Olson, K. 2015. Liquid nutritional formula for phenylketonuria patients (US 2015/0216213 A1). In United states patent application (US 2015/0216213 A1).
- Soltanizadeh, N., and L. Mirmoghtadaie. 2014. Strategies used in production of phenylalanine-free foods for PKU management. Comprehensive Reviews in Food Science and Food Safety 13 (3):287–99. doi: 10.1111/1541-4337.12057.
- Stroup, B. M., D. M. Ney, S. G. Murali, F. Rohr, S. T. Gleason, S. C. Van Calcar, and H. L. Levy. 2017. Metabolomic insights into the nutritional status of adults and adolescents with phenylketonuria consuming a low-phenylalanine diet in combination with amino acid and glycomacropeptide medical foods. Journal of Nutrition and Metabolism 2017:6859820–17. doi: 10.1155/2017/6859820.
- Su, Y., Y. Wang, C. Lu, L. Gu, C. Chang, J. Li, D. J. McClements, and Y. Yang. 2021. Removal of phenylalanine from egg white powder: Two-step enzymatic method combined with activated carbon adsorption. Process Biochemistry 104:101–9. doi: 10.1016/j.procbio.2021.03.004.
- Su, Y., Y. Wang, D. J. McClements, C. Lu, C. Chang, J. Li, L. Gu, and Y. Yang. 2021. Selective adsorption of egg white hydrolysates onto activated carbon: Establishment of physicochemical mechanisms for removing phenylalanine. Food Chemistry 364:130285. doi: 10.1016/j.foodchem.2021.130285.
- Teruya, K. I., E. Remor, and I. V. D. Schwartz. 2020. Development of an inventory to assess perceived barriers related to PKU treatment. Journal of Patient-Reported Outcomes 4 (1):29. doi: 10.1186/s41687-020-00194-w.
- Upadia, J., K. Crivelly, G. Noh, A. Cunningham, C. Cerminaro, Y. Li, M. Mckoin, M. Chenevert, and H. C. Andersson. 2024. Maximal dietary responsiveness after tetrahydrobiopterin (BH4) in 19 phenylalanine hydroxylase deficiency patients: What super-responders can expect. Molecular Genetics and Metabolism Reports 38 (January):101050. doi: 10.1016/j.ymgmr.2024.101050.
- van Calcar, S. C., and D. M. Ney. 2012. Food products made with glycomacropeptide, a low-phenylalanine whey protein, provide a new alternative to amino acid-based medical foods for nutrition management of phenylketonuria. Journal of the Academy of Nutrition and Dietetics 112 (8):1201–10. doi: 10.1016/j.jand.2012.05.004.
- van Wegberg, A. M. J., A. MacDonald, K. Ahring, A. Bélanger-Quintana, N. Blau, A. M. Bosch, A. Burlina, J. Campistol, F. Feillet, M. Giżewska, et al. 2017. The complete European guidelines on phenylketonuria: Diagnosis and treatment. Orphanet Journal of Rare Diseases 12 (1):162. doi: 10.1186/s13023-017-0685-2.
- Wang, Z., X. Zhang, L. Wang, X. Ou, and J. Huang. 2024. High Fischer ratio oligopeptides in food: Sources, functions and application prospects. Journal of Future Foods 4 (2):128–34. doi: 10.1016/j.jfutfo.2023.06.003.
- Weetch, E., and A. Macdonald. 2006. The determination of phenylalanine content of foods suitable for phenylketonuria. Journal of Human Nutrition and Dietetics: The Official Journal of the British Dietetic Association 19 (3):229–36. doi: 10.1111/j.1365-277X.2006.00696.x.
- Yu, J., and J. Muñoz-Justicia. 2020. A bibliometric overview of twitter-related studies indexed in web of science. Future Internet 12 (5):91. doi: 10.3390/fi12050091.
- Zhang, S., Y. Zheng, Z. Wu, Y. Zhang, X. Liu, Z. Luo, H. Li, H. Li, and J. Yu. 2024. Preparation of food ingredients for the PKU patients: Two-step enzymatic hydrolysis and activated carbon adsorption for the removal of phenylalanine from whey protein hydrolysates. International Journal of Dairy Technology 77 (1):132–45. doi: 10.1111/1471-0307.13026.