Abstract
The nutritional benefits of combining probiotics with plant proteins have sparked increasing research interest and drawn significant attention. The interactions between plant proteins and probiotics demonstrate substantial potential for enhancing the functionality of plant proteins. Fermented plant protein foods offer a unique blend of bioactive components and beneficial microorganisms that can enhance gut health and combat chronic diseases. Utilizing various probiotic strains and plant protein sources opens doors to develop innovative probiotic products with enhanced functionalities. Nonetheless, the mechanisms and synergistic effects of these interactions remain not fully understood. This review aims to delve into the roles of promoting health through the intricate interplay of plant proteins and probiotics. The regulatory mechanisms have been elucidated to showcase the synergistic effects, accompanied by a discussion on the challenges and future research prospects. It is essential to recognize that the interactions between plant proteins and probiotics encompass multiple mechanisms, highlighting the need for further research to address challenges in achieving a comprehensive understanding of these mechanisms and their associated health benefits.
1. Introduction
Plant-based proteins foods, characterized by low fat and calorie content, lactose-free composition, and other beneficial traits, are gaining popularity as a viable alternative for individuals seeking to replace animal proteins. Particularly, this trend resonates with vegetarians and lactose-intolerant individuals, affirming their status as rising stars in the plant nutrition market (Aimutis Citation2022; Yan et al. Citation2023). The range of available plant proteins has been steadily expanding, encompassing various sources such as cereals (oats, rice), pseudocereals (buckwheat, quinoa), legumes (soybeans, peas, mung beans, chickpeas), and nuts (almonds, walnuts). This market expansion has garnered significant interest from the industry (Hertzler et al. Citation2020).
The word "probiotic" comes from the Greek meaning "for life". Probiotics are a group of living microorganisms that play a pivotal role in the fermentation of diverse food items, spanning from vegetables and fruits to meats, dairy, and grain products. Their application not only elevates the nutritional value of these foods but also offers numerous health benefits when consumed in adequate doses (Hill et al. Citation2014). In recent years, due to the increasing demand for preventive health care by groups worldwide, the market size of probiotics has grown strongly and is expected to grow to $119.28 billion by 2028 (Intelligence Citation2024). Commonly found in fermented foods or consumed as dietary supplements, probiotics are a valuable addition to any balanced diet. The incorporation of probiotics fermentation in food preparation can alter the taste and composition of the final products. Specifically, the application of probiotics fermentation to different plant proteins can effectively break down anti-nutritional factors, thereby enhancing the overall nutritional value of the food. Additionally, probiotics have the ability to improve the flavor and texture of plant-based beverages, allowing for the mitigation of undesirable taste and texture characteristics. Compared to conventional vegetable protein drinks, probiotic-fermented plant-based protein beverages offer distinct advantages in terms of vitamin content, improved nutrient absorption and bioactivity, product stability, as well as safety considerations (Valero-Cases et al. Citation2020).
Probiotics are able to effectively regulate pH levels to improve the solubility and digestibility of plant proteins. Through fermentation, probiotics can modify the structure and functionality of plant proteins, resulting in the production of bioactive substances such as vitamins, antioxidants, and antimicrobial peptides. Furthermore, probiotics facilitate protein absorption and utilization, thereby addressing protein energy malnutrition. Additionally, probiotics alter the metabolic activity within the intestinal microbiota, maintaining a balance between protein degradation and synthesis (Rasika et al. Citation2021).
The combination of plant proteins and probiotics exhibits synergistic effects, whereby plant proteins create a favorable environment for probiotics growth and proliferation. Serving as a substrate for probiotic metabolism, plant proteins support their growth and contribute to the synthesis of beneficial metabolites. This reciprocal interaction between plant proteins and probiotics enhances their overall effectiveness in promoting gut health and facilitating nutrient absorption. The combination of protein and probiotics holds significant potential for promoting human health, with benefits including improving digestion, maintaining gut health, supporting for the immune system, enhancing mental well-being, and improving nutrient absorption (Ranjha et al. Citation2021).
The combination of multiple flavors, nutrition, food functionality, process refinement, and probiotics with plant proteins has become a focal point of current research. The fusion of plant proteins and probiotics can cater to various nutritional needs for human health while also delivering innovative taste and nutrition. These synergistic effects show significant potential in enhancing protein digestion and absorption thereby improving human health. The combination of specific probiotic strains and plant proteins is paving the way for advancing plant protein products. However, the mechanisms and challenges of these synergistic effects for health promotion necessitate further investigation. This review seeks to delve into the roles of promoting health through the interplay of plant proteins and probiotics. The regulatory mechanisms have been elucidated to demonstrate the synergistic effects, with a discussion on the challenges and prospects for future research.
2. The interactions between plant proteins and probiotics
Plant protein plays a crucial role in regulating the growth and metabolism of probiotics. Previous studies have shown that protein stimulates the synthesis of cytoplasmic proteins in probiotics, which in turn increases cell biomass and growth rate (Molska et al. Citation2022). Additionally, through fermentation (), probiotics can alter the structure, modify functionality, facilitate bioactivity availability of plant proteins, regulate the absorption, and utilization of nutrients. Plant proteins are instrumental in improving the growth and proliferation of probiotics, stimulating the synthesis of cytoplasmic proteins in probiotics, supporting energy and metabolism, as well as maintaining the integrity of cell membranes. The combination of protein and probiotics demonstrates significant potential in promoting human health, including maintaining gut barrier, altering the metabolic activity within the gut microbiota, improving gut homeostasis, and enhancing immune response. By integrating plant proteins with probiotics, it becomes possible to meet diverse nutritional requirements while simultaneously achieving innovative taste and nutrition, thus offering promising applications.
Figure 1. The interactions between plant proteins and probiotics regulate nutrients metabolism and probiotic growth and proliferation.
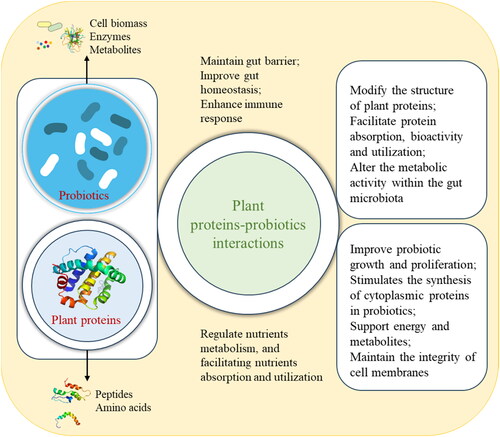
2.1. The promotion of probiotics growth and metabolism
Probiotics need a supply of nutrients for proliferation, and plant protein act as an essential growth stimulant for probiotics (Shaiken et al. Citation2023). It has demonstrated that protein promotes the synthesis of probiotic cytoplasmic proteins, thereby increasing the cell biomass and growth rate (Molska et al. Citation2022). Amino acids and peptides in protein degradation products can be converted into energy and metabolites through metabolic pathways. The energy generated by this process can be used to maintain the basic metabolic needs of cells, as well as maintaining the integrity and stability of cell membranes (Canon et al. Citation2021). The amino acids sequence in protein can affect the activity of the enzyme inside the probiotics. The types and contents of amino acids in proteins have significant effects on the growth rate and biomass of probiotics (Wang, Rosa-Sibakov, et al. Citation2022).
In addition, probiotics can use protein degradation products as carbon and nitrogen sources for growth and metabolism. Carbon and nitrogen sources have important effects on the growth and metabolism of probiotics (Xie et al. Citation2023). Protein can serve as carbon source to provide the necessary carbon elements for probiotics growth and metabolic activities (Bamigbade et al. Citation2022). At the same time, protein can also be used as a nitrogen source, in which amino acids can provide nitrogen elements required by probiotics to synthesize proteins such as cell structure and enzymes (Plavec and Berlec Citation2019). Moreover, the peptides and oligopeptides can be produced by protein hydrolysis or self-hydrolysis are deemed to regulate the activity and stability of enzymes and thus promote the growth of probiotics (Ramírez, Pineda-Hidalgo, and Rochín-Medina Citation2020). After the combination of probiotics and plant proteins, probiotics have a better survival chance, at the same time, play the physiological activity of probiotics and the growth-promoting function of prebiotics to produce health benefits to the host (Liu, Wang, and Wu Citation2021).
The effects of different types of proteins on probiotics are also different to some extent. Ding and Li (Citation2021) found that when walnut oligopeptide was added at 2.0%, the number of viable bacteria reached 2.69 × 1010 CFU/mL, which increased the metabolic rate, promoted the proliferation of probiotics, and improved the activity of bacteria. Zhang et al. (Citation2020) found that the peptides with small molecular weight from both soybean protein isolates and soybean peptides (PEPs) can be easier to effectively promote the growth of L. rhamnosus. It was also reported that the role of the soybean oligopeptide on JCM 1132 strain was manifested as promoting bacterial growth by increasing the content of tricarboxylic acid circulating intermediates, improving the metabolic capacity of L. acidophilus JCM 1132 (Li et al. Citation2020). In vitro simulated digestion tests confirmed that PEPs can act as a nitrogen source to promote probiotics growth and produce more lactic acid and acetic acid. These acids are derivatives of glucose metabolism by probiotics, demonstrating their efficacy in suppressing detrimental microorganisms (Chi et al. Citation2021).
Probiotics fermentation also produces metabolites with antibacterial properties, such as organic acids, peptides. Studies have shown that after fermentation of soy milk with probiotic B. coagulans VHProbi C08, L. rhamnosus and L. helveticus K14, it has considerable antimicrobial capacity against Escherichia coli, Listeria monocytogenes, Salmonella enteritidis, Bacillus cereus and Staphylococcus aureus (Peng et al. Citation2021). The metabolites produced after the combination of proteins and probiotics can affect the growth and proliferation of probiotics and further promote the bioactive function of probiotics (Leeuwendaal et al. Citation2022). has listed the recent studies to verify the influence of different plant proteins on probiotics growth and metabolites, promote the proliferation of probiotics. However, the specific effects can differ according to the probiotics type and multifactorial environmental. Further studies are required to fully understand how different plant proteins interact with probiotics, as well as their impact on gut health.
Table 1. The influence of different types of plant proteins on probiotics proliferation.
2.2. The enhancement of plant proteins functionality
2.2.1. The enhancement of plant proteins degradation
Proteolysis is triggered by cell envelope proteinase that breaks down proteins into polypeptides. Subsequently, dipeptides, tripeptides and oligopeptides may be taken up into the cells. In the metabolic pathway involved in metabolites of proteolysis, various enzymes play crucial roles, including cell envelope proteinase, peptidases, biosynthetic enzymes, dehydrogenase, aldolases, lyases, acyltransferases esterases, dehydrogenase, aminotransferases, decarboxylase, deiminases decarboxylase, dehydrogenase complex, and additional biosynthetic enzymes. Probiotics increase the production of protease and the enhanced activity of protease makes free amino acids a necessary factor for the proliferation of probiotics and also produces a variety of bioactive peptides (Brown et al. Citation2017). Furthermore, probiotics can induce the host to enhance the activity of digestive proteases and peptidases, as well as the release of exoenzymes involved in protein digestion (). Peptidases such as PepN and PepXP play an essential part in releasing amino acids, cell growth, and biomass formation and are also involved in the production of precursors of flavor substances (Wang and Ji Citation2019).
Figure 2. The roles of probiotics on proteolysis (A); the direct/indirect pathways of probiotics for gut microbiota-mediated proteolysis (B); and the effects of microbial metabolism on nutrient quality factors of plant food matrix (C).
FAA, free amino acids; BCAA, branch-chain amino acids; EAA, essential amino acids; IVPD, in vitro protein digestibility; IL, interleukin.
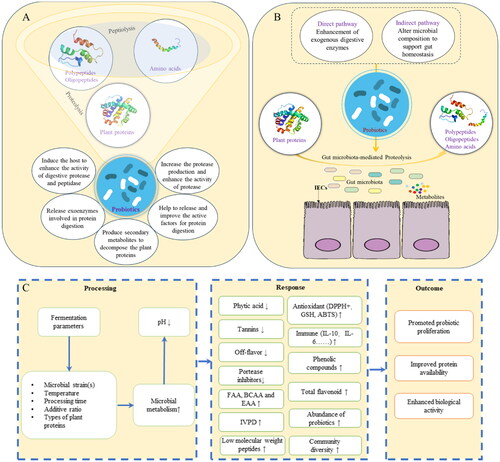
In the process of fermenting broad bean protein with the combination of Lactobacillus plantarum, Streptococcus thermophilus, and Lactobacillus acidophilus, more active protease will be produced, and plant proteins will be decomposed due to the synergistic effects between strains. After protein degradation and separation, small-molecule active polypeptides will be produced, and thus improve the nutritional quality and itself functional features (Ma et al. Citation2023). The fermentation uses proteases secreted by probiotics to hydrolyze proteins, optimize the texture, nutritional value, and bioactive substances of each plant source, and further promote the development of healthy fermented plant proteins (Engels et al. Citation2022).
In the process of fermenting plant proteins, probiotics can help to release and improve the active factors and metabolites and improve the utilization of plant proteins. Meanwhile, the probiotics will be in the lag or decline period and the secondary metabolites generated will decompose the plant proteins into small molecular peptides and free amino acids and then accumulate more amino acids and peptides (Zhao et al. Citation2022). At the same time, the variations among the strains themselves will lead to diverse metabolism and utilization capacities of plant proteins. This phenomenon may be caused by varying microorganisms producing different enzymes in the growth and reproduction process and different positions or degrees of plant proteins being cut, resulting in large differences in the amino acids composition types generated (Zhou et al. Citation2022).
2.2.2. The improvement of plant proteins digestion and absorption
Plant proteins digestion plays an important role in body health, as it can release bioactive peptides that are beneficial to overall well-being. Probiotics can influence the processes of proteins breaking down and amino acids synthesis mediated by intestinal bacteria through direct and indirect pathways (). Direct pathways include the enhancement of exogenous digestive enzymes. Indirect effects include modulation of microbial composition to support gut homeostasis, including the intestinal epithelial cells (IECs) normal morphology, thus providing an appropriate microenvironment for dietary plant proteins metabolism.
As shown, probiotics can enhance the digestion and uptake by directly influencing metabolism processes and indirectly manipulating the host gut ecosystem. Through a series of metabolic reaction processes, probiotics make biochemical changes in plant proteins and finally realize the mutual conversion between the three major substances (sugars, lipids, and proteins) or produce new substances to promote the digestion, decomposition and absorption of nutrients. Probiotics fermentation will cause enzymatic hydrolysis and acidification reactions, which will change functional properties of food and affect the advanced structure of proteins (Jiang et al. Citation2020).
The increase in protein digestibility is related to the decrease in α-helix to β-fold ratio, which produces acetic acid and lactic acid after microbial fermentation, leading to the instability of the protein secondary structure and rearrangement phenomenon (Yasar, Tosun, and Sonmez Citation2020). It significantly improves the solubility and digestibility of plant proteins and promotes amino acids production and protein degradation, which means that plant proteins treated with probiotics are more easily absorbed and utilized by the human body (García Arteaga et al. Citation2022).
Bacillus coagulans GBI-30, 608 in combination with plant protein has been proven to enhance the absorption and utilization of the protein and optimize the nutritional value in connection with protein supplementation (Keller et al. Citation2017). BC30 promotes digestion and uptake of pea, soybean, or rice protein in the upper gastrointestinal tract and maintains a healthy colon microenvironment (Kim et al. Citation2023; Walden et al. Citation2022). An in vitro study found that using Bifidobacterium animalis subsp. lactis B420, Lactococcus lactis subsp. lactis Ll-23, Lactiplantibacillus plantarum Lp-115, Lactobacillus acidophilus NCFM, Lacticaseibacillus paracasei subsp. paracasei Lpc-37, B. lactis Bl-04 and Lacticaseibacillus rhamnosus HN001 fermented plant proteins could affect the capacity of digestion while increasing bioavailability and accessibility (Marttinen et al. Citation2023).
The addition of probiotics can increase the absorption of amino acids and small peptides by plant proteins. The synergistic action of plant proteins with L. paracasei LP-DG® and LPC-S01 increases the amino acid concentration in blood, such as methionine, histidine, valine, leucine and tyrosine (Jäger et al. Citation2020). Furthermore, it promotes the utilization of proteins by enhancing the function and activity of intestinal cells, increasing the absorption surface area of IECs, improving the absorption capacity of the epithelium, and promoting peptide transport.
After the probiotics treatment, the plant protein changed from a tight ball structure to a loose wire group structure, and some groups appeared, which had a greater impact on the protein molecules, thus enhancing the absorption effect of plant proteins by the human body (Yakubu et al. Citation2022). The defect of low digestibility of plant proteins caused by the tight arrangement of protein structures is improved. Fermentation promotes the hydrolysis of proteins and the production of amino acids, which in turn increases the digestibility of plant proteins. However, different probiotics perform different abilities to produce flavor compounds for different plant proteins. has highlighted the roles of different probiotic strains and plant proteins, fermentation conditions, in vivo and in vitro experimental models, and specific change indicators to certify promote protein digestion and absorption.
Table 2. The influence of different types of probiotics on plant proteins digestion and absorption.
2.3. Probiotics fermented plant proteins to promote their bioactivity
Plant proteins possess diverse applications as bioactive peptides (BAPs) due to their wide range of functional properties. These BAPs demonstrate various activities such as antitumor, antimicrobial, antihypertensive, and antioxidant effects. The generation and roles of BAPs involve different mechanisms (Kumar et al. Citation2021): (a) Protein hydrolysis leads to the formation of bioactive hydrolysates/BAPs. (b) The enzyme angiotensin-I-converting enzyme (ACE) plays a key part in transforming angiotensin-I into its active form. Antihypertensive BAPs regulate blood pressure through suppressing ACE activity. Angiotensin-II increases blood pressure by causing vasoconstriction. (c) BAPs are believed to indirectly enhance the formation of nitric oxide or the bioavailability of bradykinin, eventually leading to vasodilation. (d) BAPs play a role in controlling the levels of low-density lipoproteins (LDL) through interaction with LDL-receptors. (e) BAPs contribute to the sequestration of free radicals formed in mitochondria and other cellular organelles, exerting their antioxidant effects. (f) BAPs exhibit potent anticancer properties and can be used as effective agents against cancer cells. (g) Antimicrobial BAPs interplay with microbial membranes and cause the eventual cell death.
Because probiotics fermentation has a cutting effect on the peptide chain in plant proteins, the specific proteases and peptidases produced by probiotics fermentation can selectively cut specific sites in plant proteins. After the synergistic action of various enzymes, longer peptide chains in plant proteins are further broken down into shorter peptide segments, yielding a series of peptides with physiological activity (Rim et al. Citation2021). Such antioxidant peptides, blood pressure-lowering peptides, antibacterial peptides, and other active peptide products enhance their nutritional value and specific functionality so that they have better bioavailability and physiological activity and are easier to be absorbed and utilized by the human body. Bioactive proteins and peptides in plant species shown to enhance health features when ingestion, such as regulating the body’s immune system response, improving chronic inflammation, lowering cholesterol levels, controlling obesity, and affecting antioxidant activity. Some studies have found that soybean protein isolates and PEPs have an impact on the growth and metabolism. This suggests that through probiotics fermentation, soybean protein isolates can produce peptides that have a positive impact on probiotics (Zhang et al. Citation2020). By fermentation and other methods, soy protein can produce potential bioactive and functional peptides, such as arginine-rich PEPs and isoleucine-rich PEPs (Zhu et al. Citation2023).
Plant protein contains a variety of bioactive substances, which can provide effective prevention and control strategies for regulating the disorders of glucose and lipid metabolism, elevations in inflammation levels, disturbances in intestinal environment, and immune abnormalities through the biological pathway combined with probiotics (Schroeder et al. Citation2017). By combining Tartary buckwheat protein with Lactobacillus plantarum ST-III, it has been comprehensively analyzed the effect of the dietary intervention mode combined with the two on regulating the disorder of lipid metabolism in mice (Zhao Citation2019).
Studies have shown that proteolytic substances can improve digestion and absorption as prebiotics by improving intestinal permeability and intestinal immunity (Aloo and Oh Citation2022). It was explored the mitigating potential of plant protein-probiotic preparations on lead poisoning in mice. The group given plant protein-probiotic preparations showed lower lead content in blood, liver and kidney tissues, higher antioxidant enzyme activity (such as superoxide dismutase and glutathione peroxidase activity), and lower malondialdehyde content (Wang, Wu, et al. Citation2021). Additionally, the liver and kidney histopathological changes of these mice were also light, which laid the foundation for further research on the application of plant protein-probiotic preparations in the field of toxicology.
Generally, food fermentation positively affects probiotic proliferation and protein availability (). Nevertheless, it is crucial to acknowledge that fermentation can also reduce the presence of substances like phytic acid, protease inhibitors and tannin. This reduction could result in a variation in health-promoting activities (Kårlund et al. Citation2020). illustrated different strains and plant proteins fermentation, and details experimental fermentation conditions, in vivo and in vitro experimental models and specific change indicators to prove the impact of fermentation of different plant proteins on nutritional functional properties and health-promoting activities.
Table 3. The combination of probiotics and plant proteins promotes their bioactivity.
3. Mechanisms of plant proteins-probiotics interactions
It has been widely accepted that probiotics fermentation products can alleviate or prevent various diseases by regulating intestinal health, establishing a close connection between probiotics and host health (Mazziotta et al. Citation2023). Probiotics, known for their ability to balance intestinal microecology, promote digestion and absorption, enhance gastrointestinal motility, boost body immunity, and alleviate allergic reactions, have been recognized as guardians of health (Sumera, Aqsa, and Imtiaz Citation2023). Research suggests that probiotics exert their beneficial effects through mechanisms such as immune response modulation, prevention of physiological stress, inhibition of pathogens, microbiota balance, and improvement of intestinal barrier function (Suez et al. Citation2019). Well-known probiotic microorganisms with a long history of use include Lactococcus, Lactobacillus, Streptococcus, Enterococcus, and Bifidobacterium.
Probiotics play a crucial role in regulating the gut microbiota, which in turn affects gut bacteria involved in proteolysis (Salam et al. Citation2023). By breaking down large molecular plant proteins into simpler forms, probiotics enhance the digestion and absorption of these nutrients in the human body. This metabolic process also leads to the production of various substances including short-chain fatty acids (SCFAs), exopolysaccharides, and vitamins. When it comes to the degradation of hard-to-digest plant proteins found in food, probiotics have the ability to break them down into smaller peptides and amino acids (), thereby improving their digestibility and nutritional value (Wang, Wu, et al. Citation2021).
Figure 3. Health promotion mechanisms of plant proteins-probiotics interactions.
Th1 cell, type 1 T helper cell; Th2 cell, type 2 T helper cell; l; Th17 cell, type 17 T helper cell Treg cell, regulatory T cell; sIgA, secreted immunoglobulin A; PPR, pattern recognition receptor; MUC, mucins; IL, Interleukin; TGF-β, transforming growth factor beta; TLR, toll-like receptor; LPS, lipopolysaccharide; ROS, reactive oxygen species.
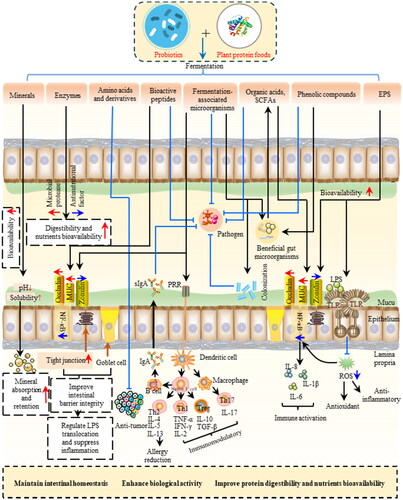
The combination of plant proteins with probiotics can effectively optimize the dietary nutrient structure needed by the human body, catering to the diverse requirement and offering health advantages (Adriana Dalila et al. Citation2023). Furthermore, the post-epidemic era has witnessed a rise in the prevalence of gastroenteritis and enteropulmonary diseases, with various health symptoms indicating the need for the consumption of high-quality protein to maintain muscle mass, boost immunity, and enhance physical fitness.
The interactions mechanisms between probiotics and plant proteins are roughly manifested in the following aspects:
Changes in plant protein solubility: The binding of probiotics to plant proteins may result in changes in plant protein solubility.
Promote plant proteins degradation: Probiotics have protease activity, which can promote the degradation of protein macromolecular aggregates into small peptides or polypeptides, thereby increasing the content of α-helix structure. The increase in this structure may lead to modifications in the function, improve the solubility and stability of proteins, and make it easier to digest and absorb.
Formation of complex: The combination of probiotics and plant proteins may form a complex, which may have new bioactivities such as antioxidant, anti-inflammatory, antibacterial, anti-allergen and immune regulation, and can also affect the combination of pathogenic bacteria and IECs, degrade anti-nutritional factors, and improve the bioavailability of proteins.
Change plant protein function: The combination of probiotics with plant proteins may change protein function. Some studies have shown that probiotics can improve the fermentation performance of plant proteins, change the emulsification, foaming, gel formation ability of proteins, etc., and improve their application value in food processing.
Plant proteins and probiotics engage in interactions such as adhesion, binding, and co-metabolism. As a signal molecule for cell attachment, proteins can bind to receptors on the surface of probiotics. This binding activates signal pathways within the cell, which subsequently affects cell attachment, growth metabolism, and probiotic function (do Carmo et al. Citation2018). Adhesion and binding represent significant mechanisms by which probiotics attach to the intestinal wall in the host intestine. Surface proteins enable probiotics to bind to IECs, facilitating their attachment to intestinal cells and enabling them to perform their functions. These interactions between proteins and probiotics, as well as co-metabolism, play a fundamental role in maintaining a balanced gut microbial community (Kieliszek et al. Citation2021).
Plant proteins combined with probiotics can produce many synergistic effects (), including the following aspects:
Increase the nutritional value: The combination of probiotics and plant proteins can improve the bioavailability of protein, promote the digestibility of protein and the growth of probiotics, and increase its nutritional value to the body.
Regulate the body’s immunity: proteins combined with probiotics can participate in the process of regulating the function of the immune system and promote the body’s resistance to diseases.
Maintain the intestinal health: Probiotics can maintain the balance of the intestinal microbiota, and protein combined with probiotics may be more easily absorbed and utilized by the intestine, contributing to intestinal environmental homeostasis.
Improve the plant protein stability: the combination of plant proteins and probiotics can improve the stability of plant proteins and extend their shelf life, thereby increasing the availability of related products.
Plant proteins in the diet encompass more than just protein; they also consist of polyphenols, minerals, fiber, and other components, creating a complex matrix. Fermented plant protein food ingredients contain various constituents that are transported to the gut, exerting an impact on both intestinal and overall health (). Fermentation enhances the bioavailability and absorption of polyphenols, minerals, and amino acids (Arghya et al. Citation2023; Vincenzo et al. Citation2024). Fermented plant protein foods offer a range of amino acids and derivatives that can function as neurotransmitters and/or immune transmitters. Additionally, these foods contain beneficial microorganisms and bioactive peptides that can inhibit the growth and colonization of intestinal pathogens. The immune system is influenced by organic acids, polysaccharides, and SCFAs, which are either directly delivered to or produced by gut microbes. These substances contribute to maintaining the integrity of the gut barrier, suppressing pathogens, and reducing inflammation (Wastyk et al. Citation2021). To gain a deeper understanding of the underlying mechanisms, randomized controlled trials and large cohort studies are necessary.
4. Challenges and perspectives
Probiotics fermentation can modify the physicochemical properties and health functions of food, resulting in distinct flavors and diversified taste and texture of plant protein-based foods. By considering the synergistic interaction between plant proteins and probiotic strains, various beneficial outcomes can be achieved, including the enrichment of active functional substances, extraction of effective components, release of flavor compounds and metabolites, generation of novel active ingredients, reduction of unpleasant flavors, and improved biological utilization (). Applying probiotics fermentation to various plant proteins can enhance the overall value of food products.
Figure 4. The research framework of the challenges and perspectives for plant proteins-probiotics interactions.
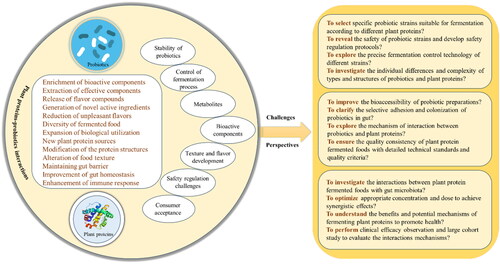
Moreover, the combination and fermentation of different probiotic strains lead to sensory and biological characteristics that are distinct to each product. It is worth to note the importance of making full use of sensory analysis research in fermented products. By designing an effective analysis program, ensure that the composition, odor and texture of the final fermented product do not produce negative effects (Gámbaro and Matthew Citation2020). This approach contributes to the specific biological and chemical composition of fermented foods and enhances the diversity of fermented products, thereby increasing their acceptance among consumers.
Currently, the regulatory impact of probiotics on gut microorganisms and their ability to maintain intestinal homeostasis remain uncertain. The stability and survival rate will be challenged in the process of processing and digestion and the resilience of probiotic preparations to harsh conditions in the gastrointestinal tract requires enhancement. Typically, the mucosa’s adhesion in the colon is insufficient, leading to poor bioaccessibility of probiotic preparations and hindering their effectiveness. The selective adhesion and colonization of probiotics in specific tissues like inflamed colon are yet to be investigated.
In the future, the health benefits of combining plant proteins with probiotics will be further strengthened, to achieve the advancements in plant protein raw materials, probiotics, and their production processes. The selection of probiotic strains is a crucial prerequisite for fermenting plant proteins. Given the wide range and variety of plant proteins and probiotic sources, it is necessary to screen specific probiotic strains suitable for fermentation based on the different properties of plant proteins. In the above process, strain screening, functional verification and high-activity preparation technology, even including the industrialization process of the final fermentation product, may face a lot of technical investment and research costs. Additionally, it is important to explore the fermentation process of different strains, design precise fermentation control technologies, and simplify the fermentation process to efficiently prepare substances in the fermentation system.
The development of functional foods, particularly those incorporating plant proteins and probiotics, remains an intricate and ongoing endeavor. Ongoing research is essential to accumulate scientific evidence that links these components to host nutrition and health. A comprehensive investigation of the mechanisms by which probiotics interact with plant proteins calls for further exploration. As shown in , the research framework is highlighted to clarify challenges and perspectives for plant proteins-probiotics interactions. It is crucial to characterize the structure of selected plant proteins, perform high-throughput sequencing screening of probiotic strains, and conduct safety studies. Furthermore, it is important to study the effects of probiotics on the digestion and absorption of plant proteins, gain a better understanding of the benefits and potential mechanisms of fermented plant proteins in promoting gut health, and explore the interactions mechanisms between plant proteins and probiotics.
In addition, due to individual variations and the intricate nature of probiotics and plant proteins, additional research is required to devise clinically efficacious combinations. Fermented foods offer a valuable amalgamation of beneficial microorganisms and bioactive compounds. By scientifically combining probiotics and plant proteins, appropriate concentrations and dosages can be selected to achieve synergistic effects, thus optimizing their incorporation and expanding their applications in the realm of health. However, it must be emphasized that the success of the laboratory scale does not mean that it can scale up production, so there are huge economic challenges to achieve the expansion from the laboratory to industrial production, such as technology maturity, large-scale production costs, market demand, results transformation, talent support, etc. Furthermore, to ensure consistent quality in plant proteins-based fermented foods, comprehensive technical standards and quality criteria need to be developed for evaluating the final food products.
Furthermore, probiotic fermentation and metabolism processes are intricate. To ensure the targeted delivery of probiotics, the use of precise control of the release mechanism and the selection of appropriate packaging technology have become critical. The research on the processing technology of composite products combining plant proteins and probiotics is still in its early stages. The interaction mechanisms among different combinations of plant proteins and probiotics are not fully understood, and the coexistence of these components in the fermentation system presents challenges for product separation and purification. Additionally, compelling evidence is essential to validate the benefits and mechanisms of probiotics and plant proteins on nutrition and health, emphasizing the importance of randomized and controlled human trials. The potential of plant protein-probiotic interactions remains largely unclear, necessitating continuous efforts to explore the diversity and possibilities of fermented plant proteins products, and achieve innovative advancements in their combination.
Author contributions
Conceptualization, Jun Du, Caili Fu, and Dejian Huang; writing—original draft preparation, Juntao Kan, Qiming Wu and Zhengying Cui; writing—review and editing, Jun Du, Caili Fu, Dejian Huang and Lin Chen; resources and visualization, Yuchen Ma, Xin Liu, Ruifang Dong and Lin Chen. All authors have read and approved the manuscript.
Ethical approval
This article does not contain any studies with human participants or animals performed by any of the authors.
Disclosure statement
The authors declare no conflict of interest.
Additional information
Funding
References
- Adriana Dalila, C., U. Adriana Cristina, C. Cristian Ovidiu, C. Lucian, C. Dana Maria, B. Ramona Flavia, and O. Neli Kinga. 2023. Plant-based beverages from germinated and ungerminated seeds, as a source of probiotics, and bioactive compounds with health benefits—part 1: Legumes. Agriculture 13 (6):1185. doi:10.3390/agriculture13061185.
- Aimutis, W. R. 2022. Plant-based proteins: The good, bad, and ugly. Annual Review of Food Science and Technology 13 (1):1–17. doi:10.1146/annurev-food-092221-041723.
- Akin, Z., and T. Ozcan. 2017. Functional properties of fermented milk produced with plant proteins. LWT 86:25–30. doi:10.1016/j.lwt.2017.07.025.
- Aloo, S. O., and D. H. Oh. 2022. The functional interplay between gut microbiota, protein hydrolysates/bioactive peptides, and obesity: A critical review on the study advances. Antioxidants (Basel, Switzerland) 11 (2):333. doi:10.3390/antiox11020333.
- Alrosan, M., T. C. Tan, A. M. Easa, S. Gammoh, M. H. Alu’datt, G. M. Aleid, M. N. Alhamad, and S. Maghaydah. 2022. Evaluation of quality and protein structure of natural water kefir-fermented quinoa protein concentrates. Food Chemistry 404 (Pt B):134614. doi:10.1016/j.foodchem.2022.134614.
- Aponte, M., F. Ungaro, I. d’Angelo, C. De Caro, R. Russo, G. Blaiotta, F. Dal Piaz, A. Calignano, and A. Miro. 2018. Improving in vivo conversion of oleuropein into hydroxytyrosol by oral granules containing probiotic Lactobacillus plantarum 299v and an Olea europaea standardized extract. International Journal of Pharmaceutics 543 (1–2):73–82. doi:10.1016/j.ijpharm.2018.03.013.
- Arghya, M., B. Samuel, D. Eirini, L. M. Maria, and D. C. Paul. 2023. Fermented foods and gastrointestinal health: Underlying mechanisms. Nature Reviews Gastroenterology & Hepatology. 21 (4):248–266. doi:10.1038/s41575-023-00869-x.
- Bamigbade, G. B., A. J. Subhash, A. Kamal-Eldin, L. Nyström, and M. Ayyash. 2022. An updated review on prebiotics: Insights on potentials of food seeds waste as source of potential prebiotics. Molecules (Basel, Switzerland) 27 (18):5947. doi:10.3390/molecules27185947.
- Boulay, M., M. Al Haddad, and F. Rul. 2020. Streptococcus thermophilus growth in soya milk: Sucrose consumption, nitrogen metabolism, soya protein hydrolysis and role of the cell-wall protease PrtS. International Journal of Food Microbiology 335:108903. doi:10.1016/j.ijfoodmicro.2020.108903.
- Brown, L., E. V. Pingitore, F. Mozzi, L. Saavedra, J. M. Villegas, and E. M. Hebert. 2017. Lactic acid bacteria as cell factories for the generation of bioactive peptides. Protein and Peptide Letters 24 (2):146–55. doi:10.2174/0929866524666161123111333.
- Brückner-Gühmann, M., E. Vasil’eva, A. Culetu, D. Duta, N. Sozer, and S. Drusch. 2019. Oat protein concentrate as alternative ingredient for non-dairy yoghurt-type product. Journal of the Science of Food and Agriculture 99 (13):5852–7. doi:10.1002/jsfa.9858.
- Budryn, G., E. Klewicka, J. Grzelczyk, I. Gałązka-Czarnecka, and R. Mostowski. 2019. Lactic acid fermentation of legume seed sprouts as a method of increasing the content of isoflavones and reducing microbial contamination. Food Chemistry 285:478–84. doi:10.1016/j.foodchem.2019.01.178.
- Çabuk, B., M. G. Nosworthy, A. K. Stone, D. R. Korber, T. Tanaka, J. D. House, and M. T. Nickerson. 2018. Effect of fermentation on the protein digestibility and levels of non-nutritive compounds of pea protein concentrate. Food Technology and Biotechnology 56 (2):257–64. doi:10.17113/ftb.56.02.18.5450.
- Cáceres, P. J., E. Peñas, C. Martínez-Villaluenga, P. García-Mora, and J. Frías. 2018. Development of a multifunctional yogurt-like product from germinated brown rice. LWT 99:306–12. doi:10.1016/j.lwt.2018.10.008.
- Canaviri Paz, P., R. J. Janny, and Å. Håkansson. 2020. Safeguarding of quinoa beverage production by fermentation with Lactobacillus plantarum DSM 9843. International Journal of Food Microbiology 324:108630. doi:10.1016/j.ijfoodmicro.2020.108630.
- Canon, F., M. B. Maillard, G. Henry, A. Thierry, and V. Gagnaire. 2021. Positive interactions between lactic acid bacteria promoted by nitrogen-based nutritional dependencies. Applied and Environmental Microbiology 87 (20): E 0105521. doi:10.1128/aem.01055-21.
- Castro-Alba, V., C. E. Lazarte, D. Perez-Rea, A.-S. Sandberg, N.-G. Carlsson, A. Almgren, B. Bergenståhl, and Y. Granfeldt. 2019. Effect of fermentation and dry roasting on the nutritional quality and sensory attributes of quinoa. Food Science & Nutrition 7 (12):3902–11. doi:10.1002/fsn3.1247.
- Cerdá-Bernad, D., E. Valero-Cases, J. J. Pastor, M. J. Frutos, and F. Pérez-Llamas. 2021. Probiotic red quinoa drinks for celiacs and lactose intolerant people: Study of functional, physicochemical and probiotic properties during fermentation and gastrointestinal digestion. International Journal of Food Sciences and Nutrition 73 (1):49–59. doi:10.1080/09637486.2021.1921707.
- Chandra-Hioe, M. V., C. H. M. Wong, and J. Arcot. 2016. The potential use of fermented chickpea and faba bean flour as food ingredients. Plant Foods for Human Nutrition (Dordrecht, Netherlands) 71 (1):90–5. doi:10.1007/s11130-016-0532-y.
- Chen, J. M., K. F. Al, L. J. Craven, S. Seney, M. Coons, H. McCormick, G. Reid, C. O’Connor, and J. P. Burton. 2020. Nutritional, microbial, and allergenic changes during the fermentation of cashew ‘cheese’ product using a quinoa-based rejuvelac starter culture. Nutrients 12 (3):648. doi:10.3390/nu12030648.
- Chi, Z., Y. Zhang, S. Xia, S. Zhu, W. Li, S. M. Aboelenin, M. Soliman, H. Li, and X. Liu. 2021. iTRAQ-based proteomic analysis of the differential effects of digested soy peptides and digested soy protein isolates on Lacticaseibacillus rhamnosus. Food Bioscience 43:101296. doi:10.1016/j.fbio.2021.101296.
- Choudhary, S., M. Singh, D. Sharma, S. Attri, K. Sharma, and G. Goel. 2018. Principal component analysis of stimulatory effect of synbiotic combination of indigenous probiotic and inulin on antioxidant activity of soymilk. Probiotics and Antimicrobial Proteins 11 (3):813–9. doi:10.1007/s12602-018-9432-9.
- Coda, R., J. Varis, M. Verni, C. G. Rizzello, and K. Katina. 2017. Improvement of the protein quality of wheat bread through faba bean sourdough addition. LWT – Food Science and Technology 82:296–302. doi:10.1016/j.lwt.2017.04.062.
- Cotârleț, M., N. Stănciuc, and G. E. Bahrim. 2020. Yarrowia lipolytica and Lactobacillus paracasei solid state fermentation as a valuable biotechnological tool for the pork lard and okara’s biotransformation. Microorganisms 8 (8):1098. doi:10.3390/microorganisms8081098.
- Curiel, J. A., R. Coda, I. Centomani, C. Summo, M. Gobbetti, and C. G. Rizzello. 2014. Exploitation of the nutritional and functional characteristics of traditional Italian legumes: The potential of sourdough fermentation. International Journal of Food Microbiology 196:51–61. doi:10.1016/j.ijfoodmicro.2014.11.032.
- D’Alessandro, M.,D. Gottardi,M. Di Nunzio,S. M. Borgonovi,C. Parolin,B. Vitali,R. Lanciotti,L. Siroli, andF. Patrignani. 2023. Improved nutritional composition and in vitro protein digestibility of fermented soy beverages produced with vaginal probiotics as adjunct cultures. Journal of Functional Foods 107:105703. doi:10.1016/j.jff.2023.105703.
- De Pasquale, I., E. Pontonio, M. Gobbetti, and C. G. Rizzello. 2019. Nutritional and functional effects of the lactic acid bacteria fermentation on gelatinized legume flours. International Journal of Food Microbiology 316:108426. doi:10.1016/j.ijfoodmicro.2019.108426.
- De Pasquale, I., M. Verni, V. Verardo, A. M. Gómez-Caravaca, and C. G. Rizzello. 2021. Nutritional and functional advantages of the use of fermented black chickpea flour for semolina-pasta fortification. Foods (Basel, Switzerland) 10 (1):182. doi:10.3390/foods10010182.
- Desfita, S., W. Sari, Y. Yusmarini, U. Pato, M. Zakłos-Szyda, and G. Budryn. 2021. Effect of fermented soymilk-honey from different probiotics on osteocalcin level in menopausal women. Nutrients 13 (10):3581. doi:10.3390/nu13103581.
- Di Renzo, T., A. Reale, F. Boscaino, and M. C. Messia. 2018. Flavoring production in kamut®, quinoa and wheat doughs fermented by Lactobacillus paracasei, Lactobacillus plantarum, and Lactobacillus brevis: A SPME-GC/MS study. Frontiers in Microbiology 9:429. doi:10.3389/fmicb.2018.00429.
- Ding, T., and Y. Li. 2021. Beneficial effect and mechanism of walnut oligopeptide on Lactobacillus plantarum Z7. Food Science & Nutrition 9 (2):672–81. doi:10.1002/fsn3.2029.
- do Carmo, F. L. R., H. Rabah, R. D. De Oliveira Carvalho, F. Gaucher, B. F. Cordeiro, S. H. da Silva, Y. Le Loir, V. Azevedo, and G. Jan. 2018. Extractable bacterial surface proteins in probiotic-host interaction. Frontiers in Microbiology 9:645. doi:10.3389/fmicb.2018.00645.
- El Youssef, C., P. Bonnarme, S. Fraud, A.-C. Péron, S. Helinck, and S. Landaud. 2020. Sensory improvement of a pea protein-based product using microbial co-cultures of lactic acid bacteria and yeasts. Foods (Basel, Switzerland) 9 (3):349. doi:10.3390/foods9030349.
- Engels, W., J. Siu, S. van Schalkwijk, W. Wesselink, S. Jacobs, and H. Bachmann. 2022. Metabolic conversions by lactic acid bacteria during plant protein fermentations. Foods (Basel, Switzerland) 11 (7):1005. doi:10.3390/foods11071005.
- Feng, L., Y. Xie, C. Peng, Y. Liu, and H. Wang. 2018. A novel antidiabetic food produced via solid-state fermentation of tartary buckwheat by L. plantarum TK9 and L. paracasei TK1501. Food Technology and Biotechnology 56 (3):373–80. doi:10.17113/ftb.56.03.18.5540.
- Fu, W.,C. Liu,X. Meng,Sha. Tao, andW. Xue. 2021. Co-culture fermentation of Pediococcus acidilactici XZ31 and yeast for enhanced degradation of wheat allergens. International Journal of Food Microbiology 347:109190. doi:10.1016/j.ijfoodmicro.2021.109190.
- Gámbaro, A. M., and B. Matthew. 2020. Chapter eight – sensory methods applied to the development of probiotic and prebiotic foods. Advances in food and nutrition research 94,:295–337. doi:10.1016/bs.afnr.2020.06.006.
- García Arteaga, V., V. Demand, K. Kern, A. Strube, M. Szardenings, I. Muranyi, P. Eisner, and U. Schweiggert-Weisz. 2022. Enzymatic hydrolysis and fermentation of pea protein isolate and its effects on antigenic proteins, functional properties, and sensory profile. Foods (Basel, Switzerland) 11 (1):118. doi:10.3390/foods11010118.
- García Arteaga, V., S. Leffler, I. Muranyi, P. Eisner, and U. Schweiggert-Weisz. 2021. Sensory profile, functional properties and molecular weight distribution of fermented pea protein isolate. Current Research in Food Science 4:1–10. doi:10.1016/j.crfs.2020.12.001.
- Hertzler, S., J. Lieblein-Boff, M. Weiler, and C. Allgeier. 2020. Plant proteins: Assessing their nutritional quality and effects on health and physical function. Nutrients 12 (12):3704. doi:10.3390/nu12123704.
- Hill, C., F. Guarner, G. Reid, G. R. Gibson, D. J. Merenstein, B. Pot, L. Morelli, R. B. Canani, H. J. Flint, S. Salminen, et al. 2014. Expert consensus document: The international scientific association for probiotics and prebiotics consensus statement on the scope and appropriate use of the term probiotic. Nature Reviews. Gastroenterology & Hepatology 11 (8):506–14. doi:10.1038/nrgastro.2014.66.
- Hu, L., Y. Li, X. Huang, C. Du, D. Huang, and X. Tao. 2023. The Effect of co-fermentation with Lactobacillus plantarum HLJ29L2 and yeast on wheat protein characteristics in sourdough and crackers. Foods (Basel, Switzerland) 12 (3):555. doi:10.3390/foods12030555.
- Hwang, C. E., S. C. Kim, D. H. Kim, H. Y. Lee, H. K. Suh, K. M. Cho, and J. H. Lee. 2020. Enhancement of isoflavone aglycone, amino acid, and CLA contents in fermented soybean yogurts using different strains: Screening of antioxidant and digestive enzyme inhibition properties. Food Chemistry 340:128199. doi:10.1016/j.foodchem.2020.128199.
- Hyeon Deok, K., L. Keun Suk, L. Kyung Eun, S. H. Joo, and K. Byung-Yong. 2023. Improved digestibility and bioavailability of pea protein following enzymatic treatment and fermentation by lactic acid bacteria. Food Science and Biotechnology 33(1):607–615. doi:10.1007/s10068-023-01335-9.
- Intelligence, M. 2024. Probiotics Market Size & Share Analysis - Growth Trends & Forecasts (2024–2029). Accessed May 8, 2024. https://www.mordorintelligence.com/industry-reports/probiotics-market.
- Jäger, R., J. Zaragoza, M. Purpura, S. Iametti, M. Marengo, G. M. Tinsley, A. J. Anzalone, J. M. Oliver, W. Fiore, A. Biffi, et al. 2020. Probiotic administration increases amino acid absorption from plant protein: A placebo-controlled, randomized, double-blind, multicenter, crossover study. Probiotics and Antimicrobial Proteins 12 (4):1330–9. doi:10.1007/s12602-020-09656-5.
- Jang, W. J., J. M. Lee, M. T. Hasan, B. J. Lee, S. G. Lim, and I. S. Kong. 2019. Effects of probiotic supplementation of a plant-based protein diet on intestinal microbial diversity, digestive enzyme activity, intestinal structure, and immunity in olive flounder (Paralichthys olivaceus). Fish & Shellfish Immunology 92:719–27. doi:10.1016/j.fsi.2019.06.056.
- Jeon, H.,H. Kim,M. Lee,J. Moon,J. Kim,J. Yang, andY. Jung. 2023. Oral Administration of Animal and Plant Protein Mixture with Lactiplantibacillus plantarum IDCC 3501 Improves Protein Digestibility. Fermentation 9 (6):560. doi:10.3390/fermentation9060560.
- Jiang, Y., J. Jia, D. Xiong, X. Xu, Y. Yang, X. Liu, and X. Duan. 2020. Effects of short-term fermentation with lactic acid bacteria on egg white: Characterization, rheological and foaming activities. Food Hydrocolloids 101:105507. doi:10.1016/j.foodhyd.2019.105507.
- Kårlund, A., C. Gómez-Gallego, J. Korhonen, O.-M. Palo-Oja, H. El-Nezami, and M. Kolehmainen. 2020. Harnessing microbes for sustainable development: Food fermentation as a tool for improving the nutritional quality of alternative protein sources. Nutrients 12 (4):1020. doi:10.3390/nu12041020.
- Keller, D., R. Van Dinter, H. Cash, S. Farmer, and K. Venema. 2017. Bacillus coagulans GBI-30, 6086 increases plant protein digestion in a dynamic, computer-controlled in vitro model of the small intestine (TIM-1). Beneficial Microbes 8 (3):491–6. doi:10.3920/bm2016.0196.
- Ketnawa, S., and Y. Ogawa. 2018. Evaluation of protein digestibility of fermented soybeans and changes in biochemical characteristics of digested fractions. Journal of Functional Foods 52:640–7. doi:10.1016/j.jff.2018.11.046.
- Kieliszek, M., K. Pobiega, K. Piwowarek, and A. M. Kot. 2021. Characteristics of the proteolytic enzymes produced by lactic acid bacteria. Molecules (Basel, Switzerland) 26 (7):1858. doi:10.3390/molecules26071858.
- Kim, J., H. Kim, H. J. Jeon, Y. H. Jung, and J. Yang. 2023. Lacticaseibacillus casei IDCC 3451 strengthen digestibility of plant-based proteins in mice. Probiotics and Antimicrobial Proteins 16 (3):927–35. doi:10.1007/s12602-023-10091-5.
- Kumar, M., M. Tomar, S. Punia, J. Dhakane-Lad, S. Dhumal, S. Changan, M. Senapathy, M. K. Berwal, V. Sampathrajan, A. A. Sayed, et al. 2021. Plant-based proteins and their multifaceted industrial applications. LWT - Food Science and Technology, 154:112620. doi:10.1016/j.lwt.2021.112620.
- Lee, J. H., B. Kim, C. E. Hwang, M. A. Haque, S. C. Kim, C. S. Lee, S. S. Kang, K. M. Cho, and D. H. Lee. 2018. Changes in conjugated linoleic acid and isoflavone contents from fermented soymilks using Lactobacillus plantarum P1201 and screening for their digestive enzyme inhibition and antioxidant properties. Journal of Functional Foods 43:17–28. doi:10.1016/j.jff.2018.01.022.
- Leeuwendaal, N. K., C. Stanton, P. W. O’Toole, and T. P. Beresford. 2022. Fermented foods, health and the gut microbiome. Nutrients 14 (7):1527. doi:10.3390/nu14071527.
- Li, Y., Y. Han, Q. Zhao, C. Tang, J. Zhang, and Y. Qin. 2022. Fermented soy and fish protein dietary sources shape ileal and colonic microbiota, improving nutrient digestibility and host health in a piglet model. Frontiers in Microbiology 13:911500. doi:10.3389/fmicb.2022.911500.
- Li, W., H. Li, Y. Zhang, L. He, C. Zhang, and X. Liu. 2021. Different effects of soybean protein and its derived peptides on the growth and metabolism of Bifidobacterium animalis subsp. animalis JCM 1190. Food & Function 12 (13):5731–44. doi:10.1039/d1fo00480h.
- Liu, Z., Y. Wang, Y. Liu, Q. Zhang, W. Li, M. Dong, and X. Rui. 2021. The conformational structural change of soy glycinin via lactic acid bacteria fermentation reduced immunoglobulin E reactivity. Foods (Basel, Switzerland) 10 (12):2969. doi:10.3390/foods10122969.
- Liu, Y., J. Wang, and C. Wu. 2021. Modulation of gut microbiota and immune system by probiotics, pre-biotics, and post-biotics. Frontiers in Nutrition 8:634897. doi:10.3389/fnut.2021.634897.
- Li, W., Y. Zhang, H. Li, C. Zhang, J. Zhang, J. Uddin, and X. Liu. 2020. Effect of soybean oligopeptide on the growth and metabolism of Lactobacillus acidophilus JCM 1132. RSC Advances 10 (28):16737–48. doi:10.1039/d0ra01632b.
- Liu, G.,M. Chu,S. Nie,Xin. Xu, andJ. Ren. 2022. Effects of Ilisha elongata protein, soy protein and whey protein on growth characteristics and adhesion of probiotics. Current Research in Food Science 5:2125–34. doi:10.1016/j.crfs.2022.10.024.
- Lorusso, A., R. Coda, M. Montemurro, and C. Rizzello. 2018. Use of selected lactic acid bacteria and quinoa flour for manufacturing novel yogurt-like beverages. Foods (Basel, Switzerland) 7 (4):51. doi:10.3390/foods7040051.
- Lorusso, A., M. Verni, M. Montemurro, R. Coda, M. Gobbetti, and C. G. Rizzello. 2016. Use of fermented quinoa flour for pasta making and evaluation of the technological and nutritional features. LWT 78:215–21. doi:10.1016/j.lwt.2016.12.046.
- Ma, Y., L. Ma, L. Han, B. Dang, W. Z, and Zhang, W. 2023. Effects of compound strains fermentation on the physicochemical properties and functional properties of broad bean protein. Food and Fermentation Industries 49 (4):183–8. doi:10.13995/j.cnki.11-1802/ts.031539.
- Manus, J., M. Millette, C. Dridi, S. Salmieri, B. R. Aguilar Uscanga, and M. Lacroix. 2021. Protein quality of a probiotic beverage enriched with pea and rice protein. Journal of Food Science 86 (8):3698–706. doi:10.1111/1750-3841.15838.
- Manus, J., M. Millette, B. R. A. Uscanga, S. Salmieri, B. Maherani, and M. Lacroix. 2021. In vitro protein digestibility and physico-chemical properties of lactic acid bacteria fermented beverages enriched with plant proteins. Journal of Food Science 86 (9):4172–82. doi:10.1111/1750-3841.15859.
- Marttinen, M., M. Anjum, M. T. Saarinen, I. Ahonen, M. J. Lehtinen, P. Nurminen, and A. Laitila. 2023. Enhancing bioaccessibility of plant protein using probiotics: An in vitro study. Nutrients 15 (18):3905. doi:10.3390/nu15183905.
- Ma, H., L. Wang, H. Yu, W. Wang, G. Wu, G. Qin, Z. Tan, Y. Wang, and H. Pang. 2022. Protease-producing lactic acid bacteria with antibacterial properties and their potential use in soybean meal fermentation. Chemical and Biological Technologies in Agriculture 9 (1):40. doi:10.1186/s40538-022-00303-5.
- Mazziotta, C., M. Tognon, F. Martini, E. Torreggiani, and J. C. Rotondo. 2023. Probiotics mechanism of action on immune cells and beneficial effects on human health. Cells 12 (1):184. doi:10.3390/cells12010184.
- Meinlschmidt, P., E. Ueberham, J. Lehmann, U. Schweiggert-Weisz, and P. Eisner. 2016. Immunoreactivity, sensory and physicochemical properties of fermented soy protein isolate. Food Chemistry 205:229–38. doi:10.1016/j.foodchem.2016.03.016.
- Molska, M., J. Reguła, M. Zielińska-Dawidziak, A. Tomczak, and M. Świeca. 2022. Starch and protein analysis in buckwheat (Fagopyrum esculentum Moench) sprouts enriched with probiotic yeast. LWT 168:113903. doi:10.1016/j.lwt.2022.113903.
- Montemurro, M., E. Pontonio, M. Gobbetti, and C. G. Rizzello. 2018. Investigation of the nutritional, functional and technological effects of the sourdough fermentation of sprouted flours. International Journal of Food Microbiology 302 (2):47–58. doi:10.1016/j.ijfoodmicro.2018.08.005.
- Ogundipe, O. O., B. M. Fasogbon, F. O. Ogundipe, O. Oredope, and R. U. Amaezenanbu. 2021. Nutritional composition of non-dairy yogurt from sprouted tigernut tubers. Journal of Food Processing and Preservation 45 (11):15884. doi:10.1111/jfpp.15884.
- Peng, S., C. Guo, S. Wu, H. Cui, H. Suo, and Z. Duan. 2021. Bioactivity and metabolomics changes of plant-based drink fermented by Bacillus coagulans VHProbi C08. LWT 156:113030. doi:10.1016/j.lwt.2021.113030.
- Plavec, T. V., and A. Berlec. 2019. Engineering of lactic acid bacteria for delivery of therapeutic proteins and peptides. Applied Microbiology and Biotechnology 103 (5):2053–66. doi:10.1007/s00253-019-09628-y.
- Pontonio, E., A. Lorusso, M. Gobbetti, and C. G. Rizzello. 2017. Use of fermented milling by-products as functional ingredient to develop a low-glycaemic index bread. Journal of Cereal Science 77:235–42. doi:10.1016/j.jcs.2017.08.022.
- Ramírez, K., K. V. Pineda-Hidalgo, and J. J. Rochín-Medina. 2020. Fermentation of spent coffee grounds by Bacillus clausii induces release of potentially bioactive peptides. LWT 138:110685. doi:10.1016/j.lwt.2020.110685.
- Ranjha, M. M. A. N., B. Shafique, M. Batool, P. Ł. Kowalczewski, Q. Shehzad, M. Usman, M. F. Manzoor, S. M. Zahra, S. Yaqub, and R. M. Aadil. 2021. Nutritional and health potential of probiotics: A review. Applied Sciences 11 (23):11204. doi:10.3390/app112311204.
- Rasika, D. M. D., J. K. Vidanarachchi, R. S. Rocha, C. F. Balthazar, A. G. Cruz, A. S. Sant’Ana, and C. S. Ranadheera. 2021. Plant-based milk substitutes as emerging probiotic carriers. Current Opinion in Food Science 38:8–20. doi:10.1016/j.cofs.2020.10.025.
- Rim, N., A. Ola, N. Moncef, and J. Mourad. 2021. Fermented protein hydrolysates: Biological activities and applications. Current Opinion in Food Science 43:120–7. doi:10.1016/j.cofs.2021.11.006.
- Rizzello, C. G., I. Losito, L. Facchini, K. Katina, F. Palmisano, M. Gobbetti, and R. Coda. 2016. Degradation of vicine, convicine and their aglycones during fermentation of faba bean flour. Scientific Reports 6 (1):32452. doi:10.1038/srep32452.
- Rizzello, C. G., M. Verni, H. Koivula, M. Montemurro, L. Seppa, M. Kemell, K. Katina, R. Coda, and M. Gobbetti. 2017. Influence of fermented faba bean flour on the nutritional, technological and sensory quality of fortified pasta. Food & Function 8 (2):860–71. doi:10.1039/c6fo01808d.
- Rocchetti, G., F. Miragoli, C. Zacconi, L. Lucini, and A. Rebecchi. 2018. Impact of cooking and fermentation by lactic acid bacteria on phenolic profile of quinoa and buckwheat seeds. Food Research International (Ottawa, Ont.) 119:886–94. doi:10.1016/j.foodres.2018.10.073.
- Rosa-Sibakov, N., M. Re, A. Karsma, A. Laitila, and E. Nordlund. 2018. Phytic acid reduction by bioprocessing as a tool to improve the in vitro digestibility of faba bean protein. Journal of Agricultural and Food Chemistry 66 (40):10394–9. doi:10.1021/acs.jafc.8b02948.
- Rui, X., J. Huang, G. Xing, Q. Zhang, W. Li, and M. Dong. 2018. Changes in soy protein immunoglobulin E reactivity, protein degradation, and conformation through fermentation with Lactobacillus plantarum strains. LWT 99:156–65. doi:10.1016/j.lwt.2018.09.034.
- Ruiz de la Bastida, A., S. Langa, Á. Peirotén, R. Fernández-Gonzalez, A. Sánchez-Jiménez, M. Maroto, J. Antonio Curiel, E. Guillamon, J. L. Arqués, A. Gutiérrez-Adán, et al. 2023. Effect of fermented soy beverage in aged female mice model. Food Research International (Ottawa, Ont.) 169:112745. doi:10.1016/j.foodres.2023.112745.
- Sáez, G. D., C. Sabater, A. Fara, and G. Zárate. 2021. Fermentation of chickpea flour with selected lactic acid bacteria for improving its nutritional and functional properties. Journal of Applied Microbiology 133 (1):181–99. doi:10.1111/jam.15401.
- Salam, A. I., J. Y. Philip, D. A. Raphael, S. E. Abdulhakim, D. W. Namesha, P. Saeed, and V. B. Rea. 2023. A review and comparative perspective on health benefits of probiotic and fermented foods. International Journal of Food Science & Technology 58:4948–64. doi:10.1111/ijfs.16619.
- Sasikumar, R., K. Vivek, and A. K. Jaiswal. 2021. Effect of spray drying conditions on the physical characteristics, amino acid profile, and bioactivity of blood fruit (Haematocarpus validus Bakh.F. Ex Forman) seed protein isolate. Journal of Food Processing and Preservation 45 (6):15568. doi:10.1111/jfpp.15568.
- Schlegel, K., N. Lidzba, E. Ueberham, P. Eisner, and U. Schweiggert-Weisz. 2021. Fermentation of lupin protein hydrolysates—effects on their functional properties, sensory profile and the allergenic potential of the major lupin allergen lup an 1. Foods (Basel, Switzerland) 10 (2):281. doi:10.3390/foods10020281.
- Schroeder, B. O., G. M. H. Birchenough, M. Ståhlman, L. Arike, M. E. V. Johansson, G. C. Hansson, and F. Bäckhed. 2017. Bifidobacteria or fiber protects against diet-induced microbiota-mediated colonic mucus deterioration. Cell Host & Microbe 23 (1):27–40.e7. doi:10.1016/j.chom.2017.11.004.
- Shaiken, T. E., S. L. Grimm, M. Siam, A. Williams, A.-H. Rezaeian, D. Kraushaar, E. Ricco, M. J. Robertson, C. Coarfa, A. Jain, et al. 2023. Transcriptome, proteome, and protein synthesis within the intracellular cytomatrix. iScience 26 (2):105965. doi:10.1016/j.isci.2023.105965.
- Sharma, R., and S. Sharma. 2021. Anti-nutrient & bioactive profile, in vitro nutrient digestibility, techno-functionality, molecular and structural interactions of foxtail millet (Setaria italica L.) as influenced by biological processing techniques. Food Chemistry 368:130815. doi:10.1016/j.foodchem.2021.130815.
- Skalickova, S., A. Ridoskova, P. Slama, J. Skladanka, P. Skarpa, I. Smykalova, J. Horacek, R. Dostalova, and P. Horky. 2022. Effect of lactic fermentation and cooking on nutrient and mineral digestibility of peas. Frontiers in Nutrition 9:838963. doi:10.3389/fnut.2022.838963.
- Suez, J., N. Zmora, E. Segal, and E. Elinav. 2019. The pros, cons, and many unknowns of probiotics. Nature Medicine 25 (5):716–29. doi:10.1038/s41591-019-0439-x.
- Sumera, Z., H. Aqsa, and K. Imtiaz. 2023. Probiotics and their beneficial health effects. Mini-Reviews in Medicinal Chemistry 24 (1):110–25. doi:10.2174/1389557523666230608163823.
- Suwapat, K., Y. Nantana, W. Kanyawee, S. Uthaiwan, and S. Chalat. 2021. Health beneficial properties of a novel plant-based probiotic drink produced by fermentation of brown rice milk with GABA-producing Lactobacillus pentosus isolated from Thai pickled weed. Journal of Functional Foods 86:104710. doi:10.1016/j.jff.2021.104710.
- Terefe, Z. K., M. N. Omwamba, and J. M. Nduko. 2021. Effect of solid state fermentation on proximate composition, antinutritional factors and in vitro protein digestibility of maize flour. Food Science & Nutrition 9 (11):6343–52. doi:10.1002/fsn3.2599.
- Tiss, M., Z. Souiy, N. B Abdeljelil, M. Njima, L. Achour, and K. Hamden. 2020. Fermented soy milk prepared using kefir grains prevents and ameliorates obesity, type 2 diabetes, hyperlipidemia and liver-kidney toxicities in HFFD-rats. Journal of Functional Foods 67:103869. doi:10.1016/j.jff.2020.103869.
- Tuccillo, F., Y. Wang, M. Edelmann, A. M. Lampi, R. Coda, and K. Katina. 2022. Fermentation conditions affect the synthesis of volatile compounds, dextran, and organic acids by Weissella confusa A16 in faba bean protein concentrate. Foods (Basel, Switzerland) 11 (22):3579. doi:10.3390/foods11223579.
- Valero-Cases, E., D. Cerdá-Bernad, J. J. Pastor, and M. J. Frutos. 2020. Non-dairy fermented beverages as potential carriers to ensure probiotics, prebiotics, and bioactive compounds arrival to the gut and their health benefits. Nutrients 12 (6):1666. doi:10.3390/nu12061666.
- Verni, M., G. De Mastro, F. De Cillis, M. Gobbetti, and C. G. Rizzello. 2019. Lactic acid bacteria fermentation to exploit the nutritional potential of mediterranean faba bean local biotypes. Food Research International (Ottawa, Ont.) 125:108571. doi:10.1016/j.foodres.2019.108571.
- Vincenzo, F., A. Gaudio, V. Petito, L. Lopetuso, and F. Scaldaferri. 2024. Gut microbiota, intestinal permeability, and systemic inflammation: A narrative review. Internal and Emergency Medicine 19 (2):275–93. doi:10.1007/s11739-023-03374-w.
- Walden, K. E., A. M. Hagele, L. S. Orr, K. N. Gross, J. M. Krieger, R. Jäger, and C. M. Kerksick. 2022. Probiotic BC30 improves amino acid absorption from plant protein concentrate in older women. Probiotics and Antimicrobial Proteins 16 (1):125–37. doi:10.1007/s12602-022-10028-4.
- Wang, Y., Y. Fu, E. Azarpazhooh, W. Li, Q. Liu, and X. Rui. 2022. Assessment of in vitro digestive behavior of lactic-acid-bacteria fermented soy proteins: A study comparing colloidal solutions and curds. Molecules (Basel, Switzerland) 27 (21):7652. doi:10.3390/molecules27217652.
- Wang, J., and H. Ji. 2019. Influence of probiotics on dietary protein digestion and utilization in the gastrointestinal tract. Current Protein & Peptide Science 20 (2):125–31. doi:10.2174/1389203719666180517100339.
- Wang, Y., J. Liu, F. Wei, X. Liu, C. Yi, and Y. Zhang. 2019. Improvement of the nutritional value, sensory properties and bioavailability of rapeseed meal fermented with mixed microorganisms. LWT 112:108238. doi:10.1016/j.lwt.2019.06.005.
- Wang, Y., N. Rosa-Sibakov, M. Edelmann, N. Sozer, K. Katina, and R. Coda. 2022. Enhancing the utilization of rapeseed protein ingredients in bread making by tailored lactic acid fermentation. Food Bioscience 50:102028. doi:10.1016/j.fbio.2022.102028.
- Wang, Y., H. Sun, B. Han, H. Y. Li, and X. L. Liu. 2022. Improvement of nutritional value, molecular weight patterns (soluble peptides), free amino acid patterns, total phenolics and antioxidant activity of fermented extrusion pretreatment rapeseed meal with Bacillus subtilis YY-1 and Saccharomyces cerevisiae YY-2. LWT 160:113280. doi:10.1016/j.lwt.2022.113280.
- Wang, Y., J. Wu, M. Lv, Z. Shao, M. Hungwe, J. Wang, X. Bai, J. Xie, Y. Wang, and W. Geng. 2021. Metabolism characteristics and the expanding applications in food industry of lactic acid bacteria. Frontiers in Bioengineering and Biotechnology 9:612285. doi:10.3389/fbioe.2021.612285.
- Wang, Z., C. Zhang, K. Lu, K. Song, X. Li, L. Wang, and S. Rahimnejad. 2021. Effects of supplementing intestinal autochthonous bacteria in plant-based diets on growth, nutrient digestibility, and gut health of bullfrogs (Lithobates catesbeianus). Frontiers in Microbiology 12:739572. doi:10.3389/fmicb.2021.739572.
- Wastyk, H. C., G. K. Fragiadakis, D. Perelman, D. Dahan, B. D. Merrill, F. B. Yu, M. Topf, C. G. Gonzalez, W. Van Treuren, S. Han, et al. 2021. Gut-microbiota-targeted diets modulate human immune status. Cell 184 (16):4137–53.e14. doi:10.1016/j.cell.2021.06.019.
- Wu, Y., Y. Huang, J. Yu, F. Wang, X. Li, Y. Liu, and X. Ma. 2022. Changes of proteins and amino acids in soymilk during lactic acid fermentation and subsequent storage. Journal of Food Measurement and Characterization 16 (6):4728–37. doi:10.1007/s11694-022-01492-1.
- Xie, Z., J. Bao, Q. Mo, L. Chen, X. Rui, M. Dong, and W. Li. 2023. Effects of different types and concentrations of thickeners on yoghurt protein bioaccessibility by an in vitro dynamic gastrointestinal digestive model. International Journal of Dairy Technology 76 (4):885–94. doi:10.1111/1471-0307.12984.
- Xing, Q., S. Dekker, K. Kyriakopoulou, R. M. Boom, E. J. Smid, and M. A. I. Schutyser. 2019. Enhanced nutritional value of chickpea protein concentrate by dry separation and solid state fermentation. Innovative Food Science & Emerging Technologies 59:102269. doi:10.1016/j.ifset.2019.102269.
- Yakubu, C. M., R. Sharma, S. Sharma, and B. Singh. 2022. Influence of alkaline fermentation time on in vitro nutrient digestibility, bio- & techno-functionality, secondary protein structure and macromolecular morphology of locust bean (Parkia biglobosa) flour. LWT 161:113295. doi:10.1016/j.lwt.2022.113295.
- Yan, X., Z. Zeng, D. J. McClements, X. Gong, P. Yu, J. Xia, and D. Gong. 2023. A review of the structure, function, and application of plant-based protein – phenolic conjugates and complexes. Comprehensive Reviews in Food Science and Food Safety 22 (2):1312–36. doi:10.1111/1541-4337.13112.
- Yang, X., C. Ke, and L. Li. 2021. Physicochemical, rheological and digestive characteristics of soy protein isolate gel induced by lactic acid bacteria. Journal of Food Engineering 292:110243. doi:10.1016/j.jfoodeng.2020.110243.
- Yasar, S., R. Tosun, and Z. Sonmez. 2020. Fungal fermentation inducing improved nutritional qualities associated with altered secondary protein structure of soybean meal determined by FTIR spectroscopy. Measurement 161:107895. doi:10.1016/j.measurement.2020.107895.
- Zahra, A., M. Johanne, R. A.-U. Blanca, S. Stéphane, M. Mathieu, and L. Monique. 2022. Physico-chemical properties and sensorial appreciation of a new fermented probiotic beverage enriched with pea and rice proteins. Plant Foods for Human Nutrition 77:112–20. doi:10.1007/s11130-021-00944-1.
- Zhai, Q., L. Yang, J. Zhao, H. Zhang, F. Tian, and W. Chen. 2018. Protective effects of dietary supplements containing probiotics, micronutrients, and plant extracts against lead toxicity in mice. Frontiers in Microbiology 9:2134. doi:10.3389/fmicb.2018.02134.
- Zhang, C., Y. Zhang, G. Liu, W. Li, S. Xia, H. Li, and X. Liu. 2020. Effects of soybean protein isolates and peptides on the growth and metabolism of Lactobacillus rhamnosus. Journal of Functional Foods 77:104335. doi:10.1016/j.jff.2020.104335.
- Zhao, S. 2019. Regulatory Function of tartary buckwheat protein and Lactobacillus plantarum on blood lipid and intestinal flora in mice. Master Diss., Shanghai Institute of Technology.
- Zhao, Y., S. Liu, X. Han, Z. Zhou, and J. Mao. 2022. Combined effects of fermentation temperature and Saccharomyces cerevisiae strains on free amino acids, flavor substances, and undesirable secondary metabolites in huangjiu fermentation. Food Microbiology 108:104091. doi:10.1016/j.fm.2022.104091.
- Zhou, X., H. Du, M. Jiang, C. Zhou, Y. Deng, X. Long, and X. Zhao. 2021. Antioxidant effect of Lactobacillus fermentum CQPC04-fermented soy milk on d-galactose-induced oxidative aging mice. Frontiers in Nutrition 8:727467. doi:10.3389/fnut.2021.727467.
- Zhou, Y., B. Ouyang, M. Duan, X. Lv, and X. Zhou. 2022. Biological characteristics of the gluten-free sourdough system fermented by Lactobacillus plantarum ST-III and its effect on dough quality and nutritional value during freezing. Food Chemistry: X 14:100350. doi:10.1016/j.fochx.2022.100350.
- Zhu, Y., G. Chen, J. Diao, and C. Wang. 2023. Recent advances in exploring and exploiting soybean functional peptides—a review. Frontiers in Nutrition 10:1185047. doi:10.3389/fnut.2023.1185047.