Abstract
AML with mutated NPM1 occurs in all age groups. Yet, the mutational pattern is not extensively studied in the very old, which may hamper appropriate risk assessment. Herein we examined 22 cases of NPM1-mutated de novo AML in patients older than 75, with a median age of 84. All diagnostic samples were sequenced aiming for coverage of the most relevant AML-associated mutations. For comparison with younger patients, we used already published data on several cohorts. A total of 76 mutations including 50 different variants were identified in 16 recurrently mutated AML genes. Compared with younger patients, a significant enrichment of TET2 and SRSF2 was observed, together with a reduced frequency of DNMT3A mutations. Our results indicate that the mutational pattern may be different in the very old as compared to younger patients with NPM1-mutated AML.
The mutational spectrum of NPM1-mutated AML in patients above 75 years displays distinct features.
A significant enrichment of TET2 and SRSF2 mutations together with a reduced frequency of DNMT3A mutations was observed in the elderly.
NPM1 mutation is a secondary event in the development of AML in the very old.
Highlights
Introduction
Acute myeloid leukemia (AML) is a biologically heterogeneous disease [Citation1,Citation2] arising from clonal expansions of hematopoietic stem cells [Citation3]. With advances in technology, in particular high-throughput sequencing techniques, important insights into the molecular landscape of the disease have been gained, with increased understanding of the genetic basis of AML. In turn, this knowledge has generated a new approach to disease classification with prognostic implications [Citation4]. Certain mutations occur earlier than other in leukemogenesis before evidence of hematological malignancy. Such mutations are thought to provide a selective advantage for expansion of clonal populations preceding progression to AML [Citation5]. This phenomenon is known as clonal hematopoiesis of indeterminate potential (CHIP) or age-related clonal hematopoiesis (ARCH), and includes genes involved in epigenetic regulation such as DNMT3A, TET2, and ASXL1 [Citation6]. Others are involved in progression to overt AML, so called driver genes, of which NPM1 is one of the most important [Citation1,Citation4]. AML with mutated NPM1 is today recognized as a distinct entity in the revised World Health Organization (WHO) classification of myeloid neoplasms. Together with AML with biallelic CEBPA mutation, it represents the only definite WHO category defined by a single-gene mutation [Citation7]. NPM1-mutations are common in AML, especially in de novo AML, occurring in approximately 30% of all adult cases [Citation7–12]. They are heterozygous and almost always occur as a four-nucleotide insertion [Citation10]. Several types have been described of which type A is the most common, constituting 80% of NPM1 mutant AML [Citation13].
AML occurs in all age groups but is mainly a disease of the elderly with a mean and median age of 68 years and 71 years, respectively, at diagnosis [Citation14–16]. Elderly patients are sometimes thought to be too frail for intensive treatment, but many patients up to 80 years seem to tolerate this, which improves early death rates and long-term survival compared with palliation [Citation15–18]. Genetic markers such as somatic mutations occurring in NPM1 or other genes are not only important for classification, but also for risk stratification [Citation19]. However, their applicability for risk assessment is possibly more relevant for the younger and middle-aged patients, since the mutational spectrum is not extensively studied in the very old [Citation20]. Therefore, it is important to deepen the knowledge of AML-associated somatic mutations in elderly for optimal care of this group of patients, who often are treated outside clinical trials. The aim of the study was to explore the mutational spectrum in older (>75 years) patients with AML. We focused on AML with mutated NPM1 since it typically presents de novo [Citation7]. The cutoff 75 years was chosen since most patients up to this age are given intensive induction treatment in Sweden [Citation16]. We found significant differences regarding the frequency of certain mutations between older and younger patients.
Material and methods
Patients, samples and immunophenotyping
The study included 22 patients older than 75 years diagnosed with NPM1-mutated AML at the department of Pathology at Lund University Hospital, Sweden, between November 2013 and June 2019. Samples from bone marrow (BM); 16 patients, or peripheral blood (PB); 6 patients, were collected and analyzed. DNA was isolated from mononuclear cells (LymphoprepTM, Abbott, Abbott Park, Illinois) using QIAamp DNA Blood Mini Kit (Qiagen, Germantown, Maryland) according to the manufacturer’s protocol. For one BM sample, DNA was captured from microscopic glass slides. Immunophenotyping was performed by multicolor flow cytometry as previously described [Citation21].
The study was approved by the Regional Ethical Review Board at Lund University (diary number 2014/505 and diary number 2017/850).
Next generation sequencing (NGS)
The Illumina Trusight Myeloid Sequencing Panel (Illumina, San Diego, CA) was used, complemented with a spike-in library for FLT3 and CEBPA to improve coverage in GC-rich areas. For Trusight Myeloid library preparation, 50 ng DNA was used from BM or PB. An additional 50 ng was used to amplify FLT3 and CEBPA with the Q5 Hot Start High-Fidelity DNA Polymerase (New England Biolabs, Ipswich, Massachusetts) and the Q5 High GC Enhancer. The following primers were used for FLT3 amplification: FLT3_F 5′-AAC TGT GCC TCC CAT TTT TG-3′ & FLT3_R 5′-CCT GAT TGT CTG TGG GGA GT-3′ and CEBPA amplification: CEBPA_F 5′-AGG CAC CGG AAT CTC CTA GT-3′ & CEBPA_R 5′-CCT GCC GGG TAT AAA AGC TG-3′. Nextera XT DNA Library Prep (Illumina) was used to generate a spike-in library.
Paired-end sequencing was performed on a NextSeq550 (Illumina) using the Mid Output Kit v2.5 (300 cycles) for both the Trusight Myeloid Sequencing Panel libraries and the spike-in libraries. Reads were aligned to the human genome (hg19) using bwa 0.7.15 [Citation22]. Variant detection was performed with freebayes 1.0.2 [Citation23] and MuTect2 (GATK 3.7) [Citation24], tumor only mode, supplied with the COSMIC (v54) [Citation25] and dbSNP (build 138) [Citation26] databases. Pindel 0.9.5b9 was used to detect FLT3-ITD [Citation27].
Variants <5% variant allele frequency (VAF) or <700 reads were discarded, as well as variants classified as artifacts. Variants were annotated with their COSMIC and dbSNP information. Variants were manually reviewed and classified according to evidence in the literature. Rare or previously unreported variants were classified according to their predicted consequence and the classification of similar variants. Variants classified as pathogenic, likely pathogenic, and a subset classified as uncertain significance, were considered clinically relevant. Sequencing data are available in Supplementary Table 1.
Comparative studies
To compare the mutational landscape in older NPM1-mutated AML patients with that of younger patients, data were retrieved from two large studies; one by Papaemmanuil et al. [Citation4] and the other by Ivey et al. [Citation28]. By using a panel of 111 genes Papaemmanuil et al. characterized 1540 AML patients (from three different clinical trials), including 418 with NPM1 mutation. 89% of all 1540 patients from two of the cohorts were younger than 66 while 11% from the third cohort were between 58–84 years. The mutational profiles for the NPM1-mutated cases were extracted from their Supplementary data [Citation4]. Ivey et al. sequenced 223 NPM1-mutated AML patients younger than 68 with a panel of 51 genes. The mutation frequencies were retrieved from their publication [Citation28]. For comparison with a cohort of elderly patients the recently published data by Renaud et al. was used [Citation29]. By using a panel of 36 genes, they characterized the mutational spectrum in AML patients older than 80 years (mean 83 years), including 17 with NPM1 mutation selected for this comparison [Citation29].
Statistical analyses
Data was analyzed using IBM SPSS version 25 and the R software version 3.6.1. Comparisons between different studies were evaluated by Fisher’s exact test. p < .05 was considered statistically significant. Regression analysis was performed to assess the correlation between blast counts and NPM1 VAF%.
Results
Patient characteristics
Of the 22 patients, 16 had the NPM1 type A mutation, 3 type B and 3 other types. The mean age at diagnosis was 83 years. Further characteristics are presented in . All patients were considered de novo AML based on available clinical, immunophenotypical and cytogenetical information. None of the patients had a complex karyotype, but minor cytogenetic aberrations were found in three patients including trisomy 8, which is a common aberration in patients with AML with mutated NPM1 [Citation7]. Another patient had a del(5q) but no other signs of myelodysplasia. The third patient displayed 45,X,-X,inv(9)(p11q13)c in 25/25 mitoses. -X could be constitutional variant, although no karyotyping on normal tissue was performed to confirm this possibility. Inv(9)(p11q13) is considered a constitutional variant.
Table 1. Characteristics of the 22 AML patients older than 75 with mutated NPM1.
Treatment strategies were heterogeneous; only two patients (76 and 77 years respectively) received intensive chemotherapy with curative intent, while other age adjusted treatment strategies were applied for the remainder including best supportive care without cytotoxic therapy (). The mean overall survival was 232 days (median 82; range 1–1622). Only one patient was alive at the end of the study in December 2020 at day 1622. Detailed characteristics for the two long-term survivors are presented in .
Table 2. Detailed characteristics of the two patients receiving intensive chemotherapy with daunorubicin and cytarabine (DA).
Characterization of AML-associated mutations and immunophenotype
A total of 76 mutations (50 different variants) were identified in 16 genes in the diagnostic samples from the 22 AML patients with NPM1 mutation (). The most common functional class of mutations involved DNA methylation genes; 15 TET2 (5 samples contained 2 different TET2 mutations), 6 DNMT3A and 6 IDH2, followed by FLT3 mutations (kinase signaling) in 9 samples (including 6 ITD and 3 TKD) and the spliceosome gene SRSF2 (5 samples). The mean number of panel mutations per patient was three.
Figure 1. AML-associated mutations, immunophenotypic characteristics and karyotype of 22 NPM1-mutated AML patients. Each column represents a single patient. ‘APL-like’, ‘Acute promyelocytic leukemia’-like phenotype i.e. CD34-/HLA-DR-. Five patients displayed two different TET2 mutations and one patient two different BCOR mutations.
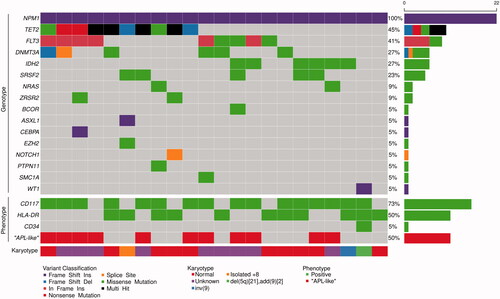
AML with mutated NPM1 often displays a characteristic immunophenotype without expression of CD34. Two major subgroups have been described; one with frequent expression of monocytic markers including CD64, CD14, CD11b and HLA-DR, most often in the absence of CD117, and the other with myeloid features including expression of CD117, but lacking monocytic differentiation [Citation7,Citation30]. CD34-/HLA-DR- is a distinctive feature of the myeloid subgroup and CD34-/HLA-DR + of the monocytic [Citation30,Citation31]. NPM1-mutated AML lacking both CD34 and HLA-DR is sometimes called acute promyelocytic leukemia-like (‘APL-like’), since the absence of these antigens mimics the characteristic phenotype of APL [Citation31]. Eleven patients showed an ‘APL-like’ phenotype of which ten belonged to the myeloid subgroup (). Co-mutation of either TET2 or IDH2 were seen in 10/11 of the ‘APL-like’ cases, whereas 6/11 of the remaining ‘non-APL-like’ cases displayed either of them. TET2 and IDH2 mutations were mutually exclusive.
The mutational profile in the elderly display distinct features
Compared to younger AML patients [Citation4,Citation28] a significant enrichment of mutations in TET2 and SRSF2 was observed (p < .001), while the frequency of DNMT3A mutations was significantly reduced (p < .001) (). There was a slight trend toward more IDH2 mutations in the elderly, but the difference did not reach statistical significance. For FLT3-ITD, no significant difference could be observed. Comparing data from the present study with those from the recently published study by Renaud et al. [Citation29] revealed similar mutation frequencies, with the possible exception of FLT3-ITD (). ASXL1 mutations, typically associated with secondary AML [Citation32], appeared equally rare (≤6%) in both older and younger patients (data not shown). For the remainder of the mutations in , the low frequency precluded meaningful analysis.
Figure 2. Gene mutation frequencies (%) of TET2, DNMT3A, IDH2, FLT3-ITD and SRSF2 in NPM1-mutated AML comparing data from the present study (n = 22; leftmost) with those of another cohort of older patients by Renaud et al. [Citation29] (n = 17; middle left) and two series of younger AML patients by Papaemmanuil et al. [Citation4] (n = 418, middle right) and Ivey et al. [Citation28] (n = 223, rightmost). p-values as determined by Fisher’s exact test are shown for comparisons of the gene mutation frequencies with those of the present study.
![Figure 2. Gene mutation frequencies (%) of TET2, DNMT3A, IDH2, FLT3-ITD and SRSF2 in NPM1-mutated AML comparing data from the present study (n = 22; leftmost) with those of another cohort of older patients by Renaud et al. [Citation29] (n = 17; middle left) and two series of younger AML patients by Papaemmanuil et al. [Citation4] (n = 418, middle right) and Ivey et al. [Citation28] (n = 223, rightmost). p-values as determined by Fisher’s exact test are shown for comparisons of the gene mutation frequencies with those of the present study.](/cms/asset/d27a7f51-e0ef-4e46-a9e9-8fa59032b31d/ilal_a_1894650_f0002_c.jpg)
NPM1 mutation is a secondary event in the development of AML
Clonal hematopoiesis is often thought to precede the development of AML. Therefore, we compared the allelic frequency (VAF) of NPM1 with genes involved in DNA methylation (DNMT3A, TET2 and IDH2) associated with such age-related proliferation. As seen in , the majority (19/22) of patients had a coexisting mutation in either DNMT3A, TET2 or IDH2. For 17 of these 19 patients, VAF% for at least one of the mutations in genes involved in DNA methylation was higher than for NPM1. For the remaining two (#4 and #7) VAF% for NPM1 and either DNMT3A or IDH2 were at the same level. This suggests that for the majority of patients, the NPM1 mutation was acquired in a preexisting clone harboring mutations in DNA methylation genes.
Figure 3. Variant allele frequency (VAF%) of coexisting mutations in DNA methylation genes (DNMT3A, TET2 and IDH2) compared to NPM1 VAF% among the 22 AML patients. Three patients (#1, 11 and 19) lacked a mutation in either of these genes. For TET2, two different mutations were found in five patients, displayed by different symbols (patients #2, 5, 10, 12 and 20). All NPM1 VAF% values are connected with a gray line for easier read-out.
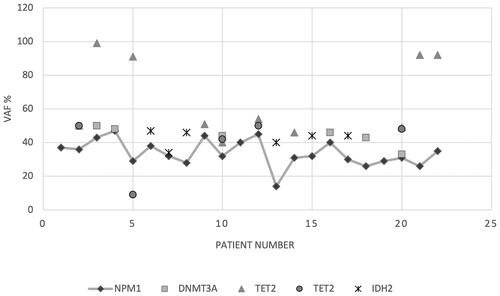
No correlation between blast frequency and NPM1 variant allele frequency (VAF)
To investigate the relation between blast counts and NPM1 VAF% in BM, regression analysis was performed with no observed correlation (R2=0.190) (Supplementary Figure 1). NPM1 VAFs ranged from 14–44% () and the BM-blast percentages from 10–90% (). For five diagnostic samples, NPM1 VAF% was approximately half or less than half of the blast count, but for the majority, NPM1 VAF% was above half, suggesting that the mutation was present in maturing leukemic cells and not only within the blast population.
Discussion
The mutational profile of AML is important for risk stratification, but the current knowledge is not necessarily translatable to the very old patients. In fact, surprisingly few studies have addressed the spectrum and relevance of the mutational landscape in this age group despite AML being primarily a disease of the elderly [Citation20]. Therefore, the aim of this study was to explore the molecular landscape in older (>75 years) NPM1-mutated AML patients. Patients with NPM1 mutation were chosen since these leukemias are often associated with de novo disease [Citation7]. Also, we deliberately sought to exclude secondary AML, which increases with age (up till approximately 80 years [Citation17,Citation33]), and would add complexity to the comparison, even though earlier studies have shown a lack of prognostic value of secondary AML in the elderly [Citation33]. Our results show a different mutational spectrum in older patients with de novo AML with mutated NPM1 as compared to published data on younger individuals.
Published comparisons between older and younger patients are limited but support our findings of certain differences regarding AML-associated mutations. Metzeler et al. found higher frequencies of TET2, SRSF2, RUNX1, IDH2, TP53 and ASXL1 mutations in the elderly and fewer WT1 mutations in a large cohort of AML patients [Citation34]. However, the age cutoff was 60 years, compared to 75 used in our comparison precluding firm conclusions about the very old. The high frequency of TET2, SRSF2 and ASXL1 mutations was confirmed by the same group in another study with an age cutoff similar to ours (>75) [Citation20]. However, in this study only intensively treated patients were included which may have biased the results. Also, Silva et al. found a high frequency of DNMT3A, SRSF2, IDH1/2, RUNX1, TET2 and ASXL1 mutations in a cohort of elderly AML patients 65 to 90 years, although no comparison with younger patients was performed [Citation35]. Using an unselected material of AML patients older than 80, Renaud et al. found more mutations in TET2, SRSF2, ASXL1, TP53 and RUNX1 as compared to younger patients [Citation29].
Among the NPM1-mutated leukemias older than 75 in the present study, TET2, FLT3, DNMT3A, IDH2 and SRSF2, in descending order of frequency, were the most frequently mutated genes. Most evaluable patients had a normal karyotype and none displayed a complex karyotype, which makes secondary AML unlikely. Furthermore, the immunophenotypes of our cases were typical of de novo AML with mutated NPM1, i.e. absence of CD34-expression in 21/22 diagnostic samples. It has been reported that AML with mutated NPM1 can be divided into different immunophenotypical subgroups, i.e. myeloid and monocytic, and that most of the myeloid cases display a phenotype reminiscent of APL [Citation30,Citation31,Citation36]. These characteristic phenotypes may be caused by mutation of different progenitor cells [Citation30]. Our frequency of ‘APL-like’ cases with the associated increase of TET2 and IDH2 mutations are in line with an earlier study by Mason et al. [Citation31]. They demonstrated a superior outcome for patients with the ‘APL-like’ subtype carrying TET2 and IDH1/2 mutations [Citation31] which could not be investigated in our study due to small sample size.
The significant enrichment of TET2 mutations in the elderly contrasted to the significant reduced frequency of DNMT3A variants, both considered CHIP-mutations () [Citation4,Citation28,Citation29]. Several types of DNMT3A mutations have been described in AML of which the ones affecting codon R882 are the most common [Citation37,Citation38] and associated with adverse prognosis [Citation39]. In contrast to Renaud et al., who found no DNMT3A R882 mutations among their DNMT3A and NPM1-co-mutated elderly patients [Citation29], we noticed the R882 mutation in 50% of our DNMT3A-mutated cases. This is similar to what has been reported by others in younger cohorts (irrespective of NPM1-mutation) [Citation4,Citation37] and distinctly different from the much lower rate (17%) in CHIP and in elderly AML patients independently of NPM1 (15%) [Citation29,Citation40]. The high VAF% of a coexisting methylation gene mutation suggests that the NPM1 mutation was a secondary event in most patients, acquired in a founding clone harboring mutations in DNA methylation genes, in line with the current view of preleukemic hits and driver genes [Citation4,Citation5,Citation41].
Interestingly, a significant increase of SRSF2 mutations was seen in the elderly. The SRSF2 mutations were co-mutated with either TET2 or with IDH2 but not with DNMT3A. Mutations in the spliceosome gene SRSF2 are highly associated with secondary AML and myelodysplastic syndrome [Citation32]. On the other hand, SRSF2 mutations are also associated with older age and CHIP [Citation40], which would offer an explanation for the observed age difference. However, the allele frequency for SRSF2 mutations was lower than or equal to that of TET2 or IDH2 suggesting a secondary event rather than an early mutation. Although not always mutually exclusive with NPM1 mutation, it is interesting to note that co-mutations of SRSF2 and NPM1 are rarely described in the literature, probably reflecting their importance as drivers of secondary or therapy-related AML on the one hand (SRSF2) and de novo AML on the other (NPM1) [Citation32]. ASXL1, another mutation associated with secondary AML [Citation32], but also with CHIP [Citation40], showed no age difference compared to SRSF2, with infrequent mutations in both younger and older patients [Citation4,Citation28]. The low frequencies of ASXL1 mutations in these presumed de novo NPM1 mutant AML contrast to the much higher numbers (32%) in secondary AML reported by Coleman-Lindsley et al. [Citation32].
The absence of correlation between the blast frequency in BM and VAF% for NPM1 contrasts to a study by Toth et al. who found a moderate correlation for NPM1, but not for FLT3, KRAS, NRAS and NPM1 analyzed together [Citation42]. Assuming a heterozygous NPM1 mutation, without copy-number aberrations and with presence exclusively in leukemic blasts, VAF% should equal half of the blast count percentages. However, in some cases the results indicated that the mutation must be present in maturing leukemic cells and not only in the leukemic blasts, since VAF% was close to or equal to the blast count. In a few cases the NPM1 mutation did not even seem to be included in the whole blast population since the VAF% was below half of the blast percentage.
A limitation of our study is its small size, including only 22 patients. Nevertheless, our results probably reflect true differences between younger and older patients since similar findings were reported by Renaud et al. [Citation29], with the possible exception of FLT3-ITD, which in their study more resembled the occurrence in the young groups (). Old AML patients are not a homologous group and some may benefit from intensive treatment [Citation15]. In general, the prognosis is highly age-dependent and even though AML is mainly a disease of the elderly they are often underrepresented in clinical trials. Some typical secondary-type AML mutations such as SRSF2 and ASXL1 are present at high frequency in patients 60 years or older, even in presumed de novo AML [Citation32], conferring a negative prognostic impact. However, the prognostic significance of these secondary-type mutations and others have not been examined in the very old above 75 years. Other studies have shown that subgroups of elderly AML patients (>75) carrying certain mutations, like IDH1, have a poor prognosis [Citation20].
In conclusion, we found a different mutational spectrum between younger patients and the very old with a significant increase of TET2 and SRSF2 mutations as well as a significant decrease of DNMT3A mutations. Further studies need to be performed to elucidate the prognostic impact of these observed differences and whether a therapeutic approach based on these findings could improve outcome.
Author contributions
LP, VL, GJ and ME conceived and designed the study. VL and GJ provided samples and clinical information. BH performed the bioinformatics. LP and ME analyzed data and wrote the report. All authors approved the final version of the report for publication.
Patient consent
Trained health professionals provided written and oral information to all patients who signed informed consent.
GLAL-2020-1615-File008.pdf
Download PDF (257.6 KB)GLAL-2020-1615-File007.pdf
Download PDF (337.9 KB)Acknowledgments
The authors would like to thank Ulf Strömberg, Region Halland, for statistical support, and the Center for Translational Genomics, Lund University, for DNA sequencing.
Disclosure statement
The authors report no conflict of interest.
Additional information
Funding
References
- Ley TJ, Miller C, Ding L, Cancer Genome Atlas Research N, et al. Genomic and epigenomic landscapes of adult de novo acute myeloid leukemia. N Engl J Med. 2013;368(22):2059–2074.
- Grimwade D, Ivey A, Huntly BJ. Molecular landscape of acute myeloid leukemia in younger adults and its clinical relevance. Blood. 2016;127(1):29–41.
- Short NJ, Rytting ME, Cortes JE. Acute myeloid leukaemia. Lancet. 2018;392(10147):593–606.
- Papaemmanuil E, Gerstung M, Bullinger L, et al. Genomic classification and prognosis in acute myeloid leukemia. N Engl J Med. 2016;374(23):2209–2221.
- Shlush LI, Zandi S, Mitchell A, HALT Pan-Leukemia Gene Panel Consortium, et al. Identification of pre-leukaemic haematopoietic stem cells in acute leukaemia. Nature. 2014;506(7488):328–333.
- Steensma DP, Bejar R, Jaiswal S, et al. Clonal hematopoiesis of indeterminate potential and its distinction from myelodysplastic syndromes. Blood. 2015;126(1):9–16.
- Swerdlow SC, Harris NL, et al. WHO Classification of Tumours of Haematopoietic and Lymphoid Tissues. 4th ed. Lyon, France: IARC Press; 2017.
- Chou WC, Tang JL, Lin LI, et al. Nucleophosmin mutations in de novo acute myeloid leukemia: the age-dependent incidences and the stability during disease evolution. Cancer Res. 2006;66(6):3310–3316.
- Falini B, Martelli MP, Bolli N, et al. Acute myeloid leukemia with mutated nucleophosmin (NPM1): is it a distinct entity? Blood. 2011;117(4):1109–1120.
- Falini B, Mecucci C, Tiacci E, GIMEMA Acute Leukemia Working Party, et al. Cytoplasmic nucleophosmin in acute myelogenous leukemia with a normal karyotype. N Engl J Med. 2005;352(3):254–266.
- Falini B, Nicoletti I, Martelli MF, et al. Acute myeloid leukemia carrying cytoplasmic/mutated nucleophosmin (NPMc + AML): biologic and clinical features. Blood. 2007;109(3):874–885.
- Juliusson G, Jadersten M, Deneberg S, et al. The prognostic impact of FLT3-ITD and NPM1 mutation in adult AML is age-dependent in the population-based setting. Blood Adv. 2020;4(6):1094–1101.
- Thiede C, Koch S, Creutzig E, 0for the Deutsche Studieninitiative Leukämie (DSIL), et al. Prevalence and prognostic impact of NPM1 mutations in 1485 adult patients with acute myeloid leukemia (AML). Blood. 2006;107(1):4011–4020.
- National Cancer Institute. Surveillance, Epidemiology, and End Results Program, 2020. [cited 2021 Feb 24]. Available from: http://seer.cancer.gov/.
- Juliusson G, Antunovic P, Derolf A, et al. Age and acute myeloid leukemia: real world data on decision to treat and outcomes from the Swedish Acute Leukemia Registry. Blood. 2009;113(18):4179–4187.
- Juliusson G. Older patients with acute myeloid leukemia benefit from intensive chemotherapy: an update from the Swedish Acute Leukemia Registry. Clin Lymphoma Myeloma Leuk. 2011;11(Suppl 1):S54–S59.
- Juliusson G, Hagberg O, Lazarevic VL, et al. Improved survival of men 50 to 75 years old with acute myeloid leukemia over a 20-year period. Blood. 2019;134(18):1558–1561.
- Lazarevic VL, Bredberg A, Lorenz F, Swedish AML Registry, et al. Acute myeloid leukemia in very old patients. Haematologica. 2018;103(12):e578–e580.
- Angenendt L, Rollig C, Montesinos P, et al. Chromosomal abnormalities and prognosis in NPM1-mutated acute myeloid leukemia: a pooled analysis of individual patient data from nine international cohorts. J Clin Oncol. 2019;37(29):2632–2642.
- Prassek VV, Rothenberg-Thurley M, Sauerland MC, et al. Genetics of acute myeloid leukemia in the elderly: mutation spectrum and clinical impact in intensively treated patients aged 75 years or older. Haematologica. 2018;103(11):1853–1861.
- Pettersson L, Leveen P, Axler O, et al. Improved minimal residual disease detection by targeted quantitative polymerase chain reaction in Nucleophosmin 1 type a mutated acute myeloid leukemia. Genes Chromosomes Cancer. 2016;55(10):750–766.
- Li H. Aligning sequence reads, clone sequences and assembly contigs with BWA-MEM. arXiv preprint arXiv: 13033997v1[q-bioGN] 2013.
- Garrison E MG. Haplotype-based variant detection from short-read sequencing. arXiv preprint arXiv:12073907v2 [q-bioGN] 2012.
- Benjamin D, Sato T, Cibulskis K, et al. Calling Somatic SNVs and Indels with Mutect2. bioRxiv 2019:861054.
- Tate JG, Bamford S, Jubb HC, et al. COSMIC: the catalogue of somatic mutations in cancer. Nucleic Acids Res. 2019;47(D1):D941–D947.
- Smigielski EM, Sirotkin K, Ward M, et al. dbSNP: a database of single nucleotide polymorphisms. Nucleic Acids Res. 2000;28(1):352–355.
- Ye K, Schulz MH, Long Q, et al. Pindel: a pattern growth approach to detect break points of large deletions and medium sized insertions from paired-end short reads. Bioinformatics. 2009;25(21):2865–2871.
- Ivey A, Hills RK, Simpson MA, et al. Assessment of minimal residual disease in standard-risk AML. N Engl J Med. 2016;374(5):422–433.
- Renaud L, Nibourel O, Marceau-Renaut A, et al. Comprehensive molecular landscape in patients older than 80 years old diagnosed with acute myeloid leukemia: a study of the French Hauts-de-France AML observatory. Am J Hematol. 2019;94(1):E24–E27.
- Liu YR, Zhu HH, Ruan GR, et al. NPM1-mutated acute myeloid leukemia of monocytic or myeloid origin exhibit distinct immunophenotypes. Leuk Res. 2013;37(7):737–741.
- Mason EF, Kuo FC, Hasserjian RP, et al. A distinct immunophenotype identifies a subset of NPM1-mutated AML with TET2 or IDH1/2 mutations and improved outcome. Am J Hematol. 2018;93(4):504–510.
- Lindsley RC, Mar BG, Mazzola E, et al. Acute myeloid leukemia ontogeny is defined by distinct somatic mutations. Blood. 2015;125(9):1367–1376.
- Hulegardh E, Nilsson C, Lazarevic V, et al. Characterization and prognostic features of secondary acute myeloid leukemia in a population-based setting: a report from the Swedish Acute Leukemia Registry. Am J Hematol. 2015;90(3):208–214.
- Metzeler KH, Herold T, Rothenberg-Thurley M, et al. Spectrum and prognostic relevance of driver gene mutations in acute myeloid leukemia. Blood. 2016;128(5):686–698.
- Silva P, Neumann M, Schroeder MP, et al. Acute myeloid leukemia in the elderly is characterized by a distinct genetic and epigenetic landscape. Leukemia. 2017;31(7):1640–1644.
- Mason EF, Hasserjian RP, Aggarwal N, et al. Blast phenotype and comutations in acute myeloid leukemia with mutated NPM1 influence disease biology and outcome. Blood Adv. 2019;3(21):3322–3332.
- Ley TJ, Ding L, Walter MJ, et al. DNMT3A mutations in acute myeloid leukemia. N Engl J Med. 2010;363(25):2424–2433.
- Park DJ, Kwon A, Cho BS, et al. Characteristics of DNMT3A mutations in acute myeloid leukemia. Blood Res. 2020;55(1):17–26.
- Kumar D, Mehta A, Panigrahi MK, et al. DNMT3A (R882) mutation features and prognostic effect in acute myeloid leukemia in Coexistent with NPM1 and FLT3 mutations. Hematol Oncol Stem Cell Ther. 2018;11(2):82–89.
- Jaiswal S, Fontanillas P, Flannick J, et al. Age-related clonal hematopoiesis associated with adverse outcomes. N Engl J Med. 2014;371(26):2488–2498.
- Morita K, Wang F, Jahn K, et al. Clonal evolution of acute myeloid leukemia revealed by high-throughput single-cell genomics. Nat Commun. 2020;11(1):5327.
- Toth LN, Green D, Peterson J, et al. Variant allele frequencies do not correlate well with myeloblast counts in a clinically validated gene sequencing panel for routine acute myeloid leukemia workup. Leuk Lymphoma. 2019;60(10):2415–2422.