Abstract
Grape seed proanthocyanidin extract (GSPE) plays a significant role in body health, including improving antioxidant capacity and maintaining lipid metabolism stability. However, whether dietary GSPE supplementation can improve lipid metabolism in finishing pigs remains unclear. Here 18 castrated male Duroc × Landrace × Yorkshire finishing pigs were randomly divided into three groups with six replicates and one pig per replicate. Pigs were fed a basal diet (control), a basal diet supplemented with 100 mg/kg GSPE, or a basal diet supplemented with 200 mg/kg GSPE for 30 days. Antioxidant analysis showed that dietary 200 mg/kg GSPE supplementation increased glutathione, total antioxidant capacity and glutathione peroxidase levels, and reduced malondialdehyde levels in serum, muscle and liver. Dietary 200 mg/kg GSPE supplementation also upregulated the mRNA and protein levels of nuclear-related factor 2 (Nrf2). Lipid metabolism analysis showed that dietary GSPE supplementation increased serum high-density lipoprotein cholesterol levels and reduced serum triglyceride and total cholesterol levels. Besides, GPSE upregulated the mRNA expression of lipolysis- and fatty acid oxidation-related genes downregulated the mRNA expression of lipogenesis-related genes, and activated the AMPK signal in finishing pigs. Together, we provided evidence that dietary GSPE supplementation improved the antioxidant capacity and lipid metabolism in finishing pigs.
Introduction
Metabolic syndrome (MS) is considered a worldwide epidemic with high socioeconomic costs and an increasing prevalence among children and young adults.Citation1 A large number of studies have shown that oxidative stress and abnormal lipid metabolism are important factors in the occurrence and development of MS.Citation1 With the misregulation of reactive oxygen species (ROS), oxidative stress occurs in the body.Citation2 It is essential for the body to maintain healthy homeostasis between the production and elimination of cellular ROS.Citation3 Oxidative stress is an important cause of many chronic diseases and is related to the excessive accumulation of free radicals. The antioxidant system is composed of enzymatic antioxidants including superoxide dismutase (SOD), catalase (CAT) and glutathione peroxidase (GPx) and non-enzymatic antioxidants, which can resist oxidative stress.Citation4–6 Oxidative stress is closely related to the excessive accumulation of lipids and lipid peroxidation. The main manifestations of abnormal lipid metabolism are the increase of serum triglyceride (TG), total cholesterol (T-CHO), low-density lipoprotein cholesterol (LDL-C) levels and the decrease of serum high-density lipoprotein cholesterol (HDL-C) levels. In recent years, plant extracts, such as resveratrol, eucommia polyphenols, rhodiola and salidroside,Citation7,Citation8 have been found to improve antioxidant capacity and lipid metabolism in the body.Citation9
Grape seed proanthocyanidin extract (GSPE) is a kind of plant-derived polyphenols isolated from grape seeds. GSPE is polymerized by different numbers of flavanol-catechin and epicatechin. Usually, the di-tetramer is called procyanidolic oligomer, and the pentamer or more is called procyanidolic polymers. GSPE is one of the most effective free radical scavengers discovered so far, especially oligomeric proanthocyanidins can scavenge free radicals and ROS in the body. Studies have shown that GSPE has antioxidant,Citation9 free radical scavenging,Citation10 anti-inflammatory, anti-tumour,Citation11 anti-radiation and anti-stress effects.Citation12 GSPE shows remarkable antioxidant capacity, and the antioxidant activity of GSPE is 50 times that of vitamin E and 20 times that of vitamin C.Citation13 As a manifestation of antioxidant function, GSPE has the potential to protect retinal tissue function by modulating oxidative stress and mitochondrial functionCitation14 and acts as an effective protector against oxidative stress-mediated pancreatic dysfunction in rats.Citation15 In addition, GPSE improves lipid metabolism by regulating the mRNA expression of lipid metabolism-related genes in rats and inhibits liver fat synthesis.Citation16 And as a health product, GPSE has been applied to prevent cardiovascular and cerebrovascular diseasesCitation17 and reduce blood lipid.Citation18 Although GSPE has been widely reported to improve antioxidant capacity and lipid metabolism in rodents, there is a distinct lack of human research. Compared to rodents, in terms of physiological function and the shape and size of organs, pigs are very similar to humans,Citation19 so pigs are the most suitable animal model for the anatomical understanding of the human body.
In our previous studies, we reported that adding GSPE to the diet during the whole fattening period could improve the antioxidant capacity of finishing pigs.Citation20 However, in order to further reduce costs, whether the addition of GSPE in the diet of finishing pigs in the later fattening stage can enhance antioxidant capacity remains unclear. In addition, it is also unknown whether dietary GSPE supplementation can improve lipid metabolism in finishing pigs. Therefore, the purpose of this study was to investigate the effects of adding GSPE on lipid metabolism and antioxidant capacity in the later fattening period of finishing pigs, and to clarify its mechanism.
Materials and methods
Ethics statement
All animal procedures were approved by the Animal Care Advisory Committee of Sichuan Agricultural University with the permission number of YYS20211220.
Experimental design and diets
GSPE was purchased from Tianjin Jianfeng Natural Product R&D Co., Ltd. (Tianjin, China), and the purity was higher than 95%. A total of 18 healthy castrated Duroc × Landrace × Yorkshire (DLY) pigs with an average body weight of 94.90 ± 0.2 kg were randomly divided into three groups with six replicates and one pig per replicate. The pigs were adaptively reared for seven days and then were randomly assigned to each of the three dietary treatments that consisted of a basal diet (control group),100 mg/kg GSPE group (a basal diet supplemented with 100 mg GSPE per kg of feed) and 200 mg/kg GSPE group (a basal diet supplemented with 200 mg GSPE per kg feed). GSPE was weighed and mixed with limestone, mineral premix, vitamin premix and amino acids, and then mixed with other substances in the formula. The basal diet () was formulated based on the Nutrient Requirements Council (Nutrient Requirements of Swine, 11th revised edition, 2012) for 100–135 kg pigs. Pigs were fed in an independent pen with the same size (2.0 m × 3.0 m) and housed in a room. Pigs were fed three times per day and free access to clean drinking water throughout the experimental period. The room temperature was maintained at 20–22 °C and relative humidity was controlled at 65–75%. The experiment lasted 30 days.
Table 1. Feed ingredients and nutrient composition of the basal diet, % as fed basis (air-dry basis).
Carcase traits and sample collection
At the end of the experiment, after 12h fasting, blood samples were obtained by jugular venipuncture. Blood samples were centrifuged at 3500 × g for 10 minutes after being staticstable for 30 minutes. The supernatant was then gathered and kept at −20 °C until analysis. After the blood samples were collected, the pigs were then transported one by one to a place about 300 metres away from the slaughterhouse. Then, every 15 minutes, one pig in each group was subjected to electric shock, blood drawing, hair removal, skin removal, and boning and splitting of the midline according to standard commercial procedures. For RNA and protein extraction, enzyme activity testing and malondialdehyde (MDA) content assessment, Longissimus dorsi (LD) muscle samples were taken from the right side of the carcase at the 10th rib and immediately frozen in liquid nitrogen and then kept at −80 °C for storage. The liver was quickly taken from the carcase’ left side, frozen in liquid nitrogen and then kept at −80 °C for storage.
Enzyme activity and malondialdehyde content
Approximately 100 mg of the liver and LD muscle samples were homogenized in pre-chilled 0.9% saline and then centrifuged at 2500 × g for 10 min at 4 °C, and the supernatant was aspirated. The supernatant was used to determine the activities of the T-AOC, T-SOD, CAT, GSH-Px and GSH and the content of malondialdehyde (MDA) in serum, LD muscle and liver using kits obtained from Nanjing Jiancheng Bioengineering Institute (Nanjing, China). Determination of total protein concentration was performed by BCA protein assay kit (Pierce) according to the manufacturer’s instructions.
Serum lipid metabolism analysis
The levels of TG, T-CHO, LDL-C and HDL-C in serum were determined using kits obtained from Nanjing Jiancheng Bioengineering Institute (Nanjing, China). The cholesterol esters and TG react with a specific reagent to eventually generate red bin compounds, which are measured by recording absorbance. HDL-C and LDL-C react with specific reagents and are measured by recording absorbance at a wavelength of 546 nm.
Real-time quantitative PCR
Total RNA from liver and LD muscle samples was extracted using RNAiso Plus reagent (TaKaRa, Dalian, China). Nano-Drop ND 2000c Spectrophotometer (Thermo Scientific, USA) was used to detect RNA concentration. One microgram of RNA was reverse transcribed into cDNA using PrimeScript RT reagent Kit with gDNA Eraser (TaKaRa, China). Real-time quantitative PCR was performed on a QuantStudio 5 or QuantStudio 6 Flex real-time PCR system (384-cell standard block) (Applied Biosystem, Foster, CA, USA) using TB Green Premix Ex Taq™ II (Tli RNaseH Plus) (TaKaRa, Dalian, China). Primer sequences used are listed in . The PCR cycling conditions were 45 cycles of 95 °C for 15 s and 60 °C for 15 s. Relative gene expression was evaluated using 2-ΔΔCt method and normalized to β-actin mRNA.
Table 2. Primers used in real-time quantitative PCR.
Western blot analysis
As previously described, the LD muscle and liver samples (about 0.1 g) were lysed in RIPA lysis buffer (Beyotime, Jiangsu, China) for 30 min at 4 °C.Citation21 The supernatant was collected after centrifugation at 12,000 × g for 15 min. The protein concentration was measured using a BCA protein assay kit (Pierce, Rockford, IL, USA) with a Nano-Drop ND 2000c spectrophotometer. Twenty micrograms of total proteins were denatured after boiling with 5 × protein loading buffer for 10 min, separated by SDS-PAGE, and transferred to a PVDF membrane (Millipore, Eschborn, Germany) using a wet Trans-Blot system (Bio-Rad). After blocking with bovine serum albumin (BSA) for 1.5 h at room temperature, membranes were incubated overnight with the primary antibodies at 4 °C, followed by the corresponding secondary antibodies (Cell Signalling Technology, Danvers, MA, USA). Primary antibodies specific for haem oxygenase 1 (HO-1) (Proteintech, cat. no. 66743-1-Ig), nuclear-related factor 2 (Nrf2) (Proteintech, cat. no. 66504-1-Ig), NAD(P)H quinone dehydrogenase 1 (NQO1) (Proteintech, cat. no. 67240-1-Ig), total AMP-activated protein kinase (AMPK) (Cell Signalling, cat. no. 5831S), phosphorylated AMPK (p-AMPK) (Cell Signalling, cat. no. 2535S) and β-actin (Trans, cat. no. HC201) were used. Target proteins were visualized using a clarity western ECL substrate (BioRad, Hercules, CA, USA) and a ChemiDoc XRS imaging system (Bio-Rad). The protein expression was quantified using Image Lab and normalised to β-actin expression.
Statistical analysis
Data expressed as means and the standard error of the mean (SEM) and were analyzed using SPSS 22.0 (SPSS, Chicago, IL, USA). All data were analyzed by a One-way analysis of variance followed by Tukey’s test. When P < 0.05, the difference was significant. When 0.05 ≤ P < 0.1, there was a trend.
Results
Antioxidant enzyme activities and MDA content
The antioxidant enzyme activities and the MDA content are listed in . Compared with the control group, 100 mg/kg GSPE reduced the MDA content in the LD muscle of finishing pigs (p < 0.05), and 200 mg/kg GSPE reduced the MDA content in the serum, LD muscle and liver of finishing pigs (p < 0.05). Data showed that 100 mg/kg GSPE increased CAT level in the serum, increased T-AOC and T-SOD levels in the LD muscle, and increased T-AOC and GSH-Px levels in the liver (p < 0.05). Similarly, 200 mg/kg GSPE increased T-AOC, T-SOD, GSH-Px and GSH levels in the serum, increased T-AOC, GSH-Px and GSH levels in the LD muscle, and increased T-AOC, T-SOD, GSH-Px, CAT and GSH levels in the liver (p < 0.05).
Table 3. Effect of dietary GSPE supplementation on antioxidant enzyme activities and MDA content of finishing pigs.
Antioxidant-related gene mRNA expression
The mRNA expression related to antioxidants is shown in . In LD muscle of finishing pigs, compared with the control group, 100 mg/kg GSPE increased SOD1, SOD2 and GR mRNA levels (p < 0.05), and 200 mg/kg GSPE increased CAT, GST and GR mRNA levels (p < 0.05) (). In the liver of finishing pigs, 100 mg/kg GSPE upregulated GR mRNA expression compared with the control group (p < 0.05), and 200 mg/kg GSPE increased SOD1, SOD2, GST and CAT mRNA levels (p < 0.05) ().
Figure 1. Effects of GSPE on antioxidant enzyme genes in LD muscle and liver. (A) The mRNA expression of antioxidant enzyme genes in LD muscle. (B) The mRNA expression of antioxidant enzyme genes in liver. Data are presented as means and SEM (n = 6). *p < 0.05, **p < 0.01, and ***p < 0.001. SOD1: superoxide dismutase 1; SOD2 : superoxide dismutase 2; GR: glutathione reductase; CAT: catalase; GST: glutathione S-transferase.
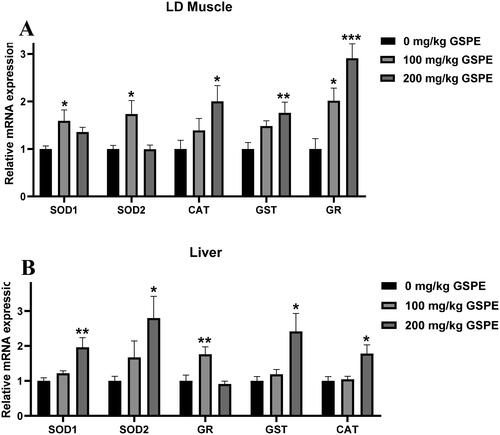
NRF2-ARE signalling pathway
The protein and mRNA levels of NRF2 pathway genes are shown in . In the liver, compared with the control group, 100 mg/kg GSPE increased the protein levels of NQO1 and HO-1 (P < 0.05), and 200 mg/kg GSPE increased NQO1, HO-1 and Nrf2 protein levels (P < 0.05) (). As shown in , in the LD muscle, 100 mg/kg GSPE increased the protein level of HO-1 compared with the control group (p < 0.05), and 200 mg/kg GSPE increased protein levels of NQO1, HO-1 and Nrf2 (p < 0.05). As shown in , 200 mg/kg GSPE increased NQO1, HO-1 and Nrf2 mRNA levels in the liver and the LD muscle (p < 0.05).
Figure 2. Effects of GSPE on expression of Nrf2 pathway genes. (A) NQO1, HO-1and Nrf2 protein levels in the liver were determined by Western blot analysis. (B) NQO1, HO-1and Nrf2 protein levels in LD muscle were determined by Western blot analysis. Equal loading was monitored with an anti-β-actin antibody. (C) NQO1, HO-1and Nrf2 mRNA levels in liver. (D) NQO1, HO-1and Nrf2 mRNA levels in LD muscle. Western blot analysis data are presented as means and SEM (n = 3). Real-time quantitative PCR data are presented as means and SEM (n = 6). *p < 0.05, **p < 0.01, and ***p < 0.001. NQO1: NAD(P)H, quinone oxidoreductase 1; Nrf2: NF-E2-related nuclear factor 2; HO-1: haem oxygenase-1.
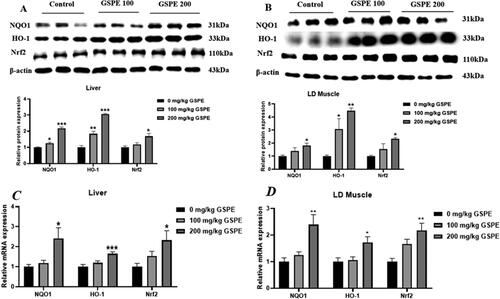
Serum lipid metabolism analysis
The lipid metabolism analysis of serum is listed in . Compared with the control group, 100 mg/kg of GSPE reduced the level of T-CHO (p < 0.05) and increased the level of HDL-C (p < 0.05), and 200 mg/kg of GSPE reduced the contents of TG and T-CHO (p < 0.05) and increased the content of HDL-C (p < 0.05).
Table 4. Effect of dietary GSPE supplementation on serum lipid metabolism of finishing pigs.
Lipid metabolism-related gene mRNA levels
The mRNA expression related to lipid metabolism is shown in . As shown in , in the LD muscle, compared with the control group, 100 mg/kg GSPE increased the mRNA levels of CPT1b, HSL, PGC-1α and Sirt1, and 200 mg/kg GSPE increased HSL, CPT1b, PPARα, PPARγ, PGC-1α and Sirt1 mRNA levels (p < 0.05). As shown in , in the liver, compared with the control group, 100 mg/kg GSPE increased the mRNA levels of CPT1a and PPARα (P < 0.05), and 200 mg/kg GSPE increased CPT1a, PPARα, PPARγ, HSL, PGC-1α and Sirt1 mRNA levels (P < 0.05).
Figure 3. Effects of GSPE on lipid metabolism gene expression in LD muscle and liver. (A) The mRNA expression of lipid metabolism genes in LD muscle, (B) The mRNA expression of lipid metabolism genes in liver. Data are presented as means and SEM (n = 6). *p < 0.05, **p < 0.01, and ***p < 0.001. CPT1a: carnitine palmitoyl-transferase-1a;CPT1b: carnitine palmitoyl transferase-1b; PPARα: peroxisome proliferator-activated receptor α; PPARγ: peroxisome proliferator-activated receptor γ; ACC: Acetyl-CoA carboxylase; HSL: hormone sensitive lipase; FAS: fatty acid synthase; PGC-1α: peroxisome proliferator-activated receptor gamma coactivator 1 alpha; Sirt1: sirtuin 1.
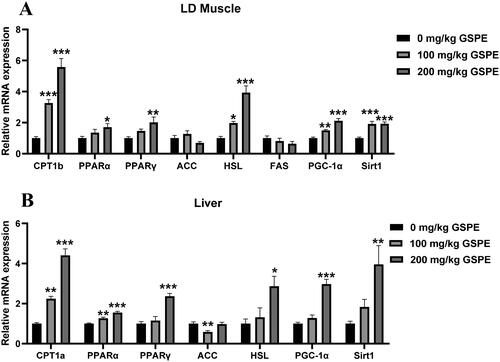
p-AMPK expression
Effect of GSPE on p-AMPK expression in LD muscle and liver is shown in . Dietary 100 mg/kg GSPE supplementation upregulated the protein expression of p-AMPK in the LD muscle compared to the control group (, p < 0.05). Dietary GSPE supplementation upregulated the protein expression of p-AMPK in the liver compared to the control group (, p < 0.05).
Figure 4. Effect of GSPE on p-AMPK expression in LD muscle and liver. (A) The p-AMPK protein expression in LD muscle. (B) The p-AMPK protein expression in liver. The p-AMPK protein level was monitored with AMPK protein. Data are presented as means and SEM (n = 3). *p < 0.05 and **p < 0.01. AMPK: AMP-activated protein kinase; p-AMPK: phospho-AMP-activated protein kinase.
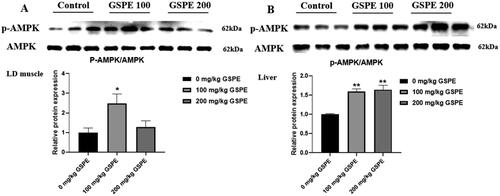
Discussion
In recent years, more and more attention has been paid to plant extracts in combating oxidative stress and disorders of lipid metabolism. GSPE has the characteristics of large yield, easy access and non-toxicity.Citation22 It was found that GSPE has excellent scavenging ability to superoxide free radicals and hydroxyl free radicals in fattening pigs.Citation13,Citation23 Therefore, we speculate that GSPE might maintain the body health by increasing the antioxidant capacity and improving the lipid metabolism.
It is well known that antioxidant capacity is closely related to free radicals in the body. In normal body activities, the production and extinction of free radicals in the body are in a state of dynamic equilibrium. Free radicals are constantly generated, and at the same time, the body’s antioxidant system will be cleared in time. When the free radicals in the body increase, it will disrupt the balance state and reduce the antioxidant capacity of the body.Citation24 The activities of T-AOC, GSH-Px, SOD, CAT and the content of MDA in the blood are important indicators reflecting the antioxidant capacity of the body.Citation6 The enzyme antioxidant system consisting primarily of T-AOC, GSH-Px, SOD and CAT is a relatively stable dynamic balance in the organism, which plays a vital role in scavenging free radicals, reducing oxidative damage and maintaining cellular structure.Citation25 In this study, the addition of 100 and 200 mg/kg GSPE increased T-AOC level and reduced MDA content in LD muscle of finishing pigs. The addition of 100 and 200 mg/kg GSPE also increased T-AOC and GSH-Px levels in liver of finishing pigs. We further examined the expression of related antioxidant genes. The results showed that the mRNA levels of antioxidant-related genes were also affected by dietary GSPE. We showed that dietary GSPE increased SOD1, SOD2, CAT, GST and GR mRNA levels in LD muscle of finishing pigs. The results showed that adding GSPE could reduce oxidative damage and improve the antioxidant capacity of finishing pigs, which are consistent with previous reports.Citation20,Citation26
Nrf2 signalling pathway plays an essential role in alleviating toxicant-induced oxidative stress and hepatotoxicity with antioxidant properties,Citation27 which is the defense pathway against external oxidation and stimuli.Citation28 Antioxidative response elements (AREs) are important protective cis-responsive elements in the body and play an important role in antioxidant effects.Citation29 Binding of Nrf2 to ARE increases the expressions of HO-1,Citation30 NQO1,Citation31 SOD,Citation32 GSH-Px,Citation33 and CAT,Citation34 which then increases the body’s resistance to oxidative stress and plays a protective role. Previous studies have demonstrated that GSPE attenuated lead-induced oxidative stress in rat kidneysCitation35 and aflatoxin B-induced oxidative damage in broilers by activating the Nrf2 signaling pathway,Citation36 and activation of the Nrf2 signalling pathway reduced renal damage in diabetic rats.Citation37 Subsequently, we investigated the protein expression of the downstream factors in the Nrf2 signalling pathway. As expected, here we showed that GSPE increased the protein expressions of HO-1, NQO1 and Nrf2 in liver and LD muscle of finishing pigs. Similar results were observed in the mRNA levels of HO-1 and NQO1. GSPE also increased the mRNA expression of HO-1, NQO1 and Nrf2 in liver and LD muscle of finishing pigs. Together, these results suggested that the positive effect of GSPE on the antioxidant capacity in finishing pigs might be achieved by the Nrf2 signalling pathway.
Disorders of lipid metabolism can lead to serious lipid-related diseases including obesity, non-alcoholic fatty liver disease and type 2 diabetes (T2DM).Citation38 Adipose tissue plays a very important role in the regulation of multiple key processes in energy homeostasis,Citation39 which is related to nutritional regulation, hormone regulation and homeostasis.Citation38 Obesity is a chronic metabolic disease caused by excessive accumulation and/or abnormal distribution of fat in the body due to the interaction of weight gain, genetic factors, environmental factors and other factors.Citation40 Normal lipid metabolism plays a vital role in the body, and excessive fat deposition is one of the manifestations of unhealthy metabolic obesity and is a key risk factor for the development of T2DM, the prevalence of which is increasing worldwide trend.Citation41 The results of this study showed that the addition of GSPE in the diet could significantly reduce the content of serum T-CHO and increase the content of serum HDL-C, suggesting that GPSE could effectively reduce fat accumulation and maintain the balance of glucose and lipid metabolism in the body, and had a significant effect of lowering blood lipid in finishing pigs.
Then, we examined key genes involved in lipid metabolism. The three critical steps of lipid metabolism are lipogenesis, lipolysis, and fatty acid oxidation. The significant organs for fatty acid synthesis and utilization include the liver, fat, and muscle tissue. To assess the effect of GSPE on lipogenesis in finishing pigs, the protein and mRNA levels associated with lipid metabolism were measured. When lipogenesis occurs, ACC catalyzes the carboxylation of acetyl-CoA, which is the rate-limiting step in de novo lipogenesis and catalyzes the carboxylation of acetyl-CoA to malonyl-CoA.Citation42 When lipid mobilization occurs, triglycerides are hydrolyzed into diglycerides and fatty acids. Our present study indicated that dietary 100 mg/kg GSPE supplementation could reduce ACC mRNA level in liver, suggesting that GSPE might inhibit fatty acid de novo synthesis, which might also be related to the decreased TG content in the liver. HSL is the rate-limiting enzyme for lipolysis and is the first step in controlling the breakdown of triglycerides.Citation43,Citation44 In this study, dietary 100 and 200 mg/kg GPSE supplementation increased mRNA expression of HSL in the LD muscle. In addition, PGC-1α, Sirt1, CPT1b and PPARα play central roles in fatty acid oxidation. PGC-1α is considered a metabolic co-activator responsible for inducing mitochondrial biogenesis and respiration, interacting with PPARα to stimulate the expression of genes such as CPT1b.Citation45 CPT-1b is the rate-limiting enzyme for fatty acid β-oxidation.Citation46 PPARα can regulate the mRNA expression level of CPT-1bCitation47 and regulate fatty acid oxidation associated with various target genes.Citation48 Sirt1 is required for the regulation of fatty acid oxidationCitation49 and is a functional regulator of PGC-1α. Sirt1 is involved in the expression of genes involved in glycolysis,Citation50 triglyceride synthesis and lipid metabolism and plays a beneficial role in regulating hepatic lipid metabolism and controlling hepatic oxidative stress.Citation51,Citation52 What is more, GSPE is involved in addressing fatal diseases such as cancer, cardiovascular and lipid metabolism diseases,Citation53 which has a certain promoting effect on lipid synthesis and decomposition. In the present study, the increased mRNA levels of PGC-1α, Sirt1, CPT1b and PPARα promoted fatty acid oxidation in the LD muscle and liver. The β-oxidation of fatty acids increases the production of pyruvate, and the oxidative decarboxylation of pyruvate increases the content of acetyl-CoA, which is the reason for the increase of total cholesterol in LD muscle.
Finally, we focused on the AMPK signalling pathway, which is a key pathway to regulate the lipid metabolism. AMPK is a key signalling molecule in lipid metabolism.Citation3 AMPK signalling pathway is involved in a variety of important metabolic regulations.Citation54,Citation55 AMPK can activate Sirt1 and regulate the activities of various energy metabolism-related transcription factors and co-regulators through deacetylation, including activation of PGC-1α, which is the main regulator of fatty acid β-oxidation and gluconeogenesis.Citation56 Therefore, the AMPK/Sirt1/PGC-1α signalling pathway is considered to be an energy homeostasis sensing system and plays an important regulatory role in energy metabolism. Here, we found that dietary GSPE supplementation increased p-AMPK (the activated form of AMPK) protein expression and PGC-1α and Sirt1 mRNA levels. The above results suggested that GSPE had a positive effect on AMPK activation, improvement of total cholesterol metabolism, and reduction of liver oxidative stress and lipid accumulation.
In conclusion, this study demonstrated that GSPE could increase the body’s antioxidant capacity through activating the Nrf2 signalling pathway when consumed in the diet. Additionally, GSPE could improve the lipid metabolism of serum, muscle, and liver, reducing lipid deposition. By considering the experimental results, adding 200 mg/kg GSPE to the diet seemed to be better.
Disclosure statement
No potential conflict of interest was reported by the author(s).
Additional information
Funding
References
- Santana-Gálvez J, Cisneros-Zevallos L, Jacobo-Velázquez DA. Chlorogenic acid: recent advances on its dual role as a food additive and a nutraceutical against metabolic syndrome. Molecules. 2017;22(3):358.
- Richard PU, Duskey JT, Stolarov S, et al. New concepts to fight oxidative stress. Expert Opin Drug Deliv. 2015;12(9):1527–1545.
- Guo ZY, Chen XL, Huang ZQ, et al. Dietary dihydromyricetin supplementation enhances antioxidant capacity and improves lipid metabolism in finishing pigs. Food Funct. 2021;12(15):6925–6935.
- Somogyi A, Rosta K, Pusztai P, et al. Antioxidant measurements. Physiol Meas. 2007;28(4):R41–R55.
- Milani P, Ambrosi G, Gammoh O, et al. SOD1 and DJ-1 converge at Nrf2 pathway. Oxid Med Cell Longev. 2013;2013:836760–836712.
- Yang H, Hua J, Wang C. Anti-oxidation and anti-aging activity of polysaccharide from Malus micromalus Makino fruit wine. Int J Biol Macromol. 2019;121:1203–1212.
- Kisková T, Kassayová M. Resveratrol action on lipid metabolism in cancer. Int J Mol Sci. 2019;20(11):2704.
- Ding H, Cao A, Li H, et al. Effects of Eucommia ulmoides leaf extracts on growth performance, antioxidant capacity and intestinal function in weaned piglets. J Anim Physiol Anim Nutr. 2020;104(4):1169–1177.
- Vitseva O, Varghese S, Chakrabarti S, et al. Grape seed and skin extracts inhibit platelet function and release of reactive oxygen intermediates. J Cardiovasc Pharmacol. 2005;46(4):445–451.
- Bayatli F, Akkuş D, Kilic E, et al. The protective effects of grape seed extract on MDA, AOPP, apoptosis and eNOS expression in testicular torsion. World J Urol. 2013;31(3):615–622.
- Tyagi A, Raina K, Gangar S, et al. Differential effect of grape seed extract against human non-small-cell lung cancer cells. Nutr Cancer. 2013;65 Suppl 1(0 1):44–53.
- Charradi K, Elkahoui S, Karkouch I, et al. Grape seed and skin extract alleviates high-fat diet-induced renal lipotoxicity and prevents copper depletion in rat. Appl Physiol Nutr Metab. 2013;38(3):259–267.
- Bagchi D, Sen CK, Ray SD, et al. Molecular mechanisms of cardioprotection by a novel grape seed proanthocyanidin extract. Mutat Res. 2003;523-524:87–97.
- Li L, Geng X, Tian L, et al. Grape seed proanthocyanidins protect retinal ganglion cells by inhibiting oxidative stress and mitochondrial alteration. Arch Pharm Res. 2020;43(10):1056–1066.
- Bashir N, Manoharan V, Miltonprabu S. Grape seed proanthocyanidins protects against cadmium induced oxidative pancreatitis in rats by attenuating oxidative stress, inflammation and apoptosis via Nrf-2/HO-1 signaling. J Nutr Biochem. 2016;32:128–141.
- Baselga-Escudero L, Bladé C, Ribas-Latre A, et al. Grape seed proanthocyanidins repress the hepatic lipid regulators miR-33 and miR-122 in rats. Mol Nutr Food Res. 2012;56(11):1636–1646.
- Del Bas JM, Crescenti A, Arola-Arnal A, et al. Intake of grape procyanidins during gestation and lactation impairs reverse cholesterol transport and increases atherogenic risk indexes in adult offspring. J Nutr Biochem. 2015;26(12):1670–1677.
- Pons Z, Guerrero L, Margalef M, et al. Effect of low molecular grape seed proanthocyanidins on blood pressure and lipid homeostasis in cafeteria diet-fed rats. J Physiol Biochem. 2014;70(2):629–637.
- Pabst R. The pig as a model for immunology research. Cell Tissue Res. 2020;380(2):287–304.
- Xu M, Chen XL, Chen DW, et al. Effects of dietary apple polyphenol supplementation on carcass traits, meat quality, muscle amino acid and fatty acid composition in finishing pigs. Food Funct. 2019;10(11):7426–7434.
- Yamakoshi J, Saito M, Kataoka S, et al. Safety evaluation of proanthocyanidin-rich extract from grape seeds. Food Chem Toxicol. 2002;40(5):599–607.
- Halliwell B, Gutteridge JM, Cross CE. Free radicals, antioxidants, and human disease. J Lab Clin Med. 1992;119(6):598–620.
- Burke NC, Scaglia G, Boland HT, et al. Influence of two-stage weaning with subsequent transport on body weight, plasma lipid peroxidation, plasma selenium, and on leukocyte glutathione peroxidase and glutathione reductase activity in beef calves. Vet Immunol Immunopathol. 2009;127(3-4):365–370.
- Liu JB, Yan HL, Zhang Y, et al. Effects of stale maize on growth performance, immunity, intestinal morphology and antioxidant capacity in broilers. Asian-Australas J Anim Sci. 2020;33(4):605–614.
- Ozkan G, Ulusoy S, Alkanat M, et al. Antiapoptotic and antioxidant effects of GSPE in preventing cyclosporine A-induced cardiotoxicity. Ren Fail. 2012;34(4):460–466.
- Jiang Y-M, Wang Y, Tan H-S, et al. Schisandrol B protects against acetaminophen-induced acute hepatotoxicity in mice via activation of the NRF2/ARE signaling pathway. Acta Pharmacol Sin. 2016;37(3):382–389.
- Wang Y-Z, Zhang Y-C, Cheng J-S, et al. Protective effects of BML-111 on cerulein-induced acute pancreatitis-associated lung injury via activation of Nrf2/ARE signaling pathway. Inflammation. 2014;37(4):1120–1133.
- Ohnuma T, Nakayama S, Anan E, et al. Activation of the Nrf2/ARE pathway via S-alkylation of cysteine 151 in the chemopreventive agent-sensor Keap1 protein by falcarindiol, a conjugated diacetylene compound. Toxicol Appl Pharmacol. 2010;244(1):27–36.
- Jarmi T, Agarwal A. Heme oxygenase and renal disease. Curr Hypertens Rep. 2009;11(1):56–62.
- Venugopal R, Jaiswal AK. Nrf1 and Nrf2 positively and c-Fos and Fra1 negatively regulate the human antioxidant response element-mediated expression of NAD(P)H:quinone oxidoreductase1 gene. Proc Natl Acad Sci U S A. 1996;93(25):14960–14965.
- McCord JM, Fridovich I. Superoxide dismutase: the first twenty years (1968–1988).Free Radic Biol Med. 1988;5(5-6):363–369.
- Banning A, Deubel S, Kluth D, et al. The GI-GPx gene is a target for Nrf2. Mol Cell Biol. 2005;25(12):4914–4923.
- Li W, Kong A-N. Molecular mechanisms of Nrf2-mediated antioxidant response. Mol Carcinog. 2009;48(2):91–104.
- Liu B, Zhang H, Tan X, et al. GSPE reduces lead-induced oxidative stress by activating the Nrf2 pathway and suppressing miR153 and GSK-3β in rat kidney. Oncotarget. 2017;8(26):42226–42237.
- Rajput SA, Sun L, Zhang N-Y, et al. Grape seed proanthocyanidin extract alleviates AflatoxinB1-induced immunotoxicity and oxidative stress via modulation of NF-κB and Nrf2 signaling pathways in broilers. Toxins. 2019;11(1):23.
- Ding Y, Li H, Li Y, et al. Protective effects of grape seed proanthocyanidins on the kidneys of diabetic rats through the Nrf2 signalling pathway. Evid Based Complement Alternat Med. 2020;2020:5205903.
- Li Y, Ma Z, Jiang S, et al. A global perspective on FOXO1 in lipid metabolism and lipid-related diseases. Prog Lipid Res. 2017;66:42–49.
- Marcelin G, Clément K. The multifaceted progenitor fates in healthy or unhealthy adipose tissue during obesity. Rev Endocr Metab Disord. 2021;22(4):1111–1119.
- Wang Y, Xue H, Sun M, et al. Prevention and control of obesity in China. Lancet Glob Health. 2019;7(9):e1166–e1167.
- Longo M, Zatterale F, Naderi J, et al. Adipose tissue dysfunction as determinant of obesity-associated metabolic complications. Int J Mol Sci. 2019;20(9):2358.
- Jones JEC, Esler WP, Patel R, et al. Inhibition of acetyl-CoA carboxylase 1 (ACC1) and 2 (ACC2) reduces proliferation and De Novo lipogenesis of EGFRvIII human glioblastoma cells. PLOS One. 2017;12(1):e0169566.
- Langfort J, Donsmark M, Ploug T, et al. Hormone-sensitive lipase in skeletal muscle. Acta Physiol Scand. 2003;178(4):397–403.
- Zechner R, Zimmermann R, Eichmann TO, et al. FAT SIGNALS–lipases and lipolysis in lipid metabolism and signaling. Cell Metab. 2012;15(3):279–291.
- Ventura-Clapier R, Garnier A, Veksler V. Transcriptional control of mitochondrial biogenesis. Cardiovasc Res. 2008;79(2):208–217.
- Peffer PL, Lin X, Odle J. Hepatic β-oxidation and carnitine palmitoyltransferase I in neonatal pigs after dietary treatments of clofibric acid, isoproterenol, and medium-chain triglycerides. Am J Physiol Regul Integr Comp Physiol. 2005;288(6):R1518–24.
- Gerhart-Hines Z, Rodgers JT, Bare O, et al. Metabolic control of muscle mitochondrial function and fatty acid oxidation through SIRT1/PGC-1α. Embo J. 2007;26(7):1913–1923.
- Zandbergen F, Plutzky J. PPARα in atherosclerosis and inflammation. Biochim Biophys Acta. 2007;1771(8):972–982.
- Sun X, Zemel MB. Leucine modulation of mitochondrial mass and oxygen consumption in skeletal muscle cells and adipocytes. Nutr Metab . 2009;6(1):26.
- Shi J, Yu J, Pohorly JE, et al. Polyphenolics in grape seeds-biochemistry and functionality. J Med Food. 2003;6(4):291–299.
- Ding R-B, Bao J, Deng C-X. Emerging roles of SIRT1 in fatty liver diseases. Int J Biol Sci. 2017;13(7):852–867.
- Kim H-S, Xiao C, Wang R-H, et al. Hepatic-specific disruption of SIRT6 in mice results in fatty liver formation due to enhanced glycolysis and triglyceride synthesis. Cell Metab. 2010;12(3):224–236.
- Caimari A, del Bas JM, Crescenti A, et al. Low doses of grape seed procyanidins reduce adiposity and improve the plasma lipid profile in hamsters. Int J Obes. 2013;37(4):576–583.
- Gall C, von Stehle JH, Weaver DR. Mammalian melatonin receptors. Cell Tissue Res. 2002;309(1):151–162.
- Serviddio G, Bellanti F, Vendemiale G. Free radical biology for medicine. Free Radic Biol Med. 2013;65:952–968.
- Cantó C, Gerhart-Hines Z, Feige JN, et al. AMPK regulates energy expenditure by modulating NAD+ metabolism and SIRT1 activity. Nature. 2009;458(7241):1056–1060.
- Cantó C, Auwerx J. PGC-1α, SIRT1 and AMPK, an energy sensing network that controls energy expenditure. Curr Opin Lipidol. 2009;20(2):98–105.