ABSTRACT
Objective
To explore the effect of renal sympathetic denervation (RSD) on left ventricle hypertrophy and the Raf/MEK/ERK signaling pathway in spontaneously hypertensive rats (SHRs).
Methods
SHRs were divided into SHR, SHR + Sham, SHR + RSD and SHR + U0126 groups, with WKY rats as the baseline controls. The blood pressure of rats was observed, while myocardial fibrosis was evaluated through Masson staining. Thereafter, real-time quantitative polymerase chain reaction (qRT-PCR) was carried out to determine the levels of myocardial-hypertrophy-related markers, and Western blotting was used to measure the activity of the Raf/MEK/ERK signaling pathway.
Results
In comparison with the WKY group, significant increases were observed in the systolic pressure and diastolic pressure of rats from the other four groups at different time points after surgery. In addition, rats in these groups had obvious increases in LVMI, renal NE and IVSd and decreases in LVEDd, LVEF and LVFS. In addition, the CVF of myocardial tissues was increased, with the upregulation of ANP, BNP and β-MHC and the downregulation of α-MHC. For the activity of the Raf/MEK/ERK signaling pathway, the levels of p-Raf/Raf, p-MEK/MEK and p-ERK1/2/ERK1/2 were all remarkably elevated (all P < .05). Further comparison with the SHR group showed that the above indexes in the rats were significantly improved in the RSD group and SHR + U0126 group (all P < .05).
Conclusion
RSD may decrease blood pressure, mitigate hypertension-induced left ventricle hypertrophy and improve cardiac function efficiently in SHRs via the suppression of the Raf/MEK/ERK signaling pathway.
Introduction
Accumulating evidence has shown that hypertension is a kind of systemic disease caused by multiple risk factors, including genetic and environmental factors, or their interactions, having great associations with the high prevalence and mortality of cerebrovascular events (Citation1,Citation2). Left ventricle hypertrophy (LVH), one of the most important target organ damages in hypertension, has been well recognized as an independent risk factor for cardiovascular morbidity and fatality, and continuous myocardial hypertrophy may eventually result in heart failure, malignant arrhythmia or even sudden death (Citation3,Citation4). Thus, how to delay or even reverse the progression of LVH is a major issue in the research and development of new strategies for the prevention and treatment of myocardial diseases (Citation5). Recent studies have indicated that renal sympathetic denervation (RSD) can efficiently decrease blood pressure (Citation6,Citation7). Mechanistically, RSD can specifically block the renal sympathetic nerves, i.e., the renal sympathetic afferent nerve-hypothalamus-renal sympathetic efferent nerve circuit decreases the activity of sympathetic nerves, thus decreasing blood pressure (Citation8,Citation9). In addition to the decrease in blood pressure, Krum H et al. also observed improved LVH and heart function after RSD (Citation10). However, although the roles of RSD in decreasing blood pressure and suppressing sympathetic pressure have been clarified, the potential mechanism affecting LVH remains to be elucidated in further studies.
The MAPK signaling pathway includes a group of evolutionarily conserved serine/threonine kinases that widely exist in mammalian cells and exert a pivotal role in various cellular biological events, such as cell growth, differentiation, development, division and apoptosis (Citation11,Citation12). ERK, also known as Raf/MEK/ERK, is one of the numerous MAPK signal transduction pathway proteins (Citation13). In the study of Roberts RE et al., animal models of hypertension manifested increased ERK activity in vascular smooth muscle cells, whereas the suppression of ERK led to decreased vascular smooth muscle cell growth and vasoconstriction (Citation14). Additionally, the suppression of the Raf/MEK/ERK1/2 signaling pathway can partially reverse the hypertrophy of myocardial cells (Citation15). More importantly, the overactivation of the renin-angiotensin-aldosterone system (RAAS) is involved in the progression of myocardial hypertension, which may be related to the activation of the Raf/MEK/ERK signaling pathway (Citation16). However, RSD can reduce blood pressure by inhibiting the overactivation of the RAAS (Citation17). As such, we inferred that RSD possibly affects the expression of the Raf/MEK/ERK signaling pathway in the development of hypertension-induced ventricle hypertrophy.
Considering the above, we attempted to explore the effect of RSD on LVH and the activity of the Raf/MEK/ERK signaling pathway in spontaneously hypertensive rats (SHRs), aiming to elucidate the potential mechanism by which RSD reduces blood pressure.
Methods
Animal grouping and RSD
Twelve-week-old male specific-pathogen-free (SPF) spontaneously hypertensive rats (SHRs) and male Wistar-Kyoto (WKY) rats weighing between 250 and 290 g were provided by Beijing Vital River Animal Experiment Co., Ltd. A total of 60 rats were randomized into the SHR, SHR + Sham, SHR + RSD and SHR + U0126 groups (15 rats per group), while fifteen 12-week-old WKY rats were selected as the baseline control (WKY group). Rats in the SHR + RSD group underwent RSD surgery. In brief, rats were acclimated for 2 weeks, and thereafter, they were anesthetized by intraperitoneal injection of pentobarbital sodium; the kidney was exposed, and the sheath-enclosed renal artery and vein at the renal hilus were visible; the sheath and the surrounding connective tissues were freed from the artery and vein to locate the renal nerves, where the surrounding nerves of renal arteries were snipped. Ethanol (95%) containing 10% phenol was applied near the artery and vein to destroy all the residual nerves. The abdominal cavity was then closed after the organs were placed in the original positions (Citation18). For rats in the Sham group, after the kidney and the renal artery and vein were exposed, normal saline was applied near the artery and vein without the removal of the surrounding connective tissues, and the wound was sutured. Rats in the SHR + U0126 group additionally received the MEK1/2 inhibitor U0126 (Sigma-Aldrich, 30 mg/kg intraperitonially) (Citation19) daily for one week, while those in the SHR group received DMSO in the same volume. After surgery, the blood pressure and heart rate of conscious rats were determined with the use of an intelligent noninvasive hematomanometer at the same time prior to feeding in the morning every week. The protocol for the animal experiments was approved by the Ethics Committee of the University.
Echocardiogram (ECG) examination
At 6 weeks after surgery, rats underwent echocardiogram examination. In brief, rats were anesthetized first and fixed in the supine position for smooth breathing for the ECG performed with the GE vivid 7 Ultrasonic Diagnostic System; a probe was used to apply the coupling agent at the left side of the rib, and under the guidance of 2-dimensional ultrasound, a standard plane of the long axis in the left ventricle was identified along the outflow tract of the left ventricle; then, the probe was rotated by 90° to identify the cross-section on the level of the papillary muscles to measure the interventricular septal thickness in diastole (IVSd), left ventricular end-systole dimension (LVESD) and left ventricular end-diastole dimension (LVEDd); finally, the left ventricular ejection fraction (LVEF) and left ventricular shortening fraction (LVFS) were calculated with the use of software.
Norepinephrine (NE) and left ventricular weight index (LVWI) determination
Rats were sacrificed at 18 weeks of age (approximately 6 weeks after surgery) to collect heart and kidney samples. Kidney tissues were first rinsed and placed in a homogenizer containing 1 mL normal saline. On ice, the tissues were homogenized manually, and the supernatant was obtained after centrifugation at 3000 r/min for 5 min. An enzyme-linked immunosorbent assay (ELISA) kit (Shanghai Jonin Biotech Co., Ltd, China) was utilized to determine the level of NE in the kidney. After sacrifice, the heart was collected rapidly, and along the atrioventricular junction, major vessels, left and right ventricles and atria were isolated and rinsed in cold PBS. Following the removal of water by filter paper, the left ventricle in the interventricular septum was weighed, and the left ventricle weight index was calculated by the LVW-to-BW ratio. Thereafter, heart samples were divided into two parts: one was stored at −80°C for Western blotting, while the other was fixed in 10% formaldehyde for 24 h, followed by dehydration, paraffin embedding and slicing to prepare sections with a thickness of 5 μm.
Masson staining
Paraffin sections were dewaxed and hydrated in running water and distilled water. Then, they were placed in Weigert-hematoxylin for 5 to 10 min. Sections were washed in distilled water and stained in Masson ponceau for 5 to 10 min. In 3% glacial acetic acid, residual ponceau was removed by the immersion of the sections for a while, and then sections were placed in 1% phosphomolybdic acid for 3 to 5 min. Without any washes in water, sections were stained directly in aniline blue for 5 min and then rinsed in 0.2% glacial acetic acid for a while. Following rinsing, sections were treated sequentially in 95% ethanol, 100% ethanol, xylene and neutral balsam. Under the optical microscope, the collagen fibers of myocardium were stained blue, while the myocardium was stained deep purple, and myocardial fibrosis was evaluated by the collagen volume fraction (CVF; the percentage of the collagen area in blue in the surface area of the myocardium).
Quantitative real-time PCR (qRT-PCR)
The total RNA extracted from the tissues was subjected to a determination of purity with the use of an ultraviolet spectrometer. With the total RNA, cDNA was prepared with the use of the PrimeScript RT kit (RR014A, TaKaRa Biotech, Beijing, China). With the cDNA and primers designed by Primer 5.0 software () and synthesized by Nanjing GeneScript Biotech Co., Ltd, qRT-PCR was conducted with the use of the PCR kit (KR011A1, Beijing Tiangen Biotech Co., Ltd, Beijing, China) according to the manufacturer’s instructions. The expression of the target genes was quantified with the formula 2−ΔΔCt, where ΔCt = Ct target gene-Ct reference gene and ΔΔCt = ΔCt experiment -ΔCt control. The relative transcription level of the mRNA of the target gene = 2−ΔΔCt.
Table 1. Primer design and the sequence
Western blotting
Tissues were homogenized to obtain the total proteins in the tissue, which were later prepared for determinations of the concentration of proteins with the BCA method. Following separation by 8% SDS-PAGE, proteins in the gel were transferred to PVDF membranes. Then, the nonspecific sites on the membrane were blocked with 5% BSA/TBST for 60 min. Proteins on the membrane were probed through incubation with the primary antibodies p-Raf, Raf, p-MEK, MEK, p-ERK1/2, ERK1/2, GAPDH at 4°C overnight and then with the secondary antibodies (horseradish-peroxidase-labeled goat anti-rabbit IgG, Abcam, Cambridge, UK) at room temperature for 120 min. After three washes in TBST, an enhanced chemiluminescence (ECL; Millipore, USA) reagent was added to the surface of membrane to develop the protein bands, and the intensity was evaluated with the use of Quantity One software. The relative expression of the target protein was calculated by the ratio of the intensity of the target protein to that of the reference protein.
Statistical analysis
SPSS 21.0 software (SPSS Inc., Chicago, USA) was utilized for data analysis, and each experiment was repeated three times. Measured data are presented as the mean ± the standard deviation and were compared between two groups by t test and among groups by one-way ANOVA. P < .05 suggested a statistically significant difference.
Results
RSD attenuates blood pressure in SHRs
As shown in , no statistically significant changes occurred in the weight or heart rate at different time points among groups (all P > .05). The blood pressure analysis demonstrated that the SBP and DBP in rats from the SHR, SHR + Sham, SHR + RSD and SHR + U0126 groups at all time points were higher than those of WKY rats (all P < .05). There were no statistically significant differences between the SHR group and the SHR + Sham group in SBP and DBP (all P > .05). After RSD or U0126 treatment, the blood pressure (SBP and DBP) of the SHR group decreased significantly compared with that of the SHR + Sham group at all time points (all P < .05).
Effect of RSD on LVMI and renal NE levels in SHRs
Compared with the WKY group, the rats in the SHR group and SHR + Sham group had significantly higher LVMI, renal NE and IVSd but lower LVEDd, LVEF and LVFS (all P < .05, ). Conversely, lower LVMI, renal NE and IVSd but higher LVEDd, LVEF and LVFS were exhibited in rats from both the SHR + RSD group and the SHR + U0126 group than in those from the SHR group (all P < .05). Furthermore, the above parameters showed no significant differences between the SHR group and the SHR + Sham group (all P > .05).
Effect of RSD on the collagen content of SHRs
Collagen content was assessed using Masson’s trichrome staining of myocardial tissue sections, and as a result, fibrotic tissues were stained blue, while myocardial cells were stained red (). The collagen volume fraction (CVF) in both the SHR group and the SHR + Sham group significantly increased in comparison with the WKY group (all P < .05). The CVF was significantly decreased in the SHR + RSD group and SHR + U0126 group compared with that in the SHR group (all P < .05). No significant difference was observed in CVF between the SHR group and the Sham group (P > .05).
Effect of RSD on myocardial-hypertrophy-associated genes in SHRs
Myocardial hypertrophic markers, including ANP, BNP, α-MHC and β-MHC, were detected with qRT-PCR (). Compared with the WKY group, the SHR group and the SHR + Sham group had significantly increased expression levels of ANP, BNP and β-MHC and decreased expression levels of α-MHC to a large extent (all P < .05). There were no differences in the expression of ANP, BNP, α-MHC and β-MHC between the SHR group and the SHR + Sham group (all P > .05). However, when compared with the SHR group, the expression levels of ANP, BNP and β-MHC in the SHR + RSD group and SHR + U0126 group were significantly downregulated with upregulated α-MHC (all P < .05).
Effect of RSD on the expression of the Raf/MEK/ERK pathway in SHRs
As indicated in , the expression levels of p-Raf/Raf, p-MEK/MEK and p-ERK1/2/ERK1/2 in the SHR group and SHR + Sham group were higher than those in the WKY group (all P < .05).
Figure 5. Comparison of the expression of Raf/MEK/ERK pathway-related proteins in rats in each group
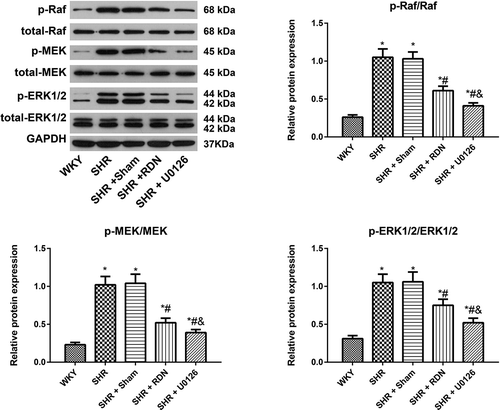
The SHR + RSD group and SHR + U0126 group exhibited a significant decrease in the expression of p-Raf/Raf, p-MEK/MEK and p-ERK1/2/ERK1/2 compared to the SHR group (all P < .05). Nevertheless, no obvious differences were identified in comparisons of these proteins between the SHR group and the SHR + Sham group (all P > .05).
Discussion
Existing evidence has indicated that SHRs at 4–6 weeks may experience a gradual increase in blood pressure, as well as an augmentation in peripheral vascular resistance without any medication or surgical intervention, which closely approximates the pathophysiological process of human primary hypertension (Citation20,Citation21). As such, SHRs were selected as the animal model in this study. According to a previous study by Li P et al., the sympathetic activity of the hypertensive animal model was decreased to attenuate hypertension and improve vascular remodeling or vascular fibrosis as a consequence of RSD (Citation22). After our observation, we indeed found that the SHRs had higher SBP and DBP than WKY rats, but the blood pressures in SHRs were decreased after RSD, exhibiting the successful establishment of the RSD models. Importantly, RSD has been shown to effectively lead to a significant decrease in blood pressure in multiple hypertensive animals, including dogs, pigs, and sheep, as well as SHRs (Citation23,Citation24). As such, we hypothesized that RSD may contribute to a delay in the development of LVH in our SHRs.
In the current research, we also found that SHRs had significant increases in LVMI and NE, and their CVF, an indicator reflecting the fibrosis of myocardium, was also augmented, but this was reversed by RSD surgery or U0126 treatment. Hypertension could give rise to an increase in cardiac afterload and then trigger a series of neurohormonal activities, finally inducing LVH (Citation25,Citation26). LVH is a major indicator for the evaluation of myocardial hypertrophy, including pathological manifestations such as the elevation of LVMI or cardiomyocyte hypertrophy as well as the aggravation of myocardial interstitial fibrosis, which could be further increased in response to the continuous enhancement of pressure overload (Citation27–30). The NE in the plasma and tissues mainly originates from the sympathetic nerve endings, and its alteration reflects the activity of the sympathetic nervous system. Specifically, increased NE levels are always discovered in patients with left ventricular dysfunction (Citation31,Citation32). Coincidently, in the work of Jianling Li et al. (Citation33), RSD not only significantly decreased NE levels in the plasma and kidneys to mitigate renal injury in SHRs but also improved diastolic function, with significant decreases in DBP, SBP and LVWI, as also demonstrated by Brandt MC et al. (Citation34). Krum et al. also found that RSD not only reduced blood pressure but also conferred a protective effect on hypertension-induced LVH (Citation10). Taken together, RSD and U0126 (an inhibitor of Raf/MEK/ERK), in our study, may increase cardiac function and improve myocardial interstitial fibrosis.
As a result, both RSD and U0126 decreased the expression of ANP, BNP and β-MHC in SHRs, with the upregulation of α-MHC. To our knowledge, the chronic overactivation of neurohormonal activities in hypertension leads to the abnormal expression of LVH-related genes. For instance, under pathological conditions, such as myocardial hypertrophy, ANP is expressed in ventricular cells and is positively correlated with the degree of myocardial hypertrophy (Citation35). BNP is a kind of ventricle-secreted polypeptide hormone that regulates cardiovascular functions, and its elevation suggests diastolic dysfunction and concentric hypertrophy in the left ventricle (Citation36,Citation37). β-MHC is the major isomer of the contractile protein in fetal ventricular cells, and after birth, it is transformed into α-MHC; under myocardial hypertrophy, persistent increases in afterload induce the expression of β-MHC in a manner parallel to the degree of ventricular hypertrophy; in addition, the decrease in the expression of α-MHC is negatively correlated to the degree of ventricular hypertrophy (Citation38–40). These indicators could indirectly reflect the pathological changes in ventricular hypertrophy. In agreement with our study, RSD ameliorated hypertension-induced ventricular hypertrophy and myocardial fibrosis by decreasing blood pressure, LVWI and CVF in SHRs, as demonstrated by Ding X et al. (Citation41). This further confirmed that RSD can significantly delay the progression of left ventricular hypertrophy in SHRs.
Finally, we discovered that both RSD and U0126 could block the activation of the Raf/MEK/ERK signaling pathway in SHRs, with decreased levels of p-Raf/Raf, p-MEK/MEK and p-ERK1/2/ERK1/2, as detected by Western blotting. Likewise, Jing L et al. reported a significant increase in the phosphorylation of ERK1/2 in the endothelial cells of the aorta and vascular smooth muscle cells (Citation42). The inhibition of MEK/ERK could improve the LVW/BW ratio and systolic blood pressure to exert antihypertrophic effects (Citation43). As documented, in response to external stimuli, Ras is transformed into an activated state, which can further activate Raf-1; as a result, MEK is activated by phosphorylation, further inducing the phosphorylation of ERK1/2, while p-ERK1/2 triggers the phosphorylation of ElK-1 L inside the nuclei. Such a cascade-like activation delivers external signals through the cell membrane and cytoplasm to the nuclei; thus, it is involved in various physiological regulations (Citation44,Citation45). It has been widely accepted that the activation of the RAAS is involved in the pathogenesis of hypertension-induced ventricular hypertrophy (Citation46), while AngII, the promotor of the RAAS, is featured with potent positive inotropic action and contractile activity, promotes the synthesis of proteins and participates in the early-stage signal transduction of LVH (Citation47,Citation48). Moreover, AngII activates the expression of the ERK signaling pathway by targeting ATl (Citation49). According to the study by Dai Z et al., RSD can mitigate ventricular interstitial fibrosis evidently and decrease the levels of AngII and RAAS activity, thereby ameliorating the diastolic function of the heart and delaying the onset of heart failure (Citation50). Thus, we inferred that RSD may delay the progression of LVH by downregulating AngII to suppress the activation of the Raf/MEK/ERK signaling pathway.
Considering the above, we concluded that RSD may suppress the activity of the Raf/MEK/ERK signaling pathway, decrease blood pressure and improve LVH and cardiac function in SHRs, which may provide theoretical evidence to guide the clinical treatment of hypertension with the application of RSD.
Declaration of interest
The authors report no conflicts of interest.
Supplemental Material
Download JPEG Image (290 KB)Supplementary material
Supplemental data for this article can be accessed on the publisher’s website
Additional information
Funding
References
- Chien KL, Hsu HC, Sung FC, Su TC, Chen MF, Lee YT. Incidence of hypertension and risk of cardiovascular events among ethnic Chinese: report from a community-based cohort study in Taiwan. J Hypertens. 2007;25(7):1355–61.doi:10.1097/HJH.0b013e3280d94313.
- Rosamond W, Flegal K, Friday G, Furie K, Go A, Greenlund K, Haase N, Ho M, Howard V, Kissela B, et al. American heart association statistics C, Stroke statistics S. Heart disease and stroke statistics–2007 update: a report from the American heart association statistics committee and stroke statistics subcommittee. Circulation. 2007;115:e69–171.doi:10.1161/CIRCULATIONAHA.106.179918.
- Bombelli M, Facchetti R, Carugo S, Madotto F, Arenare F, Quarti-Trevano F, Capra A, Giannattasio C, Dell’Oro R, Grassi G, et al. Left ventricular hypertrophy increases cardiovascular risk independently of in-office and out-of-office blood pressure values. J Hypertens. 2009;27(12):2458–64.doi:10.1097/HJH.0b013e328330b845.
- Mao Y, Tokudome T, Kishimoto I, Otani K, Nishimura H, Yamaguchi O, Otsu K, Miyazato M, Kangawa K. Endogenous ghrelin attenuates pressure overload-induced cardiac hypertrophy via a cholinergic anti-inflammatory pathway. Hypertension. 2015;65(6):1238–44.doi:10.1161/HYPERTENSIONAHA.114.04864.
- Olsen MH, Wachtell K, Ibsen H, Lindholm LH, Dahlof B, Devereux RB, Kjeldsen SE, Oikarinen L, Okin PM. Reductions in albuminuria and in electrocardiographic left ventricular hypertrophy independently improve prognosis in hypertension: the LIFE study. J Hypertens. 2016;12(4):775–81.doi:10.1097/01.hjh.0000217862.50735.dc.
- Krum H, Schlaich MP, Sobotka PA, Bohm M, Mahfoud F, Rocha-Singh K, Katholi R, Esler MD. Percutaneous renal denervation in patients with treatment-resistant hypertension: final 3-year report of the Symplicity HTN-1 study. Lancet. 2014;383:622–29.doi:10.1016/S0140-6736(13)62192-3.
- Bohm M, Mahfoud F, Ukena C, Hoppe UC, Narkiewicz K, Negoita M, Ruilope L, Schlaich MP, Schmieder RE, Whitbourn R, et al. First report of the global SYMPLICITY registry on the effect of renal artery denervation in patients with uncontrolled hypertension. Hypertension. 2015;65(4):766–74.doi:10.1161/HYPERTENSIONAHA.114.05010.
- Eriguchi M, Tsuruya K. Renal sympathetic denervation in rats. Methods Mol Biol. 2016;1397:45–52.
- Polimeni A, Curcio A, Indolfi C. Renal sympathetic denervation for treating resistant hypertension. Circ J. 2013;77(4):857–63.doi:10.1253/circj.CJ-13-0297.
- Krum H, Schlaich M, Whitbourn R, Sobotka PA, Sadowski J, Bartus K, Kapelak B, Walton A, Sievert H, Thambar S, et al. Catheter-based renal sympathetic denervation for resistant hypertension: a multicentre safety and proof-of-principle cohort study. Lancet. 2009;373(9671):1275–81.doi:10.1016/S0140-6736(09)60566-3.
- Manna PR, Stocco DM. The role of specific mitogen-activated protein kinase signaling cascades in the regulation of steroidogenesis. J Signal Transduct. 2011;2011:821615.doi:10.1155/2011/821615.
- Martinez-Soto D, Ruiz-Herrera J. Functional analysis of the MAPK pathways in fungi. Rev Iberoam Micol. 2017;34:192–202.doi:10.1016/j.riam.2017.02.006.
- De Luca A, Maiello MR, D’Alessio A, Pergameno M, The NN.RAS/RAF/MEK/ERK and the PI3K/AKT signalling pathways: role in cancer pathogenesis and implications for therapeutic approaches. Expert Opin Ther Targets. 2012;16(Suppl 2):S17–27.doi:10.1517/14728222.2011.639361.
- Roberts RE. The extracellular signal-regulated kinase (ERK) pathway: a potential therapeutic target in hypertension. J Exp Pharmacol. 2012;4:77–83.doi:10.2147/JEP.S28907.
- Lorenz K, Schmitt JP, Vidal M, Lohse MJ. Cardiac hypertrophy: targeting Raf/MEK/ERK1/2-signaling. Int J Biochem Cell Biol. 2009;41(12):2351–55.doi:10.1016/j.biocel.2009.08.002.
- Zhang P, Mende U. Regulators of G-protein signaling in the heart and their potential as therapeutic targets. Circ Res. 2011;109:320–33.doi:10.1161/CIRCRESAHA.110.231423.
- Wang L, Lu CZ, Zhang X, Luo D, Zhao B, Yu X, Xia DS, Chen X, Zhao XD. [The effect of catheter based renal synthetic denervation on renin-angiotensin-aldosterone system in patients with resistant hypertension]. Zhonghua Xin Xue Guan Bing Za Zhi. 2013;41:3–7.
- Jiang W, Tan L, Guo Y, Li X, Tang X, Yang K. Effect of renal denervation procedure on left ventricular hypertrophy of hypertensive rats and its mechanisms. Acta Cir Bras. 2012;27(11):815–20.doi:10.1590/S0102-86502012001100012.
- Ahnstedt H, Mostajeran M, Blixt FW, Warfvinge K, Ansar S, Krause DN, Edvinsson L. U0126 attenuates cerebral vasoconstriction and improves long-term neurologic outcome after stroke in female rats. J Cereb Blood Flow Metab. 2015;35(3):454–60.doi:10.1038/jcbfm.2014.217.
- Folkow B, Hallback M, Lundgren Y, Weiss L. Background of increased flow resistance and vascular reactivity in spontaneously hypertensive rats. Acta Physiol Scand. 1970;80(1):93–106.doi:10.1111/j.1748-1716.1970.tb04773.x.
- Kodavanti UP, Schladweiler MC, Ledbetter AD, Watkinson WP, Campen MJ, Winsett DW, Richards JR, Crissman KM, Hatch GE, Costa DL. The spontaneously hypertensive rat as a model of human cardiovascular disease: evidence of exacerbated cardiopulmonary injury and oxidative stress from inhaled emission particulate matter. Toxicol Appl Pharmacol. 2000;164(3):250–63.doi:10.1006/taap.2000.8899.
- Li P, Huang PP, Yang Y, Liu C, Lu Y, Wang F, Sun W, Kong XQ. Renal sympathetic denervation attenuates hypertension and vascular remodeling in renovascular hypertensive rats. J Appl Physiol (1985). 2017;122(1):121–29.doi:10.1152/japplphysiol.01019.2015.
- Azizi M, Schmieder RE, Mahfoud F, Weber MA, Daemen J, Davies J, Basile J, Kirtane AJ, Wang Y, Lobo MD, et al. Endovascular ultrasound renal denervation to treat hypertension (RADIANCE-HTN SOLO): a multicentre, international, single-blind, randomised, sham-controlled trial. Lancet. 2018;391(10137):2335–45.doi:10.1016/S0140-6736(18)31082-1.
- Katayama T, Sueta D, Kataoka K, Hasegawa Y, Koibuchi N, Toyama K, Uekawa K, Mingjie M, Nakagawa T, Maeda M, et al. Long-term renal denervation normalizes disrupted blood pressure circadian rhythm and ameliorates cardiovascular injury in a rat model of metabolic syndrome. J Am Heart Assoc. 2013;2(4):e000197.doi:10.1161/JAHA.113.000197.
- Taddei S, Nami R, Bruno RM, Quatrini I, Nuti R. Hypertension, left ventricular hypertrophy and chronic kidney disease. Heart Fail Rev. 2011;16(6):615–20.doi:10.1007/s10741-010-9197-z.
- Lepor NE, Hypertension KA. Left ventricular hypertrophy and cardiac function in patients with resistant hypertension. Rev Cardiovasc Med. 2013;14:69–71.
- Gosse P.Left ventricular hypertrophy–the problem and possible solutions. J Int Med Res. 2005;33(Suppl 1):3A–11A.doi:10.1177/14732300050330S102.
- Ozcakar ZB, Yalcinkaya F, Tutar E, Cakar N, Ucar T, Elhan A, Acar B, Yuksel S, Uncu N, Kara N, et al. Hypertension and left ventricular hypertrophy in pediatric peritoneal dialysis patients: ambulatory blood pressure monitoring and echocardiographic evaluation. Nephron Clin Pract. 2006;104(2):c101–6.doi:10.1159/000093997.
- Andersen K, Hennersdorf M, Cohnen M, Blondin D, Modder U, Poll LW. Myocardial delayed contrast enhancement in patients with arterial hypertension: initial results of cardiac MRI. Eur J Radiol. 2009;71(1):75–81.doi:10.1016/j.ejrad.2008.03.009.
- Lee Y, Kim BK, Lim YH, Kim MK, Choi BY, Shin J. The relationship between adiponectin and left ventricular mass index varies with the risk of left ventricular hypertrophy. PLoS One. 2013;8(7):e70246.doi:10.1371/journal.pone.0070246.
- Tawa M, Yamamoto S, Ohkita M, Matsumura Y. Endothelin-1 and norepinephrine overflow from cardiac sympathetic nerve endings in myocardial ischemia. Cardiol Res Pract. 2012;2012:789071.doi:10.1155/2012/789071.
- Cohn JN. Plasma norepinephrine and mortality. Clin Cardiol. 1995;18(S1):I9–12.doi:10.1002/clc.4960181304.
- Li J, He Q, Wu W, Li Q, Huang R, Pan X, Lai W. Role of the renal sympathetic nerves in renal sodium/potassium handling and renal damage in spontaneously hypertensive rats. Exp Ther Med. 2016;12(4):2547–53.doi:10.3892/etm.2016.3669.
- Brandt MC, Mahfoud F, Reda S, Schirmer SH, Erdmann E, Bohm M, Hoppe UC. Renal sympathetic denervation reduces left ventricular hypertrophy and improves cardiac function in patients with resistant hypertension. J Am Coll Cardiol. 2012;59(10):901–09.doi:10.1016/j.jacc.2011.11.034.
- Holtwick R, van Eickels M, Skryabin BV, Baba HA, Bubikat A, Begrow F, Schneider MD, Garbers DL, Kuhn M. Pressure-independent cardiac hypertrophy in mice with cardiomyocyte-restricted inactivation of the atrial natriuretic peptide receptor guanylyl cyclase-A. J Clin Invest. 2003;111(9):1399–407.doi:10.1172/JCI17061.
- Hobbs RE, Mills RM. Endogenous B-type natriuretic peptide: a limb of the regulatory response to acutely decompensated heart failure. Clin Cardiol. 2008;31(9):407–12.doi:10.1002/clc.20304.
- Liu H, Zhang YZ, Gao M, Liu BC. Elevation of B-type natriuretic peptide is a sensitive marker of left ventricular diastolic dysfunction in patients with maintenance haemodialysis. Biomarkers. 2010;15(6):533–37.doi:10.3109/1354750X.2010.493973.
- Sheridan DJ, Autelitano DJ, Wang B, Percy E, Woodcock EA, Du XJ. Beta(2)-adrenergic receptor overexpression driven by alpha-MHC promoter is downregulated in hypertrophied and failing myocardium. Cardiovasc Res. 2000;47:133–41.doi:10.1016/S0008-6363(00)00065-1.
- Pandya K, Kim HS, Smithies O. Fibrosis, not cell size, delineates beta-myosin heavy chain reexpression during cardiac hypertrophy and normal aging in vivo. Proc Natl Acad Sci U S A. 2006;103:16864–69.
- Di Domenico M, Casadonte R, Ricci P, Santini M, Frati G, Rizzo A, Carratelli CR, Lamberti M, Parrotta E, Quaresima B, et al. Cardiac and skeletal muscle expression of mutant beta-myosin heavy chains, degree of functional impairment and phenotypic heterogeneity in hypertrophic cardiomyopathy. J Cell Physiol. 2012;227(10):3471–76.doi:10.1002/jcp.24047.
- Ding X, Xu X, Yan Y, Song X, Liu S, Wang G, Su D, Jing Q, Qin Y. Effects of renal sympathetic denervation and angiotensin-converting enzyme inhibitor on left ventricular hypertrophy. Comparison in spontaneously hypertensive rats. Herz. 2015;40(4):695–701.doi:10.1007/s00059-014-4110-5.
- Jing L, Zhang JZ, Zhao L, Wang YL, Guo FY. High-expression of transforming growth factor beta1 and phosphorylation of extracellular signal-regulated protein kinase in vascular smooth muscle cells from aorta and renal arterioles of spontaneous hypertension rats. Clin Exp Hypertens. 2007;29:107–17.doi:10.1080/10641960701195447.
- Yang L, Zou X, Liang Q, Chen H, Feng J, Yan L, Wang Z, Zhou D, Li S, Yao S, et al. Sodium tanshinone IIA sulfonate depresses angiotensin II-induced cardiomyocyte hypertrophy through MEK/ERK pathway. Exp Mol Med. 2007;39(1):65–73.doi:10.1038/emm.2007.8.
- Cho HJ, Kang JH, Kwak JY, Lee TS, Lee IS, Park NG, Nakajima H, Magae J, Chang YC. Ascofuranone suppresses PMA-mediated matrix metalloproteinase-9 gene activation through the Ras/Raf/MEK/ERK- and Ap1-dependent mechanisms. Carcinogenesis. 2007;28:1104–10.doi:10.1093/carcin/bgl217.
- McCubrey JA, Steelman LS, Chappell WH, Abrams SL, Wong EW, Chang F, Lehmann B, Terrian DM, Milella M, Tafuri A, et al. Roles of the Raf/MEK/ERK pathway in cell growth, malignant transformation and drug resistance. Biochim Biophys Acta. 2007;1773:1263–84.doi:10.1016/j.bbamcr.2006.10.001.
- Freitas RR, Lopes KL, Carillo BA, Bergamaschi CT, Carmona AK, Casarini DE, Furukawa L, Heimann JC, Campos RR, Dolnikoff MS. Sympathetic and renin-angiotensin systems contribute to increased blood pressure in sucrose-fed rats. Am J Hypertens. 2007;20(6):692–98.doi:10.1016/j.amjhyper.2007.01.014.
- Dahlof B. Left ventricular hypertrophy and angiotensin II antagonists. Am J Hypertens. 2001;14(2):174–82.doi:10.1016/S0895-7061(00)01257-7.
- Kang -N-N, Fu L, Xu J, Han Y, Cao J-X, Sun J-F, Zheng M. Testosterone improves cardiac function and alters angiotensin II receptors in isoproterenol-induced heart failure. Arch Cardiovasc Dis. 2012;2012(2):68–76.doi:10.1016/j.acvd.2011.12.002.
- Guo L, Wang M, Zhang ZY, Hao L, Lou BY, Li XY, Loo WT, Jin L, Cheung MN. Angiotensin II induces interleukin-6 synthesis in osteoblasts through ERK1/2 pathway via AT1 receptor. Arch Oral Biol. 2011;56(3):205–11.doi:10.1016/j.archoralbio.2010.09.016.
- Dai Z, Yu S, Zhao Q, Meng Y, He H, Tang Y, Wang X, Xiao J, Wang X, Huang C. Renal sympathetic denervation suppresses ventricular substrate remodelling in a canine high-rate pacing model. EuroIntervention. 2014;10(3):392–99.doi:10.4244/EIJV10I3A65.