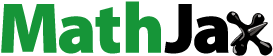
Abstract
Today (year 2020), the globally recognized problem of arsenic (As) contamination of water resources and other environments at toxic levels has been reported in all of the 20 Latin American countries. The present review indicates that As is prevalent in 200 areas across these countries. Arsenic is naturally released into the environment and mobilized from geogenic sources comprising: (i) volcanic rocks and emissions, the latter being transported over thousands of kilometers from the source, (ii) metallic mineral deposits, which get exposed to human beings and livestock through drinking water or food chain, and (iii) As-rich geothermal fluids ascending from deep geothermal reservoirs contaminate freshwater sources. The challenge for mitigation is increased manifold by mining and related activities, as As from mining sites is transported by rivers over long distances and even reaches and contaminates coastal environments. The recognition of the As problem by the authorities in several countries has led to various actions for remediation, but there is a lack of long-term strategies for such interventions. Often only total As concentration is reported, while data on As sources, mobilization, speciation, mobility and pathways are lacking which is imperative for assessing quality of any water source, i.e. public and private.
Graphical abstract
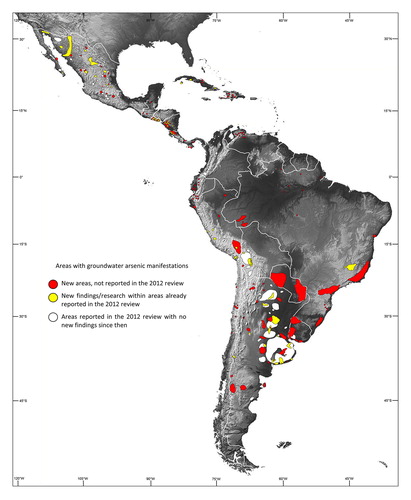
1. Introduction
During the last two decades, the presence of arsenic (As) in natural environments has finally received the recognition as a global environmental and health problem. It has also been acknowledged by authorities that the occurrence of As in different environments (groundwater, surface water, soil and sediments) is widespread in Latin America where at present (year 2020), the As problem is known in all of its 20 countries, resulting in diverse environmental and public health impacts. It took, however, over 100 years to come to acknowledge this problem. Discoveries started more than 100 years ago in Argentina where As-related health effect were described in 1913 in the area of Bell Ville in Córdoba Province and were attributed to drinking As-rich groundwater (Ayerza, Citation1917a, Citation1917b, Citation1918; Goyenechea, Citation1917). It took more than four decades to confirm As occurrence in groundwater and surface water in Mexico (1958; González Hita et al., Citation1995), Chile (1962; Borgoño & Greiber, Citation1972) and Peru (1975; Kirchmer & Castro, Citation1979). Until then, it was still believed that occurrence of As in toxic concentrations impacts the water resources only locally. It took about two more decades to change this opinion, with the widespread discoveries on the presence of As in groundwater and other environmental compartments in many new areas in these countries. In a relatively quick succession, As was discovered in several new Latin American countries, including Nicaragua (1996), El Salvador (1998), Brazil (1998/2000), Bolivia (2001), Cuba (2002), Ecuador (2005), Uruguay (2005/2006), Colombia (2007) and Guatemala (2007) (Bundschuh et al., Citation2012 and references therein) and Costa Rica (2017; Herrera-Murillo et al., Citation2017).
This increased research into As and related topics has finally confirmed that As contamination in Latin America is of the same order of relevance, as in the “classical” As impacted aquifers, such as those in the Bengal Delta in South-East Asia, where the problem has received much attention especially during the last 4 decades. However, in Latin America, there is still a challenge that much of the new knowledge is contained in internal reports or research presented at national and regional scientific events and journals, which are mostly in the Spanish language. This hinders the dissemination of the new information among the global scientific community and makes it unavailable for the researchers and decision makers, who are working on and concerned about As problems in other continents.
In Latin America, As is generally of geogenic origin. It originates mostly from young (Cenozoic to present) volcanic rocks, from where it is mobilized by physical and chemical weathering (Bundschuh et al., Citation2004; Bundschuh, Nath, et al., Citation2012; Bhattacharya et al., Citation2006; Nicolli et al., Citation2010, Citation2012; Nriagu et al., Citation2007; Raychowdhury et al., Citation2014, Delgado Quezada et al., Citation2020). A special case is the mobilization of As from wall rocks of geothermal reservoirs, being transported through ascending geothermal fluids to zones close to the earth’s surface (Birkle et al., Citation2010; López et al., Citation2012; Morales-Simfors et al., Citation2020; Tomaszewska et al., Citation2020). Volcanic emissions, such as gases and especially volcanic ash producing eruptions, are other important geological As sources (López et al., Citation2012). From these geogenic sources, As can be mobilized and transported into ground- and surface water resources used for drinking and irrigation purposes, soils (including those used for agriculture), sediments and other environments; and can move into the human food chain, adversely impacting human and animal health. Arsenic can be transported within these environments over large distances (e.g., by rivers and wind) and can cross the environmental boundaries as well. Geothermal fluids, which often have high As contents, can rise up from deep geothermal reservoirs and impact shallow aquifers and surface water bodies, which are important sources for drinking water and irrigation purposes, through deep seated fractures and fissures (López et al., Citation2012; Delgado Quezada et al., Citation2020; Tomaszewska et al., Citation2020).
In the current review, we provide a critical compilation of information and analyze progress of the related studies and outcomes on the origins, mobilization and transport to human environments in Latin America with focus on the developments during the past 10 years. As Latin America, we consider the 20 Spanish and Portuguese speaking countries in North, Central and South America and in the Caribbean, which are sovereign states. Older information is briefly resumed, as far as it is needed for understanding in wider context or where respective data or information (e.g., internal reports) has not yet been available in English. For corresponding updated knowledge on mitigation of the As problem in Latin America, we refer to Litter et al. (Citation2019a, Citation2019b, Citation2020) and Kumar et al. (Citation2019) and for health effects of As exposure to Khan et al. (Citation2020).
2. North America
2.1. Mexico
More than six decades ago, the problem of As exposure due to its high concentrations in groundwater of sedimentary aquifers was recognized in the Comarca Lagunera region in 1958, which extends over the states of Coahuila and Durango in northern Mexico (, Table SM1, Supplementary material; Cebrián et al., Citation1994; Armienta & Segovia, Citation2008, Limón-Pacheco et al., Citation2018). Four years later, in 1962, 40 cases (1 casualty) of health effects due to As were described in the City of Torreón (Comarca Lagunera, Coahuila State, ; Castro de Esparza, Citation2009). Both these reported cases can be considered as starting points of As-related (hydro) geochemical, environmental and health-related studies in Mexico (Table SM1, Supplementary material).
Figure 1. Areas in Latin America’s 20 states affected by arsenic concentrations in groundwater at concentrations toxic for human consumption without previous treatment. Shown are areas that have already have been reported in Bundschuh et al. (Citation2012) (white) with new research in the same areas since then (yellow) and new groundwater arsenic areas not yet reported in this 2012 review (red). The digital elevation model is courtesy of NASA/JPL-Caltech (http://photojournal.jpl.nasa.gov).
MEXICO (MX): Baja California St.: 1 Cerro Prieto geothermal area, 2 San Ignacio-Mulegé, Sierra Guadalupe, 3 Sierra El Mechudo, communities of San Juan de la Costa and Punta Coyote, 4 San Antonio-El Triunfo, 5 Bahía Concepción; Sonora St.: 6 Caborca, Magdalena, 7 Hermosillo, 8 Yaqui River watershed, 9 Etchojoa, 10 Colorado River delta, 11 Guadalupe Victoria, Tobarito; Chihuahua St.: 12 Santa Barbara mining area, 13 Meoqui, Delicias, Julimes, Camargo, Jimenes, 14 Tabaloapa, Aldama, Dolores, 15 Hidalgo del Parral; Nuevo León St.: 16 Monterrey; Zacatecas St.: 17 Zacatecas, 18 Concepción del Oro; Durango and Coahuila St.: 19 Comarca Lagunera; Durango St.: 20 Valle del Guadiana (Durango City and vicinity); Col. 5 de febrero, 21 Cerro de Mercado; San Luis Potosí St.: 22 Santa Ma. de la Paz, 23 Morales in San Luis Potosí city, 24 Río Verde, 25 Soledad de Graciano., 26 Matehuala; Hidalgo St.: 27 Zimapán; Aguascalientes St.: 28 Aguascalientes; Puebla St.: 29 Acoculco geothermal area, 30 Los Humeros geothermal area; Michoacán St.: 31 Los Azufres geothermal area, 32 Cuitzeo Lake; Jalisco St.: 33 Altos de Jalisco; Guanajuato St.: 34 Salamanca, 35 Acámbaro, 36 Independencia, 37 Juventino Rosas Municipality, 38 Aurora Mine Guanajuato; Morelos St.: 39 Huautla; Guerrero St.: 40 Taxco, 41 Tlamacazapa; Oaxaca St.: 42 Oaxaca; Tabasco St.: 43 Cactus-Sitio Grande, 44 Luna-Sen, 45 Jujo-Tecominoacán, 46 Pol-Chuc-Abkatún; Sinaloa St.: 47 Upper San Lorenzo River; Estado de México St.: 48 Ixtapan de la Sal and Tonatico.
GUATEMALA (GU): 1 Marlin mine, 2 Petén Itzá Lake, 3 Joaquina geothermal field, 4 Chinautla and Mixco.
EL SALVADOR (ES): 1 Coatepeque Lake, 2 Ilopango Lake, 3 Olomega Lake. For details and other areas affected see Figures 2 and 3.
HONDURAS (HN): 1 Valle de Siria.
NICARAGUA (NI): 1 Cerro Mina de Agua, 2 El Charco, Santa Rosa del Peñón, 3 Santa Cruz de la India, 4 Kinuma, 5 Zapote,
6 Llano La Tejera, 7 Tipitapa geothermal area. For a detailed As distribution map in water sources see Figure 4.
COSTA RICA (CR): 1 Rincón de la Vieja, 2 Miravalles, 3 Chocosuela-Platanar area, 4 NE Guanacaste, and Caño Negro, Gloria de Aguas Zarcas, Concepción in San Carlos in Alajuela Prov., 5 Cartago City, El Guarco, Turrialba volcano (Cartago Prov.).
PANAMA (PA): 1 Petaquilla, Colón, 2 Azuero Peninsula, 3 Damas Bay, Coiba Island, 4 Bocas de Toro Archipelago.
VENEZUELA (VE) : Lara St.: 1 Las Cuibas, Montañita and Cabudare, Palavecino Municipality, 2 Barquisimeto and Río Turbio, Iribarren Municipality, 3 Tunal Quíbor, rural zone and Caujaral, Jiménez Municipality, 4 Guarico, Río Tocuyo and Tocuyo, Moran Municipality, 6 Torres Municipality, 9 Sarare, Simón Planas Municipality, 11 Sanare, Andrés Eloy Blanco Municipality; Yaracuy St.: 7 Las Velas Yaritagua. Peña Municipality, 8 Aroa, Bolívar Municipality; Portuguesa St: 5 Guanare, Guanare Municipality, 10 Papelón, Papelón Municipality.
Colombia (CO): 1 Córdoba Dep., 2 Sucre Dep., 3 Bolivar Dep., 4 Santander Dep., 5 Antioquia Dep., 6 Cundinamarca Dep., 7 Caldas Dep., 8 Marmato mining district (Caldas Dep.), 9 Valle de Cauca Dep., 10 El Diamante gold mine (Nariño Dep.).
Ecuador (EQ): 1 Tambo River and Papallacta Lake area (Quijos County, Napo Prov.), 2 Guayllabamba, 3 Tumbaco, 4 Geothermal waters from El Carchi, Imbabura, Pichincha, Cotopaxi, and Tungurahua Prov., 5 Yacuambi River, 6 The gold artisanal mining areas of Nambija, Portovelo-Zaruma, and Ponce Enriquez, 7 Calera and Q. Calixto, 8 Intag forest region, 9 Puyango River (Calera and Amarillo rivers which are two of the tributaries of the Puyango River), 10 Portovelo (87 gold processing centers located along the Puyango-Tumbes River), 11 Provinces of Guayas and Los Ríos, and from national supermarkets in the cities of Quito, Daule, Milagros, and Babahoyo.
PERU (PE): 1 Morococha mining region/La Oroya smelting complex (Yauli Prov, Junin Dep.), 2 Rímac River basin, 3 Huaytará prov. (Huancavélica Dep.), 4 Puno (Puno Dep.), 5 Locumba valley (Tacna Dep.; Ilo City water supply), 6 Tacna area (Sama Quebrada de la Yarada, localities: Amopaya, Sama, Inclán; Tacna Dep.), 7 Mórrope Municipality (Lambayeque Dep.), 8 Pucallpa (Ucayali Dep.), 9 Altiplano-Puna, 10 Iquitos (Loreto Dep.).
BOLIVIA (BO): 1 Lower Katari basin, Cohana Bay, Titicaca Lake (T), 2 El Alto (La Paz), 3 Titicaca-Desaguadero-Poopó-Salt pans (TDPS) basin: T: Titicaca, U: Uru and Poopó lakes, 4 Poopó basin, 5 Upper Pilcomayo River basin, 6 North of Potosí Dep., 7 Lipéz and south of Potosí Dep.
CHILE (CL): 1 Chinchorro, Arica and Parinacota region, 2 Esquiña, Illpata, Camarones, Arica y Parinacota Region, 3 Puchuldiza geothermal field, Tarapacá Region, 4 Loa River basin, Chiu Chiu, Holjar and Toconce rivers, 5 El Tatio Geysers, 6 Antofagasta port, 7 Portreillo smelters, 8 Copiapó River valley, Atacama Region, 9 Valle del Elqui, El Indio, 10 El Indio, Coquimbo Region, 11 Ventanas industrial complex, 12 Maipo River basin.
ARGENTINA (AR): Principal regions: 1 Pampean plain, 2 Chaco plain, 3 Cuyo, 4 NW region and Puna, 5 Patagonian Andes, 6 Patagonia. Subregions: The subregions of 1-6 are listed in Table SM 4).
URUGUAY (UR): 1 Raigon aquifer, 2 Mercedes aquifer, 3 Chuy aquifer, 4 Guarani aquifer
PARAGUAY (PA): 1 Yenda-Toba-Tarijeño, 2 Guarani aquifer
BRAZIL (BR): Minas Gerais St.: 1 Iron Quadrangle” region (Nova Lima Dist., Santa Bárbara Dist., Ouro Preto/Mariana Dist., 2 Paracatu; São Paulo and Paraná St.: 3 Ribeira Valley region (Adrianópolis, Apiaí, Eldorado); Amapá St.: 4 Santana Dist., 5 Laranjal do Jari; Amazonas St.: 6 Anori, 7 Aruanã farm, Itacoatiara; Roraima St.: 8 Caracaraí; Acre St.: 9 Sena Madureira, 10 Rio Branco, 11 Xapuri; Mato Grosso St.: 12 Itaúba; Pará St.: 13 Santarem; Bahia St.: 14 Baía de Todos os Santos; Espirito Santo and Rio de Jaineiro St.: 15 Brazilian coast; Santa Catarina St.: 16 Coal mining areas (Lauro Müller, Treviso, Sideropólis, Criciúma); Rio Grande do Sul St.: 17 Patos Lagoon estuary.
DOMIMICAN REPUBLIC (DR): 1 Pueblo Viejo mining area, 2 Falcondo mining area, 3 Cerro de Maimón deposits, 4 La Duquesa landfill.
HAITI (HA): The Plaine du Cul-de-Zac aquifer, 2 The Etang Saumâtre Lake, 3 Trou du Nord River and Village of Fleury, Caracol Commune.
CUBA (CU): 1 Cienfuegos Bay, 2 Sagua la Grande River, northern Villa Clara Province, 3 Moa Bay, 4 Delita gold deposits, Juventud Island, 5 San Fernando mine.
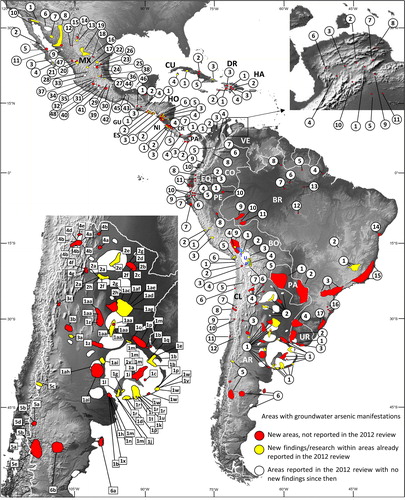
2.1.1. Arsenic in water
As by today’s knowledge (2020) As was reported in water supplies of more than half (16) of the Mexican states (31): Aguascalientes, Baja California, Chihuahua, Coahuila, Durango, Guanajuato, Hidalgo, Jalisco, Morelos, Nuevo León, Oaxaca, Puebla, San Luis Potosí, Sonora, Tlaxcala, and Zacatecas (, Table SM1, Supplementary material). By 2008, the groundwater As problem was known only in certain areas of 12 states and the number of people exposed to As in concentrations exceeding 50 μg L−1 had been estimated as 450,000 at that time (Armienta et al., Citation2008; Castro de Esparza, Citation2009). However, by 2018, about 1.5 million people (1.27% of the total population of 124,738,000 million in 2018) were estimated to have ingested water containing over 25 µg L−1 As (the national regulatory limit), and about 150,000 of them, in the highest range of 75 to 500 µg L−1 As (INCA, Citation2018). Limón-Pacheco et al. (Citation2018) estimated that around 500,000 children up to 14 years of age drink water with As levels of over 10 µg L−1, around 205,000 with 25 µg L−1, and approximately 17,500 between 75 and 500 µg L−1.
Approaches of research on As in Mexico have been quite variable. At the beginning, it was mostly targeting manifestations of chronic illness due to As exposure. However, environmental assessment studies related to As contamination were making inroads (Table SM1, Supplementary material and references therein). Nevertheless, (hydro)geochemical studies, e.g., on mobilization and mobility of As, were carried out in some areas like Concepción del Oro, Huautla, Juventino Rosas, Ixtapan de la Sal, San Antonio-El Triunfo, and Tabaloapa-Aldama (Table SM1, Supplementary material), in addition to those already included in Bundschuh, Litter, et al. (Citation2012) like Comarca Lagunera, Zimapán and Guanajuato. Research on mitigation efforts for As removal from water started only in the present century. Overviews on toxicological studies were presented by Armienta and Segovia (Citation2008), McClintock et al. (Citation2012) and Limón-Pacheco et al. (Citation2018). Treatment options and experiences were reviewed by Litter et al. (Citation2009, Citation2012, Citation2019b), Bundschuh et al. (Citation2010), and Alarcón-Herrera and Martín-Domínguez (Citation2018). Co-occurrence of As and F in groundwater was assessed by Alarcón-Herrera et al. (Citation2013). Arsenic in geothermal areas of Mexico has been reviewed by López et al. (Citation2012), Alfaro de la Torre et al. (Citation2018), Armienta et al. (Citation2018) and Morales-Simfors et al. (Citation2020). Since 2012, geochemical behavior has been studied at various geothermal areas by Villanueva-Estrada et al. (Citation2013), Páez-Sánchez et al. (Citation2013), Wurl et al. (Citation2013), Armienta et al. (Citation2014), Morales et al. (Citation2015), Morales-Arredondo et al. (Citation2018). These studies showed the importance of As sorption on Fe oxyhydroxides in submarine vents in the Gulf of California and the occurrence of geothermal As in the San Juan Bautista Londó aquifer, in Baja California Sur State (Villanueva-Estrada et al., Citation2013; Wurl et al., Citation2013). The concentration and distribution of As in geothermal wells at the Cerro Prieto geothermal power plant complex and occurrence of low levels (many of them below detection) in the groundwater at the adjacent agricultural zone (Armienta et al., Citation2014; Morales-Simfors et al., Citation2020) were reported. The role of water-rock interaction processes in the rhyolite matrix and relevance of fractures on As enrichment in groundwaters at the Bajío Guanajuatense (Central Mexico) area (Morales et al., Citation2015, Morales-Arredondo et al., Citation2018), and the presence of As in thermal springs at Cuitzeo Lake, Michoacán State (Páez-Sánchez et al., Citation2013) were also studied. Arsenic in water, co-produced during hydrocarbon exploitation has been discussed by Birkle et al. (Citation2010); however, since this study, no further data has been available.
At Comarca Lagunera, Mexico’s principle cattle-breeding area, As concentration in cow-milk and As translocation from groundwater to cows is of particular importance and was studied for the first time in Mexico by Rosas et al. (Citation1999; see also review by Bundschuh, Nath, et al., Citation2012). Monroy-Torres et al. (Citation2009) related presence of As in hair (mean of 1.3 mg kg−1) of children living in rural areas of Guanajuato to the use of contaminated water to cook beans, broths or soups, besides direct drinking of polluted well water.
2.1.1.1 Arsenic in groundwater
Environmental studies of As started slowly in Mexico during the early 1960s; first in areas from where health effects were reported, focusing on total As in drinking water drawn from wells at Comarca Lagunera region (Chávez et al., Citation1964; Cebrián et al., Citation1994). As speciation in groundwater and As sources were determined in that area (Del Razo et al., Citation1993). Initial reports from Comarca Lagunera () claimed pesticides as cause for As contamination of groundwater in this area, whereas a former hydrothermal deposit was proposed as natural As source; the last was justified by the co-occurrence of groundwater As with boron (B), fluoride (F) and lithium (Li) (González-Hita et al., Citation1991). Following up research on (hydro)geology and (hydro)geochemistry indicated mechanisms such as sulfide oxidation, release of As by dissolution of iron (Fe) and manganese (Mn) oxides and evaporative concentration increase of As in areas with dry climate causing high As groundwaters (Ortega-Guerrero, Citation2003; Molina, Citation2004; Gutiérrez-Ojeda, Citation2009). Mejía-González et al. (Citation2014) proposed that pH increase leads to release of As from sediments to the water. On the other hand, inflow of As-rich water to the granular aquifer was related to a reversal of the hydraulic gradient due to intensive pumping and a decrease of freshwater infiltration due to a dam construction (Ortega-Guerrero, Citation2017). In a sampling campaign carried out in 2013, Sariñana-Ruiz et al. (Citation2017) measured As concentrations above 10 µg L−1 in about 95% out of 59 groundwater samples (maximum: 650 µg L−1) collected at Comarca Lagunera. Other areas in northern Mexico affected by high As groundwater are Sonora (with concentrations up to 31 µg L−1) and Chihuahua states (Wyatt et al., Citation1998; Alarcón-Herrera et al., Citation2001; Roberge et al., Citation2012; Alarcón-Herrera et al., Citation2013; Reyes-Gómez et al., Citation2013) ().
Based on the hydrogeology, the geogenic origin of As was suggested for Chihuahua groundwater samples of the Meoqui-Delicias aquifer. Arsenopyrite dissolution has been associated with As presence in the northwest area of the aquifer, while a geothermal influence was identified at Julimes Municipality in the northeast, and also in the Guadiana Valley of Durango State (; Alarcón-Herrera et al., Citation2013). Igneous rocks, conglomerates and alluvial deposits were proposed as the principal As source in the aquifers of Chihuahua, San Luis Potosí, Durango, and Sonora states (Alarcón-Herrera et al., Citation2013). Particularly, in central Chihuahua, rhyolites were found to release As to the alluvial aquifer system (Reyes-Gómez et al., Citation2013). Drinking water was found to contain up to 245 µg L−1 As and up to 11.1 mg L−1 fluoride in one neighborhood (Colonia 5 de Febrero) of Durango City (Rocha-Amador et al., Citation2011).
In-depth hydrogeological and (hydro)geochemical studies in central Mexico, viz. on Independencia aquifer of Guanajuato State, the Río Verde in San Luis Potosí State and the Zimapán, Hidalgo State valleys (), showed that the groundwater As sources are natural, i.e., geogenic (Armienta et al., Citation1997, Citation2001; Mahlknecht et al., Citation2004; Ortega-Guerrero, Citation2009; Sracek et al., Citation2010). A geothermal origin has been identified in other zones of Guanajuato State (Juventino Rosas) and Estado de México State (Ixtapan de la Sal and Tonatico) (Morales-Arredondo et al., Citation2018) ().
2.1.1.2 Arsenic in surface water
Arsenic concentrations in water and sediments were studied for three storage lakes (El Granero, La Boquilla, Las Vírgenes) in Chihuahua State during the period 2005–2006. In the lake sediments, mean As content was between 9.11 and 16.62 mg kg−1, without showing remarkable differences between the individual lakes and seasons (Hernández-Garcia et al., Citation2008). In lake water, concentrations of As were between 6 and 21 µg L−1; however, with exception of a single sample (Las Virgenes Lake, sampled in spring 2006, 170 µg L−1; Hernández-García, Citation2007). Arsenic has been also reported from other storage reservoirs in Chihuahua State; namely, Chuviscar, Chihuahua, El Rejón and San Marcos. Their sediments were sampled in three different seasons (spring, summer, autumn) of 2008 and showed mean As concentrations between 3.147 and 7.307 mg kg−1; with no water sample exceeding the WHO guideline value for drinking water (10 µg L−1; Cano de los Ríos, Citation2010; Pérez Vargas, Citation2010). For the As detected in the storage lakes, a component of residual waters has been proposed as source by Hernández-García (Citation2007); however, agrochemicals could be another important anthropogenic source contributing As. In addition, weathering and leaching of acid igneous rocks and sulfide minerals could be a geogenic source of As. At Chelem Lagoon in southern Mexico (Yucatán State), concentrations of As were found to be above sediment NOAA Apparent Effect Threshold (AET) Screening Quick Reference Tables (35 mg kg−1; Arcega-Cabrera et al., Citation2015). Sediments collected near the river mouth of Cuitzeo Lake, Michoacán State, showed a moderate zinc and arsenic pollution. However, fractionation results indicated a medium potential risk to the biota, since these elements could be released to the lake ecosystem, depending on the environmental conditions (Villalobos-Castañeda et al., Citation2010).
Gutiérrez et al. (Citation2008) identified sources of As in water and sediments in the middle of the Río Conchos basin, Chihuahua State. The spatial distribution in the geological and hydrogeological framework, together with correlation analyses of As with Sb, Cu and Bi, suggested that As was provided mainly by the mineralization in the area, although sewage could also be an As source to the river water.
2.2.3. Arsenic in soils and sediments
Concentration of As and its sources and mobility have been studied for river, lake, and coastal sediments in the states of Baja California, Chihuahua, Guerrero, Hidalgo and Sonora. Whereas no As contamination of coastal sediments from the La Paz Lagoon (Sonora State) was reported (Shumilin et al., Citation2001; Jara-Marini & García-Rico, Citation2006), sediment core samples from the remnant delta of the Colorado River, Baja California Norte State () were reported to have As contamination traced to the pesticides applied in the first half of the 19th century in the catchment area of the river (Daesslé et al., Citation2009).
River sediments are often impacted by mine tailings, frequently located on or close to the river banks at mining sites impacting the local settlements and the downstream area of the river (García et al., Citation2001; Gutiérrez-Ruiz et al., Citation2007; Gutiérrez & Carreón, Citation2008; Espinosa et al., Citation2009). At the San Antonio mining district (Baja California Sur State), Shumilin et al. (Citation2015) examined the mobility of As by treating samples with various extractants in the dry stream sediments of the area. Reactive As was found to increase downstream, probably as a result of As release from the mineral sulfide fraction of the tailings. Páez-Osuna et al. (Citation2015) evaluated As (and heavy metals) concentrations in water and suspended sediments at San Lorenzo River (northwest Mexico) following a tailing spill upstream.
Total, bioaccessible (leached by simulated human gastric and intestinal phases) and mobile (leached by CO2-rich water, pH = 5.5) concentrations of As and F were determined in soils at Comarca Lagunera to assess the environmental behavior of As; thereby it was found that the bioaccessible percentage reached 63% in the gastric phase for As (Sariñana-Ruiz et al., Citation2017). Ruiz-Huerta et al. (Citation2017) determined total As concentrations (up to 172 mg kg−1) and geochemical controls on available As in soils irrigated with As-polluted water in Matehuala, San Luis Potosí State. Arsenic was found to be highly soluble in water in soils rich in calcite and lower in those rich in gypsum. However, As bioconcentration in maize crops showed the opposite trend. High bioaccumulation, up to 2.5 times of bioconcentration and 45% of translocation indicated a significant risk for the population consuming the crops irrigated with As-rich water.
2.2.4. Arsenic in mining areas
In different mining areas of Mexico, As occurrence has been reported in different environments (ground- and surface-water, soil and sediment, tailings and other waste products). Arsenic released naturally from geogenic sources or through mining activities to groundwater has been studied at different sites (Table SM1, Supplementary material; Castro-Larragoitia et al., Citation1997; Armienta et al., Citation1997, Citation2001; Carrillo-Chávez et al., Citation2000; Razo, Carrizales, et al., Citation2004; Rodríguez et al., Citation2005; Esteller et al., Citation2015). Mineralogical and petrochemical analysis, together with hydrogeochemical modeling, established oxidation of arsenopyrite and dissolution of scorodite as mechanisms for As mobilization into a deep limestone aquifer at Zimapán (central Mexico) (Armienta et al., Citation1997, Citation2001).
In the Zimapán area, deep aquifers contaminated by As from dissolution of natural As sources could be distinguished from shallow wells with As contamination originating from tailings by chemometric and isotopic approaches (Sracek et al., Citation2010). In one of the mining areas of San Luis Potosí State, As presence has been reported in mine tailings, soils and water in Santa María de la Paz, (; Castro-Larragoitia et al., Citation1997). A later study in this zone indicated that oxidation of arsenopyrite and natural sulfide dissolution at high pH and under oxidizing conditions are the principal mechanisms of As release into groundwater (Razo, Carrizales, et al., Citation2004). In the mining area of San Antonio-El Triunfo (Baja California Sur State, ), high As concentrations were reported close to the mining waste piles (), for which principal hydrogeochemical processes were identified along the groundwater flow path, viz. adsorption of As onto iron-hydroxides surfaces and precipitation of calcite (Carrillo-Chávez et al., Citation2000). In the southern section of the mining district, As in groundwater was investigated and possible As sources were determined (Wurl et al., Citation2014). Concentrations of As (and heavy metals) were determined in waste rocks, tailings, stream water and sediments, groundwater and soils to assess their sources and environmental fate in the mining zone of Concepción del Oro, Zacatecas (Castro-Larragoitia et al., Citation2013).
Mining-related environmental impacts, including As mobilization from different types of mining wastes and release through smelter dusts and fumes as well as its dispersion, were studied at different mining and ore processing sites in the states of Baja California, Chihuahua, Guerrero, Guanajuato, Hidalgo, San Luis Potosí and Sonora (, Table SM1, Supplementary material). Geochemical and detailed mineralogical studies have been used to outline the processes and related mechanisms for As (and heavy metals) mobilization into different environments (surface- and groundwater, soil, sediment, atmosphere, etc.: Talavera Mendoza et al. (Citation2005), Gutiérrez-Ruiz et al. (Citation2007), Romero et al. (Citation2006, Citation2008) and Armienta et al. (Citation2012). The mobility of As has been assessed by mineralogical and physico-chemical approaches and sequential extraction experiments have been applied to mine tailings from mining areas in the states of Hidalgo, Guanajuato and Chihuahua (Méndez & Armienta, Citation2003; García-Meza et al., Citation2004, Citation2006; Ramos-Arroyo & Siebe, Citation2007; Armienta et al., Citation2012, Carrillo-Chávez et al., Citation2014; Gavilán García et al., Citation2017; Zúñiga-Vázquez et al., Citation2019). Isotopic characteristics, (hydro)geochemical modeling and leaching experiments were combined to assess the mobilization of As from mine tailings and smelter slags and the mobility of As toward different adjacent environments at the mining areas of Guanajuato, Santa María de la Paz and Taxco (, Table SM1, Supplementary material) (Manz & Castro, Citation1997; Talavera Mendoza et al., Citation2006; Mendoza-Amézquita et al., Citation2006; Martínez-Villegas et al., Citation2013; Carrillo-Chávez et al., Citation2014; Dótor-Almazán et al., Citation2017).
The contamination of soil by As originating from tailings and ore smelting activities has been accessed at different mining sites. The influence of historic/legacy mining and ore processing sites with its tailings and residues from smelting activities on As concentration and horizontal and vertical (with depth) distribution pattern of As in soils have been investigated at Zimapán mining area (), and respective liability has been determined (Ongley et al., Citation2007). Further investigations in the same area assessed the mobility of As (and other heavy metals) in soils impacted by tailings with distinct degrees of oxidation by applying sequential extraction in surrounding soils (Armienta et al., Citation2016). The influence of historic (inactive) tailings has also been researched at the mining area of Santa Barbara in the Chihuahua State (), determining As concentrations in soil (total and soluble fractions; Gutiérrez, Citation2007). Another assessment of the environmental impact of an abandoned historic mining sites was done in the San Antonio el Triunfo area (Baja California Sur State), where As concentrations have been determined in soils and water (Carrillo-Chávez et al., Citation2000; Naranjo-Pulido et al., Citation2002; Wurl et al., Citation2018). Arsenic bioaccessibility assessment of soils from the Santa Maria de la Paz mining area (San Luis Potosí State) found a 4-times higher As bioaccessibility in soils impacted by a former smelter than soil impacted by mining waste (using PBET method – in vitro Physiologically Based Extraction Test; Razo, Téllez, et al., Citation2004). At Cerro de Mercado (Durango State, , Table SM1, Supplementary material), presence of arsenopyrite, galena and As-bearing mineral phases (As atomic % from 0.31 to 3.75 for n = 10, according to EDS analyses) in soils have been found near active and old tailing impoundments of this Fe-oxide rich mining district (Morales et al., Citation2015).
3. Central America
3.1. Guatemala
Guatemala presents few published studies during the last decade that have investigated As. These studies include arsenic in drinking water (Prado et al., Citation2016), arsenic in soils for anthropological studies of the Maya civilization (Battistel et al., Citation2018), possible contamination of the Marlin Mine (Basu et al., Citation2010), and geochemical and thermal studies at the Joaquina geothermal field (Libbey et al., Citation2015).
3.1.1. Arsenic in water
Guatemala, and 10 other Latin American countries, have adopted the WHO drinking water standard for As (10 µg L−1; Bundschuh, Litter, et al., Citation2012 and references therein; WHO, Citation2017). The Department of Toxicology of the School of Chemistry and Pharmacy of the Universidad de San Carlos de Guatemala (Prado et al., Citation2016) investigated the levels of dissolved As in drinking water from different areas of the Municipality of Guatemala City using atomic absorption spectrometry with hydride generation (Spectrometer Perkin Elmer Model 700). Results of this study showed that 25% of the samples had As concentrations above the WHO and the national drinking water guideline. Among the As contaminated samples, half were from water wells and the other half from the municipal network. Actual values of the concentrations and sample locations were not reported.
3.1.2. Arsenic in soils and sediments
Six cores from the Lake Petén Itzá were analyzed for heavy metals, As, rare earth elements, organic and inorganic carbon and organic matter content (Battistel et al., Citation2018) with the purpose to reconstruct the environmental history of the area. Principal component analysis was applied to the results of the different samples at different depths (up to 3 m). Results were also compared with oxygen isotopes and pollen content, as reported in other works (Rosenmeier et al., Citation2002; Wahl et al., Citation2006). The core layers were also dated using radiocarbon techniques and encompassed the time period between 5500 a BP to the present.
This time period included the Preclassic (4000–1700 a BP) and Classic Periods (1700–1000 a BP), when Maya tribes extensively cleared land for agriculture. Concentrations of As ranged from 4.5 to 15.6 mg kg−1 (mean 7.05 mg kg−1, s.d. 1.82 mg kg−1). Enrichment factors for the different elements were calculated using a simple formula proposed by Reimann and De Caritat (Citation2000). The temporal profiles of enrichment factors for Hg and As showed one peak for Hg around 2250 a BP, while As showed two distinct peaks at 1330 and 2500 a BP, respectively. These peaks exceeded several times the background enrichment factors for Hg and As, and are assumed to be due to Hg and/or As that was atmospherically transported to the basin, such as during volcanic eruptions. Comparison with volcanic eruptions studies in the region suggests that these peaks were produced by eruptions from Chichon, Fuego, and Acatenango volcanoes.
3.1.3. Arsenic in mining areas
The operation of the Marlin Mine in Guatemala had been associated with As related health problem in the population close to the mine. Basu et al. (Citation2010) studied the heavy metal and As content of different environmental phases and in biological samples of the local population. Al, Mn, Co, Ni, Cu, Zn, As, Cd, Pb, and Hg concentrations were determined in blood and urine of 23 participants, 5 of them being miners. All the metals in the biological samples were lower than reference values for toxic effects reported by the United States Centers for Disease Control and Prevention (US CDC) and the Handbook on the Toxicology of Metals (Nordberg et al., Citation2007), except for Cd in blood (median 0.0012 mg L−1, range: 0.74–2.4 µg L−1, US CDC reference value <1 µg L−1) and within acceptable levels in urine (median 0.11 µg L−1, range: 0.05–0.2 µg L−1, US CDC reference value <1 µg L−1). Arsenic in the blood samples (median 3.9 µg L−1; range: 3.2–8.5 µg L−1) and in urine (median 0.06 µg L−1; range: 0.04–16.7 g L−1) was lower than the reference values of 0–5 µg L−1 for US CDC for blood, and <100 µg L−1 for US CDC and <50 µg L−1 reported in the Handbook of Toxicology for urine. However, when the distance of the household to the mine was considered, the concentrations of Cr, Ni, Cu, Zn, As, Cd, and Hg in urine were higher for the people living closer to the mine. For the river waters draining the area (four sites were sampled twice), concentrations of Al, Mn, Co and Zn were lower upstream from the mine than downstream. Arsenic concentrations were higher for the upstream sample than for two of the three downstream sites, probably reflecting geogenic As of the area. Sediments were also sampled and analyzed at four sites with results similar to the trends of the respective water samples, with lower concentrations upstream from the mine and higher concentrations downstream. However, the differences were smaller in the case of sediments.
3.1.4. Arsenic from other sources
The Joaquina geothermal field has been investigated for the concentrations of CO2, Hg, Sb and As in soils and soil temperature in the thermal areas, together with chemical and isotopic composition of the discharged fluids, chemical composition of the cores of drilled boreholes, lithology and geological structures such as faults (Libbey et al., Citation2015). Joaquina geothermal field is a non-magmatic, deep-circulation-type system similar to those in Honduras (i.e., Platanares and Azacualpa). In these systems, meteoric waters penetrate deep into the crust and are heated to high temperatures (∼175–185 °C for Joaquina). Fluids ascend to the surface due to buoyancy along the high permeability pathways of faults, which present a complex structure in this region close to the Motagua fault system. Fluid flow is focused within the NE-trending left-lateral and ESE- striking normal faults. The composition of the water discharged at Joaquina is Na-bicarbonate (-sulfate)-rich with low Cl reflecting the lack of a magmatic component and the chemical composition of the metamorphic host rocks. The presence of sulfur, CO2 and CH4 and its isotopic composition suggest interactions between the hydrothermal fluids and metasedimentary rocks at depth. In the thermal areas with fumarolic discharges, temperature, CO2, Hg, Sb seem to be well correlated, reflecting the enrichment of this elements at the soil-air interface. For As in soils, Libbey et al. (Citation2015) have identified four population data: the background population in the soils of this area ranges from 0.5 to 7.1 mg kg−1, the anomalous population ranges from 7.1 to 24.0 mg kg−1, the highly anomalous population from 24.0 to 74.9 mg kg−1, and the very highly anomalous population from 74.9 to 107.6 mg kg−1. The profile of As obtained with the chemical composition of core samples collected from boreholes ranges from less than 50 to more than 1000 mg kg−1.
3.2. El Salvador
During the last decade, several studies in different fields in El Salvador have included determination of As. In addition to As in geothermal areas (López et al., Citation2012) and caldera lakes in El Salvador (López et al., Citation2004; López et al., Citation2009), other natural and anthropic systems also present high concentrations of As: potable drinking water, water supply wells; surface waters in rivers and lakes; soil, sediment and water of a pesticide contaminated site; and mining areas in the eastern part of the country.
3.2.1. Arsenic in water
Arsenic has been studied in potable water (groundwater) and surface waters of El Salvador by the National Institute of Health (Quinteros, Citation2014) and the Ministry of the Environment and Natural Resources (MARN, Citation2017).
3.2.1.1 Arsenic in groundwater
Arsenic in potable water and water supply wells has been investigated during the last decade in El Salvador. In 2014, the National Institute of Health of the Ministry of Environment in El Salvador carried out an investigation to determine arsenic in potable water in the country (; Quinteros, Citation2014). A total of 49 water samples were analyzed from 49 different municipalities out of 262 in the country. Out of 49 samples, 43 were from the water distribution network and six directly from wells. Higher concentrations of arsenic in potable water were found close to Ilopango Lake and San Salvador City. The sampled area represents a population of 2.24 million, which is 35.5% of the total population of El Salvador. Concentrations of As ranged from 5 to 78 µg L−1, with one sample having a concentration of 160 µg L−1 that belongs to a well located in a region explored for gold deposits. 21 out of 49 samples presented concentrations of As higher than the national norm (10 µg L−1) and represent 56.8% of the population in the investigated areas. These results indicate that a great proportion of El Salvador population is likely to drink water with arsenic higher than the standard for drinking water.
Drinking water in El Salvador is extracted from 71 aquifers of variable size (MARN, Citation2017) associated with the active volcanoes in the country (Morales-Simfors et al., Citation2020), as highly fractured volcanic rocks – blocky lava flows associated with high silica content in basaltic andesitic and rhyolitic rocks – from these aquifers. Two volcanic aquifers are quite important in the region, as they provide water to two large cities in the country: the aquifers associated with San Salvador and San Miguel volcanoes (see location of volcanoes in ). Arsenic concentrations for wells located in San Miguel volcanic aquifer have concentrations ranging from 13 to 31 µg L−1. For wells in San Salvador volcanic aquifer, As concentrations range from 15 to 64 µg L−1. The highest As concentration of 73 µg L−1 occurs in a well close to the town of Santa Rosa de Lima (). This well also presents high temperature (66 °C) and it is obviously having some geothermal fluid input.
Figure 3. Arsenic in rivers of El Salvador (after Mejía López, Citation2019). Data from MARN (Citation2017). Insert represents the mined region of El Salvador and the rivers affected by mine contamination.
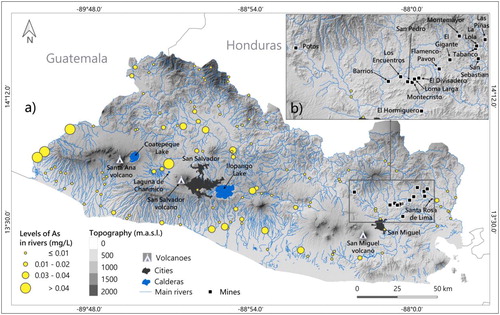
3.2.1.2 Arsenic in surface water
The Ministry of Environment and Natural Resources (MARN) in El Salvador often tests the river waters for contaminants like heavy metals and arsenic, biochemical oxygen demand (BOD), chemical oxygen demand (COD), dissolved oxygen, cyanide, phosphate, sulfate, alkalinity, fluoride, chloride, sulfide, nitrate, nitrate, ammonium, and field parameters such as pH, electrical conductivity, and total dissolved solids, as it is reported in the last collected data in 2017 (MARN, Citation2017). Researchers from the Ministry of Health (MINSAL) have analyzed the data and constructed maps of the concentrations of different ions, including As (Mejia López, Citation2019). The distribution of As in the rivers of El Salvador is shown in . The average detectable As concentration in the rivers of El Salvador is 13 µg L−1, ranging from a minimum detectable concentration of 0.23 to a maximum of 105 µg L−1. The highest concentrations of As in river water occur near the El Salvador-Guatemala border (close to the geothermal fields of Ahuachapán and Chipilapa) and in the slopes of San Salvador Volcano (). The water quality of the rivers in El Salvador for 2017, according to their WQI (Water Quality Index), presented 32% with good water quality (total analyzed samples 117), 59% regular, and 9% bad, showing an improvement from previous years (MARN, Citation2017). For example, in the previous data collection in 2013, while 5% of the sites presented good water quality, 73% presented regular, 17% bad and 5% very bad water quality. However, in terms of As, it should be noted that two of the three sites with very high As in the Paz River (border with Guatemala) have been classified as “good” water in the MARN report, and the third sampling point with also high arsenic in this river, has been assigned “regular” water quality. These 3 sites present concentrations of 101–105 µg L−1 of As. The WQI index considers the following parameters: dissolved oxygen, fecal coliforms, pH, BOD5, nitrate, phosphate, temperature, turbidity, and total dissolved solids. It is clear that consideration of heavy metals and arsenic should also be included in the calculation of the WQI.
Three volcanic lakes have been investigated recently in El Salvador (Cabassi et al., Citation2019): Coatepeque Lake in Coatepeque caldera, which is part of the Izalco-Santa Ana-Coatepeque volcanic complex, Ilopango Lake nested in Ilopango caldera, and Chanmico Lake, which is located on a monogenic crater related to the San Salvador Volcano (see ). Ilopango and Coatepeque have depth reaching around 240 and 115 m, respectively, and have resurgent domes close to the caldera walls, and in the case of Ilopango, one dome (Islas Quemadas) at the center of the lake. Ilopango and Coatepeque present periods of stratification from around April to October and periods of convection between October and April, mostly due to variations in wind velocity rather than big changes in temperature (López et al., Citation2004, Citation2009; McCutcheon, Citation1998). Cabassi et al. (Citation2019) do not report the date of the samples and profiles of field parameters, but they found the three lakes stratified, which suggests they worked some time during the stratification period. The recent data reported in Cabassi et al. (Citation2019) describe the composition of Coatepeque and Ilopango lakes as a Na-Cl type water with high concentration of bicarbonate (323–353 mg CaCO3 L−1 for Coatepeque, and 330–366 mg CaCO3 L−1 for Ilopango), pH ranging from 7.8 to 8.8 for Coatepeque and from 7.5 to 9.1 for Ilopango with a decreasing trend with depth for both lakes. Hydrothermal fluids input are suggested to feed these two lakes, as it is evidenced by the Cl/Br–molar ratios ≤ 650, and high As, B, Li and Si contents and Cl–/ ratio >1 (Cabassi et al., Citation2019). Arsenic concentrations found in Cabassi et al. (Citation2019) study were in general lower than previous studies (López et al., Citation2004, Citation2009; McCutcheon, Citation1998) and ranged from 88 to 104 µg L−1 for Coatepeque, and 88 to 644 µg L−1 for Ilopango Lake. In both the lakes, a mild decreasing trend with depth can be observed. In comparison, Chanmico presents a Mg-HCO3 type water with lower total dissolved solids than Ilopango and Coatepeque lakes, the high Mg concentration suggest interactions with mafic/ultramafic rocks (Cabassi et al., Citation2019). Chanmico is a shallower lake and sampling in the Cabassi et al. (Citation2019) study reached 47 m of depth. Chanmico Lake water has a pH ranging from 7.3 to 9.3 that decreases with depth, bicarbonate concentrations ranging from 310 to 522 mg CaCO3 L−1 that increases with depth, and As concentrations ranging from 6.1 to 13 µg L−1 that increases with depth. This contrasting behavior of As with depth of the two caldera lakes with respect to Chanmico Lake envisages further investigation.
3.2.3. Arsenic in soils and sediments
Arsenic in soil, sediment and water was investigated in a pesticide contaminated site in El Salvador. Loma del Gallo, a rural community located close to the Pacific coast in central El Salvador, was investigated for the concentration of heavy metals and As (Quinteros et al., Citation2017). The reason for this research was that a pesticide factory (QUIMAGRO) abandoned large amounts of pesticides during the 1980s and the population was suffering of illnesses like chronic kidney disease of unknown cause (CKDu). The investigation had the task to identify the extent of the contamination through analysis of samples of ground and surface waters in the vicinity of the factory and the community. Water wells and samples from the streams that cross the area were analyzed. Groundwater analysis results showed hardness values ranging from 19 to 247 mg L−1, with a mean of 67 mg L−1, and pH values varying between 6.1 and 7.3. Arsenic was detected in 8 of the 13 sampled wells, with the highest value of 12 µg L−1 and a mean of 0.5 µg L−1. Cadmium was also detected in two wells, with 4 µg L−1 as the highest value. For the surface waters, pH ranged from 6.9 to 7.9, with an outlier of 10 in a sampling point located in the northwestern part of the study area. Arsenic was detected in all surface sampling points, ranging from 7 to 26 µg L−1, with variability in all the sampling regions that could have been caused by the volcanic origin of the soils in the area. Cadmium was detected in five surface samples downstream from the factory with values ranging from 0.08 to 0.1 µg L−1 in the streams crossing the sugar cane plantations, suggesting that agrochemicals used in sugar cane cultivation could be the source for Cd.
3.2.4. Arsenic in mining areas
Mining exploitation in El Salvador started during the colonial time with at least 15 gold and silver mines that were exploited, one of them (San Sebastian, ) closed the industrial exploitation as late as 1998. Three departments of the country have been affected by that exploitation (Morazán, San Miguel and La Unión, ). Discharges of acid mine drainage have been identified in some of the mines and the region has been studied by government organizations, consulting companies and non-government organizations. In 2017, the National Congress (Asamblea Legislativa) of El Salvador emitted a law that forbids exploitation of metal mines, underground as well as open pit mines. The law also contemplates the closing of the abandoned mines and possible environmental remediation. For that reason, several studies have been carried out about the contamination of rivers, groundwater, sediments, and mine waste in the mining areas (Ticay et al., Citation2015; Domínguez et al., Citation2015; CEICOM, Citation2010a). Data from those studies was used by López (Citation2019) to analyze the results of concentration of heavy metals and As in the different environmental phases. However, it should be noted that the extension of the soil contamination with Hg, As and other metals and metalloids in the mined areas has not been evaluated yet. Unfortunately, those soils are often used for agricultural purposes and animal pasture with great risk of incorporation of the contaminants to the food web.
In the Domínguez et al. (Citation2015) study, nineteen well and spring waters were analyzed. In general, the concentrations were lower than the standards for drinking water of El Salvador, As was not detected in all the samples. However, sulfate and iron concentrations were high in samples from wells located close to San Sebastian mine (), suggesting likely mine water contamination. For the stream waters, fifteen samples were collected, five of which had pH lower than 3 and the rest of them had pH higher than 6. Sulfate was also high for those samples (between 500 and 2290 mg L−1). Of the heavy metals, iron, lead, cadmium and zinc presented values higher than the US EPA (United States Environmental Protection Agency) criteria for aquatic life. The area with highest values was for those samples located in the vicinity of the San Sebastian mine (). For As, all the surface waters presented values lower than the criterion for aquatic life (150 µg L−1), probably due to sorption on abundant iron hydroxide sediments produced by iron oxidation in acid mine drainage sites. Eight of the fifteen samples presented detectable As with values ranging from 7 to 17 µg L−1.
For the sediments, 24 samples of the rivers in the region were analyzed. The obtained values were compared with the concentration limits presented by the United States Environmental Protection Agency (US EPA, Citation2001). Note that in the sediments, As, Cu, Pb, Zn, Hg, and Cd have values higher than the norm with the highest values close to San Sebastian mine. Arsenic in sediments was detected in all the samples with values ranging from 2.6 to 138.6 mg kg−1. Arsenic concentration was higher than the limit for aquatic life (8.2 mg kg−1) in 14 of the 24 samples (). For the mine waste abandoned in the mining areas without any protection for the population, fifteen samples were analyzed (Domínguez et al., Citation2015). López (Citation2019) compared the concentrations with the limits for agricultural soils established in Canada, instead of the standard for industrial soils, because the soils in this area are used for agricultural purposes. The sediments associated with the mine waste materials, revealed high levels of metals and As. However, the levels of Hg were below the limit for agricultural soils (6.6 mg kg−1) in Canada (CCME, Citation2019). This is surprising, because amalgamation was the preferred method used in the mineral processing in these mines. However, amalgamation also leads to volatilization of mercury from the ore material, which could explain the low concentrations. Arsenic was detected in the fifteen mining waste sites with concentration ranging from 6.1 to 76.4 mg kg−1. Concentrations of As higher than the values for agricultural soils (12 mg kg−1) were found in six of the fifteen samples, with the highest values in spoils of the mines Las Piñas, San Sebastian, Montecristo, and El Hormiguero ().
CEICOM (Citation2010a) carried out a study to determine the contamination produced by San Sebastian mine. Two wells, four surface waters samples and water discharged at the mine opening were sampled during the dry and the wet season. The results during the wet season were similar to those reported in the Domínguez et al. (Citation2015) study. In both the studies, As was low or below detection limit in the surficial and well waters, but higher closer to the mine opening, with 182 µg L−1 at the mine opening and two other values of 16 and 12 µg L−1 in other two surface water samples. Mercury was non-detectable as in the Domínguez et al. (Citation2015) study. During the dry season, arsenic was not detected in all the samples. Higher flows discharged from the mine during the wet season can explain this behavior. During the wet season the water level within the mine pool should increase, as a result of washing of the sulfide oxidation products from the mine walls and discharging water with As and other metalloids and metals.
CEICOM (Citation2010b) also studied the soils and sediments of a village in the vicinity of El Divisadero mine (see ). Four soil and one sediment samples were analyzed. Results showed that all the analyzed metals (As, Pb, Al, Zn and Cd) have levels higher than the limit for agricultural soils of Canada and the US EPA standard for sediments. In the case of As, soil concentrations ranged from 9.4 to 72.9 mg kg−1, with 3 of the samples with values considerable higher than 12 mg kg−1, the Canadian limit for agricultural soils (CCME, Citation2019). For the sediment sample, As was 21.2 mg kg−1 also higher than the US EPA limit of 8.2 mg kg−1.
All these investigations show that river sediments and soils are contaminated with As and heavy metals in this mined region of El Salvador, presenting a health risk for the population, as well for aquatic and terrestrial life. A more detailed study of mine waste contamination in each site and the extension of the contamination in the rivers is suggested.
3.3. Honduras
There is no peer-reviewed literature on As for Honduras. However, some work has been done in student thesis and probably other sources. Arsenic in rice cultivated in Honduras has been studied by Pazmiño Sánchez and Mendieta Arguello (Citation2018).
3.3.1. Arsenic from other sources
The purpose of the Pazmiño Sánchez and Mendieta Arguello (Citation2018) study was to determine the accumulation of As in two rice varieties DICTA Playitas and DICTA Comayagua, as a function of the type of irrigation and type of cultivation. The two types of irrigation used in the experiment were flood irrigation and drip irrigation. Two cultivation types were investigated: direct and indirect. Direct is when the seed is planted directly in the soils, indirect is when the seed is first planted in a greenhouse and the small plants are transferred later to the cultivation parcel. Eight different land blocks were planted using different combination of the studied factors. Results showed that the yield using flood irrigation methods was higher than for drip irrigation. Concentration of As in the initial irrigation water was 2.3 µg L−1 and in the soils was 12.64 mg kg−1. The final concentration of As in the soils where drip irrigation was applied was higher than the As concentration in the soils where flood irrigation was applied, 20.02 and 12.64 mg kg−1, respectively. In comparison, concentration of As in rice was higher in the rice cultivated with flood irrigation than in drip irrigation, as well as in rice cultivated using direct planting methods. Concentrations of As in rice cultivated by direct methods and under flood irrigation conditions were 4.52 and 3.07 mg kg−1 for Comayagua and Playitas rice, respectively. Indirect methods of planting gave also low values for As concentration in rice. Arsenic concentration was 1.8 µg L−1 for the drip method, direct and indirect planting methods, for the two kinds of rice. Indirect methods of planting and flooding method of irrigation gave also 0.0018 mg kg−1 As for both types of rice. This research shows that flood irrigation and indirect planting are probably the best methods to cultivate rice in this area, because a good yield with low concentration of As is produced.
3.4. Nicaragua
It has been 25 years since the detection of the highest concentration of As (1320 µg L−1) in groundwater for human consumption till date (2020) in the country, from a community well drilled at El Zapote in the Sébaco Valley of Nicaragua (Aguilar et al., Citation2000; Gómez, Citation2002). Since then different studies have been carried out in certain communities in 12 of the 16 departments in which the country is divided, as well as in the two autonomous regions (RACCN: Autonomous Region of the North Caribbean Basin and RACCS: Autonomous Region of the South Caribbean Basin). It is estimated that 327 communities, distributed in 11 departments have As concentrations that exceed the limit established by WHO (Citation2011) guideline for human consumption (Table SM2, Supplementary material).
According to the multiple studies carried out in the country, the presence of As in water sources is considered to be of geogenic origin, associated with: (1) volcanism in the Nicaragua Depression and the ascent arsenic rich geothermal waters through faults; (2) the gold veins in the mining districts; and (3) the Cenozoic volcanic and sedimentary rocks. Arsenic contamination is reported in all the five geomorphological provinces in which the country is divided (Figure SM1, Supplementary material). In this section the total As data available for the period 2010–2019 have been presented; however, it is likely that there are more sites with As presence in the country, but the respective information is not available to the public.
3.4.1. Arsenic in water
The first study related to As in Nicaragua (INAA, Citation1996) was carried out in the Central Plateau Province, where As is present in the ferric and sulfate rocks (as arsenopyrite) due to the Cenozoic volcanism, and is also associated to epithermal and gold veins in mining districts. After this study, several investigations have been carried out within this geomorphological province, determining the presence of As in groundwater (INETER, Citation2010; INETER et al., Citation2011; Estrada Guevara, Citation2012; Hagström & Rydstedt, Citation2015; Admiraal et al., Citation2015), as well as some studies that focused on the toxic effects of arsenic on the health of the population. By 2010, As has been detected in high concentrations (> 10 μg L−1; from 0 to 325 µg L−1, mean 19.82 µg L−1, n = 268) in water wells and springs located in the communities living on the slopes of the Telica-Rota-San Jacinto and Momotombo-El Hoyo-Monte Galán volcanic complexes, belonging to the volcanic mountain range Los Maribios. This volcanic chain is composed of recent volcanic and Quaternary sedimentary rocks; and is located in the NW sector of the Nicaragua Depression (Nuevas Esperanzas, Citation2010; OPOMS-Nicaragua & Nuevas Esperanzas, Citation2011; Nuevas Esperanzas, Citation2013; CIRA/UNAN-Managua & Altamirano Espinoza, Citation2017; Genie Zepeda & González Zambrana, Citation2017).
In 2014, a new delimitation of the country’s river basins was carried out under the Pfafstetter methodology (INETER et al., Citation2014), according to which Nicaragua was divided into six water units; five of these water units pour into the Caribbean Sea and one into the Pacific Ocean (). According to the existing arsenic information in the country and available for publication, the six water units have As concentrations in their underground and surface waters.
Figure 4. Left: Arsenic concentrations (in μg L−1) in water samples from Nicaragua (2010-2019). Right: Water units, 42 according to Pfafstetter Methodology.
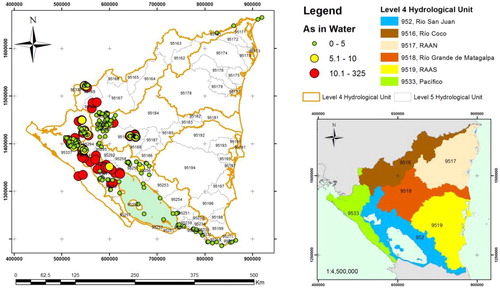
3.4.1.1. Arsenic in groundwater
During the last 10 years, a total 553 sites monitored in the country showed a range of As concentrations from 0.0 to 325 µg L−1 in water, mean: 15.0 µg L−1; of these, 462 correspond to goundwater sources (springs, excavated and drilled wells), in ranges from 0.0 to 325 µg L−1, mean: 16.40 µg L−1 (Delgado Quezada et al., Citation2020 and references therein; Admiraal et al., Citation2015; Estrada Guevara, Citation2012; IAEA & CIRA/UNAN-Managua, Citation2010; CIRA/UNAN-Managua, Citation2011a, Citation2011b; Citation2011c; González Rodríguez & PIENSA-UNI, Citation2016; CIRA/UNAN-Managua et al., Citation2017); and the rest, n = 91, corresponds to surface waters (rivers, lakes, lagoons) in a range of 0.0 to 153.4 µg L−1, mean: 7.89 µg L−1 (Delgado Quezada et al., Citation2020 and references therein; Admiraal et al., Citation2015; Estrada Guevara, Citation2012; IAEA & CIRA/UNAN-Managua, Citation2010; CIRA/UNAN-Managua, Citation2011a; Citation2011b; Citation2011c; González Rodríguez & PIENSA-UNI, Citation2016; CIRA/UNAN-Managua et al., Citation2017). Of this total, 176 sites (31.83%) have reported As concentrations >10 µg L−1, which is the established maximum permissible value for human consumption (WHO, Citation2011); out of which, 156 (33.77%) are groundwater and 20 (21.98%) surface water.
Arsenic distribution, both in surface and groundwater, is mainly observed in the water unit 952 (San Juan de Nicaragua River), which includes: Lake Apanás-Asturias, the tectonic origin lakes Xolotlán and Cocibolca, volcanic lagoons and the San Juan River of Nicaragua. Scatter As distribution follows in water units 9533 (The Pacific), 9518 (Río Grande de Matagalpa), 95197 (between Río Escondido and Punta Gorda, in the RACCS), and 9516 (Río Coco, in the RACCN).
In , it is observed that concentrations of As (10.1–325 µg L−1) are found on the Pacific volcanic mountain range, following Los Maribios 1 and 2 faults (Figure SM1, Supplementary material); therefore, its presence is mostly associated to the Quaternary volcanism. However, As is also present in the Paleozoic-Mesozoic rocks, which corresponds to the oldest formation in the country, wherein As presence is related to epithermal veins. Moreover, As is present not only in soil, sediments and rocks, but also is also associated with the volcanism and ore mineral deposits in the Central Plateau.
The highest concentration of As reported during 2010–2019, was for a dug well used by 15 people that lived or worked in an agricultural farm called El Olocotón, located in the Municipality of Telica (OPS/OMS-Nicaragua & Nuevas Esperanzas, Citation2011). The wells assessed in this study are from six communities located in the 95337-water unit, corresponding to the sub-basin of the Estero Real River on the Pacific watershed. These communities live on the slopes of the Telica Volcano, in Los Maribios volcanic mountain range, which corresponds to the youngest geological formation in the country (volcanic and sedimentary Quaternary). It is likely that the water from these wells is a mixture of the waters from the shallow alluvial aquifer with the waters enriched in As rising from the geothermal reservoir.
3.4.1.2. Arsenic in surface water
The surface water data indicate that As is mainly concentrated within the water unit 952 (Río San Juan de Nicaragua), as follows: starting in the north with <0.99 µg L−1 arsenic in the waters from the artificial lake of Apanás-Asturias (MARENA, Citation2013), through the tributary river system of Río Viejo sub-basin (0.5–23.58 µg L−1 of arsenic; MARENA, Citation2013), which unloads into Lake Xolotlán. Arsenic values in waters from Lake Xolotlán range from 22.57 to 26.56 µg L−1 (CIRA/UNAN-Managua, Citation2016). In the interlakes zone, As values in the Tipitapa River (24.42-25.8 µg L−1; INETER et al., Citation2011) and Tisma Lagoon (26.70 µg L−1; INETER et al., Citation2011) are very similar to those found in Lake Xolotlán; as the river approaches the Lake Cocibolca, As values drop to 17 µg L−1 (INETER et al., Citation2011), to finally reach values of <0.99 µg L−1 in the waters of Lake Cocibolca (CIRA/UNAN-Managua, Citation2016). Finally, along the San Juan River of Nicaragua River and its tributaries, As values are 2.02 µg L−1 (CIRA/UNAN-Managua, Citation2012).
Within the 952-water unit, As is also present in the lagoons of volcanic origin. The available information reports a timely data in the center of the Tiscapa Lagoon (1.59 µg L−1; CIRA/UNAN-Managua & Delgado Quezada, Citation2019a, Citation2019b). In the Masaya Lagoon, at five sites monitored from the surface to a maximum depth of 60 m, As concentrations were below detection limit (ENACAL, Citation2013). In the Apoyo Lagoon, there are previous studies on arsenic in the water of this lagoon and in groundwater adjacent to it; however, in a study conducted in 2010, arsenic concentrations of 153.40 and 192 µg L−1 were determined (CIRA/UNAN-Managua et al., Citation2010).
In the 952-water unit, special attention should be given to: (1) the Apoyo Lagoon system and the groundwater flow dynamics between this water body and Lake Cocibolca, due to evidence of As water transport through decreasing gradients of arsenic concentrations in wells located between these two water bodies (Vásquez-Prada et al., Citation2008); and (2) to water transfer and subsequent transport of pollutants from Lake Xolotlán to Lake Cocibolca, when the water level of Lake Xolotlán exceeds 41 m a.s.l. Therefore, the chemical and microbiological water quality monitoring carried out within the interlakes zone has been a priority for the Government of Nicaragua. According to the Article 97 of the General Water Law (Law # 620; GRUN and ANA (Gobierno de Reconciliación y Unidad Nacional & Autoridad Nacional del Agua), Citation2010), protection, conservation and destination of the waters of the Lake Nicaragua or Cocibolca is regulated, which is the current source of drinking water supply for three municipalities in the country (ENACAL, Citation2008, Citation2011; Tablada, Citation2008), and probably for other municipalities in the near future.
To date, monitoring of As concentrations in rivers is limited. This is because groundwater is the main source of drinking water supply in the country. However, the available information indicates that in 49 rivers that flow into the Caribbean watershed, As concentrations from 0.5 to 25.8 µg L−1 (INETER, Citation2010; CIRA/UNAN-Managua, Citation2011b, Citation2011c, Citation2012, Citation2016; INETER, IAEA, & CIRA/UNAN-Managua, Citation2011; MARENA, Citation2013) have been reported. It is worth mentioning that the highest concentrations were found in the Tipitapa River that flows into Lake Cocibolca. From the data available for rivers that drain to the Pacific watershed, As concentrations range from 0.5 to 5.39 µg L−1 (OPS/OMS Nicaragua & Nuevas Esperanzas, Citation2011; Soza Ferrufino, Citation2020).
3.4.2. Arsenic in soils and sediments
In studies prior to 2010, in addition to its presence in the water, the presence of As in sediments, soils and rocks in communities in the country was also detected (Lacayo Romero et al., Citation1991; CIRA/UNAN-Managua et al., Citation1999; Altamirano Espinoza, Citation2005; Altamirano Espinoza & Bundschuh, Citation2009). During 2009–2019, only one research analyzed arsenic content in rocks, ranging from 4 to 32 mg/kg of total arsenic, mean: 9.68 mg/kg, n = 34, was carried out (Hagström & Rydstedt, Citation2015); and in surface sediments from the 952-water unit; e.g., in Lake Xolotlán (1.16–3.76 g kg−1) and Lake Cocibolca (0.20–1.68 g kg−1) (CIRA/UNAN-Managua, Citation2016), in the San Juan of Nicaragua River (0.36–1.40 g kg−1; CIRA/UNAN-Managua, Citation2012), and in the 95197 water unit, in Bluefields Bay (0.84–2.69 g kg−1; Levy Brooks, Citation2016).
3.4.3. Arsenic in mining areas
As mentioned previously, the arsenic of natural origin is associated with the gold veins from the Cenozoic material, both in mining districts and in other territories of the country. However, information on arsenic concentrations present in mining districts is not available to the public; the only work carried out in the Siuna mining district was in 2004, where arsenic was analyzed in the water and soil, as well as in the nails of adults and infants (Wickre et al., Citation2004). The results from this study, reported arsenic in water from <0.03 to 73.63 µg L−1; in household soil from 6.5 to 1263.4 µg g−1, and in four samples from the mining tailings ranged from 20.7 to 47.8 µg g−1 (Wickre et al., Citation2004). Fingernail samples from 130 individuals (age from 11 months to 85 years) were taken for arsenic analysis, resulting in a range from 0.036 to 5.115 µg g−1 (Wickre et al., Citation2004).
3.5. Costa Rica
The presence of As contamination has been reported in the aqueducts from northern Costa Rica and also in water supplies for human consumption in the Central Valley. Arsenic in water is mainly associated with the volcanic rocks, their weathering products and geothermal fluids. Despite the fact that at several places the reported As concentrations are higher than the national regulatory limit of 10 µg L−1 for drinking water (La Gaceta, Citation2005; Colina Blanco, Citation2015), only a few studies have investigated As in surface and groundwater, or the information is not available to the public. Costa Rica has several active volcanoes, which have their period of activity as indicated in parenthesis: Irazú (1963–1965), Poás (1953–1955, 1989–1994), Turrialba (2010, 2013–2016), Arenal (1975, 1988, 2000), Rincón de la Vieja (1967, 1991, 1995, 1998), that have caused volcanic crises in the past (Abarca & Alvarado, Citation2017), and may be considered as a serious threat for the economy and the health of the inhabitants. These volcanoes released As and other contaminants (e.g., F, B, Li, Hg, Sb, H2S) to the water, which may enter the food chain, producing serious problems to the humans, animals and biota. More information about As concentrations in northern Costa Rica and the Central Valley can be found in the works of Hammarlund and Piñones (Citation2009), Bundschuh, Litter, et al. (Citation2012), López et al. (Citation2012), Morales-Simfors et al. Citation(2020), among others.
3.5.1. Arsenic in water
3.5.1.1 Arsenic in groundwater
In northern Costa Rica, total-As concentrations were analyzed on samples from aqueducts in 59 districts (1600 samples) in the Province of Guanacaste by Mora-Alvarado et al. (Citation2015), in order to analyze the association of As-contaminated drinking water intake and the Standardized Morbidity Index (SMI) for Chronic Kidney Disease (CKD). Total-As data show that among the 421 studied aqueducts, only 17 (4.00%) display high As concentrations in the localities of Bagaces (32.04 µg L−1), Cañas (25.1 µg L−1) and Bebedero (15.13 µg L−1), all exceeding As concentration guideline value for drinking water (10 µg L−1; WHO, Citation2003). In the remaining districts (56) of the Guanacaste Province, As concentrations range from 1.0 to 3.62 µg L−1. Although As contaminated water has been reported in the district of Guanacaste, it is not a common risk factor in the zones affected by the epidemic CKD. Nevertheless, it is necessary to carry out more studies taking into account different common risk factors in the CKD affected districts. Studies carried out by the National Water Laboratory in Costa Rica (LNA) and reported by Colina Blanco (Citation2015) detected high As contents in drinking water sources in the localities of Hotel de Cañas (180 µg L−1), Aguacaliente (62 µg L−1), Vergel (26 µg L−1), Montenegro (20 µg L−1), all in the Province of Guanacaste. High As concentrations have been documented by Hammarlund and Piñones (Citation2009) in geothermal wells (11,900–29,100 µg L−1 and 7800–13,000 µg L−1) in thermal springs (10–4600 µg L−1 and 10–10,900 µg L−1) in the Miravalles and Rincón de la Vieja geothermal areas (northern Costa Rica), respectively. The same authors reported that As concentrations exceed the WHO guideline limit for safe drinking water (10 µg L−1) in 35 of the 50 samples in the studied area.
LNA (Colina Blanco, Citation2015) has detected high (toxic) As concentrations in drinking water supplies in the localities of Caño Negro (186 µg L−1), Gloria de Aguas Zarcas (27 µg L−1) and Concepción in San Carlos (Alajuela Province, northern Costa Rica). The As reported by LNA in San Carlos and Guanacaste is present in the form of As(V) and As(III) and may be of anthropogenic origin.
3.5.1.2. Arsenic in surface water
In the Central Valley of Costa Rica, As concentration has been documented in the City of Cartago by Montero-Campos et al. (Citation2010) in a study about As determination in water supplies (118 spring samples) for human consumption. The studies determined that none of the sampled springs contained detectable amounts of >5 µg L−1 As(III). However, in El Guarco (Cartago Province), an As concentration of about 18 µg L−1 has been detected in a deep well; however, the concentration was below the recommended limit of 10 µg L−1 in a pit at a construction area (Montero-Campos, pers. commun., November 15, 2019).
Hammarlund and Piñones (Citation2009) documented high As concentrations in thermal springs (10-4600 µg L−1 and 10-10,900 µg L−1) and in cold surface waters (<10 µg L−1) in the Miravalles and Rincón de la Vieja geothermal areas (northern Costa Rica), respectively.
3.5.2. Arsenic from other sources
Arsenic was also analyzed in the Poás and Turrialba volcanic fields (Central Valley). In Poás volcanic field (Heredia Province), As concentrations ranged from 210 to 16,000 µg kg−1 for the monitoring period of 1980–2002 (Martínez Cruz, Citation2008). In the same study, the author reported the losses of crops, especially coffee plantations, caused by the period of enhanced acidic deposition in the year 1994 in the vicinity of the volcanoes. Arsenic concentrations of <0.005 µg L−1 have been reported in Turrialba volcanic rocks (Cartago Province) by Piazza et al. (Citation2015). Lücke and Calderón (Citation2016) identified an anomalous high content of As2O5 at 0.491 wt.% (4910 mg kg−1, 1 sample) in the ashes from the 2014 to 2015 volcanic eruption through Scanning Electron Microscope (SEM) and Energy Dispersive X-Ray Spectroscopy (EDX), which may be the result of enrichment by the hydrothermal system or due to the dissolution of the volcanic glass present in the ash and its downward percolation leading to the enrichment of arsenic in the aquifer rocks. Further work on the behavior, mobility of As and other contaminants uptake is necessary for better understanding of their bioavailability/bioaccessibility in surface and groundwater environments.
3.6. Panama
Arsenic distribution in Panama has only been studied marginally or the data have not been officially published, although several monitoring programs for restoring the surface and groundwater systems in the country have been implemented. Data on human exposure to As from drinking water is very limited. Some studies investigating trace metals, including As in rocks, sediments and water have been carried out in the Colón Province (southwest of Panama City), Azuero peninsula (SW Panama), Bahia Damas, Gulf of Chiriqui (west Panama), Bocas de Toro (NW Panama), besides an ongoing study (2019-) on “Trace Elements and Toxicity of Sediments” in the Panama Channel Basin that commenced by the University of Panama and the University of Cartagena, Colombia. Panama is part of “the Golden Belt of the North”, which extends from Central America and includes the entire Panamanian territory (Nelson & Ganoza, Citation2011).
3.6.1. Arsenic in soils and sediments
The increasing industrial growth in the Bay of Panama has raised concern about heavy metals and metalloids pollution and its impact on the marine ecosystem. However, in Bahia Damas, Isla de Coiba in the Gulf of Chiriqui (West Panama), As concentration in sediments (Cat 2 sample) was below the detection limit (0.004 mg kg−1; Araúz, pers. commun., 2019).
Berry et al. (Citation2013) analyzed heavy metals (Cd, Cu, Zn, Hg) and As in sediments and tissues of the scleractinian corals Porites furcata and Agaricia tenuifolia in five reef sites from Bocas de Toro archipelago (NW Panama). Results indicate that As and Cd concentrations were lower than those of Cu and Zn. The highest concentration of each contaminant was measured in P. furcata tissues, indicating that this species has a higher affinity for metal/metalloid accumulation and storage than A. tenifolia. The order of heavy metals and metalloids found in reef sediments was Zn > Cu > As > Cd > Hg. Thus, Cu absorbed to the sediments within the Almirante Bay could be negatively impacting biota; however, the sediments have concentrations within natural environmental background levels for As, Cd, Zn and Hg. The distribution of metal pollution throughout the bay is likely facilitated by the resuspension of dredged sediments, water flow created by the inlet and outlets, discharge from rivers within the bay and the sediment plume origination from the Changuinola River. The waters of Changuinola River are used for agriculture and local livestock breeding (Cornejo et al., Citation2017).
3.6.2. Arsenic in mining areas
There are several gold, silver and copper deposits in the territory that have been exploited since the colonial time. In one of the largest epithermal deposits of gold-bearing Cu-porphyry of Oligocene age in the world, the Petaquilla deposit in Donoso, Colón Province, southeast of Panama City, As concentrations in drill cores (B07061 Botija well) reached values from <2000 to 161,000 µg L−1. The highest values of As (161,000 µg L−1) with 0.15% Cu were sampled between 108.5 to 109.6 m of depth (Flores Rojas, Citation2010). Before the mining activities started, the communities were supplied with water from the rivers (e.g., San Juan, Petaquilla, Caimito); however, nowadays the mining activities have affected the crops and water supplies in the region (Arcia, Citation2018). Studies on the mobilization and release into water sources of As and other contaminants due to mining activities have not been carried out so far in the region. In Cerro Quema, Azuero peninsula (SW Oanma) of Late Campanian to Maastrichtian age, the average of enrichment factors for the oxide and sulfide ores have been reported in drill cores and surface deposits from high-sulfidation epithermal Au-Cu deposits. In the drill samples As varied from 12,000–27,400,000 µg kg−1 in the sulfide ore and 11,000–810,000 µg kg−1 in the oxide ore, while in surface As varied from 15,000–28,000 µg kg−1 in sulfide ores and 15,000–3,700,000 µg kg−1 in oxide ores. The results show that sulfide ores have also the highest concentration of Cu, Zn, As (2.74%) and Cd in the whole-rock data (Corral et al., Citation2018). Most communities in this region have their own water aqueduct and the river valleys (Tonosí, Joaquín and Oria rivers) where the mining concession contains headwaters are used for agricultural purposes on the national level, producing milk, beef, rice, vegetables and fruits (Degray & Miles, Citation2014). Studies of pollution and human exposure to As and other contaminants in the zone due to the accumulation of As and heavy metals from gold tailing dams are also missing.
3.6.3. Arsenic from other sources
Another study assessing contents of trace metals and As in Panama is by Neary Ross (Citation2015), who analyzed Panamanian marine turtles (six sea turtle species) and related implications for human consumption. Turtles and their eggs are an important source of food and income in some countries, including Panama. Median As contents were 0.12 ± 0.04 mg kg−1 and 0.12 ± 0.06 mg/kg−1 for Green and Olive Ridley eggs, respectively, while Hg being the most toxic element in comparison to As and Cd. Elemental contents were in the order Zn > Fe > Se > Cu > Mn > As > Ce > Ni > Mo > Hg > Pb > Co. According to WHO (Citation2010), organic As species, which are abundant in seafood, are less harmful to health compared to inorganic species, and are rapidly eliminated by the body.
4. South America
4.1. Venezuela
In the Bolivarian Republic of Venezuela, the occurrence of As has not been considered as an important issue yet and only few data could be found about As concentrations in the environment, biota, human fluids (blood and urine) and tissues or in food. Total As levels were determined during the year 2006 in sugar cane soils and foliar tissue from sugar cane crops of Palavecino Municipality, Río Turbio Valley, Lara State (VE-01 in ; Yépez et al., Citation2007), for which samples were taken from six farms. The As levels in soil ranged from 5.75 to 12.13 mg kg−1, and those in the foliar tissue from 0.24 to 0.29 mg kg−1. Even though the sampled sites are under risk of As contamination due to the intensive use of As-containing pesticides, the observed As concentration in foliar tissue and soils are significantly lower than those found in risk zones. The As levels were determined in rice grains, from San Cristóbal Municipality, Táchira State, supermarkets, since there is a large concern about its effect on human health. Venezuelan rice falls into an intermediate category of mean As concentrations (0.062–0.089 mg kg−1), approximately half of those reported for rice from the USA and Europe (Zavala & Duxbury, Citation2008). Arsenic data in the period 2010–2020 is shown as follows.
4.1.1. Arsenic in water
4.1.1.1 Arsenic en surface water; Lotic waters
During the period 2010–2012, a time line of physicochemical parameters of Río Turbio water, an important freshwater body of Lara State, was established (VE-01 and VE-02 in ; Adan, Citation2010, Adan et al., Citation2014). The surface river water is used for drinking water supply and irrigation at the high basin; while in medium and low basin, it is used mainly for irrigation. It is important to remark that the Río Turbio houses an important aquifer, used for the drinking water supply of the City of Cabudare (VE-01 in ). The As concentration was determined by total reflection X-ray fluorescence at five sampling points, covering high, medium and low river basin. All measured As concentrations were below the national regulatory drinking water limit (50 µg L−1; Decreto 883, 1995). In the high basin (point Buena Vista) with agricultural exposure and low basin (points Santa Rosa and Guardagallo) with industrial and urban exposure, As levels were less than 2 µg L−1, i.e., below the WHO limit of 10 µg L−1. In the medium basin, with the influence of diffuse contamination sources, viz. waste waters and the action of a high population density (Barquisimeto City has more than 1 million inhabitants), As concentration was 6 ± 1 µg L−1, also below the WHO limit of 10 µg L−1. The highest value was found at the confluence of Río Claro with the Río Turbio (14 ± 3 µg L−1), and it is above the WHO limit of 10 µg L−1, which may be explained in terms of geological differences with the previous sampling sites.
During the years 2012–2014, As concentration was determined in water from Tabure Creek, Palavecino Municipality, Lara State (VE-01 in ) (Ojeda, Citation2012; Poleo et al., Citation2014). This creek feeds the Río Turbio basin containing an important aquifer, and is located in a protected zone, the Terepaima National Park. The selected sample sites were two small dams, the first one labeled Dique Arriba in the upstream area is located inside the wet forest and the second one named Dique Abajo corresponds to the low zone located in a dry forest close to the City of Cabudare, Lara State. The As content was determined directly on water samples, using total reflection X-ray fluorescence. Arsenic concentrations in water from both sites are below the national limit of 50 µg L−1 established for drinking water (Dique Arriba: 6.5–18 µg L−1; Dique Abajo: <1 µg L−1). However, at Dique Arriba sampling point, As levels above the WHO limit of 10 µg L−1 were found. This point is located inside Terepaima National Park, in a rain forest, at the Andean Mountains foothills and was used for coffee and potato production during the twentieth century.
4.1.1.2. Arsenic in water for human consumption and agricultural, agro-industrial, industrial and touristic purposes
The As levels in water (surface, well, ponds, runoff lagoons and bottle) were determined (unpublished data) in the samples of the west-central region in the states of Lara, Portuguesa and Yaracuy (). Arsenic concentrations in water used for agricultural, agro-industrial, industrial and touristic purposes are shown in , whereas shows As concentrations for water used for human consumption (tap and bottle water). All the water samples have As concentrations below the maximum allowed national As level for drinking water (50 µg L−1), with the exception of two sites: Caujaral, Jiménez Municipality, VE-03, Lara State (230 µg L−1 for a lagoon) and from Guanare Municipality, Portuguesa State (VE-05), Agropecuaria Santa Rita. These two sites are devoted to intensive agricultural production. The high As levels from these two sites could be associated with the type of the activity and to the use of As-containing agrochemicals, crop management and irrigation practices, rather than originating from natural sources. Considering the 10 µg L−1 WHO guideline value, all the samples are below this level, with the exception of the afore mentioned and a sample in the northwest of Iribarren Municipality, Pavia, corresponding to waste water (13 ± 3 µg L−1), a drinking water well sample at Las Velas Yaritagua, Peña Municipality, Yaracuy State, VE-07 in (23 ± 1 µg L−1), an agricultural well water sample from Torres Municipality, Lara State, VE-06 (13 ± 3 µg L−1) and an industrial waste water in Cabudare, Palavecino Municipality, Lara State, VE-01 (30 µg L−1).
Table 1. Arsenic levels in water for animal, agricultural touristic and industrial production at the West Center region of Venezuela in the states of Lara, Portuguesa and Yaracuy. Source: Own unpublished data.
Table 2. Levels of Arsenic in human consumption water in municipalities of Lara, Yaracuy and Portuguesa states, Venezuela.
4.1.2. Arsenic in soils, sediments and vegetal tissues
For the evaluation of As removal in landfill soils from the main landfill of Barquisimeto (northwest, VE-02 in ), Iribarren Municipality, Lara State, using earthworms, As was analyzed in five landfill yards (Marcó Parra et al., Citation2010). These levels can be considered to represent the highest expected values due to the nature of the sampled zone, a landfill. The lowest level was found for Prophylaxis yard, at 0–10 cm depth (37.65 mg kg−1). For the Carnichos yards, closed yard, gardens and residential, the As levels were in the range of 58.20–78.58 mg kg−1 at 0–10 cm depth. For the depth of 10–20 cm, the range was 61.20–73.50 mg kg−1.
During 2014–2016, a study on the soils of the Terepaima National Park and its urban surroundings or protection zone was conducted (Hernández et al., Citation2018). The study area was confined to the medium basin of Tabure Creek, at Palavecino Municipality (VE-01 in ). In a plot of 86 ha, a grid of 68 sampling points at two depths (0–10 cm and 10–20 cm) with a distance of 100 m in between them was selected. Over 70% of the soil samples had As concentration below 40 mg kg−1 in the background concentration range of 0.1–40 mg kg−1, 40 mg kg−1 being a threshold level established after a conservative risk analysis, according to the US EPA guidelines (Dudka & Mille, Citation1999). The highest As levels are associated to the points located inside the campus of the Universidad Centroccidental Lisandro Alvarado, in an improvised illegal landfill. Some points with high As levels of up to 306 mg kg−1 are located inside the park, which in the middle of the 20th century was devoted to the coffee cultivation. The numbers and frequency (%) of samples for the different concentration ranges are shown in .
Table 3. Arsenic concentration ranges in soils of Terepaima National Park, Campus and University Park (Palavecino Municipality, Lara State). Source: Own unpublished data.
A study of soil and ornamental vegetation was carried out in a demarcated area of 5 hectares at the Lara Avenue, Barquisimeto City, Iribarren Municipality. This is one of the most important avenues with high traffic and commercial activity, and allows evaluating the associated risk in an urban context, with intervened land. The data constitute a baseline and are shown in . Measured As levels in soil are low (<5 mg kg−1) for most samples. Only one sample has a concentration value higher than the threshold level of 40 mg kg−1, corresponding to an urban garden with intensive use of agrochemicals. Regarding the As levels in foliar tissue, roots and stem of the urban vegetation, the highest values were found in roots for the specie Gliriscidia sepium (5.9 ± 0.3 mg kg−1). The rest of the analyzed species had accumulated As levels lower than 1.2 mg kg−1 in roots and in many cases, levels were lower than 0.5 mg kg−1 (). As expected, As concentrations in roots were higher than those in stems, leaves or fruits and seeds. The results indicate a low risk of As contamination, as the sampled place corresponded to a critical point due to high urban traffic and intense commercial activities.
Table 4. Concentration of arsenic in soil and vegetation at a main avenue, (Lara Avenue) Barquisimeto City, Iribarren Municipality, Lara State. Source: own unpublished data.
4.1.3. Arsenic from other sources
The As concentration was determined in different animal food samples, available at the market in the west-central region of Venezuela, and used by small and large producers of animals and animal products (unpublished data). This allows an evaluation of the risk in the food chain and also for the spread of As by using the animal manure as raw material for organic fertilizers. The results in show that As levels of all samples are lower than the values established by the European Union (EU) for animal feed and minerals (EU, Citation2013), which established levels below 2 mg kg−1 for feed raw materials and 12 mg kg−1 for mineral feed or supplement. Therefore, there is no risk related to As in animal food at present in Venezuela.
Table 5. Arsenic concentration in animal food at the westcentral region of Venezuela. Source: own unpublished data.
The analysis of organic fertilizers allows evaluating the impact of As dispersion. For different sites worldwide, it has been demonstrated that the systematic application of contaminated fertilizers contributes to the increase of trace element levels in soil and water and in consequence enter the food chain (Jayasumana et al., Citation2015; Gong et al., Citation2019). It was reported that manures and organic fertilizers were a major source contributing trace elements to the environment (Gong et al., Citation2019).
In Venezuela, the As concentrations found in organic fertilizers, agrochemicals and raw materials () were lower than those reported from China, other countries as Austria, UK, Canada Switzerland (Gong et al., Citation2019) and Sri Lanka (Jayasumana et al., Citation2015). It has been observed that As levels in the west-central region of Venezuela were lower than the maximum acceptable concentration (10 mg kg−1) in many countries, as reported by Gong et al. (Citation2019). The highest values were found in two samples from the main farm of Jiménez Municipality at Lara State (10 and 12 mg kg−1). These samples corresponded to chicken mortality and egg wastes composts ().
Table 6. Arsenic concentrations in organic fertilizers, agrochemicals and raw materials. Source: own unpublished data.
4.2. Colombia
The geology of Colombia indicates the existence of rocks with As-bearing minerals, especially in the mining areas. However, very few studies exist about As contents in rocks, soils, sediments, and water. The first studies conducted in the country concerning the presence of As relates it with arsenopyrite, mainly associated with precious metal veins in the departments of Antioquia, Caldas, Quindio, Tolima, Cauca, Nariño y Santander (Ingeominas, Citation1987). The following subsections include updated information (after Alonso et al., Citation2014) of the studies reporting the presence and concentrations of As in groundwater, superficial waters, and soil and sediments, at different locations in Colombia. Table SM3 summarizes the information of studies that reported As concentrations in these environmental matrices.
4.2.1. Arsenic in water
Colombia has a great groundwater potential, since approximately 75% of the territory presents favorable geological conditions for aquifers with high yield (Vesga & Peñalosa, Citation2018). The regional areas with the greatest groundwater exploitation and study cases correspond to Guajira, Cundinamarca, Valle del Cauca, Cesar, Norte de Santander, Tolima, Córdoba, Magdalena, Sucre, Atlántico and Boyacá. Although in recent years it has been possible to find several studies on both surface and groundwater quality in Colombia, the data available for As concentrations are still very scarce, especially in groundwater. It is important to stress that in Colombia there are no available reports of As concentration in groundwater used for drinking purposes. The Bogotá savannah, with almost ten million inhabitants, is the most important agro-industrial center in the country, and its water demand is supplied mainly by the Bogotá River systems and the Chingaza mountain range. According to the Colombian Geological Survey, water from the Bogotá River has traditionally been used to irrigate crops of flowers, vegetables, grasses, among others (IDEAM, Citation2010).
4.2.1.1 Arsenic in groundwater
Cacua (Citation2004) reported a preliminary evaluation of the water quality in two wells, located in the metropolitan area of Bucaramanga, at the Santander Department. The concentrations of As were 3.0 µg L−1 and 4.0 µg L−1, respectively. However, although these values were below 10 µg L−1, other physicochemical parameters and Pb concentration indicated that the wells water required treatment for human consumption. Ángel and Fierro (Citation2012) reported As concentrations up to 13 µg L−1 in the upper basin of the Suratá River (Santander), near the Municipality of California, which again is above the As limit specified in Colombian legislation for drinking water. In this study area, gold ores containing arsenopyrite (FeAsS) and byproducts of the mining processes are the main As sources. However, no action has been taken until now to assess the presence and impact of As. In the same area, Alonso and Castillo (Citation2014) demonstrated that there is a very high As contamination downstream from the mining area, with As values of 9.1–419.4 mg kg−1 in sediments and 8.2–52.0 µg L−1 in water, respectively, of the Suratá River.
A detailed study over a period of four years (2011–2014) of the El Carrasco municipal landfill, where both industrial and domestic wastes are dumped, and the most important sanitary landfill in the Department of Santander, located in Bucaramanga, identified that the site does not have a groundwater monitoring network (Niño et al., Citation2016). The effect of leachate from the municipal landfill on the quality of groundwater was evaluated using computer tools (Modflow, a groundwater flow model). Further, it was evaluated the physicochemical and microbiological quality of the water from 10 wells located downstream of the landfill, from which groundwater is taken. The local environmental authorities authorized the use of water from these wells for industrial management in the city (for car wash use). The results showed very high As values in the Bucaramanga deep aquifer, in an area influenced by motor park (4200 µg L−1). This is again above the As limit specified in Colombian legislation for industrial use (100 µg L−1) (MPSDS, Citation2014). This study demonstrated the effect of the mishandling of the El Carrasco landfill, which has been closed recently.
In several municipalities of the Córdoba and Sucre departments, the source for water supply is the groundwater from Morroa aquifer. Hernández and Marrugo (Citation2016) evaluated the concentrations of As and trihalomethanes (THMs) in drinking water and determined the associated risk from its intake among the population of Chinú (Córdoba) and Corozal (Sucre). Samples of drinking and water stored in pools, used for human consumption, were taken at two seasons of the year (dry and rainy season). Mean value of As concentration was 2.26 µg L−1 in the dry season and 2.44 µg L−1 in the rainy season at the Municipality of Chinú, and 1.9 µg L−1 both in both seasons at the Municipality of Corozal. Although As concentrations did not exceed the limit of 10 µg L−1, in both water types, 33% of the samples from Corozal and 83% of those from Chinú presented fecal coliforms.
González-Martínez et al. (Citation2018) studied the exposure of the population to As through drinking water in the municipalities of Margarita and San Fernando (SW Bolivar Department). Eligible participants in this study had been living in these municipalities for at least 5 years and often drinking groundwater. The As concentrations in samples from drinking water wells in the Margarita and San Fernando towns, ranged from 10-96 µg L−1 (mean 34.6 ± 24.7 µg L−1), all exceeding the limit of 10 µg L−1 for drinking water. In addition, the authors evaluated the exposure through intake of this water using the lifetime average daily dose (LADD mg kg−1 day−1) and the hazard quotient (HQ). As reported by González-Martínez et al. (Citation2018), LADD stands for the amount of As to which an individual is exposed daily from one or more sources and is expressed on the basis of mg of As per kg body weight per day, while the HQ is obtained by dividing the LADD by the US EPA’s reference dose (RfD) of As (0.3 mg kg−1 day−1), for non-cancer outcomes such as hyperpigmentation, keratosis and possible vascular complications (US EPA, Citation2001). Thus, a HQ above 1 is considered a risk for a potential adverse health effect, whereas an HQ below 1 is not considered a risk. LADD was 0.31 mg kg−1 weight day−1 and the HQ was above 1. Therefore, these results show that the study population have a chronically exposure to As by drinking groundwater and a potential risk to its health.
4.2.1.2. Arsenic in surface water
The Bogotá River basin waters are and have been the object of study by different researchers and public and private entities over the past 60 years, who revealed that they are contaminated with different types of organic and inorganic substances, within which have been identified potentially toxic elements. Vargas et al. (Citation2004) analyzed As, Cd, Pb, Zn, Mo, Mn, Cr, Co, Cu and Ni in soils of the Bogotá River basin, from its source in the Guacheneque moor, Municipality of Villapinzón, to its mouth in the Magdalena River, at the City of Girardot (Cundinamarca Department). The total area of the basin is approximately 6000 km2. The elemental distribution in the basin’s soils differentiates two zones: (i) Lower River Basin, and (ii) Upper River Basin, from its source to the Muña reservoir in Sibaté, which has lower concentrations for almost all elements compared to the Lower Basin. The pH in the lower basin is mostly basic (6.6–9.0), while in the upper basin it is acidic to about neutral (4.0–6.5). It is worth noting that Cd is enriched in a greater number of locations in the upper basin, while the highest concentrations of As (30 mg kg−1) were found only in the lower basin (municipalities of Anapoima and Cachipay). The presence of As in these soils, in addition to the geographical contribution, can be related to agricultural activities that take place in these areas (use of fertilizers, insecticides, fungicides, and livestock waste). These soils should be studied in more detail to determine the amplitude of As presence, since they are located in one of the most important agricultural areas of the country.
In another study, Vargas et al. (Citation2001) analyzed data of metals and metalloids present in surface water from 16 points located in the Bogotá River basin. The data reported correspond to 67 samples taken mainly at the La Turbia stream (Sesquilé Municipality), Bahamón River (Cachipay Municipality), La Carbonera stream (La Mesa Municipality), La Honda stream (Tena Municipality), Socha stream (La Calera Municipality) and Tunjuelito River (Usme Municipality). The As concentrations in the Bogotá River basin were on mean 0.43 ± 0.57 µg L−1, a lower concentration than the WHO (Citation2011) guideline for As in drinking water (10 µg L−1). Similar results were reported by Espinosa and Güiza (Citation2005), with As concentrations of 0.03–2.73 µg L−1 in surface water of the upper Bogotá River basin.
4.2.2. Arsenic in soils and sediments
In Colombia, most of the mineralogical, geochemical and hydrogeological studies of soils and sediments have been the result of investigations by the Colombian Geological Survey (Servicio Geológico Colombiano - SGC), which has also produced site maps for those areas where studies have been conducted. This information is available online (http://www.sgc.gov.co). Among its main reports are the geochemical studies on the country’s soils and sediments, viz. the Geochemical Atlas of Colombia, Ultra Low Density Map and the Map of Geochemical Abnormalities of Colombia (Vargas et al., Citation2004; Prieto & García, Citation2005; Ingeominas, Citation2009). González et al. (Citation2005) reported As levels of 1.0–4.0 mg kg−1 in unconsolidated recent sediments of rivers and creeks that make up the Atrato River basin in the southwest zone of the Antioquia Department, mainly in the municipalities of Urrao and Vigía del Fuerte. The presence of arsenopyrite has been reported at the El Diamante gold mine, in the Nariño Department (Bustos Rodriguez et al., Citation2007), and in the Marmato mining district, at the Caldas Department (Prieto, Citation1998). Bundschuh, Litter, et al. (Citation2012) reported high As concentration in Marmato River water samples, at the Marmato mining district. The river sediments show seasonal variation of As concentrations (dry season 10–850 mg kg−1; wet season 440–1400 mg kg−1). Acid mine drainage has been suggested as one of the main sources of surface water contamination by As in the area (Alonso et al., Citation2014).
A recently published atlas covering 60% of Colombia’s territory reports that the As content in sediments range between 0.5 and 21.1 mg kg−1 (SGC, Citation2016). However, higher As concentrations, between 21.1 and 700 mg kg−1, were found in some sites located mainly in the western area of the Bogotá savannah. This is one of the main agricultural regions of the country, where for many years the Bogotá River is the main source of irrigation water. The other zones identified on the map are located in the Municipality of Marmato (Caldas Department) and in the north of the country, in the departments of Sucre and Bolivar.
These areas have been widely studied, mainly due to the presence of Hg, which is widespread due to illegal mining. In 2009 and 2010, the same entity performed a geochemical evaluation for a medical geology assessment in the Momposina Depression at the Mompos, Magangué and San Marcos sectors, covering an area of 1000 km2 in the north of the country. García et al. (Citation2010) presented the results of the floodplains sediments geochemical sampling in the region for the superficial and deep horizons. The regional sampling of ULD (Ultra Low Density) showed anomalous high concentrations of elements, such as As (13–35 mg kg−1), in almost all stations, which are in the range found in natural background values but exceed the mean value. The As background content in soil typically ranges from 1 to 40 mg/kg, with a mean of 5 mg/kg (CCME, Citation2002; Redon et al., Citation2013). The Momposina Depression is the confluence and deposition zone of the rivers Magdalena, Cauca and San Jorge.
A comprehensive review, from the 1970s to 2014, identified the presence of As in different matrices (soils, sediments and water) across Colombia (Alonso et al., Citation2014). It is inferred from these studies that the occurrence of As in water resources could be attributed to human activities, mainly mining (geogenic As sources). In recent years, the growth of mining industries in the country, mainly of gold and coal, together with the fact that there is not a consistent framework that incorporates a risk-based approach to remediation of contaminated sites, evidenced by Arias et al. (Citation2018) and other studies carried out in several areas of the country, confirm the presence of toxic elements, especially Hg, as well as Pb and Cd, in areas affected by mining activities. However, studies on As in groundwater and other water sources are still incipient. Although there are entities that regulate water quality in Colombia, most of the surface water that is supplied for agricultural activities is of precarious quality. This, added to phenomena such as “El Niño”, causes episodes such as one reported recently in the country where 48 municipalities were declared in public calamity due to water shortages (Semana, Citation2019).
A study was carried out to analyze the presence of As, Cd, Pb, Cu and Hg in fresh milk, soil, superficial waters and grass from animal farms in the towns of San Pedro and Entrerríos, two of the main livestock sectors of the Antioquia Department, where the soil is fertilized with wastewater, by-products of recycled water treatment and sludge from the City of Medellín, collected from the San Fernando plant (Londoño, Citation2014). Samples (140) were analyzed for two seasons from February to March 2010 (summer season) and in December 2010 (winter season). Arsenic values found in grass, soil and water samples were 0.301, 0.508 and 0.111 mg kg−1, respectively. According to the results, the authors affirm that the most dangerous toxic elements, taking into account their concentrations, were As and Cu due to a continuous irrigation of farms for several years with wastewater and fertilization with wastewater biosolids. These could create risks of translocation to the food chain and thus affect animal health, and possibly human health, due to heavy metal and metalloid contamination.
The existing coal reserves of Colombia are very large compared to those of other countries in Latin America (BP, Citation2019). Therefore, it is vital to monitor that the exploitation of this resource is done in an environment friendly way. Mahecha et al. (Citation2016) performed a quantification analysis of As and Hg in coal, soil, leaf tissue and water sources samples from sites nearby the coal mining areas of Guachetá (Cundinamarca Department). In coal samples, As levels were 0.19–2.90 mg kg−1, while Hg levels were 0.09–0.30 mg kg−1, which did not exceed the permissible limits. In soil samples, As levels were 1.98–7.19 mg kg−1. It is important to note that the higher values were observed in soils from a crop located approximately 1 km from the coal mines. In addition, very acidic pH values were measured, which was associated with high Al saturation, low percentage of organic matter and low edaphic levels. In the soils closest to the mine, As was lower (1.98 mg kg−1). Authors say this could be explained due to the direction of air currents in the area that take As not directly around the mine but to distant soils of the coal mines, where it is found in higher concentration. In the samples taken from water sources none of the elements were detected.
A recent study (Alonso et al., Citation2020) was carried out to evaluate the effect of the Vetas-California gold mining district on As distribution in stream sediments and surface water samples from Suratá River, located in the southwestern area of Santurbán paramo. The results highlighted a high As content (reaching up to 484 mg kg–1) in stream sediment sampled near the gold mining district. For both sediment and water, the relative As content along the Suratá River exceeded the background content by 220 and 64.9 times, respectively.
4.3. Ecuador
Reported studies on As in Ecuador have related its presence in the environment to geothermal sources and mining activities. Cumbal et al. (Citation2010) measured 2–969 µg L−1 As in water of hot springs, and 1.6–717.6 mg kg−1 in sediments sampled in the Andean geothermal areas of north-central Ecuador. In another study, in the Papallacta Lake, As concentrations up to 369 µg L−1 at the surface and 289–351 µg L−1 at depth were measured. These high values are caused by the input of geothermal waters with As contents up to 7852 µg L−1 into the lake (Cumbal et al., Citation2009; Bundschuh, Litter, et al., Citation2012).
4.3.1. Arsenic in water
4.3.1.1. Arsenic in groundwater
A recent review by Morales-Simfors et al. (Citation2020) reported As concentrations, physicochemical parameters and major ions in Inbabura Province, Pichincha geothermal province, and Napo, Cotopaxi, Tungurahua, Chimborazo and Santa Elena provinces. Overall, As concentrations varied from 1 to 5712 µg L−1 with the highest concentrations in the geothermal springs close to the Tambo River (up to 5712 µg L−1), Nono spring (3950 µg L−1), Papallacta thermal springs (up to 3203 µg L−1), and Chachimbiro spring (1250 µg L−1). Mature waters (Na-Cl type) presented the highest As concentrations due to water-rock interaction in the geothermal reservoir during a long time.
4.3.1.2. Arsenic in surface water
The water quality of the Yacuambi River (SW Ecuador) was investigated to evaluate associated risk to the population and ecosystems of the tropical Andean area that is considered to be among the most biodiverse in the world. Measurement of pH, electrical conductivity, dissolved oxygen, temperature, color, phosphates, nitrite, nitrate, biochemical oxygen demand, chemical oxygen demand, total solids, turbidity, metals and metalloids (Ba, Cd, Cr, Pb, As and Hg), pesticides and fecal coliforms were done for the samples collected at six sites along the river during four sampling campaigns. According to the water quality index (WQI), the river water is of good to medium quality. However, arsenic, color and fecal coliforms exceeded the limits for human consumption in all the samples. Lead threshold of preservation of aquatic life was exceeded in all samples and As, pH, nitrite and nitrate in some samples. In addition, due to As concentrations and presence of fecal coliforms, water is not adequate for irrigation (Villa-Achupallas et al., Citation2018). Another study was carried out for the rivers located in the artisanal gold mining areas of Nambija, Portovelo-Zaruma, and Ponce Enriquez in southern Ecuador (Carling et al., Citation2013). Dissolved and particulate trace elements (Co, Cr, Hg, Mn, Ni, Ti, U, V, As, Cd, Pb, Se, Sb and Zn) showed distinct concentration predominance in different areas, reflecting the local geology and mining processes. The highest contents of As, Cd, Pb and Se were measured at Calera. Dissolved concentrations of As (up to about 60 µg L−1) exceeded WHO guidelines for drinking water in most of the mining area. Arsenic drinking water guideline was exceeded at Quebrada Calixto. Another study by Knee and Encalada (Citation2014) investigated the water quality of five river systems and 15 community water supply systems in the Intag forest region, located in northwestern Ecuador. Measured parameters included turbidity, temperature, dissolved oxygen, pH, fecal indicator bacteria (FIB), nitrate, phosphate, ammonium, Ni, Mn, Cu, Zn, Cd, Pb, As, Cr, discharge and aquatic invertebrate diversity. This area is important for its high biodiversity, and large deposits of copper and other minerals. High As concentrations were found to be related to hot springs, abandoned mining boreholes and wastewater discharges, while the lowest contamination was found in the streams located in the forest area.
4.3.3. Arsenic in mining areas
The influence of gold and silver mining on As, Hg, Pb, and Mn contamination in the Puyango River basin, located in southwestern Ecuador, was studied by García et al. (Citation2012). About 110 ore processing plants are located on the banks of the Calera and Amarillo rivers, which are two of the tributaries of the Puyango River. The study determined the seasonal variation of physicochemical parameters and heavy metal and metalloid concentrations in surface waters, sediments and particulate matter. Low As concentrations (up to 0.63 µg L−1) were measured in surface waters within the pristine zone, while As reached 25 µg L−1 in the dry season at the mining zone. On the other hand, As concentration was 161 mg kg−1 in sediments at one pristine point due to the presence of arsenopyrite, but reached 3260 mg kg−1 in the mining area. Besides, up to 153 µg L−1 As were measured in particulate matter in the dry season. Results from this study led to the use of filters to retain the particulate matter in the affected communities, and later to the installation of drinking water plants.
Marshall et al. (Citation2018) reported a study to evaluate the mobility of Hg, Cd, Cu, Pb and Zn in the Puyango-Tumbes River in relation to gold processing plants. This river flows from Ecuador to Peru, and is part of the border between those countries. In Portovelo, located in southern Ecuador, there are 87 gold processing centers along the Puyango-Tumbes River that process poly-metallic ores using a combination of mercury amalgamation and/or cyanidation. Currently, both the countries are taking actions to decontaminate the river (Marshall et al., Citation2018). The reported study determined total Hg, total As, Cd, Cu, Pb and Zn in filtered water and sediment samples along the river from 2012 to 2014. Mercury isotopes were also determined in tailings and sediment samples. High concentration of the analyzed elements, above the CCME (Canadian Council of Ministers of the Environment) thresholds for the protection of aquatic life (As = 5 µg L−1, Cd = 0.09 µg L−1, Pb = 2.5 µg L−1, Cu = 3.5 µg L−1, Zn = 30 µg L−1, Hg = 0.026 µg L−1 in water; As = 17 mg kg−1, Cd = 4.2 mg kg−1, Pb = 112 mg kg−1, Cu = 108 mg kg−1, Zn = 271 mg kg−1, Hg = 0.486 mg kg−1 in sediments) was reported in the water and sediments downstream from the processing plants. Arsenic reached the concentrations 3000 times over the LTE (Long Term Effect) value of 5 µg L−1, and 740 times over the PEL (Probable Effect Level) value of 17 mg kg−1. Arsenopyrite is the main source of As in the tailings.
4.3.4. Arsenic from other sources
Since Ecuador is one of the main consumers of rice in South America, various studies, listed below, have been performed to evaluate the influence of As on health through the consumption of rice. Otero et al. (Citation2016) determined As concentrations in water (36 flood water samples from the rice fields, 16 from irrigation channels, 12 from wells, 9 from rivers close to the rice fields), soil, rice plants and commercial sold rice cultivated in Ecuador from local markets in the provinces of Guayas and Los Ríos that produce more than 93% of the rice in the country. Physico-chemical parameters and dissolved As were determined in water samples. Total and bioavailable As, total organic C, texture, total Fe and amorphous Fe oxyhydroxides (FeOx) were analyzed in soil samples. Total, inorganic (∑As(III), As(V)), and organic As (∑DMA, MMA) were determined in plant parts (dehusked rice grain, stem, leaves, and husk). Measured As concentrations in water were mostly below 10 µg L−1, and were also low in soil (4.48 ± 3 mg kg−1). Total As in rice grains ranged from 0.042 to 0.125 mg kg−1 d.w. (dry weight) and was significantly lower than in leaves (up to 0.286 mg kg−1 d.w.) and stems (up to 0.201 mg kg−1 d.w.). Concentrations of FeOx are negatively correlated with total As in plants, while As in stems and leaves were linearly correlated with total As in the soil and flood water. Arsenic was mostly in the inorganic form in the plants (>80% of total As). Concentrations of As in flood water and soil were highly correlated with total As in rice grain (a mean of 0.125 ± 0.044 mg kg−1 total As) fitting a logarithmic model. This concentration in rice grain is below, but not far from that established As concentration (0.2 mg kg−1) by the Codex Alimentarius Commission (Citation2014).
Another study focused on estimating the health risks in the Ecuadorian population due to the ingestion of As in rice. The rice samples were obtained from paddies in the provinces of Guayas and Los Ríos, and from national supermarkets in the cities of Quito, Daule, Milagos and Babahoyo. The study showed that the Estimated Daily Intake (EDI) of As for infants living in urban areas of Ecuador is around four times higher than European children, but lower than that reported for Brazil, Bangladesh and India. A 3 per 10,000 excess lifetime risk was estimated for adults, but 10 per 10,000 in rural and 20 per 10,000 in urban areas for children. The relative weight of rice with respect to total exposure considering drinking water was estimated from the correlation between EDI and concentration of As in hair. Results showed that rice is a more important exposure source with respect to tap water (Nunes & Otero, Citation2017).
4.4. Peru
Arsenic has been detected in ground- and surface waters at various Peruvian locations, mainly related to water-rock interactions and mining operations. However, purely anthropogenic sources may also be contaminating surficial waters. In a previous review, Bundschuh, Litter, et al. (Citation2012) reported high As concentrations in the Locumba River and Puente de Santa Rosa up to 1680 µg L−1 and 1630 µg L−1, respectively. Arsenic, mainly As(V), was also identified in the area of Yucamane Volcano, and in the Rímac River basin, eastern Lima City. Since then, diverse studies have been carried out to determine As contents and possible sources at other sites. In addition, As concentrations above WHO and national standards (both 10 µg L−1) in drinking water have led authorities to take emergency actions in some towns.
4.4.1. Arsenic in water
In 2012, a study was carried out to determine the As concentrations in groundwater and surface water bodies used as drinking water sources in areas with historical or current mining activities. That study also aimed at evaluating the accuracy of the Arsenic Econo Quick (EQ) field testing kit for measuring As concentrations (George et al., Citation2014). Samples were collected from 151 sources and analyzed by ICP-MS, and by EQ kit in the field. Measured As concentration was above WHO recommended limit in 85%, and above 50 µg L−1 in 56% of the 111 groundwater samples, and above the WHO limit in 50% of the 40 surface water samples. The highest concentrations were found in wells at Juliaca (1.2–193.1 µg L−1), and Caracoto (31.9–113.1 µg L−1). All of the well samples at Caracoto, and 95% of those at Juliaca exceeded the WHO’s recommended limit. However, water from all these wells is used as drinking water by the families unaware of high As concentrations. Samples collected from the section of the Rímac River running through Lima have As levels between 14.6 and 42.5 µg L−1. High As concentrations may be related to mine tailings, a smelter, a refinery, a legacy mine located close to the river, and to other manufacturing wastes from Lima City. The study showed that the EQ kit could be used for a rapid identification of As contamination in drinking water. The authors called attention to the As problem in Peru and the need for an immediate solution to avoid As exposure.
4.4.1.1. Arsenic in groundwater
de Meyer et al. (Citation2017) evaluated As presence in groundwater from Western Amazonia in the Iquitos and Pucallpa regions. Two types of aquifers vulnerable to geogenic contaminants such as As were identified. The first type, Aquifer Type 1, is hosted in modern river deposits of “whitewaters” rivers. In these aquifers, As and Mn are mobilized by reductive dissolution. Values of pH are near-neutral, concentrations of As and Mn reach up to 700 µg L−1 and 4 mg L−1, respectively. The second type, Aquifer Type 3, is an unconfined pure sand aquifer enriched in Al, as a consequence of acidic conditions. The acidic, high Al conditions occur in pure sand formations at catchments of other “blackwater” rivers in the Amazon basin, with pH ranging from 4.2 to 5.5 and Al concentrations up to 3.3 mg L−1. Groundwater from other deeper or older aquifers (Aquifers Types 2 and 4) is generally without geogenic contaminants, e.g., in the Ucayali Formation; however, some of them have As >10 µg L−1 and Mn > 200 µg L−1, requiring careful monitoring. In general, groundwater from older and deeper aquifers is of good quality.
Problems related to As contamination of drinking water have increased at specific sites. In September 2016, As concentrations above national drinking water standards of 10 µg L−1 were identified in a well of the Mórrope Municipality, northwestern Peru. Here, water extraction from an older well was proposed as an alternative to provide safe water to the population; however, As content (30 µg L−1) in this well water was also above drinking water standards. Arsenic was identified in other wells, some of which also contain Pb (0.016–0.044 mg L−1) above WHO drinking water standards (10 µg L−1). Therefore, an emergency disaster supreme decree, based on water contamination, was approved on May 2, 2018 in some localities of Mórrope. This plan included water distribution by cistern vehicles to the affected population and medical attention to their As exposure related health issues. A project aiming at providing a permanent solution to this problem recommended supply of good quality water through gravity transport and drilling of deep wells, as water with safe levels of heavy metals and metalloids was found below 150 m depth. Community education and actions to increase awareness on As related health risk were also carried out in the affected towns focused on the general public, teachers, authorities and medical professionals (Uturunco & Paredes, Citation2019). Arsenic concentrations (up to 100 µg L−1) ten times the drinking water standard, were reported in wells at 14 localities of Mórrope district by Ingemmet (Geologic, Mining and Metallurgic Institute) in October 2018. A permanent monitoring, temporal or permanent closure of contaminated wells, drilling of a new well to gain insight on the aquifer characteristics and further studies to determine the origin and evolution of As were recommended by the specialist of Ingemmet, Danitza Machaca (Andina, Citation2018). To solve the As problem and provide good quality water to Peruvian population, the government announced on October 2, 2018, the construction of the first As treatment plant in Jauja Province. This plant will benefit more than 30,000 inhabitants living in the Jauja, Yauyos and Sausa districts (Peru, Citation2018). The start of operations of this plant in June 2019 was announced in May 2019 (Andina, Citation2019). However, no information could be found about the current operation of this plant in June 2019. On the other hand, on October 28, 2019, the communication office of the Peruvian government informed the installation of filter kits for As removal in 2036 houses in Mórrope district, Lambayeque region (Andina, Citation2019).
A study carried out in 2017 showed urinary As concentrations in the dwellers of the Cairani and Camilaca districts in Tacna region above the toxicity reference values established by the World Health Organization (Ale-Mauricio et al., Citation2018). Arsenic presence in drinking water above the WHO guide and up to 680 µg L−1 has been ascribed to a geogenic source, namely volcanic soils in the Tacna region. A median of 601.61 mg kg−1 creatinine was determined in urine samples from 103 dwellers in the Cairani district. Notwithstanding As concentrations below WHO guide in drinking water, a median of 30.24 mg kg−1 creatinine was found in 71 inhabitants in Camilaca district. Another study (Villa-Gonzales et al., Citation2018) aimed to evaluate the performance of a household filter, found 510 µg L−1 of As in water of Sama River, located also in the Tacna region.
4.4.1.2. Arsenic in surface water
Morales Cabrera et al. (Citation2018) studied the environmental risk of As and B in the Samba and Locumba basins in Tacna. Two sampling campaigns of drinking water reservoirs were carried out in August and November, 2016, and April and June, 2017. Mean As concentrations in the respective months were 73, 298, 287 and 711 µg L−1, surpassing WHO guidelines and drinking water standards of Peru. Boron concentrations reached also high values with 8.681 mg L−1 in August and 4.148 mg L−1 in November 2016. The studies concluded that the superficial waters were not suitable for water supply and present an environmental and human health risk.
4.4.2. Arsenic in mining areas
Reuer et al. (Citation2012) carried out a reconnaissance study to evaluate the impact of a nonferrous smelting complex at La Oroya, Peru on children´s health, for which Pb, As and Cd in drinking water, indoor dust and soil were measured at five sites at distances from 1 to 26 km from the complex. Median As concentrations reached up to 1658 mg kg−1 in soil, up to 1071 mg kg−1 in indoor dust and up to 11.38 µg L−1 in drinking water. Median concentrations of the analyzed elements in soils decreased exponentially with distance from the smelter. Arsenic concentrations in indoor dust also showed a general decreasing trend with distance. Arsenic in drinking water reached the highest concentrations (11.4 µg L−1) in Yauli, followed by La Oroya Antigua (8.5 µg L−1). Exposure to heavy metals and metalloids was estimated using the Integrated Exposure Uptake Biokinetic Model for Lead in Children dose-response model. Results suggested significant As and Pb contributions from soil and indoor dust, and recommend the need of remediation (Reuer et al., Citation2012).
4.4.3. Arsenic from other sources
Tapia, Murray, et al. (Citation2019) conducted a comprehensive study of As distribution, origin and geochemistry of As in the Altiplano-Puna plateau, which includes areas of Argentina, Chile, Bolivia and Peru. The plateau is located within the Central Volcanic Zone of the Andes. While most of the inhabitants of this area correspond to Bolivia, 1,194,495 live in Peru (INEI Perú, Citation2017). Among the natural sources, the highest concentrations of As in solids were related to mineral deposits, saline precipitates and volcanic rocks. Besides, the highest As concentrations in solution were measured in brines, saline water, and hot springs. Mining activities also contribute to As contamination through the exposure of sulfide minerals and acid mine drainage. Reported mean concentrations of As in water bodies within the Altiplano-Puna included in this study were 200 µg L−1 in groundwater, 1900 µg L−1 in hot springs, 600 µg L−1 in rivers and lakes, 2800 µg L−1 in rivers affected by acid mine drainage, and 100 µg L−1 in tap water. The authors emphasize on the need of more studies in this geographic area to identify the mineral phase and physicochemical mechanisms involved in the release of As to surficial and groundwater from volcanic rocks, the effect of the dry and wet seasons on As mobility in the plateau, the number of people at risk and specific methods to remove As from water.
The presence of As in Peruvian surface and groundwater has recently been recognized as a potential threat to bioaccumulation of As in the food-chain especially rice. Recent studies by Mondal et al. (Citation2020) analyzed the levels of As in rice from the Tumbes River basin in northern Peru. The average concentrations of As was found to be 8.6 ± 7.8 mg kg−1 based on the analyses of 30 samples of soils from agricultural fields. Arsenic concentration in rice collected from 29 locations in the Tumbes basin was found to range in between 68 and 345 mg kg−1 with an average of 168 ± 71 mg kg−1.
Another research focused on the evaluation of human adaptation to high As environmental concentrations in inhabitants of the Argentinian Andes, Peru and Colombia (Schlebusch et al., Citation2015). Arsenic concentrations in urine showed that inhabitants of the northern Argentinean Andes have an efficient methylation and excretion of dimethylated arsenic and less excretion of monomethylated metabolite. Genotype studies suggested that AS3MT locus has been the target of selection for adaptation to an As-rich environment of the Argentinian population that has been possibly exposed to As for 7000 years or more. Besides, Mark (Citation2017) investigated the likely causes of melanoma in Peruvian mummies buried more than 2400 years ago, and mentioned As exposure as a probable cause. On the other hand, the studied Peruvian population living at Lima City, which are exposed to much less environmental As, does not present a genetic differentiation to tolerate As.
4.5. Bolivia
The Bolivian Highlands and its limiting mountain chains to the west and east, known as Western and Eastern Cordillera, contain different geogenic As sources, comprising volcanic rocks, geothermal fluids and volcanic emissions (gases, ash), which impact its different environments with As, including ground- and surface water, sediments, soils and atmosphere, consequently affecting the human food chain and human health. Release of As into these environments can locally be accelerated through present and past mining and ore processing activities, most of them located in the Eastern Cordillera in the departments of La Paz, Oruro and Potosí. Arsenic research has been concentrated in (i) the Pilcomayo River basin () and (ii) the endorheic Titicaca-Desaguadero-Poopó-Salt pans (TDPS) basin, the Poopó Lake basin in particular ().
4.5.1. Arsenic in water
4.5.1.1 Arsenic in groundwater
In recent research after 2010, two generalized trends for As have been identified in groundwater: (a) high concentrations in arid zone in the range from 100 to 250 µg L−1 in southern and northwestern regions, and (b) concentrations below 50 µg L−1 (the national regulatory As limit for drinking water) in the remaining part of the watershed. The Eh values in groundwater ranged from 141 to 218 mV (mean 179 mV), indicating moderately reducing conditions. There was no analytical speciation of As, but based on the Eh-pH diagram and pH values in the range from 6.4 to 7.9, it was interpreted that As was generally present as arsenate species H2AsO4 −. In surface water, predominant species was HAsO4 2 −, and concentrations were in the range from 5.6 to 32.4 µg L−1. Potential sources and processes leading to the enrichment of As and trace metals were oxidation and weathering of As-rich pyrite and arsenopyrite in mineralized areas and desorption from hydrous ferric oxides (HFO). Volcanic ash was suggested as main As source in non-mining areas.
Geogenic As was studied in the area south of the Poopó Lake (Ormachea Muñoz et al., Citation2013). This is a semiarid area with predominantly volcanic rocks. Groundwater and surface water samples were collected at 24 sites in the dry and rainy seasons. Groundwater was of Na-Cl-HCO3 or Ca-Na-HCO3 type with neutral or slightly alkaline pH and oxidizing redox status. Concentrations of As were from detection limit of 5.2 to 207 µg L−1 (mean 72 µg L−1), i.e., well above the WHO guideline value. Highest As concentrations were found in lowlands close to the lake, suggesting relatively long residence time of groundwater. Values of Eh were moderately reducing, in the range from 135 to 278 mV (mean 175 mV) and pH values from 6.57 to 7.94 (mean 7.57). Analytical speciation using disposable cartridges indicated that most As is present as arsenate As(V). Results of geochemical modeling identified as principal species of arsenate and H3AsO3 as principal species of arsenite present only in minor amounts. Also reported were high B concentrations (mean 1902 µg L−1), combined with high salinity, rendering the groundwater unsuitable for drinking. Sequential extraction of sediments together with calculation of saturation indices for water indicated presence of ferric oxyhydroxides, which are important adsorbents of As. High pH values and potential competition with HCO3 for adsorption sites can be responsible for As release and mobility.
Arsenic and trace metals were also studied at mining regions located in the Antequera and Poopó sub-basins (Ramos Ramos et al., Citation2014). Samples were collected during the rainy season and As, Cd, and Mn exceeded the WHO drinking water guidelines in more than 50% of samples. In groundwater, As concentrations were from below detection limit of 5.6 to 364 µg L−1 and in surface water concentrations were from below detection limit to 30,749 µg L−1, the last one in sample of leakage from mine tailings. Factor analysis was applied to identify principal processes responsible for water chemistry. Factors based on groundwater data included plagioclase, gypsum, halite dissolution, sulfide oxidation, release of As and other trace elements under acid pH conditions. Factors based on surface water data included mobilization of trace metals at low pH, dissolution of evaporate salts, neutralization of acid mine drainage and As release due to dissolution of Fe and Mn oxyhydroxides.
Arsenic and other trace elements in hot and cold-water springs east to the Poopó Lake basin were investigated by Ormachea Muñoz et al. (Citation2015). Values of pH of thermal springs were from 6.3 to 8.3 (mean 7.0), and values of Eh were from +106 to +204 mV (mean 172 mV). Principal ions were Na+ and Cl− and predominant water types were Na-Cl and Na-Cl-HCO3. Arsenic ranged from 7.8 to 65.3 µg L−1. Arsenic was speciated using disposable cartridges). As(III) predominated in 8 samples, As(V) in 3 samples and in 5 samples there were similar concentrations of As(III) and As(V). Values of Eh calculated on the basis of the As(III)/As(V) pair were much lower compared to field measured Eh values, indicating strong redox disequilibrium. The disequilibrium was interpreted as a consequence of mixing of deep reduced water with shallow cold water and slow adjustment of As(III) to new redox conditions. Sediments sampled at hot spring outlets had high contents of ferric oxyhydroxides, suggesting As attenuation by adsorption and co-precipitation. Surprisingly, As concentrations in cold springs were higher than in thermal springs and ranging from 5.6 to 233.2 µg L−1. It was concluded that thermal waters are not the main As source in shallow aquifers, which probably contain only a small fraction (or none) of water from deep geothermal reservoirs and their high temperature results from steam heating or conduction.
The geochemistry of As in groundwater and river water in the region south of the Poopó Lake was investigated by Ormachea Muñoz et al. (Citation2016). Surface waters were alkaline with pH 8.2–8.7 and oxidized with dissolved oxygen (DO) values from 2.5 to 6.6 mg L−1. The predominant water type was Na-Cl-HCO3 and As concentrations ranged from 8.6 to 117 µg L−1 with As(V) as predominant oxidation state. Also reported were high concentrations of Li from 1.1 to 4.4 mg L−1, but concentrations of other trace elements were low. In water wells, Eh ranged from 70 to 461 mV (mean 294 mV), in piezometers from 18 to 376 mV (mean 277 mV) and in surface water from 4.3 to 284.4 mV (mean 155.8 mV). Concentrations of As in water wells were from 3.3 to 433.4 µg L−1 (mean 99.2 µg L−1) in piezometers from 12.7 to 3497 µg L−1 (the highest As concentration found in the Bolivian Altiplano) with mean value of 404.8 µg L−1. In surface water, As concentrations were from 8.6 to 117.4 µg L−1 (mean 73.6 µg L−1). About 90% of investigated wells exceeded the WHO limit of 10 µg L−1 for As. There was no analytical As speciation in this study, but As(V) and is expected to be dominant on the basis of Eh and pH values. The Li concentrations ranged from 0.05 to 31.6 mg L−1. High salinity as a consequence of intense evaporation was also reported. Principal cations were Na+ and Ca2+, principal anions were Cl-,
and
Elevated As concentrations were linked to alkaline pH and evaporation, as confirmed by stable isotopes.
The most recent studies from the Lower Katari Basin were published by Quino Lima et al. (Citation2019,
Citation2020). The study area was located close to the Cohana Bay in the Titicaca Lake, where sediments are mostly of fluvio-glacial and fluvio-lacustrine origin. Samples were collected during wet season and included both groundwater (31 samples) and surface water (6 samples). Both have alkaline pH (groundwater 7.2–8.3, surface water 6.7–8.5) and moderately oxidizing corrected Eh values (groundwater 167.9–235.4 mV, surface water 240.9–245.7 mV). Groundwater water types are variable with 48% Ca-HCO3 type, 19% Ca-Cl type, and 16% Na-HCO3 type. Concentrations of As in groundwater are 0.75–89.7 µg L−1 and exceed in 48% of samples the WHO guideline (10 µg L−1). In surface water, only 1 sample (11.7 µg L−1) was above the WHO guideline. Concentrations of As are higher in wells close to the Titicaca Lake and based on speciation, the dominant are As(V) species ( and
). Increased As concentrations were contributed to geological factors, viz. alteration of volcanic rocks. Another important contaminant was found to be
with the range 0.2–221.4, where 23% of wells have As concentrations higher than the WHO guideline value.
4.5.1.2. Arsenic in surface water
The TDPS basin ranges from the Titicaca Lake in the north, which drains through the Desaguadero River to the south, flowing over the Andean Highland before discharging about 500 km southwards into Poopó Lake. Other rivers discharge from the east (sulfide mining areas in the Eastern Cordillera as As source) and the south (geothermal manifestations as As sources). Occasionally, when the water level of Poopó Lake is high, its water drains into Lacajahuira River and subsequently into Coipasa salt pan. Research on As is concentrated on Poopó Lake basin were reviewed by Bundschuh, Litter, et al. (Citation2012), Bundschuh, Nath, et al. (Citation2012). The findings of significant research since then are presented in the following section.
After 2010, research on As in the Poopó Lake basin was intensified. Hydrogeochemical data were collected for both surface and groundwater in a small watershed of the Poopó catchment. Samples were taken around the Poopó Lake and the smaller connected Uru-Uru Lake to its north (Ramos Ramos et al., Citation2012). The surface waters contained high concentrations of sulfate, As, Zn, and Pb linked to acid mine drainage.
4.5.2. Arsenic in mining areas
Pilcomayo River and its tributaries originate in the Western Cordillera, home of many mining activities and source of As, and flows down to the lowland in the foreland of the Andes. Arsenic contamination at Porco mine received global attention after breach of a tailings dam on August 29, 1996 that has caused until September 1, release of 235,000 m3 As-rich tailings (Macklin et al., Citation1996). This event triggered a series of research on As and heavy metals in water, suspended load, sediments, agricultural soils, food chain (fish, vegetables) and related human impacts. The results of these investigations are mostly documented in unpublished reports, which have been compiled in detail in two reviews (see Bundschuh, Litter, et al., Citation2012; Bundschuh, Nath, et al., Citation2012 and references therein). Since then, no new As data or information from this area has been reported.
In summary, in Bolivia As(V) seems to be predominant in most groundwater samples around the Poopó Lake, but values of Eh calculated on the basis of As(III)/As(V) pair indicate strong redox disequilibrium. This suggests that speciation of As based on the Eh-pH diagram should be interpreted with caution. Principal source of As in the Poopó Lake area seems to be geogenic, i.e., the alteration of volcanic rocks, but As concentrations can be increased by other processes, such as evaporation and also locally by mining activities and redox reactions (dissolution of Fe and Mn oxyhydroxides) linked to the discharge of organic-rich waste waters. On the other hand, thermal waters were discarded as a significant As source. The outcome of global spatial dependence analysis indicates a positive and statistically significant autocorrelation for six trace elements such as As, Al, Sb, F, B and Mn, and there is statistical evidence that the spatial dependency of the trace elements are not random but controlled by specific natural or anthropogenic factors (see Quino Lima et al., Citation2019 for details).
4.6. Chile
Chile is located along the southwestern border of South America and is limited to the east by the Andes. Its population of about 18 million is distributed among sixteen administrative regions (), of which the Metropolitan Region is the most densely populated. The Andean Cordillera of Chile is divided into the following three zones based on their distinctive geological characteristics: the Central (CVZ), Southern (SVZ), and Austral (AVZ) Volcanic Zones (). From north to south, these volcanic zones are separated by the Pampean Flat Slab (PFS), and Patagonian Volcanic Gap (PVG), respectively (Stern, Citation2004). Arsenic in Chile is mostly enriched in the environment, especially in the northern regions of the country (e.g., Oyarzún et al., Citation2004; Romero et al., Citation2003).
Figure 5. Arsenic distribution and concentration box plots of distinct water types (brines: Risacher et al., Citation1999; Tapia, Davenport, et al., Citation2018; saline water: Risacher et al., Citation1999; Tapia, Davenport, et al., Citation2018; hot springs: Aguilera et al., Citation2014; Chong et al., Citation2010; Hauser, Citation1997; Lemus et al., Citation2015; Pérez, Citation1999; Risacher & Hauser, Citation2008; Sánchez-Yañez et al., Citation2017; Wrage et al., Citation2017; surface water: DGA, Citation2017; Lagomarsino et al., Citation2018; Risacher et al., Citation1999; Romero et al., Citation2003; Tapia, Davenport, et al., Citation2018; groundwater: DGA, Citation2017; Leybourne & Cameron, Citation2008; Soto, Citation2010) and solid materials (tailing impoundments: SERNAGEOMIN, Citation2017; continental sediment: Astudillo et al., Citation2015; Baeza et al., Citation2014; Barrera et al., Citation2017; Espinoza et al., Citation2016; Lacassie et al., Citation2014; Lagomarsino et al., Citation2018; Narváez et al., Citation2007; Oyarzún et al., Citation2004; Palma-Fleming et al., Citation2012; Romero et al., Citation2003; marine sediment: Valdés & Tapia, Citation2019; soil: Agriquem, Citation2009; Alfaro et al., 2017; CENMA, Citation2014; De Gregori et al., Citation2003; López, Citation2014; Parra et al., Citation2014; PSG, Citation2015; PSG, Citation2016; volcanic and intrusive rocks: Benavides, Citation2006; Mamani et al., Citation2010; Marschik et al., Citation2003) found in the Central Volcanic Zone, Pampean Flat Slab, and Southern Volcanic Zone of Chile. The dashed horizontal lines in the box plots correspond to reference concentrations for comparison; for water (left-hand box plots), a reference concentration of 10 µg L−1 is shown (NCH Citation409, Citation409, Citation2006; WHO, Citation2011), and for solid materials (right-hand box plots), the mean As value found in the continental crust is shown (Hu & Gao, Citation2008). The distinct administrative regions of Chile are also numbered and shown in plan view from north to south: (XV) Arica and Parinacota, (I) Tarapacá, (II) Antofagasta, (III) Atacama, (IV) Coquimbo, (V) Valparaíso, (XIII) Metropolitan Region (where Santiago is the capital), (VI) Libertador Bernardo O'Higgins, (VII) Maule, (XVI) Ñuble, (VIII) Bio Bio, (IX) Araucanía, (XIV) Los Ríos, (X) Los Lagos, (XI) Aysén, (XII) Magallanes.
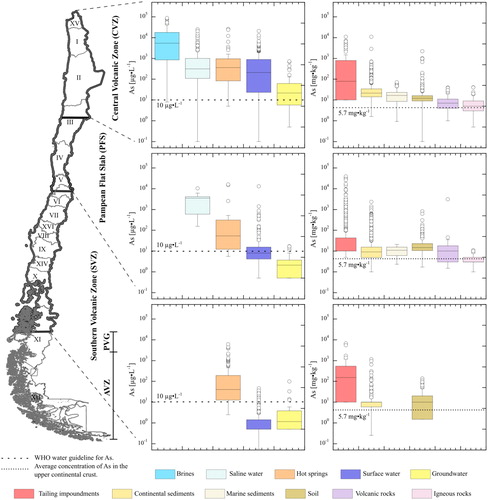
4.6.1. Arsenic in water
Throughout the country, water is mostly present in the form of surface water, cold and hot springs, in addition to groundwater. Of the distinct water types present, the highest concentrations of As are found in brines (up to 87,600 µg L−1; Risacher et al., Citation1999), followed by saline water and hot springs in the CVZ (up to 25,000 µg L−1; Risacher et al., Citation1999 and 45,000 µg L−1; Risacher & Hauser, Citation2008, respectively), saline water in the PFS (up to 10,400 µg L−1; Risacher et al., Citation1999), and hot springs in the SVZ (up to 10,000 µg L−1 at the Pemehue thermal springs (Hauser, Citation1997; ).
Surface water is characterized by higher concentrations of total and dissolved As in the northern regions of the country, and previous studies have shown that the difference between concentration of dissolved versus total As is not significant in samples obtained there (Leybourne & Cameron, Citation2008; Tapia, Davenport, et al., Citation2018). In the Antofagasta Region of northern Chile for example, additional water resources were needed to supply inhabitants following an increase in population due to increased mining activities in the mid-20th century. These new water resources included the Holajar and Toconce rivers, which were reported to contain 800 µg L−1 and 1300 µg L−1 of As, respectively (Smith et al., Citation1998), resulting in a chronic exposure of this element to the population between 1958 and 1970 (Marshall et al., Citation2007; Smith et al., Citation1998). In addition, a 10-year (2008–2017) compilation of data from the Dirección General de Aguas (DGA, Directorate General of Water), a government agency that provides the country’s water-related information, shows that As is highly concentrated in the northern administrative regions of Arica and Parinacota, Tarapacá, and Antofagasta (XIV, I, and II in , respectively). This compilation also shows that the total As values tend to decrease toward southern Chile, attributable to increased precipitation and dilution, as well as the absence of As-rich evaporites, brines, and saline water (Tapia, Rodríguez, et al., Citation2019).
The concentration of As also varies as a function of elevation in Chile. For instance, in the PFS, water quality monitored throughout the course of the Copiapó River (, Figure SM3) shows that in most of the sections of the river, As concentrations were found to be below the Chilean guideline for drinking water of 10 µg L−1 (NCH 409, Citation2006). However, in 2002, in the upper section of the catchment within the Andean Cordillera, certain tributaries have relatively higher As concentrations (>10 µg L−1), of geogenic origin, affirmed by the presence of As-bearing mineralization in those areas. A detailed analysis of As levels, based on historical data from DGA, was recently performed by Vega et al. (Citation2018), which indicates that the Loa, Elqui, and Lluta river catchments present higher As concentrations in the streams as well as groundwater.
Table 7. Variation of Arsenic concentration along the course of the Copiapó River.
Furthermore, bottled water in Chile has been found to be contaminated with As: a study performed by Daniele et al. (Citation2019) on the Chilean bottled water from the mineral springs, as identified by the brands, concluded that 3 out of 10 (30%) top selling brands contain As values above the Chilean water legislation of 10 µg L−1, reaching a maximum of 18.97 µg L−1 (for a popular brand).
4.6.2. Arsenic in soils and sediments
The available information related to As in soils, sediments, and rocks indicates its natural presence in some volcanic rocks and sediments of the Arica and Parinacota, Tarapacá, Atacama, and Coquimbo administrative regions (Tapia, Rodríguez, et al., Citation2019), as well as in marine sediments (Valdés & Tapia, Citation2019). For instance, As content in volcanic rock ranges between 1.0 and 3105 mg kg−1 (median 7 mg kg−1) and concentrations in sediments from the Arica and Parinacota region range from 1.6 to 2886 mg kg−1 (median 18 mg kg−1) (Tapia, Rodríguez, et al., Citation2019). Additionally, previous studies have reported important As values in sinter structures of the Puchuldiza geothermal field and El Tatio Geysers (Nicolau et al., Citation2014; Sánchez-Yañez et al., Citation2017), as well as in saline precipitates of the Atacama Desert (Tapia, Davenport, et al., Citation2018; Tapia, González, et al. Citation2018), evaporites of the Altiplano-Puna plateau (Alonso et al., Citation1991; Risacher et al., Citation1999), high sulfidation epithermal deposit of El Indio (Jannas et al., Citation1990), and sediments of the Elqui River basin (Oyarzún et al., Citation2006, Citation2004). Oyarzún et al. (Citation2004) indicated that Holocene lacustrine sediments (BP 9640 ± 40) exhibit high As concentrations (747 ± 544 mg kg−1, n = 14) in close proximity to the Au deposit of El Indio, and the presence of As there is mostly related to iron oxides and oxyhydroxides. On the other hand, a recent case study performed in the Copiapó River valley (, CL8) at the northern border of the PFS () showed that mud and airborne dust samples presented As concentrations below 100 mg kg−1 (this study).
In 2018, a study was performed on public playgrounds for children to assess the soil quality in the Bio Bio Region of south-central Chile. It identified that the average As values range from 18.82 to 23.53 mg kg−1 at the different studied sites. Using international guidelines for soil contamination (VROM, Citation2000 and ADEC, Citation2010), analysis of the pollutants showed that there was no health risk for children in the studied playgrounds of that region (Rodríguez-Oroz et al., Citation2018).
4.6.3. Arsenic in mining areas
In Chile, the following sources generally show high concentrations of As: (i) evaporites of the Altiplano-Puna plateau (Alonso et al., Citation1991), (ii) brines (Risacher et al., Citation1999), (iii) volcanic rocks (Tapia, González, et al., Citation2018), (iv) hot springs (Cortecci et al., Citation2005; Risacher & Hauser, Citation2008; Sánchez-Yañez et al., Citation2017; Tapia, Davenport, et al., Citation2018), and (v) certain mineral deposits, such as Eocene-Oligocene porphyry copper (e.g., La Escondida; Padilla Garza et al., Citation2001) and epithermal deposits (e.g., El Indio; Jannas et al., Citation1990). In brines (TDS ≥ 50,000 mg L−1), dissolved As in the Chilean Altiplano-Puna plateau presents an average of 14,200 µg L−1 (data from Risacher et al., Citation1999; Tapia, Davenport, et al., Citation2018), and in El Tatio Geysers, extremely high values of up to 45,000 µg L−1 have been measured (Risacher & Hauser, Citation2008). Copper concentrates generated from porphyry copper deposits in Chile can present elevated concentrations of As. For example, a 0.79% (7900 mg kg−1) concentration of As is found in a Chuquicamata copper concentrate (Fuentes et al., Citation2009), where As is mostly related to tennantite (Cu12As4S13) and enargite (Cu3AsS4), decreasing the economic value of the concentrate (Long et al., Citation2012). In El Indio Mine, the copper concentrate contains 8% (80,000 mg kg−1) of As, which is mainly related to enargite (Oyarzún et al., Citation2004).
4.6.4. Arsenic from other sources
Apart from the various natural sources of As in Chile, anthropogenic contamination of this metalloid is also present. One notable example corresponds to the Port of Antofagasta, where 22 out of 300 young children attending kindergartens in the close vicinity showed levels of As in urine of up to 43 µg L−1 (Minería Chilena, Citation2015). In the city dust, values ranging between 127 and 1492 mg kg−1 of As have been detected in a radius of less than 0.5 km from the port (Table 2 in Tapia, Valdés, et al., Citation2018). These As values are considered to be the highest ever reported in the city dust (239 ± 269 mg kg−1; Table 8 in Tapia, Valdés, et al., Citation2018) and an average of 50% is bioaccessible (Table 8 in Tapia, Valdés, et al., Citation2018). Another example where As pollution has been detected is in close proximity to the Potrerillos Smelter in the northern Atacama Region with average values of 730 µg L−1 and 463 mg kg−1 in water and sediment, respectively. Occasional heavy rains, such as the precipitation event of March 2015, have been able to mobilize dissolved and particulate As in the surface environment and create a hydraulic connection between the El Salado Alto and El Salado Bajo basins. Following this high precipitation event of 2015, the average concentration of dissolved As increased from 2 µg L−1 in 2014 to 287 µg L−1 two months after the flooding, with a subsequent decrease to 19 µg L−1 one year after the flooding, when the hydraulic disconnection was restored between El Salado Alto and El Salado Bajo (Tapia, Davenport, et al., Citation2018). At the Ventanas Industrial Complex in the Valparaíso Region of central Chile, a soil mapping survey indicated that concentrations of As exist over 40 mg kg−1, with a maximum of 824 mg kg−1 in close proximity to this facility (Tume et al., Citation2019). Median concentrations of As there are 13.4 mg kg−1 higher than median concentrations of As in urban soils of Arica (11 mg kg−1), northern Chile (Tume et al., Citation2019; Tapia, Rodríguez, et al., Citation2019). As a consequence, students attending La Greda School in 2011, 500 m to the east from the smelter, had considerable amounts of As in their blood (El Mostrador, Citation2011). Furthermore, elevated As concentrations in the catchment area of the Copiapó River, where a contributing factor includes nearby mining activities (present study), showed values within the range of approximately 2 and 15 µg L−1 (Figure SM3). Tailing impoundments sampled by the National Geology and Mining Service of Chile (SERNAGEOMIN, Citation2017) show that the highest values of As within solid materials reach up to 60% As (603,458 mg kg−1) ().
4.7. Brazil (without Guarani aquifer)
In the Brazilian territory, the studies on As (or including As) have identified As-related issues in 13 out of 26 states (). However, studies are very punctual, or concentrated only in few study areas, viz. the Iron Quadrangle (, BR1). The country is mostly affected by natural As, but As from anthropogenic sources have also developed in the last decade. The first is related to volcanic activity and weathering of rocks, which results in mineral dissolution and/or release in wind-blown dusts, hydrothermal ore deposits and seasalt (De Carlo et al., Citation2014; Alloway et al., Citation2016; WHO, Citation2017). The second is related to mining, metal smelting, pesticide/herbicide/fertilizers production and use, combustion of fossil fuels, timber and leather treatment, industrial effluents, and glass processing (WHO, Citation2017).
The study of environmental problems involving As in Brazil has a history of approach that considered soil, water and sediment as main matrices. In recent years, however, the diversity of evaluated matrices has increased with the analysis of foods such as rice, beef, chicken, seafood and bee pollen, with the main objective of ascertaining the risk of As exposure to the population (Morgano et al., Citation2010; Batista et al., Citation2011; Lemos et al., Citation2014; Azevedo et al., Citation2018) (). At the same time, studies were started on human samples (biomarkers), and levels of As in urine, blood and hair have been determined as a tool to expand the evaluation of As contamination in Brazil (Santos et al., Citation2003; Sakuma, Citation2004; Freire et al., Citation2015).
Although As-focused studies are concentrated in Brazil, mainly in the states of Minas Gerais, São Paulo, Paraná, Amazonas and Rio de Janeiro, studies have also been reported from other states, viz, Acre, Amapá, Roraima, Mato Grosso, Bahia, Santa Catarina and Rio Grande do Sul (Burger et al., Citation2009; Hatje et al., Citation2010; Batista et al., Citation2011; Mirlean et al., Citation2014; Corguinha et al., Citation2015; Costa et al., Citation2015; Freire et al., Citation2015; Rosolen et al., Citation2015; Santos et al., Citation2015; Silva et al., Citation2015; Freitas et al., Citation2017; Cagnin et al., Citation2017; de Meyer et al., Citation2017; Dolci et al., Citation2017; Gao et al., Citation2018; Silva et al., Citation2018; Carvalho et al., Citation2018, Paraguassú et al., Citation2019; da Silva Junior et al., Citation2019; Baeyens et al., Citation2019; Costa et al., Citation2019) ().
Among the studies carried out in Brazil, most are related to sources arising from the processes of mining and refining metals or the presence of geochemical anomalies added to the industrial influence.
4.7.1. Arsenic in water
Regarding water bodies, the main anthropogenic sources of As occur due to the discharge of industrial effluents, mining and leaching of soil contaminated by phosphate fertilizers (De Carlo et al., Citation2014). Even considering a population that consumes As-free water, the use of contaminated water for irrigation poses a risk of soil contamination and exposure to the food chain through plants and animals that will feed humans (Bundschuh, Nath, et al., Citation2012; Datta et al., Citation2012). Ingestion of contaminated water, along with the consumption of contaminated food, stands out as the main route of exposure of the human organism to As (Chung et al., Citation2014).
4.7.1.1 Arsenic in groundwater
Groundwater is likely to contain higher levels of dissolved As than surface water, which occurs due to the lower availability of suspended particulate matter (SPM), the weathering of rocks and the natural process of percolation water filtration at various soil profile layers (Schaefer et al., Citation2017; Machado et al., Citation2019).
Brazil is one of the world’s most privileged countries with both surface water and groundwater sources. It has two of the largest aquifers in the world: Alter do Chão, under the states of Pará and Amazonas; and Guarani, under the states of Goiás, Mato Grosso, Mato Grosso do Sul, Minas Gerais, Parana, Rio Grande do Sul, Santa Catarina and São Paulo, reserves that also are shared with Argentina, Paraguay and Uruguay (Sindico et al., Citation2018). At the same time, despite strategic importance of its groundwater reserves, the country lacks strategic management, experiencing cyclical supply and pollution crises, even in regions of intense use of aquifers.
The water from the Guaraní Aquifer System (GAS) is not always suitable to be consumed without treatment, as exemplified by Gastmans et al. (Citation2010). The so-called “Thermal Corridor” of Uruguay River, located in the triple border between Argentina, Brazil and Uruguay, presents high contents of As (>10 µg L−1 in groundwaters from this area), being the water used for recreational purposes in this region, and is discussed in Section 4.11.
Brazil has 12% of the surface fresh water of the planet, hosting large hydrographic basins, such as those of the São Francisco, Paraná and Amazonas rivers (Machado, Citation2003). However, Brazil has serious problems of basic sanitation, with only 50.3% of the Brazilian households with access to the sewage system, which makes the population susceptible to contamination by toxic elements (SNIS, Citation2015). This section critically assesses the problems of environmental contamination by As in Brazil during the last 10 years, highlighting the gaps and providing recommendations for new research in the area.
In Brazil, few studies have evaluated the presence of As in groundwater, with some records in the SE, such as in the states of Minas Gerais, São Paulo and Rio de Janeiro. In Minas Gerais stands out the region of the Iron Quadrangle (, BR 1), that is reference in mining activities. In the Iron Quadrangle, As is present in rocks that host auriferous sulfide deposits (Borba et al., Citation2003; Borba & Figueiredo, Citation2004). The highest concentrations of As were reported in this region, reaching a maximum value of 2980 µg L−1 in groundwater (Silva et al., Citation2018; Carvalho et al., Citation2018, Paraguassú et al., Citation2019).
In the Municipality of Jundiaí, State of São Paulo, As was evaluated in water from domestic wells resulting from the application of phosphate fertilizers containing As in adjacent soils. The maximum content of As found was 170 µg L−1 (Campos, Citation2002; Souza et al., Citation2019). In the State of Rio de Janeiro, As was detected in aquifers in the Paraíba do Sul River delta, and its source and factors controlling its distribution were evaluated by Mirlean et al. (Citation2014). In this study, the As content in the groundwater was found to reach a maximum value of 17.2 µg L−1 and it was reported that the geological structure of the Paraíba do Sul River delta resembles the problem areas with As contamination in Asian deltas. In 1993, the World Health Organization (WHO) has reduced the maximum guideline limit for As in drinking water from 50 to 10 g L−1, based on its adverse effects on human health (WHO, Citation2017). In Brazil, the maximum potability standards are determined by Ordinance N°. 2,914, on December 12th, 2011 and whose maximum limit is 10 µg L−1 (Brazil, Citation2011).
However, there remains considerable uncertainty if the 10 μg L−1 is a safe limit ensures protection of human health from the adverse effects of As (Ahmad & Bhattacharya, Citation2019). In this sense, the search for efforts to reduce the upper limit of arsenic considered safe for human portability (e.g., as is already the case in New Jersey and Denmark, which establishes 5 μg L−1 of arsenic in drinking water and The Netherlands where drinking water companies are making efforts to reduce the As in drinking water to less than 1 μg L−1) (Ahmad et al., Citation2018; Ersbøll et al., Citation2018; Ahmad & Bhattacharya, Citation2019). However, the As extraction and determination methods used in conventional treatments are not efficient to the point of obtaining such low final concentrations (WHO, Citation2017).
The National Council of Environment (CONAMA) Resolution N° 420/2009 provides guiding values for the presence of chemicals and also establishes a reference value of As in groundwater as 10 μg L−1 (CONAMA, Citation2009). The reference value is taken as the concentration of a given substance in which, exceeding this value, it may cause direct or indirect risks to human health, requiring intervention (e.g., treatment) regarding the use of this water for drinking (CONAMA, Citation2009). CONAMA Resolution N° 357/2005 – delimits the maximum values of As for surface bodies and determines their possible uses according to their classification. For vegetable and aquaculture irrigation, the maximum permitted value (MPV) of As is 10 μg L−1 and for animal consumption, irrigation of trees, cereals and forage, the As limit rises to 33 μg L−1 (CONAMA, Citation2005). CONAMA Resolution N°. 396/2008 deals exclusively with groundwater framing. In this case, the MPV for irrigation is not explicit and the MPV for animals thirst-quenching is 200 μg L−1 (CONAMA, Citation2008) ().
Table 8. As content in soils (mg kg−1) and in water bodies (µg/L−1) according to Brazilian legislation.
There is no record of studies evaluating As dispersion from groundwater use for irrigation, leaving a significant gap in the literature, considering As toxicity. Because of the high toxicity and little available information on As soil behavior studies, there is an urgent need for studies that can assist in risk prediction and mitigation of As contaminated areas (Campos et al., Citation2013; Machado et al., Citation2019).
The problem of As in groundwater is of utmost importance and public interest, because there is a relationship of water imbalance between the spatial availability of water and the spatial pattern of consumption by urban centers in Brazil (Mitchell et al., Citation2019). In addition to this, the pollution of surface water sources and the increase in generation of electric power from fossil fuel power plants – consuming significant quantities of surface water - were essential factors for increasing demand of groundwater for various purposes, viz. human consumption, irrigation, industry and leisure (Zoby, Citation2008; Mitchell et al., Citation2019). The climatic and geological conditions of Brazil favor the formation of aquifer systems with sedimentary formations giving rise to productive aquifers occupying about 4,130,000 km2, which is approximately 48% of the national territory and has been intensively used (Mitchell et al., Citation2019).
4.7.1.2. Arsenic in surface water
In the SE region of Brazil, the Paraíba do Sul River basin is a water system of socioeconomic importance for the states of Rio de Janeiro, São Paulo and Minas Gerais, and is the recipient of industrial and residential wastes along its course (Nicolite et al., Citation2009). The lower basin of the Paraíba do Sul River basin is related to the northern plain of the State of Rio de Janeiro, extending from the city of Itaocara to the mouth, in Atafona, district of the Municipality of São João da Barra (Pinelli et al., Citation2011).
Effluents from industrial and municipal sewage are important anthropogenic sources of As for suspended particulate matter (SPM). In agricultural areas, the application of insecticides, herbicides, fungicides and fertilizers, in addition to soil erosion, affect the content of As in water (Smedley & Kinniburgh, Citation2002). Despite the sugar cane monoculture occurring since the 18th century in the Municipality of Campos in the study area there are no reports of the presence of point sources of As. Thus, it is possible that the geochemical association of As with Au (Borba et al., Citation2003) is responsible for the diffuse and natural occurrence of As, since the extraction of Au was performed in the 1980s in the Pomba and Muriaé rivers.
Other studies carried out in Brazilian river basins evaluated the relationship between As and Fe in sediments. In the São Francisco River basin and the Velhas River, it was demonstrated by sequential extraction that even at high concentrations the As remains practically immobile in the sediment due to its adsorption to Fe (Pereira et al., Citation2007; Rezende, Citation2009). In the Paraíba do Sul River basin delta (Mirlean et al., Citation2014) and in the Paracatu River (State of Minas Gerais) (Rezende et al., Citation2015; Bidone et al., Citation2014; Bidone et al., Citation2018), a significant positive correlation between As and Fe oxides was observed in surface sediment. In the Paracatu River a reduction of As in the dissolved fraction of the water column from 110 to 31 µg L−1 was observed, for example, as Fe increased in the sediment (3–9%), suggesting a higher retention of As by Fe oxides in the sediment (Bidone et al., Citation2014; Bidone et al., Citation2018).
de Meyer et al. (Citation2017) provide first insights on the presence and distribution of arsenic in groundwater resources of the Amazon Basin. The high concentrations of As (<0.5–715 µg L−1), Al (<5–3.3 mg L−1) or Mn (3–4 mg L−1) in groundwater along the populated floodplains of the Amazon River require urgent investigation on possible health effects.
Scarpelli (Citation2010) noted the similarity between sediments enriched with As deposited on the banks of the rivers that drain the Himalayas, a region known worldwide for contamination with As, with the Andes sediments deposited on the banks of the Amazonian rivers. The As concentration gradient found in the Amazon basin reinforces that this region can be an important source of As toxic to the population (Fernandes et al., Citation2018). In addition, there is a lack of comprehensive information on the background levels of As in the soils of the Amazon basin, given that the most recent studies have focused only on specific eastern Amazon states (da Silva Junior et al., Citation2019).
4.7.1.3 Arsenic in seawater
The coastal zone impacted by As-laden river water and sediments discharging into the Atlantic Ocean can also reach high levels (, BR15) (Mirlean et al., Citation2011, Citation2012, Citation2013, Citation2014, Citation2016; Cagnin et al., Citation2017; Dolci et al., Citation2017; Gao et al., Citation2018; Baeyens et al., Citation2019).
In the Bay of Todos Santos (BA) (, BR 14) the presence of petrochemical, chemical, metallurgical and port industries are responsible for high concentrations of As in the sediment, which shows values up to 30 mg kg−1 (Hatje et al., Citation2010). In a study conducted by Mirlean et al. (Citation2014) in the Paraíba do Sul River basin delta samples of coastal sediments of lakes/intertidal marshes were analyzed in order to determine the source of As. The concentration of As in the sediment reached values up to 270 mg kg−1. Due to the lack of connection of the interdune lakes/swamps with the fluvial channel, the atmospheric entrance of the As was considered as the main source for the sediments.
In relation to the marine sediments, the anthropogenic activity also influences the concentration of As. High As contents (up to 50 mg kg−1) in Patos Lagoon estuarine sediments have previously been reported, and about 80% of the As there is found in a bioavailable form (Mirlean et al., Citation2003; Mirlean et al., Citation2012; Costa et al., Citation2019). In a review carried out by Baeyens et al. (Citation2019) were found 270 mg kg−1 in the gyttja of the interdunes, 120 mg kg−1 in beach sands at the coasts of the states of Bahia and Espírito Santo and up to 108 mg kg−1 in the Doce River continental shelf. Total As concentrations above the Brazilian legislation threshold for marine sediments 70 mg kg−1 in beach sand and surface sediments (, BR 17).
4.7.2. Arsenic in soils and sediments
A major source of As in agricultural soils is the use of continued use of as-rich water for crop irrigation (Gillispie et al., Citation2015). Soils rich in clay minerals, which have smaller particles and larger surface area, have a higher ability to adsorb As (Nriagu et al., Citation2007). Speciation and mobility of As in soil is highly influenced by oxidation and reduction processes. The chemical forms of As adsorb primarily to Fe and Al (hydro) oxides present in the soil, with arsenate being adsorbed more strongly (Takahashi et al., Citation2004).
An average concentration of 5.2 mg kg−1 As was found in Brazilian oxisols, the most common soil type in the country (Campos et al., Citation2007). A recent study using an unprecedented databank based on laboratory analyses (including rare earth elements), proximal and remote sensing, geographical information system operations, and pedological information revealed that the median soil As content in Brazilian soils ranged from 0.14 to 41.1 mg kg−1 in reference soils, and 0.28 to 58.3 mg kg−1 in agricultural soils. Predictive models of topsoil As content using machine learning (ML) algorithms showed that the most important environmental covariables governing As content in Brazilian soils, which were arranged in 4 natural groups, were temperature, soil organic carbon (SOC), clay, sand, and TiO2 (Menezes et al., Citation2020). Increasing soil pH may lead to reduced As adsorption on Fe and Al oxides by OH− competition for binding sites (Kader et al., Citation2016). Below pH 8.5, As adsorption to oxyhydroxides is high and at pH >6, arsenite is more strongly adsorbed to minerals than arsenate occurring the maximum adsorption of anions near pKa1 (Herbel & Fendorf, Citation2006). The dissociation constants of As(III) exists predominately as H3AsO3 are pKa1 = 9.22; pKa2 = 12.10; pKa3 = 13.40. The dissociation constants of As (V) as H2AsO4 and HAsO4 are pKa1 = 2.22; pKa2 = 6.96; pKa3 = 11.5 in natural aqueous environments (Sadiq et al., Citation1983; Masue et al., Citation2007).
Reducing conditions can lead to precipitation of As containing sulfide minerals. Thus, it is expected to find low As content in the gaqueous phase when there is a high free sulfide content (Nriagu et al., Citation2007). Soils with high sulfide concentration under reducing conditions (swamps, for example) tend to have higher concentrations of As (Smedley & Kinniburgh, Citation2002). Mining activities and the use of agricultural inputs can increase As concentration to critical soil levels (Smedley & Kinniburgh, Citation2002).
The labile fraction of As present in soil is available to plants, contributing to the accumulation of As in edible parts, exposing As to human ingestion (Joseph et al., Citation2015). The bioavailability of As in soil is difficult to predict as it depends on factors such as soil hydrogeology and geochemistry, climate, height of the saturated soil zone and its fluctuation, among others (Uddh-Söderberg et al., Citation2015).
da Silva Junior et al. (Citation2019) observed that the total concentration of As ranged from 0.98 to 41.71 mg kg1, with values generally increasing from the topsoil (0–20 cm) to the deepest layer (40–60 cm) in all the studied sites in the Amazon basin (, BR 4-13).
In Brazil, CONAMA Resolution N° 420/2009 (CONAMA, Citation2009) provides criteria and guiding values for soil quality regarding the presence of As and other chemical substances. This resolution establishes quality reference values (QRV), prevention values (PV) and investigation values (IV). QRV is the concentration that defines the natural quality of the soil, with each state in the Union being responsible for establishing this limit, given the national heterogeneity of the soils. PV is the concentration that, above this limit, the soil may not be able to sustain its main functions and harmful effects to the environment can be seen. The IV, which also includes groundwater, is considered to be the concentration of a certain substance in which, exceeding this value, it may cause direct or indirect risks to human health, requiring intervention in the use of the contaminated matrix (CONAMA, Citation2009). The national PV determined in the resolution is 15 mg kg−1. Regarding IV, it is separated according to its use. IV is 35 mg kg−1 for agricultural soils, 55 mg kg−1 for residential soils and 150 mg kg−1 for industrial soils ().
Once the As concentration in the soil is below the QRV established by the state it is in (class 1), no action is required on the spot. Soils with As concentrations greater than the established QRV and less than or equal to the PV (class 2), may require an assessment by the environmental agency, not necessarily involving investigation. Soils with As concentration values between PV and IV established for their type of use (class 3) require source identification, assessment of natural occurrence, control of contamination sources and monitoring of soil and groundwater quality. Soils with a concentration of As above the IV established for their type of use, the area is considered unsuitable and a series of measures must be taken in order to eliminate or amortize the risk.
4.7.3. Arsenic in mining areas
The As problem is concentrated mainly in four regions:
Iron Quadrangle” (, BR1) in Minas Gerais State, where As is released into the environment through activities related to Au mining (Matschullat et al., Citation2000; Deschamps et al., Citation2002; Borba et al., Citation2003, Borba & Figueiredo, Citation2004; Mello et al., Citation2006; Deschamps & Matschullat, Citation2007; Bundschuh, Litter, et al., Citation2012; Silva et al., Citation2018; Carvalho et al., Citation2018, Paraguassú et al., Citation2019).
Ribeira Valley (, BR3) in São Paulo and Paraná states, which is highly contaminated due to mining, processing and refining of Pb-Zn-Ag ores, in which As is naturally found in rocks and soils (Figueiredo et al., Citation2003, Citation2007; Abreu & Figueiredo, Citation2014; Akcil & Koldas, Citation2006; Blodau, Citation2006; Campaner & Silva, Citation2009; Cha et al., Citation2018).
The Amazon region (, BR4-13), including the area of the Municipality of Santana, in the State of Amapá, where As is associated with manganese (Mn) ores exploited during the last five decades (Santos et al., Citation2003; Mandal et al., Citation2004; Bundschuh et al., Citation2017, de Meyer et al., Citation2017, da Silva Junior et al., Citation2019).
In the City of Paracatu (northwest of Minas Gerais) (, BR 2) found measurable levels of contamination pronounced arsenic from the history of Au mining activity (Bundschuh et al., Citation2017). However, despite the high concentrations of total As, studies show that it is very low As bioaccessibility in tailings in the gold mining area in Paracatu, as it is mainly associated with poorly crystalline Fe arsenate (Ono et al., Citation2012; Guilherme et al., Citation2014; Rezende et al., Citation2015; Freitas et al., Citation2015). On the other hand, a study conducted by Ng et al. (Citation2019) shows that the total As intake is about 10% of the JECFA BMDL0.5 of 3 μg kg−1 b.w. per day, and the combined risk based on the cancer slope calculation is similar to the arsenic intake from the consumption of 2 L of water containing 10 μg L−1 of As. Food was the main contributor of the daily total intake of arsenic with rice and beans being the most significant ones (Ng et al., Citation2019).
A study was conducted by Cagnin et al. (Citation2017) on the Doce River, site of a massive ore mining where the tailings dam collapsed in November 2015. Millions of liters of tailings were dumped into the river and hit the mainland shelf of Brazil, causing the largest environmental disaster of the country (Segura et al., Citation2016). These data provide an important perspective on contamination on continental shelves and encourage monitoring of the mine’s environmental impacts (Cagnin et al., Citation2017).
In that sense, the studies on the water, mud and sediments in the river right after the disaster, and one year after it, was conducted by different organizations (IBAMA, Citation2015; CPRM, Citation2015; GEMG, Citation2015; GIAIA, Citation2016; IGAM, Citation2016; ANA, Citation2016; Segura et al., Citation2016). They found high levels of Mn, As, Cd, Se and Pb in the Doce River water. While the sediment and water assessment performed by Embrapa (Citation2015) did not detect dissolved metals in the water that characterize contamination caused by the disaster, as the heavy metal concentrations in the water were consistent with previous records. In this sense, the results presented in the study conducted by Davila et al. (Citation2020) demonstrate a scenario of predisaster contamination for the Doce River watershed. These authors conclude that the deposited tailings are not a time-bomb for heavy metals contamination in the region (Davila et al., Citation2020).
However, in November 2019, it was reported in the main Brazilian newspapers (https://g1.globo.com/mg/minas-gerais/noticia/2019/11/07/estudo-feito-em-mariana-e-barra-longa-atingidas-pela-lama-da-samarco-aponta-perigo-urgente-para-a-saude-publica.ghtml) that eleven residents of the City of Barra Longa (MG), who performed toxicological tests in 2018 were diagnosed with nickel poisoning, according to a report from the Health and Sustainability Institute sent to the Public Prosecution Service (ISS, Citation2017). For half of the participants, blood As levels were altered. In contrast to the rural districts of Bento Rodrigues and Paracatu, whose residents were relocated to Mariana after their villages were buried by the dam’s collapse, most of those affected in Barra Longa were not relocated. They remained in the city during its reconstruction. Part of the mud was removed over a year after the disaster, part was grounded on the football field and the exhibition grounds, part was turned into a cobblestone and part dried, turned to dust and spread across the city with the movement of trucks and heavy vehicles. Exposure to dust, according to the report, may be linked to some of the symptoms described by the population (Vormittag et al., Citation2018).
Similarly, one month after the Córrego do Feijão mine dam rupture occurred in 2018, residents of Brumadinho and the region began to have the same symptoms as the populations affected by tailings dam collapsed Mariana; itching and skin irritation, respiratory allergies and hair loss (Vormittag et al., Citation2018, Khan et al., Citation2020). Across the Paraopeba basin, about 1.3 million people may be contaminated with heavy metals. Due to As toxicity and potential exposure to humans (ATSDR, Citation2019), it is important to warn people living in the studied locations about the risks related to the use of the soil (Davila et al., Citation2020).
Arsenic hydrological pollution in the Santa Catarina region (, BR 16), on the other hand, is caused by the leading to acid mine drainage of coal mining and processing operations (Campaner et al., Citation2014, Freitas et al., Citation2017)
4.7.4. Arsenic from other sources
In the State of Paraná, a study was carried out to evaluate the exposure of the population (samples were obtained from 54 farmers and 108 healthy unexposed individuals) to As through blood analysis (Rocha et al., Citation2015). Samples of blood serum from vineyard farmers were analyzed to test the presence of As due to the use of pesticides with toxic metal(loid)s in those crops. Blood samples were collected at three distinct times: two in summer and one in winter. In winter the decrease of heat and rainfall reduces the proliferation of pests and the plants become more resistant. Thus, in this period, smaller amounts of pesticides are used when compared to summer. The content of As present in blood serum was positively correlated with the amount of pesticide used at specific times of the year, with higher contents in summer (3.4 and 3.8 µg L−1) compared to winter (2.1 µg L−1) ().
Table 9. Arsenic content in hair, urine and blood, meat and liver of cattle and chicken (μg g−1) obtained in different studies in Brazil regions, states or cities.
In Rio Branco, in the State of Acre, Freire et al. (Citation2015) determined the level of exposure to As in blood donors (n = 1183) (). Arsenic was found in almost all donors (99.5%), with higher levels in males, older adults and smokers. In this study, the lack of association between As in blood and water contamination in Rio Branco demonstrates greater importance of other As sources, such as smoking and occupational exposures (ATSDR, Citation2007).
In Campos dos Goytacazes (Rio de Janeiro), a study was carried out to estimate the risk of exposure of the population to As by consumption of meat and liver of cattle and chicken and the probability of cancer occurrence (Caldas et al., Citation2016) (). As concentration was determined in 58% of the total samples (n = 250), but the concentration of this element in food items, as well as the estimated tolerable intake, was below that established by legislation. Meats in general have higher values of As compared to vegetables. A study by EFSA (Citation2014) showed that this food group has the highest concentrations of inorganic As. The maximum limits of Brazilian legislation are: 0.5 mg kg−1 (muscle and eggs) and 1 mg kg−1 (viscera and fish) (ANVISA, Citation2013). Specifically, in relation to chicken, the most consumed animal type by Brazilians (OECD, Citation2016), the highest concentration values are usually found in the kidneys and liver, as they are organs of filtration and metabolization (Ghosh et al., Citation2012; Caldas et al., Citation2016). Since As is bioaccumulative, broilers raised in environments with exposure to high concentrations of this element will reflect these high concentrations in their tissues. Studies conducted in countries with known As contamination in water, such as Bangladesh and Pakistan, show As concentration values well above what Brazilian law allows for chicken cuts (EFSA Citation2009; Ghosh et al., Citation2012; Kazi et al., Citation2013).
In the case of Brazil, in order to prevent foods with high concentrations of As reaching the end consumer, ANVISA (Citation2013) establishes maximum As concentration values in foods. The maximum allowable quantities vary according to the origin and type of the food in question.
The Codex Alimentarius Commission of the Food, the joint intergovernmental body of FAO and WHO (FAO/WHO Codex Alimentarius, Citation1995) has set benchmarks for certain types of food. For butters and other edible oils and fats, the recommended limit is 0.1 mg kg−1. The recommended limits for food salts and polished rice are 0.2 and 0.5 mg kg−1 respectively. The European Union recommends only maximum values for rice and rice-based products. These range from 0.1 mg kg−1 for baby food rice to 0.3 mg kg−1 for rice-based cakes, cookies and waffles. In other food items, each country is responsible for its legislation (EC, Citation2015).
The maximum limits of As in Brazilian legislation are very broad for plant types and range from 0.1 mg kg−1 (vegetables in general) to 0.8 mg kg−1 (nuts). In general, As concentrations vary considerably for each plant type, soil type and irrigation water quality (Bundschuh, Nath, et al., Citation2012).
The Government of Brazil determined, through Ordinance N°. 31, on January 29th, 2002 (Brazil, Citation2002), the cancelation of the registration of all products formulated with active ingredients based on As in the area of feed, besides the manufacture products intended for animal feed for the purpose of promoting growth or improvement of animal performance. Nevertheless, Caldas et al. (Citation2016) found As concentrations in 3 out of 4 feed brands (0.79 ± 0.49; 1.03 ± 0.56 and 0.35 ± 0.37 mg kg−1) and in 3 out of 4 feed brands. mineral supplement (0.79 ± 0.49; 1.03 ± 0.56 and 0.35 ± 0.37 mg kg−1) for chicken sold in Campos dos Goytacazes, São Fidélis and São Francisco do Itabapoana, cities located in the north and northwest of the State of Rio de Janeiro, which may expose consumers of the region’s chickens to As. Despite the low As content reported in the samples, meat and liver consumption represents a source of As for the population in question, but just considering these sources in isolation, there is no risk.
4.8. Paraguay (without Guarani aquifer)
4.8.1. Arsenic in water
Arsenic contamination in Paraguay is reported in the Yrenda-Toba-Tarijeño and the transboundry Guarani Aquifer systems. The last will be discussed separately in section 4.11 as it forms a single geological unit that needs including all the countries over which it is extended. The Yrenda-Toba-Tarijeño (SAYTT) aquifer system is a regional, semi-confined to confined aquifer of fresh, brackish and saline water, western region of Paraguay, covering an area of about 197,280 km2 (Larroza & Fariña, Citation2005). It is located in the Paraguayan Chaco, which borders the plains of Bolivian Chaco in the north and west and the Argentinean Chaco to the south and the Paraguay River to the east (Larroza & Fariña, Citation2005). The most important aquifer recharge by rivers is through Pilcomayo River (Larroza & Fariña, Citation2005; Fariña et al., Citation2018). In Bolivia, mining activities since the year 1540 and release of respective heavy metals and metalloids, including As, resulted in continuous impact on downstream areas, e.g., the collapse of a lead-zinc tailing dam at the Porco mine Potosí (Bolivia, see Bundschuh, Litter, et al., Citation2012; Bundschuh, Nath, et al., Citation2012), where the toxic effects of the spill in the mining area of Potosí were apparent up to 800 km downstream in Chaco, Paraguay-Argentina (Garcia-Guinea & Harffy, Citation1998). Release of As and other contaminants into Pilcomayo River, the water of which recharges the SAYTT aquifer system in Paraguay is of particular concern, especially because many communities use the water for drinking, washing and irrigation purposes as well as animal husbandry (Bundschuh, Nath, et al., Citation2012). It is probably that these communities close to the Pilcomayo are being directly exposed to As (Archer et al., Citation2005) during several years.
According to Fariña et al. (Citation2018), the SAYTT aquifer system includes three hydrogeological zones (A, B, C): A. This zone is located in the borders with Bolivia and Argentina and covers an area of 25600 km2. Arsenic concentrations in freshwater varies from 3.0 to 120 µg L−1 for samples (T 21.5–31.4 °C, pH 7.0–9.0, TDS 0–1000 mg L−1). The salinity and the percent of SO4 2 − and Cl− increase with the flow direction (from NW to SE, as all Chaco), except in the Bolivia border, where are found medium to coarse grained sand and gravel. These waters are of Ca/Mg-HCO3 type, or in some cases of Na-HCO3 type. Cl− content is very low in this type of groundwater. B. This zone is located toward the east along zone A. It covers an area of 57,600 km2 and it is mainly formed of brackish or saline waters with As concentrations of about 3.0 µg L−1 for 6 samples (T 25.5–31.2 °C, pH 6.6–8.1, TDS 1000–10,000 mg L−1). The waters are Na-SO4 and Na-Cl type. The zone has an increase contact with evaporites of Cenozoic sediments. The salinity of these waters increases toward to the east. The waters are mainly used for livestock watering and after desalination for human purposes. C. This zone is located to the east side of zone B and covers an area of 11,4080 km2 of saline groundwater (>10,000 mg L−1) in the direction of the groundwater flow. The water contains chloride, sodium sulfate with low bicarbonate content. Arsenic concentration has not been reported in this zone.
4.9. Uruguay (without Guarani aquifer)
4.9.1. Arsenic in water
Three aquifers (Raigón, Mercedes, Chuy) have been studied in southwestern Uruguay (Manganelli et al., Citation2007; Goso et al., Citation2008; Mañay et al., Citation2013), where As content in groundwater indicate values above the 10 µg L−1 drinking water limit. Of these, the Mercedes aquifer showed the highest As concentrations (up to 58 µg L−1) (Manganelli et al., Citation2007). The waters of these aquifers are mainly used for agriculture, drinking, domestic use, tourism and raising cattle.
The Plio-Pleistocene detrital Raigón aquifer spreads over an area of about 1800 km2 and is widely exploited in southwestern Uruguay (Heinzen et al., Citation2003). It has a mean well depth of about 30 to 40 m with a discharge rate of 20 to >30 m3 h−1 (Manganelli et al., Citation2007). It is located in the Santa Lucia sedimentary basin, which is a southwestern segment of an extensional and dextral wrenching corridor related to the early Atlantic break-up pull-apart system (Rossello et al., Citation2018). The main surface drainage systems in the aquifer are La Plata and San Jose rivers (Manganelli et al., Citation2007; Mañay et al., Citation2014). In the San Jose (Libertad City) Department, As concentration of about 1.4–24.2 µg L−1 are reported (Manganelli et al., Citation2007; Goso et al., Citation2008; Mañay et al., Citation2013), while in Canelones Department, As concentration varies from 3.4 to 11.8 µg L−1 (Manganelli et al., Citation2007). Groundwater is predominantly of Na-HCO3 and Na-Cl type (Mañay et al., Citation2013).
Manganelli et al. (Citation2007) have found that distal sediments of the Raigón aquifer in the San Jose Department have groundwater with higher As concentrations compared to groundwater from proximal facies, for example Canelones Department south Paraguay. According to Mañay et al. (Citation2013), anomalous As concentrations (>7 mg kg−1) analyzed in a few samples of sediments of Raigón aquifer allowed them to postulate an anthropogenic origin, probably due to the application in the past of arsenical pesticides and herbicides. Pistón et al. (Citation2013) and Mañay et al. (Citation2013), in a study of the groundwater (37 water samples; max: 24.19 µg L−1), found that 80% samples with As levels exceed the 10 µg L−1 WHO guideline, and 11% exceed the 20 µg L−1 of the Uruguayan regulatory limit for drinking water. In the Kiyú region, nine sediment samples of Raigón and Libertad formations were analyzed and As concentrations varied from 9820 to 1180 µg L−1, leading to the supposition that parts the population, as well as industrial and agricultural activities, are consuming water with too high As concentrations.
The Late Cretaceous Mercedes aquifer is located in the Parana hydrogeological province (Heinzen et al., Citation1986), where the mean well depth in the Paisandú area ranged from 40 to 100 m and has a discharge rate of 15–25 m3 h−1 (Manganelli et al., Citation2007). This aquifer is widely exploited for agricultural purposes in the center and south of the west littoral of the Uruguay River (Goso et al., Citation2006). The concentrations of As range from 9.9 to 23.0 µg L−1 (T 17.1, pH 7.4) and from 10.5 to 58.0 µg L−1 (T 21.1, pH 7.4) in the Young and New Palmira areas, respectively (Manganelli et al., Citation2007). The waters of the Mercedes aquifer are of Ca-HCO3 type in Young and Na-HCO3 type in Nueva Palmira cities, however one sample was of Ca-SO4 type in the Young area (Montaño et al., Citation2006).
Another important aquifer where As is reported is the Pleistocene detrital Chuy aquifer in Ciudad de la Costa (Canelones Department) (Goso, Citation1972). This aquifer extends over nearly the entire La Plata River and Atlantic Ocean coasts in Uruguay, where the mean well depth of this aquifer is from <10 m and a discharge rate of 10–20 m3 h−1 (Manganelli et al., Citation2007). Groundwater is generally fresh and of good quality, and provides an excellent water supply source for urban and rural population (Almagro & Custodio, Citation2004). The As concentration varies from 0.1 to 41.1 µg L−1, (T 21.3, pH 7.9) (Manganelli et al., Citation2007), indicating that at some sites As concentrations exceed the WHO guideline value. The aquifer waters are of Na-HCO3 and Na-Cl types (Montaño et al., Citation2006).
However, according to Mañay et al. (Citation2019), only 28% of all drinking water supplied by OSE (State Company Obras Sanitarias del Estado) is provided from groundwater sources, while the majority of the population receives drinking water from surface water source
4.10. Argentina (without Guarani aquifer)
Globally, Argentina is one of most affected countries by chronic As exposure (Bardach et al., Citation2015; Bundschuh, Litter, et al., Citation2012; Bundschuh, Nath, et al., Citation2012, Litter et al., Citation2019a, Citation2019c; Villaamil Lepori, Citation2015). In fact, it was the first country in Latin America where As-related diseases were described (Goyenechea, Citation1917). The main region where these problems are concentrated is the Chaco-Pampean Plain (over 1 × 106 km2), which is partially affected by high As in groundwater (<4–5300 µg L−1; Smedley et al., Citation2005), in addition to other associated trace elements (F, U, V, B, Se, Mo, etc.) and high levels of salinity and/or hardness. This As-affected region extends from the Paraguay border in the North to the Patagonian Plateau in the South and from the Pampean Hills in the west to the Paraná and de la Plata rivers in the east (Smedley et al., Citation2002; Bundschuh, Litter, et al., Citation2012; Nicolli et al., Citation2010, Citation2012). At present, in this region, the population at risk of high As concentrations in drinking water is estimated to be around four million people (Bardach et al., Citation2015). However, high concentration of dissolved As in surface water and groundwater have been reported also from other areas of Argentina, including the Andes range (Nieva et al., Citation2016, Murray et al., Citation2014), as well as in Cuyo (Gomez et al., Citation2019) and Patagonia (Sandali & Diez, Citation2004; Nievas et al., Citation2013; Ruggieri et al., Citation2012).
Current legislation has adopted the WHO guidelines of 10 µg L−1, instead of 50 µg L−1 initially indicated by the Argentine Food Code (Código Alimentario Argentino, Citation2012). Nevertheless, a grace period of 5 years was established in 2007 and an addendum was granted in 2012 until the completion of epidemiological studies to be performed by governmental authorities (Secretaría de Políticas, Regulación e Institutos y Secretaría de Agricultura, Ganadería y Pesca, Código Alimentario Argentino, Resolución Conjunta 34/2012 y 50/2012, Citation2012). It must be stated that the national limit is not applied to all the provinces, but they regulate the permissible As concentration by their own norms. High As concentrations in natural waters represent an important issue not only for drinking water, but also for biotransference from As-contaminated water to food chain (Pérez Carrera et al., Citation2015). Recent developments regarding As origin, mobility and transfer to human environments in Argentina have been summarized in Table SM4 (Supplementary material), in which the main topics and findings are listed together with the regions under research. The studies were divided into six regions: (1) Pampean Plain; (2) Chaco Plain; (3) Cuyo, (4) NW region and Puna, (5) Patagonian Andes; and, (6) Patagonia. In these regions are mapped according to (1) the new areas not already included in Bundschuh, Litter, et al. (Citation2012); (2) new findings within areas already reported; and, (3) areas previously reported with no significant findings since then. Several other works addressing different topics, such as development of As remediation technologies and As impacts on human health, have also been published during the past 10 years. Since they are beyond the scope of this work, the reader is encouraged to look at some of the very comprehensive reviews already published (Litter et al., Citation2019b; Kumar et al., Citation2019).
4.10.1. Arsenic in water
4.10.1.1. Arsenic in groundwater
After releasing from soils and sediments (see subsection 4.10.2), As may adopt different chemical forms that depend on the water chemistry. In natural hydrological systems, As exists most commonly as arsenate [As(V), e.g., and
] and arsenite [As(III), e.g., and
]. Due to the oxidizing conditions that usually prevail in the Chaco-Pampean region, and to the slightly to moderately alkaline conditions, As is commonly found as
and
(e.g., Borzi et al., Citation2015; Nicolli et al., Citation2010; Díaz et al., Citation2016 and many others). However, some new works indicate the presence of As(III) in solution in proportions larger than previously thought. Watts et al. (Citation2010), after collecting groundwater and surface water in Ingeniero Luiggi, La Pampa Province, determined that a significant proportion of total As (mean: 46.8%; max: 78%) was present as As(III). Similar results were obtained by Sierra et al. (Citation2018) and Sierra (Citation2019) in the Claromecó fluvial basin in Buenos Aires Province, where As(III) reached a maximum of 60% of the total As in some samples. In groundwaters from Santiago del Estero and La Pampa, measured As(III) reached 70% of the total, although As(V) was the dominant form in most cases (Bejarano-Sifuentes & Nordberg, Citation2003; Bhattacharya et al., Citation2006; Raychowdhury et al., Citation2014; Coomar et al., Citation2019; Aullón Alcaine et al., Citation2020). Ward et al. (Citation2014) also found high As(III) concentrations in groundwater from different provinces in Argentina. Gomez et al. (Citation2014) measured As(III) concentrations in Mendoza, probably coming from deep reducing aquifers. These findings bring to light the importance of measuring As speciation, since its toxicity, mobility and absorptivity is dependent upon its chemical form. Hall et al. (Citation1999) indicated the importance of separating As species in-field (e.g., using resin-filled cartridges), because rapid changes in speciation may occur after sampling and during transport to labs, thus rendering questionable the results.
There is a general consensus that a wide variability in As concentrations exists in aquifers from the Chaco-Pampean Plain even within very short distances, both laterally and in depth (Nicolli et al., Citation2005; Bhattacharya et al., Citation2006; Aullón Alcaine et al., Citation2020; Blanco et al., Citation2006, Citation2012; Sierra et al., Citation2016, Citation2018). This fact strongly suggests the interaction of many local factors, such as geomorphology and surface relief, soil type, texture and mineralogy, depositional environment, residence time, hydrothermal and anthropic activity, etc. (Blanco et al., Citation2012; Nicolli et al., Citation2011; Raychowdhury et al., Citation2014; Sracek et al., Citation2009), which determines the origin, mobility and distribution of As. Regarding the depositional and postdepositional processes, Sosa et al. (Citation2019) have identified different amounts of As in sediments that depend on their age. When studying the deposits in the Claromecó fluvial basin, Buenos Aires Province, they found that Quaternary sediments are more enriched in As than Neogene ones (12.8 ± 9.3 and 5.0 ± 2.1 mg kg−1, respectively). Moreover, the pedogenetic units and hydromorphic paleosols and soils contained 23.9 ± 5.1 and 14.5 ± 11.5 mg kg−1 of total As, which were significantly higher than other fluvio-eolic Quaternary units. These findings were related to the environmental conditions, where the ancient position of water table and hydraulic gradients are relevant factors that affected such contents (i.e., higher groundwater levels correspond to large hydraulic gradient and therefore shorter residence time). In the same area, Sierra et al. (Citation2018) analyzed As concentrations in groundwater in relation to geomorphic units where boreholes were located. They observed that groundwater quality was different between the Neogene caltretized hills and the quaternary fluvio-eolic floodplain. In the hills, groundwaters showed lower electrical conductivity and lower As contents, when compared to the floodplain, where groundwater presented higher electrical conductivities and higher As contents. They considered the first case as a groundwater recharge zone and the second as a discharge zone, where likely mixing of regional flux occurs. Something similar was described by Díaz et al. (Citation2016) in the southern Pampean Plain. Moreover, these areas are characterized by flatlands and soft positive relief in which hydraulic gradients are relatively low (< 6%). In this context, vertical infiltration of rainwater and evapotranspiration are the dominant water movements, which may enhance water-sediment interactions (Fiorentino et al., Citation2007; Borzi et al., Citation2015).
Among all the hydrogeochemical factors that may exert some control on As mobility, only few appear to be responsible for the high As concentrations in groundwaters from Chaco-Pampean region. Since the main source has been attributed to volcanic glasses, dissolution may provide the primary As to groundwater (Nicolli et al., Citation2012). However, many other minerals (e.g., mafic minerals; see subsection 4.10.2) are also potential sources in current environmental conditions (Blanco et al., Citation2012; Vital et al., Citation2018). Dissolution of silicates in loess and volcanic glasses is favored under oxidizing conditions by alkaline pH, which originates mainly in the recharge zones and is controlled by carbonate dissolution. Silicate dissolution (e.g., albite) may also release Na+, H4SiO4 and to the environment, which would also explain the usually good correlation between Na+ and As. Na is a common element that is part of the crystalline structure of the calcosodic plagioclases, which are found in significant proportions in loessic sediments. Pedogenic processes favor silicate weathering controlling As availability to recharge water (Díaz et al., Citation2015, Citation2016).
Calcite equilibrium has also been proposed as a mechanism for As control (and other trace elements) in groundwater (Limbozzi, Citation2011; Vital et al., Citation2019). Both dissolution and co-precipitation are thermodynamically possible in these environments, as demonstrated by laboratory experiments. Since most groundwaters are usually over-saturated with respect to calcite (e.g., Zabala et al., Citation2016; Sierra et al., Citation2018; Gomez et al., Citation2019; Díaz et al., Citation2016), dissolution would be restricted to specific sites such as recharge areas, where acidic pH from rainwater may trigger this process. On the other hand, Ca sequestration by means of cation exchange reactions (Ca2+ by Na+) and/or fluorite precipitation are processes that may induce calcite dissolution, as well. Cation exchange coupled to calcite dissolution increases pH, thus contributing to desorption of oxyanionic species of As. Therefore, in order to determine to what extent both calcite dissolution and precipitation develop in this region, further data and research are still required.
After dissolution from primary source (see subsection 4.10.2), whatever mechanisms had occurred, As may re-adsorb as an oxyanion onto Fe-Mn-Al oxides (in order of adsorption capacity). However, under the hydrochemical conditions usually found in most groundwaters in the Chaco-Pampean Plain, desorption from the surface of such minerals is especially favored. At a slightly alkaline pH (7.0–7.5), Fe-oxides surfaces (and even more, Al and Mn oxides) begin to be negatively charged (zero point of charge for goethite is 7.5; Cristiano et al., Citation2011) and oxyanions such as
are repelled. Moreover,
is known to be a good competitor for adsorption sites (Appelo et al., Citation2002) and it is likely to be the main one since it is present always in high concentration. These facts explain the positive correlation usually found in groundwaters for pH and
with As (Nicolli et al., Citation2012; Bundschuh, Litter, et al., Citation2012; Borzi et al., Citation2015, Zabala et al., Citation2016). High silica in groundwaters (> 60 mg L−1 and even more), which is likely to be at or near saturation, also contributes to As desorption from oxides (Su & Puls, Citation2001; Scanlon et al., Citation2009). Oxyanion formed by trace elements could also compete for adsorption sites, but due to their minor concentrations, the effect is expected to be of lower impact on As release. Finally, the presence of
(related to human activities may be also an important factor that promotes As desorption). In short, regardless the competing ion, desorption from Fe oxides is a key process in controlling As contents in groundwaters.
Another less studied process is the counterion effect (Scanlon et al., Citation2009), which has been demonstrated in laboratory. It consists in the change of a Ca-rich to a Na-rich water that may trigger the desorption of As from metal oxides (Masue et al., Citation2007). Higher ionic strength decreases outer sphere surface complexation, causing As to be mobilized from oxides. Raychowdhury et al. (Citation2014) have explained the good positive correlation between Na/Ca0.5 and As a result of this effect in groundwaters from Mendoza. This has been validated by the means of hydrogeochemical modeling. Evapotranspiration is a process that may increase As concentration but also is a key process in favoring high ionic strength in groundwaters (Nicolli et al., Citation2012). Evaporation increases pH and salinity in groundwater, facilitating the dissolution of volcanic glass shards and consequently increasing mobility of As and other trace elements (Giménez et al., Citation2013). This process is more effective in shallow aquifers of the central and the northern Chaco-Pampean Plain where climate is more arid and semiarid (Nicolli et al., Citation2010).
4.10.1.2. Arsenic in surface water
Arsenic, in general, has been related to groundwater since larger residence time favors the interaction between solid phases and water, and therefore, the occurrence of the already mentioned hydrogeochemical processes. Recently, some efforts have been focused on the study of As concentrations in surface waters in several parts of Argentina. In the Pampean Plain, dissolved As concentrations in surface waters are generally under 50 µg L−1 and thus, comparatively less affected than respective groundwaters (Avigliano et al., Citation2015; Perez Carrera and Fernandez Cirelli, Citation2013; Othax et al., Citation2013; D’Urso et al., Citation2013; Bécher-Quinodóz et al., Citation2015; Sosa et al., Citation2019). Nevertheless, in Pergamino Creek and Chasicó Lake (Buenos Aires Province) As concentrations in surface water are higher than in groundwater (Reynoso & Andriulo, Citation2013; Puntoriero et al., Citation2014; Volpedo et al., Citation2013). In this case, evaporation could be a significant process affecting the water quality. Closer to the Andes range, surface waters may be affected by geothermal activity, which is characterized also by low pH (from 0.82 to 6.38) and Eh (from 25 to 542 mV), and As concentration that reaches 3783 µg L−1 (Farnfield et al., Citation2012). In Patagonia, monitored surface waters in proximities of a uranium mine district revealed As concentrations between 8 and 53 µg L−1 (Nievas et al., Citation2013).
Exposure of freshwater biota to As-rich water may be relevant for fisheries and, more in general, for the diary intake of many people. One of these works is the one by Rodriguez Castro et al. (Citation2015), which shows that in the Buenos Aires Province 32 streams exceeded the arsenic recommended guidelines for human consumption of 10 µg L−1 and six exceeded recommended values for aquatic organisms’ protection of 50 µg L−1. Rosso et al. (Citation2013) found that, on average, fish from the Pampa Plain showed higher (1.76 mg kg−1) As in muscle than the upper limit suggested for several freshwater fish species (Donohue and Abernathy, Citation1999). Moreover, in the arsenic-rich ecosystems of the Pampa Plain, some species (e.g., Odontesthes bonariensis and Bryconamericus iheringii) showed low concentrations of As and negative and low correlations coefficients with abiotic compartments, suggesting that these species may be able to regulate the level of As in muscle. Conversely, the concentration of As in other species like the scale-less silurid Rhamdia quelen closely followed the level of As in water and sediments. In this case, the intimate interaction between this large catfish and sediments may help to explain this pattern.
Avigliano et al. (Citation2015) summarized that the levels of As in fish species from different zones of Argentina are below the recommended maximum levels established by the Argentinean Food Codex (1 mg kg−1 on wet weight basis) and the US Environmental Protection Agency (1.3 mg kg−1 for As, on wet weight basis). However, according to the European Food Safety Authority (EFSA, Citation2010), the lower limit on the benchmark dose for a 10% response (BMDL) values associated with health risk for As ranged from 4.9 to 24 µg kg−1 of body weight per week. Considering these values, a 60 kg person must consume more than 0.38–1.9 kg of silverside muscle (with an As average concentration of 0.76 mg kg−1 wet weight basis) per week to have health problems. In Argentina, the average fish consumption rate is 0.1–0.2 kg per week (FAO, Citation2012). However, the consumption rate is much lower than that calculated for other trace elements (e.g., Pb) from de La Plata River. This suggests that lagoon-side and riverside communities, that may consume greater amounts of fish, are exposed to higher risk of chronic exposure to As (Avigliano et al., Citation2015).
4.10.2. Arsenic in soils and sediments
The primary As source has been commonly ascribed to volcanic-glass shards present in soils and sediments as well as in volcanic ash layers (Bundschuh et al., Citation2004; Bundschuh, Litter, et al., Citation2012; Nicolli et al., Citation2012). These materials are dispersed in the loess and loess-like sediments in percentages of 15% in the sand fraction (Bhattacharya et al., Citation2006; Sracek et al., Citation2009; Sosa et al., Citation2019) and more than 60% in the silt fraction (Teruggi, Citation1957), while in the volcanic ash layers can reach up to 95% (Bundschuh et al., Citation2004). Since the Neogene to present, these sediments were transported by wind and rivers from the Andes range all over the Chaco-Pampean Plain, giving it its particular uniform and flat morphology. In geological time span, As was released under favorable conditions and later precipitated or adsorbed on Fe, Al, Mn oxyhydroxides from where desorption could have occurred as a fast process, according to changing geochemical conditions in time and space (Bhattacharya et al., Citation2006; Bundschuh et al., Citation2011; Bundschuh, Litter, et al., Citation2012).
Some advances regarding As sources have been attained in the last years. Bia et al. (Citation2015), after studying modern volcanic ashes by means of X-ray photoelectron spectroscopy (XPS), have recently showed that there is an important enrichment of As in ash surfaces. After deconvolution of XPS spectra, authors interpreted that As may be in two forms: As(III) as replacing Fe(II) in arsenian pyrite and As(V), (interpreted as arsenate), which is present as adsorbed and/or poorly crystalline scorodite-like phase (Fe(III)AsO4·2H2O) formed by oxidative dissolution of As-bearing sulfides. The main proportion of As is within the more stable silicate structure of the volcanic glasses. They also performed kinetics experiments, where they showed that a rapid release of As occurs in the first 90 minutes, followed afterwards by a much slower dissolution. Although these authors analyzed modern volcanic ashes for these experiments, they speculated that this mechanism may be the one responsible for releasing As from the ancient ashes present in loess and loess-like sediments. After deposition, the contact with surface run-off and infiltration water could trigger this initial mechanism of relatively rapid As release. In contrast, the dissolution of the glassy fraction is expected to be slower and occur under more extreme conditions, such as acidic or alkaline pH.
Subsequently, and in the same line, Bia et al. (Citation2017) explored the speciation in different ash samples using X-ray adsorption spectroscopy (XAS). Their spectra interpretation showed that As is in the form of hydroxide species (As(OH)3), together with deprotonated species (), within the aluminosilicate structure of modern volcanic ashes. Despite this, while applying this technique to ancient ashes (∼125 ky), authors found that an additional minor proportion appeared in the form of As(V), but coordinated in its second shell by a cation, likely Si, Al or Na. This arrangement suggests a coordination of As(V) within the Al-Si network. This finding suggests that oxidation would begin within the glass lattice. An advanced weathering would lead to the release of As (either As(III) and As(V)) atoms from within this structure.
Ash dissolution mechanisms were investigated by Ruggieri et al. (Citation2012), who performed some lixiviation studies by batch and column experiments, using ashes from the Chaiten Volcano obtained after its 2008 eruption. Dissolution kinetics was explained by the occurrence of different mechanisms and the composition of ash particles, which agreed with the findings of earlier works (Oelkers & Gislason, Citation2001; Oelkers et al., Citation2009). The dissolution of silica, regardless of its grade of crystallinity, begins with the water absorption near a Si–O–Si group and is followed by the formation of a new Si–O bond with oxygen of the adsorbed water and the cleavage of the Si–O–Si group. The silicate glass dissolution becomes still more complex by the presence of other silicate like alkali feldspar, the dissolution of which develops in three steps, including alkaline ions exchange, generation of a residual layer rich in Si and a slower stage where feldspars interact with solution (incongruent dissolution).
Other authors, however, state that As does not originate exclusively from volcanic material. Blanco et al. (Citation2006, Citation2012; and references therein) have found high As concentrations in several accessory minerals that compose the different fractions of loessic sediments. Moreover, there are also differences between the light and heavy sand sub-fractions in the studied soils, which showed that As contents are similar and even higher than those found in volcanic glass (8.8–29.0 mg kg−1 in the light and 15.0–26.0 mg kg−1 in the heavy fraction). Vital et al. (Citation2018) have also showed the importance of accessory minerals in controlling the chemistry of groundwater. These minerals are rarely detectable by XRD and therefore, other techniques, such as scanning electron microscopy (SEM) and energy dispersive X-ray spectroscopy (EDS), must be used to deduce the presence of minor mineral constituents. In this line of research, Dietrich et al. (Citation2016) and Cacciabue et al. (Citation2016) have performed sequential extraction schemes over different loessic samples, which were specifically designed for loessic sediments. In agreement with Bia et al. (Citation2017), approximately 50% of As resides in silicates (no differentiation between glass and other silicate minerals can be done by this methodology). However, the other 50% of the As is hosted in more labile fractions, such as carbonates, oxy-hydroxides (low and high crystallinity) and adsorbed onto different mineral phases. Limbozzi (Citation2011), after studying caliche layers (locally known as “tosca”) from soils within the southern Pampa region, revealed that carbonates may scavenge important quantities of As. Therefore, this material may turn into As sink or source depending on current hydrogeochemical conditions. In this sense, Vital et al. (Citation2019) performed some flow-through experiments to assess the specific role of calcite in controlling the mobility of As and other trace elements in Pampean aquifer. They found that As and those trace elements (F, V, Sr and Ba) have a strong correlation with Ca, suggesting that they are released to the solution as a result of calcite dissolution. Something similar was demonstrated by Puccia et al. (Citation2015), after analyzing pore waters from the unsaturated zone, later validated by hydrogeochemical modeling.
Fluorapatite is another mineral that has been considered as a potential source of As. García et al. (Citation2014) performed some batch kinetic experiments, and proposed that under slightly acidic conditions, fluorapatite may dissolve and release F and also As simultaneously, which is known to be held within this mineral structure. These mechanisms would explain, at least in part, the usually found good correlation between As and F in groundwaters and surface waters.
Much knowledge has been gained in the last ten years regarding As sources, processes of mobility and characterization of water chemistry. Still, and in the opinion of these authors, much more research is needed to overcome the apparently “anarchic” pattern usually observed especially in groundwater, where As behavior is difficult to be predicted. This particular complexity, which is characteristic of Chaco-Pampean Plain, renders groundwater exploration an always challenging task.
4.11. The Guarani Aquifer System (Uruguay, Argentina, Paraguay, Brazil)
The Guarani Aquifer System (GAS) is one of the largest underground reservoirs in the world, with about 46,280 km3 of water (Sindico et al., Citation2018). It is located in the eastern and south-central portion of South America, under the territories of Uruguay, Argentina, Paraguay and Brazil and has an estimated total surface area of 1,195,000 km2. Almost 70% of this total is under Brazilian territory, in the states of Mato Grosso do Sul (213,700 km2); Rio Grande do Sul (157,600 km2), Sao Paulo (155,800 km2), Paraná (131,300 km2), Goiás (55,000 km2), Minas Gerais (51,300 km2), Santa Catarina (49,200 km2) and Mato Grosso (26,400 km2) (Wendland et al., Citation2014; Sindico et al., Citation2018). The groundwater chemistry evolves from neutral pH, Ca-HCO3 type in recharge area toward high pH, Na-HCO3 type in deep confined zone as a consequence of cation exchange and dissolution of carbonates (Sracek & Hirata, Citation2002; Hirata et al., Citation2011).
The current exploitation of its water sources surpasses 1.0 km3 year−1, 93.6% in Brazil (of which about 80% are in the State of São Paulo), 2.8% in Uruguay, 2.3% in Paraguay and 1.3% in Argentina (Sindico et al., Citation2018). Despite the generally good quality of GAS waters, anomalous concentrations of As (>50 µg L−1) have been described, such as those recognized by Fariña et al. (Citation2007) in wells that capture waters of hydrogeological units underlying the basalts, in the localities of Toro Blanco, San Juan de Nepomuceno and Coronel Bogado, in Paraguay. The authors attribute a natural origin to these occurrences, possibly associated to the reduction of As-rich goethite present in the sandstones. In the NW of Uruguay between the border of Uruguay and Argentina along the Uruguay River thermal corridor, high As concentrations (from <10 to 85 µg L−1) have also been reported in water samples (LAVELIN, Citation2008). In this area, there is an intense economic thermal tourism activity. Thousands of tourists (670,000 tourists in 2017 (35% more than 2016) and 6.9% in January 2019 (in comparison to January 2018); El País, Citation2019) visit the thermal resources every year (e.g., Almirón, Arapey, Daimán, Guaviyú, Salto Grande, San Nicanor). The origin of balneology in the region is associated to oil exploration efforts that began more than 60 years ago (Pesce, Citation2002). The types of these thermal waters range from Ca-HCO3 to Na-Cl types with increased presence of sulfates.
Bonotto and Elliot (Citation2017) carried out an investigation on the GAS with a transect in São Paulo State, Brazil, which involving analysis of trace elements, REEs and stable isotopes (B, Sr) in rainwater and groundwater samples. They found As concentrations exceeding the 10 µg L−1 limit for drinking water in one groundwater sample in Paraguaçu Paulista (19.62 µg L−1). However, this water is not used for human consumption, only for recreational purposes (thermal pools) (Bonotto & Elliot, Citation2017).
The concentrations of As are positively correlated with those of Cr and U, which corroborates the hypothesis that As desorption processes, from Fe oxides/hydroxides, in environments with high pH, are responsible for the release of these elements into groundwater along the Uruguay River thermal corridor (Argentina-Brazil-Uruguay), more specifically, Buena Vista and Sanga do Cabral formations (Gastmans et al., Citation2010). The source for As is associated with the intense magmatic activity that occurred at the end of Permian and early Triassic, which contributed with significant amounts of volcanic material, especially volcanic ash, recognized in the units underlying the GAS, which are frequently associated with anomalous concentrations of As. Calarge et al. (Citation2006) described occurrences of bentonite layers, the origin of which is associated with the deposition of volcanic ash in rocks of the Sanga do Cabral formations in Rio Grande do Sul, and Yaguari in Uruguay. According to these authors, alteration processes associated with the deposition of these ashes in lagoon environments generated bentonite deposits. In one of these occurrences, in Bañado de Medina, the As concentrations range from 7.5 to 30 mg kg−1. In addition, the occurrence of Fe oxides/hydroxides coatings observed in the neopaleozoic units (Buena Vista and Sanga do Cabral formations), covering the sandstone framework grains, in a quantity higher than that observed in the sandstones belonging to the GAS, suggests that these units are the likely source for the As found in groundwater along the Uruguay River thermal corridor (Argentine-Brazil-Uruguay) (Stollenwerk, Citation2003; Gastmans et al., Citation2010; Kirchheim et al., Citation2019). The occurrence of volcanic ash in the adjacent units that make up the GAS; the lithic composition of the rock forming grains of the aquifer system and the widespread occurrence of coatings of iron oxides covering the sandstone grains of Sanga do Cabral (BUV, in Brazil) and Buena Vista (Argentina and Uruguay) formations indicate that the main source of As present in the groundwaters along the Uruguay River thermal corridor is related to these units (Gastmans et al., Citation2010).
Thus, although the use of water in the region is essentially thermal (recreation/tourism), and there is no definition of safe limits of concentrations of As to which users can be exposed in this scenario, in the elaboration of projects of new perforations in the area should take into account the real possibility of occurrence of As concentrations above acceptable limits in Buena Vista or Sanga do Cabral formations (Smedley & Kinniburgh, Citation2002; Gastmans, Chang, et al., Citation2010). Research of the impact of As and other contaminants on human health has to be considered in further studies, especially due to the rapid expansion and popularity of the thermal water resources that are attracting thousands of tourists every year.
5. Caribbean region
The Greater Antilles host some of the world’s most important deposits of bauxite and lateritic nickel as well as gold, silver, copper, zinc, manganese, cobalt and chromium resources (Nelson et al., Citation2011). In this region there are serious water contamination problems, most of them are originated from mining and related activities and purely anthropogenic industrial activities, including agriculture. However, sedimentation, salt water intrusion into coastal aquifers, as well as remobilization of sediments through flooding, landslide and droughts may impact the water resources and put the health of the population at risk. Arsenic data in sediments, surface and groundwater are poorly documented. However, a short description about the Dominican Republic, Haiti and Cuba is discussed in this study.
5.1. Dominican Republic
5.1.1. Arsenic in mining areas
Mining of high-sulfidation epithermal deposits in Pueblo Viejo (central Dominican Republic) has caused serious environmental problems due to the formation of acid mine drainage associated with the oxidative dissolution of sulfides and sulfosalts (Grandia et al., Citation2009). Historical mining activity polluted the majority of the surface water sources in the Pueblo Viejo area (Tobey, Citation2004). However, in the present, the water from the mine has been treated and requires complying with the quality standards established by the Dominican Republic government and the World Bank (Cardenas et al., Citation2018). This treated water is discharged to the Margajita spring, which runs through the Pueblo Viejo mining concession, and drains into the Yuna River, upstream from the Hatillo freshwater reservoir, used for drinking water supply. Another watercourse that also passes through the mining concession area is the Maguaca River, which joins Yuna River below the Hatillo reservoir. Alarcón et al. (Citation2012) reported As values of 13–690 µg L−1 and <1–100 µg L−1 in surface waters from Margajita spring and the Magauca River, respectively; both are associated with volcanic rocks. The Yuna River, is one of the largest of the Hispaniola and is part of the Yuna watershed, which has an area of about 5498 km2 and supplies water to the Hatillo, Blanco, Arroyón, Tireito, Yuboa, Rincón, Guaygüí dams and dikes that divert water to aqueducts and irrigation channels for agriculture fields (Tobey, Citation2004). The Hatillo dam is one of the Caribbean’s largest fresh-water lakes, located southwest of Cotuí in Sánchez Ramírez Province (central Dominican Republic). Pueblo Viejo is hosted by the Lower Cretaceous Los Ranchos volcanoclastic rocks that extend across the eastern half of the Dominican Republic (Cardenas et al., Citation2018). According to Cardenas et al. (Citation2018), the mineralization in the area is mainly pyrite, with lesser amounts of sphalerite and enargite. The highest gold concentration in the studied area is associated with microcrystalline pyrite, which is also the mineral with the highest As content. This type of pyrite is the most prone to oxidation and the most difficult to liberate, as it forms complex intergrowths within the rock and with sphalerite. The same authors found that As content ranged from 260 to 1650 mg kg−1, where most of the As was dissolved and precipitated during pressure oxidation (POX) test. Tailings and waste rock from the mine area are deposited in the el Llagal valley, where a tributary of the Maguaca River flows.
Another important ore deposit in the region is the nickel laterite at the Falcondo mining area (central Dominican Republic) developed over Loma Caribe peridotite, which is divided in two major metavolcanic units: the Maimón Formation (NE) of Lower Cretaceous age, and the Duarte Complex (SW) of Late Jurassic or Early Cretaceous age (Lewis et al., Citation2002). Aiglsperger (Citation2015, Citation2016) found platinum group elements (PGE) in one of the deposits of the Falcondo mining area, the Loma Peguera Ni laterites, where As in limonite grains varied between 0.20 and 0.62 wt% (about 2000-6200 mg kg−1) and in laurite grains was 0.01 wt%; (about 100 mg kg−1) as measured by means of electron microprobe analyzer (EMPA). Arsenic content in sphalerite was 0.09 wt.% (about 900 mg kg−1) and in tennantite 16.1–18.5 wt.% (about 1600–1801 mg kg−1) using EMPA has also been reported in a mineralogical and geochemical study in Cerro de Maimón (central Dominican Republic; Torró et al., Citation2016). In the same area of the Cu–Zn deposit of Cerro de Maimón, Andreu et al. (Citation2010) reported As concentrations of 5.2% (using EMPA) in Ag bearing minerals mixed together with barite cemented by colloform goethite, their location and shape suggesting late remobilization. This ore deposit is located within the Malmón Formation, which hosts most of the massive sulfide occurrences in the Dominican Republic (Andreu et al., Citation2015).
5.1.2. Arsenic from other sources
Arsenic concentrations between 1.6 and 103.0 mg L−1 (for 164 samples) in sediments and soils have also been reported in a study carried out by Zorrilla Fernández (Citation2017) in one the biggest landfills of the Dominican Republic, La Duquesa, located in the Santo Domingo Norte Municipality, 15 km northeast of Santo Domingo City. High concentrations of trace metals and metalloids (Ag, Al, As, Au, Ba, Be, Bi, Br, Cd) have contaminated the soil and tributaries near the landfill area during the past 20 years, affecting several communities (Municipio Santo Domingo Norte, Guaricano, Los Casabes, Higuero Abajo, Duquesa, Higuerito, Jucagua). According to INSAPROMA (Citation2014), 83% of the landfills in Dominican Republic are placed in areas where aquifers are located and 75% are close to surface waters (<1000 m), in a violation to the Environmental Management Plan for Non-Hazardous Solid Waste. For example, the nearest watercourse from the Duquesa landfill is the Isabela River, a tributary of the most important river of Santo Domingo, the Ozama River, which flows into the Caribbean Sea carrying in its way several toxic contaminants (Zorrilla Fernández, Citation2017).
5.2. Haiti
5.2.1. Arsenic in water
Water resources in Haiti are inadequately distributed and poorly managed with regard to their critical role in establishing long-term water and food security (Adamson et al., Citation2017). According to Widmer et al. (Citation2018), water quality has been degraded because of social and demographic changes over time; 62% of urban and 34% of rural residents have access to distributed water (within a radius of 500 m around a water point). Water treatment practices are not effective and nearly 32% (3 out of 10 households) drinking water is untreated. In some communities, saline contamination in urban aquifers, e.g., the Plaine du Cul-de-Zac aquifer (southeastern Haiti) is of great environmental concern. This is one of the most important and largest aquifers of Haiti, supplying a population of over two million, as well as, to the agricultural, industrial and commercial sectors (Adamson et al., Citation2017). In an evaluation of the aquifer, Adamson et al. (Citation2017) reported As concentrations from <10 to 25 µg L−1. The highest value (25 µg L−1) corresponds to a site (Sibert 7) sampled in October 2016, for which values of other parameters are: total alkalinity 380 mg L−1, electrical conductivity 690 µS cm−1, Cl 20.8 mg L−1, F 0.85 mg L−1. Arsenic values partly exceed the WHO guideline value for drinking water (10 µg L−1), so part of the population receiving water from this aquifer may be at risk. Salt water intrusions into the aquifer may be a concern due to overexploitation, and if deeper wells are drilled, tapped rock formations may contain ancient brackish groundwater that can have elevated As concentrations (Widmer et al., Citation2018). However, more research is needed in order to explain the high contents of As in water found during the year 2016 by Adamson et al. (Citation2017). In the brackish water from the Lake of Étang Saumâtre (i.e., Lac Azuei, Lago de Fondo, or Yanagua, eastern Haiti), Eisen-Cuadra et al. (Citation2013) reported average concentration of five trace metals, Cr 70.11 mg kg−1, As 0.88 mg kg−1, Cu 10.65 mg kg−1, Pb 2.02 mg kg−1 and Zn 9.03 mg kg−1 in core sections from lake sediments. These results showed that the highest concentrations occur toward the top of the sediment core (more recently), suggesting an increase in depositional flux in recent years, whereas lower concentrations and greater stability occur toward the bottom of the core. In some groundwater wells (6 sites) surrounding the Étang Saumâtre Lake, As concentrations varied from 280 to 8660 µg L−1 (T: 24.9–29.3, pH 7.2–8.5, TDS 8.4-536 mg L−1; Hannigan, pers. commun., 2019). The Étang Saumâtre Lake is used for drinking and irrigation water supply and for fishing, the last constituting a potential risk for metal-induced health effects for the inhabitants of the communities of Thomazeau, Ganthier and Fond Parisien (Eisen-Cuadra et al., Citation2013).
Arsenic of geogenic origin is reported from surface water (As 1030 µg L−1, pH 7.6, TDS 392 mg L−1, EC 387.0 µS cm−1) from Trou du Nord River and groundwater (As 1110 µg L−1, pH 8.1, TDS 262.7 mg L−1, EC 551.0 µS cm−1) in the village of Fleury, Caracol commune (northeastern Haiti; Findley & Côte, Citation2011; ENVIRON, Citation2011). In the same survey, Findley and Côte (Citation2011) found high amounts of Ba (4.0 mg L−1) suggesting that water in Trou du Nord River and Fleury will require additional treatment before human consumption to reduce the level of both contaminants to WHO guideline levels. The village of Caracol and communal sections are fed with potable water with hand pumps and public water taps. Some people also use unprotected water sources, vulnerable to surface contamination, for household water supply. Furthermore, in the Caracol region, a 150 ha industrial park was developed in the lower portion of Trou de Nord River watershed, which hosts export-oriented garment manufacturing units and other businesses (Findley & Côte, Citation2011), which may further increase environmental impacts to the neighboring communities in the future, if the necessary environmental regulations are not complied. Distribution of metals from six different water sources has also been measured in Cap-Haitien by Rivera-Nuñez et al. (Citation2018). Cap-Haitien is the second largest city in the northern coast of Haiti, with a population of about 190,000. Arsenic concentrations were measured in public water supply lines (0.16–57.81 µg L−1, 31 samples), private and communal wells (1.01–5.4 µg L−1, 21 samples), water kiosks (0.20–1.89 µg L−1, 19 samples), water sachets (0.02–0.09 µg L−1, 9 samples), water reservoirs (0.21-9.29 µg L−1, 6 samples) and water drums or concrete (0.43–0.84 µg L−1, 2 samples). The results showed that As concentration was above the WHO guideline and the US EPA drinking water standard (10 µg L−1) in a sampling location far away from the coast. Despite these preliminary data, it has to be mentioned that As and other contaminants data is sparse in the country, and therefore efforts are needed to monitor As exposure.
5.2.2. Arsenic in mining areas
Another important potential source of water contamination by trace metals and metalloids is mining which is mainly located along the Copper-Silver-Gold mineralization belt. However, the authors could not find any data of As contents in surface and groundwater in these mining sites.
5.3. Cuba
A review of the contamination of water resources by As, which is mostly caused by natural lixiviation of ore deposit or released anthropogenically through mining and industrial activities in Cuba was compiled by Bundschuh, Litter, et al. (Citation2012). The following information consists of an update, considering the works published after 2012. The natural occurrence of As and heavy metals in Cuban soils was described by Rodríguez Alfaro et al. (Citation2015), who found that the natural distribution of heavy metals and metalloids followed the following order: Fe > Mn > Ni > Cr > Ba > V>Zn > Cu > Pb > Co > As > Sb > Ag > Cd > Mo > Hg, where As concentration was about 20.3 mg kg−1, and the Quality Reference Values (QRVs) for As were 19.0 mg kg−1. The QRVs are based on the analysis of metal and metalloid content in soil with minimal anthropic interference (Bidone et al., Citation2014). However, the same authors found significant correlations between trace elements and Fe concentration in soils with different levels of Fe, where As concentration varied between 0.600 and 92.60 mg kg−1. High metal and metalloid concentrations in Cuban soils are greatly influenced by the geology of the island. However, the anomalous concentration of certain metals and metalloids, e.g., Cu, Ni, Ni, Sb, As in soils indicate the need for further research regarding their origin, bioavailability, bioaccessibility and risk for human health. A serious problem concerning food security in Cuba is that organic fertilizers and substrates used in urban agriculture and vegetables contain trace elements, including As, causing a high risk to human health. Therefore, concentrations of Cd, Pb, As, Se, Hg, Ni and Cr in composts and substrates were assessed in a study carried out during the first national-wide survey of trace elements in Cuban urban agriculture by Rodríguez Alfaro et al. (Citation2015). The results showed that Cd, Pb, As, Se, Hg, Ni and Cr in organic fertilizers used in Cuba were within permissible limits and do not constitute a risk to human health.
5.3.1. Arsenic in mining areas
Arsenic has been reported in the Cavo Moa Bay (NE of the Holguín Province, Cuba), where one of the largest global reserves of nickel and cobalt are located. This region has been impacted by human activity since the middle of the 20th century, causing great environmental deterioration due to mining and metallurgical activities (Cervantes-Guerra et al., Citation2011). The Ni laterites in the Moa Bay mining area have been developed from weathering of hydrated ultramafic rocks of the Moa-Baracoa ophiolitic massif (Aiglsperger, Citation2015). The highest As concentrations were found in the sediments at the mouth of Moa (153 mg kg−1) and Cayo Guam rivers (123 mg kg−1) rivers (Cervantes-Guerra et al., Citation2011). From the same area, Cervantes-Guerra et al. (Citation2017) reported As concentrations of 126 mg kg−1 and 106 mg kg−1 from sediments of Moa and Cayo Guam rivers, respectively. The high As concentrations decrease toward the southeast of the coastline. Furthermore, large quantities of As, Cu, Pb and Zn have been reported in spots, localized at the south of Cayo Moa Grande (Cervantes-Guerra et al., Citation2011). Land erosion around the Moa Bay has increased due to land use, causing transportation of more than 106 m³ year−1 of eroded sediments into the bay, especially during the periods of heavy rains (Fernández, Citation2009). Sediments obtained from the delta of Moa River have a black coloration, very similar to the industrial tailings deposited near the mouth of the river (Cervantes-Guerra et al., Citation2017). According to Cervantes-Guerra et al. (Citation2011, Citation2017), the degree of pollution of the Moa Bay sediments has the following order of geoaccumulation: Pb < Mn < Co < Cu < As < Zn < Ni < Fe < Cr < Al (from unpolluted to very strongly polluted), causing a high ecotoxicological risk to the marine environments.
Arsenic and heavy metals contamination in mine tailings and sediments has been reported in the surface- and groundwater of the Delita gold deposits (Juventud Island, southern Cuba). Delita is part of the metamorphic massif of the Juventud Island, which is represented by a Jurassic terrigenous-carbonate lithological complex, which has been metamorphosed to greenschists (Bortnikov et al., Citation1989; Toujaguez et al., Citation2013). This epithermal deposit was formed in three stages: an early quartz-arsenopyrite stage, a quartz-sphaleritic-sulfantimonite stage and a quartz-stibnite stage (Bortnikov et al., Citation1989). The Delita mineralizations have been exploited since the Colonial time (Toujague de la Rosa & Molerio León, Citation2013), so high As concentrations (up to 3.5%) and other contaminants have been reported in the weathered material from old mine tailings, which were used to fill the path to a beach located close to the Delita gold mine (Toujaguez et al., Citation2013). In the coastal area, high As concentrations up to 575 µg L−1 has been reported, sourced from arsenopyrite bearing mine tailings, which are transported by surface runoff toward the sea (Toujaguez et al., Citation2013). In some water wells (V-28, S-7, S-9) located in the Delita area, measured As concentrations of 11.6, 15.0 and 18.8 µg L−1 respectively exceeded the drinking water standard of 10 µg L−1, respectively (Bundschuh, Litter, et al., Citation2012). According to Toujaguez et al. (Citation2013), the total As concentrations in four mine tailings (R1, R2, R3 and R4) are 8650 ± 1021, 1666 ± 62.1, 35372 ± 4413 and 1085 ± 44.4 mg kg−1, respectively. The highest gastrointestinal As bioaccessibility was found in tailing R4 (438 ± 3.46 mg kg−1), which is followed by R3 (1750 ± 407 mg kg−1), indicating that these materials present the greatest risk associated with accidental ingestion, especially for children.
Contamination by acid mine drainage has also been reported in the Cu–Zn San Fernando mine and surrounding area (central Cuba) by Milián Rodríguez (Citation2012). This ore deposit was mined from its discovery in 1827 to its closure in 1961, and recent drilling proved that the mineralization is still open along strike (Torró et al., Citation2016) and, as a result several trace metals and metalloids are mobilized, leading to high concentrations of As in superficial waters (660–1900 µg L−1), as well as of other toxic contaminants (e.g., Cu, Zn, Cd, Hg) that caused pollution of water, sediments and soils from San Fernando mine until the Arimao River basin (southern San Fernando deposit), which recharges in the Avilés dam (Milián Rodríguez, Citation2012). The ore deposit at San Fernando occurs within the stratigraphically lowest unit of the Los Pasos Formation, where several volcanogenic massive sulfide deposits (VMS) are found. In a study of the mineralogy and geochemistry in VMS deposits from Cuba and the Dominican Republic, Torró et al. (Citation2016) reported the mean of the distribution of the concentration of As in sphalerite (0.06 wt.%, 600 mg kg−1), tennantite (16.3–18.9 wt.%, 163,000–189,000 mg kg−1), tetrahedrite (0.9–4.4 wt.%, 9000–44,000 mg kg−1) and arsenopyrite (36.8–43.4 wt.%, 368,000–434,000 mg kg−1) and other elements in the ore by means of electron-microprobe analysis. The water with As and other contaminants are used for fishing, agriculture and in the dry season for drinking water supply to the communities of Palmira, Potrerillo and the Cruces channel (Milián Rodríguez, Citation2012).
5.3.2. Arsenic from other sources
According to Alfonso-Hernández et al. (Citation2012), total As in marine food from Cienfuegos Bay (southern coast of Cuba) was determined in crustaceans (26.5 mg kg−1), mollusks (22.0 mg kg−1) and muscle tissues of certain species of fish (10.2 mg kg−1) in dry weight (d.w.), using an Energy Dispersive X-Ray Fluorescence (EDXRF) method. Arsenic content (0.010–4.820 mg kg−1 d.w.) in marine products was also reported in the west coast of Cienfuegos (Alfonso-Hernández et al., Citation2004). The concentration of As have increased 12 times during the last 30 years in the bay; the origin may be due to waste from the fertilizer industry in Cienfuegos (Alfonso-Hernández et al., Citation2012). Water mass in Cienfuegos Bay receives a fresh water contribution from Caunao, Arimao, Damují and Salado rivers, altering (reducing) the salinity and concentration of organic material and contaminants entering into the bay (Garcés et al., Citation2012). The Cienfuegos Bay is one of the major industrial sectors (e.g., fertilizer and biochemical factories, sugar mills, paper plant, oil refinery) in Cuba, and is extremely vulnerable to anthropogenic pollution. Another example of As in marine species (Clarias gariepinus) has been investigated by Lima et al. (Citation2013), who have reported As (0.010–0.110 mg kg−1), Hg and Se concentration in fish tissue of Clarias gariepinus from Sagua la Grande River (north of Villa Clara Province, central Cuba). Here, As is mainly presented as organic species that are much less toxic than inorganic As. However, Se sources in fish have to be investigated in the region, in order to determinate the sources and its exposure to humans.
6. Conclusions
This study collected, compiled and analyzed information on the occurrence and impacts of As exposure in Latin America from over 200 principal sites of diverse extents and relevance. In all the 20 Latin American countries, As was found to impact water sources and environment, thus exposing humans to a significant risk. Arsenic originates from different, mostly geogenic, and locally anthropogenic As sources, from which it is mobilized to different environments, including ground- and surface water sources, soils and sediments and biota. The impacts found in all of the Latin American countries, which comprise in number about 10% of all states in the world, discerns the high relevance of the Latin American As contamination scenario within the global As problem. However, analyses show that the As problem in the individual Latin American states is of diverse nature and scale. The most important geogenic As sources and mobilization mechanisms that lead to direct exposure through drinking water or indirect exposure via the human food chain are:
Volcanic rocks and products of volcanic emissions, such as volcanic ashes. Thereby, the principal areas from where As is mobilized into environmental systems are young volcanic rocks (Cenozoic to recent) found in the vicinity of active volcanoes (active volcanic zone along the Pacific coast, stretching from Mexico to the southernmost part of Argentina and Chile, including the Trans-Mexican Volcanic Belt); however, mobilized As also occurs in the volcanic rocks found in the areas of former volcanism (back-arc volcanoes). Water from aquifers (hardrock and sedimentary aquifers the latter comprising the weathering products) often show As concentrations exceeding the WHO guideline value of 10 µg L−1. Volcanic emissions, in particular volcanic ash containing As, which can be transported over long distances, covering 100’s or 1000’s of kilometers, are relevant. Since most of the ash is in the form of amorphous glass, it is highly water-soluble so that even low As contents – through accumulation – lead to high environmental As contamination. A well-known example is the Chaco-Pampean Plain (Argentina), where volcanic ash derived and accumulated due to longer periods of Andean volcanism (Cenozoic to recent) over millions of years is found dispersed or as distinct layers in loess-type sediments impacting (mostly shallow) groundwater with As. In addition, in the extension of the Argentine Chaco Plain into Bolivia and Paraguay, As has also been reported. This way of As exposure impacts populated areas and therefore constitutes a significant public health risk. In the rock formations underlying the Guarani aquifer system, which is an important regional water source and transboundary aquifer shared between Brazil, Paraguay, Uruguay and Argentina, volcanic ash (neopaleozoic) is considered a source for the locally elevated As and also F concentrations found in groundwater of this aquifer system.
Hydrothermal, sulfidic and other types of metallic mineral deposits constitute another important source from which As is released by natural processes, such as lixiviation and chemical weathering. However, its mobilization into the environment can be accelerated by several orders of magnitude through mining and related activities (ore processing, enrichment, refining, etc.). Since mining areas are often located in sparsely populated areas, their direct impact is often limited to workers (occupational hazard) and their families living around the mines. However, As can be transported through rivers over thousands of kilometers contaminating areas along their courses, where often most of the rural population lives – adversely impacting on drinking water supply and irrigated areas used for food production – and even reach and contaminate coastal areas, as it has been recognized in the cases of Brazil (Doce River), Peru (Rímac River) and Chile (Loa River).
Geothermal fluids (water/gases), which rise up from depth and mix with near-surface environments, including surface and groundwater sources. Especially the geothermal fluids from active volcanic areas have high As contents of several to several tens of mg/L (e.g., Los Humeros in central Mexico and El Tatio in the north of Chile).
Purely anthropogenic As sources, such as pesticides have been locally reported. For example, As from present and past pesticide applications have been reported in this review from Mexico, El Salvador and Venezuela. However, detailed studies are lacking and, in most cases As from anthropogenic sources are compounded with geogenic sources of contamination.
A combination of spatial and geostatistical methods such as inverse distance weighting interpolation (IDW) and local indicator of spatial association (LISA) are needed to understand the trends in the distribution of As and other associated elements in groundwater and soil ecosystems. Statistical hierarchical clustering analysis (HCA) and geospatial techniques such as the Moran index needs to be integrated together with conventional hydrogeochemical studies to determine the spatial autocorrelation and the localized index for understanding the patterns of spatial variability of As and other related trace elements in different groundwater system across the LA countries.
Additionally, risk assessment studies need to be carried out in the entire LA region to evaluate the exposure and assessments of the effect of As on human health. Exposure models should be developed through different methods, taking into account the exposure of As to humans identified to be ‘intake of water’ and ‘intake of As contaminated plants (specifically grains, and leafy vegetables), intake of meat and milk, intake of fish', but even other pathways that include intake of soil, inhalation of dust and dermal contact which might cause the most impact on human health.
The significance, and respective high relevance for governmental interactions to tackle the As problem is well-recognized since decades in countries such as Mexico, Chile, Argentina, but more recently also in Nicaragua. The problem is of similar importance in other Latin American countries like Peru, Colombia and Venezuela, but the lack of data and information hampers a thorough assessment of the problem. Data availability is the largest hurdle to overcome. In consequence, As must become a standard parameter for any analysis of water sample to provide a first, very basic assessment, starting with those water sources used for drinking and irrigation. Assessment of As sources, mobilization, environmental and human health impacts, and evaluation of detailed water quality analyses are therefore needed for application of appropriate remediation. Data on sediments and suspended matter in water is limited. However, As can be sorbed into these phases and transported with water flow. Prevention of As-related impacts is essential especially in mining and geothermal operations in particular, as well as in industrial and agricultural activities, which requires stringent regulations, monitoring and methods of compliance by the governments of the different Latin American countries. Assessment of the problem and its mitigation is undoubtedly a key challenge, which needs to be considered within the governments’ promises to meet the 2030 UN Sustainable Development Goals.
best_a_1770527_sm6606.pdf
Download PDF (1.7 MB)Acknowledgements
The authors want to thank the anonymous reviewers for their helpful comments, which improved the manuscript, as well as the support of different organizations. Lue-Meru Marco Parral: To the projects: FONACIT 2011000961. Registered at CDCHT as RAG-004-2012 and CDCHT-UCLA, 007-AG- 2015. J. Schneider (Brazil) (Project PNPD 88887.363100 / 2019-00) acknowledge Capes for providing postPhD scholarship. Marta Litter thanks the Agencia Nacional de Promoción Científica y Tecnológica (ANPCyT) from Argentina under PICT-2015-208, and BioCriticalMetals – ERAMIN 2015 grants. Sebastian Dietrich (Argentina) thanks the Agencia Nacional de Promoción Científica y Tecnológica (ANPCyT), Proyecto PICT 1805/2014. Valeria Delgado Quezada and Maximina Altamirano Espinoza thank PhD Bayardo González Rodríguez for some of the data provided for this article: 1) the study “Mecanismos de contaminacion de arsénico en comunidades rurales de Nicaragua. Caso de estudio: Muy Muy, Matagalpa”, where he had the collaboration of the Master’s student in Environmental Engineering from PIENSA-UNI, Ing. Eduardo Israel Velásquez Castillo to whom we thank his participation; and 2) for the study “The Arsenic Project. A Multidisciplinary Project in Nicaragua” carried out by Master´s students from TU Delft, with the collaboration from Brabant Water, Royal HaskoningDHV, ANA, Iv Groep, UNI and CISTA/UNAN-León.
References
- Abarca, T. , & Alvarado , G. E. (2017). Pérdidas económicas en valor presente al año 2016 causadas por el vulcanismo en Costa Rica entre 1953 y 2016. Revista Geológica de América Central, 56, 37–50. https://doi.org/10.15517/rgac.v0i56.29235
- Abreu, M. C. , & Figueiredo, B. R. (2014). Mapeamento geoquímico de arsênio e metais pesados em solo da unidade Piririca, Vale do Ribeira (SP) [Paper presentation]. In Proceedings of the 41th Brazilian Geological Congress, João Pessoa, Paraíba, Brazil.
- Adamson, J. K. , Miner, J. , & Rochat, P.-Y. (2017). Une évaluation de l’aquifère de la Plaine du Cul-de-Sac et de son potentiel pour desservir Canaan Rapport Final, Northwater International & Resodlo, S.A, 3–41.
- Adan, G. (2010). Evaluación fisicoquímica de la contaminación por descargas de aguas residuales en la sub-cuencas media y baja del Río Turbio. Specialization degree work. Instituto Universitario Experimental Tecnológico Andrés Eloy Blanco.
- Adan, G. E. , Marcó Parra, L. M. , Guédez, M. , Colmenarez, A. M. , Juana, A. , Torres, G. G. , Jesús Rojas, Y. S. , & Durán, R. (2014). Línea de tiempo de parámetros físico-químicos del agua del Río Rurbio para la gestión mediante el modelo ARCAL-RLA 010. Observador Del Conocimiento , 2 (6), 65–78.
- ADEC (2010). Assessment level for soil, sediment and water. Australian Department of Environment and Conservation. http://www.esdat.net/Environmental%20Standards/Australia/WA/Assessment%20Levels%20-%202010.pdf.
- Admiraal, M. , Couasnon, A. , Huijzenveld, T. , Hutten, R. , Schölvinck, O. , & van Veen, N. (2015). The Arsenic Project - A Multidisciplinary Project in Nicaragua . TU Delft.
- Agriquem (2009). Análisis químico de suelos de la ciudad de Arica. Diagnóstico de la calidad química del suelo y subsuelo urbano ciudad de Arica, para aplicar en caso necesario medidas de gestión y de regulación. Agriquem y Gobierno Regional de Arica y Parinacota.
- Aguilar, E. , Parra, M. , Cantillo, L. , & Gómez, A. (2000). Chronic arsenic toxicity in El Zapote - Nicaragua 1996. Medicina Cutánea Ibero-Latino-Americana , 28 (4), 168–173.
- Aguilera, F. , Honores, C. , Lemus, M. , Neira, H. , Pérez, Y. , & Rojas, J. (2014). Evaluación del recurso geotérmico de la Región de Los Lagos (Informe Registrado No. IR-14-57). SERNAGEOMIN, Santiago de Chile, Chile.
- Ahmad, A. , & Bhattacharya, P. (2019). Arsenic in drinking water: Is 10 μg/L a safe limit? Current Pollution Reports , 5 (1), 1–3. (https://doi.org/10.1007/s40726-019-0102-7
- Ahmad, A. , Cornelissen, E. , van de Wetering, S. , van Dijk, T. , van Genuchten, C. , Bundschuh, J. , van der Wal, A. , & Bhattacharya, P. (2018). Arsenite removal in groundwater treatment plants by sequential permanganate-ferric treatment. Journal of Water Process Engineering , 26 , 221–229. https://doi.org/10.1016/j.jwpe.2018.10.014
- Aiglsperger, T. H. (2015). Mineralogy and geochemistry of the platinum group elements (PGE), rare earth elements (REE) and scandium in nickel laterites [PhD Thesis]. Facultat de Geologia, Universitat de Barcelona.
- Aiglsperger, T. , Proenza, J. A. , Lewis, J. F. , Labrador, M. , Svojtka, M. , Rojas-Purón, A. , Longo, F. , & Ďurišová, J. (2016). Critical metals (REE, Sc, PGE) in Ni laterites from Cuba and the Dominican Republic. Ore Geology Reviews , 73 , 127–147. https://doi.org/10.1016/j.oregeorev.2015.10.010
- Akcil, A. , & Koldas, S. (2006). Acid mine drainage (AMD): Causes, treatment and case studies. Journal of Cleaner Production , 14 (12-13), 1139–1145. https://doi.org/10.1016/j.jclepro.2004.09.006
- Alarcón, M. T. , Llorens, E. , & Poch, M. (2012). Remoción de arsénico para consumo humano en Latinoamérica. Centro de Investigación en Materiales Avanzados, S.C, Chihuahua, Mexico.
- Alarcón-Herrera, M. T. , & Martín-Domínguez, A. (2018). Tecnologías y avances científicos. In L.M. Del Razo , J.M. Ledón , M.N. Velasco (Eds) Hacia el cumplimiento del derecho humano al agua. Arsénico y fluoruro en agua: Riesgos y perspectivas desde la Sociedad civil y la academia en México . Secretaría de Gobernación.
- Alarcón-Herrera, M. T. , Flores-Montenegro, I. , Romero-Navar, P. , Martín-Domínguez, I. R. , & Trejo-Vázquez, R. (2001). Contenido de arsénico en el agua potable del Valle del Guadiana, México. Ingeniería Hidráulica en México , XVI (4), 63–70.
- Alarcón-Herrera, M. T. , Bundschuh, J. , Nath, B. , Nicolli, H. , Gutiérrez, M. , Reyes-Gómez, V. M. , Nuñez, D. , Martin-Domínguez, I. R. , & Sracek, O. (2013). Co-occurrence of arsenic and fluoride in groundwater of semi-arid regions in Latin America: Genesis, mobility and remediation. Journal of Hazardous Materials , 262 , 960–969. https://doi.org/10.1016/j.jhazmat.2012.08.005
- Ale-Mauricio, D. A. , Villa, G. , Gastañaga, M. , & del, C. (2018). [Urinaryse arnic concentrations in adult population of two districts from the Tacna Region, Peru, 2017]. Revista Peruana de Medicina Experimental y Salud Publica , 35 (2), 183–189. https://doi.org/10.17843/rpmesp.2018.352.3693
- Alfaro de la Torre, C. , Deogracias Ortiz Pérez, M. , Alarcón, M. T. , Martínez Cruz, D. A. , & Ledón, J. M. (2018). Concentraciones de arsénico y fluoruro en agua subterránea. In L. M. Del Razo , J. M. Ledón , M. N. Velasco (Eds.), Hacia el cumplimiento del derecho humano al agua. Arsénico y fluoruro en agua: Riesgos y perspectivas desde la Sociedad civil y la academia en México (pp. 38–56). Secretaría de Gobernación.
- Alfonso-Hernández, C. M. , Pérez Santana, S. , Brunori, C. , Morabito, R. , Delfanti, R. , & Papucci, C. (2004). Historical trend in heavy metal pollution in the sediments of Cienfuegos Bay (Cuba), defined by 210Pb and 137Cs geochronology. International Conference on Isotopes in Environmental Studies. Aquatic Forum, IAEA-CN-118/170, 313–314.
- Alfonso-Hernández, C. M. , Gómez Batista, M. , & Díaz Asencio, M. (2012). Total arsenic in marine organisms from Cienfuegos Bay (Cuba). Food Chemistry , 130 (4), 973–976.
- Alloway, B. , Centeno, J. A. , Finkelman, R. B. , Fuge, R. , Lindh, U. , & Smedley, P. (2016). In O. Selinus (Ed.), Essentials of medical geology . Springer.
- Almagro, L. , & Custodio, E. (2004). Caracterización hidrogeoquímica de las aguas subterráneas de la Formación Chuy, en la franja costera del Este del Uruguay, fronteriza con Brasil. Boletín Geológico y Minero , 115 , 357–378.
- Alonso, D. L. & Castillo , E. (2014) Arsenic availability in a contaminated area of Vetas-California gold mining distrtrict - Santander, Colombia. In: One Century of the Discovery of Arsenicosis in Latin America (1914-2014) As2014: Proceedings of the 5th International Congress on Arsenic in the Environment, May 11-16, 2014, Buenos Aires, Argentina, 1:242–244.
- Alonso, D. L. , Latorre, S. , Castillo, E. , & Brandão, P. F. B. (2014). Environmental occurrence of arsenic in Colombia: A review. Environmental Pollution , 186 , 272–281. https://doi.org/10.1016/j.envpol.2013.12.009
- Alonso, D. L. , Pérez, R. , Okio, C. K. Y. A. , & Castillo, E. (2020). Assessment of mining activity on arsenic contamination in surface water and sediments in southwestern area of Santurbán paramo, Colombia. Journal of Environmental Management , 264 , 110478–110410. https://doi.org/10.1016/j.jenvman.2020.110478
- Alonso, R. N. , Jordan, T. E. , Tabbutt, K. T. , & Vandervoort, D. S. (1991). Giant evaporite belts of the Neogene central Andes. Geology , 19 (4), 401–404. https://doi.org/10.1130/0091-7613(1991)019<0401:GEBOTN>2.3.CO;2
- Altamirano Espinoza, M. (2005). Distribución de la contaminación natural por arsénico en el agua subterránea de la subcuenca suroeste del Valle de Sébaco-Matagalpa [MSc Thesis]. Universidad Nacional Autónoma de Nicaragua.
- Altamirano Espinoza, M. , & Bundschuh, J. (2009). Natural arsenic groundwater contamination of the sedimentary aquifers of southwestern Sébaco valley, Nicaragua. In J. Bundschuh , M. Armienta , P. Birkle , P. Bhattacharya , J. Matschullat , A. Mukherjee (Eds.), Natural Arsenic in Groundwaters of Latin America . CRC Press. pp. 109–122.
- ANA (2016). Encarte da ANA reúne informações sobre Rio Doce antes e depois do rompimento da barragem de Mariana, Autoridad Nacional del Agua (ANA), Brazil. http://www2.ana.gov.br/Paginas/imprensa/noticia.aspx?id_noticia=12964
- Andina (2018). Agua de pozos en Mórrope contiene arsénico 9 veces más de lo permitido. Andina (Agencia Peruana de Noticias). Retrieved from https://andina.pe/agencia/noticia-agua-pozos-morrope-contiene-arsenico-9-veces-mas-de-permitido-728855.aspx.
- Andina (2019). Nueva planta de tratamiento de agua beneficiará a 33,000 pobladores de Jauja. Andina (Agencia Peruana de Noticias). Retrieved from https://andina.pe/agencia/noticia-nueva-planta-tratamiento-agua-beneficiara-a-33000-pobladores-jauja-753352.aspx.
- Andreu, E. , Proenza, J. A. , Tauler, E. , Chavez, C. , & Espaillat, J. (2010). Gold and lodargyrite in the Gossan of Cerro de Maimón Deposit (Central Dominican Republic). Revista de la Sociedad Española de Mineralogía. Resumen SEM , 2010 (13), 41–42.
- Andreu, E. , Torró, I. , Proenza, J. A. , Domenech, C. , García Casco, A. , Villanova de Benavent, C. , Chavez, C. , Espaillat, J. , & Lewis, J. F. (2015). Weathering profile of the Cerro de Maimón VMS deposit (Dominican Republic): Textures, mineralogy, gossan evolution and mobility of gold and silver. Ore Geology Reviews , 65 , 165–179. https://doi.org/10.1016/j.oregeorev.2014.09.015
- Ángel, A. , & Fierro, J. (2012). Análisis y modelamiento del comportamiento de fluidos líquidos de pilas de escombros en minería de oro. Bogotá . Universidad Nacional de Colombia, Departamento de Geociencias.
- ANVISA (2013). Dispõe sobre o Regulamento Técnico MERCOSUL sobre Limites Máximos de Contaminantes Inorgânicos em Alimentos. Resolução RDC 42, Agência Nacional de Vigilância Sanitária, Brazil.
- Appelo, C. A. J. , van der Weiden, M. J. J. , Tournassat, C. , & Charlet, L. (2002). Surface complexation of ferrous iron and carbonate con ferrihydrite and the mobilization of arsenic. Environmental Science & Technology , 36 (14), 3096–3103. https://doi.org/10.1021/es010130n
- Arcega-Cabrera, F. , Garza, R. , Noreña, E. , & Oceguera, I. (2015). Impacts of geochemical and environmental factors on seasonal variation of heavy metals in a coastal lagoon Yucatan, Mexico. Bulletin of Environmental Contamination and Toxicology , 94 (1), 58–65. https://doi.org/10.1007/s00128-014-1416-1
- Archer, J. , Hudson-Edwards, K. A. , Preston, D. , Howarth, R. J. , & Linge, K. (2005). Aqueous exposure and uptake of arsenic by riverside communities affected by mining contamination in the Rio Pilcomayo basin, Bolivia. Mineralogical Magazine , 69 (5), 719–736. https://doi.org/10.1180/0026461056950283
- Arcia, J. (2018). Copper mine destroying forest in Panama’s Mesoamerican Biological Corridor . Forest Trackers, Global Forest Reporting Network. https://news.mongabay.com/2018/12/copper-mine-destroying-forests-in-panamas-mesoamerican-biological-corridor/
- Arias, V. , Rodriguez, A. , Bardos, P. , & Naidu, R. (2018). Contaminated land in Colombia: A critical review of current status and future approach for the management of contaminated sites. Science of the Total Environment , 618 , 199–209. https://doi.org/10.1016/j.scitotenv.2017.10.245
- Armienta, M. A. , & Segovia, N. (2008). Arsenic and fluoride in the groundwater of Mexico. Environmental Geochemistry and Health , 30 (4), 345–353. https://doi.org/10.1007/s10653-008-9167-8
- Armienta, M. A. , Rodríguez, R. , Aguayo, A. , Ceniceros, N. , Villaseñor, G. , & Cruz, O. (1997). Arsenic contamination of groundwater at Zimapán. Hydrogeology Journal , 5 (2), 39–46. https://doi.org/10.1007/s100400050111
- Armienta, M. A. , Villaseñor, G. , Rodríguez, R. , Ongley, L. K. , & Mango, H. (2001). The role of arsenic- bearing rocks in groundwater pollution at Zimapán Valley, México. Environmental Geology , 40 (4-5), 571–581. https://doi.org/10.1007/s002540000220
- Armienta, M. A. , Amat, P. D. , Larios, T. , & López, D. L. (2008). América Central y México. In J. Bundschuh , AP. Carrera , M. Litter (Eds.), Distribución del Arsénico en las Regiones Ibérica e Iberoamericana (pp. 187–210.). Editorial Programa Iberoamericano de Ciencia y Tecnología para el Desarrollo.
- Armienta, M. A. , Villaseñor, G. , Cruz, O. , Ceniceros, N. , Aguayo, A. , & Morton, O. (2012). Geochemical processes and mobilization of toxic metals and metalloids in an As-rich base metal waste pile in Zimapán, Central Mexico. Applied Geochemistry , 27 (11), 2225–2237. https://doi.org/10.1016/j.apgeochem.2012.01.015
- Armienta, M. A. , Rodríguez, R. , Ceniceros, N. , Cruz, O. , Aguayo, A. , Morales, P. , & Cienfuegos, E. (2014). Groundwater quality and geothermal energy. The case of Cerro Prieto Geothermal Field. Renewable Energy , 63 , 236–254. https://doi.org/10.1016/j.renene.2013.09.018
- Armienta, M. A. , Mugica, V. , Reséndiz, I. , & Gutiérrez, M. (2016). Arsenic and metals mobility in soils impacted by tailings at Zimapán, México. Journal of Soils and Sediments , 16 (4), 1267–1278. https://doi.org/10.1007/s11368-015-1244-x
- Armienta, M. A. , Cardona, A. , Labastida, I. , Alfaro de la Torre, C. , & Ballinas Casarrubias, M. L. (2018). Situación de la presencia de arsénico y fluoruro en aguas subterráneas en México. In L.M. Del Razo , J.M. Ledón , M.N. Velasco (Eds.), Hacia el cumplimiento del derecho humano al agua. Arsénico y fluoruro en agua: Riesgos y perspectivas desde la sociedad civil y la academia (pp. 21–30). Secretaría de Gobernación.
- Astudillo, F. , Baeza, L. , Barrera, J. , Carrasco, F. , Castillo, P. , Espinoza, F. , Figueroa, M. , Juan, L. , Miralles, C. , Muñoz, N. , & Ramírez, C. (2015). Base de datos de geoquímica de sedimentos de la Hoja Pisagua, regiones de Tarapacá y de Arica y Parinacota (No. Base de Datos 03). Sernageomin, Santiago de Chile, Chile.
- ATSDR (2007). Toxicological profile for Arsenic. Agency for Toxic Substances and Disease Registry, U.S. Department of Health and Human Services, Public Health Service, Atlanta, Georgia, U.S.A. https://www.atsdr.cdc.gov/toxprofiles/tp.asp?id=22&tid=3
- ATSDR (2019). Priority list of hazard substances. Agency for Toxic Substances and Disease Registry, U.S. Department of Health and Human Services, Division of Toxicology and Human Health Sciences, Atlanta, Georgia, U.S.A. https://www.atsdr.cdc.gov/spl/index.html#2019spl
- Aullón Alcaine, A. , Schulz, C. , Bundschuh, J. , Jacks, G. , Thunvik, R. , Gustafsson, J. P. , Mörth, C. M. , Sracek, O. , Ahmad, A. , & Bhattacharya, P. (2020). Hydrogeochemical controls on the mobility of arsenic, fluoride and other geogenic co-contaminants in the shallow aquifers of northeastern La Pampa Province in Argentina. Science of the Total Environment , 715 , 136671. https://doi.org/10.1016/j.scitotenv.2020.136671
- Avigliano, E. , Schenone, N. F. , Volpedo, A. V. , Goessler, W. , & Fernández Cirelli, A. (2015). Heavy metals and trace elements in muscle of silverside (Odontesthes bonariensis) and water from different environments (Argentina): Aquatic pollution and consumption effect approach. Science of the Total Environment , 506-507 , 102–108. https://doi.org/10.1016/j.scitotenv.2014.10.119
- Ayerza, A. (1917a). Arsenicismo regional endemico (keratodermia y melanodermia combinadas. Boletín de la Academia Nacional de Medicina , 2–3 , 11–24.
- Ayerza, A. (1917b). Arsenicismo regional endémico (keratodermia y melanodermia combinadas (Continuación). Boletín de la Academia Nacional de Medicina , 2–3 , 41–55.
- Ayerza, A. (1918). Arsenicismo regional endémico (keratodermia y melanodermia combinadas) (Continuación). Boletín de la. Academia Nacional de Medicina , 1–24.
- Azevedo, L. S. , Pestana, I. A. , Meneguelli-Souza, A. C. , De Souza Ramos, B. , Pessanha, D. R. , Caldas, D. , Almeida, M. G. , & Souza, C. M. M. (2018). Risk of exposure to total and inorganic arsenic by meat intake among different age groups from Brazil: A probabilistic assessment. Environmental Science and Pollution Research International , 25 (35), 35471–35478. https://doi.org/10.1007/s11356-018-3512-y
- Baeyens, W. , Mirlean, N. , Bundschuh, J. , Winter, N. , Baisch, P. , Silva Júnior, F. M. R. , & Gao, Y. (2019). Arsenic enrichment in sediments and beaches of Brazilian coastal waters. A review. Science of the Total Environment , 681 , 143–154. https://doi.org/10.1016/j.scitotenv.2019.05.126
- Baeza, L. , Juan, L. , Astudillo, F. , Barrera, J. , Carrasco, F. , Castillo, P. , Espinoza, F. , Figueroa, M. , Miralles, C. , Muñoz, N. , Ramírez, C. , & Salinas, P. (2014). Base de datos de geoquímica de sedimentos de la Hoja Arica, región de Arica y Parinacota (02), Base de Datos. Sernageomin, Servicio Nacional de Geología y Minería, Santiago de Chile, Chile.
- Bardach, A. E. , Ciapponi, A. , Soto, N. , Chaparro, M. R. , Calderón, M. , Briatore, A. , Cadoppi, N. , Tassara, R. , & Litter, M. (2015). Epidemiology of chronic disease related to arsenic in Argentina: A systematic review. Science of the Total Environment , 538 , 802–816. https://doi.org/10.1016/j.scitotenv.2015.08.070
- Barrera, J. , Roth, T. , & Juan, L. (2017). Línea base geoquímica de sedimentos fluviales de la cuenca del Río Aysén, XI Región Aysén del General Carlos Ibáñez del Campo, Chile, Informe Registrado IR-17-67. Servicio Nacional de Geología y Minería, Santiago de Chile, Chile.
- Basu, N. , Abare, M. , Buchanan, S. , Cryderman, D. , Nam, D. H. , Sirkin, S. , Schmitt, S. , & Hu, H. (2010). A combined ecological and epidemiologic investigation of metal exposures amongst Indigenous peoples near the Marlin Mine in Western Guatemala. Science of the Total Environment , 409 (1), 70–77. https://doi.org/10.1016/j.scitotenv.2010.09.041
- Batista, B. L. , Souza, J. M. , De Souza, S. S. , & Barbosa, F. (2011). Speciation of arsenic in rice and estimation of daily intake of different arsenic species by Brazilians through rice consumption. Journal of Hazardous Materials , 191 (1-3), 342–348. https://doi.org/10.1016/j.jhazmat.2011.04.087
- Battistel, D. , Roman, M. , Marchetti, A. , Kehrwald, N. M. , Radaelli, M. , Balliana, E. , Toscano, G. , & Barbante, C. (2018). Anthropogenic impact in the Maya Lowlands of Petén, Guatemala, during the last 5500 years. Journal of Quaternary Science , 33 (2), 166–176. https://doi.org/10.1002/jqs.3013
- Bécher-Quinodóz, F. N. , Blarasin, M. T. , & Panarello, H. O. (2015). Modelado geoquímico e isotópico de las relaciones agua superficial-subterránea. Revista de la Asociación Geológica Argentina , 72 , 506–518.
- Bejarano-Sifuentes, G. , & Nordberg, E. (2003). Mobilisation of arsenic in the Rio Dulce alluvial cone, Santiago del Estero Province, Argentina. TRITA-LWR Master Thesis, Department of Land and Water Resources Engineering, Royal Institute of Technology (KTH), Stockholm, Sweden.
- Benavides, J. (2006). Iron oxide-copper-gold deposits of the Mantoverde area, northern Chile: Ore genesis and exploration guidelines [PhD Thesis]. Queen’s University, Canada.
- Berry, K. L. E. , Seemann, J. , Dellwig, O. , Struck, U. , Wild, C. , & Leinfelder, R. R. (2013). Sources and spatial distribution of heavy metals in scleractinian coral tissues and sediments from the Bocas de Toro Archipelago, Panama. Environmental Monitoring and Assessment , 185 (11), 9089–9099. https://doi.org/10.1007/s10661-013-3238-8
- Bhattacharya, P. , Claesson, M. , Bundschuh, J. , Sracek, O. , Fagerberg, J. , Jacks, G. , Martin, R. A. , Storniolo, A. R. , & Thir, J. M. (2006). Distribution and mobility of arsenic in the Río Dulce Alluvial aquifers in Santiago del Estero Province, Argentina. Science of the Total Environment , 358 (1-3), 97–120. https://doi.org/10.1016/j.scitotenv.2005.04.048
- Bia, G. , Borgnino, L. , Gaiero, D. , & García, M. G. (2015). Arsenic-bearing phases in south Andean volcanic ashes: Implications for As mobility in aquatic environments. Chemical Geology , 393-394 , 26–35. https://doi.org/10.1016/j.chemgeo.2014.10.007
- Bia, G. , García, M. G. , & Borgnino, L. (2017). Changes in the As solid speciation during weathering of volcanic ashes. A XAS study on Patagonian ashes and Chaco-Pampean loess. Geochimica et Cosmochimica Acta , 212 (1), 119–132. https://doi.org/10.1016/j.gca.2017.06.016
- Bidone, E. D. , Castilhos, Z. C. , Bertolino, L. C. , Santos, M. C. B. , Silva, R. S. V. , & Cesar, R. G. (2014). Arsenic in soils and sediments from Paracatu, Brazil. In M. I. Litter , H. B. Nicolli , J. M. Meichtry , N. Quici , J. Bundschuh , P. Bhattacharya , R. Naidu , (Eds.), V International Congress of Arsenic in the Environment – As2014 (pp. 113–115). CRC Press.
- Bidone, E. , Cesar, R. , Santos, M. C. , Sierpe, R. , Silva-Filho, E. V. , Kutter, V. , Dias da Silva, L. I. , & Castilhos, Z. (2018). Mass balance of arsenic fluxes in rivers impacted by gold mining activities in Paracatu (Minas Gerais State, Brazil). Environmental Science and Pollution Research , 25 (9), 9085–9910. https://doi.org/10.1007/s11356-018-1215-z
- Birkle, P. , Bundschuh, J. , & Sracek, O. (2010). Mechanisms of arsenic enrichment in geothermal and petroleum reservoirs fluids in Mexico. Water Research , 44 (19), 5605–5617. https://doi.org/10.1016/j.watres.2010.05.046
- Blanco, M. C. , Paoloni, J. D. , Morrás, H. J. M. , Fiorentino, C. E. , & Sequeira, M. (2006). Content and distribution of arsenic in soils, sediments and groundwater environments of the southern Pampa Region, Argentina. Environmental Toxicology , 21 (6), 561–574. https://doi.org/10.1002/tox.20219
- Blanco, M. C. , Paoloni, J. D. , Morrás, H. , Fiorentino, C. , Sequeira, M. E. , Amiotti, N. N. , Bravo, O. , Díaz, S. , & Espósito, M. (2012). Partition of arsenic in soils sediments and the origin of naturally elevated concentrations in groundwater of the southern Pampa region (Argentina). Environmental Earth Sciences , 66 (7), 2075–2084. https://doi.org/10.1007/s12665-011-1433-x
- Blodau, C. (2006). A review of acidity generation and consumption in acidic coal mine lakes and their watersheds. Science of the Total Environment , 369 (1-3), 307–332. https://doi.org/10.1016/j.scitotenv.2006.05.004
- Bonotto, D. M. , & Elliot , T. (2017). Trace elements, REEs and stable isotopes (B, Sr) in GAS groundwater, São Paulo State, Brazil. Environtal Earth Sciences, 76, 265 https://doi.org/10.1007/s12665-017-6590-0
- Borba, R. P. , Figueiredo, B. R. , Rawlins, B. , & Matschullat, J. (2003). Geochemical distribution of arsenic in waters, sediments and weathered gold mineralized rocks from Iron Quadrangle, Brazil. Environmental Geology , 44 (1), 39–52. https://doi.org/10.1007/s00254-002-0733-6
- Borba, R. P. , & Figueiredo, B. R. (2004). A influência das condições geoquímicas na oxidação da arsenopirita e na mobilidade do arsênio em ambientes superficiais tropicais. Revista Brasileira de Geociências , 34 (4), 489–500. https://doi.org/10.25249/0375-7536.2004344489500
- Borgoño, J. , & Greiber, R. (1972). Epidemiological study of arsenicism in the city of Antofagasta. Trace Substances in Environmental Health , 5 , 13–24.
- Bortnikov, N. S. , Kramer, K. L. , Genkin, A. D. , & Kul'nev, A. S. (1989). The mineralogy and conditions of formation of the Delita gold deposit, Cuba. International Geology Review , 31 (11), 1158–1171. https://doi.org/10.1080/00206818909465968
- Borzi, G. E. , García, L. , & Carol, E. S. (2015). Geochemical processes regulating F−, As and NO3 − content in the groundwater of a sector of the Pampean Region, Argentina. Science of the Total Environment , 530-531 , 154–162. https://doi.org/10.1016/j.scitotenv.2015.05.072
- BP (2019). Statistical Review of World Energy 2019–68th Edition. https://www.bp.com/content/dam/bp/business-sites/en/global/corporate/pdfs/energy-economics/statistical-review/bp-stats-review-2019-full-report.pdf (Accessed February 4, 2020).
- Brazil (2002). Ministério da Agricultura, Pecuária e Abastecimento. Portaria (31). Diário Oficial da União.
- Brazil (2011). Dispõe sobre os procedimentos de controle e de vigilância da qualidade da água para consumo humano e seu padrão de potabilidade. Ministério da Saúde. Portaria (2.914). Diário Oficial da União.
- Bundschuh, J. , Farias, B. , Martin, R. , Storniolo, A. , Bhattacharya, P. , Cortes, J. , Bonorino, G. , & Albouy, R. (2004). Groundwater arsenic in the Chaco-Pampean Plain, Argentina. Applied Geochemistry , 19 (2), 231–243. https://doi.org/10.1016/j.apgeochem.2003.09.009
- Bundschuh, J. , Litter, M. , Ciminelli, V. , Morgada, M. E. , Cornejo, L. , Garrido Hoyos, S. , Hoinkis, J. , Alarcón-Herrera, M. T. , Armienta, M. A. , & Bhattacharya, P. (2010). Emerging mitigation needs and sustainable options for solving the arsenic problems of rural and isolated urban areas in Iberoamerica – A critical analysis. Water Research , 44 (19), 5828–5845. https://doi.org/10.1016/j.watres.2010.04.001
- Bundschuh, J. , Bhattacharya, P. , Sracek, O. , Mellano, M. F. , Ramírez, A. E. , Storniolo, A. , Martín, R. A. , Cortés, J. , Litter, M. I. , & Jean, J.-S. (2011). Arsenic removal from groundwater of the Chaco-Pampean Plain (Argentina) using natural geological materials as adsorbents. Journal of Environmental Science and Health, Part A , 46 (11), 1297–1214. https://doi.org/10.1080/10934529.2011.598838
- Bundschuh, J. , Litter, M. I. , Parvez, F. , Román-Ross, G. , Nicolli, H. B. , Jean, J.-S. , Liu, C.-W. , López, D. , Armienta, M. A. , Guilherme, L. R. G. , Cuevas, A. G. , Cornejo, L. , Cumbal, L. , & Toujaguez, R. (2012). One century of arsenic exposure in Latin America: A review of history and occurrence from 14 countries. Science of the Total Environment , 429 , 2–35. https://doi.org/10.1016/j.scitotenv.2011.06.024
- Bundschuh, J. , Nath, B. , Bhattacharya, P. , Liu, C.-W. , Armienta, M. A. , Moreno López, M. V. , Lopez, D. L. , Jean, J.-S. , Cornejo, L. , Fagundes, L. , Macedo, L. , & Tenuta Filho, A. (2012). Arsenic in the human food chain: The Latin American perspective. Science of the Total Environment , 429 , 92–106. https://doi.org/10.1016/j.scitotenv.2011.09.069
- Bundschuh, J. , Maity, J. P. , Mushtaq, S. , Vithanage, M. , Seneweera, S. , Schneider, J. , Bhattacharya, P. , Khan, N. I. , Hamawand, I. , Guilherme, L. R. G. , Reardon-Smith, K. , Parvez, F. , Morales-Simfors, N. , Ghaze, S. , Pudmenzky, C. , Kouadio, L. , & Chen, C. Y. (2017). Medical geology in the framework of the sustainable development goals. Science of the Total Environment , 581-582 (58), 87–104. https://doi.org/10.1016/j.scitotenv.2016.11.208
- Burger, J. , Jeitner, C. , Schneider, L. , Vogt, R. , & Gochfeld, M. (2009). Arsenic, cadmium, chromium, lead, mercury, and selenium levels in blood of four species of turtles from the Amazon in Brazil. Journal of Environmental Science and Health, Part A , 73 (1), 33–40. https://doi.org/10.1080/15287390903248877
- Bustos Rodriguez, H. , Oyola Lozano, D. , Rojas Martínez, Y. A. , Pérez Alcázar, G. A. , Flege, S. , Balogh, A. G. , Cabri, L. J. , & Tubrett, M. (2007). Mineralogical analysis of auriferous ores from the El Diamante mine, Colombia. Hyperfine Interactions , 175 (1-3), 195–206. https://doi.org/10.1007/s10751-008-9603-2
- Cabassi, J. , Capecchiacci, F. , Magi, F. , Vaselli, O. , Tassi, F. , Montalvo, F. , Esquivel, I. , Grassa, F. , & Caprai, A. (2019). Water and dissolved gas geochemistry at Coatepeque, Ilopango and Chanmico volcanic lakes (El Salvador, Central America). Journal of Volcanology and Geothermal Research , 378 , 1–15. https://doi.org/10.1016/j.jvolgeores.2019.04.009
- Cacciabue, L. , Dietrich, S. , Sierra, L. , Bea, S. A. , Weinzettel, P. A. , & García, M. G. (2016). Study of arsenic availability in Pampean loess sediments using a sequential extraction procedure. In Arsenic Research and Global Sustainability. Proceedings of the Sixth International Congress on Arsenic in the Environment - As2016, June 19-23, 2016, CRC Press. p. 167.
- Cacua, I. (2004). Caracterización físico-química y microbiológica para aguas subterráneas en zona de influencia del Acueducto Metropolitano de Bucaramanga e implementación de los métodos de análisis para arsénico, selenio y flúor . Bucaramanga, Universidad Industrial de Santander, Bucaramanga, Santander.
- Cagnin, R. C. , Quaresma, V. S. , Chaillou, G. , Franco, T. , & Bastos, A. C. (2017). Arsenic enrichment in sediment on the eastern continental shelf of Brazil. Science of the Total Environment , 607-608 , 304–316. https://doi.org/10.1016/j.scitotenv.2017.06.162
- Calarge, L. M. , Meunier, A. , Lanson, B. , & Formoso, M. L. L. (2006). Chemical signature of two Permian volcanic ash deposits whitin a bentonite bed from Melo, Uruguay. Anais da Academia Brasileira de Ciências , 78 (3), 525–541. https://doi.org/10.1590/S0001-37652006000300012
- Caldas, D. , Pestana, I. A. , Almeida, M. G. , Henry, F. C. , Salomão, M. S. M. B. , & Souza, C. M. M. (2016). Risk of ingesting As, Cd, and Pb in animal products in north Rio de Janeiro State. Chemosphere , 164 , 508–515. https://doi.org/10.1016/j.chemosphere.2016.08.130
- Campaner, V. P. , & Silva, W. L. (2009). Processos físico-químicos em drenagem ácida de mina em mineração de carvão no sul do Brasil. Química Nova , 32 (1), 146–152. https://doi.org/10.1590/S0100-40422009000100028
- Campaner, V. P. , Luiz-Silva, W. , & Machado, W. (2014). Geochemistry of acid mine drainage from a coal mining area and processes controlling metal attenuation in stream waters, southern Brazil. Anais da Academia Brasileira de Ciencias , 86 (2), 539–554. https://doi.org/10.1590/0001-37652014113712
- Campos, M. L. , Guilherme, L. R. G. , Lopes, R. S. , Antunes, A. S. , Marques, J. J. G. S. M. , & Curi, N. (2007). Teor e capacidade máxima de adsorção de arsênio em Latossolos brasileiros. Revista Brasileira de Ciência Do Solo , 31 (6), 1311–1308. https://doi.org/10.1590/S0100-06832007000600010
- Campos, M. L. , Guilherme, L. R. G. , Antunes, A. S. , & Borges, K. S. C. (2013). Teor de arsênio e adsorção competitiva arsênio/fosfato e arsênio/sulfato em solos de Minas Gerais, Brasil. Ciência Rural , 43 (6), 985–991. https://doi.org/10.1590/S0103-84782013005000057
- Campos, V. (2002). Arsenic in groundwater affected by phosphate fertilizers at São Paulo, Brazil. Environmental Geology , 42 (1), 83–87. https://doi.org/10.1007/s00254-002-0540-0
- Cano de los Ríos, M. P. (2010). Contaminación del agua por metales pesados en presas del municipio de Chihuahua Chihuahua, Chih., Mexico. Bachelor Thesis, Facultad de Zootecnia y Ecología, Universidad Autónoma de Chihuahua, Chihuahua, Mexico.
- Cardenas, R. , Miranda, H. , & Krutzelmann, H. (2018). Technical report on the Pueblo Viejo Mine, Sanchez Ramirez Province, Dominican Republic. Technical Report (43-101), 1–29.
- Carling, G. T. , Díaz, X. , Ponce, M. , Perez, L. , Nasimba, L. , Pazmino, E. , Rudd, A. , Merugu, S. , Fernandez, D. P. , Gale, B. K. , & Johnson, W. P. (2013). Particulate and dissolved trace element concentrations in three southern Ecuador rivers impacted by artisanal gold mining. Water, Air, & Soil Pollution , 224 (2), 1415. https://doi.org/10.1007/s11270-012-1415-y
- Carrillo-Chávez, A. , Drever, J. , & Martínez, M. (2000). Arsenic content and ground water geochemistry of the San Antonio-El Triunfo, El Carrizal and Los Planes aquifers in southermost Baja California México. Environmental Geology , 39 (11), 1295–1303. https://doi.org/10.1007/s002540000153
- Carrillo-Chávez, A. , Salas-Megchún, E. , Levresse, G. , Muñoz-Torres, C. , Pérez-Arvizu, O. , & Gerke, T. (2014). Geochemistry and mineralogy of mine-waste material from a “skarn-type” deposit in central Mexico: Modeling geochemical controls of metals in the surface environment. Journal of Geochemical Exploration , 144 , 28–36. https://doi.org/10.1016/j.gexplo.2014.03.017
- Carvalho, G. O. , Pinhero, A. A. , Sousa, D. M. , Padilha, J. A. , Souza, J. S. , Galvão, P. M. , Paiva, T. C. , Freire, A. S. , Santelli, R. E. , Malm, O. , & Torres, J. P. M. (2018). Metals and arsenic in water supply for riverine communities affected by the largest environmental disaster in Brazil: The dam collapse on Doce River. Orbital. The Electronic Journal of Chemistry , 10 , 299–307.
- Castro de Esparza, M. L. (2009). The presence of arsenic in drinking water in Latin America and its effect on public health. In J. Bundschuh , M. A. Armienta , P. Birkle , P. Bhattacharya , J. Matschullat , A.B. Mukherjee , (Eds.), Natural arsenic in groundwater of Latin America (pp. 17–29). CRC Press.
- Castro-Larragoitia, J. , Krama, U. , & Puchelt, H. (1997). 200 years of mining acitivities at La Paz/San Luis Potosí/Mexico - Consequences for environment and geochemical exploration. Journal of Geochemical Exploration , 58 (1), 81–91. https://doi.org/10.1016/S0375-6742(96)00054-4
- Castro-Larragoitia, J. , Kramar, U. , Monroy, M. , Viera, F. , & García, E. (2013). Heavy metal and arsenic dispersion in a copper-skarn mining district in a Mexican semi-arid environment: Sources, pathways and fate. Environmental Earth Sciences , 69 (6), 1915–1929. https://doi.org/10.1007/s12665-012-2024-1
- CCME (2002). Canadian Sediment Quality Guidelines for the Protection of Aquatic Life. In: Canadian Environment Quality Guidelines, Canadian Council of Ministers of the Environment (CCME). http://ceqg-rcqe.ccme.ca/en/index.html#void
- CCME (2019). Soil Quality Guidelines for the Protection of Environmental and Human Health, Canadian Council of Ministers of the Environment. http://st-ts.ccme.ca/en/index.html
- Cebrián, M. E. , Albores, A. , García-Vargas, G. , Del Razo, L. M. , & Ostrosky-Wegman, P. (1994). Chronic arsenic poisoning in humans: The case of Mexico. In J. O. Nriagu (Ed.), Arsenic in the environment, Part II: Human health and ecosystem effects (pp. 93–107). John Wiley & Sons, Inc.
- CEICOM (2010a). Análisis de la calidad de agua y su relación con la salud y calidad de vida de los pobladores del Río San Sebastián, en la zona de minas San Sebastián, Municipio de Santa Rosa de Lima, Departamento de La Unión, El Salvador. Unpublished report, Centro de Investigación sobre Inversión y Comercio (CEICOM), San Salvador, El Salvador.
- CEICOM (2010b). Determinación de metales pesados en suelos, sedimentos y semilla de maíz, en áreas agrícolas expuestas a desechos de minería metálica, y su repercusión en la cadena alimenticia. El Divisadero, Morazán, El Salvador. Unpublished report, Centro de Investigación sobre Inversión y Comercio (CEICOM), San Salvador, El Salvador.
- CENMA (2014). Diagnóstico regional de suelos abandonados con potencial presencia de contaminantes. Informe Final Versión 4, Centro Nacional del Medio Ambiente (CENMA), Santiago de Chile, Chile.
- Cervantes-Guerra, Y. , Almaguer Carmenates, Y. , Pierra Conde, A. , Orozco Melgar, G. , & Gursky, J. H. (2011). Metales traza en sedimentos de la bahía de Cayo Moa (Cuba): Evaluación preliminar de la contaminación. Minería y Geología , 27 (4), 1–19.
- Cervantes-Guerra, Y. , Pierra Conde, A. , Rodríguez Infante, A. , Almaguer Carmenates, Y. , Gursky, J. H. , Van Caneghem, J. , & Vandecasteele, C. (2017). Metal accumulation in surface sediment of the urban and industrial coastal area. Minería y Geología , 33 (2), 235–250.
- Cha, J. D. , Lourenço, D. B. , & Korkes, F. (2018). Analysis of the association between bladder carcinoma and arsenic concentration in soil and water in southeast Brazil. International Braz j Urol , 44 (5), 906–913. https://doi.org/10.1590/s1677-5538.ibju.2017.0543
- Chávez, A. , Pérez, C. , Tovar, E. , & Gramilla, M. (1964). Estudios en una comunidad con arsenicismo crénico endémico. Salud Pública de México , 6 , 421–433.
- Chong, G. , Pueyo, J. J. , & Demergasso, C. (2010). The borate deposits in Chile. Andean Geology , 27 , 99–119.
- Chung, J. Y. , Yu, S. D. , & Hong, Y. S. (2014). Environmental source of arsenic exposure. Journal of Preventive Medicine and Public Health = Yebang Uihakhoe Chi , 47 (5), 253–257. https://doi.org/10.3961/jpmph.14.036
- CIRA/UNAN-Managua (2011a). Resultados de análisis para el Programa de Maestría. Jinotega, Nicaragua . CIRA/UNAN-Managua.
- CIRA/UNAN-Managua (2011b). Datos internos del Laboratorio de Contaminantes Metálicos (Río Coco) . CIRA/UNAN-Managua.
- CIRA/UNAN-Managua (2011c). Datos internos del Laboratorio de Contaminantes Metálicos (Masaya). CIRA/UNAN-Managua.
- CIRA/UNAN-Managua (2012). Contribución al establecimiento de la línea de base ambiental del Rio San Juan de Nicaragua-2012 . CIRA/UNAN-Managua.
- CIRA/UNAN-Managua (2016). Project CIRA/UNAN Nicaragua and CSRSR Taiwan .
- CIRA/UNAN-Managua , & Altamirano Espinoza, M. (2017). Estudio de la contaminación natural por arsénico en las aguas subterráneas de la comunidad rural “La Fuente”, para sugerir y promover el uso de fuentes alternativas de agua segura. Municipio de La Paz Centro, León, Nicaragua. CIRA/UNAN-Managua, Managua, Nicaragua.
- CIRA/UNAN-Managua , & Delgado Quezada, V. (2019a). Tiscapa, arsenic analysis. CIRA/UNAN- Managua .,
- CIRA/UNAN-Managua , & Delgado Quezada, V. (2019b). Resultados analíticos de arsénico en la microcuenca del Río Dipilto .
- CIRA/UNAN-Managua , Sarria Sacasa, K. , del, C. , & Lacayo Membreño, J. (1999). Determinación de metales pesados en sedimentos del Lago Cocibolca, Nicaragua. Managua. CIRA/UNAN-Managua, Departamento de Micropoluentes - Sección Adsorción Atómica, Managua, Nicaragua.
- CIRA/UNAN-Managua , Calderón Palma, H. L. , & Flores Meza, Y. (2010). Evaluación de la dinámica de la Laguna de Apoyo mediante trazadores isotópicos y geoquímicos . CIRA/UNAN-Managua.
- CIRA/UNAN-Managua , Rivas Navarrete, K. , Rojas Cerda, K. , & Altamirano Espinoza, M. (2017). Informar a los pobladores de El Descanso (La Breña Tres), Municipio de Nandaime, sobre la calidad física, química y microbiológica de sus fuentes de agua, Departamento de Granada. Informe final Fondos de Proyectos de Investigación (FPI) UNAN-Managua, Managua, Nicaragua.
- Codex Alimentarius Commission (2014). Thirty-seventh Session CICG, Geneva, Switzerland, 14–18 July 2014, http://www.fao.org/news/story/en/item/238558/icode/
- Código Alimentario Argentino (2012). Bebidas hídricas, aguas y aguas gasificadas. Capítulo XII. http://www.anmat.gov.ar/alimentos/codigoa/CAPITULO_XII.pdf.
- Colina Blanco, A. (2015). Remoción de arsénico en aguas contaminadas utilizando fibras vegetales funcionalizadas con un polímero policatiónico como intercambiador iónico [Licenciate Thesis]. University of Costa Rica.
- CONAMA (2005). Conselho Nacional do Meio Ambiente N° 357/2005. Dispõe sobre a classificação dos corpos de água e diretrizes ambientais para o seu enquadramento, bem como estabelece as condições e padrões de lançamento de efluentes, e dá outras providências. Publicação DOU (053), 58–63.
- CONAMA (2008). Conselho Nacional do Meio Ambiente N° 396/2008.Dispõe sobre a classificação e diretrizes ambientais para o enquadramento das águas subterrâneas e dá outras providências. Publicação DOU (66), 66–68.
- CONAMA (2009). Conselho Nacional do Meio Ambiente. Resolução N° 420, de 28 de dezembro de 2009 [internet]. Dispõe sobre critérios e valores orientadores de qualidade do solo quanto à presença de substâncias químicas e estabelece diretrizes para o gerenciamento ambiental de áreas contaminadas por essas substâncias em decorrência de atividades antrópicas. Brasília, DF: Diário Oficial [da] União (249), 81-84. http://www.mma.gov.br/port/conama/legiabre.cfm?codlegi=620.
- Coomar, P. , Mukherjee, A. , Bhattacharya, P. , Bundschuh, J. , Verma, S. , Fryar, A. E. , Ramos Ramos, O. E. , Ormachea Muñoz, M. , Gupta, S. , Mahanta, C. , Quino, I. , & Thunvik, R. (2019). Contrasting controls on hydrogeochemistry of arsenic-enriched groundwater in the homologous tectonic settings of Andean and Himalayan basin aquifers, Latin America and South Asia. Science of the Total Environment , 689 , 1370–1387. https://doi.org/10.1016/j.scitotenv.2019.05.444
- Corguinha, A. P. B. , Souza, G. A. , Gonçalves, V. C. , Carvalho, C. A. , Lima, W. E. A. , Martins, F. A. D. , Yamanaka, C. H. , Francisco, E. A. B. , & Guilherme, L. R. G. (2015). Assessing arsenic, cadmium, and lead contents in major crops in Brazil for food safety purposes. Journal of Food Composition and Analysis , 37 , 143–150. https://doi.org/10.1016/j.jfca.2014.08.004
- Cornejo, A. E. , López-López, R. A. , Ruiz-Picos, J. E. , Sedeño-Días, B. , Armitage, B. , Arefina, C. , Nieto, A. , Tuñon, M. , Molinar, T. , Abrego, E. , Pérez, A. R. , Tuñon, J. , Magué, A. , Rodríguez, J. , Pineda, J. , Cubilla, J. , & Quintero, A. (2017). Diagnóstico de la condición ambiental de los afluentes superficiales de Panamá. Instituto Conmemorativo Gorgas de Estudios de la Salud, Ministerio de Ambiente, Panama.
- Corral, I. , Corbella, M. , Gómez-Gras, D. , & Griera, A. (2018). Trace-metal content of the Cerro Quema Au-Cu deposit (Azuero Peninsula, Panama): Implication for exploration. Boletín de la Sociedad Geológica Mexicana , 70 (2), 549–565. https://doi.org/10.18268/BSGM2018v70n2a14
- Cortecci, G. , Boschetti, T. , Mussi, M. , Lameli, C. H. , Mucchino, C. , & Barbieri, M. (2005). New chemical and original isotopic data on waters from El Tatio geothermal field, northern Chile. Geochemical Journal , 39 (6), 547–571. https://doi.org/10.2343/geochemj.39.547
- Costa, L. , Mirlean, N. , Quintana, G. , Adebayo, S. , & Johannesson, K. (2019). Distribution and geochemistry of arsenic in sediments of the world’s largest choked estuary: The Patos Lagoon, Brazil. Estuaries and Coasts , 42 (7), 1896–1911. https://doi.org/10.1007/s12237-019-00596-0
- Costa, R. V. F. , Leite, M. G. P. , Mendonça, F. P. C. , & Nalini, H. A. Jr. (2015). Geochemical mapping of arsenic in surface waters and stream sediments of the Quadrilátero Ferrífero, Brazil. Rem: Revista Escola de Minas , 68 (1), 43–51. https://doi.org/10.1590/0370-44672015680077
- CPRM (2015). Monitoramento especial do Rio Doce. Relatório 02: Geoquímica. Segunda campanha de campo 12 a 23 de novembro de 2015. Companhia de Pesquisa de Recursos Minerais (CPRM), Belo Horizonte, Brazil. http://portal1.snirh.gov.br/arquivos/Doce/CPRM/relatorios/RT_02_2015_MONIT_ESP_BACIA_RIO_DOCE_v4_5.pdf
- Cristiano, E. , Hu, Y. J. , Siegfried, M. , Kaplan, D. , & Nitsche, H. (2011). A comparison of point of zero charge measurement methodology. Clays and Clay Minerals , 59 (2), 107–115. https://doi.org/10.1346/CCMN.2011.0590201
- Cumbal, L. H. , Bundschuh, J. , Aguirre, V. , Murgueitio, E. , Tipán, I. , & Chávez, C. (2009). The origin of arsenic in sediments from Papallacta Lake area in Ecuador. In J. Bundschuh , M. A. Armienta , P. Birkle , P. Bhattacharya , J. Matschullat , A. B. Mukherjee (Eds.), Natural Arsenic in Groundwaters of Latin America (pp. 81–90). CRC Press.
- Cumbal, L. , Vallejo, P. , Rodriguez, B. , & Lopez, D. (2010). Arsenic in geothermal sources at the north-central Andean region of Ecuador: Concentrations and mechanisms of mobility. Environmental Earth Sciences , 61 (2), 299–310. https://doi.org/10.1007/s12665-009-0343-7
- D’Urso, C. , Rodríguez Areal, M. , Marchisio, P. , López, J. , Sales, A. , Rodríguez, G. , & Rodríguez, M. (2013). Caracterización hidroquímica del Valle de Tafí, Provincia de Tucumán, República Argentina. Acta Geológica Lilloana , 25 , 9–20.
- Daesslé, L. W. , Lugo-Ibarra, K. C. , Tobschall, H. J. , Melo, M. , Gutiérrez-Galindo, E. A. , García- Hernández, J. , & Álvarez, L. G. (2009). Accumulation of As, Pb, and Cu associated with the recent sedimentary processes in the Colorado Delta, south of the United States-Mexico boundary. Archives of Environmental Contamination and Toxicology , 56 (4), 680–692. https://doi.org/10.1007/s00244-008-9218-2
- Daniele, L. , Cannatelli, C. , Buscher, J. T. , & Bonatici, G. (2019). Chemical composition of Chilean bottled waters: Anomalous values and possible effects on human health. Science of the Total Environment , 689 , 526–533. https://doi.org/10.1016/j.scitotenv.2019.06.165
- da Silva Júnior, E. C. , Martins, G. C. , de Oliveira Wadt, L. H. , da Silva, K. E. , de Lima, R. M. B. , Batista, K. D. , Guedes, M. C. , de Oliveira Junior, R. C. , Reis, A. R. , Lopes, G. , de Menezes, M. D. , Broadley, M. R. , Young, S. D. , & Guilherme, L. R. G. (2019). Natural variation of arsenic fractions in soils of the Brazilian Amazon. Science of the Total Environment , 687 , 1219–1231. https://doi.org/10.1016/j.scitotenv.2019.05.446
- Datta, B. K. , Bhar, M. K. , Patra, P. H. , Majumdar, D. , Dey, R. R. , Sarkar, S. , Mandal, T. K. , & Chakraborty, A. K. (2012). Effect of environmental exposure of arsenic on cattle and poultry in Nadia district, West Bengal, India. Toxicology International , 19 (1), 59–62. https://doi.org/10.4103/0971-6580.94511
- Davila, R. B. , Fontes, M. P. F. , Pacheco, A. A. , & Ferreira, M. S. (2020). Heavy metals in iron ore tailings and floodplain soils affected by the Samarco dam collapse in Brazil. Science of the Total Environment , 709 , 136151. https://doi.org/10.1016/j.scitotenv.2019.136151
- De Carlo, E. H. , Tomlinson, M. S. , deGelleke, L. E. , & Thomas, S. (2014). Distribution and abundance of arsenic in the soil and Sediments of Oahu, Hawaii. Aquatic Geochemistry , 20 (2-3), 87–113. https://doi.org/10.1007/s10498-013-9212-9
- De Gregori, I. , Fuentes, E. , Rojas, M. , Pinochet, H. , & Potin-Gautier, M. (2003). Monitoring of copper, arsenic and antimony levels in agricultural soils impacted and non-impacted by mining activities, from three regions in Chile. Journal of Environmental Monitoring: Jem , 5 (2), 287–295. https://doi.org/10.1039/b211469k
- de Meyer, C. M. C. , Rodríguez, J. M. , Carpio, E. A. , García, P. A. , Stengel, C. , & Berg, M. (2017). Arsenic, manganese and aluminum contamination in groundwater resources of western Amazonia, Peru. Science of the Total Environment , 607-608 , 1437–1450. https://doi.org/10.1016/j.scitotenv.2017.07.059
- Decreto N° 883 (1995). Normas para la clasificación y el control de la calidad de los cuerpos de agua y vertidos o efluentes líquidos. In Gaceta Oficial de la República de Venezuela (5.02. Extraordinario del 18 de Diciembre de 1995).
- Degray, A. , & Miles, C. (2014). An analysis of the hydrological and socioeconomic impacts of the Cerro Quema open pit mine (Final Report.). ENVR 451, Panama, 1–59.
- Delgado Quezada, V. , Altamirano Espinoza, M. , & Bundschuh, J. (2020). Arsenic in geoenvironments of Nicaragua: Exposure, health effects, mitigation and future needs. Science of the Total Environment , 716 , 136527. https://doi.org/10.1016/j.scitotenv.2020.136527
- Del Razo, L. M. , Corona, J. C. , García-Vargas, G. , Albores, A. , & Cebrián, M. E. (1993). The oxidation states of arsenic in well water from a chronic arsenicism area of northern Mexico. Environmental Pollution , 80 (1), 91–94. https://doi.org/10.1016/0269-7491(90)90111-O
- Deschamps, E. , Ciminelli, V. S. T. , Lange, F. T. , Matschullat, J. , Raue, B. , & Schmidt, H. (2002). Soil and sediment geochemistry of the Iron Quadrangle, Brazil: The case of arsenic. Journal of Soils and Sediments , 2 (4), 216–222. https://doi.org/10.1007/BF02991043
- Deschamps, E. , & Matschullat, J. (2007). Arsênio antropogênico e natural. Um estudo em regiões do Quadrilátero Ferrífero. Belo Horizonte . Fundacao Estadual do Meio Ambiente.
- DGA (2017). Información oficial hidrometeorológica y de calidad de aguas en línea (octubre 2010 - noviembre 2017). Dirección General de Aguas, data. http://snia.dga.cl/BNAConsultas/.
- Díaz, S. L. , Blanco, M. D. C. , Schmidt, E. S. , Amiotti, N. M. , & Espósito, M. E. (2015). Geodisponibilidad de arsénico, hierro y sodio en suelos de la cuenca El Divisorio [Buenos Aires, Argentina]. Ciencia Del Suelo , 33 (2), 303–312.
- Díaz, S. L. , Espósito, M. E. , Blanco, M. C. , Amiotti, N. M. , Schmidt, E. S. , Sequeira, M. E. , Paoloni, J. D. , & Nicolli, H. B. (2016). Control factors of the spatial distribution of arsenic and other associated elements in loess soils and waters of the southern Pampa (Argentina). CATENA , 140 , 205–216. https://doi.org/10.1016/j.catena.2016.01.013
- Dietrich, S. , Bea, S. A. , Weinzettel, P. , Torres, E. , & Ayora, C. (2016). Occurrence and distribution of arsenic in the sediments of a carbonate-rich unsaturated zone. Environmental Earth Sciences , 75 (2), 90. https://doi.org/10.1007/s12665-015-4892-7
- Dolci, N. N. , Sá, F. , da Costa Machado, E. , Krul, R. , & Neto, R. R. (2017). Trace elements in feathers and eggshells of brown booby Sula leucogaster in the Marine National Park of Currais Islands, Brazil. Environ Monit Assess , 189 (10), 496 https://doi.org/10.1007/s10661-017-6190-1
- Domínguez, J. P. , Moya, M. , Rodríguez, E. , Panameño, P. , & Linares, J. (2015). Evaluación final de riesgos y propuesta de medidas de remediación en 15 pasivos ambientales mineros de El Salvador. Reporte de consultoría presentado al Ministerio de Economía de El Salvador por la firma Biosistemas, S.A. de C.V., Fundación Maquilishuatl, FIAES, MINEC, San Salvador, El Salvador.
- Donohue, J. M. , & Abernathy, C. O. (1999). Exposure to inorganic arsenic from fish and shellfish. In W. R. Chappell, C. O. Abernathy, & R. L. Calderon (Eds.), Arsenic exposure and health effects (pp. 89–98). Oxford, UK: Elsevier.
- Dótor-Almazán, A. , Armienta-Hernández, M. A. , Talavera-Mendoza, O. , & Ruiz, J. (2017). Geochemical behavior of Cu and sulfur isotopes in the tropical mining region of Taxco, Guerrero (southern Mexico). Chemical Geology , 471 , 1–12. https://doi.org/10.1016/j.chemgeo.2017.09.005
- Dudka, S. W. , & Mille, P. (1999). Permissible concentrations of arsenic and lead in soils based on risk assessment. Water, Air, and Soil Pollution , 113 (1/4), 127–132. https://doi.org/10.1023/A:1005028905396
- EC (2015). Commission Regulation (EU) 2015/1006 of 25 June 2015 amending Regulation (EC) No 1881/2006 as regards maximum levels of inorganic arsenic in foodstuffs. Official Journal of the European Union , 161 (14), 1993–1995.
- EFSA (2009). Scientific opinion on arsenic in food. EFSA Journal , 7 (10), 1351–1550.
- EFSA (2010). EFSA Panel on Contaminants in the Food Chain. (CONTAM); Scientific opinion on lead in food. EFSA Journal , 8 (4), 1570.
- EFSA (2014). Dietary exposure to inorganic arsenic in the European population. EFSA Journal , 12 (3), 3597.
- Eisen-Cuadra, A. , Christian, A. D. , Dorval, E. , Broadaway, B. , Herron, J. , & Hanningan, R. E. (2013). Metal geochemistry of a brackish lake: Étang Saumâtre, Haiti. In P. Censi , T. Darrah , Y. Erel (Eds.), Medical geochemistry (pp. 149–166). Springer.
- El Mostrador (2011). Escuela La Greda será clausurada tras confirmarse presencia de arsénico y plomo en los niños - El Mostrador. https://www.elmostrador.cl/noticias/pais/2011/07/29/escuela-la-greda-cerrara-definitivamente-tras-confirmarse-presencia-de-arsenico-y-plomo-en-los-ninos/
- El País (2019). Récord de visitantes en el litoral de termas. https://www.elpais.com.uy/informacion/record-visitantes-litoral-termas.html
- EMBRAPA (2015). Tragédia em Mariana: Produção agropecuária em áreas atingidas está comprometida. Empresa Brasileira de Pesquisa Agropecuária (EMBRAPA). https://ww.embrapa.br/busca-de-noticias/-/noticia/8410974/tragedia-em-mariana-producao-agropecuaria-em-areas-atingidas-esta-comprometida
- ENACAL (2008). Gobierno Sandinista hace realidad el agua potable para Juigalpa. Empresa Nicaragüense de Acueductos y Alcantarillados (ENACAL), Managua, Nicaragua. https://www.enacal.com.ni/proyecto/15-02-2017-1.html
- ENACAL (2011). Proyecto Programa de Abastecimiento de Agua Potable y Alcantarillado Sanitara para San Juan del Sur. ENACAL, Managua, Nicaragua. https://www.enacal.com.ni/proyecto/04-04-2018-6.html.
- ENACAL (2013). Establecimiento de la línea de base sobre la calidad actual del agua y sedimentos de la laguna de Masaya - Año 2012/2013. Empresa Nicaragüense de Acueductos y Alcantarillados (ENACAL), CIRA/UNAN-Managua, Managua, Nicaragua.
- ENVIRON (2011). Preliminary hydrological assessment for the development of an industrial park in Haiti. Project (01-27631), Inter-American Development Bank and the ENVIRON International Corporation, Washington, DC, U.S., 1-31. doi.org/ https://doi.org/10.1007/978-94-007-4372-4_9
- Ersbøll, A. K. , Monrad, M. , Sørensen, M. , Baastrup, R. , Hansen, B. , Bach, F. W. , Tjønneland, A. , Overvad, K. , & Raaschou-Nielsen, O. (2018). Low-level exposure to arsenic in drinking water and incidence rate of stroke: A cohort study in Denmark. Environment International , 120 , 72–80. https://doi.org/10.1016/j.envint.2018.07.040
- Espinosa, A. , Güiza, S. (2005). Mapa de concentración puntual de arsénico en aguas. Plancha 209 Zipaquirá - Atlas Geoquímico de Colombia. Bogotá. Instituto Colombiano de Geología y Minería, Ingeominas. http://recordcenter.sgc.gov.co/B11/22006010002740/documento/pdf/2105027401101000.pdf
- Espinosa, E. , Armienta, M. A. , Cruz, O. , Aguayo, A. , & Ceniceros, N. (2009). Geochemical distribution of arsenic, cadmium, lead and zinc in river sediments affected by tailings in Zimapán, a historical polymetalic mining zone of México. Environmental Geology , 58 (7), 1467–1477. https://doi.org/10.1007/s00254-008-1649-6
- Espinoza, F. , Astudillo, F. , Baeza, L. , Carrasco, F. , Castillo, P. , Lacassie, J. , Miralles, C. , Muñoz, N. , & Ramírez, C. (2016). Base de datos de geoquímica de sedimentos de la Hoja La Serena, región de Coquimbo. Base de Datos (04), Sernageomin, Monografías. Servicio Nacional de Geología y Minería, Santiago de Chile, Chile.
- Esteller, M. , Domínguez, E. , Garrido, S. , & Avilés, M. (2015). Groundwater pollution by arsenic and other toxic elements in an abandoned silver mine, Mexico. Environmental Earth Sciences , 74 (4), 2893–2906. https://doi.org/10.1007/s12665-015-4315-9
- Estrada Guevara, M. (2012). Calidad del agua y manejo de sus diferentes niveles para el óptimo rendimiento del cultivo del arroz, en el Valle de Sébaco, durante el periodo Julio-Diciembre 2011. MSc Thesis, Universidad Nacional Autónoma de Nicaragua, Managua, Nicaragua.
- EU (2013). Commission Regulation (EU) No 1275/2013 of 6 December 2013 amending Annex I to Directive 2002/32/EC of the European Parliament and of the Council as regards maximum levels for arsenic, cadmium, lead, nitrites, volatile mustard oil and harmful botanical impurities Text with EEA relevance; European Union (EU), Brussles, Belgium. http://extwprlegs1.fao.org/docs/pdf/eur129053.pdf.
- FAO (2012). The state of world fisheries and aquaculture . Food and Agriculture Organization of the United Nations. www.fao.org/3/a-i2727e.pdf
- FAO/WHO Codex Alimentarius (1995). General Standard for Contaminants and Toxins in Food and Feed. Adopted in 1995. (CXS 193-1995) Revised in 1997, 2006, 2008, 2009. Amended in 2010, 2012, 2013, 2014, 2015.
- Fariña, S. , Schmidt, G. , & Vassolo, S. (2007). Uso sostenible del sistema acuífero Guaraní en la Región Oriental del Paraguay SEAM/BGR, 2007. Geología e Hidrogeología. Proyecto SAG-PY (2), Asunción, Uruguay.
- Fariña, S. , Larroza, F. , Báez, J. , Cabral, N. , & Mallen, G. (2018). Mapa hidrogeológico del sistema acuífero Yrenda-Toba-Tarijeño (SAYTT) en la República de Paraguay [Paper presentation]. XIV Congreso Latinoamericano de Hidrología Subtérranea y X Congreso Argentino de Hidrogeología, Argentina. Universidad de Salta, 1–9.
- Farnfield, H. R. , Marcilla, A. L. , & Ward, N. I. (2012). Arsenic speciation and trace element analysis of the volcanic Rio Agrio and the geothermal waters of Copahue, Argentina. Science of the Total Environment , 433 , 371–378. https://doi.org/10.1016/j.scitotenv.2012.05.098
- Fernandes, A. R. , Souza, E. S. , de, de Souza Braz, A. M. , Birani, S. M. , & Alleoni, L. R. F. (2018). Quality reference values and background concentrations of potentially toxic elements in soils from the eastern Amazon, Brazil. Journal of Geochemical Exploration , 190 , 453–463. https://doi.org/10.1016/j.gexplo.2018.04.012
- Fernández, Z. (2009). Plan de MIZC para minimizar el azolvamiento en la bahía de Cayo Moa [MSc Thesis]. Universidad de Oriente.
- Figueiredo, B. R. , Cunha, F. G. , Paoliello, M. M. B. , Capitani, E. M. , Sakuma, A. , & Enzweiler, J. (2003). Environment and human exposure to lead, cadmium and arsenic in the Ribeira Valley, southeastern Brazil. Proceedings of the 6th International Symposium on Environmental Geochemistry, Edinburgh, Scotland.
- Figueiredo, B. R. , Borba, R. P. , & Angélica, R. S. (2007). Arsenic occurrence in Brazil and human exposure. Environmental Geochemistry and Health , 29 (2), 109–118. https://doi.org/10.1007/s10653-006-9074-9
- Findley, M. , & Côte, M. (2011). Environment assessment of the USAID/Haiti North Park Power Project. Report of the environmental assessment of the USAID/Haiti North Park Power Project.
- Fiorentino, C. E. , Paoloni, J. D. , Sequeira, M. E. , & Arosteguy, P. (2007). The presence of vanadium in groundwater of southeastern extreme the Pampean region Argentina: Relationship with other chemical elements. Journal of Contaminant Hydrology , 93 (1-4), 122–129. https://doi.org/10.1016/j.jconhyd.2007.02.001
- Flores Rojas, K. J. (2010). Porfido de Cobre del Proyecto Petaquilla área de Botija-Panama. Thesis. Universidad Nacional de Ingeniería, Lima, Peru.
- Freire, C. , Koifman, R. J. , Fujimoto, D. , Souza, V. C. O. , Barbosa, F., Jr. , & Koifman, S. (2015). Reference values of cadmium, arsenic and manganese in blood and factors associated with exposure levels among adult population of Rio Branco, Acre, Brazil. Chemosphere , 128 , 70–78. https://doi.org/10.1016/j.chemosphere.2014.12.083
- Freitas, E. T. F. , Montoro, L. A. , Gasparon, M. , & Ciminelli, V. S. T. (2015). Natural attenuation of arsenic in the environment by immobilization in nanostructured hematite. Chemosphere , 138 , 340–347. https://doi.org/10.1016/j.chemosphere.2015.05.101
- Freitas, L. A. , Rambo, C. L. , Franscescon, F. , Barros, A. F. P. , Lucca, G. S. , Siebel, A. M. , Scapinello, J. , Lucas, E. M. , & Magro, J. D. (2017). Coal extraction causes sediment toxicity in aquatic environments in Santa Catarina, Brazil. Revista Ambiente & Agua , 12 (4). https://doi.org/10.4136/ambi-agua.2036
- Fuentes, G. , Viñals, J. , & Herreros, O. (2009). Hydrothermal purification and enrichment of Chilean copper concentrates. Part 2: The behavior of the bulk concentrates. Hydrometallurgy , 95 (1-2), 113–120. https://doi.org/10.1016/j.hydromet.2008.05.004
- Gao, Y. , Baisch, P. , Mirlean, N. , da Silva, F. M. R., Jr. Van Larebeke, N. , Baeyens, W. , & Leermakers, M. (2018). Arsenic speciation in fish and shellfish from the North Sea (Southern Bight) and Açu Port area (Brazil) and health risks related to seafood consumption. Chemosphere , 191 , 89–96. https://doi.org/10.1016/j.chemosphere.2017.10.031
- Garcés, Y. , Betanzon, A. , Lopeztegui, L. , & Artiles, A. (2012). Hydrological characteristics of Cienfuegos Bay, Cuba, related to the presence of the invasive green mussel Perna viridis . International Journal of Marine Science , 2 (2), 12–17. https://doi.org/10.5376/ijms.2012.02.0002
- García, A. , Armienta, M. A. , & Cruz, O. (2001). Sources, distribution and fate of arsenic along the Toliman River, Zimapán, México. In Acreman M.C. (Ed.), Hydro-ecology: Linking hydrology and aquatic ecology , vol. 266, pp. 57–64. IAHS.
- García, D. , Prieto, G. , Páez, I. , Rojas, A. M. , García, G. (2010). Evaluación geoquímica para geología médica en la depresión Momposina. Instituto Colombiano de Geología y Minería – Ingeominas, Bogotá, Colombia. https://catalogo.sgc.gov.co/cgi-bin/koha/opac-detail.pl?biblionumber=49163
- García, M. E. , Betancourt, O. , Cueva, E. , Jean Remy, D. , & Gimaraes, J. R. (2012). Mining and seasonal variation of the metal’s concentration in the Puyango River basin—Ecuador. Journal of Environmental Protection , 03 (11), 1542–1550. https://doi.org/10.4236/jep.2012.311170
- García, M. G. , Borgnino, L. , Bia, G. , & Depetris, P. J. (2014). Mechanisms of arsenic and fluoride release from Chaco-Pampean sediments (Argentina). International Journal of Environment and Health , 7 (1), 41–57. https://doi.org/10.1504/IJENVH.2014.060122
- García-Guinea, J. , & Harffy, M. (1998). Bolivian mining pollution: Past, present and future. Ambio , 27 (5), 251–253.
- García-Meza, J. V. , Ramos, E. , Carrillo-Chávez, A. , & Duran-de-Bazúa, C. (2004). Mineralogical and chemical characterization of historical mine tailings from the Valenciana mine, Guanajuato, Mexico: Environmental implications. Bulletin of Environmental Contamination and Toxicology , 72 (1), 170–177. https://doi.org/10.1007/s00128-003-0256-1
- García-Meza, J. V. , Carrillo-Chávez, A. , & Morton-Bermea, O. (2006). Sequential extractions on mine tailings samples after and before bioassays: Implications on the speciation of metals during microbial re-colonization. Environmental Geology , 49 (3), 437–448. https://doi.org/10.1007/s00254-005-0101-4
- Gastmans, D. , Veroslavski, G. , Kiang Chang, H. , Marmisolle, J. , & Oleaga, A. (2010). Influence of hydrostratigraphic framework in arsenic occurrence in groundwater along the Uruguay River thermal corridor (Argentine-Brazil-Uruguay). Geociencias , 29 (1), 105–120.
- Gastmans, D. , Chang, H. K. , & Hutcheon, I. (2010). Groundwater geochemical evolution in the northern portion of the Guarani Aquifer System (Brazil) and its relationship to diagenetic features. Applied Geochemistry , 25 (1), 16–36. https://doi.org/10.1016/j.apgeochem.2009.09.024
- Gavilán García, I. C. , Fernández Villagómez, G. , Menchaca Pérez, A. , Barraza Torres, A. , & Gavilán García, A. (2017). Policy proposal for metals speciation in tailings contaminated soils: A case study in Chihuahua, Mexico. Journal of the Mexican Chemical Society , 61 (1), 14–22. https://doi.org/10.29356/jmcs.v61i1.123
- GEMG (2015). Relatório: Avaliação dos efeitos e desdobramentos do rompimento da Barragem de Fundão em Mariana-MG - Decreto nº 46.892/2015, Secretaria de Estado de Desenvolvimento Regional, Política Urbana e Gestão Metropolitana, Governo de Estado de Minas Gerais, Brazil. http://www.saudeesustentabilidade.org.br/wp-content/uploads/2017/04/RELAT%C3%93RIO_GREENPEACE_18.04.17_FINAL.pdf
- Genie Zepeda, E. , & González Zambrana, R. (2017). Efectos a la salud por exposición crónica a arsénico en agua de bebida en habitantes adultos de comunidades rurales del Municipio Larreynaga-Malpaisillo. Monografía, UNAN-León, Facultad de Ciencias Médicas, León, Nicaragua.
- George, C. M. , Sima, L. , Arias, M. H. , Mihalic, J. , Cabrera, L. Z. , Danz, D. , Checkley, W. , & Gilman, R. H. (2014). Arsenic exposure in drinking water: An unrecognized health threat in Peru. Bulletin of the World Health Organization , 92 (8), 565–572. https://doi.org/10.2471/BLT.13.128496
- GIAIA (2016). Relatório técnico – determinação de metais na bacia do Rio Doce (período: Dezembro 2015 a abril 2016); Grupo Independente para Avaliação de Impacto Ambiental (GIAIA), May 2016. http://giaia.eco.br/wp-content/uploads/2016/06/Relatorio–GIAIA_Metais_Vivian_revisto5.pdf
- Gillispie, E. C. , Sowers, T. D. , Duckworth, O. W. , & Polizzotto, M. L. (2015). Soil pollution due to irrigation with arsenic-contaminated groundwater: Current state of science. Current Pollution Reports , 1 (1), 1–12. https://doi.org/10.1007/s40726-015-0001-5
- Ghosh, A. , Awal, M. A. , Majumder, S. , Sikder, M. H. , & Rao, D. R. (2012). Arsenic residues in broiler meat and excreta at arsenic prone areas of Bangladesh. Bangladesh Journal of Pharmacology , 7 (3), 178–185. https://doi.org/10.3329/bjp.v7i3.11435
- Giménez, M. C. , Blanes, P. S. , Buchhamer, E. E. , Osicka, R. M. , Morisio, Y. , & Farías, S. S. (2013). Assessment of heavy metals concentration in arsenic contaminated groundwater of the Chaco Plain, Argentina. ISRN Environmental Chemistry , 2013 , 1–12. https://doi.org/10.1155/2013/930207
- Gómez, C. A. (2002). Monitoreo y atención de intoxicados con arsénico en El Zapote, Municipio de San Isidro - Departamento de Matagalpa, Nicaragua 1994–2002 . UNICEF.
- Gomez, M. L. , Aranibar, J. N. , Wuilloud, R. G. , Rubio, M. C. , Martinez, D. E. , Soria, N. D. , Monasterio, R. , Villagra, P. , & Goirán, S. B. (2014). Hydrogeology and hydrogeochemical modeling in phreatic aquifer of NE Mendoza, Argentina. Journal of Iberian Geology , 40 (3), 521–538. https://doi.org/10.5209/rev_JIGE.2014.v40.n3.43302
- Gomez, L. , Canizo, B. , Lana, B. , Zalazar, G. , Wuilloud, R. , & Aravena, R. (2019). Hydrochemical processes, variability and natural background levels of arsenic in groundwater of northeastern Mendoza, Argentina. Journal of Iberian Geology , 45 (3), 365–382. https://doi.org/10.1007/s41513-018-00099-0
- Gong, Q. , Chen, P. , Shi, R. , Gao, Y. , Zheng, S. A. , Xu, Y. , Shao, C. , & Zheng, X. (2019). Health assessment of trace metal concentrations in organic fertilizer in northern China. International Journal of Environmental Research and Public Health , 16 (6), 1031. https://doi.org/10.3390/ijerph16061031
- González, L. , Rodríguez, G. , Muñoz, R. (2005). Plancha 144 Anexo 144-4 Mapa de concentración puntual sedimentos activos finos de As. Bogotá: Instituto Colombiano de Geología y Minería, Ingeominas. http://recordcenter.sgc.gov.co/B12/23008010002782/mapa/pdf/2105027821300003.pdf.
- Gonzálaez- Hita, L. , Sá nchez, L. , & Mata. I. (1991). Estudio hidrogeoquímico e isotópico del acuífero granular de la Comarca Lagunera. Instituto Mexicano de Tecnología del Agua. Technical Report (unpublished).
- González Hita, L. , Sánchez Díaz, F. , & Mata Arellano, I. (1995). Estudio hidrogeoquímico e isotópico del acuífero granular de la Comarca Lagunera, México. In L. González-Hita , L. Sánchez , & I. Mata . Estudios de Hidrogeología Isotópica en América Latina 1994 – Isotope Hydrology Investigations in Latin America 1994 . IAEA- TECDOC-835, IAEA. pp. 337–376.
- González-Martínez, F. , Sánchez-Rodas, D. , Cáceres, D. , Martínez, M. , Quiñones, L. , & Johnson-Restrepo, B. (2018). Arsenic exposure, profiles of urinary arsenic species, and polymorphism effects of glutathione-s-transferase and metallothioneins. Chemosphere , 212 , 927–936. https://doi.org/10.1016/j.chemosphere.2018.08.139
- González Rodríguez, B. & PIENSA-UNI ( 2016). Mecanismos de contaminación de arsénico en comunidades rurales de Nicaragua. Caso de estudio: Muy Muy, Matagalpa. Report, Managua, Nicaragua.
- Goso, C. , Manganelli, A. , Fernández-Turiel, J. L. , García Valles, M. , Gimeno, D. , & Pérez, C. (2006). Contaminación por arsénico en aguas del acuífero Mercedes (Uruguay). Nota Breve, Revista SUG , 13 , 65–66.
- Goso, C. , Fernández-Turiel, J. L. , Guerequiz, R. , García Valles, M. , Gimeno, D. , Mañay, N. , & Manganelli, A. (2008). Arsénico en algunos acuíferos del Uruguay. Nota Breve. Revista SUG , 15 , 98–99.
- Goso, H. (1972). El Cuaternario Uruguayo (Uruguayan Quaternary). Proyecto, Estudio Levantamiento de Suelos. Ed. Mimeogr.
- Goyenechea, M. (1917). Sobre la nueva enfermedad descubierta en Bell-Ville. Revista Médica de Rosario , 1917 (7), 485.
- Grandia, F. , Salas, J. , Arcos, D. , Archambault, A. , & Cottard, F. (2009). Impacto del drenaje ácido de explotaciones mineras en la cuenca del Río Margajita y Embalse de Hatillo (República Dominicana). Boletín Geológico y Minero , 120 (4), 595–606.
- GRUN & ANA (2010). Ley General de Aguas N° 620 y Reglamento de la Ley General de Aguas Nacionales, Mapa de Cuencas, Decreto (44). Published in: La Gaceta No. 169, 4 September 2007, Gobierno de Reconciliación y Unidad Nacional (GRUN) y Autoridad Nacional del Agua (ANA) Managua, Nicaragua.
- Guilherme, L. R. G. , Ono, F. B. , Cantoni, M. , Abreu, C. A. , Coscione, A. R. , Tappero, R. , & Sparks, D. (2014). Bioaccessibility of arsenic in a gold mine area in Brazil: Why is it so low? In M. I. Litter , H. B. Nicolli , M. Meichtry , N. Quici , J. Bundschuh , P. Bhattacharya , R. Naidu , (Eds.), One Century of the Discovery of Arsenicosis in Latin America (1914–2014) – As2014. Proceedings of the 5th International Congress on Arsenic in the Environment.
- Gutiérrez, M. , & Carreón, E. (2008). Contenido de As, Ba, y Cu en sedimentos y su asociación con depósitos minerales en el noreste de Chihuahua. Medio Ambiente Desarrollo Sustentable , 11 , 108–117.
- Gutiérrez, M. , Martinez-Pina, C. , Luo, J. , & Mickus, K. (2008). Geochemical processes contributing to the contamination of soil and surface waters in the Rio Conchos basin, Mexico. Geosphere , 4 (3), 600–611. https://doi.org/10.1130/GES00160.1
- Gutiérrez, S. (2007). Caracterización química de las patinas negras utilizadas en la momificación de las momias negras de la cultura Chinchorro [Thesis]. Universidad de Tarapacá. Arica, Chile. Chile.
- Gutiérrez-Ojeda, C. (2009). Determining the origin of arsenic in the Lagunera region aquifer, Mexico using geochemical modeling. In J. Bundschuh , M. A. Armienta , P. Birkle , P. Bhattacharya , J. Matschullat , A. B. Mukherjee (Eds.), Natural arsenic in groundwater of Latin America (pp. 163–170.). CRC Press.
- Gutiérrez-Ruiz, M. , Romero, F. M. , & González-Hernández, G. (2007). Suelos y sedimentos afectados por la dispersión de jales inactivos de sulfuros metálicos en la zona minera de Santa Bárbara, Chihuahua, México. Revista Mexicana de Ciencias Geológicas , 24 , 170–184.
- Hagström, A. , & Rydstedt, A. (2015). A hydrochemical Investigation and socioeconomic assessment in Rio Zapomeca River basin focusing on arsenic contamination [MSc Thesis]. Lund University.
- Hall, G. E. M. , Pelchat, J. C. , & Gauthier, G. (1999). Stability of inorganic arsenic (III) and arsenic(V) in water samples. Journal of Analytical Atomic Spectrometry , 14 (2), 205–213. https://doi.org/10.1039/a807498d
- Hammarlund, L. , & Piñones, J. (2009). Arsenic in geothermal waters of Costa Rica. TRITA-LWR (Master Thesis). A Minor Field Study. KTH, Stockholm, Sweden.
- Hatje, V. , Macedo, S. M. , Jesus, R. M. , Cotrim, G. , Garcia, K. S. , Queiroz, A. F. , & Ferreira, S. L. C. (2010). Inorganic As speciation and bioavailability in estuarine sediments of Todos os Santos Bay, BA, Brazil. Marine Pollution Bulletin , 60 (12), 2225–2232. https://doi.org/10.1016/j.marpolbul.2010.08.014
- Hauser, A. (1997). Catastro y caracterización de las fuentes de aguas minerales y termales de Chile. Boletín 50, Servicio Nacional de Geología y Minería, ISSN, 0020–3939.
- Heinzen, W. , Velozo, C. , Carrión, R. , Cardozo, L. , Madracho, H. , & Massa, E. (1986). Carta hidrogeológica del Uruguay, 1:200000, DINAMIGE, Montevideo, Uruguay.
- Heinzen, W. , Carrión, R. , Massa, E. , Pena, S. , Stapff, M. (2003). Mapa hidrogeológico del Uruguay. DINAMIGE. http://www.dinamige.gub.uy/ch25.htm
- Herbel, M. , & Fendorf, S. (2006). Biogeochemical processes controlling the speciation and transport of arsenic within iron coated sands. Chemical Geology , 228 (1-3), 16–32. https://doi.org/10.1016/j.chemgeo.2005.11.016
- Hernández, M. , & Marrugo, J. (2016). Trihalomethanes and arsenic in drinking water in Chinú and Corozal municipalities in Colombia: Health risk assessment. Ingeniería y Desarrollo , 34 , 88–115. Retrieved from http://www.scielo.org.co/pdf/inde/v34n1/v34n1a05.pdf (accessed January 2020).
- Hernández, W. , Marcó, L. , Torres, D. , & Romero, P. (2018). Variabilidad espacial del pH y del contenido de Fe2O3 en suelos de la cuenca del Río Tabure del Estado Lara. Ciencia y Tecnología , 11 (1), 18–27. https://doi.org/10.18779/cyt.v11i1.202
- Hernández-García, Y. (2007). Metales pesados y arsénico en agua y sedimento de embalses artificiales del Estado de Chihuahua México. Chihuahua, Chih., Mexico. MSc Thesis, Facultad de Zootecnia y Ecología. Universidad Autónoma de Chihuahua, Chihuahua, Mexico.
- Hernández-Garcia, Y. , Sosa-Cerecedo, M. , Moreno, M. , Alcalá, J. , & Puga, S. R. (2008). Evaluación de la contaminación por metales pesados y arsénico en el sedimento en embalses del Estado de Chihuahua, México. Revista Latinoamericana de Recursos Naturales , 8 , 89–94.
- Herrera-Murillo, J. , Mora-Campos, D. , Suarez-Serrano, A. , Villalobos-Cháves, M. , Salas-Jiménez, P. , Gamboa-Jiménez, A. , & Anchía-Leitón, D. (2017). Determinación de los niveles de arsénico presentes en sistemas de abastecimiento de agua de las regiones Chorotega y Huetar norte de Costa Rica, América Central. Costa Rica. Universidad Nacional de Costa Rica, San José, Costa Rica.
- Hirata, R. , Gesicki, A. , Sracek, O. , Bertolo, R. , Giannini, P. C. , & Aravena, R. (2011). Relation between sedimentary framework and hydrogeology in the Guarani Aquifer System in São Paulo State, Brazil. Journal of South American Earth Sciences , 31 (4), 444–456. https://doi.org/10.1016/j.jsames.2011.03.006
- Hu, Z. , & Gao, S. (2008). Upper crustal abundances of trace elements: A revision and update. Chemical Geology , 253 (3-4), 205–221. https://doi.org/10.1016/j.chemgeo.2008.05.010
- IAEA & CIRA/UNAN-Managua (2010). Proyecto de Nacional de Cooperación Técnica NIC/8/012. Aplicación de técnicas nucleares en el Plan de Gestión de la Cuenca 69 de los Grandes Lagos de Nicaragua (Informe Final). OIEA and CIRA/UNAN-Managua.
- IBAMA (2015). Laudo técnico preliminar. Impactos ambientais decorrentes do desastre envolvendo o rompimento da barragem de Fundão, em Mariana, Minas Gerais, Instituto Brasileiro do Meio Ambiente e dos Recursos Naturais Renováveis (IBAMA), Brazil. http://www.ibama.gov.br/phocadownload/barragemdefundao/laudos/laudo_tecnico_preliminar_Ibama.pdf.
- IDEAM (2010). Estudio Nacional de Agua. Cap. 5 Estimación de la demanda de agua. http://documentacion.ideam.gov.co/openbiblio/bvirtual/021888/CAP5.pdf.
- IGAM (2016). Monitoramento da qualidade das águas superficiais do Rio doce no Estado de Minas Gerais. Relatório técnico: Acompanhamento da Qualidade das Águas do Rio Doce após o Rompimento da Barragem da Samarco no Distrito de Bento Rodrigues – Mariana/MG. Governo do Estado de Minas Gerais. Sistema Estadual de Meio Ambiente, Instituto Mineiro de Gestão das Águas (IGAM). http://portal1.snirh.gov.br/arquivos/Doce/IGAM/relatorios/RelatorioTecnico_01fev2016.pdf.
- INAA (1996). Informe de actividades de investigación de pozos perforados y excavados en comunidades rurales de San Isidro Matagalpa. Instituto Nicaragüense de Acueductos y Alcantarillados. Nicaragua.
- INCA (2018). Inventario Nacional de Calidad del Agua. www.gapmaps.org.
- INEI Perú (2017). Población y vivienda, Instituto Nacional de Estadística e Informática del Perú.
- INETER (2010). Estudio de Caracterización Hidrogeológica e Hidrogeoquímica de la Subcuenca Mayales.
- INETER, IAEA, & CIRA/UNAN-Managua (2011). Evaluación del impacto de la calidad del agua del Lago Xolotlán sobre el Río Tipitapa y el área inmediata en el Lago Cocibolca. Estación lluviosa Octubre 2010 y Seca Abril 2011. Managua, Nicaragua.
- INETER, ANA, UNI, & GIZ (2014). Delimitación de cuencas hidrográficas en Nicaragua bajo la metodología de Pfafstetter . INETER.
- Ingeominas (1987). Recursos Minerales de Colombia, Tomo I, 2a Ed. Bogotá. Publicaciones Geológicas Especiales de Ingeominas, Instituto Colombiano de Geología y Minería.
- Ingeominas (2009). Mapa de Anomalías Geoquímicas de Colombia. Bogotá. Instituto Colombiano de Geología y Minería. Ingeominas. http://recordcenter.sgc.gov.co/B11/22006100024453/documento/pdf/2105244531101000.pdf.
- INSAPROMA (2014). Proyecto de Fortalecimiento de la Capacidad Institucional en el Manejo Integral de los Residuos Sólidos a Nivel Nacional en la República Dominicana. https://insaproma.com/wp-content/uploads/2016/10/Situación-actual-de-disposición-final-de-la-basura.pdf
- ISS (2017). Avaliação dos riscos em saúde da população de Barra Longa/MG afetada pelo desastre. Instituto Saúde e Sustentabilidade, Brazil. http://www.saudeesustentabilidade.org.br/wp-content/uploads/2017/04/RELAT%C3%93RIO_GREENPEACE_18.04.17_FINAL.pdf
- Jannas, R. R. , Beane, R. E. , Ahler, B. A. , & Brosnahan, D. R. (1990). Gold and copper mineralization at the El Indio deposit, Chile. Journal of Geochemical Exploration , 36 (1-3), 233–266. https://doi.org/10.1016/0375-6742(90)90057-H
- Jara-Marini, M. E. , & García-Rico, L. (2006). Distribution of arsenic in three geochemical fractions of surface sediments from coastal sites of Sonora, Gulf of California, Mexico. Bulletin of Environmental Contamination and Toxicology , 76 (4), 677–683. https://doi.org/10.1007/s00128-006-0973-3
- Jayasumana, C. , Fonseka, S. , Fernando, A. , Jayalath, K. , Amarasinghe, M. , Siribaddana, S. , Gunatilake, S. , Paranagama, P. (2015). Phosphate fertilizer is a main source of arsenic in areas affected with chronic kidney disease of unknown etiology in Sri Lanka. SpringerPlus, 4, 90. Retrieved from https://www.ncbi.nlm.nih.gov/pmc/articles/PMC4348354/.
- Joseph, T. , Dubey, B. , & McBean, E. A. (2015). A critical review of arsenic exposures for Bangladeshi adults. Science of the Total Environment , 527-528 , 540–551. https://doi.org/10.1016/j.scitotenv.2015.05.035
- Kader, M. , Lamb, D. T. , Megharaj, M. , & Naidu, R. (2016). Sorption parameters as a predictor of arsenic phytotoxicity in Australian soils. Geoderma , 265 , 103–110. https://doi.org/10.1016/j.geoderma.2015.11.019
- Kazi, T. G. , Shah, A. Q. , Afridi, H. I. , Shah, N. A. , & Arain, M. B. (2013). Hazardous impact of organic arsenical compounds in chicken feed on different tissues of broiler chicken and manure. Ecotoxicology and Environmental Safety , 87 , 120–123.
- Khan, K. M. , Chakraborty, R. , Bundschuh, J. , Bhattacharya, P. , & Parvez, F. (2020). Health effects of arsenic exposure in Latin America: An overview of the past eight years of research . Science of the Total Environment , 710 , 136071. https://doi.org/10.1016/j.scitotenv.2019.136071
- Kirchheim, R. , Gastmans, D. , Chang, H. K. , & Gilmore, T. E. (2019). The use of isotopes in evolving groundwater circulation models of regional continental aquifers: The case of the Guarani Aquifer System. Hydrological Processes , 33 (17), 2266–2278. https://doi.org/10.1002/hyp.13476
- Kirchmer, C. J. , & Castro, M. L. (1979). Tratamiento para la remoción de arsénico en aguas con alto contenido de Magnesio - Río Locumba, Perú. ACODAL , 89 , 11–30.
- Knee, K. L. , & Encalada, A. C. (2014). Land use and water quality in a rural cloud forest region (Intag, Ecuador), River. River Research and Applications , 30 (3), 385–401. https://doi.org/10.1002/rra.2634
- Kumar, R. , Patel, M. , Singh, P. , Bundschuh, J. , Pittman, C. U., Jr. , Trakal, L. , & Mohan, D. (2019). Emerging technologies for arsenic removal from drinking water in rural and peri-urban areas: Methods, experience from, and options for Latin America . Science of the Total Environment , 694 , 133427. https://doi.org/10.1016/j.scitotenv.2019.07.233
- La Gaceta (2005). Reglamento para la Calidad del Agua Potable. Decreto No 32327-S, La Gaceta (84). https://www.ministeriodesalud.go.cr/gestores_en_salud/comision_agua_segura/legislacion/CAS_reglamento_calidad_agua_potable.pdf
- Lacassie, J. , Astudillo, F. , Baeza, L. , Barrera, J. , Carrasco, F. , Castillo, P. , Espinoza, F. , Figueroa, M. , Muñoz, N. , Ramírez, C. , & Salinas, P. (2014). Base de Datos de Geoquímica de sedimentos de la Hoja Iquique, región de Tarapacá (01), Base de Datos. Sernageomin, Servicio Nacional de Geología y Minería, Santiago de Chile, Chile.
- Lacayo Romero, M. , Cruz, A. , Lacayo, J. , Calero, S. , & Fomsgaard, I. (1991). Arsénico total en sedimentos, peces y agua en el Lago Xolotlán (Managua). Presented at: Taller Regional de Limnología Aplicada al Lago Xolotlán para su Recuperación y Aprovechamiento, 8-10 July 1991, Centro para la Investigaciones en Recursos Acuáticos (CIRA), Managua, Nicaragua.
- Lagomarsino, C. , Quiroz, C. , & Tapia, J. (2018). Geoquímica de As, Cu y Mo en cuencas contrastantes de Chile. XV Congreso Geológico Chileno, Concepción, Chile.
- Larroza, F. , & Fariña, S. (2005). Caracterización hidrogeológica del Sistema Acuífero Yrenda (SAY) en Paraguay: Recurso compartido con Argentina y Bolivia [Paper presentation]. IV Congreso Argentino de Hidrogeología, Río Cuarto, Córdoba. Argentina, II, 125–134.
- LAVELIN (2008). Proyecto de Protección Ambiental y Desarrollo Sostenible del Sistema Acuífero Guaraní. Hidroquímica, resultados finales de laboratorio (SNC LAVALIN). Zona Operativa Sur. Protocolo Base, Anexo 5.
- Lemos, M. J. N. , Nascimento, E. S. , Maihara, V. A. , Silva, P. S. C. , & Landgraf, M. (2014). Evaluation of As, Se and Zn in octopus samples in different points of sales of the distribution chain in Brazil. Journal of Radioanalytical and Nuclear Chemistry , 301 (2), 573–579. https://doi.org/10.1007/s10967-014-3167-1
- Lemus, C. , Honores, C. , Aguilera, F. , Pérez, Y. , Morales, D. , Cáceres, D. , & Neira, H. (2015). Evaluación de los recursos geotérmicos de la Región de Los Ríos. Informe Registrado (IR-15-59). SERNAGEOMIN, Santiago de Chile, Chile.
- Levy Brooks, Y. (2016). Contaminación orgánica e inorgánica de la bahía de Bluefields y evaluación del riesgo por la ingesta de mercurio y plomo a través del consumo de peces y ostiones, 2011–2012. Universidad Nacional Autónoma de Nicaragua. MSc Thesis.
- Lewis, J. F. , Escuder-Viruete, J. , Hernaiz-Huerta, P. P. , Gutiérrez, G. , Draper, G. , & Pérez-Estaún, A. (2002). Subdivisión geoquímica del arco de Isla Circum-Caribeño, Cordillera Central Dominicana: Implicaciones para la formación, acreción y crecimiento cortical en un ambiente intraoceánico. Acta Geologica Hispanica , 37 , 81–122.
- Leybourne, M. I. , & Cameron, E. M. (2008). Source, transport, and fate of rhenium, selenium, molybdenum, arsenic, and copper in groundwater associated with porphyry-Cu deposits, Atacama Desert, Chile. Chemical Geology , 247 (1-2), 208–228. https://doi.org/10.1016/j.chemgeo.2007.10.017
- Libbey, R. B. , Williams-Jones, A. E. , Melosh, B. L. , & Backeberg, N. R. (2015). Characterization of geothermal activity along the North American - Caribbean Plate boundary in Guatemala: The Joaquina geothermal field. Geothermics , 56 , 17–34. https://doi.org/10.1016/j.geothermics.2015.03.002
- Lima, L. , López-Heras, I. , Pedrero, Z. , Olivares Rieumont, S. , Madrid, Y. , Cámara, C. , & De la Rosa, D. (2013). Levels of arsenic, mercury and selenium in Clarias gariepinus from Sagua la Grande River. Annales de Limnologie - International Journal of Limnology , 49 (2), 113–119. https://doi.org/10.1051/limn/2013046
- Limbozzi, F. (2011). Elementos traza en el agua subterránea. Rol de la zona no saturada como fuente de aporte de flúor. PhD Thesis, Universidad Nacional del Sur, Bahia Blanca, Argentina.
- Limón-Pacheco, J. H. , Jiménez-Córdova, M. I. , Cárdenas-González, M. , Sánchez Retana, I. M. , Gonsebatt, M. E. , & Del Razo, L. M. (2018). Potential co-exposure to arsenic and fluoride and biomonitoring equivalents for Mexican children. Annals of Global Health , 84 (2), 257–217. https://doi.org/10.29024/aogh.913
- Litter, M. I. , Armienta, M. A. , & Farías, S. S. (Eds.). (2009). Metodologías analíticas para la determinación y especiación de arsénico en aguas y suelos. CYTED. http://www.cnea.gov.ar/xxi/ambiental/iberoarsen/default.asp.
- Litter, M. I. , Alarcón-Herrera, M. T. , Arenas, M. J. , Armienta, M. A. , Avilés, M. , Cáceres, R. E. , Cipriani, H. N. , Cornejo, L. , Dias, L. E. , Fernández Cirelli, A. , Farfán, E. M. , Garrido, S. , Lorenzo, L. , Morgada, M. E. , Olmos-Márquezd, M. A. , & Pérez-Carrera, A. (2012). Small-scale and household methods to remove arsenic from water for drinking purposes in Latin America. Science of the Total Environment , 429 , 107–121. https://doi.org/10.1016/j.scitotenv.2011.05.004
- Litter, M. I. , Ingallinella, A. M. , Olmos, V. , Savio, M. , Difeo, G. , Botto, L. , Farfán Torres, E. M. , Taylor, S. , Frangie, S. , Herkovits, J. , Schalamuk, I. , González, M. J. , Berardozzi, E. , García Einschlag, F. S. , Bhattacharya, P. , & Ahmad, A. (2019a). Arsenic in Argentina: Occurrence, human health, legislation and determination. Science of the Total Environment , 676 , 756–766. https://doi.org/10.1016/j.scitotenv.2019.04.262
- Litter, M. I. , Ingallinella, A. M. , Olmos, V. , Savio, M. , Difeo, G. , Botto, L. , Farfán Torres, E. M. , Taylor, S. , Frangie, S. , Herkovits, J. , Schalamuk, I. , González, M. J. , Berardozzi, E. , García Einschlag, F. S. , Bhattacharya, P. , & Ahmad, A. (2019b). Arsenic in Argentina: Technologies for arsenic removal from groundwater sources, investment costs and waste management practices. Science of the Total Environment , 690 , 778–789. https://doi.org/10.1016/j.scitotenv.2019.06.358
- Litter, M. I. , Armienta, M. A. , Villanueva Estrada, R. E. , Villaamil Lepori, E. C. , & Olmos, V. (2020). Arsenic in Latin America: Part II. In S. Srivastava (Ed.), Arsenic in drinking water and food . Springer.
- Londoño, L. F. (2014). Heavy metals in dairy herds in the municipalities of San Pedro and Entrerríos, Antioquia, Colombia . Doctoral Thesis. Universidad de León. https://buleria.unileon.es/handle/10612/3667
- Long, G. , Peng, Y. , & Bradshaw, D. (2012). A review of copper-arsenic mineral removal from copper concentrates. Minerals Engineering , 36-38 , 179–186. https://doi.org/10.1016/j.mineng.2012.03.032
- López, D. L. , Ransom, L. , Perez, N. , Hernandez, P. , & Monterrosa, J. (2004). Dynamics of diffuse degassing at Ilopango Caldera, El Salvador. In W. I. Rose , J. J. Bommer , D. L. López , M. J. Carr , J. J. Major (Eds.), Natural Hazards in El Salvador (vol. 375, pp. 191–202). Geological Society of America Special Paper.
- López, D. L. , Ransom, L. , Monterrosa, J. , Soriano, T. , Barahona, F. , Olmos, R. , & Bundschuh, J. (2009). Volcanic arsenic and boron pollution of Ilopango Lake, El Salvador. In J. Bundschuh , M. Armienta , P. Birkle , P. Bhattacharya , J. Matschullat , A.B. Mukherjee (Eds.), Natural Arsenic in Groundwater of Latin America (pp. 129–143). Taylor & Francis.
- López, D. L. , Bundschuh, J. , Birkle, P. , Armienta, M. A. , Cumbal, L. , Sracek, O. , Cornejo, L. , & Ormachea, M. (2012). Arsenic in volcanic geothermal fluids of Latin America. Science of the Total Environment , 429 , 57–75. https://doi.org/10.1016/j.scitotenv.2011.08.043
- López, L. F. (2014). Exploraciones geoquímicas de yacimientos bajo cobertura transportada en el distrito de Inca de Oro [Evolución de Regolito y paisaje e impactos en métodos geoquímicos indirectos. Magíster Thesis]. Universidad de Chile. Atacama, Chile: Santiago de Chile. Chile.
- López, D. L. (2019). El Cierre y Remediación de Minas Abandonadas en El Salvador. Report presented to the Mesa Nacional Frente la Mineria, San Salvador, El Salvador.
- Lücke, O. H. , & Calderón, A. (2016). Characterization of the ashes from the 2014-2015 Turrialba Volcano eruptions by means of scanning electron microscopy and energy dispersive X-ray spectroscopy. Revista Geológica de América Central , 54 , 109–123. https://doi.org/10.15517/rgac.v54i0.23281
- Machado, C. J. S. (2003). Recursos hídricos e cidadania no Brasil: Limites, alternativas e desafios. Ambiente & Sociedade , 6 (2), 121–136. https://doi.org/10.1590/S1414-753X2003000300008
- Machado, I. , Bühl, V. , & Mañay, N. (2019). Total arsenic and inorganic arsenic speciation in groundwater intended for human consumption in Uruguay: Correlation with fluoride, iron, manganese and sulfate. Science of the Total Environment , 681 , 497–502. https://doi.org/10.1016/j.scitotenv.2019.05.107
- Macklin, M. G. , Payne, I. , Preston, D. , & Sedwick, C. (1996). Review of the Porco mine tailings dam burst and associated mining waste problems, Pilcomayo basin, Bolivia. Report to the UK Overseas Development Agency.
- Mahecha, C. , Chaparro, S. , Saray, M. , & Reynoso, K. (2016). Assessment of arsenic and mercury pollution derived from the coal mining in some areas of the Guacheta (Cundinamarca) Municipality. Revista Ciencia Desarrollo e Innovación , 2 , 30–36. https://revistas.udca.edu.co/index.php/rcdi/article/view/489/414.
- Mahlknecht, J. , Steinich, B. , & Navarro de LeÓN, I. (2004). Groundwater chemistry and mass transfers in the Independence aquifer, central Mexico, by using multivariate statistics and mass-balance models. Environmental Geology , 45 (6), 781–795. https://doi.org/10.1007/s00254-003-0938-3
- Mamani, M. , Wörner, G. , & Sempere, T. (2010). Geochemical variations in igneous rocks of the Central Andean orocline (13°S to 18°S): Tracing crustal thickening and magma generation through time and space. Geological Society of America Bulletin , 122 (1-2), 162–182. https://doi.org/10.1130/B26538.1
- Mañay, N. , Goso, C. , Pistón, M. , Fernández-Turiel, J. L. , García Vallés, M. , Rejas, M. , & Guerequiz, R. (2013). Groundwater arsenic content in Raigón aquifer system (San José, Uruguay). Revista SUG , 18 , 20–38.
- Mañay, N. , Pistón, M. , & Goso, C. (2014). Arsenic environmental and health issues in Uruguay: A multidisciplinary approach. In M. Litter , H. B. Nicolli , M. Meichtry , N. Quici , J. Bundschuh , P. Bhattacharya , R. Naidu (Eds.), One Century of the Discovery of Arsenicosis in Latin America (1914-2014). Proceedings of the 5th International Congress on Arsenic in the Environment (pp. 485–487). CRC Press.
- Mañay, N. , Pistón, M. , Cáceres, M. , Pizzorno, P. , & Bühl, V. (2019). An overview of environmental arsenic issues and exposure risks in Uruguay. Science of the Total Environment , 686 , 590–598. https://doi.org/10.1016/j.scitotenv.2019.05.443
- Mandal, B. K. , Ogra, Y. , Anzai, K. , & Suzuki, K. T. (2004). Speciation of arsenic in biological samples. Toxicology and Applied Pharmacology , 198 (3), 307–318. https://doi.org/10.1016/j.taap.2003.10.030
- Manganelli, A. , Goso, C. , Guerequiz, R. , Fernández Turiel, J. L. , García Vallès, M. , Gimeno, D. , & Pérez, C. (2007). Groundwater arsenic distribution in south-western Uruguay. Environmental Geology , 53 (4), 827–834. https://doi.org/10.1007/s00254-007-0695-9
- Manz, M. , & Castro, J. (1997). The environmental hazard caused by smelter slags from the Sta. Maria de la Plaz mining district in Mexico. Environmental Pollution , 98 (1), 7–13. https://doi.org/10.1016/S0269-7491(97)00107-3
- Marcó Parra, L. M. , Vázquez, C. , Macchi H, L. M. , Urdaneta, C. , Amaya, J. , Arroyo Cortez, J. , & Matute, S. (2010). Use of earthworms (Eisenia fetida) and vermicompost in the processing and safe management of hazardous solid and liquid wastes with high metal contents. International Journal of Global Environmental Issues , 10 (3/4), 214–224. https://doi.org/10.1504/IJGENVI.2010.037267
- MARENA (2013). Estudio de calidad y disponibilidad de los recursos hídricos en la subcuenca del Rio Viejo. Final report. Managua, Nicaragua.
- Mariño, E. E. , Ávila, G. T. , Bhattacharya, P. , & Schulz, C. J. (2020). The occurrence of arsenic and other trace elements in groundwaters of the southwestern Chaco-Pampean plain, Argentina. Journal of South American Earth Sciences , 100 , 102547. https://doi.org/10.1016/j.jsames.2020.102547
- Mark, S. (2017). A review of the evidence for melanoma in nine Inca mummies. International Journal of Osteoarchaeology , 27 (4), 573–579. https://doi.org/10.1002/oa.2580
- MARN (2017). Informe de la calidad del agua de los rios de El Salvador. Unidad de Comunicaciones MARN, Ministerio de Ambiente y Recursos Naturales de El Salvador, San Salvador, El Salvador.
- Marschik, R. , Fontignie, D. , Chiaradia, M. , & Voldet, P. (2003). Geochemical and Sr-Nd-Pb-O isotope composition of granitoids of the Early Cretaceous Copiapó plutonic complex (27°30′S), Chile. Journal of South American Earth Sciences , 16 (5), 381–398. https://doi.org/10.1016/S0895-9811(03)00104-4
- Marshall, G. , Ferreccio, C. , Yuan, Y. , Bates, M. N. , Steinmaus, C. , Selvin, S. , Liaw, J. , & Smith, A. H. (2007). Fifty-year study of lung and bladder cancer mortality in Chile related to arsenic in drinking water. Journal of the National Cancer Institute , 99 (12), 920–928. https://doi.org/10.1093/jnci/djm004
- Marshall, B. G. , Marcello, M. , Veiga, R. J. , Kaplan, R. , Adler Miserendino, G. , Schudel, B. A. , Bergquist, J. R. D. , Guimarães, L. , Sobral, G. S. , & Gonzalez-Mueller, C. (2018). Evidence of transboundary mercury and other pollutants in the Puyango-Tumbes River basin, Ecuador-Peru. Environmental Science: Processes & Impacts , 20 (4), 632–641. https://doi.org/10.1039/C7EM00504K
- Martínez Cruz, M. (2008). Geochemical evolution of the acid crater lake of Poás Volcano (Costa Rica): Insights into volcanic-hydrothermal processes [PhD Thesis]. Utrecht University.
- Martínez-Villegas, N. , Briones-Gallardo, R. , Ramos-Leal, J. A. , Avalos-Borja, M. , Castañón- Sandoval, A. D. , Razo-Flores, E. , & Villalobos, M. (2013). Arsenic mobility controlled by solid calcium arsenates: A case study in Mexico showcasing a potentially widespread environmental problem. Environmental Pollution (Barking, Essex: 1987) , 176 , 114–122. https://doi.org/10.1016/j.envpol.2012.12.025
- Masue, Y. , Loeppert, R. H. , & Kramer, T. A. (2007). Arsenate and arsenite adsorption and desorption behavior on coprecipitated aluminum: Iron hydroxides. Environmental Science & Technology , 41 (3), 837–842. https://doi.org/10.1021/es061160z
- Matschullat, J. , Perobelli Borba, R. , Deschamps, E. , Ribeiro Figueiredo, B. , Gabrio, T. , & Schwenk, M. (2000). Human and environmental contamination in the Iron Quadrangle, Brazil. Applied Geochemistry , 15 (2), 181–190. https://doi.org/10.1016/S0883-2927(99)00039-6
- McClintock, T. R. , Chen, Y. , Bundschuh, J. , Oliver, J. T. , Navoni, J. , Olmos, V. , Villaamil Lepori, E. , Ahsan, H. , & Parvez, F. (2012). Arsenic exposure in Latin America: Biomarkers, risk assessments and related health effects. Science of the Total Environment , 429 , 76–91. https://doi.org/10.1016/j.scitotenv.2011.08.051
- McCutcheon, M. (1998). Anthropogenic and volcanic pollution at Coatepeque Lake, El Salvador [BSc Thesis]. Ohio University.
- Mejía-González, M. A. , González-Hita, L. , Briones-Gallardo, R. , Cardona-Benavides, A. , & Soto-Navarro, P. (2014). Mechanisms that release arsenic to the groundwater of the Laguna Region, states of Coahuila and Durango, Mexico. Water Technology and Sciences, V , (1), 71–82.
- Mejia López, R. (2019). Distribución de las concentraciones de metales pesados presentes en agua superficial en El Salvador, 2018. Instituto Nacional de Salud, Unidad de Investigaciones en Salud, Ministerio de Salud, El Salvador.
- Mello, J. , Roy, W. , Talbott, J. , & Stucki, J. (2006). Mineralogy and arsenic mobility in arsenic-rich Brazilian soils and sediments. Journal of Soils and Sediments , 6 (1), 9–19. https://doi.org/10.1065/jss2005.09.144
- Méndez, M. , & Armienta, M. A. (2003). Arsenic phase distribution in Zimapán Mine Tailings, Mexico. Geofísica Internacional , 42 , 131–140.
- Mendoza-Amézquita, E. , Armienta-Hernández, M. A. , Ayora, C. , Soler, A. , & Ramos-Ramírez, E. (2006). Potencial de lixiviación de elementos traza en jales de las minas La Asunción y Las Torres, en el Distrito Minero de Guanajuato, México. Revista Mexicana de Ciencias Geológicas , 23 , 75–83.
- Menezes, M. D. , Bispo, F. H. A. , Faria, W. M. , Gonçalves, M. G. M. , Curi, N. , & Guilherme, L. R. G. (2020). Modeling arsenic content in Brazilian soils: What is relevant? Science of the Total Environment , 712 , 136511. https://doi.org/10.1016/j.scitotenv.2020.136511
- Milián Rodríguez, E. A. (2012). Estudio geoquímico ambiental por contaminación de elementos tóxicos en la explotación del Yacimiento polimetälico San Fernando de Cuba Central [MSc Thesis]. Universidad de Pinar del Río, Cuba.
- Minería Chilena (2015). Encuentran arsénico y plomo en niños cerca del puerto de Antofagasta. Minería Chilena, Santiago de Chile, Chile. Retrieved from http://www.mch.cl/2015/01/23/estudio-determina-presencia-de-arsenico-y-plomo-en-ninos-de-antofagasta/ (accessed 10.31.18).
- Mirlean, N. , Andrus, V. E. , Baisch, P. , Griep, G. , & Casartelli, M. R. (2003). Arsenic pollution in Patos Lagoon estuarine sediments, Brazil. Marine Pollution Bulletin , 46 (11), 1480–1484. https://doi.org/10.1016/S0025-326X(03)00257-1
- Mirlean, N. , Baisch, P. , Travassos, M. P. , & Nassar, C. (2011). Calcareous algae bioclast contribution to sediment enrichment by arsenic on the Brazilian subtropical coast. Geo-Marine Letters , 31 (1), 65–73. https://doi.org/10.1007/s00367-010-0215-x
- Mirlean, N. , Medeanic, S. , Garcia, F. A. , Travassos, M. P. , & Baisch, P. (2012). Arsenic enrichment in shelf and coastal sediment of Brazilian subtropics. Continental Shelf Research , 35 , 129–136. https://doi.org/10.1016/j.csr.2012.01.006
- Mirlean, N. , Garcia, F. , Baisch, P. , Quintana, G. C. , & Agnes, F. (2013). Sandy beaches contamination by arsenic, a result of nearshore sediment diagenesis and transport (Brazilian coastline). Estuarine, Coastal and Shelf Science , 135 , 241–247. https://doi.org/10.1016/j.ecss.2013.10.020
- Mirlean, N. , Baisch, P. , & Diniz, D. (2014). Arsenic in groundwater of the Paraiba do Sul delta, Brazil: An atmospheric source?. Science of the Total Environment , 482-483 , 148–156. https://doi.org/10.1016/j.scitotenv.2014.02.138
- Mirlean, N. , Baisch, P. , Garcia, F. , Seus, E. , Silva-Silveira, E. , & Vicenti, J. (2016). Coralline algae and arsenic fixation in near shore sediments. Regional Studies in Marine Science , 3 , 83–88. https://doi.org/10.1016/j.rsma.2015.06.005
- Mitchell, K. , Forde, M. , & Neptune, A. (2019). Water Quality in the Americas: Caribbean-Grenada. In Water Quality in the Americas. The Inter-American Network of Academies of Sciences IANAS ., pp. 355–367.
- Molina, A. (2004). Estudio hidrogeoquímico en la Comarca Lagunera [México. MSc Thesis]. National Autonomous University of Mexico.
- Mondal, D. , Periche, R. , Tineo, B. , Bermejo, L. A. , Rahman, M. M. , Siddique, A. B. , Rahman, M. A. , Solis, J. L. , & Cruz, G. J. F. (2020). Arsenic in Peruvian rice cultivated in the major rice growing region of Tumbes River basin. Chemosphere , 241 , 125070. https://doi.org/10.1016/j.chemosphere.2019.125070
- Monroy-Torres, R. , Macías, A. E. , Gallaga-Solorzano, J. C. , Santiago-García, E. J. , & Hernández, I. (2009). Arsenic in Mexican children exposed to contaminated well water. Ecology of Food and Nutrition , 48 (1), 59–75. https://doi.org/10.1080/03670240802575519
- Montaño, J. , Gagliardi, S. , & Montaño, M. (2006). Recursos hídricos subterráneos del Uruguay. Boletín Geológico Minero , 117 (1), 201–222.
- Montero-Campos, V. , Quesada-Kimsey, J. , Ledezma-Espinoza, A. , & Sandoval-Mora, J. A. (2010). Determinación de arsénico en abastecimientos de agua para consumo humano de la Provincia de Cartago, Costa Rica. Acta Médica Costarricense. Colegio de Médicos y Cirujanos , 96–101.
- Mora-Alvarado, D. , Urbina-Campos, A. , & Chamizo-García, H. (2015). Estudio ecológico sobre insuficiencia renal crónica y arsénico en las aguas para consumo humano por distritos de Guanacaste. Revista Tecnología en Marcha , 28 (2), 102–115. https://doi.org/10.18845/tm.v28i2.2337
- Morales, I. , Villanueva-Estrada, R. E. , Rodríguez, R. , & Armienta, M. A. (2015). Geological, hydrogeological, and geothermal factors associated to the origin of arsenic, fluoride, and groundwater temperature in a volcanic environment “El Bajío Guanajuatense”, Mexico. Environmental Earth Sciences , 74 (6), 5403–5415. https://doi.org/10.1007/s12665-015-4554-9
- Morales Cabrera, D. , Avendaño Cáceres, E. , Zevallos Ramos, D. , Fernández Prado, J. , & Mendoza Rodas, Z. (2018). Environmental risk due to arsenic and boron in the watersheds Sama y Locumba from Perú. MEDISAN , 22 (4), 406.
- Morales-Arredondo, J. I. , Esteller-Alberich, M. V. , Armienta Hernández, M. A. , & Martínez-Florentino, T. A. K. (2018). Characterizing the hydrogeochemistry of two low-temperature thermal systems in Central Mexico. Journal of Geochemical Exploration , 185 , 93–104. https://doi.org/10.1016/j.gexplo.2017.11.006
- Morales-Simfors, N. , Bundschuh, J. , Herath, I. , Inguaggiato, C. , Caselli, A. T. , Tapia, J. , Choquehuayta, F. E. A. , Armienta, M. A. , Ormachea, M. , Joseph, E. , & López, D. L. (2020). Arsenic in Latin America: A critical overview on the geochemistry of arsenic originating from geothermal features and volcanic emissions for solving its environmental consequences. Science of the Total Environment , 716 , 135564. https://doi.org/10.1016/j.scitotenv.2019.135564
- Morgano, M. A. , Martins, M. C. T. , Rabonato, L. C. , Milani, R. F. , Yotsuyanagi, K. , & Rodriguez-Amaya, D. B. (2010). Inorganic contaminants in bee pollen from southeastern Brazil. Journal of Agricultural and Food Chemistry , 58 (11), 6876–6883. https://doi.org/10.1021/jf100433p
- MPSDS (2014). Resolución 1207 del Ministerio de la Protección Social y Desarrollo Sostenible (MPSDS). Bogotá, Colombia.
- Murray, J. , Kirschbaum, A. , García, M. G. , Borgnino, L. , & Guimaraes, E. M. (2014). Arsenic solid speciation in tailings of the abandoned Pan de Azúcar mine, northwestern Argentina. In One century of the discovery of arsenicosis in Latin America (1914-2014) - As2014: Proceedings of the 5th International Congress on Arsenic in the Environment (pp. 234–235). CRC Press.
- Naranjo-Pulido, A. , Romero-Schmidt, H. , Mendez-Rodríguez, L. , Acosta-Vargas, B. , & Ortega-Rubio, A. (2002). Soil arsenic contamination in the Cape Region, B.C.S., Mexico. Journal of Environmental Biology , 23 (4), 347–352.
- Narváez, J. , Richter, P. , & Toral, M. I. (2007). Preliminary physical chemical characterization of river waters and sediments affected by copper mining activity in central Chile: Application of multivariate analysis. Journal of the Chilean Chemical Society , 52 (3), 1261–1265. https://doi.org/10.4067/S0717-97072007000300016
- NCH 409 (2006). Norma Calidad del Agua Potable, NCH 409/1. http://www.doh.gov.cl/APR/documentos/Documents/Normas%20NCh%20409%20Calidad%20y%20Muestreo%20del%20Agua%20Potable%20EEO.pdf.
- Neary Ross, D. A. (2015). Trace metal content in Panamanian marine turtles, its potential to differentiate populations, and implication for human consumption [MSc Thesis]. McGill University.
- Nelson, C. E. , & Ganoza, J. (2011). Mineralización de oro en la franja aurífera de Veraguas, Panamá. Revista Geológica de América Central , 22 (22), 87–100. https://doi.org/10.15517/rgac.v0i22.8589
- Nelson, C. E. , Proenza, J. A. , Lewis, J. F. , & López-Kramer, J. (2011). The metallogenic evolution of the Greater Antilles. Geologica Acta , 9 (3-4), 229–264.
- Ng, J. C. , Ciminelli, V. , Gasparon, M. , & Caldeira, C. (2019). Health risk apportionment of arsenic from multiple exposure pathways in Paracatu, a gold mining town in Brazil. Science of the Total Environment , 673 , 36–43. https://doi.org/10.1016/j.scitotenv.2019.04.048
- Nicolau, C. , Reich, M. , & Lynne, B. (2014). Physico-chemical and environmental controls on siliceous sinter formation at the high-altitude El Tatio geothermal field, Chile. Journal of Volcanology and Geothermal Research , 282 , 60–76. https://doi.org/10.1016/j.jvolgeores.2014.06.012
- Nicolite, M. , Truccolo, E. C. , Schettini, C. A. F. , & Carvalho, C. E. V. d. (2009). Oscilação do nível de água e a co-oscilação da maré astronômica no baixo estuário do Rio Paraíba do Sul, RJ. Revista Brasileira de Geofísica , 27 (2), 225–239. https://doi.org/10.1590/S0102-261X2009000200006
- Nicolli, H. B. , Garcí a, J. W. , Falcó n, C. M. , & Smedley, P. L. (2011). Mobilization of arsenic and other trace elements of health concern in groundwater from the Salí River Basin, Tucumán Province, Argentina. Environmental Geochemistry and Health. https://doi.org/10.1007/s10653-011-9429-8
- Nicolli, H. B. , Tineo, A. , García, J. W. , & Falcón, C. M. (2005). Caracterización hidrogeoquímica y presencia de arsénico en las aguas subterráneas de la cuenca del Río Salí, Provincia de Tucumán, Argentina. In G. Galindo , J. L. Fernández Turiel , M. A. Parada , D. Gimeno Torrente (Eds.), Arsénico en aguas: Origen, movilidad y tratamiento (pp. 93–102). IV Cong. Hidrogeológico Argentino.
- Nicolli, H. B. , Bundschuh, J. , García, J. W. , Falcón, C. M. , & Jean, J.-S. (2010). Sources and controls for the mobility of arsenic in oxidizing groundwaters from loess-type sediments in arid/semi-arid dry climates - evidence from the Chaco-Pampean Plain (Argentina). Water Research , 44 (19), 5589–5604. https://doi.org/10.1016/j.watres.2010.09.029
- Nicolli, H. B. , García, J. W. , Falcón, C. M. , & Smedley, P. (2012). Mobilization of arsenic and other trace elements of health concern in groundwater from the Salí River Basin, Tucumán Province, Argentina. Environmental Geochemistry and Health , 34 (2), 251–262. https://doi.org/10.1007/s10653-011-9429-8
- Nicolli, H. B. , Bundschuh, J. , Blanco, M. C. , Tujchneider, O. C. , Panarello, H. O. , Dapeña, C. , & Rusansky, J. E. (2012). Arsenic and associated trace-elements in groundwater from the Chaco-Pampean Plain, Argentina: Results from 100 years of research. Science of the Total Environment , 429 , 36–56. https://doi.org/10.1016/j.scitotenv.2012.04.048
- Nieva, N. E. , Borgnino, L. , Locati, F. , & García, M. G. (2016). Mineralogical control on arsenic release during sediment-water interaction in abandoned mine wastes from the Argentina Puna. Science of the Total Environment , 550 , 1141–1151. https://doi.org/10.1016/j.scitotenv.2016.01.147
- Nievas, H. O. , Caruso, M. , Pizzio, F. , Ferri, F. O. , & Pérez, S. (2013). Monitoreo ambiental de aguas superficiales y subterráneas, consideración de áreas sensibles, distrito uranífero Pichiñán Este, Departamento Paso de Indios, Provincia del Chubut. Revista de la Asociación Geológica Argentina , 70 (3), 327–334.
- Niño, L. , Ramón, J. , & Ramón, J. (2016). Physiochemical contamination of aquifers due to leachates from the El Carrasco, Bucaramanga, landfill. Producción + Limpia , 11 , 66–74. http://www.scielo.org.co/pdf/pml/v11n1/v11n1a07.pdf (accessed January 2020).
- Nordberg, G. , Fowler, B.A. , & Nordberg, M. (Eds.). (2007). Handbook on the toxicology of metals . Academic Press.
- Nriagu, J. O. , Bhattacharya, P. , Mukherjee, A. B. , Bundschuh, J. , Zevenhoven, R. , & Loeppert, R. H. (2007). Arsenic in soil and groundwater: An overview. In Trace metals and other contaminants in the environment (vol. 9, pp. 3–60). Elsevier.
- Nuevas Esperanzas (2010). Estudio de arsénico en Telica. Una investigación de la extensión, las causas y los efectos de la contaminación por arsénico en el Municipio de Telica, Departamento de León. Telica, Nicaragua.
- Nuevas Esperanzas (2013). Annual Report. León, Nicaragua.
- Nunes, L. M. , & Otero, X. (2017). Quantification of health risks in Ecuadorian population due to dietary ingestion of arsenic in rice. Environmental Science and Pollution Research International , 24 (35), 27457–27468. https://doi.org/10.1007/s11356-017-0265-y
- OECD (2016). Meat consumption (indicator), The Organization for Economic Co-operation and Development (OECD). https://data.oecd.org/agroutput/meat-consumption.htm-en
- Oelkers, E. H. , & Gislason, S. R. (2001). The mechanism, rates, and consequences of basaltic glass dissolution: I. An experimental study of the dissolution rates of basaltic glass as a function of aqueous Al, Si, and oxalic acid concentration at 25 °C and pH = 3 and 11. Geochimica et Cosmochimica Acta , 65 (21), 3703–3719. https://doi.org/10.1016/S0016-7037(01)00664-0
- Oelkers, E. H. , Golubev, S. V. , Chairat, C. , Pokrovsky, O. S. , & Schott, J. (2009). The surface chemistry of multi-oxide silicates. Geochimica et Cosmochimica Acta , 73 (16), 4617–4634. https://doi.org/10.1016/j.gca.2009.05.028
- Ojeda, G. (2012, October). Determinación del índice de calidad de agua de la Quebrada Tabure bajo los lineamientos del proyecto ARCAL RLA 010. (Trabajo especial de grado Universidad Centroccidental, Lisandro Alvarado). Ingeniería Agronómica. Barquisimeto, Estado Lara, Venezuela.
- Ongley, L. K. , Sherman, L. , Armienta, A. , Concilio, A. , & Ferguson-Salinas, C. (2007). Arsenic in the soils of Zimapán, Mexico. Environmental Pollution (Pollution) , 145 (3), 793–799. https://doi.org/10.1016/j.envpol.2006.05.014
- Ono, F. , Guilherme, L. R. G. , Penido, E. , Carvalho, G. , Hale, B. , Toujaguez, R. , & Bundschuh, J. (2012). Arsenic bioaccessibility in a gold mining area: A health risk assessment for children. Environmental Geochemistry and Health , 34 (4), 457–465. https://doi.org/10.1007/s10653-011-9444-9
- OPS/OMS-Nicaragua, & Nuevas Esperanzas (2011). Estudio de la contaminación del agua por arsénico en el Municipio de Telica, León, Nicaragua.
- Ormachea Muñoz, M. , Wern, H. , Johnsson, F. , Bhattacharya, P. , Sracek, O. , Thunvik, R. , Quintanilla, J. , & Bundschuh, J. (2013). Geogenic arsenic and other trace elements in the shallow hydrogeologic system of Southern Poopó Basin, Bolivian Altiplano. Journal of Hazardous Materials , 262 , 924–940. https://doi.org/10.1016/j.jhazmat.2013.06.078
- Ormachea Muñoz, M. , Bhattacharya, P. , Sracek, O. , Ramos Ramos, O. , Quintanilla Aguirre, J. , Bundschuh, J. , & Maity, J. P. (2015). Arsenic and other trace elements in thermal springs and in cold waters from drinking water wells on the Bolivian Altiplano. Journal of South American Earth Sciences , 60 , 10–20. https://doi.org/10.1016/j.jsames.2015.02.006
- Ormachea Muñoz, M. , Aróstegui, J. L. , Bhattacharya, P. , Sracek, O. , García Moreno, M. E. , Kohfahl, C. , Quintanilla Aguirre, J. , Díaz, J. H. , & Bundschuh, J. (2016). Geochemistry of naturally occurring arsenic in groundwater and surface-water in the southern part of the Poopó Lake basin, Bolivian Altiplano. Groundwater for Sustainable Development , 2-3 , 104–116. https://doi.org/10.1016/j.gsd.2016.04.002
- Ortega-Guerrero, A. (2003). Origin and geochemical evolution of groundwater in a closed-basin clayey aquitard, Northern Mexico. Journal of Hydrology , 284 (1-4), 26–44. https://doi.org/10.1016/S0022-1694(03)00239-7
- Ortega-Guerrero, A. (2017). Evaporative concentration of arsenic in groundwater: Health and environmental implications, La Laguna Region, Mexico. Environ Geochem Health , 39 (5), 987–1003. https://doi.org/10.1007/s10653-016-9866-5
- Ortega-Guerrero, M. A. (2009). Presencia, distribución, hidrogeoquímica y origen de arsénico, fluoruro y otros elementos traza disueltos en agua subterránea, a escala de cuenca hidrológica tributaria de Lerma-Chapala, México. Revista Mexicana de Ciencias Geológicas , 26 (1), 143–161.
- Otero, X. L. , Tierra, W. , Atiaga, O. , Guanoluisa, D. , Nunes, L. M. , Ferreira, T. O. , & Ruales, J. (2016). Arsenic in rice agrosystems (water, soil and rice plants) in Guayas and Los Ríos provinces, Ecuador. Science of the Total Environment , 573 , 778–787. https://doi.org/10.1016/j.scitotenv.2016.08.162
- Othax, N. , Peluso, F. , Castelain, J. G. , Rodríguez, L. , & Dubny, S. (2013). Riesgo sanitario integrado por sustancias presentes en recursos hídricos de Tres Arroyos, Argentina. Acta Bioquímica Clínica Latinoamericana , 47 (4), 681–692.
- Oyarzún, R. , Lillo, J. , Higueras, P. , Oyarzún, J. , & Maturana, H. (2004). Strong arsenic enrichment in sediments from the Elqui watershed, Northern Chile: Industrial (gold mining at El Indio-Tambo district) vs. geologic processes. Journal of Geochemical Exploration , 84 (2), 53–64. https://doi.org/10.1016/j.gexplo.2004.03.002
- Oyarzún, R. , Lillo, J. , Oyarzún, J. , Higueras, P. , & Maturana, H. (2006). Strong metal anomalies in stream sediments from semiarid watersheds in northern Chile: When geological and structural analyses contribute to understanding environmental disturbances. International Geology Review , 48 (12), 1133–1144. https://doi.org/10.2747/0020-6814.48.12.1133
- Padilla Garza, R. A. , Titley, S. R. , & Pimentel B, F. (2001). Geology of the Escondida porphyry copper deposit, Antofagasta Region, Chile. Economic Geology , 96 (2), 307–324. https://doi.org/10.2113/gsecongeo.96.2.307
- Páez-Osuna, F. , Bojórquez-Leyva, H. , Bergés-Tiznado, M. , Rubio-Hernández, O. A. , Fierro-Sañudo, J. F. , Ramírez-Rochín, J. , & León-Cañedo, J. A. (2015). Heavy metals in waters and suspended sediments affected by a mine tailing spill in the upper San Lorenzo River, northwestern México. Bulletin of Environmental Contamination and Toxicology , 94 (5), 583–588. https://doi.org/10.1007/s00128-015-1473-0
- Páez-Sánchez, A. , Alfaro-Cuevas-Villanueva, R. , Cortés-Martínez, R. , & Segovia, N. (2013). Arsenic content and physicochemical parameters of water from wells and thermal springs at Cuitzeo Lake basin, Mexico. IJIRSET , 2 (12), 7731–7740.
- Palma-Fleming, H. , Quiroz, E. , Campillay, C. , Figueroa, M. , Varas, A. , Velásquez, D. , Jara, B. , & Palma-Larrea, X. (2012). Temporal and spatial trends of total aliphatic hydrocarbons of diesel range and trace elements in sediments and mussels of the Corral Bay area, Valdivia, Southern Central Chile. Journal of the Chilean Chemical Society , 57 (2), 1074–1082. https://doi.org/10.4067/S0717-97072012000200003
- Paraguassú, L. , Leite, M. G. P. , Moreira, F. W. A. , Mendonça, F. P. C. , & Eskinazi-Sant’Anna, E. M. (2019). Impacts of mining in artificial lake of Iron Quadrangle-MG: Past marks and changes of the present. Environmental Earth Sciences , 78 (5), 167. https://doi.org/10.1007/s12665-019-8158-7
- Parra, S. , Bravo, M. A. , Quiroz, W. , Moreno, T. , Karanasiou, A. , Font, O. , Vidal, V. , & Cereceda, F. (2014). Distribution of trace elements in particle size fractions for contaminated soils by a copper smelting from different zones of the Puchuncaví Valley (Chile). Chemosphere , 111 , 513–521. https://doi.org/10.1016/j.chemosphere.2014.03.127
- Pazmiño Sánchez, G. F. , & Mendieta Arguello, R. A. (2018). Thesis Influencia de dos sistemas de riego en la absorción de arsénico, y el crecimiento y rendimiento de dos variedaded de arroz (Oryza sativa) bajo siembra directa e indirecta. BSc Escuela Agricola Panamericana, Zamorano, Honduras.
- Pereira, M. dS. S,. Winter, E,. Guimarães, J. R. , Rath, S. , & Fostier, A. H. (2007). A simple voltammetric procedure for speciation and evaluation of As removal from water. Environmental Chemistry Letters , 5 (3), 137–141. https://doi.org/10.1007/s10311-007-0094-1
- Pérez, Y. (1999). Fuentes de aguas termales de la cordillera Andina del centro-sur de Chile (39-42oS). Boletín 54. SERNAGEOMIN, Santiago, Chile.
- Perez Carrera, A. , & Fernandez Cirelli, A. (2013). Niveles de arsénico y vanadio en aguas naturales en el Departamento de Unión, sudeste de la Provincia de Córdoba, Argentina. AUGMDOMUS, 5, Special Number (I), Aguas. ISSN, 1852–1218.
- Pérez Carrera, A. L. , Volpedo, A. , & Fernandez Cirelli, A. (2015). Arsénico. Del agua a los alimentos. Ciencia e Investigación , 65 (2), 37–44.
- Perez Vargas, M. R. (2010). Contaminación del sedimento de presas del municipio de Chihuahua Chihuahua, Chih., Mexico. Bachelor Thesis, Facultad de Zootecnia y Ecología, Universidad Autónoma de Chihuahua.
- Peru (2018). Agua potable quedará libre de arsénico con primera planta tratamiento que operará en Jauja, Plataforma digital única del Gobierno Peruano. https://www.gob.pe/institucion/vivienda/noticias/19685-agua-potable-quedara-libre-de-arsenico-con-primera-planta-tratamiento-que-operara-en-jauja.
- Pesce, A. H. (2002). Thermal spas: An economical development alternative long both sides of the Uruguay River. GHC Bulletin , 22–28.
- Piazza, A. , Di, Rizzo, A. L. , Barberi, F. , Carapezza, M. L. , Astis, G. , de, Romano, C. , & Sortino, F. (2015). Geochemistry of the mantle source and magma feeding system beneath Turrialba Volcano, Costa Rica. Lithos , 232 , 319–335. https://doi.org/10.1016/j.lithos.2015.07.012
- Pinelli, J. A. , Silva, A. G. , Reis, B. J. , Catelani, C. S. , Souza, W. C. , & Nunes, T. C. O. (2011). Relatório técnico - bacia do Rio Paraíba do Sul - subsídios às ações de melhoria da gestão. AGEVAP - Associação Pró-Gestão das Águas da Bacia Hidrográfica do Rio Paraíba do Sul, Brazil.
- Pistón, M. , Mañay, N. , Goso, C. , Fernandez Turiel, J. L. , Rejas, M. , & Gerequiz, R. (2013). Groundwater arsenic content in Raigón Aquifer System (San José, Uruguay). Revista SUG , 18 , 20–38.
- Poleo, G. , Marcó, L. , Piña, R. , Giordani, L. , Segura, Y. , & Torres, G. (2014). Sistema piloto para la gestión del agua, los residuos sólidos y líquidos y su aprovechamiento. Avance. Observador de Conocimiento , 2 (2), 27–34. https://issuu.com/oncti/docs/rocv2n2
- Prado, F. , González, M. E. , Hernández, M. , Guzmán, C. , Chaulon, M. G. , Cóbar, S. , Donis, M. , & Rivera, C. (2016). Preliminary study of total levels of dissolved arsenic in drinking water of different zones of the Municipality of Guatemala, Department of Guatemala. Toxicology Letters , 259 , S122. https://doi.org/10.1016/j.toxlet.2016.07.313
- Prieto, G. (1998). Geochemistry of heavy metals derived from gold-bearing sulphide minerals in the Marmato District (Colombia). Journal of Geochemical Exploration , 64 (1-3), 215–222. https://doi.org/10.1016/S0375-6742(98)00034-X
- Prieto, G. , & García, G. (2005). Atlas geoquímico de Colombia - mapeo de ultra baja densidad (UBD) . Instituto Colombiano de Geología y Minería–Ingeominas. http://recordcenter.sgc.gov.co/B11/22006100020002/documento/pdf/2105200021101000.pdf (accessed February 2019).
- PSG (2015). Muestreo de suelos para las comunas de Quintero y Puchuncaví, Región de Valparaíso. Ministerio Del Medio Ambiente, Licitación ID: 608897-135-LP14.
- PSG (2016). Evaluación confirmatoria sitio específico del riesgo en sectores relacionados con disposición de residuos sólidos domiciliarios (RSD) y asimilables: Vertedero Lepún (Lago Ranco) y Vertedero Asquee (Mariquina), Región de Los Ríos. Ministerio Del Medio Ambiente, Licitación ID 613925-1-LE16.
- Puccia, V. , Limbozzi, F. , & Avena, M. (2015). Arsenic in porewaters of the unsaturated zone of an Argentinean watershed: Adsorption and competition with carbonate as important processes that regulate its concentration. Aquatic Geochemistry , 21 (6), 513–534. https://doi.org/10.1007/s10498-015-9271-1
- Puntoriero, M. L. , Volpedo, A. , & Fernández Cirelli, A. (2014). Riesgo para la población rural en zonas con alto contenido de arsénico. Acta Toxicológica Argentina , 22 (1), 15–22.
- Quino Lima, I. , Ramos Ramos, O. , Ormachea Muñoz, M. , Quintanilla Aguirre, J. , Duwig, C. , Maity, J. P. , Sracek, O. , & Bhattacharya, P. (2020). Spatial dependency of arsenic, antimony, boron and other trace elements in the shallow groundwater systems of the Lower Katari Basin, Bolivian Altiplano. Science of the Total Environment , 719 , 137505. https://doi.org/10.1016/j.scitotenv.2020.137505
- Quino Lima, I. , Ormachea Muñoz, M. , Ramos Ramos, O. E. , Bhattacharya, P. , Quispe Choque, R. , Quintanilla Aguirre, J. , & Sracek, O. (2019). Hydrogeochemical assessment in the Lower Katari Basin. Groundwater for Sustainable Development , 8 , 281–2913. https://doi.org/10.1016/j.gsd.2018.11.013
- Quinteros, E. (2014). Arsénico en agua de consumo en El Salvador. http://www.xeologosdelmundu.org/wp-content/uploads/2015/01/Congreso-Agua-Memoria-Tecnica-2014.pdf
- Quinteros, E. , Ribó, A. , Mejía, R. , López, A. , Belteton, W. , Comandari, A. , Orantes, C. M. , Pleites, E. B. , Hernández, C. E. , & López, D. L. (2017). Heavy metals and pesticide exposure from agricultural activities and former agrochemical factory in a Salvadoran rural community. Environmental Science and Pollution Research , 24 (2), 1662–1676. https://doi.org/10.1007/s11356-016-7899-z
- Ramos-Arroyo, Y. R. , & Siebe, C. (2007). Weathering of sulphide minerals and trace element speciation in tailings of various ages in the Guanajuato mining district, Mexico. CATENA , 71 , 497–506.
- Ramos Ramos, O. E. , Cáceres, L. F. , Ormachea Muñoz, M. R. , Bhattacharya, P. , Quino, I. , Quintanilla, J. , Sracek, O. , Thunvik, R. , Bundschuh, J. , & García, M. E. (2012). Sources and behavior of arsenic and trace elements in groundwater and surface water in the Poopó Lake basin, Bolivian Altiplano. Environmental Earth Sciences , 66 (3), 793–807. https://doi.org/10.1007/s12665-011-1288-1
- Ramos Ramos, O. E. , Rötting, T. S. , French, M. , Sracek, O. , Bundschuh, J. , Quintanilla, J. , & Bhattacharya, P. (2014). Geochemical processes controlling mobilization of arsenic and trace elements in shallow aquifers and surface waters in the Antequera and Poopó mining regions, Bolivian Altiplano. Journal of Hydrology , 518 , 421–433. https://doi.org/10.1016/j.jhydrol.2014.08.019
- Raychowdhury, N. , Mukherjee, A. , Bhattacharya, P. , Johannesson, K. , Bundschuh, J. , Bejarano Sifuentes, G. , Nordberg, E. , Martin, R. A. , & Storniolo, A. R. (2014). Provenance and fate of arsenic and other solutes in the Chaco-Pampean Plain of the Andean foreland, Argentina: From perspectives of hydrogeochemical modeling and regional tectonic setting. Journal of Hydrology , 518 , 300–316. https://doi.org/10.1016/j.jhydrol.2013.07.003
- Razo, I. , Carrizales, L. , Castro, J. , Díaz-Barriga, F. , & Monroy, M. (2004). Arsenic and heavy metal pollution of soil, water and sediments in a semi-arid climate mining area in Mexico. Water, Air, & Soil Pollution , 152 (1-4), 129–152. https://doi.org/10.1023/B:WATE.0000015350.14520.c1
- Razo, I. , Téllez, J. , Monroy, M. , Carrizales, L. , Díaz-Barriga, F. , & Castro, J. (2004). As and Pb bioaccesibility in polluted soils from a mining site under semiarid climate in Mexico. Proceedings Tailings and Mine Waste’04. Taylor & Francis Group, 173–182.
- Redon, P.-O. , Bur, T. , Guiresse, M. , Probst, J.-L. , Toiser, A. , Revel, J.-C. , Jolivet, C. , & Probst, A. (2013). Modelling trace metal background to evaluate anthropogenic contamination in arable soils of south-western France. Geoderma , 206 , 112–122. https://doi.org/10.1016/j.geoderma.2013.04.023
- Reimann, C. , & De Caritat, P. (2000). Intrinsic flaws of element enrichment factors (EFs) in environmental geochemistry. Environmental Science & Technology , 34 (24), 5084–5091. https://doi.org/10.1021/es001339o
- Reuer, M. K. , Bower, N. W. , Koball, J. H. , Hinostroza, E. , De la Torre Marcas, M. E. , Hurtado Surichaqui, J. A. , & Echevarria, S. (2012). Lead, arsenic, and cadmium contamination and its impact on children’s health in La Oroya, Peru. ISRN Public Health , 2012 , 1–12. Article ID 231458. https://doi.org/10.5402/2012/231458
- Reyes-Gómez, V. , Alarcón, M. , Gutiérrez, M. , & Ñunez, D. (2013). Fluoride and arsenic in an alluvial aquifer system in Chihuahua, Mexico: Contaminant levels, potential sources, and co-occurrence. Water, Air, & Soil Pollution , 224 (2), 1–15. https://doi.org/10.1007/s11270-013-1433-4
- Reynoso, L. , & Andriulo, A. (2013). Estado actual de la calidad del agua en la cuenca del arroyo pergamino. INTA. Estación Experimental Agropecuaria Pergamino. http://produccion-animal.com.ar/agua_cono_sur_de_america/45-calidad_agua_cuenca_Pergamino.pdf
- Rezende, P. S. (2009). Avaliação da Distribuição e Mobilidade de Elementos Traço em Sedimentos da Bacia Hidrográfica do Rio São Francisco. Dissertação de Mestrado. Instituto de Ciências Exatas, Departamento de Química Analítica, Universidade Federal de Minas Gerais, Belo Horizonte, Brazil, 128 p.
- Rezende, P. S. , Costa, L. M. , & Windmöller, C. C. (2015). Arsenic mobility in sediments from Paracatu River basin, MG, Brazil. Archives of Environmental Contamination and Toxicology , 68 (3), 588–602. https://doi.org/10.1007/s00244-015-0134-y
- Risacher, F. , & Hauser, A. (2008). Catastro de las principales fuentes de aguas termales de Chile (12302–1). IRD and SERNAGEOMIN, Santiago de Chile, Chile.
- Risacher, F. , Alonso, H. , & Salazar, C. (1999). Geoquímica de aguas en cuencas cerradas: I, II y III regiones - Chile. S.I.T. N° 51, Santiago de Chile, Chile.
- Rivera-Núñez, Z. , Pan, Z. , Dulience, B. , Becker, H. , Steensma, J. , Hobson, A. , Giammar, D. E. , & Iannotti, L. L. (2018). Water metal contaminants in a potentially mineral-deficient population of Haiti. International Journal of Environmental Health Research , 28 (6), 626–634. https://doi.org/10.1080/09603123.2018.1499880
- Roberge, J. , O’Rourke, M. K. , Meza-Montenegro, M. M. , Gutiérrez-Millán, L. E. , Burgess, J. L. , & Harris, R. B. (2012). Binational arsenic exposure survey: Methodology and estimated arsenic intake from drinking water and urinary arsenic concentrations. International Journal of Environmental Research and Public Health , 9 (4), 1051–1067. https://doi.org/10.3390/ijerph9041051
- Rocha, G. H. O. , Lini, R. S. , Barbosa, F., Jr Batista, B. L. , Souza, V. C. O. , Nerilo, S. B. , Bando, E. , Mossini, S. A. G. , & Nishiyama, P. (2015). Exposure to heavy metals due to pesticide use by vineyard farmers. International Archives of Occupational and Environmental Health , 88 (7), 875–880. https://doi.org/10.1007/s00420-014-1010-1
- Rocha-Amador, D. O. , Calderón, J. , Carrizales, L. , Costilla-Salazar, R. , & Pérez-Maldonado, I. N. (2011). Apoptosis of peripheral blood mononuclear cells in children exposed to arsenic and fluoride. Environmental Toxicology and Pharmacology , 32 (3), 399–405. https://doi.org/10.1016/j.etap.2011.08.004
- Rodríguez, R. , Armienta, M. A. , & Mejía Gómez, J. A. (2005). Arsenic contamination of the Salamanca aquifer system in Mexico: A risk analysis. In J. Bundschuh , P. Bhattacharya , D. Chandrasekharam (Eds.), Natural arsenic in groundwater: Occurrence, remediation and management (pp. 77–83). Taylor & Francis Group.
- Rodríguez Alfaro, M. , Araújo do Nascimento, C. W. , Muñiz Ugarte, O. , Montero, A. , De Aguiar Accioly, A. M. , Calero Martin, B. , Limeres Jiménez, T. , & Ginebra Aguilar, M. (2015). First national-wide survey of trace elements in Cuban urban agriculture. Agronomy for Sustainable Development , 37 (27), 1–7.
- Rodriguez Castro, M. C. , Urrea, G. , & Guasch, H. (2015). Influence of the interaction between phosphate and arsenate on periphyton’s growth and its nutrient uptake capacity. Science of the Total Environment , 503-504 , 122–132. https://doi.org/10.1016/j.scitotenv.2014.06.094
- Rodríguez-Oroz, D. , Vidal, R. , Fernandoy, F. , Lambert, F. , & Quiero, F. (2018). Metal concentrations and source identification in Chilean public children’s playgrounds. Environmental Monitoring and Assessment , 190 (12), 703. https://doi.org/10.1007/s10661-018-7056-x
- Romero, F. M. , Armienta, M. A. , Villaseñor, G. , & González, J. L. (2006). Mineralogical constraints on the mobility of arsenic in tailings from Zimapán, Hidalgo, Mexico. International Journal of Environment and Pollution , 26 (1/2/3), 23–40. https://doi.org/10.1504/IJEP.2006.009097
- Romero, F. M. , Armienta, M. A. , Gutiérrez, M. E. , & Villaseñor, G. (2008). Factores geológicos y climáticos que determinan la peligrosidad y el impacto ambiental de jales mineros. Revista Internacional de Contaminación Ambiental , 24 , 43–54.
- Romero, L. , Alonso, H. , Campano, P. , Fanfani, L. , Cidu, R. , Dadea, C. , Keegan, T. , Thornton, I. , & Farago, M. (2003). Arsenic enrichment in waters and sediments of the Río Loa (Second Region, Chile). Applied Geochemistry , 18 (9), 1399–1416. https://doi.org/10.1016/S0883-2927(03)00059-3
- Rosas, I. , Belmont, R. , Armienta, A. , & Baez, A. (1999). Arsenic concentrations in water, soil, milk and forage in Comarca Lagunera, Mexico. Water, Air, and Soil Pollution , 112 (1/2), 133–149. https://doi.org/10.1023/A:1005095900193
- Rosenmeier, M. F. , Hodell, D. A. , Brenner, M. , Curtis, J. H. , Martin, J. B. , Anselmetti, F. S. , Ariztegui, D. , & Guilderson, T. P. (2002). Influence of the vegetation change on hydrology: Implications for paleoclimatic interpretation of lacustrine d18O records. Journal of Paleolimnology , 27 (1), 117–131. https://doi.org/10.1023/A:1013535930777
- Rosolen, V. , De-Campos, A. B. , Govone, J. S. , & Rocha, C. (2015). Contamination of wetland soils and floodplain sediments from agricultural activities in the Cerrado Biome (State of Minas Gerais, Brazil). CATENA , 128 , 203–210. https://doi.org/10.1016/j.catena.2015.02.007
- Rossello, E. A. , Veroslavsky, G. , de Santa Ana, H. , & Rodríguez, P. (2018). Geology of the Río de la Plata and the surrounding areas of Argentina and Uruguay related to the evolution of the Atlantic margin. Journal of South American Earth Sciences , 83 , 147–164. https://doi.org/10.1016/j.jsames.2017.12.010
- Rosso, J. J. , Schenone, N. F. , Pérez Carrera, A. , & Fernández Cirelli, A. (2013). Concentration of arsenic in water, sediments and fish species from naturally contaminated rivers. Environmental Geochemistry and Health , 35 (2), 201–214. https://doi.org/10.1007/s10653-012-9476-9
- Ruggieri, F. , Fernandez-Turiel, J. L. , Saavedra, J. , Gimeno, D. , Polanco, E. , Amigo, A. , Galindo, G. , & Caselli, A. (2012). Contribution of volcanic ashes to the regional geochemical balance: The 2008 eruption of Chaitén Volcano, Southern Chile. Science of the Total Environment , 425 , 75–88. https://doi.org/10.1016/j.scitotenv.2012.03.011
- Ruiz-Huerta, E. A. , de la Garza Varela, A. , Gómez-Bernal, J. M. , Castillo, F. , Avalos-Borja, M. , SenGupta, B. , & Martínez-Villegas, N. (2017). Arsenic contamination in irrigation water, agricultural soil and maize crop from an abandoned smelter site in Matehuala, Mexico. Journal of Hazardous Materials , 339 , 330–339.
- Sadiq, M. , Zaidi, T. H. , & Mian, A. A. (1983). Environmental behavior of arsenic in soils: Theoretical. Water, Air, & Soil Pollution , 20 (4), 369–377. https://doi.org/10.1007/BF00208511
- Sakuma, A. M. A. (2004). Avaliação da exposição humana ao arsênio no Alto Vale do Ribeira , Brasil. Doctoral Thesis, Universidade Estadual de Campinas.
- Sánchez-Yañez, C. , Reich, M. , Leisen, M. , Morata, D. , & Barra, F. (2017). Geochemistry of metals and metalloids in siliceous sinter deposits: Implications for elemental partitioning into silica phases. Applied Geochemistry , 80 , 112–133. https://doi.org/10.1016/j.apgeochem.2017.03.008
- Sandali, G. , & Diez, E. (2004). Determinación del contenido de arsénico en agua de consumo humano en la Provincia del Chubut. Acta Toxicológica Argentina , 12 , 1–46.
- Santos, E. C. d O. , Jesus, I. M. d. , Brabo, E. d S. , Fayal, K. F. , Sá Filho, G. C. , Lima, M. d O. , Miranda, A. M. M. , Mascarenhas, A. S. , Sá, L. L. C. d. , Silva, A. P. d. , & Câmara, V. d M. (2003). Exposição ao mercúrio e ao arsênio em estados da Amazônia: Síntese dos estudos do Instituto Evandro Chagas/FUNASA. Revista Brasileira de Epidemiologia , 6 (2), 171–185. https://doi.org/10.1590/S1415-790X2003000200010
- Santos, M. J. , Tarley, C. R. T. , Cunha, I. , Zapelini, I. , Galunin, E. , Bleinroth, D. , Vieira, I. , & Abrão, T. (2015). Leachability of major and minor elements from soils and sediments of an abandoned coal mining area in southern Brazil. Environmental Monitoring and Assessment , 187 (3), 1–13. https://doi.org/10.1007/s10661-015-4271-6
- Sariñana-Ruiz, Y. A. , Vazquez-Arenas, J. , Sosa-Rodríguez, F. S. , Labastida, I. , Armienta, M. A. , Aragon-Piña, A. , Escobedo-Bretado, M. A. , Gonzalez-Valdez, L. S. , Ponce-Peña, P. , Ramírez- Aldaba, H. , & Lara, R. H. (2017). Assessment of arsenic and fluorine in surface soil to determine environmental and health risk factors in the Comarca Lagunera, Mexico. Chemosphere , 178 , 391–401. https://doi.org/10.1016/j.chemosphere.2017.03.032
- Scanlon, B. R. , Nicot, J. P. , Reedy, R. C. , Kurtzman, D. , Mukherjee, A. , & Nordstrom, D. K. (2009). Elevated naturally occurring arsenic in a semiarid oxidizing system, Southern High Plains aquifer, Texas, USA. Applied Geochemistry , 24 (11), 2061–2071. https://doi.org/10.1016/j.apgeochem.2009.08.004
- Scarpelli, W. (2010). Arsênio. Alerta de perigo. http://www.cprm.gov.br/publique/media/gestao_territorial/geologia_medica/slides_palestraII.pdf
- Schaefer, C. E. , Andaya, C. , Burant, A. , Condee, C. W. , Urtiaga, A. , Strathmann, T. J. , & Higgins, C. P. (2017). Electrochemical treatment of perfluorooctanoic acid and perfluorooctane sulfonate: Insights into mechanisms and application to groundwater treatment. Chemical Engineering Journal , 317 , 424–432. https://doi.org/10.1016/j.cej.2017.02.107
- Schlebusch, K. , Gattepaille, L. , Engström, K. , Vahter, M. , Jakobsson, M. , & Broberg, K. (2015). Human adaptation to arsenic-rich environments. Molecular Biology and Evolution , 32 (6), 1544–1555. https://doi.org/10.1093/molbev/msv046
- Secretaría de Políticas, Regulación e Institutos y Secretaría de Agricultura, Ganadería y Pesca, Código Alimentario Argentino, Resolución Conjunta 34/2012 y 50/2012 (2012). https://www.ecolex.org/details/legislation/resolucion-conjunta-no-342012-y-502012-prorrogase-el-plazo-de-cinco-anos-previsto-en-los-articulos-982-y-983-del-codigo-alimentario-argentino-lex-faoc110418/
- Segura, F. R. , Nunes, E. A. , Paniz, F. P. , Paulelli, A. C. C. , Rodrigues, G. B. , Braga, G. U. B. , Filho, W. R. P. , Barbosa, F., Jr. , Cerchiaro, G. , Silva, F. F. , & Batista, B. L. (2016). Potential risks of the residue from Samarco’’s mine dam burst (Bento Rodrigues, Brazil). Environmental Pollution , 218 , 813–825. https://doi.org/10.1016/j.envpol.2016.08.005
- Semana (2019). 48 municipios se declararon en calamidad pública por desabastecimiento de agua. Revista Semana. https://sostenibilidad.semana.com/impacto/articulo/48-municipios-se-declararon-en-calamidad-publica-por-desabastecimiento-de-agua/42929
- SERNAGEOMIN (2017). Datos de geoquímica de depósitos de relaves de Chile. Servicion Nacional de Geología y Minería. https://www.sernageomin.cl/deposito-de-relaves/
- SGC . (2016). Atlas Geoquímico de Colombia. Concentración de Arsénico (As). Bogotá: Servicio Geológico Colombiano (SGC). http://srvags.sgc.gov.co/Archivos_Geoportal/Recursos_Minerales/UNIDOS_ATLAS/As.pdf
- Shumilin, E. , Páez-Osuna, F. , Green-Ruiz, C. , Sapozhnikov, D. , Rodrı́guez-Meza, G. D. , & Godı́nez-Orta, L. , (2001). Arsenic, antimony, selenium and other trace elements in sediments of the La Paz Lagoon, Peninsula of Baja California Mexico. Marine Pollution Bulletin , 42 (3), 174–178. https://doi.org/10.1016/S0025-326X(00)00123-5
- Shumilin, E. , Mirlean, N. , Choumiline, K. , & Ostrooumov, M. (2015). Increasing arsenic mobility in the fine fraction of the dry stream sediments of the semi-arid San Antonio gold mining district (Baja California peninsula, Mexico). Environmental Earth Sciences , 73 (8), 4689–4700. https://doi.org/10.1007/s12665-014-3753-0
- Sierra, L. (2019). Nuevas metodologías en la exploración del agua subterránea en base a la investigación del origen y la movilidad del arsénico en el acuífero Pampeano PhD Thesis, Facultad de Ciencias Exactas, Físicas y Naturales, Universidad Nacional de Córdoba, Córdoba, Argentina. ISBN 978-987-86-2042-2048.
- Sierra, L. , Weinzettel, P. , Dietrich, S. , Bea, S. , & Cacciabue, L. (2016). Caracterización del acuífero pampeano mediante la utilización del perfilaje de espectroscopía gamma natural en una perforación de estudio. IX Congreso Argentino de Hidrogeología y VII Seminario Hispano-Latinoamericano Sobre Temas Actuales de la Hidrología Subterránea, 248–256.
- Sierra, L. , Weinzettel, P. , Dietrich, S. , Cacciabue, L. , Bea, S. , Basso, M. , & Kruse, E. (2018). Estudio de la variabilidad de la concentración de Arsénico en un sector experimental de la cuenca del Arroyo Claromecó [Paper presentation]. Hidrología Regional Actas Del XIV Congreso Latinoamericano de Hidrogeología, I, Salta, Argentina.
- Silva, D. C. , Bellato, C. R. , Marques Neto, J. O. , & Fontes, M. F. (2018). Arsenic and trace metals in water and sedimento of the Velhas River, southeastern Iron Quadrangle region, Minas Gerais, Brazil. Química Nova , 41 (9), 1011–1018. https://doi.org/10.21577/0100-4042.20170275
- Silva, Y. J. A. B. , Nascimento, C. W. A. , Cantalice, J. R. B. , Silva, Y. J. A. B. , & Cruz, C. M. C. A. (2015). Watershed-scale assessment of background concentrations and guidance values for heavy metals in soils from a semiarid and coastal zone of Brazil. Environmental Monitoring and Assessment , 187 (9), 1–10. https://doi.org/10.1007/s10661-015-4782-1
- Sindico, F. , Hirata, R. , & Manganelli, A. (2018). The Guarani Aquifer System: From a beacon of hope to a question mark in the governance of transboundary aquifers. Journal of Hydrology: Regional Studies , 20 , 49–59. https://doi.org/10.1016/j.ejrh.2018.04.008
- Smedley, P. L. , & Kinniburgh, D. G. (2002). A review of the source, behaviour and distribution of arsenic in natural waters. Applied Geochemistry , 17 (5), 517–568. https://doi.org/10.1016/S0883-2927(02)00018-5
- Smedley, P. L. , Nicolli, H. B. , Macdonald, D. M. J. , Barros, A. J. , & Tullio, J. O. (2002). Hydrogeochemistry of arsenic and other inorganic constituents in groundwater from La Pampa, Argentina. Applied Geochemistry , 17 (3), 259–284. https://doi.org/10.1016/S0883-2927(01)00082-8
- Smedley, P. L. , Kinniburgh, D. G. , Macdonald, D. M. J. , Nicolli, H. B. , Barros, A. J. , Tullio, J. O. , Pearce, J. M. , & Alonso, M. S. (2005). Arsenic associations in sediments from the loess aquifer of La Pampa, Argentina. Applied Geochemistry , 20 (5), 989–1016. https://doi.org/10.1016/j.apgeochem.2004.10.005
- Smith, A. H. , Goycolea, M. , Haque, R. , & Biggs, M. L. (1998). Marked increase in bladder and lung cancer mortality in a region of Northern Chile due to arsenic in drinking water. American Journal of Epidemiology , 147 (7), 660–669. https://doi.org/10.1093/oxfordjournals.aje.a009507
- SNIS (2015). Diagnóstico SNIS de 2015. Sistema Nacional de Informação sobre Saneamento (SNIS), Brazil. http://www.snis.gov.br/diagnostico-residuos-solidos/diagnostico-rs-2015.
- Sosa, N. N. , Kulkarni, H. V. , Datta, S. , Beilinson, E. , Porfido, C. , Spagnuolo, M. , Zárate, M. , & Surber, J. (2019). Occurrence and distribution of high arsenic in sediments and groundwater of the Claromecó fluvial basin, southern Pampean Plain (Argentina). Science of the Total Environment , 695 , 133673. https://doi.org/10.1016/j.scitotenv.2019.133673
- Soto, C. (2010). Hidrogeología e hidroquímica de aguas subterráneas en el Distrito Inca de Oro, Región de Atacama: Procesos de interacción agua-roca y dispersión geoquímica . M.Sc. Thesis, Universidad de Chile.
- Souza, A. C. M. , Almeida, M. G. , Pestana, I. A. , & Souza, C. M. M. (2019). Arsenic exposure and effects in humans: A mini-review in Brazil. Archives of Environmental Contamination and Toxicology , 76 , 357–365.
- Soza Ferrufino, E. (2020). Disponibilidad del potencial hídrico y la contaminación por arsénico con fines de gestión sostenible de la cuenca El Sauce, Departamento de León . M.Sc. Thesis, Universidad Nacional Autónoma de Nicaragua.
- Sracek, O. , & Hirata, R. (2002). Geochemical and stable isotopic evolution of the Guarani Aquifer System in the State of São Paulo, Brazil. Hydrogeology Journal , 10 (6), 643–655. https://doi.org/10.1007/s10040-002-0222-8
- Sracek, O. , Novák, M. , Sulovský, P. , Martin, R. , Bundschuh, J. , & Bhattacharya, P. (2009). Mineralogical study of arsenic-enriched aquifer sediments at Santiago del Estero, northwest Argentina. In J. Bundschuh , M. A. Armienta , P. Birkle , P. Bhattacharya , J. Matschullat , A. B. Mukherjee (Eds.), Natural arsenic in groundwater of Latin America - Occurrence, health impact and remediation (pp. 61–68.). CRC Press/Balkema.
- Sracek, O. , Armienta, M. A. , Rodríguez, R. , & Villaseñor, G. (2010). Discrimination between diffuse and point sources of arsenic at Zimapán, Hidalgo State, Mexico. Journal of Environmental Monitoring: JEM , 12 (1), 329–337. https://doi.org/10.1039/B911873J
- Stern, C. R. (2004). Active Andean volcanism: Its geologic and tectonic setting. Revista Geológica de Chile , 31 (2), 161–206. https://doi.org/10.4067/S0716-02082004000200001
- Stollenwerk, K. G. (2003). Geochemical processes controlling transport of arsenic in groundwater: A review of adsortion. In A. H. Welch , K. G. Stollenwerk (Eds.), Arsenic in groundwater: Geochemistry and occurrence (pp. 68–100). Kluwer Academic Publishers.
- Su, C. M. , & Puls, R. W. (2001). Arsenate and arsenite removal by zerovalent iron: Effects of phosphate, silicate, carbonate, borate, sulfate, chromate, molybdate, and nitrate, relative to chloride. Environmental Science & Technology , 35 (22), 4562–4568. https://doi.org/10.1021/es010768z
- Tablada, Y. (2008, December 28). Cocibolca ya sale grifos Juigalpa. El Nuevo Diario. https://www.elnuevodiario.com.ni/contactoend/36179-cocibolca-ya-sale-grifos-juigalpa/
- Takahashi, Y. , Minamikawa, R. , Hattori, K. H. , Kurishima, K. , Kihou, N. , & Yuita, K. (2004). Arsenic behavior in paddy fields during the cycle of flooded and non-flooded periods. Environmental Science & Technology , 38 (4), 1038–1044. https://doi.org/10.1021/es034383n
- Talavera Mendoza, O. , Yta, M. , Moreno, T. O. , Dótor, A. A. , Flores, M. N. , & Duarte, G. C. (2005). Mineralogy and geochemistry of sulfide-bearing tailings from silver mines in the Taxco, México area to evaluate their potential environmental impact. Geofísica Internacional , 44 , 49–64.
- Talavera Mendoza, O. , Armienta Hernández, M. A. , García Abundis, J. , & Flores Mundo, N. (2006). Geochemistry of leachates from the El Fraile sulfide tailings piles in Taxco, Guerrero, southern Mexico. Environmental Geochemistry and Health , 28 (3), 243–255. https://doi.org/10.1007/s10653-005-9037-6
- Tapia, J. , Davenport, J. , Townley, B. , Dorador, C. , Schneider, B. , Tolorza, V. , & von Tümpling, W. (2018). Sources, enrichment, and redistribution of As, Cd, Cu, Li, Mo, and Sb in the northern Atacama region, Chile: Implications for arid watersheds affected by mining. Journal of Geochemical Exploration , 185 , 33–51. https://doi.org/10.1016/j.gexplo.2017.10.021
- Tapia, J. , González, R. , Townley, B. , Oliveros, V. , Álvarez, F. , Aguilar, G. , Menzies, A. , & Calderón, M. (2018). Geology and geochemistry of the Atacama Desert. Antonie Van Leeuwenhoek , 111 (8), 1273–1291. https://doi.org/10.1007/s10482-018-1024 https://doi.org/10.1007/s10482-018-1024-x
- Tapia, J. , Valdés, J. , Orrego, R. , Tchernitchin, A. , Dorador, C. , Bolados, A. , & Harrod, C. (2018). Geologic and anthropogenic sources of contamination in settled dust of a historic mining port city in northern Chile: Health risk implications. PeerJ , 6 , e4699. https://doi.org/10.7717/peerj.4699
- Tapia, J. , Murray, J. , Ormachea, M. , Tirado, N. , & Nordstrom, D. K. (2019). Origin, distribution, and geochemistry of arsenic in the Altiplano-Puna plateau of Argentina, Bolivia, Chile, and Perú. Science of the Total Environment , 678 , 309–325. https://doi.org/10.1016/j.scitotenv.2019.04.084
- Tapia, J. , Rodríguez, M. P. , Castillo, P. , González, R. , Rodríguez, C. , Valdés, A. , Townley, B. , & Fuentes, G. (2019). Arsenic and copper in Chile and the development of environmental standards. In: Chile: Environmental history, perspectives and challenges . Chapter 7, 241–285, ISBN 978-1-53615-665-2, Nova Publishers.
- Teruggi, M. E. (1957). The nature and origin of Argentine loess. Journal of Sedimentary Research , 27 , 322–332.
- Ticay, S. , Vásquez Hernández, J. A. , Fagoaga, W. A. , Martínez Batres, R. C. , & Joares, O. M. (2015). Inventario y Diagnóstico de 15 Antiguos Trabajos Mineros. Reporte de consultoría presentado al Ministerio de Economía de El Salvador.
- Tobey, J. (2004). Impacts of altered freshwater flows to estuaries: Yuna watershed and Samana Bay estuary. Draft Profile, USAID, Washington. https://www.crc.uri.edu/download/14_LevelOneProfileDraft_SamanaBay_2004.pdf
- Tomaszewska, B. , Bundschuh, J. , Pająk, L. , Dendys, M. , Delgado Quezada, V. , Bodzek, M. , Armienta, M. A. , Muñoz, M. O. , & Kasztelewicz, A. (2020). Use of low-enthalpy and waste geothermal energy sources to solve arsenic problems in freshwater production in selected regions of Latin America using a process membrane distillation – Research into model solutions. Science of the Total Environment , 714 , 136853. https://doi.org/10.1016/j.scitotenv.2020.136853
- Torró, L. , Garcia-Casco, A. , Proenza, J. A. , Blanco-Quintero, I. F. , Gutiérrez-Alonso, G. , & Lewis, J. F. (2016). High-pressure greenschist to blueschist facies transition in the Maimón Formation (Dominican Republic) suggests mid-Cretaceous subduction of the Early Cretaceous Caribbean arc. Lithos , 266-267 , 309–331. https://doi.org/10.1016/j.lithos.2016.10.026
- Torró, L. , Proenza, J. A. , Melgarejo, J. C. , Alfonso, P. , Farré de Pablo, J. , Colomer, J. M. , García-Casco, A. , Gubern, A. , Gallardo, E. , Cazañas, X. , Chávez, C. , Del Carpio, R. , León, P. , Nelson, C. E. , & Lewis, J. F. (2016). Mineralogy, geochemistry and sulfur isotope characterization of Cerro de Maimón (Dominican Republic), San Fernando and Antonio (Cuba) Lower Cretaceous VMS deposits: Formation during subduction initiation of the proto-Caribbean lithosphere within a for-arc. Ore Geology Reviews , 72 (1), 794–817. https://doi.org/10.1016/j.oregeorev.2015.09.017
- Toujaguez, R. , Ono, F. B. , Martins, V. , Cabrera, P. P. , Blanco, A. V. , Bundschuh, J. , & Guilherme, L. R. G. (2013). Arsenic bioaccessibility in gold mine tailings of Delita, Cuba. Journal of Hazardous Materials , 262 , 1004–1013. https://doi.org/10.1016/j.jhazmat.2013.01.045
- Toujague de la Rosa, R. , & Molerio León, L. F. (2013). Análisis multivariado de la contaminación por arsénico en las aguas subterráneas de mina Delita, Cuba. Revista Electrónica de la Agencia de Medio Ambiente , 13 (24), 1–9.
- Tume, P. , Barrueto, K. , Olguin, M. , Torres, J. , Cifuentes, J. , Ferraro, F. , Roca, N. , Bech, J. , Cornejo, O. (2019). The influence of the industrial area on the pollution outside its borders: A case study from Quintero and Puchuncavi districts, Chile. Environmental Geochemistry and Health. https://doi.org/10.1007/s10653-019-00423-2
- Uddh-Söderberg, T. E. , Gunnarsson, S. J. , Hogmalm, K. J. , Lindegård, M. I. B. G. , & Augustsson, A. L. M. (2015). An assessment of health risks associated with arsenic exposure via consumption of homegrown vegetables near contaminated glassworks sites. Science of the Total Environment , 536 , 189–197. https://doi.org/10.1016/j.scitotenv.2015.07.018
- US EPA (2001). Arsenic in drinking water. Fact Sheet: Drinking water standard for arsenic . U.S. Environmental Protection Agency (US EPA). EPA 815-F-00-015.
- Uturunco, C. , Paredes, H. , N. (2019). Informe de Emergencia N° 214 - 05/03/2019/COEN - INDECI/15:20 HORAS (Informe N° 35) Contaminación por arsénico y plomo en el agua subterránea del Distrito de Mórrope - Lambayeque. https://www.indeci.gob.pe/wp-content/uploads/2019/04/INFORME-DE-EMERGENCIA-N%C2%BA-456-13ABR2019-CONTAMINACI%C3%93N-DE-ARS%C3%89NICO-Y-PLOMO-EN-EL-AGUA-EN-EL-DISTRITO-DE-M%C3%93RROPE-LAMBAYEQUE-39.pdf
- Valdés, J. , & Tapia, J. (2019). Spatial monitoring of metals and As in coastal sediments of northern Chile: An evaluation of background values for the analysis of local environmental conditions. Marine Pollution Bulletin , 145 , 624–640. https://doi.org/10.1016/j.marpolbul.2019.06.036
- Vargas, O. , González, L. M. , Prieto, G. , Espinosa, A. , Matamoros, A. , Sánchez, L. H. , Perilla, C. E. , Garzón, T. , García, G. I. (2001). Levantamiento geoquímico en la Plancha 5-09 - Informe Técnico. Bogotá: Instituto Colombiano de Geología y Minería - Ingeominas. http://recordcenter.sgc.gov.co/B3/12007100002656/documento/pdf/0101026561103000.pdf.
- Vargas, O. , Prieto, G. , González, L. M. , & Matamoros, A. (2004). Geoquímica de metales pesados en suelos de la cuenca del Río Bogotá. Instituto Colombiano de Geología y Minería - Ingeominas. http://recordcenter.sgc.gov.co/B11/23008001024383/Documento/pdf/Geoqu%C3%ADmica%20de%20Metales%20Pesados%20en%20Suelos%20de%20la%20Cuenca%20del%20r%C3%ADo%20Bogot%C3%A1.pdf.
- Vásquez-Prada, B. D. , Ortega, J. , Alonso Marín, E. , Cerrato, D. , & Sánchez Navarro, J. A. (2008). Estudio hidrogeológico de la Laguna de Apoyo (Nicaragua) y propuestas para su gestión. Boletín Geológico y Minero , 119 (1), 137–148.
- Vega, A. S. , Lizama, K. , & Pastén, P. A. (2018). Water quality: Trends and challenges. In G. Donoso (Ed.), Water policy in Chile. Global issues in water policy (vol. 21). Springer.
- Vesga, A. M. , Peñalosa, P. (2018). Reporte de avance del Estudio Nacional del Agua - ENA (2018). Instituto de Hidrología, Meteorología y Estudios Ambientales – IDEAM, Bogotá, Colombia. www.andi.com.co/Uploads/Cartilla_ENA_%202018.pd.
- Villa-Achupallas, M. , Rosado, D. , Aguilar, S. , & Galindo-Riaño, M. D. (2018). Water quality in the tropical Andes hotspot: The Yacuambi River (southeastern Ecuador). Science of the Total Environment , 633 , 50–58. https://doi.org/10.1016/j.scitotenv.2018.03.165
- Villaamil Lepori, E. C. (2015). Hidroarsenicismo crónico regional endémico en Argentina. Acta Bioquímica Clínica Latinoamericana , 49 , 83–104.
- Villa-Gonzales, G. F. , Huamaní-Pacsi, C. , Chávez-Ruiz, M. , & Huamaní-Azorza, J. A. (2018). [Evaluation of the arsenic removal in superficial waters using home filters]. Rev Peru Med Exp Salud Publica , 35 (4), 652–526. https://doi.org/10.17843/rpmesp.2018.354.3715
- Villalobos-Castañeda, B. , Alfaro-Cuevas, R. , Cortés-Martínez, R. , Martínez-Miranda, V. , & Márquez- Benavides, L. (2010). Distribution and partitioning of iron, zinc, and arsenic in surface sediments in the Grande River mouth to Cuitzeo Lake, Mexico. Environmental Monitoring and Assessment , 166 (1-4), 331–346. https://doi.org/10.1007/s10661-009-1005-7
- Villanueva-Estrada, R. E. , Prol-Ledesma, R. M. , Rodríguez-Díaz, A. A. , Canet, C. , & Armienta, M. A. (2013). Arsenic in hotsprings of Bahía Concepción, Baja California Peninsula, México. Chemical Geology , 348 , 27–36. https://doi.org/10.1016/j.chemgeo.2012.09.008
- Vital, M. , Daval, D. , Clément, A. , Quiroga, S. , Fritz, B. , & Martinez, D. E. (2018). Importance of accessory minerals for the control of water chemistry of the Pampean aquifer, Province of Buenos Aires, Argentina. CATENA , 160 , 112–123. https://doi.org/10.1016/j.catena.2017.09.005
- Vital, M. , Martínez, D. E. , Babay, P. , Quiroga, S. , Clément, A. , & Daval, D. (2019). Control of the mobilization of arsenic and other natural pollutants in groundwater by calcium carbonate concretions in the Pampean Aquifer, southeast of the Buenos Aires Province, Argentina. Science of the Total Environment , 674 , 532–543. https://doi.org/10.1016/j.scitotenv.2019.04.151
- Volpedo, A. , Puntoriero, M. L. , & Fernández Cirelli, A. (2013). Riesgo potencial de las altas concentraciones de arsénico en el Lago Chasicó (Buenos Aires, Argentina). Actas 7mo. Congreso de Medio Ambiente AUGM., La Plata, Argentina.
- Vormittag, E. M. P. A. A. , Oliveira, M. A. , & Gleriano, J. S. (2018). Health evaluation of the Barra Longa population affected by the disaster in Mariana county. Ambiente & Sociedade , 21 (0), e01222. https://doi.org/10.1590/1809-4422asoc0122r2vu18l1ao
- VROM (2000). Circular on target values and intervention values for soil remediation, Annex A: Target values, soil remediation intervention values and indicative levels for serious contamination . Dutch Ministry of Housing, Spatial Planning and Environment.
- Wahl, D. , Byrne, R. , Schreiner, T. , & Hansen, R. (2006). Holocene vegetation change in the northern Petén and its implications for Maya prehistory. Quaternary Research , 65 (3), 380–389. https://doi.org/10.1016/j.yqres.2005.10.004
- Ward, N. I. , Lord, G. , Farnfield, H. , O’Reilly, J. , Al Rawahi, W. , Watts, M. J. , & Marcilla, A. L. (2014). In Arsenic speciation analysis of water in Argentina [Paper presentation]. Proceeding of the 5th International Congress on Arsenic in the Environment, Taylor and Francis Group, London, pp. 201–203.
- Watts, M. J. , O’Reilly, J. , Marcilla, A. L. , Shaw, R. A. , & Ward, N. I. (2010). Field based speciation of arsenic in UK and Argentinean water samples. Environmental Geochemistry and Health , 32 (6), 479–490. https://doi.org/10.1007/s10653-010-9321-y
- Wendland, E. , Rabelo, J. , Roehrig, J. (2014). Guarani Aquifer System – The strategical water source in South America: Institute für Tropentechnologie, Germany. http://www.geologiadelparaguay.com/Guaran%C3%AD-Aquifer-System.pdf.
- WHO (2003). Arsenic in drinking-water. Background document for preparation of WHO guidelines for drinking-water quality . World Health Organization. WHO/SDE?WSH?03.04.75.
- WHO (2010). Exposure to arsenic: A major public health concern. In: Preventing disease through healthy environments . World Health Organization. https://www.who.int/ipcs/features/10chemicals_en.
- WHO (2011). Guidelines for drinking-water quality, 4th edition, incorporating the 1st addendum . World Health Organization.
- WHO (2017). Guidelines for drinking-water quality: Fourth edition incorporating first addendum . 4th ed. World Health Organization.
- Wickre, J. B. , Folt, C. L. , Sturup, S. , & Karagas, M. R. (2004). Environmental exposure and fingernail analysis of arsenic and mercury in children and adults in a Nicaraguan gold mining community. Archives of Environmental Health , 59 (8), 400–409. https://doi.org/10.3200/AEOH.59.8.400-409
- Widmer, J. M. , Sergile, F. , Cheremond, Y. , & Morris, G. (2018). A vision for water in Haiti 2018. Emerging Infectious Diseases , 24 (10), 1–21. https://doi.org/10.3201/eid2410.180693
- Wrage, J. , Tardani, D. , Reich, M. , Daniele, L. , Arancibia, G. , Cembrano, J. , Sánchez-Alfaro, P. , Morata, D. , & Pérez-Moreno, R. (2017). Geochemistry of thermal waters in the Southern Volcanic Zone, Chile – Implications for structural controls on geothermal fluid composition. Chemical Geology , 466 , 545–561. https://doi.org/10.1016/j.chemgeo.2017.07.004
- Wurl, J. , Rodríguez, L. C. M. , Cassassuce, F. , Gutiérrez, G. M. , & Velázquez, E. R. (2013). Geothermal water in the San Juan Bautista Londó aquifer, BCS, Mexico. Procedia Earth and Planetary Science , 7 , 900–903. https://doi.org/10.1016/j.proeps.2013.03.124
- Wurl, J. , Mendez-Rodriguez, L. , & Acosta-Vargas, B. (2014). Arsenic content in groundwater from the southern part of the San Antonio-El Triunfo mining district, Baja California Sur, Mexico. Journal of Hydrology , 518 , 447–459. https://doi.org/10.1016/j.jhydrol.2014.05.009
- Wurl, J. , Lamadrid, M. I. , Mendez-Rodriguez, L. , & Acosta-Vargas, B. (2018). Arsenic concentration in the surface water of a former mining area: The La Junta Creek, Baja California Sur, Mexico. International Journal of Environmental Research and Public Health , 15 (3), 437. https://doi.org/10.3390/ijerph15030437
- Wyatt, C. J. , Fimbres, C. , Romo, L. , Méndez, R. O. , & Grijalva, M. (1998). Incidence of heavy metal contamination in water supplies in Northern Mexico. Environmental Research , 76 (2), 114–119. https://doi.org/10.1006/enrs.1997.3795
- Yépez, M. , de, L. A. , Reina, M. , Segura, F. , Amaya, J. , Arrieche, B. , Arroyo, J. , Marcó, L. , & Matute, S. (2007). Determination of Arsenic in sugar cane soils and foliar tissue from Municipio Palavecino, Lara State, Venezuela by hydride generation atomic absorption spectroscopy. Journal of Environmental Science, an Indian Journal , 2 (3), 200–204. https://www.tsijournals.com/articles/a-determination-of-arsenic-in-sugar-cane-soils-and-foliar-tissue-from-municipio-lara-state-venezuela-by-hydride-generati.pdf.
- Zabala, M. E. , Martínez, S. , Manzano, M. , & Vives, L. (2016). Groundwater chemical baseline values to assess the recovery plan in the Matanza-Riachuelo River basin, Argentina. Science of the Total Environment , 541 , 1516–1530. https://doi.org/10.1016/j.scitotenv.2015.10.006
- Zavala, Y. J. , & Duxbury, J. M. (2008). Arsenic in rice: I. Estimating normal levels of total arsenic in rice grain. Environmental Science & Technology , 42 (10), 3856–3860. https://doi.org/10.1021/es702747y
- Zoby, J. L. G. (2008). Panorama da qualidade das águas subterrâneas no Brasil [Paper presentation]. XV Congresso Brasileiro de Águas Subterrâneas, Natal, Brazil.
- Zorrilla Fernández , S. A. (2017). Aplicación de la geoquímica al estudio del vertedero de Duquesa en Santo Domingo, República Dominicana: Propuesta de tratamiento pasivo de los lixiviados. Tesis (Master), E.T.S.I. de Minas y Energía (UPM).
- Zúñiga-Vázquez, D. , Armienta, M. A. , Deng, Y. , Cruz, O. , Aguayo, A. , & Ceniceros, N. (2019). Evaluation of Fe, Zn, Pb, Cd and As mobility from tailings by sequential extraction and experiments under imposed physico-chemical conditions. Geochemistry: Exploration, Environment, Analysis , 19 (2), 129–137. https://doi.org/10.1144/geochem2018-041