ABSTRACT
Introduction
The peripheral stress response, consisting of the autonomic nervous system (ANS) and hypothalamic pituitary adrenal-axis (HPA-axis), functions to maintain homeostasis in response to stressors. Cervical spine manual therapy has been shown to differentially modulate the stress response in healthy populations. No study has investigated whether cervical spine mobilizations can differentially modulate the stress response in individuals with persistent post-concussion symptoms (PPCS), a population characterized by a dysfunctional stress response.
Methods
A randomized, controlled, parallel design trial was performed to investigate whether upper or lower cervical spine mobilization can differentially modulate components of the stress response in individuals with PPCS. The outcomes were salivary cortisol (sCOR) concentration (primary) and the HRV metric, rMSSD, measured with a smartphone application (secondary). Nineteen males diagnosed with PPCS, aged 19–35, were included. Participants were randomly assigned into either intervention group, upper (n = 10) or lower (n = 9) cervical spine mobilization. Each outcome was collected at different time points, pre- and post-intervention. Statistical analyses were performed using the Friedman’s Two-Way ANOVA, Mann-Whitney U test, and Wilcoxon Signed Rank Test.
Results
There was a statistically significant within-group reduction in sCOR concentration 30 minutes following lower cervical spine mobilizations and statistically significant within-group increase in rMSSD 30 minutes following upper cervical spine mobilizations.
Conclusion
The results of this trial provide preliminary evidence for cervical spine mobilizations to differentially modulate components of the stress response at specific time points. Understanding the mechanisms of the effect of cervical spine mobilizations on the stress response provides a novel rationale for selecting cervical spine mobilizations to rehabilitate individuals with PPCS.
Introduction
A dysfunctional stress response may underpin the development and/or maintenance of symptoms in a range of disorders, such as persistent post-concussion symptoms (PPCS) [Citation1,Citation2]. The stress response maintains homeostasis in response to physical, physiological, and/or psychological stressors [Citation3]. The stress response has central and peripheral components [Citation4–7]. Peripheral components consist of the autonomic nervous system (ANS) and hypothalamic pituitary adrenal-axis (HPA-axis), which work bidirectionally and interchangeably [Citation4,Citation8,Citation9]. For the purpose of this trial, ‘stress response’ refers to the peripheral components. In response to a concussion event, the stress response initially activates the faster acting but shorter lasting ANS response, which then communicates with the HPA-axis for the slower-acting but longer lasting endocrine response [Citation3,Citation4,Citation8,Citation9]. An acute stress response is adaptive [Citation10]. Whereas a chronic or dysfunctional stress response, either under or over-active, is maladaptive and may lead to many signs and symptoms, such as executive or cognitive dysfunction, depression, or fatigue [Citation3,Citation9]. These symptoms are commonly observed in those with PPCS [Citation11], consistent with a dysfunctional stress response. Further supporting this concept, ANS dysfunction, indicated by changes in heart rate variability (HRV), has been observed in individuals with PPCS [Citation12–14]. Reduced cortisol levels in individuals with concussion, indicating HPA-axis dysfunction, have been associated with a larger quantity of symptoms, severity of symptoms, and delayed medical clearance [Citation15–18].
Cervical spine manual therapy has the potential to modulate the stress response, in addition to local effects. Cervical spine manual therapy is recommended following concussion [Citation11,Citation19–21]. As well as reducing symptoms characteristic of cervical musculoskeletal dysfunction, such as pain, stiffness, headache, and dizziness [Citation11,Citation22–29], it has been reported that such interventions reduce fogginess and fatigue [Citation30]. Various mechanisms of effects of cervical manual therapy in those with PPCS have been postulated, such as its effect on the local musculoskeletal system or upper cervical afferents [Citation20,Citation31]. It is plausible, however, that individuals with PPCS could benefit from manual therapy applied to the cervical spine due to other mechanisms, such as modulation of the stress response, even in the absence of cervical musculoskeletal dysfunction [Citation32].
Currently, no study has investigated the physiological response of cervical spine manual therapy on peripheral components of the stress response simultaneously or whether there was a differential physiological response depending on the target location of cervical spine manual therapy in individuals with PPCS. This proof-of-concept (POC) trial aimed to explore the physiological effects of a single session of upper or lower cervical spine mobilization in males with PPCS by comparing the immediate and short-term changes in the (1) HPA-axis response, measured with salivary cortisol (sCOR) [Citation33], and (2) ANS response, measured with the HRV metric root mean squared of successive RR intervals (rMSSD) [Citation34]. It is currently unknown whether these physiological changes correlate with changes in clinical symptoms. Therefore, a patient-reported outcome measure, the Rivermead Post Concussion Symptoms Questionnnaire (RPQ), was used as a secondary analysis to [Citation3] explore any possible relationship between changes in the HPA-axis and ANS response with changes in clinical symptoms. This study may provide a deeper understanding of the mechanisms of cervical spine mobilization in a population characterized by a dysfunctional stress response.
Methods
Study design
A POC trial using a randomized-controlled, parallel, repeated measures design was conducted to evaluate the effects of two intervention groups, upper versus lower cervical mobilization, on the HPA-axis (sCOR concentration), the ANS (HRV), and a clinical outcome (RPQ). This study employed a 1:1 allocation ratio, using a block length of six. A parallel design was chosen over a crossover design due the potential for a carryover effect from manual therapy applied to the cervical spine in individuals with PPCS [Citation35]. The study was reported in accordance with the CONSORT 2010 statement: updated guidelines for reporting parallel group randomized trials [Citation36] (Appendix 1) and TIDieR checklist (Appendix 2). Ethical approval was obtained from the Health and Disability Ethics Committee (2022 EXP 12,201). A protocol was registered a priori with Australia New Zealand Clinical Trials Registry (ACTRN12622000479707). All participants signed informed consent prior to enrollment in the study.
Participants
Males with PPCS, aged between 21 and 35, who owned an iPhone, were recruited from the local community. This age range was selected due to the maturation of the ANS before the age of 20 [Citation37], and decline of the endocrine system after the age of 35 [Citation38]. Due to slow recruitment, the inclusion criteria were broadened to males with PPCS, aged between 19 and 35. To be diagnosed with PPCS, individuals must have had an initial diagnosis of concussion or similar term by a physician, with symptoms persisting beyond 14 days [Citation39]. To confirm the medical diagnosis of PPCS within the New Zealand context, the participant must have a national accident insurance (Accident Compensation Corporation, ACC) number relating to their initial diagnosis.
Participants were excluded if they took systemic glucocorticoid or cardioactive medication or had an endocrine, central nervous system, cardiovascular, mental health, co-morbid musculoskeletal, history of serious medical/pathologic or psychiatric disorder, moderate or severe traumatic brain injury, were clinically asymptomatic at any point during the trial before the intervention, or were unable to refrain from external treatment sessions or changes in treatment from days five to seven (). Participants could be prescribed psychotropic medication for treatment of concussion-related symptoms (e.g. headache) and were permitted to enroll in the trial provided they were not prescribed serotonin-norepinephrine reuptake inhibitors (e.g. desvenlafaxine) given the impact of such medication on autonomic function and HRV [Citation40]. Participants were also excluded if they had any contraindications and on a case-by-case basis if they had any precautions to manual therapy [Citation41]. Females were excluded due to the differential effect of sex [Citation42,Citation43] and the menstrual cycle [Citation44–46], on the outcomes of interest.
Figure 1. Timing of outcome measurement. During each intervention, sCOR concentrations and HRV data were collected in clinic at three timepoints, baseline or five-minutes prior to the intervention (pre-5), then five-minutes (post-5) and thirty-minutes (post-30) following the intervention. Baseline sCOR samples were collected at home on day five and six between the hours of 2200–0000 (baseline-pm). sCOR samples were then collected the night following the intervention between the hours of 2200–0000 (post-pm). HRV data was collected each morning on waking, at home, between the hours of 0600–0800. Day two to seven were baseline HRV data (baseline-am). Day eight was post-intervention HRV data (post-am). RPQ data was filled out at the same time as sCOR samples were collected on day six (baseline-pm) and seven (post-pm), and HRV data on day seven (baseline-am) and eight (post-am).
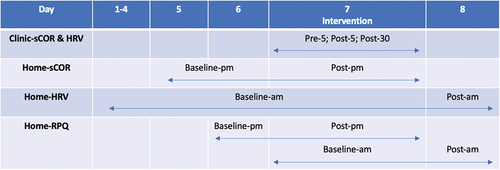
Intervention
A mobilization technique was performed on all participants in the supine position. These techniques consisted of low frequency, mid-range, bilateral, and alternating oscillations of either the upper cervical spine (C0–1 and C1–2) or lower cervical spine (C6–7 and C7-T1) [Citation32]. The frequency was 3 Hz [Citation47]. Each level was mobilized for three sets of two-minutes, separated by a 30-second rest period. All interventions were performed by the same operator, a New Zealand registered physiotherapist with a Master’s degree in orthopedic manipulative therapy and 9 years clinical experience. The mobilization techniques are described in detail in Appendix 3.
Setting
To control for potential contextual influences, each intervention was performed in the same room at the School of Physiotherapy, University of Otago [Citation48] for each participant, between the hours of 1300 and 1700. Temperature was accounted for, and no natural light was allowed to enter the room. The average temperature for the upper cervical mobilization group was 21.69° (0.79 SD), and lower cervical mobilization group was 20.76° (1.23 SD).
Outcome measures data collection
Salivary cortisol
Cortisol is a hormone released into the blood stream by the adrenal cortex and is the end product of the HPA-axis and a proxy measure of HPA-axis activity [Citation49,Citation50]. Following saturation in the blood, cortisol will overflow into saliva [Citation51]. Whole saliva samples were collected with SalivaBio’s 2 mL cryovials and Saliva Collection Aids (Salimetrics, State College, PA) via the unstimulated passive drool technique [Citation33] (Appendix 4). This technique is noninvasive and the most reliable method for collecting sCOR samples [Citation33]. The minimal clinically important difference for cortisol is currently unknown [Citation52].
Participants collected sCOR samples at six time points (). Cortisol exhibits a diurnal decline, with peak concentrations in the morning 30–45 minutes after awakening and a gradual decline over the course of the day, reaching a minimum around midnight [Citation53–55]. Midnight sCOR samples have the highest reliability when assessing chronic HPA-axis tone [Citation56]. The primary outcome was sCOR concentration the night post-intervention (post-pm).
Heart rate variability
HRV is the beat-to-beat variation of R–R intervals from the cardiac cycle [Citation57]. HRV was utilized to provide a noninvasive measure of cardiac ANS activity, predominately parasympathetic nervous system (PSNS) activity [Citation57,Citation58]. An iPhone application, Camera HRV (A.S.M.A. B.V.), was used to measure HRV with a technique called photoplethysmography (PPG). PPG uses the smartphones light to illuminate the skin and detect blood volume variation during a cardiac cycle [Citation59]. PPG is a reliable way to measure HRV and is validated when compared to electrocardiogram, the gold standard of HRV collection [Citation60–62]. Many metrics exist to quantify HRV [Citation57,Citation63]. For this study, rMSSD was chosen as the primary time domain metric to quantify PSNS activity [Citation34]. When measured with PPG, rMSSD has a low standard error of measurement less 6% [Citation64]. The smallest practical or meaningful change for HRV has been reported to be 3% [Citation65]. Appendix 5 outlines various factors accounted for which can affect sCOR and HRV.
Participants collected HRV data at 11 different timepoints (). Baseline measurements were collected over seven consecutive mornings prior to the intervention [Citation66,Citation67]. The day one measurement was omitted from analysis as a practice measurement. The gold standard for HRV measurement is in the middle of the night while the individual is sleeping when PSNS activity is at its highest [Citation68]. This timing is not possible when using a smartphone application; hence, our measurements were taken first thing in the morning upon waking, when the participant should still be in a PSNS dominant state [Citation69,Citation70]. Participants were asked to breath spontaneously [Citation63] and measure their HRV for two-minutes [Citation63,Citation71], in the supine position [Citation61,Citation63], prior to sCOR collection, to minimize the autonomic effect of position change on HRV [Citation72].
Rivermead post-concussion symptoms questionnaire
The RPQ is a valid [Citation73,Citation74] and reliable [Citation75] self-report outcome measure used to assess the severity of 16 of the most reported symptoms post-concussion in comparison to individuals pre-injury baseline [Citation75]. The RPQ was used to ascertain any trend between changes in physiological outcomes (sCOR and HRV) and symptoms after a single intervention. Hence, participants filled out the RPQ at the same time as when their physiological outcomes were taken (). The suggested minimally clinically important difference (MCID) in total RPQ scoring is 4.6 points [Citation76].
Salivary cortisol data analysis
Salivary cortisol samples were analyzed with a Salimetrics ELISA immunoassay kit by a physiologist at a University of Otago physiology laboratory in accordance with the standard procedures (Appendix 6).
Heart rate variability data analysis
Raw RR intervals were exported, deidentified, stored, processed, and cleaned through Kubios HRV Standard version 3.5.0 (Biosignal Analysis and Medical Imaging Group, Kuopio, Finland) (Appendix 7).
Procedure
Refer to Appendix 8 for the procedure.
Randomisation and blinding
At enrollment, each participant selected a sealed opaque envelope containing their participation number to prospectively code saliva collection vials. A research assistant, independent to the outcome assessor, used a computerized random number generator to develop a 1:1 ratio randomization table with a block length of six, linking the participation number into intervention groups, either upper or lower cervical spine mobilization. It was not possible to blind the treating clinician [Citation77] and is unlikely the participants were blinded to which intervention they received. The outcome assessor only analyzed the de-identified data.
Power calculation
An a priori sample size calculation was not performed due to budget restraints. The trial aimed to recruit a maximum sample size of n = 30, with n = 15 (1:1 ratio) in each arm of the parallel design. The backwards, ‘participants I can get’ approach to sample size calculation was an acceptable method in POC trials [Citation78,Citation79].
Statistical analyses
Participant characteristics and outcome variables are presented with descriptive statistics. Data normality and skewness were checked by visual inspection of histograms, and formally with the Shapiro-Wilk test [Citation80]. Data and residuals were non-normally distributed, despite data transformation and outlier correction [Citation81]. Outliers were assessed through box and whisker plots and were winsorized if correlated with a confounding factor from participants clinical diary [Citation82].
Non-parametric tests were used for the non-normally distributed data [Citation83], from a small sample size [Citation84]. Within the clinic, a Friedman’s two-way ANOVA tested the within-group factor (time) on the outcomes [Citation85]. A Bonferroni correction adjusted for multiple post-hoc comparisons [Citation85] and accounted for a type one error [Citation86]. The Mann-Whitney U test was used for between-group, pairwise comparison [Citation86]. A pairwise comparison was performed with the Wilcoxon-Signed Rank Test for the within-group measurements taken at home [Citation87]. Effect size was calculated with the formula r = z/√N [Citation86]. The missing data were minimal and handled with pairwise deletion [Citation88]. Data analysis was performed using IBM SPSS Statistics version 28.0 (IBM Corporation, Armonk, NY). Effect sizes, level of significance, and 95% confidence intervals are presented in the results [Citation89–91].
Results
The flow of participants is presented in [Citation92]. Data collection occurred between the 25th of May 2022 and 7th of April 2023. Nineteen males participated in this study. Participant characteristics are described in . No participants were lost from this study. Two HRV datasets were excluded due to operator and assessor error. Two individual HRV measures were lost due to operator error. Fifteen individual RPQ scores were lost due to participant’s forgetting to fill out the outcome. No adverse reactions were reported following any manual therapy interventions. Two sCOR data points (2.1%) were winsorized, one due to the participant receiving external treatment/smoking, and the other due to the participant performing vigorous exercise.
Table 1. Baseline participant characteristics (n = 19).
Salivary cortisol
Clinic
Within groups, there was a statistically significant change in sCOR levels following lower cervical mobilization, X2 [Citation2] = 9.556. A Bonferroni correction revealed a statistically significant reduction in sCOR levels from pre-5 (Md = 0.325) to post-30 (Md = 0.167). There was no statistically significant change in sCOR levels following upper cervical mobilization, X2 [Citation2] = 1.800.
Among the groups, there was no statistically significant difference in sCOR concentration following upper cervical and lower cervical mobilization at post-5, U = 58.000, z = 1.061, or post-30, U = 63.000, z = 1.470 (, ).
Table 2. Clinical measurements of salivary cortisol and rMSSD.
Home night
Within the groups, there was no statistically significant change in sCOR levels from baseline-pm to post-pm following upper cervical, z = −0.153, or lower cervical mobilization, z = −0.296.
Among the groups, there was no statistically significant difference in sCOR levels at post-pm following upper cervical and lower cervical mobilization, U = 46.000, z = 0.082 (, ).
Table 3. Home measurements of salivary cortisol and rMSSD.
Heart rate variability: rMSSD
Clinic
Within the groups, there was a statistically significant change in rMSSD following upper cervical mobilization, X2 [Citation2] = 8.222. A Bonferroni correction revealed a statistically significant increase in rMSSD from pre-5 (Md = 34.047) to post-30 (Md = 46.921). There was no statistically significant change in rMSSD following lower cervical mobilization, X2 [Citation2] = 5.250.
Among the groups, there was no statistically significant difference in rMSSD following upper cervical and lower cervical mobilization at post-5, U = 50.000, z = 1.347, or post-30, U = 38.000, z = 0.192 (, ).
Home morning
Within the groups, there was no statistically significant change in rMSSD from baseline-am to post-am following upper cervical, z = 0.700, or lower cervical mobilization, z = 1.183.
Among the groups, there was no statistically significant difference in rMSSD post-am following upper cervical and lower cervical mobilization, U = 35.000, z = 0.315 (, ).
Rivermead post-concussion symptoms questionnaire
Home night
Within the groups, there was no statistically significant change in RPQ scores from baseline-pm to post-pm following upper cervical, z = −0.339, or lower cervical mobilization, z = −1.022.
Among the groups, there was no statistically significant difference in RPQ scores post-pm following upper cervical and lower cervical mobilization, U = 24.500, z = 0.000 (, ).
Table 4. RPQ scores.
Home morning
Within groups, there was a statistically significant increase in RPQ scores from baseline-am (Md = 13.000) to post-am (Md = 18.500) following upper cervical mobilization, z = −2.032. There was no statistically significant change in RPQ score from baseline-am to post-am following lower cervical mobilization, z = −0.341.
Between groups, there was no statistically significant difference in RPQ scores post-am following upper cervical and lower cervical mobilization, U = 24.000, z = −0.842 (, ).
Figure 3. Clinic measurement of salivary cortisol (figure includes 95% confidence interval error bars).
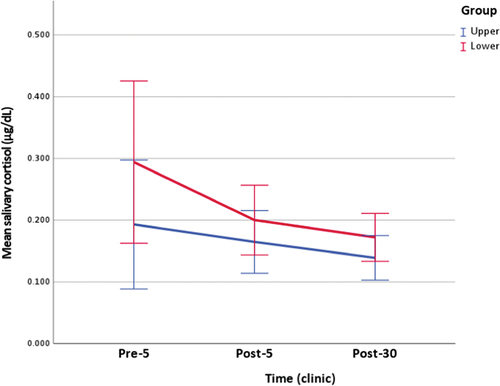
Discussion
Cervical spine manual therapy has the potential to modulate the stress response. To the authors' knowledge, this is the first study investigating whether there was a differential physiological response depending on the target location of cervical spine manual therapy in individuals with PPCS. This POC trial aimed to explore the physiological effects of a single session of upper or lower cervical spine mobilization in males with PPCS by comparing the immediate and short-term changes in the (1) HPA-axis response and (2) ANS response.
Salivary cortisol
There was a trend for a differential HPA-axis response between the two groups the night following the intervention (). Albeit non-significant, sCOR concentration increased the night following lower cervical mobilization and decreased the night following upper cervical mobilization. This was the primary outcome of the trial. This response can be explained by the underlying anatomy and function of the HPA-axis in the context of the stress response. The upper cervical spine is in close anatomical proximity to the brainstem and its PSNS cranial nerves [Citation93,Citation94], whereas the middle and lower cervical spine is in close anatomical proximity to the middle and inferior cervical (or stellate) ganglion, which are paravertebral ganglia of the sympathetic nervous system (SNS) [Citation95]. Considering the classical stress response, involving increased activity in the SNS and HPA-axis and decreased activity in the PSNS, upper cervical mobilization stimulating the PSNS centers is likely to downregulate the HPA axis and lower cervical mobilization stimulating the SNS centers is likely to upregulate the HPA-axis [Citation9]. The trend for a differential response occurring the night following the intervention is supported when considering the underlying autonomic anatomy and its relationship with the HPA-axis, along with the endocrine component of the stress response being slower-acting but longer-lasting [Citation10].
There was a statistically significant within-group decrease in sCOR concentration from pre-5 to post-30 following lower cervical mobilizations (), which is supported by a large effect size [Citation96]. This indicates a downregulation of cortisol secretion from the HPA-axis 30 minutes following lower cervical mobilization. This finding is supported by another study on healthy males, which also found a statistically significant reduction in sCOR concentration following lower cervical spine mobilizations [Citation32]. Within the exercise science literature, the response to lower cervical spine mobilizations (initial decrease in sCOR concentration 30 minutes after the intervention followed by a reciprocal increase the night following the intervention) has been termed the ‘rebound effect’ [Citation97]. Albeit a different population (Achilles tendinopathy), intervention (thoracic manipulation), and outcome (salivary testosterone to cortisol ratio), the same trend was shown following a thoracic manipulation [Citation98]. In this study, there was an initial decrease in the T/C ratio, followed by a reciprocal increase 6 hours later [Citation98]. Considering that both regions house components of the SNS [Citation95,Citation99] and the bidirectional and interchangeable communication between the peripheral components of the stress response [Citation10], the thoracic manipulation could have the same effect on the SNS and subsequent HPA-axis as the lower cervical mobilizations. This highlights the importance of longitudinal measurement of sCOR, as single measurements may miss or misconstrue the true interaction.
Heart rate variability
There was a statistically significant within-group increase in rMSSD from pre-5 to post-30 following upper cervical mobilization () which is supported by a large effect size [Citation96]. An increase in rMSSD represents an increase in PSNS activation and an increased ability to adjust the internal environment in response to stressors [Citation100,Citation101]. This finding supports the hypothesis of a differential response based on the underlying anatomy and the function of the ANS in the context of the stress response. The upper cervical spine is in close anatomical proximity to the PSNS [Citation93,Citation94], and the ANS is the fast-acting component of the stress response [Citation3,Citation4,Citation8,Citation9]. Despite a different population (acute neck pain), intervention (manipulation), primary HRV metrics (SDNN, nuHF), and tools (ECG), the finding of an increase in rMSSD is supported by a study on individuals with acute neck pain, where upper cervical manipulation increased HRV metrics indicative of PSNS activation [Citation102].
Rivermead post concussion symptoms questionnaire
A trend was shown for changes in symptoms at the same time as the physiological outcomes were taken. The increase in RPQ scores following upper cervical spine mobilization () occurred with a decrease in sCOR concentration (post-pm) and a slight increase in rMSSD (post-am). In contrast, the decrease in RPQ scores following lower cervical spine mobilization () occurred with an increase in sCOR concentration (post-pm) and rMSSD (post-am). Caution must be taken when interpreting symptom changes with changes in the underlying physiology, as this study was not powered with the appropriate regression analyses. Additionally, the changes in RPQ scores did not reach clinical significance [Citation76].
Strengths and limitations
Stringent inclusion criterion and careful control of many potential confounders strengthen internal validity. Robust internal validity does limit the study’s external validity. Missing data were minimal for physiological outcomes (0% for sCOR, 2.4% for rMSSD), but not patient self-reported outcome measures (15.8% for RPQ), despite utilizing reminder texts. There was no attrition of participants. Further strengths of this trial is that it examined both components of the stress response in unison in a population characterized by a dysfunctional stress response and collected patient-reported outcome data (RPQ) concurrently with physiological outcomes. This was a starting point for interpreting the clinical relevance of physiological changes following manual therapy.
A primary limitation of this POC trial is its small sample size, potentially leading to a type II error and underpowered trial [Citation103]. Significant variability in the data, evidenced by the 95% confidence intervals, supports the notion of a type II error, potentially washing out differential effects between and within groups. External pressures of funding and time explain why recruitment ceased and only 19 participants were included. It is possible that lowering the age range to increase recruitment biased the results. A non-specified post-hoc subgroup analysis [Citation104] revealed that two additional participants below the original age of 21 did not alter the results. The small sample size and variability within the data meant the normality assumption was violated, and parametric statistical analyses could not be performed to obtain a group × time interaction. Another potential limitation is the lack of a sham or control group. This work aimed not to investigate efficacy but whether there was a differential response, which did not require a sham or control group.
Implications for clinical practice and recommendations for future research
The results of this trial provide preliminary evidence to support further investigation for the selective use of cervical spine manual therapy to target specific components of the stress response and offers clinicians who mobilize the cervical spine for musculoskeletal dysfunction (e.g. pain and joint stiffness) alternative considerations for the mechanisms of effect of their intervention. Considering the rebound effect and the trend for increasing cortisol concentrations the night following lower cervical spine mobilizations, this intervention may be a novel treatment to increase cortisol concentrations in individuals who develop PPCS, assuming the cortisol concentration remains low from their concussion [Citation15–18]. A reduction in PSNS activity has been implicated in individuals with PPCS [Citation105,Citation106]. The results of the present POC trial indicate that selective cervical spine mobilizations could be used to increase PSNS activity. Considering the shared mechanisms of a dysfunctional stress response, the results of this trial may be generalizable to other populations characterized by low cortisol levels, such as osteoarthritis [Citation107], rheumatoid arthritis [Citation108], or long-COVID [Citation109–111], or characterized by low PSNS activity, such as postural orthostatic tachycardia syndrome [Citation112] or fibromyalgia [Citation113].
Future studies should include larger sample sizes over extended periods to clarify the trends in stress response physiology and symptoms. A control and/or sham group should be included to isolate the treatment effect. Females sustain higher rates of concussion and have greater time lost from participation [Citation114], therefore must be included in future studies. Wider age ranges should also be included in future studies to increase generalizability of the results. Another logical step would be to sub-group or phenotype patients into categories, termed ‘clinical phenotyping’ [Citation115], such as a dampened or heightened stress response, who may respond to upper or lower cervical spine mobilizations based on their underlying symptoms and physiology (mechanisms informed intervention or precision-based medicine). Following this, an adequately powered clinical trial could be developed, aligning specific treatment to the individual’s subgroup and underlying pathophysiology.
Conclusion
This POC trial provides preliminary evidence that manual therapy applied to separate locations within the cervical spine may differentially modulate the stress response in people with PPCS. Thirty-minutes following lower cervical spine mobilization, there was a statistically significant within-group reduction in sCOR concentration, reflecting a downregulation of HPA-axis activity. Thirty-minutes following upper cervical spine mobilization, there was a statistically significant increase in rMSSD, reflecting an increase in PSNS activity. These results support further investigation on the selective use of cervical spine manual therapy to differentially modulate the stress response.
Author contribution
Concept development: GF, CCh, EK, KS, ASG, CCo, ST
Literature search: GF
Design: GF, CCh, EK, KS, ASG, CCo, RK, ST
Recruitment: GF, CCh
Interventions and participant management: GF
Data collection: GF
Data analysis: GF, MRB, RK
Manuscript preparation: GF
Commented/edited final manuscript: GF, CCh, EK, MRB, KS, ASG, CCo, RK, ST
Supplemental Material
Download MS Word (823.3 KB)Disclosure statement
No potential conflict of interest was reported by the author(s).
Data availability statement
The data that support the findings of this study are available from the corresponding author upon reasonable request.
Supplementary material
Supplemental data for this article can be accessed online at https://doi.org/10.1080/10669817.2024.2363018
Additional information
Funding
Notes on contributors
Gerard Farrell
Gerard Farrell is a PhD candidate at the Centre for Health, Activity and Rehabilitation Research, School of Physiotherapy at the University of Otago. His research interests include the neuroendocrine mechanisms of manual therapy, the part a dysfunctional stress response has to play in the development and maintenance of various clinical populations, and the role manual therapy plays in the treatment of persistent post-concussion symptoms and other populations characterized by a dysfunctional stress response.
Cathy Chapple
Cathy Chapple is a senior lecturer at the Centre for Health, Activity and Rehabilitation Research, School of Physiotherapy at the University of Otago. Her research interests are in the management of people with osteoarthritis. She is also investigating manual therapy for osteoarthritis patients and other musculoskeletal conditions including the role of the cervical spine in persistent post-concussion symptoms.
Ewan Kennedy
Ewan Kennedy is a senior lecturer at the Centre for Health, Activity and Rehabilitation Research, School of Physiotherapy at the University of Otago. His research interests are in musculoskeletal disorders, concussion, and clinical education. Recent work has explored overlap between cervical spine and concussion injuries, with a focus on improving health service delivery.
Matthew Reily-Bell
Matthew Bell is a PhD candidate at the Department of Physiology, HeartOtago, School of Biomedical Sciences. His PhD is investigating CRISPR/Cas13 as a tool for the knockdown of microRNA unregulated in diabetic heart disease.
Kesava Sampath
Kesava Sampath is a senior lecturer at the Centre of Health and Social Practice, Waikato Institute of Technology, Hamilton, Waikato, New Zealand. His research work focuses on the mechanisms underpinning manual therapy practice. Currently, he leads a research project investigating the usage of bio-psycho-social model of care by New Zealand osteopaths while treating people with persistent musculoskeletal pain. He is a visiting research fellow at the University of Technology, Sydney, where he is part of a global osteopathic network and actively collaborates/contributes to other projects. He is also an adjunct researcher at the Ara Institute of Canterbury and supervises post-graduate nursing research students.
Angela Spontelli Gisselman
Angela Spontelli Gisselman is an assistant professor at Tufts University School of Medicine. Her main research interests are in the role of health metrics, such as heart rate variability (an index of the autonomic nervous system), and their ability to influence decision-making in rehabilitation, such as post-concussion rehabilitation. In addition to this line of research, other areas of interest include heart rate variability and temporomandibular disorders; the use of thermal imaging in tendinopathy; examination and management of shoulder injuries; load monitoring technology and post-operative rehabilitation.
Chad Cook
Chad Cook is a clinical researcher, physical therapist, and professional advocate with a history of clinical care excellence and service. His passions include refining and improving the patient examination process and validating tools used in day-to-day physical therapist practice. Dr. Cook has authored or co-authored three textbooks, has published over 250 peer-reviewed manuscripts, and lectures internationally on orthopedic examination and treatment. He is a fellow of the American Academy of Orthopaedic Manual Physical Therapists; has specialized in manual therapy for over 19 years. His main areas of research interest are in examination and conservative or surgical treatment of orthopedic-related conditions.
Rajesh Katare
Rajesh Katare is an associate professor at the Department of Physiology, HeartOtago, School of Biomedical Sciences. His main research interests include investigating the molecular mechanisms involved in the development of cardiovascular complications in diabetes, the role of microRNAs in cardiovascular diseases, the development of novel genetic and stem cell therapies for the treatment of ischemic and non-ischemic cardiovascular complications.
Steve Tumilty
Steve Tumilty is one of the few clinician scientists in Physiotherapy Worldwide and one of only few Registered Physiotherapy Specialists in New Zealand. He has an interest in blurring the boundaries between the manual therapy professions. The majority of his clinical experience has been in the outpatient musculoskeletal practice settings in the UK, Germany, and New Zealand. He also has experience in professional sports and Occupational Health Physiotherapy. Dr. Tumilty’s current research interests are in modulation of the Hypothalamus-Pituitary Axis using manual interventions, and the influence of the autonomic nervous system on musculoskeletal pain and healing.
References
- Farrell G, Wang S, Chapple C, et al. Dysfunction of the stress response in individuals with persistent post-concussion symptoms: a scoping review. Phys Ther Rev. 2022;27(5):1–22. doi: 10.1080/10833196.2022.2096195
- Weil ZM, White B, Whitehead B, et al. The role of the stress system in recovery after traumatic brain injury: a tribute to Bruce S. McEwen Neurobiol Stress. 2022;19:100467. doi: 10.1016/j.ynstr.2022.100467
- Chrousos GP. Stress and disorders of the stress system. Nat Rev Endocrinol. 2009;5(7):374–381. doi: 10.1038/nrendo.2009.106
- Agorastos A, Heinig A, Stiedl O, et al. Vagal effects of endocrine HPA axis challenges on resting autonomic activity assessed by heart rate variability measures in healthy humans. Psychoneuroendocrinology. 2019;102:196–203. doi: 10.1016/j.psyneuen.2018.12.017
- Nicolaides NC, Kyratzi E, Lamprokostopoulou A, et al. Stress, the stress system and the role of glucocorticoids. Neuroimmunomodulation. 2015;22(1–2):6–19. doi: 10.1159/000362736
- Sawicki CM, Humeidan ML, Sheridan JF. Neuroimmune interactions in pain and stress: an interdisciplinary approach. Neuroscientist. 2020;27(2):113–128. doi: 10.1177/1073858420914747
- Tsigos C, Chrousos GP. Hypothalamic–pituitary–adrenal axis, neuroendocrine factors and stress. J Psychosom Res. 2002;53(4):865–871. doi: 10.1016/S0022-3999(02)00429-4
- Herman JP. Regulation of hypothalamo-pituitary-adrenocortical responses to stressors by the nucleus of the solitary tract/dorsal vagal complex. Cell Mol Neurobiol. 2018;38(1):25–35. doi: 10.1007/s10571-017-0543-8
- Godoy LD, Rossignoli MT, Delfino-Pereira P, et al. A comprehensive overview on stress neurobiology: basic concepts and clinical implications. Front Behav Neurosci. 2018;12:1–23. doi: 10.3389/fnbeh.2018.00127
- Schuurmans AAT, Nijhof KS, Cima M, et al. Alterations of autonomic nervous system and HPA axis basal activity and reactivity to acute stress: a comparison of traumatized adolescents and healthy controls. Stress. 2021;24(6):876–887. doi: 10.1080/10253890.2021.1900108
- Dwyer B, Katz DI. Postconcussion syndrome. Handb Clin Neurol. 2018;158:163–178.
- Coffman CA, Kay JJM, Saba KM, et al. Predictive value of subacute heart rate variability for determining outcome following adolescent concussion. J Clin Med. 2021;10(1):1–17. doi: 10.3390/jcm10010161
- Anderson FL, Hellwinkel JE, Montjoy M, et al. Change in heart rate variability after concussion in a collegiate soccer player. Neurotrauma Rep. 2020;1(1):88–92. doi: 10.1089/neur.2020.0003
- Lagos L, Thompson J, Vaschillo E. A preliminary study: heart rate variability biofeedback for treatment of postconcussion syndrome. Biofeedback. 2013;41(3):136–143. doi: 10.5298/1081-5937-41.3.02
- Ritchie EV, Emery C, Debert CT. Analysis of serum cortisol to predict recovery in paediatric sport-related concussion. Brain Inj. 2018;32(4):523–528. doi: 10.1080/02699052.2018.1429662
- Di Battista AP, Rhind SG, Churchill N, et al. Peripheral blood neuroendocrine hormones are associated with clinical indices of sport-related concussion. Sci Rep. 2019;9(1):1–10. doi: 10.1038/s41598-019-54923-3
- Villegas E, Hartsock MJ, Aben B, et al. Association between altered cortisol profiles and neurobehavioral impairment after mild traumatic brain injury in college students. J Neurotrauma. 2022;39(11–12):809–820. doi: 10.1089/neu.2021.0495
- Tabor J, La P, Kline G, et al. Saliva cortisol as a biomarker of injury in youth sport-related concussion. J Neurotrauma. 2022;39:1–13.
- Ellis MJ, McDonald PJ, Olson A, et al. Cervical spine dysfunction following pediatric sports-related head trauma. J Head Trauma Rehabil. 2019;34(2):103–110. doi: 10.1097/HTR.0000000000000411
- Marshall CM, Vernon H, Leddy JJ, et al. The role of the cervical spine in post-concussion syndrome. Phys Sportsmed. 2015;43(3):274–284. doi: 10.1080/00913847.2015.1064301
- Schneider KJ. Concussion part II: rehabilitation - the need for a multifaceted approach. Musculoskeletal Sci Pract. 2019;42:151–161. doi: 10.1016/j.msksp.2019.01.006
- Gross A, Miller J, D’Sylva J, et al. Manipulation or mobilisation for neck pain: a Cochrane review. Man Ther. 2010;15(4):315–333. doi: 10.1016/j.math.2010.04.002
- Gross A, Langevin P, Burnie SJ, et al. Manipulation and mobilisation for neck pain contrasted against an inactive control or another active treatment. Cochrane Database Syst Rev. 2015;2015(9):CD004249. doi: 10.1002/14651858.CD004249.pub4
- Dunning JR, Cleland JA, Waldrop MA, et al. Upper cervical and upper thoracic thrust manipulation versus nonthrust mobilization in patients with mechanical neck pain: a multicenter randomized clinical trial. J Orthop Sports Phys Ther. 2012;42(1):5–18. doi: 10.2519/jospt.2012.3894
- Reid SA, Rivett DA. Manual therapy treatment of cervicogenic dizziness: a systematic review. Man Ther. 2005;10(1):4–13. doi: 10.1016/j.math.2004.03.006
- Reid SA, Callister R, Snodgrass SJ, et al. Manual therapy for cervicogenic dizziness: long-term outcomes of a randomised trial. Man Ther. 2015;20(1):148–156. doi: 10.1016/j.math.2014.08.003
- Reid SA, Callister R, Katekar MG, et al. Effects of cervical spine manual therapy on range of motion, head repositioning, and balance in participants with cervicogenic dizziness: a randomized controlled trial. Arch Phys Med Rehabil. 2014;95(9):1603–1612. doi: 10.1016/j.apmr.2014.04.009
- Reid SA, Rivett DA, Katekar MG, et al. Sustained natural apophyseal glides (SNAGs) are an effective treatment for cervicogenic dizziness. Man Ther. 2008;13(4):357–366. doi: 10.1016/j.math.2007.03.006
- Dunning JR, Butts R, Mourad F, et al. Upper cervical and upper thoracic manipulation versus mobilization and exercise in patients with cervicogenic headache: a multi-center randomized clinical trial. BMC Musculoskelet Disord. 2016;17(1):64. doi: 10.1186/s12891-016-0912-3
- Kennedy E, Chapple C, Quinn D, et al. Can the neck contribute to persistent symptoms post concussion? long-term follow up from a prospective descriptive case series. J Man Manip Ther. 2021;29(5):1–13. doi: 10.1080/10669817.2021.1920276
- Ellis MJ, Leddy JJ, Willer B. Physiological, vestibulo-ocular and cervicogenic post-concussion disorders: an evidence-based classification system with directions for treatment. Brain Inj. 2015;29(2):238–248. doi: 10.3109/02699052.2014.965207
- Farrell G, Bell M, Chapple C, et al. Autonomic nervous system and endocrine system response to upper and lower cervical spine mobilization in healthy male adults: a randomized crossover trial. J Man Manip Ther. 2023;31(6):1–14. doi: 10.1080/10669817.2023.2177071
- Groschl M. Current status of salivary hormone analysis. Clin Chem. 2008;54(11):1759–1769. doi: 10.1373/clinchem.2008.108910
- Shaffer F, McCraty R, Zerr CL. A healthy heart is not a metronome: an integrative review of the heart’s anatomy and heart rate variability. Front psychol. 2014;5:1–19. doi: 10.3389/fpsyg.2014.01040
- Schneider KJ, Meeuwisse WH, Nettel-Aguirre A, et al. Cervicovestibular rehabilitation in sport-related concussion: a randomised controlled trial. Br J Sports Med. 2014;48(17):1294–1298. doi: 10.1136/bjsports-2013-093267
- Moher D, Hopewell S, Schulz KF, et al. CONSORT 2010 explanation and elaboration: updated guidelines for reporting parallel group randomised trials. Br Med J. 2010;340(mar23 1):c869. doi: 10.1136/bmj.c869
- Silvetti MS, Drago F, Ragonese P. Heart rate variability in healthy children and adolescents is partially related to age and gender. Int J Cardiol. 2001;81(2):169–174. doi: 10.1016/S0167-5273(01)00537-X
- Feldman HA, Longcope C, Derby CA, et al. Age trends in the level of serum testosterone and other hormones in middle-aged men: longitudinal results from the Massachusetts male aging study. J Clin Endocrinol Metab. 2002;87(2):589–598. doi: 10.1210/jcem.87.2.8201
- McCrory P, Meeuwisse W, Dvorak J, et al. Consensus statement on concussion in sport-the 5th international conference on concussion in sport held in berlin, October 2016. Br J Sports Med. 2017;51(11):838–847. doi: 10.1136/bjsports-2017-097699
- Agorastos A, Kellner M, Stiedl O, et al. Blunted autonomic reactivity to pharmacological panic challenge under long-term escitalopram treatment in healthy men. Int J Neuropsychopharmacol. 2014;18(5):1–11. doi: 10.1093/ijnp/pyu053
- Hutting N, Kerry R, Coppieters MW, et al. Considerations to improve the safety of cervical spine manual therapy. Musculoskeletal Sci Pract. 2018;33:41–45. doi: 10.1016/j.msksp.2017.11.003
- Koenig J, Thayer JF. Sex differences in healthy human heart rate variability: a meta-analysis. Neurosci Biobehav Rev. 2016;64:288–310. doi: 10.1016/j.neubiorev.2016.03.007
- Van Cauter E, Leproult R, Kupfer DJ. Effects of gender and age on the levels and circadian rhythmicity of plasma cortisol. J Clin Endocrinol Metab. 1996;81(7):2468–2473. doi: 10.1210/jcem.81.7.8675562
- Saeki Y, Atogami F, Takahashi K, et al. Reflex control of autonomic function induced by posture change during the menstrual cycle. J Auton Nerv Syst. 1997;66(1–2):69–74. doi: 10.1016/S0165-1838(97)00067-2
- Sato N, Miyake S, Ji A, et al. Power spectral analysis of heart rate variability in healthy young women during the normal menstrual cycle. Psychosom Med. 1995;57(4):331–335. doi: 10.1097/00006842-199507000-00004
- Schmalenberger KM, Eisenlohr-Moul TA, Jarczok MN, et al. Menstrual cycle changes in vagally-mediated heart rate variability are associated with progesterone: evidence from two within-person studies. J Clin Med. 2020;9(3):1–20. doi: 10.3390/jcm9030617
- Piekarz V, Perry J. An investigation into the effects of applying a lumbar Maitland mobilisation at different frequencies on sympathetic nervous system activity levels in the lower limb. Man Ther. 2016;23:83–89. doi: 10.1016/j.math.2016.01.001
- Karim N, Hasan J, Ali S. Heart rate variability - a review. J Basic Appl Scis. 2011;7:71–77.
- Strahler J, Skoluda N, Kappert MB, et al. Simultaneous measurement of salivary cortisol and alpha-amylase: application and recommendations. Neurosci Biobehav Rev. 2017;83:657–677. doi: 10.1016/j.neubiorev.2017.08.015
- Sladek CD, Michelini LC, Stachenfeld NS, et al. Endocrine-autonomic linkages. Compr Physiol. 2015;5:1281–1323.
- Bozovic D, Racic M, Ivkovic N. Salivary cortisol levels as a biological marker of stress reaction. Med Archives. 2013;67(5):374–377. doi: 10.5455/medarh.2013.67.374-377
- Colombi T. The effects induced by spinal manipulative therapy on the immune and endocrine systems. Medicina (B Aires). 2019;55(8):1–12. doi: 10.3390/medicina55080448
- Adam EK, Kumari M. Assessing salivary cortisol in large-scale, epidemiological research. Psychoneuroendocrinology. 2009;34(10):1423–1436. doi: 10.1016/j.psyneuen.2009.06.011
- Nater UM, Rohleder N, Schlotz W, et al. Determinants of the diurnal course of salivary alpha-amylase. Psychoneuroendocrinology. 2007;32(4):392–401. doi: 10.1016/j.psyneuen.2007.02.007
- Touitou Y, Haus E. Alterations with aging of the endocrine and neuroendocrine circadian system in humans. Chronobiol Int. 2000;17(3):369–390. doi: 10.1081/CBI-100101052
- Golden SH, Wand GS, Malhotra S, et al. Reliability of hypothalamic–pituitary–adrenal axis assessment methods for use in population-based studies. Eur J Epidemiol. 2011;26(7):511–525. doi: 10.1007/s10654-011-9585-2
- Electrophysiology TFOTESOCTNA. Heart rate variability: standards of measurement, physiological interpretation and clinical use. task force of the European society of cardiology and the north American society of pacing and electrophysiology. Circulation. 1996;93(5):1043–1065. doi: 10.1161/01.CIR.93.5.1043
- Del Paso Ga R, Langewitz W, Mulder LJM, et al. The utility of low frequency heart rate variability as an index of sympathetic cardiac tone: a review with emphasis on a reanalysis of previous studies. Psychophysiology. 2013;50(5):477–487. doi: 10.1111/psyp.12027
- Allen J. Photoplethysmography and its application in clinical physiological measurement. Physiol Meas. 2007;28(3):R1–R39. doi: 10.1088/0967-3334/28/3/R01
- Plews DJ, Scott B, Altini M, et al. Comparison of heart-rate-variability recording with smartphone photoplethysmography, polar H7 chest strap, and electrocardiography. Int J Sports Physiol Perform. 2017;12(10):1324–1328. doi: 10.1123/ijspp.2016-0668
- Pernice R, Javorka M, Krohova J, et al. Reliability of short-term heart rate variability indexes assessed through photoplethysmography. In: Annual International Conference IEEE England Medical Biological Society; Honolulu, Hawaii, USA; 2018. p. 5610–513.
- Heathers JA. Smartphone-enabled pulse rate variability: an alternative methodology for the collection of heart rate variability in psychophysiological research. Int J Psychophysiol. 2013;89(3):297–304. doi: 10.1016/j.ijpsycho.2013.05.017
- Laborde S, Mosley E, Thayer JF. Heart rate variability and cardiac vagal tone in psychophysiological research – recommendations for experiment planning, data analysis, and data reporting. Frontiers In Psychology. 2017;8:1–18. doi: 10.3389/fpsyg.2017.00213
- Moya-Ramon M, Mateo-March M, Pena-Gonzalez I, et al. Validity and reliability of different smartphones applications to measure HRV during short and ultra-short measurements in elite athletes. Comput Methods Programs Biomed. 2022;217:106696. doi: 10.1016/j.cmpb.2022.106696
- Al Haddad H, Laursen P, Chollet D, et al. Reliability of resting and postexercise heart rate measures. Int J Sports Med. 2011;32(8):598–605. doi: 10.1055/s-0031-1275356
- Plews DJ, Laursen PB, Kilding AE, et al. Heart rate variability in elite triathletes, is variation in variability the key to effective training? a case comparison. Eur J Appl Physiol. 2012;112(11):3729–3741. doi: 10.1007/s00421-012-2354-4
- Plews DJ, Laursen PB, Kilding AE, et al. Evaluating training adaptation with heart-rate measures: a methodological comparison. Int J Sports Physiol Perform. 2013;8(6):688–691. doi: 10.1123/ijspp.8.6.688
- Kim H-S, Yoon K-H, Cho J-H. Diurnal heart rate variability fluctuations in normal volunteers. J Diabetes Sci Technol. 2014;8(2):431–433. doi: 10.1177/1932296813519013
- Van Eekelen APJ, Houtveen JH, Kerkhof GA. Circadian variation in cardiac autonomic activity: reactivity measurements to different types of stressors. Chronobiol Int. 2004;21(1):107–129. doi: 10.1081/CBI-120027983
- Massin MM. Circadian rhythm of heart rate and heart rate variability. Arch Dis Child. 2000;83(2):179–182. doi: 10.1136/adc.83.2.179
- Esco MR, Flatt AA. Ultra-short-term heart rate variability indexes at rest and post-exercise in athletes: evaluating the agreement with accepted recommendations. J Sports Sci Med. 2014;13(3):535–541.
- Young FLS, Leicht AS. Short-term stability of resting heart rate variability: influence of position and gender. Appl Physiol Nutr Metab. 2011;36(2):210–218. doi: 10.1139/h10-103
- King NS. Emotional, neuropsychological, and organic factors: their use in the prediction of persisting postconcussion symptoms after moderate and mild head injuries. J Neurol Neurosurg Psychiatry. 1996;61(1):75–81. doi: 10.1136/jnnp.61.1.75
- Ingebrigtsen T, Waterloo K, Marup-Jensen S, et al. Quantification of post-concussion symptoms 3 months after minor head injury in 100 consecutive patients. J Neurol. 1998;245(9):609–612. doi: 10.1007/s004150050254
- King NS, Crawford S, Wenden FJ, et al. The rivermead post concussion symptoms questionnaire: a measure of symptoms commonly experienced after head injury and its reliability. J Neurol. 1995;242(9):587–592. doi: 10.1007/BF00868811
- Mercier LJ, Fung TS, Harris AD, et al. Improving symptom burden in adults with persistent post-concussive symptoms: a randomized aerobic exercise trial protocol. BMC Neurol. 2020;20(1):1–9. doi: 10.1186/s12883-020-1622-x
- Cook C, Sheets C. Clinical equipoise and personal equipoise: two necessary ingredients for reducing bias in manual therapy trials. J Man Manip Ther. 2011;19(1):55–57. doi: 10.1179/106698111X12899036752014
- Verhagen A, L-M Y. Editorial: what about sample size? Musculoskeletal Sci Pract. 2021;54:102405. doi: 10.1016/j.msksp.2021.102405
- Ting N, Chen D-G, Ho S, et al. Design a proof of concept trial. Singapore: Springer Singapore; 2017. p. 75–92.
- Mohd Razali N, Yap B. Power comparisons of Shapiro-wilk, kolmogorov-Smirnov, lilliefors and Anderson-darling tests. J Stat Model Anal. 2011;2:21–33.
- Lo S, Andrews S. To transform or not to transform: using generalized linear mixed models to analyse reaction time data. Front psychol. 2015;6:1171. doi: 10.3389/fpsyg.2015.01171
- Lien D, Balakrishnan N. On regression analysis with data cleaning via trimming, winsorization, and dichotomization. Commun Stat-Simul C. 2005;34(4):839–849. doi: 10.1080/03610910500307695
- Putt ME, Chinchilli VM. Nonparametric approaches to the analysis of crossover studies. Stat Sci. 2004;19(4):712–719. doi: 10.1214/088342304000000611
- Koch GG. The use of non-parametric methods in the statistical analysis of the two-period change-over design. Biometrics. 1972;28(2):577–584. doi: 10.2307/2556170
- Pereira DG, Afonso A, Medeiros FM. Overview of friedman’s test and post-hoc analysis. Commun Stat-Simul. 2014;44(10):2636–2653. doi: 10.1080/03610918.2014.931971
- Pallant J. SPSS Survival Manual. London: Routledge; 2020.
- Woolson RF. Wilcoxon Signed-Rank Test. In: Armitage P, Colton T, editors. Encyclopedia of Biostatistics. 2005. doi: 10.1002/0470011815.b2a15177
- Burke S. Missing values, outliers, robust statistics & non-parametric methods. Stat Data Anal. 2001;2:19.
- Sullivan GM, Feinn R. Using effect size—or why the p value is not enough. J Grad Med Educ. 2012;4(3):279–282. doi: 10.4300/JGME-D-12-00156.1
- Altman DG, Gore SM, Gardner MJ, et al. Statistical guidelines for contributors to medical journals. Br Med J. 1983;286(6376):1489–1493. doi: 10.1136/bmj.286.6376.1489
- Sterne JAC. Sifting the evidence-what’s wrong with significance tests? Another comment on the role of statistical methods. Br Med J. 2001;322(7280):226–231. doi: 10.1136/bmj.322.7280.226
- Dwan K, Li T, Altman DG, et al. CONSORT 2010 statement: extension to randomised crossover trials. Br Med J. 2019;l4378. doi: 10.1136/bmj.l4378
- Damodaran O, Rizk E, Rodriguez J, et al. Cranial nerve assessment: a concise guide to clinical examination. Clin Anat. 2014;27(1):25–30. doi: 10.1002/ca.22336
- Bland JH, Boushey DR. Anatomy and physiology of the cervical spine. Semin Arthritis Rheum. 1990;20(1):1–20. doi: 10.1016/0049-0172(90)90090-3
- Elias M. Cervical sympathetic and stellate ganglion blocks. Pain Physician. 2000;3(3):294–304. doi: 10.36076/ppj.2000/3/294
- Cohen J. Statistical power analysis for the behavioral sciences. 2nd ed. Hillside (NJ): Lawrence Erlbaum Associates; 1988.
- Cunniffe B, Hore AJ, Whitcombe DM, et al. Time course of changes in immuneoendocrine markers following an international rugby game. Eur J Appl Physiol. 2010;108(1):113–122. doi: 10.1007/s00421-009-1200-9
- Kovanur Sampath K, Mani R, Katare R, et al. Thoracic spinal manipulation effect on neuroendocrine response in people with achilles tendinopathy: a randomized crossover trial. J Manipulative Physiol Ther. 2021;44(5):420–431. doi: 10.1016/j.jmpt.2021.06.001
- Patel TR. Chapter 36 - anatomy of the sympathetic nervous system. In: Tubbs R, Rizk E, Shoja M, Loukas M, Barbaro N Spinner R, editors. Nerves and Nerve Injuries. San Diego: Academic Press; 2015. p. 495–506.
- Thayer JF, Åhs F, Fredrikson M, et al. A meta-analysis of heart rate variability and neuroimaging studies: implications for heart rate variability as a marker of stress and health. Neurosci Biobehav Rev. 2012;36(2):747–756. doi: 10.1016/j.neubiorev.2011.11.009
- Thayer JF, Sternberg E. Beyond heart rate variability: vagal regulation of allostatic systems. Ann N Y Acad Sci. 2006;1088(1):361–372. doi: 10.1196/annals.1366.014
- Win NN, Jorgensen AM, Chen YS, et al. Effects of upper and lower cervical spinal manipulative therapy on blood pressure and heart rate variability in volunteers and patients with neck pain: a randomized controlled, cross-over, preliminary study. J Chiropr Med. 2015;14(1):1–9. doi: 10.1016/j.jcm.2014.12.005
- Altman DG. Practical statistics for medical research. New York: Chapman and Hall/CRC; 1990.
- Burke JF, Sussman JB, Kent DM, et al. Three simple rules to ensure reasonably credible subgroup analyses. Br Med J. 2015;h5651. doi: 10.1136/bmj.h5651
- Abaji JP, Curnier D, Moore RD, et al. Persisting effects of concussion on heart rate variability during physical exertion. J Neurotrauma. 2016;33(9):811–817. doi: 10.1089/neu.2015.3989
- Pertab JL, Merkley TL, Cramond AJ, et al. Concussion and the autonomic nervous system: an introduction to the field and the results of a systematic review. NeuroRehabilitation. 2018;42(4):397–427. doi: 10.3233/NRE-172298
- Carlesso LC, Sturgeon JA, Zautra AJ. Exploring the relationship between disease-related pain and cortisol levels in women with osteoarthritis. Osteoarthritis Cartilage. 2016;24(12):2048–2054. doi: 10.1016/j.joca.2016.06.018
- Evers AW, Verhoeven EW, van Middendorp H, et al. Does stress affect the joints? Daily stressors, stress vulnerability, immune and HPA axis activity, and short-term disease and symptom fluctuations in rheumatoid arthritis. Ann Rheum Dis. 2014;73(9):1683–1688. doi: 10.1136/annrheumdis-2012-203143
- Hashim M, Athar S, Gaba WH. New onset adrenal insufficiency in a patient with COVID-19. BMJ Case Rep. 2021;14(1):e237690. doi: 10.1136/bcr-2020-237690
- Kanczkowski W, Evert K, Stadtmüller M, et al. COVID-19 targets human adrenal glands. Lancet Diabetes Endocrinol. 2022;10(1):13–16. doi: 10.1016/S2213-8587(21)00291-6
- Kanczkowski W, Beuschlein F, Bornstein SR. Is there a role for the adrenal glands in long COVID? Nat Rev Endocrinol. 2022;18(8):451–452. doi: 10.1038/s41574-022-00700-8
- Swai J, Hu Z, Zhao X, et al. Heart rate and heart rate variability comparison between postural orthostatic tachycardia syndrome versus healthy participants; a systematic review and meta-analysis. BMC Cardiovasc Disord. 2019;19(1):1–12. doi: 10.1186/s12872-019-01298-y
- Martins DF, Viseux FJF, Salm DC, et al. The role of the vagus nerve in fibromyalgia syndrome. Neurosci Biobehav Rev. 2021;131:1136–1149. doi: 10.1016/j.neubiorev.2021.10.021
- Covassin T, Moran RM, Elbin RJ. Sex differences in reported concussion injury rates and time loss from participation: an update of the national collegiate athletic association injury surveillance program from 2004-2005 through 2008-2009. J Athl Train. 2016;51(3):189–194. doi: 10.4085/1062-6050-51.3.05
- Damian K, Chad C, Kenneth L, et al. Time to evolve: the applicability of pain phenotyping in manual therapy. J Man Manip Ther. 2022;30(2):61–67. doi: 10.1080/10669817.2022.2052560
- Kudielka BM, Gierens A, Hellhammer DH, et al. Salivary cortisol in ambulatory assessment-some dos, some don’ts, and some open questions. Psychosom Med. 2012;74(4):418–431. doi: 10.1097/PSY.0b013e31825434c7
- Chrousos GP, Gold PW. The concepts of stress and stress system disorders: overview of physical and behavioral homeostasis. J Am Med Assoc. 1992;267(9):1244–1252. doi: 10.1001/jama.1992.03480090092034
- Maes M, Mommen K, Hendrickx D, et al. Components of biological variation, including seasonality, in blood concentrations of TSH, TT3, FT4, PRL, cortisol and testosterone in healthy volunteers. Clin Endocrinol (Oxf). 1997;46(5):587–598. doi: 10.1046/j.1365-2265.1997.1881002.x
- Hellhammer DH, Wust S, Kudielka BM. Salivary cortisol as a biomarker in stress research. Psychoneuroendocrinology. 2009;34(2):163–171. doi: 10.1016/j.psyneuen.2008.10.026
- Stalder T, Kirschbaum C, Kudielka BM, et al. Assessment of the cortisol awakening response: expert consensus guidelines. Psychoneuroendocrinology. 2016;63:414–432. doi: 10.1016/j.psyneuen.2015.10.010
- Kudielka BM, Hellhammer DH, Wüst S. Why do we respond so differently? Reviewing determinants of human salivary cortisol responses to challenge. Psychoneuroendocrinology. 2009;34(1):2–18. doi: 10.1016/j.psyneuen.2008.10.004
- Wolfram M, Bellingrath S, Kudielka BM. The cortisol awakening response (CAR) across the female menstrual cycle. Psychoneuroendocrinology. 2011;36(6):905–912. doi: 10.1016/j.psyneuen.2010.12.006
- Hutchison MG, Mainwaring L, Senthinathan A, et al. Psychological and physiological markers of stress in concussed athletes across recovery milestones. J Head Trauma Rehabil. 2017;32(3):38–48. doi: 10.1097/HTR.0000000000000252
- Hansen ÅM, Garde AH, Persson R. Sources of biological and methodological variation in salivary cortisol and their impact on measurement among healthy adults: a review. Scand J Clin Lab Invest. 2008;68(6):448–458. doi: 10.1080/00365510701819127
- Malfliet A, Lluch Girbés E, Pecos‐Martin D, et al. The influence of treatment expectations on clinical outcomes and cortisol levels in patients with chronic neck pain: an experimental study. Pain Pract. 2019;19(4):370–381. doi: 10.1111/papr.12749
- Stein PK, Pu Y. Heart rate variability, sleep and sleep disorders. Sleep Med Rev. 2012;16(1):47–66. doi: 10.1016/j.smrv.2011.02.005
- Stanley J, Peake JM, Buchheit M. Cardiac parasympathetic reactivation following exercise: implications for training prescription. Sports Med. 2013;43(12):1259–1277. doi: 10.1007/s40279-013-0083-4
- Lu C-L, Zou X, Orr WC, et al. Postprandial changes of sympathovagal balance measured by heart rate variability. Dig Dis Sci. 1999;44(4):857–861. doi: 10.1023/A:1026698800742
- Zimmermann-Viehoff F, Thayer J, Koenig J, et al. Short-term effects of espresso coffee on heart rate variability and blood pressure in habitual and non-habitual coffee consumers – a randomized crossover study. Nutr Neurosci. 2016;19(4):169–175. doi: 10.1179/1476830515Y.0000000018
- Inoue N, Kuroda K, Sugimoto A, et al. Autonomic nervous responses according to preference for the odor of jasmine tea. Biosci Biotechnol Biochem. 2003;67(6):1206–1214. doi: 10.1271/bbb.67.1206
- Quintana DS, Guastella AJ, McGregor IS, et al. Moderate alcohol intake is related to increased heart rate variability in young adults: implications for health and well-being. Psychophysiology. 2013;50(12):1202–1208. doi: 10.1111/psyp.12134
- Quintana DS, McGregor IS, Guastella AJ, et al. A meta-analysis on the impact of alcohol dependence on short-term resting-state heart rate variability: implications for cardiovascular risk. Alcohol Clin Exp Res. 2013;37:E23–E9. doi: 10.1111/j.1530-0277.2012.01913.x
- Fagius J, Karhuvaara S. Sympathetic activity and blood pressure increases with bladder distension in humans. Hypertension. 1989;14(5):511–517. doi: 10.1161/01.HYP.14.5.511
- Ben-Dror I, Weissman A, Leurer MK, et al. Alterations of heart rate variability in women with overactive bladder syndrome. Int Urogynecol J. 2012;23(8):1081–1086. doi: 10.1007/s00192-012-1738-7
- Heathers JAJ. Everything hertz: methodological issues in short-term frequency-domain HRV. Front Physiol. 2014;5:1–15. doi: 10.3389/fphys.2014.00177
- O’Brien IA, O’Hare P, Corrall RJ. Heart rate variability in healthy subjects: effect of age and the derivation of normal ranges for tests of autonomic function. Heart. 1986;55(4):348–354. doi: 10.1136/hrt.55.4.348
- Gisselman AS, D’Amico M, Smoliga JM. Optimizing intersession reliability of heart rate variability—the effects of artifact correction and breathing type. J Strength Cond Res. 2020;34(11). doi: 10.1519/JSC.0000000000002258
- Flatt AA, Esco MR. Heart rate variability stabilization in athletes: towards more convenient data acquisition. Clin Physiol Funct Imaging. 2016;36(5):331–336. doi: 10.1111/cpf.12233
- Quintana DS, Alvares GA, Heathers JAJ. Guidelines for reporting articles on psychiatry and heart rate variability (GRAPH): recommendations to advance research communication. Transl Psychiatry. 2016;6(5):1–10. doi: 10.1038/tp.2016.73
- Tarvainen MP, Niskanen J-P, Lipponen JA, et al. Kubios HRV – heart rate variability analysis software. Comput Methods Programs Biomed. 2014;113(1):210–220. doi: 10.1016/j.cmpb.2013.07.024
- van Eck MM, Nicolson NA. Perceived stress and salivary cortisol in daily life. Ann Behav Med. 1994;16(3):221–227.
- Smith KJ, Rosenberg DL, Timothy Haight G. An assessment of the psychometric properties of the perceived stress scale-10 (PSS10) with business and accounting students. Account Perspect. 2014;13(1):29–59. doi: 10.1111/1911-3838.12023