Abstract
The effect of oil-in-water submicron emulsion (SE) droplet surface charge on absolute bioavailability of a poorly water-soluble drug (griseofulvin, as model drug) after oral administration was studied in conscious rat. Positively, negatively, and neutrally charged SE were designed and characterized (size, polydispersity index, zeta potential, and pH). Three emulsion formulations, whose compositions included 40% oil phase and differed only in the nature of the emulsifying agent, were retained. Only the positively charged SE showed a higher area under the plasma concentration–time curve (AUC0 → ∞) in comparison with the tablet and with the other SE.
INTRODUCTION
The potential use of oil-in-water (o/w) emulsion to enhance gastrointestinal absorption of poorly soluble drugs has already been outlined (CitationCarrigan and Bates 1973). Several studies showed that the incorporation of drugs administered orally in o/w emulsion significantly increased the bioavailability compared with the equivalent aqueous suspension (CitationKakemi et al. 1972a, Citationb; CitationBates and Carrigan 1975; CitationBates and Sequeira 1975; CitationKimura et al. 1989; CitationKararli et al. 1992), the oily suspension (CitationCarrigan and Bates 1973), or the oily solution (CitationWagner, Gerard, and Kaiser 1966; Toguchi, Ogawa, and Shimamoto 1990).
Moreover, the submicron droplet size of o/w emulsion is able to improve the absorption compared with coarse emulsion (CitationNicolaos et al. 2003) or aqueous solution (CitationTiwari and Amiji 2006).
Another factor to be taken into consideration is the droplet's surface charge. From the previous in vivo studies performed with submicron o/w emulsion administered topically (CitationEzra et al. 1996; CitationYouenang Piemi et al. 1999; CitationKlang, Abdulrazik, and Benita 2000; CitationAbdulrazik et al. 2001) and nanoparticles (CitationEl-Shabouri 2002) or Self-Emulsifying Drug Delivery System (SEDDS) (CitationGershanik and Benita 1996) administered orally, the main conclusion is that positively charged colloidal drug carriers increase the potential uptake of slightly soluble drugs when compared with neutrally or negatively charged ones, thus improving their bioavailability. Recently, in vitro efficacy of a novel schistosomicidal drug was improved by incorporation into a cationic nanoemulsion (CitationDe Araujo et al. 2007).
The aim of our study was to investigate the influence of the surface charge of an o/w submicron emulsion on the bioavailability of a poorly soluble drug. Griseofulvin was chosen as a model drug for this investigation taking into consideration its very low aqueous solubility (CitationTur, Ching, and Baie 1997), its incomplete absorption (CitationChiou and Riegelman 1971; CitationKadir et al. 1986), and the lack of charge at neutral pH to ensure the lack of interaction with the surface charge of the droplets (CitationWashington 1996).
MATERIALS AND METHODS
Materials
Griseofulvin (Synopharm GmBh & Co, Barsbuttel, Germany) was purchased from Inresa (Bartenheim, France). Griseofulvin tablets (Grisefulline®) were provided by Sanofi-Synthelabo (Gentilly, France). Purified soybean oil was purchased from Société Industrielle des Oléagineux (Saint Laurent Blangy, France). Medium-chain-triglycerides (MCT) of caprylic and capric acids (Miglyol 812N®) and blends of mono-(50%), di-(40%), and triesters (8%) of caprilic and capric acids (Imwitor 742®) were purchased from Condea (Witten, Germany). Short-chain-triglycerides (SCT) (triacetin and tributyrin) and stearylamine, a cationic lipid, were purchased from Sigma-Aldrich (Saint Quentin Fallavier, France). Soybean lecithin (Lipoïd S40® and S100®) and egg lecithin (Lipoïd E80®) were kindly provided by Lipoïd GmBh (Ludwigshafen, Germany). Poloxamer 188 was purchased from BASF (Ludwigshafen, Germany). Polysorbate 80 and 85 (Tween 80® and 85®) were kindly provided by Uniqema (Everberg, Belgium). Macrogol 400 was purchased from Condea (Marl, Germany). All other ingredients used were of pharmaceutical grade.
Model Drug Solubility Assessment
The solubility of griseofulvin was assessed either in different pure oil phases (soybean oil, Miglyol 812N®, Imwitor 742®, tributyrin, and triacetin) or in blends of triacetin and emulsifying agents (polysorbate 80, polysorbate 85, poloxamer 188), according to the approached solubility method (CitationMulak and Cotty 1975).
Emulsion Formulation
The goal was to obtain submicron emulsions incorporating a sufficient amount of the model drug. The formulated emulsions, in spite of the different surface charges (negative, positive, or neutral), also had to exhibit quite a similar composition to allow further comparison for the in vivo pharmacokinetic study.
The optimization of the emulsion formulation was conducted in two stages. First, without incorporating the drug (unloaded emulsion) to investigate the emulsification capacity with no influences of the drug and second, by incorporating the model drug into the oily phase of the satisfactory formulations obtained in the first stage.
Emulsion Preparation
Griseofulvin was first dissolved in a blend of triacetin and polysorbate 85. Lecithins and stearylamine, when used, were dissolved in MCT oil or soybean oil. These two oil phases and aqueous phase were heated to 70°C. Both phases were then mixed and emulsified by a phase inversion method (CitationBecher 1965) using a high shear mixer (Ultraturrax® T25, Ika, Staufen, Germany). The emulsion was then stirred for 10 min in an ice bath using an ultrasonic homogenizer (Sonifier® 450, Bransen, Danbury, United States) to obtain a submicron emulsion.
Emulsion Characterization
Visual observations were made to assess macroscopic behavior, possible creaming, coalescence, and phase separation.
Particle size, polydispersity index (PI), and zeta potential measurements were performed using a Zetasizer Nano ZS® (Malvern Instruments, Orsay, France). Particle size and Pl were measured by photon correlation spectroscopy (PCS) et 25°C and 90° scattering angle. Zeta potential determinations were based on droplet electrophoretic mobility in aqueous medium. Each emulsion sample was diluted with purified water (1: 1000).
The pH of the emulsion samples was measured using a pH meter (phm 210, Meterlab, Copenhagen, Denmark).
The morphological characterization of emulsions was obtained by transmission electron microscopy (TEM). Griseofulvin loaded and unloaded emulsions were diluted 20-fold in water. A drop of the solution was placed on a copper grid with Formvar films Cu 200 Mesh® (Euromedex, Mundolsheime, France). Negative staining was then performed by adding a drop of uranyl acetate solution (2% w/v). The excess fluid was removed with filter paper. The grids were examined under transmission electron microscope (JEM-100S, JEOL, Tokyo, Japan) at accelerating voltage of 80 kV.
Only a short-term stability assessment of the optimized formulations at room temperature (+20°C) was monitored by visual observation and by measuring the zeta potential, the droplet size, Pl, and the pH over a period of 28 days. The objective of the study was to compare the effect of the surface droplet charge on the absorption of a model drug in vivo.
Control Formulations
Two control formulations were determined: a parenteral formulation and a control oral form. The parenteral solution containing 20 mg/mL was obtained by dissolving griseofulvin in macrogol 400. This solution was autoclaved for 20 min at 121°C.
Griseofulvin tablets (Grisefulline® 500 mg, Sanofi-Synthelabo, Gentilly, France) were used as the control oral formulation in this study and were dispersed in water. To allow further comparisons related to the in vivo pharmacokinetic study, the final concentration of the griseofulvin dispersion was fixed at 0.3%, like the emulsion formulation final concentration.
Rat Experimentation
The pharmacokinetic study was performed according to the Waeldele and Stoclet method (Citation1973) in conscious adult male Wistar rats (Charles River, Saint Aubin Les Elbeuf, France) weighing 316 ± 23 g. They were treated as recommended in the “Guide for the Care and Use of Laboratory Animals” prepared by the Institute of Laboratory Animal Resources.
The study was performed on six rats for the parenteral solution and the negatively charged submicron emulsion and on five rats for the positively charged submicron emulsion, the neutrally charged submicron emulsion and the tablet.
Before surgery, anesthesia was performed on the rats by an intraperitoneal injection of pentobarbital (50 mg/kg). A polyethylene catheter was permanently implanted into the thoracic aorta through the left carotid. The free end of the catheter was externalized at the base of the neck and covered with a small rubber plug to clamp the cannula. After surgery, the rats were placed in restraining cages. Blood sampling was realized via this cannula. Before and between every blood sampling, the cannula was filled with an aqueous solution of 0.9% of sodium chloride containing sodic heparin (100 IU/mL).
The rats were fasted overnight prior to the experiment and for 12 hr after the administration of the drug. Free access to water was allowed throughout the experiment. Oral formulations were delivered by an oral intubation catheter. Parenteral solution was injected through the catheter. The griseofulvin dose was 10 mg/kg whatever the galenic form administered. Oral and parenteral formulations were freshly prepared before administration.
During each experiment, 0.3 mL of blood was collected from the catheter and put into heparinized tubes at 1, 2, 3, 4, 5, 6, 8, 12, and 24 hr for oral formulations and at 15, 30, 60, and 90 min, 2, 4, 6, 12, and 24 hr for parenteral solution.
One pharmacokinetic study was performed on each rat because of a decrease of the bioavailability of griseofulvin after repeated administration (CitationBates and Carrigan 1975; CitationSchafer-Korting 1993).
HPLC Analysis of Griseofulvin Plasma Level
The level of griseofulvin in plasma samples was measured by a sensitive and specific HPLC method modified from that described by CitationZia et al. (1980). The plasma samples were deproteinized with an equal volume of acetonitrile containing 2.5 μg/mL of p-phenylphenol. A total of 50 μL of the supernatant was analyzed through an optimized system composed of a reversed phase column (Lichrocart® 250-4 Lichrospher 100 RP-18 5 μm, VWR International, Fontenay sous Bois, France) and a mobile phase solvent of 45% acetonitrile in 0.1 M acetic acid. The flow rate was 1 mL/min, and the separation was carried out at room temperature and monitored at 291 nm. The linearity (r2 = 0.993) and both between- and within-day reproducibility were assessed. The interassay and intraassay coefficient of variation were within the range 8.7–3.6% and 6.0–3.5% for griseofulvin concentration between 0.09 and 12 μg/mL. For this HPLC method, the limit of quantification was 0.06 μg/mL.
Pharmacokinetic Parameter Analysis
Griseofulvin pharmacokinetic parameters were determined with a noncompartmental analysis except the C0 of the parenteral solution which was estimated with a bicompartmental model. Elimination rate constant (ke) was estimated by linear least-square regression on the final plasmatic concentrations of griseofulvin. The half-life of elimination (t1/2) was calculated with the following equation: t1/2 = ln2/ke. For oral administration, Cmax and time to reach Cmax (Tmax) were determined through the observation of individual animal concentrations versus time curves. The area under the plasma concentration curve (AUC0 → ∞) was calculated with the trapezoidal rule and an extrapolation to infinite. The absolute bioavailability F of the oral dosage forms was estimated as the AUC0 → ∞ ratio of the oral dosage form to the parenteral solution.
The griseofulvin disposition profiles were determined by the deconvolution method. The rate of disposition dA%/dt was calculated between time n − 1 and time n + 1 from the percentage of available drugs as follows:
All these calculations were made with Kinetica 2.0 Software (Innaphase, France).
Statistical Analysis
A Mann–Whitney test was used to compare Tmax and absorption rate. An ANOVA test was used to compare the other pharmacokinetic parameters. The drug disposition profile comparison was carried out using the similarity test (CitationMoore 1996). This test was divided into a two-step process as follows: estimation of the difference factor f1 and evaluation of the similarity factor f2. To be able to conclude that a similarity exists between two curves, f2 must be superior to 50% and f1 inferior to 10%. Statistical significance was defined as p < .05.
RESULTS
Griseofulvin Solubility
The solubility results of griseofulvin are summarized in . Griseofulvin was neither soluble in soybean oil nor in MCT oil. Its solubility was higher in SCT and increased when the length of the chain decreased. The best solubility was obtained in triacetin (25 mg/g). Moreover, this solubility was improved by adding polysorbates emulsifying agents.
TABLE 1 Approached solubility of griseofulvin in different oil phases
Emulsion Formulations
Twenty-six formulations of unloaded emulsions have been investigated for emulsification capacity. gives a summary of the corresponding compositions assessed. Among the 26 formulations, 11 have shown a failure of the emulsification capacity with immediate phase disruption, 6 were nonsubmicronic, and 6 were discarded because of an inappropriate zeta potential. The remaining 3 formulations were assessed for incorporation of the model drug.
TABLE 2 Composition of the investigated unloaded emulsions
The compositions of submicron emulsions incorporating the model drug are given in . Because of the macroscopic precipitation of the drug with the highest concentration (0.5%), only the A1, B1, and C1 formulations have been retained for the pharmacokinetic study.
TABLE 3 Compositions (w/w %) of the emulsions incorporating the model drug at two griseofulvin concentrations
Characteristics of Emulsion Formulations
Characteristics (particle size, polydispersity index, zeta potential, and pH) of freshly prepared positively, negatively, and neutrally charged loaded or unloaded submicron emulsions are given in . Whatever the charge, the loaded emulsions exhibited a typical Gaussian distribution without droplets above 1 μm. The particle size distribution is given in . Differences in droplet size were observed between the three groups of emulsion. Negative submicron emulsions exhibited the lowest droplet sizes (under 200 nm), and neutral emulsions exhibited the highest droplet sizes (above 300 nm). Positive emulsion droplet sizes were in between. Moreover, polydispersity indices were similar for the positive and negative submicron emulsions and ≤0.1, and neutral emulsions showed a polydispersity index around 0.2. A slight difference of pH values between the three groups of formulations was observed. Globally, the characteristics of unloaded formulations were similar to those of the emulsions incorporating the model drug, showing no influence of griseofulvin inclusion into emulsions.
FIG. 1 Particle size distribution of freshly prepared (a) negatively, (b) positively, and (c) neutrally charged SE. Results are mean ± SD error bar of three determinations.
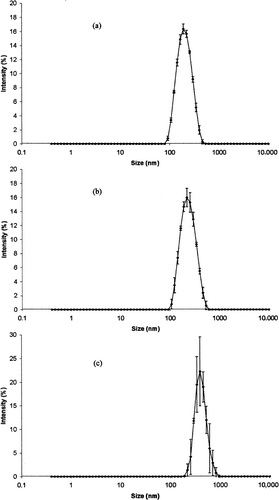
TABLE 4 Characteristics at day 0 of griseofulvin loaded and unloaded submicron emulsion
Results of TEM morphological characterization of the submicron emulsions distinguished three different structures related to the droplet surface charge and independent of the inclusion of the model drug. Results of TEM analysis of the loaded submicron emulsion are shown in . Shape differences can be noticed between the three groups. Negative emulsions exhibited droplets with typical globular shape, neutral emulsions exhibited irregular globular shape droplets, and positive emulsions handbag-like structures.
FIG. 2 TEM structure of the droplets of loaded (a) negatively, (b) positively, and (c) neutrally charged submicron emulsions.
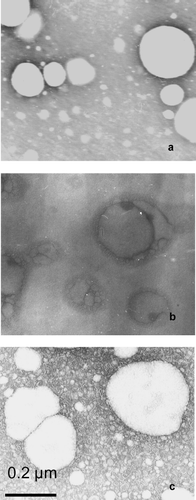
The short assessment of the stability of the emulsions showed that there were no modifications of the characteristics (particle size, PI, zeta potential, pH) of the negatively and positively charged emulsions stored at 20°C during 28 days (data not shown). On the contrary, the neutral emulsions exhibited a very short shelf life with a preservation of the initial characteristics during 3 hr only. Loaded and unloaded neutral emulsions were not submicronic 6 hr after preparation, exhibiting a maximum droplet size of approximately 5500 nm. The low stability of the neutral emulsion has justified for the in vivo evaluation to investigate only freshly prepared emulsions used within 2 hr after the preparation.
Pharmacokinetic Study
The griseofulvin plasma disappearance curves after intra-aortic injection and oral administration are represented in and .
FIG. 3 Griseofulvin plasma level concentration after intra-aortic administration of griseofulvin (n = 6). Results are mean ± SEM error bar.
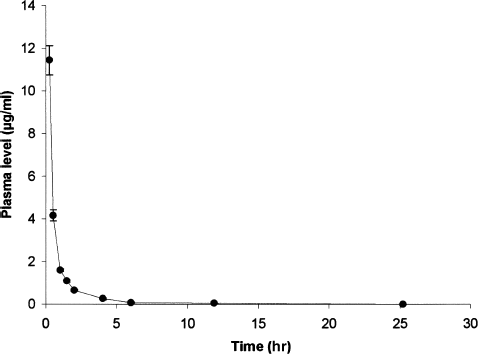
FIG. 4 Griseofulvin plasma level concentration after oral administration of positively charged SE (♦, n = 5), negatively charged SE (▵, n = 6), neutrally charged SE (▪, n = 5), and tablet (□, n = 5). SE: submicron emulsion. Results are mean ± SEM error bar.
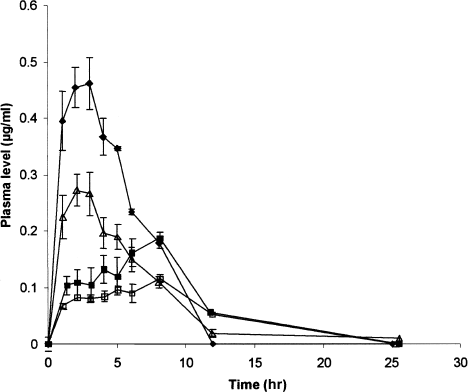
The results of pharmacokinetic study show a high inter-subject variability probably due to the experiment and the drug itself.
Pharmacokinetic parameters (mean ± SD) obtained after intra-aortic injection of griseofulvin (n = 6) show a distribution half-time of 0.13 ± 0.05 hr (t1/2α), an elimination half-time of 1.30 ± 0.80 hr (t1/2β), a clearance of 0.88 ± 0.25 l hr−1kg−1, a Co of 34.48 ± 16.90 μg/mL, and an AUCo → ∞ of 12.08 ± 3.39 μg hr mL−1.
Pharmacokinetic parameters obtained by noncompartmental analysis after oral administration of griseofulvin are listed in . Cmax of positively and negatively charged submicron emulsion is significantly higher than the tablet p < .05). Tmax is significantly higher for neutrally charged submicron emulsion and tablet in comparison with positively and negatively charged submicron emulsion (P < .05). There is no significant difference between the AUC0 → ∞ of negatively charged submicron emulsion, neutrally charged submicron emulsion, and tablet. Their absolute bioavailabilities are of the same order. In contrast, the positively charged submicron emulsion presents a significant increase of its AUC0 → ∞ (p < .05) and consequently, an increase of its bioavailability compared with the other oral formulations. The t1/2 and clearances of oral and parenteral formulations are not significantly different.
TABLE 5 Pharmacokinetic parameters of oral formulations
The griseofulvin disposition amount profiles and the disposition rates obtained by the deconvolution analysis during the first 12 hr are displayed in and . The statistical test () shows that the amount of griseofulvin absorbed was influenced by the type of submicron emulsion used ().
FIG. 5 Median cumulative percent dose absorbed versus time plot (a) and mean input absorption rate (b) extracted using deconvolution analysis for positively charged SE (♦, n = 5), negatively charged SE (▵, n = 6), neutrally charged SE (▪, n = 5), and tablet (□, n = 5). SE: submicron emulsion.
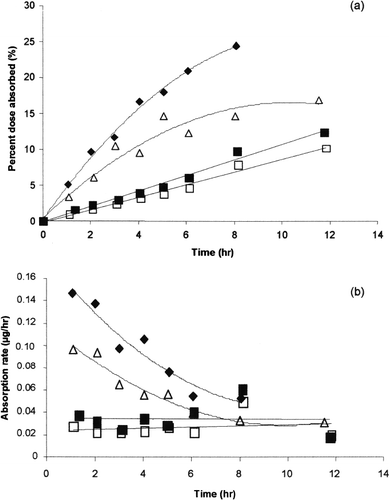
TABLE 6 Drug disposition profiles comparison (similarity test)
TABLE 7 Absorption parameters of oral formulations
The absolute bioavailability of griseofulvin as measured by the maximum percentage of the dose absorbed is consistent with the calculated values based on the ratio of AUCoral/AUCiv. There is no significant difference in the maximal amount of griseofulvin absorbed from negatively charged submicron emulsion, neutrally charged submicron emulsion, or tablet. In contrast, positively charged submicron emulsion shows a significant increase in its maximal amount of absorbed griseofulvin (p < .05). The positively and negatively charged submicron emulsions show a significantly higher absorption rate in comparison with the neutrally charged submicron and the tablet. The absorption rate is higher for the positively charged emulsion compared with the negatively charged one (p < .02).
DISCUSSION
Emulsion Formulation
The main objective was to formulate submicron emulsions with a controlled granulometry and a similar composition exhibiting positive, negative, or neutral charges at the droplet surface of the emulsion.
Optimization of the Formulation With Regard to the Oily Phase
The choice of the oily phase was constrained by the poor solubility of the model drug in the usual digestible oily phases used for submicron emulsion formulation (e.g., Long-chain-triglycerides (LCT), MCT). Only triacetin (SCT) was able to incorporate a satisfactory amount of griseofulvin. Triacetin has already been used for the emulsion formulation of a very poorly soluble drug, paclitaxel (CitationTarr, Sambandan, and Yalkowsky 1987). But the emulsion obtained was not submicronic and exhibited a poor stability, which has been correlated by the authors to a poor capacity of emulsification. This poor capacity of emulsification was confirmed in our preformulation study. The unloaded emulsions formulated including 20% of triacetin oil phase were unable to form an emulsion with either lecithin or a blend of nonionic surfactants (polysorbate 80/poloxamer 188) as emulsifying agents.
The use of lecithin, which is the reference emulsifying agent used in the submicron emulsion (CitationYang and Benita 2000), was not dispersible in the SCT oil, and the blend of nonionic surfactants was unable to form an emulsion giving an immediate phase disruption at the end of the emulsification process. The strategy followed was then to reduce the amount of triacetin by mixing it with conventional oily phases. An amelioration was obtained by the mixing of triacetin (1:1) or (1:3) with soybean oil or MCT oil, but the emulsions obtained were nonsubmicronic whatever the emulsifying agent used. Among the nonionic surfactive agents, only polysorbate 85 was able to give a submicron emulsion. Taking into consideration the solubility of griseofulvin, the mixing with longer triglyceride chains was correlated to a decrease in the solubility of the drug. Finally, only a 40% oil phase with a blend of triacetin/MCT (1:3) including 2.4% lecithin and 2% polysorbate 85 was able to form a submicron emulsion with a potential sufficient amount of incorporable drug. The formulation of a 40% oil phase submicron emulsion including nonconventional lipids is of interest with regard to the formulations of submicron emulsion of 10–20% of oil phase currently available, including mainly naturalor hemisynthetic lipids, fatty acids, and oils such as medium-or long-chain triglycerides (CitationYang and Benita 2000).
Optimization of the Emulsion Formulation With Regard to the Droplet Charge
Considering the conventional formulation of submicron emulsion, the droplet charge is usually negative and the charge is given by lecithin (charged phospholipids) (CitationBenita 1999). Because of the high proportion of oil phase, the most commonly used lecithin (Lipoïd® E80) gave a zeta potential of −33 mV (data not shown) lower than the conventional values of −45 mV (CitationWashington 1996). To improve the negative zeta potential, the use of Lipoïd® S40 solved the problem and was able to give a satisfactory value of zeta potential. Granulometric parameters were in conformity with conventional negative submicron emulsions with polydispersity index under 0.1, suggesting a narrow size distribution similar to commercialized injectable submicron emulsions (CitationHedeman et al. 1996). Morphological evaluation showed homogeneous regular globular shape droplets, which is a typical structure of triglyceride emulsions (CitationTeixeira et al. 2000).
The use of cationic lipids, stearylamine (CitationElbaz et al. 1993; CitationKlang et al. 1994; CitationZeevi et al. 1994; CitationKlang, Abdulrazik, and Benita 2000; CitationFang et al. 2004) or oleylamine (CitationRabinovich Guilett et al. 2004), has already been described for the formulation of positive submicron emulsions. Usual formulations of positively charged submicron emulsions include the use of lecithin with poloxamer 188 (CitationElbaz et al. 1993; CitationKlang et al. 1994; CitationKlang, Abdulrazik, and Benita 2000; CitationTamilvanan et al. 2005). Our formulations with lecithin and poloxamer were not submicronic (data not shown), so we substituted poloxamer 188 by polysorbate 85 (data not shown). We tried to decrease the amount of negative charge given by the lecithin by using Lipoïd® E80 and E100. Lipoïd® E100 is the lecithin least rich in charged phospholipids (CitationWashington 1996) but was unable to form an emulsion with 40% oil phase formulation. Lipoïd® E80 was able to give a positive submicron emulsion (+53 mV) with 0.2% of stearylamine. A higher concentration of stearylamine (0.5%) usually used in literature (CitationYouenang Piemi et al. 1999) gave an immediate phase disruption. The retained formulations exhibited good granulometric parameters with a low droplet size (around 220 nm) and a good polydispersity index (around 0.1) indicating a narrow distribution. Morphological analysis by TEM showing handbag-like structures is in accordance with previous studies performed on triglyceride emulsion and can be related to the presence of stearylamine (CitationTeixeira et al. 2000).
The formulation of a neutrally charged emulsion was a real challenge because it is contrary to the basic concept of stabilization of the interfacial film by electrostatic charges either positive or negative (CitationWashington 1996). The choice of Lipoïd® E100, a slightly purer grade of lecithin which has very little surface charge (CitationWashington 1996), was not able to give an emulsion except when poloxamer 188 was added to the formulation, but the zeta potential was still negative (−21 mV) (data not shown). To reach the target, we chose to neutralize the surface charge given by the Lipoïd® E80 (better for emulsification) by a small amount of stearylamine (0.01%). We were then able to perform a submicron emulsion with approximately no surface charge (−1.2 to +1 mV). In comparison with the negative and positive emulsions, particle size was higher (around 320 nm) and polydispersity index was around 0.2 giving an indication of a less stable system (CitationHaskell 1998). Moreover, morphological structure analysis by TEM () showed aggregation tendency of the droplets. The instability of the neutrally charged submicron emulsion could be related to the lack of charge. Zeta potential is known to be a predictive factor for stability (CitationWashington 1996). Emulsion with zeta potential values in the range −10 to +10 mV is found to be unstable (CitationBenita and Levy 1993; Le Roy Boehm and Fessi 2000). All in all, as expected, in conformity with the granulometric parameter analysis, the morphological aspect, and the zeta potential, this formulation was poorly stable: the structure was preserved for 3 hr; at 6 hr, the granulometric profile was modified. To avoid a problem of interpretation related to stability, all administered emulsions in rats were freshly prepared and used within 2 hr for the pharmacokinetic study.
Emulsions Incorporating the Model Drug
The griseofulvin solubility was the limiting factor and only 0.3% of the drug could be incorporated in the emulsion. At 0.5%, the drug visually precipitated. As expected, the lack of charge of the drug did not influence the formulation concerning the granulometric characteristic, zeta potential, and morphological structure. Griseofulvin can be classified in the group III of the Washington classification (1996) “drug poorly soluble both in water and in oil phase” and subclassified in c “uncharged at the formulation pH.” In accordance to the Washington classification (1996), drugs classified in the group III require complex emulsion formulation methods due to their solubility characteristics; this accounts for the complexity of the formulations performed in our study. On the other hand, the fact that the drug was uncharged (c subclassification) was a choice criterion for our study to ensure the lack of contribution of the drug to the surface charge of the droplets. Globally, the granulometric and morphological characteristics of the unloaded and loaded formulations were similar, indicating the lack of influence of the drug on the emulsifying capacity and stability.
Pharmacokinetic Study
Pharmacokinetic Parameters
The plasma disappearance curve for griseofulvin after intra-arterial injection is biexponential with similar distribution and elimination half-life to those reported by CitationLin and Symchowicz (1975) after intravenous injection in rats.
For oral formulations, two groups of pharmacokinetic profiles according to the Tmax are distinguished: positively and negatively charged submicron emulsions with a Tmax of about 2 hr versus neutrally charged submicron emulsion and tablet with a Tmax of about 5 hr. Similar results are reported in literature after oral administration of 10 mg/kg of griseofulvin from a coarse emulsion and a suspension to rabbits (CitationTur, Ch'ng, and Baie 1997).
Influence of the Formulation on Absorption of Griseofulvin
Previous studies reported that the percentage of the dose of griseofulvin absorbed ranged from 28 to 60% following oral administration of 50 to 100 mg/kg of an aqueous suspension in the rat (Bames and Boothroyd 1961; CitationSymchowicz and Wong 1966; CitationLin and Symchowicz 1975). This percentage ranged from 27 to 72% following administration of a tablet of 500 mg in humans (CitationRowland, Riegelman, and Epstein 1968). In our study, absorption of griseofulvin from the tablet is lower, probably due to a lower amount administered.
CitationCarrigan and Bates (1973) reported that the bioavailability of griseofulvin in rats given in a coarse oil-in-water emulsion (containing polysorbate, edible fat, corn oil, and water) was 2.5 times greater than an oil suspension (containing polysorbate and corn oil) and 1.6 times greater than an aqueous suspension (containing polysorbate and water). In our results, only the positively charged submicron emulsion led to a bioavailability 2.3 times higher than the tablet form, and no significant difference was found between the tablet and the negatively and neutrally charged emulsions. Moreover, the submicron characteristic was not a factor of bioavailability enhancement contrary to what has been shown for cefpodoxime proxetil (CitationNicolaos et al. 2003) or tacrolimus (CitationUno et al. 1999). Two hypotheses could explain these differences: the presence of SCT and the amount of oil simultaneously ingested with the drug.
Comparing the bioavailability of penclomedine from emulsion with different oil phases, CitationMyers and Stella (1992) showed that the tributyrin emulsion had a lower AUC than the other ones containing LCT or MCT.
In our study, rats received about 1.5 times less oil than in the study of CitationBates and Carrigan (1975). For the same ratio of emulsified lipids ingested with griseofulvin in man, it was shown that the bioavailability was better when the amount of lipids ingested was higher (CitationBates et al. 1977).
When comparing absorption (), the mean input rate time profile indicates that absorption was zero-order for the neutrally charged submicron emulsion and the tablet and one-order for the positively and negatively charged submicron emulsions. It seems that positively or negatively charged emulsions are able to modify the absorption properties of griseofulvin.
Influence of the Droplet Charge on Griseofulvin Absorption
The positively charged submicron emulsion did not show a similar absorption profile when compared with the negatively and neutrally charged ones. The bioavailability of griseofulvin and the absorption rate were enhanced when griseofulvin was administered from positively charged submicron emulsion compared with negatively and neutrally charged submicron emulsions. No significant difference was found between negatively and neutrally charged submicron emulsions. The droplet size is equivalent for the three emulsions and should not influence the results.
The enhancement of oral bioavailability by a cationic charge is in agreement with literature. The benefit of cationic charge has already been demonstrated for other nano dispersed systems, like SEDDS of progesterone (CitationGershanik and Benita 1996) or nanoparticles of cyclosporin (CitationEl Shabouri 2002), and has been correlated to the interaction between the positively charged droplets and the negative charge of the gastrointestinal tract (CitationGershanik, Benzeno, and Benita 1998; CitationGershanik et al. 2000).
In conclusion, this study suggests that the type of charge plays on important role in its ability to improve the absorption rate and the bioavailability of a poorly water-soluble drug. This work shows that submicron emulsion when positively charged is also able to improve the oral absorption of poorly soluble drugs like other nano dispersed systems. The formulation of the submicron emulsion results from a compromise between the solubility of the drug to be incorporated and the emulsifying capacity. Our work also shows the possibility to perform a stable submicron emulsion with 40% oil phase, which offers the possibility to both enhance the amount of drug to be included and preserve the interesting characteristics of the dispersed system in terms of small droplet size. This formulation approach could be used to improve the oral bioavailability of other poorly water soluble and poorly absorbable drugs.
We thank Pr Viviane Tricottet, René Lai-Kuen, Laboratoire de Microscopie Electronique, Faculté des Sciences Pharmaceutiques et Biologiques, Paris 5, 4 avenue de l'Observatoire, Paris, 75006, France, for their technical assistance in TEM characterization of the submicron emulsions.
REFERENCES
- M. Abdulrazik, S. Tamilvanan, K. Khoury, and S. Benita. (2001). Oculer delivery of cyclosporin A. II. Effect of submicron emulsion's surface charge on ocular distribution of topical cyclosporin A. STP Pharm. Sci. 11:427–432.
- M. J. Barnes, and B. Boothroyd. (1961). The metabolism of griseofulvin in mammals. Biochem. J. 78:41–43.
- T. R. Bates, and P. J. Carrigan. (1975). Apparent absorption kinetics of micronized griseofulvin after its oral administration on single- and multiple-dose regimens to rats as a corn oil-in-water emulsion and aqueous suspension. J. Pharm. Sci. 64:1475–1481.
- T. R. Bates, H. J. Pieniaszek, J. L. Sequeira, and J. E. Rassmussen. (1977). Gastrointestinal absorption of griseofulvin from corn oil-in-water emulsions. Arch. Dermatol. 113:302–306.
- T. R. Bates, and J. A. Sequeira. (1975). Bioavailability of micronized griseofulvin from corn oil-in-water emulsion, aqueous suspension, and commercial tablet dosage forms in humans. J. Pharm. Sci. 64:793–797.
- P. Becher. Technique of emulsificationEmulsions: Theory and Practice, 2nd ed. Reinhold, New York, (1965) 267–325.
- S. Benita. (1999). Prevention of topical and ocular oxidative stress by positively charged submicron emulsion. Biomed. Pharmacother. 53:193–206.
- S. Benita, and M. Y. Levy. (1993). Submicron emulsions as colloidal drug carriers for intravenous administration: comprehensive physicochemical characterization. J. Pharm. Sci. 82:1069–1079.
- P. J. Carrigan, and T. R. Bates. (1973). Biopharmaceutics of drugs administered in lipid-containing dosage forms. I: GI absorption of griseofulvin from an oil-in-water emulsion in the rat. J. Pharm. Sci. 62:1476–1479.
- W. L. Chiou, and S. Riegelman. (1971). Absorption characteristics of solid dispersed and micronized griseofulvin in man. J. Pharm. Sci. 60:1376–1380.
- S. C. De Araujo, A. C. De Mattos, H. F. Teixeira, P. M. Coelho, D. L. Nelson, and M. C. De Oliveira. (2007). Improvement of in vitro efficacy of a novel schistosomicidal drug by incorporation into nanoemulsions. Int. J. Pharm. 337:307–315.
- E. Elbaz, A. Zeevi, S. Klang, and S. Benita. (1993). Positively charged submicron emulsions—a new type of colloidal drug carrier. Int. J. Pharm. 96:R1–R6.
- M. H. El-shabouri. (2002). Positively charged nanoparticles for improving the oral bioavailability of cyclosporin-A. Int. J. Pharm. 249:101–108.
- R. Ezra, S. Benita, I. Ginsburg, and R. Kohen. (1996). Prevention of oxidative damage in fibroblast cell cultures and rat skin by positively changed submicron emulsion of α-tocopherol. Eur. J. Pharm. Biopharm. 42:294–298.
- J. Y. Fang, Y. L. Leu, C. C. Chang, C. H. Lin, and Y. H. Tsai. (2004). Lipid nano/submicron emulsions as vehicles for topical flurbiproien delivery. Drug deliv. 11:97–105.
- T. Gershanik, and S. Benita. (1996). Positively charged self-emulsifying oil formulation for improving oral bioavailability of progesterone. Pharm. Dev. Technol. 1:147–157.
- T. Gershanik, S. Benzeno, and S. Benita. (1998). Interaction of self-emulsifying lipid drug delivery system with the everted rat intestinal mucosa as a function of droplet size and surface charge. Pharm. Res. 15:863–869.
- T. Gershanik, E. Haltner, C. M. Lehr, and S. Benita. (2000). Charge-dependent interaction of self-emulsifying oil formulations with Caco-2 cells monolayers: binding, effects on barrier function and cytotoxicity. Int. J. Pharm. 211:29–36.
- R. J. Haskell. (1998). Characterisation of submicron systems via optical methods. J. Pharm. Sci. 87:125–129.
- H. Hedeman, M. Lück, T. Blunk, S. Frkjaer, and R. H. Müller. (1996). Fat emulsions based on structured lipids (1-3-specific triglycerides) an investigation of the in vitro interaction with plasma proteins. Clin. Nutr. 15:175–178.
- S. Kadir, T. Murakami, Y. Higashi, and N. Yata. (1986). Gastrointestinal absorption of griseofulvin from liquid organic acids and esters in rats. Int. J. Pharm. 33:235–242.
- K. Kakemi, H. Sezaki, S. Muranishi, H. Ogata, and K. Giga. (1972a). Mechanism of intestinal absorption of drugs from oil in water emulsions. II. Absorption from oily solution. Chem. Pharm. Bull. 20:715–720.
- K. Kakemi, H. Sezaki, S. Muranishi, H. Ogata, and S. Isemura. (1972b). Mechanism of intestinal absorption of drugs from oil in water emulsions. I. Chem. Pharm. Bull. 20:708–714.
- T. T. Kararli, T. E. Needham, M. Griffin, G. Schoenhard, L. J. Ferro, and L. Alcorn. (1992). Oral delivery of a renin inhibitor compound using emulsion formulations. Pharm. Res. 9:888–893.
- T. Kimura, K. Takeda, A. Kageyu, M. Toda, Y. Kurosaki, and T. Nakayama. (1989). Intestinal absorption of dolichol from emulsions and liposomes in rats. Chem. Pharm. Bull. 37:463–466.
- S. H. Klang, M. Abdulrazik, and S. Benita. (2000). Influence of emulsion droplet surface charge on indomethacin ocular tissue distribution. Pharm. Dev. Technol. 5:521–532.
- S. H. Klang, J. Frucht-Pery, A. Hoffman, and S. Benita. (1994). Physicochemical characterization and acute toxicity evaluation of a positively charged submicron emulsion vehicle. J. Pharm. Pharmacol. 46:986–993.
- A. L. Le Roy Boehm, and H. Fessi. (2000). Pharmaceutical applications of zeta potential - Interest for colloidal drug carriers characterization. J. Pharm. Belg. 55:40–48.
- C. Lin, and S. Symchowicz. (1975). Absorption, distribution, metabolism, and excretion of griseofulvin in man and animals. Drug Metab. Rev. 4:75–95.
- J. Moore. (1996). Mathematical comparison of dissolution profiles. Pharm. Technol. 20:64–74.
- G. Mulak, and J. Cotty. (1975). Solubilite et constante dielectrique. Sci. Technol. Pharm. 4:281–288.
- R. A. Myers, and V. J. Stella. (1992). Systemic bioavailability of penolomedine (NSC-338720) from oil-in-water emulsions administered intraduodenally to rats. Int. J. Pharm. 78:217–226.
- G. Nicolaos, S. Crauste-Manciet, R. Farinotti, and D. Brossard. (2003). Improvement of cefpodoxime proxetil oral absorption in rats by an oil-in-water submicron emulsion. Int. J. Pharm. 263:165–171.
- L. Rabinovich-Guilatt, P. Couvreur, G. Lambert, D. Goldstein, S. Benita, and C. Dubernet. (2004). Extensive surface studies help to analyse zeta potential data: the case of cationic emulsions. Chem. Phys. Lipids 131:1–13.
- M. Rowland, S. Riegelman, and L. Epstein. (1968). Absorption kinetics of griseofulvin in man. J. Pharm. Sci. 57:984–989.
- M. Schafer-Korting. (1993). Pharmacokinetic optimisation of oral antifungal therapy. Clin. Pharmacokinet. 25:329–341.
- S. Symchowicz, and K. K. Wong. (1966). Metabolism of griseofulvin-14C; studies in vivo. Biochem. Pharmacol. 15:1595–1600.
- S. Tamilvanan, S. Schmidt, R. H. Müller, and S. Benita. (2005). In vitro adsorption of plasma proteins onto the surface (charges) modified-submicron emulsions for intravenous administration. Eur. J. Pharm. Biopharm. 59:1–7.
- B. D. Tarr, T. G. Sambandan, and S. H. Yalkowsky. (1987). A new parenteral emulsion for the administration of taxol. Pharm. Res. 4:162–165.
- H. Teixeira, C. Dubernet, V. Rosilio, S. Benita, J. Lepault, I. Erk, and P. Couvreur. (2000). New bicompartmental structures are observed when stearylamine is mixed with triglyceride emulsions. Pharm. Res. 17:1329–1332.
- S. B. Tiwari, and M. M. Amiji. (2006). Improved oral delivery of paclitaxel following administration in nanoemulsion formulations. J. Nanosci. Nanotechnol. 6:3215–3221.
- H. Togushi, Y. Ogawa, and T. Shimamoto. (1990). Effects of the physicochemical properties of the emulsion formulation on the bioavailability of ethyl-2-chloro-3-[4-(2-methyl-2-phenylpropyloxy)phenyl]propionate in rats. Chem. Pharm. Bull. 38:2797–2800.
- K. M. Tur, H. S. Ch'ng, and S. Baie. (1997). Use of bioadhesive polymer to improve the bioavailability of griseofulvin. Int. J. Pharm. 148:63–71.
- T. Uno, T. Kazui, Y. Suzuki, H. Hashimoto, K. Suzuki, and B. A. H. Muhammad. (1999). Pharmacokinetic advantages of a newly developped tacrolimus oil-in-water-type emulsion via the enteral route. Lipids 34:249–254.
- G. Waeldele, and J. C. Stoclet. (1973). Permanent catheterization of the thoracic aorta. Direct measure of blood pressure, injection of substances, and blood sampling in the waking rat. J. Physiol. 66:357–366.
- J. G. Wagner, E. S. Gerard, and D. G. Kaiser. (1966). The effect of the dosage form on serum levels of indoxole. Clin. Pharmacol. Ther. 7:610–619.
- C. Washington. (1996). Stability of lipid emulsions for drug delivery. Adv. Drug Deliv. Rev. 20:131–145.
- S. H. Yang, and S. Benita. (2000). Enhanced absorption and drug targeting by positively charged submicron emulsions. Drug Deliv. Res. 50:476–486.
- M. P. Youenang Piemi, D. Korner, S. Benita, and J. P. Marty. (1999). Positively and negatively charged submicron emulsions for enhanced topical delivery of antifungal drugs. J. Control Release 58:177–187.
- A. Zeevi, S. Klang, V. Alard, F. Brossard, and S. Benita. (1994). The design and characterization of a positively charged submicron emulsion containing a sunscreen agent. Int. J. Pharm. 108:57–68.
- H. Zia, W. J. Proveaux, J. P. O'donnel, and J. K. Ma. (1980). Chromatographic analysis of griseofulvin and metabolites in biological fluids. J. Chromatograph. 181:77–84.