Abstract
It has previously been reported that a peptide sequence of T7 phage protein p17 mediates uptake of its cargo by liver parenchymal cells. The aim of this study was to identify the phage-binding receptor. The involvement of LRP was confirmed by the observations that phage binding to Hepa 1c1c7 cells was inhibited by the LRP-binding receptor-associated protein, LRP-deficient mouse embryonic fibroblasts bound phage with lower efficiency than their wild-type counterparts, and using mouse models with ablated LRP liver expression. The identification of LRP as a cognate receptor for this sequence offers a new ligand-receptor combination for hepatocyte delivery of therapeutic agents.
Keywords::
Abbreviations: | ||
ASGPr, | = | asialoglycoprotein receptor |
GPr, | = | galactose particle receptor |
HSPG, | = | heparan sulfate proteoglycans |
Lf, | = | lactoferrin; LDL, low density lipoprotein |
LDLr, | = | low density lipoprotein receptor |
LRP, | = | LDLr-related protein |
MEF, | = | mouse embryonic fibroblasts |
Introduction
We have previously reported that a peptide sequence present in the T7 phage tail fiber protein (p17) efficiently targets phage particles and p17 derivatives to hepatocytes in vivo. Up to 95% of injected phage is taken up by liver, with ∼ up to 80% of the phage being associated with hepatocytes. The rest of the liver-sequestered phage is mostly associated with Kupffer cells (CitationSokoloff et al., 2003; CitationLudtke et al., 2007). The phage reaches hepatocytes within 5 min after injection. Preinjection of asialofetuin has no effect on phage uptake, suggesting that the uptake is not mediated by asialoglycoprotein receptor (ASGPr) (CitationSokoloff et al., 2003). Immunohistochemistry studies have shown that a large portion of hepatocyte-associated phage is internalized (CitationSokoloff et al., 2003; CitationLudtke et al., 2007). A synthetic analogue of the p17 sequence imparts hepatocyte targeting onto fusion proteins, polymers, siRNA, liposomes, and DNA particles (CitationWong et al., 2006). While these observations suggest that the p17 sequence can potentially be used as a hepatocyte targeting ligand in various delivery applications, the mechanism of the p17 interaction with hepatocytes has so far remained unknown. The study identifies LRP as a major hepatocyte receptor that mediates phage uptake.
Materials and methods
In vitro phage binding assay
Hepa 1c1c7 cells were obtained from ATCC (cat. # CRL-2026). Wild-type and LRP-deficient MEF were obtained from ATCC (cat. # CRL-2214 and CRL-2216, respectively). Approximately 400,000 cells were plated into 35 mm wells and grown overnight in 10% FCS in DMEM. Cells were washed once with PBS and then fixed with 2% formaldehyde (Sigma, St. Louis, MO) for 30 min at RT and washed three times with 2 ml portions of PBS. Cell treatments were conducted as described in the figure legends. 109 pfu of phage was applied to the cells in 1 ml of OptiMEM (Invitrogen) and incubated at 37°C for 30 min. Unbound phage was removed by washing cells three times with 2 ml portions of PBS. Bound phage was eluted with 0.5 ml of 1% SDS in TBS for 10 min at RT. The amount of eluted phage was determined using a plaque assay (CitationNovagen, 2000).
Tail vein injections
109 or 1011 pfu of phage was slowly injected via the tail vein of mice in 250–300 μl of PBS. After 30 min, the liver was perfused with 30–50 ml of PBS containing 1 U/ml heparin through a 24 gage catheter inserted into the inferior vena cava and placed into a 50 ml tube containing 20 ml of T7 lysis buffer (PBS/2% Triton X-100/1M NaCl) (CitationSokoloff et al., 2003). Livers were homogenized and infective phage content was determined using a plaque assay (CitationSokoloff et al., 2003).
LRP gene ablation experiments
Adenovirus expressing Cre from the CMV promoter (Ad5CMVCre) was purchased from the Gene Transfer Vector Core at the University of Iowa. Approximately 3 × 1011 Ad5CMVCre particles (∼ 3 × 109 pfu) was injected into the tail vein of mice following a protocol described by CitationRohlmann et al. (1996). Twenty days later, when the liver LRP expression level was expected to decrease by ∼ 100% (CitationRohlmann et al., 1998), 1011 pfu of phage was injected. The amount of liver-sequestered phage was determined 30 min later as described above in the section Tail vein injections. Mice lacking LRP in their hepatocytes were produced by crossing LRP-Flox +/+ LDLr −/− mice (CitationRohlmann et al., 1998) with Cre +/+ mice (B6.Cg-Tg(Alb-cre)21Mgn/J) expressing Cre from the albumin promoter (Jackson Lab; Bar Harbor, ME). F2 progeny and backrosses between F2 and LRP-Flox +/+ LDLr −/− mice were used in all experiments. Genotyping was done following an established protocol (CitationRohlmann et al., 1998). Phage detection in liver by immunohistochemistry was described previously (CitationSokoloff et al., 2003). Briefly, frozen liver sections (5–7 μm) were fixed in 2% formaldehyde, blocked with 10% normal donkey serum, and incubated with rabbit anti-T7 antibody (IgG fraction depleted against Hela cells) followed by Cy3-conjugated donkey anti-rabbit antibody. Stained sections were counterstained with To-Pro-3 to visualize nuclei and with Alexa 488-phalloidin to visualize actin filaments near basolateral and bile canalicular membranes. Sections were analyzed under a confocal Zeiss LSM 510 microscope and images were constructed as a flattened projection of a stack of twelve 0.4 μm optical sections.
Statistics
Phage liver and blood contents were compared among groups using 1-way analysis of variance (ANOVA). Group comparisons were adjusted for the phage concentration using 2-way ANOVA. P-values were adjusted for multiple pairwise comparisons using Tukey’s Honest Significant Difference method. A two-sided 5%-level test result was regarded as statistically significant.
Results
Development of a phage binding assay
To facilitate the identification of the P17-binding hepatocyte receptor, an in vitro phage-binding assay was developed based on the observation that the T7 phage 20-6F, which targeted hepatocytes in vivo (CitationSokoloff et al., 2003), efficiently bound to immortalized mouse hepatocytes, Hepa 1c1c7 (). Approximately 2–4% of input phage 20-6F were bound under these conditions. The phage binding was specific since its efficiency was dramatically reduced when HeLa cells were substituted for Hepa 1c1c7 cells or when phage 20-6F was replaced with mutant phage 20-6 that did not target hepatocytes in vivo (CitationSokoloff et al., 2003). The phage binding efficiency for these two sets of conditions was ∼ 0.1% and ∼ 0.01%, respectively. For direct quantitation of phage binding, Hepa 1c1c7 cells were fixed first with formaldehyde, which did not significantly affect the phage binding activity of these cells while blocking phage internalization. The suppression of phage internalization by incubating cells at 4°C could not be employed as it interfered, for undetermined reasons, with phage-cell interactions (data not shown).
Figure 1. Phage binding to Hepa 1c1c7 cells and MEF. (A and B) Hepa 1c1c7 cells incubated with liver-targeting phage 20-6F in OptiMEM in the presence or absence of Lf or RAP. (C) Wild-type and LRP-deficient MEF (congenic cell line PEA-13) incubated with liver-targeting phage 20-6F or non-targeting phage 20-6 in OptiMEM. The amount of cell-bound phage was measured by a plaque assay. Duplicate samples were used. Data are shown as mean ± SE.
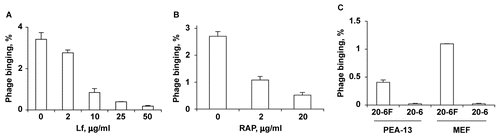
Phage binding to Hepa 1c1c7 cells and hepatocytes is inhibited by Lf
Phage binding to Hepa 1c1c7 cells was tested in the presence of potential binding competitors that reacted with major hepatocyte receptors (CitationShayakhmetov et al., 2005). Polymerized BSA, asialofetuin and low density lipoprotein (LDL), which react with the scavenger receptor SR-BI, ASGPr and LDL receptor (LDLr), respectively, had no significant effect on phage binding (data not shown). In contrast, bovine lactoferrin (Lf), which reacts with LRP and heparan sulfate proteoglycans (HSPG) (CitationHerz & Strickland, 2001), considerably inhibited phage binding in a dose-dependent manner (). Bovine Lf reacts with LRP due to the presence of a positively charged cluster of four R residues at the Lf N-terminus, which relates it to the major LRP ligand, ApoE (CitationHuettinger et al., 1988; Citation1992). Notably, the phage p17 targeting sequence also contains several positively charged residues that are critical for hepatocyte targeting (CitationSokoloff et al., 2003). Using our newly designed p17 display system, with the p17 targeting sequence replaced with a peptide display site, we have found that all p17-displayed random sequences selected for hepatocyte targeting are also positively charged (CitationLudtke et al., 2007).
Phage binding to Hepa 1c1c7 cells in vitro is mediated by LRP
To determine whether phage bound to LRP or HSPG or both, Hepa 1c1c7 cells were incubated with phage after being treated with heparinase I or heparinase III. These treatments had no effect, suggesting that HSPG did not contribute to phage binding. The involvement of LRP in the binding process was confirmed by the observations that the LRP-binding protein RAP (receptor-associated protein) strongly inhibited phage binding to Hepa 1c1c7 cells (), and that the LRP-deficient mouse embryonic fibroblasts (MEF; line PEA-13) bound phage far less efficiently than wild type MEF (). Overall, these observations provided strong evidence that LRP served as a phage-binding receptor in vitro. The preinjection of mice with 1 mg of Lf 5 min prior to injection of phage reduced the efficiency of phage uptake by liver from ∼ 72 to ∼ 40%, which suggested that LRP also reacted with phage in vivo. Asialofetuin was injected as a negative control (CitationSokoloff et al., 2003), and showed no effect on phage uptake (data not shown).
LRP mediates phage binding to hepatocytes in vivo
Phage 20-6F is taken up in vivo by both hepatocytes and Kupffer cells, with up to 80% of liver-sequestered phage being associated with the hepatocyte fraction (CitationSokoloff et al., 2003). Since LRP is expressed both by hepatocytes and macrophages (CitationMoestrup, Gliemann, & Pallesen, 1992; CitationLupu et al., 1994), the phage uptake by hepatocytes and Kupffer cells could proceed through the same, LRP-mediated mechanism. The general LRP involvement in phage uptake by liver was demonstrated employing a ‘floxed’ LRP mouse model, in which the LRP gene was removed from liver cells by injection of Cre-expressing adenovirus (CitationRohlmann et al., 1998). Mice without liver LRP showed ∼ 3-fold reduction (p = 0.0002) in liver phage uptake as determined by a plaque assay (, closed bars). A combination of confocal microscopy and immunohistochemistry (CitationSokoloff et al., 2003) showed that just a small portion of the phage sequestered by liver in LRP-deficient mice was inside hepatocytes, with the bulk of phage being present in clusters that apparently corresponded to Kupffer cells (). The decrease in phage uptake was predictably accompanied by an increase (p = 0.0002) in the proportion of injected phage that remained in the blood (, open bars).
Figure 2. Reduced phage uptake by LRP-deficient liver in Ad5/Cre-injected mice. (A) Phage contents in liver and blood were determined by a plaque assay. Data are shown as mean ± SD, n = 3. (B) Immunohistochemistry of normal liver after phage injection. (C) Immunohistochemistry of LRP-deficient liver after phage injection. Red, green, and blue colors correspond to phage, actin, and nuclei, respectively.
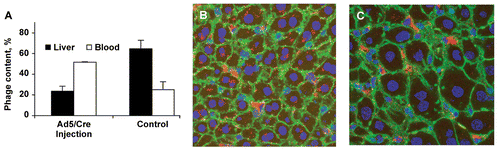
The role of LRP in phage uptake by hepatocytes was more specifically demonstrated in another ‘floxed’ mouse model lacking LRP, where Cre was specifically expressed in hepatocytes from the albumin promoter. The liver phage uptake in LRP−/LDLr+ mice, as compared to normal mice (LRP+/LDLr+), was reduced by ∼ 50% (; p = 0.002). The reduction was corroborated by immunohistochemistry data (). It appeared that LRP+/LDLr+ hepatocytes took up phage somewhat more efficiently than those lacking LDLr (LRP+/LDLr−; ). However, the difference did not reach the level of statistical significance (p = 0.06). The role of LDLr was also assessed by comparing the phage uptake by liver in LRP−/LDLr+ and LRP−/LDLr− mice. No statistically significant difference was observed for this combination either (; p = 0.86). Moderate contribution of LDLr to the phage uptake by hepatocytes was detected in immunohistochemical experiments, where the residual phage uptake observed in LRP−/LDLr+ mice declined to nearly undetectable levels in LRP−/LDLr− mice ().
Figure 3. Reduced phage uptake by LRP-deficient liver in ‘floxed’ LRP mice expressing Cre. (A) Liver phage uptake as determined by a plaque assay. Data are shown as mean ± SD, n = 4. (B) Confocal images of immunostained LRP+LDLr+ liver sections (Flox+/+, Cre−, LDLr+/+). (C) Confocal images of immunostained LRP−LDLr+ liver sections (Flox+/+, Cre+, LDLr+/+). (D) Confocal images of immunostained LRP−LDLr+ liver sections (Flox+/+, Cre+, LDLr−/−). Red, green, and blue image colors correspond to phage, actin, and nuclei, respectively.
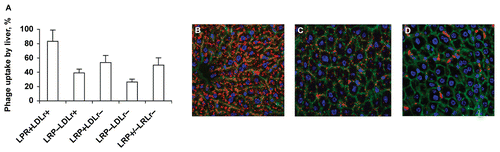
Discussion
This study demonstrates that the T7 phage uptake by hepatocytes is largely mediated by an LDLr family member, LRP. LRP binds a broad variety of ligands, including lipoproteins, clotting factors, proteinases, proteinase-inhibitor complexes, intracellular proteins, extracellular matrix proteins, viruses, and bacterial toxins (CitationHerz & Strickland, 2001). Complement-type, cysteine-rich repeats clustered in four of five structural units commonly found in the LDLr family members are largely responsible for the bulk of the LRP binding activity. The majority of ligand-binding sites are located in the LRP clusters II and IV, with most ligands binding equally well to both clusters. Most ligands bind to LRP with high affinity due to their interactions with multiple ligand-binding repeats (CitationWillnow & Herz, 1994; CitationSpringer, 1998). The result from recent studies suggests that LRP mediates the liver uptake of adenoviral particles complexed to blood proteins from the coagulation and complement cascades, such as FIX, FX, and complement C4-binding protein (CitationShayakhmetov et al., 2005; CitationKalyuzhniy et al., 2008; CitationWaddington et al., 2008).
Detectable amounts of LRP mRNA are observed in spleen, thymus, bone, heart, kidney, muscle, and placenta. The highest levels of LRP mRNA are observed in liver, brain, lung, and intestine (CitationHerz et al., 1988; CitationMoestrup et al., 1992). Detailed immunohistological analysis showed that LRP is expressed in a variety of cell types, including hepatocytes, Kupffer cells, lymph and spleen node macrophages, fibroblasts, smooth muscle cells, monocytes, syncitiotrophoblasts, astrocytes, and several types of epithelial cells (CitationMoestrup et al., 1992; CitationLupu et al., 1994). However, the LRP-mediated endocytosis of apoE-enriched chylomicron remnants, beta-VLDL, and LRP antibodies occurs largely in liver, where both hepatocytes and Kupffer cells are accessible to small particles due to vasculature fenestration (CitationKita, Brown, et al., 1982; CitationKita, Goldstein, et al., 1982; CitationHerz et al., 1990). The predominant occurrence of the LRP internalization activity in liver is consistent with our observations that up to 95% of T7 phage injected into mice is recovered from liver provided that the phage is rendered ‘invisible’ to natural IgM antibodies by removing its coat protein C-terminus (CitationSokoloff et al., 2000, Citation2003).
Importantly, LRP expression is not observed in normal endothelial cells despite the presence in these cells of some LRP mRNA (CitationMoestrup et al., 1992; CitationLupu et al., 1994). Nor is LRP expressed in mature blood cells, dendritic cells, or lymphoid cells (CitationMoestrup et al., 1992). The absence of LRP expression in these cells, which are readily accessible to blood-born LRP-targeting particles and proteins, is an important prerequisite for the therapeutic use of LRP-targeted bulky agents, as it essentially limits the targeting of blood-restricted agents to hepatocytes and macrophages. Macrophages can be differentially and reversibly suppressed, which would enhance the specificity of hepatocyte targeting (CitationSokoloff et al., 2003; CitationLudtke et al., 2007; CitationRussell et al., 2007). Also, internalized phage is degraded by Kupffer cells much faster than by hepatocytes (CitationSokoloff et al., 2003), which suggests that the endosomal release and functional expression in Kupffer cells of genes, oligonuclotides, and siRNA is less likely than in hepatocytes. Due to the LRP expression in many tissues, specific LRP-mediated targeting of liver cells is restricted to particles and proteins that are large enough to avoid significant extravasation (CitationHerz et al., 1990). The contribution of LDLr to the p17 targeting of hepatocytes is rather limited and is likely to be even smaller in normal animals as compared to LRP knock-outs, since the latter over-express LRP by at least 2-fold (CitationRohlmann et al., 1998). The limited role of LDLr in phage uptake is consistent with our previous observations that only the phage-displayed sequence derived from ApoE, which reacts with both LRP and LDLr, but not the sequence derived from ApoB, which mostly reacts with LDLr, mediates efficient phage internalization by hepatocytes (CitationLudtke et al., 2007).
Unlike adenoviruses that react with LRP via binding first to LRP-reactive plasma proteins, T7 phage evidently binds to liver cells via direct phage-LRP interactions. Using the same methodology that was successfully employed earlier to identify the blood factors required for adenovirus-LRP interactions (CitationShayakhmetov et al., 2005; CitationKalyuzhniy et al., 2008; CitationWaddington et al., 2008), we found that phage which was passed through perfused liver (in the absence of blood) was taken up by liver cells as efficiently as if injected through the tail vein (data not shown). Direct phage-LRP interactions also were suggested by phage binding to cultured cells in OptiMEM ().
The LRP delivery route is rather similar in terms of its cell specificity to the ASGPr route. In addition to the ASGPr expressed on hepatocytes, the ASGPr-reacting oligosaccharide ligands typically bind to the galactose particle receptor (GPr) expressed on Kupffer cells (CitationLehrman, Haltiwanger, & Hill, 1986; CitationLehrman & Hill, 1986; CitationSliedregt et al., 1999; CitationRensen et al., 2001). The uptake ratio between the ASGPr and GPr is defined in a complex manner by the size of a targeting agent and the surface distribution and exposure of Gal ligands. As with the LRP route, the specificity of the ASGPr route is enhanced by suppressing Kupffer cells (CitationLehrman, Pizzo, et al., 1986; CitationBijsterbosch & Van Berkel, 1990; CitationBiessen et al., 1994; CitationKuiper et al., 1994; CitationSliedregt et al., 1999). The specificity of the ASGPr route also is increased by blocking the expression of ASGPr on activated T cells (CitationPark, Kim, & Cho, 2006).
To summarize, this study demonstrates that the LRP targeting by the protein-incorporated or conjugated synthetic p17 sequence represents a new promising ligand-receptor combination for therapeutic delivery of particulate and proteinaceous agents to hepatocytes. This targeting route is suited particularly well for the hepatocyte delivery of proteinaceous agents that are readily amenable to genetic but not chemical modifications.
Acknowledgements
This study was partially supported by the NIH grant R01DK065090. The authors would like to thank Joachim Herz for the useful discussion of this study. Michael Evans’ help with conducting statistical analysis is greatly appreciated.
Declaration of interest: Dr Jon A. Wolff has a proprietary interest in Mirus Bio Corp. The authors alone are responsible for the content and writing of the paper.
References
- Biessen, E.A., Bakkeren, H.F., Beuting, D.M., Kuiper, J., Van Berkel, T.J. (1994). Ligand size is a major determinant of high-affinity binding of fucose- and galactose-exposing (lipo)proteins by the hepatic fucose receptor. Biochem J. 299, 291–6.
- Bijsterbosch, M.K., Van Berkel, T.J. (1990). Uptake of lactosylated low-density lipoprotein by galactose-specific receptors in rat liver. Biochem J. 270, 233–9.
- Herz, J., Hamann, U., Rogne, S., Myklebost, O., Gausepohl, H., Stanley, K.K. (1988). Surface location and high affinity for calcium of a 500-kd liver membrane protein closely related to the LDL-receptor suggest a physiological role as lipoprotein receptor. Embo J. 7, 4119–27.
- Herz, J., Kowal, R.C., Ho, Y.K., Brown, M.S., Goldstein, J.L. (1990). Low density lipoprotein receptor-related protein mediates endocytosis of monoclonal antibodies in cultured cells and rabbit liver. J Biol Chem. 265, 21355–62.
- Herz, J., Strickland, D.K. (2001). LRP: a multifunctional scavenger and signaling receptor. J Clin Invest. 108, 779–84.
- Huettinger, M., Retzek, H., Eder, M., Goldenberg, H. (1988). Characteristics of chylomicron remnant uptake into rat liver. Clin Biochem. 21, 87–92.
- Huettinger, M., Retzek, H., Hermann, M., Goldenberg, H. (1992). Lactoferrin specifically inhibits endocytosis of chylomicron remnants but not alpha-macroglobulin. J Biol Chem. 267, 18551–7.
- Kalyuzhniy, O., Di Paolo N.C., Silvestry, M., Hofherr, S.E., Barry, M.A., Stewart, P.L., Shayakhmetov, D.M. (2008). Adenovirus serotype 5 hexon is critical for virus infection of hepatocytes in vivo. Proc Natl Acad Sci USA. 105, 5483–8.
- Kita, T., Brown, M.S., Bilheimer, D.W., Goldstein, J.L. (1982). Delayed clearance of very low density and intermediate density lipoproteins with enhanced conversion to low density lipoprotein in WHHL rabbits. Proc Natl Acad Sci USA. 79, 5693–7.
- Kita, T., Goldstein, J.L., Brown, M.S., Watanabe, Y., Hornick, C.A., Havel, R.J. (1982). Hepatic uptake of chylomicron remnants in WHHL rabbits: a mechanism genetically distinct from the low density lipoprotein receptor. Proc Natl Acad Sci USA. 79, 3623–7.
- Kuiper, J., Bakkeren, H.F., Biessen, E.A., Van Berkel, T.J. (1994). Characterization of the interaction of galactose-exposing particles with rat Kupffer cells. Biochem J. 299, 285–90.
- Lehrman, M.A., Hill, R.L. (1986). The binding of fucose-containing glycoproteins by hepatic lectins. Purification of a fucose-binding lectin from rat liver. J Biol Chem. 261, 7419–25.
- Lehrman, M.A., Haltiwanger, R.S., Hill, R.L. (1986). The binding of fucose-containing glycoproteins by hepatic lectins. The binding specificity of the rat liver fucose lectin. J Biol Chem. 261, 7426–32.
- Lehrman, M.A., Pizzo, S.V., Imber, M.J., Hill, R.L. (1986). The binding of fucose-containing glycoproteins by hepatic lectins. Re-examination of the clearance from blood and the binding to membrane receptors and pure lectins. J Biol Chem. 261, 7412–8.
- Ludtke, J.J., Sololoff, A.V., Wong, S.C., Zhang, G., Wolff, J.A. (2007). In vivo selection and validation of liver-specific ligands using a new T7 phage peptide display system. Drug Deliv. 14, 357–69.
- Lupu, F., Heim, D., Bachmann, F., Kruithof, E.K. (1994). Expression of LDL receptor-related protein/alpha 2-macroglobulin receptor in human normal and atherosclerotic arteries. Arterioscler Thromb. 14, 1438–44.
- Moestrup, S.K., Gliemann, J., Pallesen, G. (1992). Distribution of the alpha 2-macroglobulin receptor/low density lipoprotein receptor-related protein in human tissues. Cell Tissue Res. 269, 375–82.
- Novagen. (2000). T7Select System Manual. Madison, WI: Novagen.
- Park, J.H., Kim, K.L., Cho, E.W. (2006). Detection of surface asialoglycoprotein receptor expression in hepatic and extra-hepatic cells using a novel monoclonal antibody. Biotechnol Lett. 28, 1061–9.
- Rensen, P.C., Sliedregt, L.A., Ferns, M., Kieviet, E., van Rossenberg, S.M., van Leeuwen, S.H., van Berkel T.J., Biessen, E.A. (2001). Determination of the upper size limit for uptake and processing of ligands by the asialoglycoprotein receptor on hepatocytes in vitro and in vivo. J Biol Chem. 276, 37577–84.
- Rohlmann, A., Gotthardt, M., Willnow, T.E., Hammer, R.E., Herz, J. (1996). Sustained somatic gene inactivation by viral transfer of Cre recombinase. Nat Biotechnol. 14, 1562–5.
- Rohlmann, A., Gotthardt, M., Hammer, R.E., Herz, J. (1998). Inducible inactivation of hepatic LRP gene by cre-mediated recombination confirms role of LRP in clearance of chylomicron remnants. J Clin Invest. 101, 689–95.
- Russell, R.G., Xia, Z., Dunford, J.E., Oppermann, U., Kwaasi, A., Hulley, P.A., Kavanagh, K.L., Triffitt, J.T., Lundy, M.W., Phipps, R.J., Barnett, B.L., Coxon, F.P., Rogers, M.J., Watts, N.B., Ebetino, F.H. (2007). Bisphosphonates: an update on mechanisms of action and how these relate to clinical efficacy. Ann NY Acad Sci. 1117, 209–57.
- Shayakhmetov, D.M., Gaggar, A., Ni, S., Li, Z.Y., Lieber, A. (2005). Adenovirus binding to blood factors results in liver cell infection and hepatotoxicity. J Virol 79, 7478–91.
- Sliedregt, L.A., Rensen, P.C., Rump, E.T., van Santbrink, P.J., Bijsterbosch, M.K., Valentijn, A.R., van der Marel, G.A., van Boom, J.H., van Berkel, T.J., Biessen, E.A. (1999). Design and synthesis of novel amphiphilic dendritic galactosides for selective targeting of liposomes to the hepatic asialoglycoprotein receptor. J Med Chem. 42, 609–18.
- Sokoloff, A.V., Bock, I., Zhang, G., Sebestyen, M.G., Wolff, J.A. (2000). The interactions of peptides with the innate immune system studied with use of T7 phage peptide display [comment]. Mol Ther. 2, 131–9.
- Sokoloff, A.V., Wong, S.C., Ludtke, J.J., Sebestyen, M.G., Subbotin, V.M., Zhang, G., Budker, T., Bachhuber, M., Sumita, Y., Wolff, J.A. (2003). A new peptide ligand that targets particles and heterologous proteins to hepatocytes in vivo. Mol Ther. 8, 867–72.
- Springer, T.A. (1998). An extracellular beta-propeller module predicted in lipoprotein and scavenger receptors, tyrosine kinases, epidermal growth factor precursor, and extracellular matrix components. J Mol Biol. 283, 837–62.
- Waddington, S.N., McVey, J.H., Bhella, D., Parker, A.L., Barker, K., Atoda, H., Pink, R., Buckley, S.M., Greig, J.A., Denby, L., Custers, J., Morita, T., Francischetti, I.M., Monteiro, R.Q., Barouch, D.H., van Rooijen, N., Napoli, C., Havenga, M.J., Nicklin, S.A., Baker, A.H. (2008). Adenovirus serotype 5 hexon mediates liver gene transfer. Cell. 132, 397–409.
- Willnow, T.E., Herz, J. (1994). Genetic deficiency in low density lipoprotein receptor-related protein confers cellular resistance to Pseudomonas exotoxin A. Evidence that this protein is required for uptake and degradation of multiple ligands. J Cell Sci. 107, 719–26.
- Wong, S.C., Wakefield, D., Klein, J., Monahan, S.D., Rosema, D.B., Lewis, D.L., Higgs, L., Ludtke, J.J., Sokoloff, A.V., Wolff, J.A. (2006). Hepatocyte targeting of nucleic acid complexes and liposomes by a T7 phage p17 peptide. Mol Pharm. 3, 386–97.