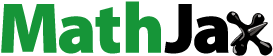
Abstract
It was found that propranolol hydrochloride (PNL), which is a beta-blocker used for hypertension treatment, has a potent spermicidal activity through local anesthetic activity or beta-blocking effect on sperm cells subsequently it could be used as a contraceptive remedy. This study aimed to entrap PNL into invasomes (INVs) and then formulate it as a locally acting contraceptive gel. PNL-loaded mucoadhesive INVs were prepared via the thin-film hydration technique. The D-optimal design was utilized to fabricate INVs employing lipid concentration (X1), terpenes concentration (X2), terpenes type (X3), and chitosan concentration (X4) as independent variables, while their impact was observed for entrapment efficiency percent (Y1; EE%), particle size (Y2; PS), zeta potential (Y3; ZP), and amount of drug released after 6 h (Y4; Q6h). Design Expert® was bestowed to nominate the desired formula. The selected INV was subjected to further studies and formulated into a mucoadhesive gel for ex-vivo and in-vivo investigations. The optimum INV showed a spherical shape with EE% of 65.01 ± 1.24%, PS of 243.75 ± 8.13 nm, PDI of 0.203 ± 0.01, ZP of 49.80 ± 0.42 mV, and Q6h of 53.16 ± 0.73%. Differential scanning calorimetry study asserted the capability of INVs to entrap PNL. Permeation studies confirmed the desired sustained effect of PNL-loaded INVs-gel compared to PNL-gel, INVs, and PNL solution. Sperm motility assay proved the potency of INVs-gel to inhibit sperm motility. Besides, the histopathological investigation verified the tolerability of the prepared INVs-gel. Taken together, the gained data justified the efficacy of PNL-loaded INVs-gel as a potential locally acting contraceptive.
Introduction
Contraception is considered an important part of unremitting efforts to decrease pregnancy. Several effective contraceptive approaches are available, such as oral contraceptives, abstinence, barrier techniques, injections, and implants Various research outcomes have displayed a diversity of oral contraceptives with moderate measures of hormones subsequently decreasing their undesirable effects (Greydanus et al., Citation2001). Spermicides are regarded as non-hormonal locally acting contraceptives. When they are present in vaginal tissues throughout intercourse, they immobilize or kill sperm without causing any systemic actions (Iyer & Poddar, Citation2008). The merits of spermicides include ease of administration, safety, and acceptability. Furthermore, they are suitable for short time utilization or as adjuvants with other methods. There are four classes of spermicidal agents: surface-active agents, enzyme inhibitors, membrane stabilizing agents, and sulfhydryl binding agents (Jalalvandi et al., Citation2021).
Nonoxynol-9 is a common surface-active agent spermicide. It is commercially available as vaginal pessaries, acts by destroying the lipids in the sperm, and causes excessive loss of sperm motion. On the other side, it causes ulcers to genital tissues and inflammation, especially if utilized daily. As a result, spermicides without detergent-type membrane toxicity might provide-significant merit over detergent-type spermicides (Tasdighi et al., Citation2012). Membrane-stabilizing drugs such as quinine, propranolol hydrochloride (PNL), chlorpromazine, and phenoxybenzamine were reported to restrain the motility of spermatozoa in-vitro (Moudgil et al., Citation2002). Hong et al. (Citation1981) studies and clinical studies by Zipper et al. (Citation1983), supported that PNL could be used as a spermicide when administered vaginally.
PNL is known as a beta-blocker that is used mainly for hypertension, angina, myocardial infarction, arrhythmias, and sinus tachycardia (Abruzzo et al., Citation2012). Several mechanisms have been suggested for the justification of PNL spermicidal action. As previously mentioned, PNL has localized anesthetic or membrane-stabilizing action with long duration and short latency. The potency of locally acting anesthetics is based on their lipid solubility, where the sperm plasma membrane consists of lipoprotein, and thus the immobilizing action on the sperm might occur (Srivastava & Coutinho, 2010). PNL is composed of a hydrophilic molecule with a hydrophobic aliphatic chain that might provide amphiphilic character to PNL (Ubrich et al., Citation2004). We speculate that PNL amphiphilic nature might aid in PNL adsorption onto sperm cells. On the other hand, both alpha and beta-adrenergic receptors have been located on human sperm cells. It has been reported that drugs able to block adrenergic receptors might affect the sperm’s ability to move (Adeoya-Osiguwa et al., Citation2006). Additionally, PNL might cause vesicle formation in sperm cells that might significantly damage their integrity (Nicotra & Senatori, Citation1992).
It is worth mentioning that PNL has been fabricated as spermicidal vaginal gel and vaginal film (Tasdighi et al., Citation2012; Borumand et al., Citation2014). According to our understanding, no paper has been published yet regarding loading PNL in nano-system that might sustain PNL spermicidal activity. In the present study invasomes (INVs) (terpenes enriched vesicles) have been utilized as carriers for PNL. INVs are elastic nano-vesicular systems containing phospholipids, terpenes, and/or ethanol (Qadri et al., Citation2017; Tawfik et al., Citation2020). Terpenes were previously proved synergetic effects as antifungal and anti-inflammatory with several drugs loaded into INVs (Albash et al., Citation2021a; Citation2021b). Moreover, it was previously reported that the addition of terpenes in extended semen had an immediate spermicidal effect (Cavalleri et al., Citation2018).
The human vagina appears as an S-shaped fibromuscular collapsible tube between 6 and 10 cm long. The vaginal wall consists of three layers: the epithelial layer, the muscular coat, and the tunica adventitia. The vaginal route can be used for both local and systemic administration (Daoud et al., Citation2017). In a topical technique of contraception, the bio-actives are transported through the vaginal wall into fluids and mucus present in the vagina resulting in the maintenance of a concentration sufficient to immobilize or kill sperms (Iyer & Poddar, Citation2008). Vaginal products must be designed for women’s convenience and must have the following criteria: no side effects during intercourse; colorless and odorless; might be topically applied before coitus; no leakage, no irritation, burning, or swelling; and suitable to be inserted easily (Vermani & Garg, Citation2000).
The vaginal route seems to be suitable for mucoadhesive drug delivery systems for managing mainly local conditions, or for utilization in contraception (Das Neves & Bahia, Citation2006). The major merit of mucoadhesive systems is the ability to augment the residence time, thus decreasing the frequency of applications. The most utilized mucoadhesive vaginal drug delivery systems are semi-solid gels. Gels are polymeric matrices consisting of little quantities of solids, scattered in great quantities of liquid, yet having a more solid-like character. Among vaginal dosage forms, gels are characterized by their easy manufacture and the capability to extend and adhere to vaginal walls (Woodley, Citation2001). Furthermore, due to their high-water composition, they provide the additional advantage of a hydrating and lubricating effect. The strength of lubrication supplied by a product will crucially determine its acceptance and usage (Das Neves & Bahia, Citation2006). A bioadhesive vaginal dosage form could not be importantly composed of a medicinal substance but may be utilized as a moisturizer for dry vagina syndrome (Ahuja et al., Citation1997). Either polycarbophil or Carbopol has been utilized as the bioadhesive polymer in several vaginal dosage forms. It is essential to assess the bioadhesion aspects of polymers and formulations in a simulated vaginal environment to choose the suitable polymers in terms of bioadhesion to vaginal walls.
The success of PNL as a vaginal spermicide has already been described in the literature. Hence, in this research trials were designed to develop PNL-loaded mucoadhesive INVs gel that not only has lubricating action but also prolongs adhesion to the vaginal tissues for an expanded time, that might maintain longer contraceptive action.
Materials
Propranolol hydrochloride (PNL) was supplied by kahira Pharmaceutical Co. (Cairo, Egypt). Phosphatidylcholine from soya bean (PC), chitosan (Mw 260,000 Da), and mucin were obtained from Sigma Aldrich Chemical Co. (St. Louis, MO, USA). Cineole and limonene were bought from Alfa Aesar (Germany). Methanol and chloroform were gained from El-Nasr Pharmaceutical Co. (Cairo, Egypt). Carbopol 934 P was acquired from Goodrich Chemical Co. (USA).
Methods
Preparation of PNL-INVs
INVs were prepared by using two types of terpenes (cineole, and limonene) at various concentrations through the thin-film hydration method () (El-Nabarawi et al., Citation2018). Firstly, terpenes and PC were weighted in a round bottom flask and dissolved in 10 mL methanol: chloroform (2:1) v/v. By keeping pressure under vacuum for 30 min, the organic phase was evaporated at 40 °C utilizing a rotatory evaporator (Heidolph VV 2000, Germany) at 150 rpm hence a thin film of INVs was formed. The film was hydrated using 10 mL phosphate buffer pH4.6 containing (50 mg PNL) at 40 °C. To maintain complete hydration of invasomal film, glass beads were used for 45 min. The INVs were stored at 4 °C for maturation. To improve the mucoadhesive properties of INVs chitosan-coated INVs were prepared. Briefly, chitosan (0.3 and 0.6%) w/v was dissolved into 1% glacial acetic acid and 10 ml water then added to equivalent volume from the previously prepared INVs then stirred for 15 min.
Table 1. D-optimal design for optimization of PNL loaded INVs.
Characterization of PNL-INVs
Determination of entrapment efficiency percentage (EE%)
One ml from the prepared INVs was centrifuged at 20,000 rpm for 1 h at 4 °C employing a cooling-centrifuge (Sigma 3 K 30, Germany). The clear supernatant was subsequently diluted and PNL concentration was determined at λmax 289 nm (Kraisit et al., Citation2018), using a UV-VIS spectrophotometer (Shimadzu UV1650, Japan). EE% was calculated by employing the sequent equation (Mosallam et al., Citation2021):
(Eq. 1)
(Eq. 1)
All evaluations were completed in triplicate ± SD.
Determination of particle size (PS), polydispersity index (PDI), and zeta potential (ZP)
The PS and PDI of INVs were measured for the fabricated INVs employing a Malvern Zetasizer 2000 (Malvern Instruments Ltd., Malvern, UK). The ZP assessment was done by observing the electrophoretic motion of the particles. The evaluations were performed after dilution (Imam et al., Citation2017; Hassan et al., Citation2018). All measurements were performed in triplicate ± SD.
Determination of amount of drug released after 6 hrs (Q6h)
The in-vitro release was performed employing a locally fabricated Franz’s diffusion cell with an area of 0.785 cm2. The cellulose membrane was subjected in the middle between the donor and receptor compartments. 1 mL of INVs dispersions (5 mg PNL) was placed in the donor cells. The receptor compartment was filled with 50 mL of phosphate buffer (pH 4.6) at 37 ± 1 °C. At a predetermined time, 1 mL of permeation media was withdrawn, and an equal volume of fresh media was added into the receiver compartment (Borumand et al., Citation2014). Samples were removed at 1, 2, 3, 4, 5 and 6 hrs then tested through a UV spectrophotometer at λmax 289 nm. Trials were completed in triplicates as mean ± SD.
D-optimal experimental design
A D-optimal experiment was performed to estimate the effect of several factors during INVs formation adopting Design expert® (Stat Ease, Inc., Minneapolis, USA). The design demanded making 20 trials. Four factors were inspected: lipid concentration (mg) (X1), terpenes concentration (%) (X2), terpenes type (X3), and chitosan concentration (%) (X4) that opted as independent variables, whilst EE% (Y1), PS (Y2), ZP (Y3) and Q6h (Y4) opted as dependent variables ().
Selection of the optimum PNL -INVs
The selection of the optimum INV resulted from the desirability function that allowed the inspection of all responses simultaneously. The selection was decided to obtain a suggestion with sustained Q6h (%), highest ZP, and reasonable PS and EE%. The solution with the highest desirability was selected ().
Transmission electron microscopy (TEM)
The shape of the optimum INV was examined by employing TEM (Joel JEM 1230, Japan). The vesicular dispersion of INVs was placed as a thin film on a grid, stained by phosphotungstic acid 1.5% then inspected and photographed (Abdellatif et al., Citation2017).
Differential scanning calorimetry (DSC)
The thermal assessment of PNL, PC, and the lyophilized optimum INV was carried out by DSC (Shimadzu Corp., Japan) standardized with indium. Five mg of each specimen was placed in a standard aluminum pan and heated in a temperature range of 10-250 °C at a scanning rate of 5 °C/min under nitrogen flow (Abd-Elsalam et al., Citation2018).
Stability study
The stability of the optimum INV was monitored to estimate vesicles’ growth, drug leakage, or any change. The optimum INV was conserved at the refrigerator for 3 months and its stability was inspected by comparing the PS, PDI, EE%, ZP, and Q6h of the conserved INVs with the freshly prepared INVs. Further, the system was visually assessed for any sedimentation (Albash et al., Citation2021c). Statistical significance was performed by Student’s t-test utilizing SPSS® software 22.0.
Evaluation of mucoadhesion aspects of INVs
The mucoadhesion aspects of optimum INV were assessed where 1% w/v of mucin solution was admixed with an equivalent quantity of INVs dispersion through mixing via stirrer. The stirring was maintained for five minutes, and the mixture was subjected to equate overnight. The surface charge of mucin alone and INVs with mucin was assessed by ZP evaluation as previously mentioned (Abdellatif et al., Citation2020).
Preparation of PNL loaded mucoadhesive INVs gel
To enhance the vaginal adhesion of PNL, the optimum INV was loaded into a gel matrix. In brief, 0.5% (w/v) Carbopol 934 P was dispersed in 10 ml water by mixing at 500 rpm employing a magnetic stirrer for 1 h to produce a Carbopol 943 P solution. Then, PNL-loaded INVs equivalent to 50 mg PNL was mixed with the equivalent quantity of Carbopol 934 P solution. Finally, triethanolamine was added to augment the pH of the Carbopol 934 P mixture, and the formation of gel took place (Jana et al., Citation2014). A control gel containing the same amount of PNL was fabricated as previously described for comparative assessment.
Gel characterization
Visual inspection
The appearance, homogeneity, and precipitation of freshly fabricated gels (PNL-gel, and INVs loaded gel) were inspected by visual examination under black and white surface (Abdelmonem et al., Citation2018).
Determination of pH
The pH of PNL- gel and the optimum INVs loaded gel was evaluated, in triplicate, using a calibrated pH meter (Hanna, type 211, Romania). The average reading was recorded (Abdellatif et al., Citation2021).
Viscosity
The viscosity of fabricated gels (PNL-gel, and INVs loaded gel) was evaluated with no dilution employing Brookfield DV III ultra V6.0 RV cone and plate rheometer (Brookfield Engineering Laboratories, Inc., Middleboro, MA) using spindle # CPE40 at 25 ± 0.5 °C. All the experiments were made in three times. The spindle was maintained at 30 r.p.m and 60 s − 1 as a shear rate (Abdellatif et al., Citation2021).
Ex-vivo permeation studies
Permeation experiments were implemented by Franz’s diffusion cell with a diffusion area of 0.785 cm2. Rat vaginal tissue was mounted between the donor and receptor compartments (Albash et al., Citation2020). 1 mL of PNL solution, INVs, PNL-gel, and INVs loaded gel equivalent to 5 mg was placed in the donor compartment. The receptor compartment was filled with 50 mL of phosphate buffer (pH 4.6) at 37 ± 1 °C. At a predetermined time, 1 mL of permeation media was withdrawn, and an equal volume of fresh media was added to the receiver cell (Abdellatif et al., Citation2020). The samples were evaluated utilizing a validated HPLC method. Statistical significance was inspected by one-way ANOVA employing SPSS® software. Post hoc analysis proceeded via Tukey’s honestly significant difference (HSD) test.
In-vitro spermicidal activity assessment
Sperm motility assay
10 μl of the optimum INV loaded into gel-plus spermatozoa were added to the slide, and the sperm motility was evaluated by light microscope using the computer-assisted sperm analysis (CASA) method (Wakimoto et al., Citation2018). The percent of sperm motility was determined by monitoring, and recording progressive, non-progressive, and immotile sperm, and the results were the average of six determinations (Ghafarizadeh et al., Citation2021).
In-vivo studies
Animals
Eighteen female Sprague Dawley rats with an average weight of (150-200 gm) were housed at 25 ± 2 °C, with the 12:12 hours light-dark cycle. Rats were furnished with food and water ad libitum. Animal procedures were accepted by the Research Ethics Committee at the Faculty of Pharmacy, Cairo University, Egypt in compliance with the national regulatory standards set via the animal care committee at Cairo University (PI2816).
Histopathologic evaluation
Animals were categorized into three groups 6 rats per group. Group one behaved as a negative control, group two received PNL gel, and group three received INVs-loaded gel, respectively. The treatments were supplied per group once daily. Each group was subdivided into two groups of three animals in which the first group was subjected to a short-term application while the other received a long-term application of treatments. After mercy killing, vaginal tissues were dissected out and preserved in 10% formalin. After fixing, samples were handled through the passage in alcohol and xylene and then added into paraffin. Sections were divided, stained, then visualized by a light microscope (Abdellatif et al., Citation2020).
Results and discussion
Optimization of INVs using D-optimal design
Several trials were performed to evaluate the impact of the independent variables. Response surface methodology is based on the experimental design that aims to determine the best variables for a specific target of the response, utilizing minimal experiments (Aboelazayem et al., Citation2018). The prepared INVs were optimized via applying D-optimal design using Design-expert® which produced 20 experimental trials. The model selected was quadratic for EE%, and Q6h (%) while it was linear and 2FI for PS and ZP, respectively. For adequate- precision a ratio greater than 4 is preferred which was observed for all dependent variables as illustrated in . The predicted R2 values were in correspondence with the adjusted R2 in all dependent variables ().
Table 2. Output data of the D-optimal analysis of INVs formulations and predicted and observed values for the selected INV (INV14).
Effect of formulation variables on the EE%
Entrapment of drugs via PC containing formulae provides the required delivery, good stability, protection, and permeability based on lipid constituents and aspects. EE% of PNL-loaded INVs ranged from 52.05 ± 1.56 to 85.17 ± 0.19%. The reasonable EE% of PNL inside INVs might be related to PC are ampholytic surfactants capable to augment the lipo-solubility of drugs, hence enhancing PNL EE% inside INVs (Abd-Elsalam et al., Citation2018). The effect of the independent variables, lipid concentration (X1), terpenes concentration (X2), terpenes type (X3), and chitosan concentration (X4) on the EE% of PNL in INVs illustrated in and is graphically explained as three-dimensional (3-D) surface plots in . Lipid concentration (X1) had a significant effect on EE% (p = 0.0053). It was obvious that EE% increased by augmenting the concentration of lipid from 100 to 200 mg our results complied with Singh et al. (Citation2005) who stated that EE% declined as lipid amount diminished which might be related to the reduction in the space available for PNL loading inside INVs. Moreover, PC may form shell-like structures embracing the drug this might enhance the stability of the formulated INVs and inhibit the leakage of the drug to the external media (Radwan et al., Citation2020). Terpenes concentration (X2) had a significant impact on EE% (p = 0.0021) as EE% increased by augmenting the concentration of terpenes from 0.5 to 1.5%. It was previously reported that the addition of terpenes to PC bilayers induces structural changes increasing lamellarity. As the lamellarity of INVs increases, it provides more area for drug entrapment inside INVs (Subongkot et al., Citation2012). Considering terpenes type (X3) (p = 0.4589) the factor was not significant in the response. On the other hand, chitosan concentration (X4) (p < 0.0001) had a significant negative impact on EE% of PNL. This could be related to the surface charge of both PNL and chitosan. PNL is positively charged bioactive (Abruzzo et al., Citation2012). Additionally, chitosan bears a positive charge, it was expected that both PNL and chitosan might compete for PC, based on their affinity, EE% of PNL decreased. The previous findings were observed when leuprolide (positively charged) was incorporated inside chitosan-coated liposomes (Guo et al., Citation2003).
Figure 1. Effect of formulation variables on EE% of PNL-INVs (a-b) and PS (c-d).
EE%: Entrapment Efficiency Percentage, PS: Particle Size, PNL: Propranolol Hydrochloride, INVs: Invasomes.
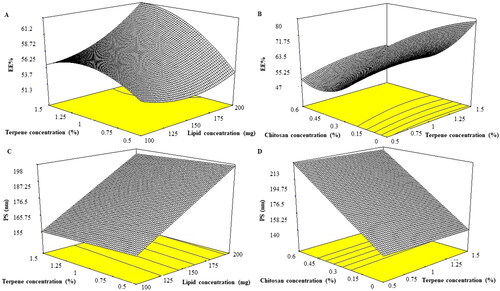
Table 3. Experimental runs, independent variables, and measured response of the D-optimal design of PNL loaded INVs.
Effect of formulation variables on PS
PS of the prepared INVs ranged from 60.20 ± 0.84 to 263.20 ± 0.84 nm. The impact of lipid concentration (X1), terpenes concentration (X2), terpenes type (X3) and chitosan concentration (X4) on the PS of PNL loaded INVs is shown in and is graphically explained as three-dimensional (3-D) surface plots in . Lipid concentration (X1) affected PS of PNL loaded INVs significantly (p = 0.0028). It was reported by augmenting PC concentration the PS increased this might be related to PC tending to form thick bilayers around INVs that subsequently increased the PS of the formed INVs (Shreya et al., Citation2016). For both terpenes concentration (X2) and terpenes type (X3), the factors were not significant in the response with p values of 0.6591 and 0.1914, respectively. Concerning chitosan concentration (X4) (p = 0.0006) by increasing chitosan concentration there was a linear increase in the PS of the formed INVs. It was reported that by augmenting the concentration of chitosan, the dispersion viscosity might increase and result in larger vesicle formation (Patil & Sawant, Citation2011).
Evaluation of PDI
A zero value of PDI manifests as a monodisperse nanosystem. Conversely, a PDI value of 1 resembles polydisperse vesicles (Al-Mahallawi et al., Citation2015). PDI values ranged from 0.136 ± 0.01 to 0.607 ± 0.03 () this viewed that the fabricated INVs were polydisperse but within the acceptable range (Stetefeld et al., Citation2016).
Effect of formulation variables on ZP
Vesicle agglomeration might not happen for nano-system that have a ZP of |30|mV or more due to electrostatic repulsion (Albash et al., Citation2019). ANOVA results demonstrated that chitosan concentration (X4), displayed a significant impact on ZP (P˂0.0001) as depicted in and graphically explained as response 3-D plots in . ZP values ranged from −22.45 ± 1.06 to +53.45 ± 1.06 mV. As observed, ZP values fluctuated between negative and positive values. The negative values of uncoated INVs could be explained due to the presence of PC as it was reported that the head of PC is directed in a manner that the phosphatidyl group is outside but the choline group is inside when they are present in a low ionic strength medium that might impart a negative surface charge (Makino et al., Citation1991). It is worth mentioning that Tawfik et al. (Citation2020) prepared Agomelatine-loaded INVs by using limonene and cineole as terpenes and their ZP ranged from −33.3 ± 2.8 to −73 ± 3.6 supporting our finding for negative surface charge subsequently good colloidal stability of INVs. On the other side, the addition of chitosan resulted in positive ZP values INVs. The positive charge of chitosan is due to the protonation of the amine group available on the surface of chitosan that forms the coating surrounding the vesicles (Shukla et al., Citation2020). Further, positive ZP increased as the chitosan concentration increased our findings were following Bashiri et al.,(Citation2020). as they found that positive ZP values increased by increasing chitosan concentration from 0.1 to 0.15% upon preparing nanostructured lipid carriers coated with chitosan due to an increment in the number of amine groups that reside on the surface of particles.
Effect of formulation variables on Q6h
The quantity of PNL emitted after 6 hrs ranged from 39.74 ± 1.37 to 73.16 ± 2.23% (). The effect of lipid concentration (X1), terpenes concentration (X2), terpenes type (X3), and chitosan concentration (X4) is graphically explained in 3-D surface plots in . Lipid concentration (X1) displayed no significant influence on Q6h (p = 0.0519). Terpenes concentration (X2) (p = 0.0008) showed that by augmenting terpenes concentration from 0.5 to 1.5% the amount of PNL released decreased. For terpenes type (X3) (p = 0.0042), limonene resulted in a higher amount of PNL released compared to cineole. The higher release profiles of INVs containing limonene might be ascribed to its low boiling point. Limonene has a lower boiling point (176 °C), followed by cineole (177 °C). The lower the boiling points of the terpene, the weaker the coherence of the molecules or self-association (El-Nabarawi et al., Citation2018). Regarding chitosan concentration (X4) (p < 0.0001), as observed increasing chitosan concentration resulted in a high amount of PNL released from INVs. The previous phenomenon was observed previously upon preparation of Indomethacin-loaded chitosan microspheres. As the authors reported upon increasing chitosan the release of Indomethacin was enhanced due to chitosan swelling that resulted in a lower crosslinking degree of chitosan and subsequently aided in high PNL release (Orienti et al, Citation1996). In addition, chitosan possesses a hydrophilic character that might aid in enhancing PNL releases upon increasing its concentration to INVs (Meng et al., Citation2011).
Determination of the selected INV
INVs were optimized by the constraints in with the relegation of the non-significant response (PDI). The best values of the variables were gained by numerical optimization relied on the desirability function employing the Design-Expert® software. A suggested optimum formula (INV14) containing 200 mg PC, 1.5% cineole, and chitosan at 0.6%. The predicted and observed values of INVs (INV14) are depicted in . The high resemblance between the observed and predicted responses of INV might validate the design to anticipate the responses.
Transmission electron microscopy (TEM)
proved that the optimum INV was a spherical invasome with narrow size distribution and no INVs with irregular morphology were shown. INVs PS determined by Zetasizer was in an accordance with that visualized employing TEM. This also represented that the coating process did not produce agglomeration or aggregation of INVs (Dung et al., Citation2009).
Differential scanning calorimetry (DSC)
PNL, PC, and optimum INV DSC thermograms are illustrated in . PNL thermogram viewed an endothermic peak at 166.91 °C, proving the crystalline nature of PNL (Chaturvedi et al., Citation2010). In addition, PC showed two endothermal peaks. The first peak (159.9 °C) was described as mild, showing hot movements of polarity parts of PC. The second peak manifested at 234.6 °C, that might be attributed to the transition from gel to liquid crystal state and the melting of the carbon-hydrogen chain in PC, and changes in isomers or the crystal (Ruan et al., Citation2010). However, the lyophilized optimum INV did not have a sharp peak. This might reflect that PNL, when formulated as INV was entrapped inside the INVs in an amorphous state (Elsenosy et al., Citation2020).
Stability study
The visual checking of the stocked INVs did not view any INVs sedimentation through the storage time. Moreover, PS, PDI, ZP, EE%, and Q6h measurements of the stocked optimum INV were 220.00 ± 12.00 nm, 0.347 ± 0.034, 47.87 ± 1.23 mV, 64.00 ± 1.00%, and 56.00 ± 2.00% that illustrated insignificant difference from the freshly prepared INVs (p > 0.05). These consequences might reveal the excellent stability of INVs which might be correlated to the steric effect generated via chitosan coating (Goyal et al., Citation2016).
Evaluation of mucoadhesion aspects of INVs
Mucin surface charge was −9.17 ± 0.02 mV shifted by combing with INVs (INV14). A positive value was gained from the complexes created between mucin and INV14, whereas the negative ZP charge of the mucin might be neutralized by the positive charge of the INV14 adhered to mucin. It was previously found that if the component has a mucoadhesive character it might change the surface aspects of the mucin (Albash et al., Citation2021b). The change from negative mucin ZP to a positive mucin ZP value of 17.86 ± 0.30 mV demonstrated the mucoadhesive property of INV14.
Gel characterization
Visual inspection
All fabricated gels revealed homogenous consistency with no precipitation or aggregation.
Determination of pH
The observed pH value for all formulae ranged 5.2 ± 0.01 to 5.4 ± 0.20, which is considered suitable for vaginal application.
Viscosity
PNL-gel, and INVs-gel formulations showed high viscosity values of 2063.50 ± 211.50 cp, and 3188.50 ± 39.50 cp, respectively. In general, mucoadhesive gel preparations are characterized by high viscosity. Previous studies reported that for obtaining a gel with a desirable viscosity for vaginal route it must be ranged from of 200 to 5000 cp supporting that both gel formulae with good viscosity (Mourtas et al., Citation2010). In addition, we speculate the higher viscosity values of INVs-gel compared to PNL-gel due to the presence of both phospholipids, and terpenes our results were in accordance with the previously reported literature (Ensign et al., Citation2014).
Ex-vivo permeation
The efficacy of drug transport to mucus membranes is based on the retention and distribution of drugs on the mucosal membranes and their infiltration via the mucus membranes (Abdellatif et al., Citation2020). Cervical mucus acts as an excellent site for the retention and distribution of spermicides and, at the same time, as the main barrier to sperm movement (Han et al., Citation2017). From , it is distinct that the quantity of PNL permeated from optimum INV loaded gel was significantly (p < 0.5) the lowest related to PNL-gel and INV14 and PNL solution. The significant lowest permeability from INVs loaded gel could be explained in the light of two reasons. First, it is well known that cationic drugs (as PNL) form complexes with either anionic surfactants or polymers and that the complexes might have an impact on the emission of the bio-active through the matrix. The complex formation is possibly the cause for the observed reduction in the total permeation of the drug from the Carbopol matrix (Perez-Marcos et al., Citation1994). Second, Carbopol gel is known to produce a controlled release system due to the increment in viscosity of the fabricated system (Jaiswal et al., Citation2016). Further, the high-bioadhesive potential of the formulated gel could be ascribed to the aspects of the anionic polymer; carbopol which has several carboxylic moieties that might form H-bonding with the mucus membranes and hence, resulting in excellent bioadhesion and sustained drug effect (Mahmoud et al., Citation2020). The previous reasons justified the significant sustained effect from INVs loaded gel compared to PNL- gel, INVs, and PNL solution. It is worth noting, that the amphoteric property of PNL justified its highest permeation profile compared to other formulae.
In-vitro spermicidal activity assessment
Sperm motility assay
Motility is an essential aspect of sperm and determination of this parameter is critical for sperm evaluation. CASA is a technique devised to take the image from a microscope through visualization of sperm on the video to provide quantitative information on the kinematic behavior of sperm, and subsequently permit objective assessment of sperm kinetics (Wakimoto et al., Citation2018). In this research, it was shown that the motility of sperm was significantly terminated in samples treated with optimum INV-loaded gel after 10 sec of incubation (). In addition, the sperm cell integrity (without flagella) was damaged as presented in . This could be justified as follows; firstly, PNL decreased sperm motion by its membrane stabilizing activity in addition to its ability to block the beta receptors present in sperm cells as previously mentioned. Secondly, the existence of cineole might induce oxidative damage to spermatozoa by reactive oxygen species (Dörsam et al., Citation2015). The findings of this research are consistent with the previous results indicating that the essential oils as cineole (at concentrations of 1% and 0.1%) in semen had immediate spermicidal potential that was evaluated by CASA and demonstrated a significant increase of damaged cells (Cavalleri et al., Citation2018).
Figure 6. Light microscope photomicrographs showing sperm cells A) before addition of PNL-INV gel B) after addition of PNL-INV gel.
PNL: Propranolol Hydrochloride, INV: Invasome.
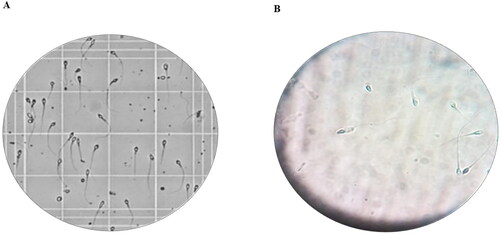
Table 4. Comparison of sperm motility before and after addition of optimum INV loaded gel.
In-vivo studies
Histological assessment of samples from the negative control group as illustrated in () displayed no histopathological alteration with the ordinary histological composition of the stratified mucosal epithelium with the underlying lamina propria. For group II treated with PNL-gel mild edema was observed in the lamina propria and submucosal layers after short-term application. On the other hand, there was no histopathological alternation after long-term application. Further, in group III treated with INVs-gel, there was no histopathological alteration in the vaginal tissues with the absence of inflammatory cells in both short and long-term application groups. The previous findings confirmed the safety of INVs gel as a topically administered spermicide. In addition, this investigation validated the possibility of utilization of INVs gel on daily basis without any inflammation.
Figure 7. Light microscope photomicrographs showing histopathological sections (hematoxylin and eosin stained) of rat vagina normal control (group I), rat vagina treated with PNL gel (group II) and rat vagina treated with INVs gel (group III) with magnification power of 16X to illustrate all vagina layers (Left side) and magnification power of 40X (Right side).
PNL: Propranolol Hydrochloride, INV: Invasome.
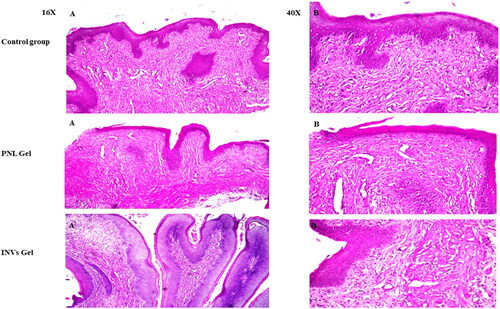
Conclusion
Propranolol hydrochloride (PNL) loaded invasomes (INVs) were created by a thin film hydration approach by D-optimal design, after a screening experiment that illustrated the importance of different variables on INVs aspects. The optimum INV (INV14) contained 200 mg phospholipid, 1.5% cineole and 0.6% chitosan. The optimum INV was stable upon refrigeration for 3 months. Transmission electron microscopy displayed spherical INVs without aggregation. Ex-vivo studies concluded sustained effect, good vaginal deposition, and accumulation of PNL-loaded INV gel, compared to PNL gel, INVs, and PNL solution. Further, sperm motility assay proved the powerful inhibitory effect of INVs-gel. Histopathological studies demonstrated the safety of PNL-loaded INV gel for vaginal application to rats. Hence, it could be concluded that PNL-loaded INV gel could be considered a safe and effective formula that can be used to prolong the deposition of PNL within vaginal layers, to control birth, with minimized systemic side effects.
Disclosure statement
No potential conflict of interest was reported by the authors.
Funding
The author(s) reported there is no funding associated with the work featured in this article.
References
- Abdelmonem R, Nabarawi M, Attia A. (2018). Development of novel bioadhesive granisetron hydrochloride spanlastic gel and insert for brain targeting and study their effects on rats. Drug Deliv 25:70–7.
- Abd-Elsalam WH, El-Helaly SN, Ahmed MA, Al-mahallawi AM. (2018). Preparation of novel phospholipid-based sonocomplexes for improved intestinal permeability of rosuvastatin: in vitro characterization, dynamic simulation, Caco-2 cell line permeation and in vivo assessment studies. Int J Pharm 548:375–84.
- Abdellatif MM, Khalil IA, Elakkad YE, et al. (2020). Formulation and characterization of sertaconazole nitrate mucoadhesive liposomes for vaginal candidiasis. Int J Nanomed 15:4079–90.
- Abdellatif MM, Khalil IA, Khalil MAF. (2017). Sertaconazole nitrate loaded nanovesicular systems for targeting skin fungal infection: in-vitro, ex-vivo and in-vivo evaluation. Int J Pharm 527:1–11.
- Abdellatif MM, Elakkad YE, Elwakeel AA, et al. (2021). Formulation and characterization of propolis and tea tree oil nanoemulsion loaded with clindamycin hydrochloride for wound healing: in-vitro and in-vivo wound healing assessment. Saudi Pharm J 29:1238–49.
- Aboelazayem O, Gadalla M, Saha B. (2018). Biodiesel production from waste cooking oil via supercritical methanol: optimisation and reactor simulation. Renew Energy 124:144–54.
- Abruzzo A, Bigucci F, Cerchiara T, et al. (2012). Mucoadhesive chitosan/gelatin films for buccal delivery of propranolol hydrochloride. Carbohydr Polym 87:581–8.
- Adeoya-Osiguwa SA, Gibbons R, Fraser LR. (2006). Identification of functional α2- and β-adrenergic receptors in mammalian spermatozoa. Hum Reprod 21:1555–63.
- Ahuja A, Khar RK, Ali J. (1997). Mucoadhesive drug delivery systems. Drug Dev Ind Pharm 23:489–515.
- Al-Mahallawi AM, Abdelbary AA, Aburahma MH. (2015). Investigating the potential of employing bilosomes as a novel vesicular carrier for transdermal delivery of tenoxicam. Int J Pharm 485:329–40.
- Albash R, Abdelbary AA, Refai H, El-Nabarawi MA. (2019). Use of transethosomes for enhancing the transdermal delivery of olmesartan medoxomil: in vitro, ex vivo, and in vivo evaluation. Int J Nanomed 14:1953–68.
- Albash R, Elmahboub Y, Baraka K, et al. (2020). Ultra-deformable liposomes containing terpenes (terpesomes) loaded fenticonazole nitrate for treatment of vaginal candidiasis: Box-Behnken design optimization, comparative ex vivo and in vivo studies. Drug Deliv 27:1514–23.
- Albash R, Al-Mahallawi AM, Hassan M, Alaa-Eldin AA. (2021a). Development and optimization of terpene-enriched vesicles (terpesomes) for effective ocular delivery of fenticonazole nitrate: in vitro characterization and in vivo assessment. Int J Nanomed 16:609–21.
- Albash R, Abdellatif MM, Hassan M, Badawi NM. (2021b). Tailoring terpesomes and leciplex for the effective ocular conveyance of moxifloxacin hydrochloride (Comparative assessment): in-vitro, ex-vivo, and in-vivo evaluation. Int J Nanomed 16:5247–63.
- Albash R, Yousry C, Al-Mahallawi AM, Alaa-Eldin AA. (2021c). Utilization of PEGylated cerosomes for effective topical delivery of fenticonazole nitrate: in-vitro characterization, statistical optimization, and in-vivo assessment. Drug Deliv 28:1–9.
- Bashiri S, Ghanbarzadeh B, Ayaseh A, et al. (2020). Preparation and characterization of chitosan-coated nanostructured lipid carriers (CH-NLC) containing cinnamon essential oil for enriching milk and anti-oxidant activity. Lwt 119:108836.
- Borumand M, Mortazavi SA, Jafari Azar Z, Teymouri Rad R. (2014). Development and in vitro evaluation of a novel contraceptive vaginal mucoadhesive propranolol hydrochloride film. J Drug Deliv Sci Technol 24:637–44.
- Cavalleri R, Becker JS, Pavan AM, et al. (2018). Essential oils rich in monoterpenes are unsuitable as additives to boar semen extender. Andrologia 50:e13074–10.
- Chaturvedi K, Umadevi S, Vaghani S. (2010). Floating matrix dosage form for propranolol hydrochloride based on gas formation technique: development and in vitro evaluation. Sci Pharm 78:927–39.
- Das Neves J, Bahia MF. (2006). Gels as vaginal drug delivery systems. Int J Pharm 318:1–14.
- Daoud WM, Rajab MA, Rajab NA. (2017). Propranolol HCL vaginal contraceptive hollow-type suppositories. World J Pharm Res 28:6(13):86–99.
- Dörsam B, Wu CF, Efferth T, et al. (2015). The eucalyptus oil ingredient 1,8-cineol induces oxidative DNA damage. Arch Toxicol 89:797–805.
- Dung DTK, Hai TH, Phuc LH, et al. (2009). Preparation and characterization of magnetic nanoparticles with chitosan coating. J Phys: Conf Ser 187:012036–11.
- El-Nabarawi MA, Shamma RN, Farouk F, Nasralla SM. (2018). Dapsone-loaded invasomes as a potential treatment of acne: preparation, characterization, and in vivo skin deposition assay. AAPS PharmSciTech 19:2174–84.
- Ensign LM, Cone R, Hanes J. (2014). Nanoparticle-based drug delivery to the vagina: a review. J Control Release 190:500–14.
- Elsenosy FM, Abdelbary GA, Elshafeey AH, et al. (2020). Brain targeting of duloxetine hcl via intranasal delivery of loaded cubosomal gel: in vitro characterization, ex vivo permeation, and in vivo biodistribution studies. Int J Nanomedicine 15:9517–37.
- Ghafarizadeh AA, Malmir M, Naderi Noreini S, et al. (2021). The effect of vitamin E on sperm motility and viability in asthenoteratozoospermic men: in vitro study. Andrologia 53:1–6.
- Goyal N, Rastogi D, Jassal M, Agrawal AK. (2016). Chitosan as a potential stabilizing agent for titania nanoparticle dispersions for preparation of multifunctional cotton fabric. Carbohydr Polym 154:167–75.
- Greydanus DE, Patel DR, Rimsza ME. (2001). Contraception in the adolescent: an update. Pediatrics 107:562–73.
- Guo J, Ping Q, Jiang G, et al. (2003). Chitosan-coated liposomes: characterization and interaction with leuprolide. Int J Pharm 260:167–73. 24
- Han L, Taub R, Jensen JT. (2017). Cervical mucus and contraception: what we know and what we don’t. Contraception 96:310–21.
- Hassan DH, Abdelmonem R, Abdellatif MM. (2018). Formulation and characterization of carvedilol leciplex for glaucoma treatment: in-vitro, ex-vivo and in-vivo study. Pharmaceutics 10:197.
- Hong C, Saintonge CD, Turner P. (1981). The inhibitory action of procaine, (+)-propranolol and (+/-)- propranolol on human sperm motility: antagonism by caffeine. Br J Clin Pharmacol 12:751–3.
- Iyer V, Poddar SS. (2008). Update on nonoxynol-9 as vaginal spermicide. Eur J Contracept Reprod Health Care 13:339–50.
- Imam SS, Ahad A, Aqil M, et al. (2017). Formulation by design based risperidone nano soft lipid vesicle as a new strategy for enhanced transdermal drug delivery: in-vitro characterization, and in-vivo appraisal Syed. Mater Sci Eng C 75:1198–205.
- Jaiswal M, Kumar A, Sharma S. (2016). Nanoemulsions loaded Carbopol® 934 based gel for intranasal delivery of neuroprotective Centella asiatica extract: in–vitro and ex–vivo permeation study. J Pharm Investig 46:79–89.
- Jalalvandi E, Jafari H, Amorim CA, et al. (2021). Vaginal administration of contraceptives. Sci Pharm 89:1–18.
- Jana S, Manna S, Nayak AK, et al. (2014). Carbopol gel containing chitosan-egg albumin nanoparticles for transdermal aceclofenac delivery. Colloids Surf B Biointerfaces 114:36–44.
- Kraisit P, Limmatvapirat S, Luangtana-Anan M, Sriamornsak P. (2018). Buccal administration of mucoadhesive blend films saturated with propranolol loaded nanoparticles. Asian J Pharm Sci 13:34–43.
- Mahmoud RA, Hussein AK, Nasef GA, Mansour HF. (2020). Oxiconazole nitrate solid lipid nanoparticles: formulation, in-vitro characterization and clinical assessment of an analogous loaded carbopol gel. Drug Dev Ind Pharm 46:706–16.
- Makino K, Yamada T, Kimura M, et al. (1991). Temperature- and ionic strength-induced conformational changes in the lipid head group region of liposomes as suggested by zeta potential data. Biophys Chem 41:175–83.
- Meng ZX, Zheng W, Li L, Zheng YF. (2011). Fabrication, characterization and in vitro drug release behavior of electrospun PLGA/chitosan nanofibrous scaffold. Mater Chem Phys 41:175–83.
- Mosallam S, Ragaie MH, Moftah NH, et al. (2021). Use of novasomes as a vesicular carrier for improving the topical delivery of terconazole: in vitro characterization, in vivo assessment and exploratory clinical experimentation. Int J Nanomed 16:119–32. 8
- Moudgil P, Gupta A, Sharma A, et al. (2002). Spermicidal efficacy of some membrane stabilizers. Pharmacol Rev Commun 12:241–8.
- Nicotra A, Senatori O. (1992). B- Adrenergic antagonists and carp (Cypvinus cavpio L.) sperm motility. J Fish Biol 41:667–9.
- Mourtas S, Mao J, Parsy CC, et al. (2010). Liposomal gels for vaginal delivery of the microbicide mc-1220: preparation and in vivo vaginal toxicity and Nano Life 01: 195–205.
- Patil SB, Sawant KK. (2011). Chitosan microspheres as a delivery system for nasal insufflation. Colloids Surf B Biointerfaces 84:384–9.
- Perez-Marcos B, Ford JL, Armstrong DJ, et al. (1994). Release of propranolol hydrochloride from matrix tablets containing hydroxypropylmethylcellulose K4M and carbopol 974. Int J Pharm 111:251–9.
- Qadri GR, Ahad A, Aqil M, et al. (2017). Invasomes of isradipine for enhanced transdermal delivery against hypertension: formulation, characterization, and in vivo pharmacodynamic study. Artif Cells Nanomed Biotechnol 45:139–45.
- Radwan SAA, El-Maadawy WH, Yousry C, et al. (2020). Zein/phospholipid composite nanoparticles for successful delivery of gallic acid into ahscs: influence of size, surface charge, and vitamin a coupling. Int J Nanomed 15:7995–8018.
- Ruan J, Liu J, Zhu D, et al. (2010). Preparation and evaluation of self-nanoemulsified drug delivery systems (SNEDDSs) of matrine based on drug-phospholipid complex technique. Int J Pharm 386:282–90.
- Shreya AB, Managuli RS, Menon J, et al. (2016). Nano-transfersomal formulations for transdermal delivery of asenapine maleate: in vitro and in vivo performance evaluations. J Liposome Res 26:221–32.
- Shukla SK, Chan A, Parvathaneni V, Gupta V. (2020). Metformin-loaded chitosomes for treatment of malignant pleural mesothelioma – a rare thoracic cancer. Int J Biol Macromol 26:221–32.
- Singh B, Mehta G, Kumar R, et al. (2005). Design, development and optimization of nimesulide-loaded liposomal systems for topical application. Curr Drug Deliv 2:143–53.
- Srivastava S, Coutinho E. (2010). Adrenergic antagonist propranolol as a novel, effective spermicide: an NMR study. Int J Pharm Pharm Sci 2:196–200.
- Stetefeld J, McKenna SA, Patel TR. (2016). Dynamic light scattering: a practical guide and applications in biomedical sciences. Biophys Rev 8:409–27.
- Subongkot T, Opanasopit P, Rojanarata T, Ngawhirunpat T. (2012). Effect of limonene and 1,8 cineole on the skin penetration of fluorescein sodium deformable liposomes. AMR 506:449–52.
- Tasdighi E, Azar ZJ, Mortazavi SA. (2012). Development and In-vitro evaluation of a contraceptive vagino-adhesive propranolol hydrochloride gel. Iran J Pharm Res 11:13–26.
- Tawfik MA, Tadros MI, Mohamed MI, El-Helaly SN. (2020). Low-frequency versus high-frequency ultrasound-mediated transdermal delivery of agomelatine-loaded invasomes: development, optimization and in-vivo pharmacokinetic assessment. Int J Nanomed 15:8893–910.
- Ubrich N, Bouillot P, Pellerin C, et al. (2004). Preparation and characterization of propranolol hydrochloride nanoparticles: a comparative study. J Control Release 97:291–300. 18
- Vermani K, Garg S. (2000). The scope and potential of vaginal drug delivery. Pharm Sci Technol Today 13:359–64.
- Wakimoto Y, Fukui A, Kojima T, et al. (2018). Application of computer-aided sperm analysis (CASA) for detecting sperm-immobilizing antibody. Am J Reprod Immunol 79:e12814.
- Woodley J. (2001). Bioadhesion: new possibilities for drug administration? Clin Pharmacokinet 40:77–84.
- Orienti I, Aiedeh K, Gianasi E, et al. (1996). Indomethacin loaded chitosan microspheres. Correlation between the erosion process and release kinetics. J Microencapsul 13:463–72.
- Zipper J, Wheeler RG, Potts DM, Rivera M. (1983). Propranolol as a novel, effective spermicide: preliminary findings. Br Med J (Clin Res Ed) 287:1245–6.