Abstract
In the Australian state of Victoria, the city of Melbourne endured the world’s longest number of lockdown days, with severe government health orders and travel restrictions in place for extended periods of 2020 and 2021. In common with others, we found the provision of field teaching in introductory geology, structural geology, and volcanology, and the online replacement of practical instruction in petrology and petrography to be the greatest pedagogical challenges. We developed and used a range of different virtual field excursions that, given time and travel constraints imposed on us, were necessarily “low-tech” and non-immersive. Despite this, our students largely engaged enthusiastically with the virtual excursions, met many preexisting learning goals, and gained additional skills, particularly in regional-scale geological synthesis. In teaching petrology and petrography online, curated resources improved student understanding of some fundamental concepts, and it was advantageous that students were all assessed using identical imagery, rather than one sample from a non-identical class-set. On the other hand, we found we were less able to train students in the advanced skills of thin section interpretation. Assessment changes associated with online teaching have resulted in a permanent shift from low-level recall-style assessments to instead emphasizing higher-level synthesis and “geological thinking” skills. Our efforts throughout the pandemic demonstrated the value of instructor-student engagement and yielded teaching resources that have subsequently enhanced our face-to-face teaching and increased flexibility for students. Moreover, the COVID-19 pandemic has highlighted the importance of collaborative teaching practice and we have increasingly seen the benefits of local and national-scale teaching networks for peer support and for resource sharing.
Introduction
Globally, government approaches to managing the COVID-19 pandemic began in earnest as case numbers accelerated rapidly in March 2020. In the Australian state of Victoria, both The University of Melbourne and Monash University responded to Federal and Victorian Government Health orders by pausing teaching from March 24, 2020, and establishing fully online “virtual campuses” from March 30, 2020. This meant that students and staff had just three weeks of regular in-person classes in the 2020 undergraduate teaching year before pivoting to “emergency remote delivery of teaching.” It was a dramatic, unprecedented transition with <1 week’s notice for academics to develop and implement new approaches to facilitate teaching and learning online (Martin, Citation2020). In the Victoria capital of Melbourne, remote learning continued throughout most of 2020 and for extended periods of 2021, with prolonged community lockdowns that were accompanied by strict travel restrictions meaning access to field sites and teaching materials was seriously compromised. Melbourne endured the world’s longest and, at many times, most restrictive lockdowns with community stay-at-home orders and travel restrictions in place for a total of 262 days over the period from March 2020 to November 2021, including 150 days on which government health orders limited essential travel to <5 km from home (Vally & Bennett, Citation2021).
With large distances between population centers, as well as large numbers of rural and remote communities, Australia is ideally suited for distance education and online learning (e.g., Latchem, Citation2018). But before the COVID-19 pandemic, remote higher education in Australia remained a highly unusual pathway for students. Although a wide range of courses was offered to students online—in recent decades largely through the Open Universities Australia consortium—online learning accounted for only 1.5% of students in higher education in Australia in 2019 (Australian Department of Education statistics—https://www.education.gov.au/resources/higher-education-statistics). Only the University of New England (UNE)—a pioneer of dual-mode study in Australia (Latchem, Citation2018)—offered a Bachelor of Science in Geoscience via distance education. But even the UNE degree required attendance at intensive residential courses at different stages of the degree program and a fully remote geoscience major was not available. Both the University of Southern Queensland (USQ) and Central Queensland University (CQU) offered a selection of online geoscience subjects that were largely focused on upskilling industry employees rather than providing a geoscience major. Like the degree offered at the UNE, the USQ and CQU subjects similarly required some intensive residential components.
Elsewhere—including at The University of Melbourne and Monash University—almost all Australian academics had no experience preparing or delivering teaching online. Given the unprecedented circumstances and the large number of academics needing support at the same time, most academics, including ourselves, had no guidance from educational designers or support staff. Instead, we were faced with teaching highly practical, field-based subjects using unfamiliar online tools, essentially without support. Moreover, unlike some disciplines, technology-integrated learning was not already particularly well established in Earth sciences teaching, with our subjects relying heavily on practical work using physical rock and mineral samples and microscopes, as well as field teaching, often in remote locations. At the same time as the educational challenge, many staff were also managing caring responsibilities, including elder care, home-schooling, and/or child-care, together with the acute—and later chronic—stresses associated with being isolated from family, friends, and colleagues. The rapid pivot, the timing of the pandemic with respect to the beginning of the teaching year, and the protracted nature and extent of the community lockdown meant Melbourne academics faced one of the hardest educational challenges in the developed world.
Our students were in similarly challenging circumstances. Before the pandemic, around 40% of students at the University of Melbourne and 34% of students at Monash University were international (Australian Department of Education, 2020). In 2020, in the natural and physical sciences at the University of Melbourne, 34% of students were international and at Monash University 18% of students were international (Australian Department of Education, 2020). This percentage can be somewhat lower for students in geoscience classes but nonetheless, significant numbers of our students either: (1) returned to their home countries before widespread border closures, meaning participation in online classes was compromised by unfavorable time zones and/or internet connectivity issues; or (2) remained in Australia, often profoundly isolated from family and broader support networks. Both student cohorts faced equally impactful challenges to learning throughout the pandemic. Our domestic students also faced myriad challenges, including (1) internal state border closures that persisted throughout 2020 and 2021; (2) financial stressors associated with the inability to work to support themselves (particularly in the hospitality and retail sectors); and (3) a range of challenging personal situations including a lack of study space, internet connectivity and/or computing facilities. University staff and students—together with the broader community—experienced complex and evolving mental health effects during the prolonged and repeated community lockdown periods (e.g., Butterworth et al., Citation2022; Czeisler et al., Citation2021) with a large number of people reporting higher levels of stress and an inability to manage challenges (Currie, Citation2020; Dodd et al., Citation2021).
In common with geoscience educators globally, key pedagogical challenges for our remote delivery of teaching were the sub-disciplines of field and structural geology, and petrology and petrography (including both hands-on practicals with rocks and minerals and examination of rocks under the microscope)—both topics that students find challenging to learn and instructors find challenging to teach (e.g., King, Citation2012). In parallel to these pedagogical challenges, we were also acutely aware and concerned about student engagement and staff and student well-being.
In this commentary, we focus on our experiences developing and using virtual excursions and teaching petrography and petrology, presenting a qualitative discussion of the approaches we took, their effectiveness, and the insights they provided. We have focused on these areas as they represented two of our greatest challenges but at the same time also produced the most insights into our teaching practice.
Educational researchers have documented and discussed the profound differences between online teaching, blended-learning and “emergency” remote delivery of teaching, a difference well-known before the COVID-19 pandemic (e.g., Alammary et al., Citation2014; Bernard et al., Citation2004; Crawford-Ferre & Wiest, Citation2012; Simonson et al., Citation2011). As noted by Hodges et al. (Citation2020) any evaluation of emergency response teaching is complex and simple comparisons of previous face-to-face learning with pandemic online teaching cannot be appropriate. Without question, the situation for Melbourne academics was very much “emergency pandemic instruction” that cannot be compared to appropriately resourced online teaching programs developed following evidence-based-research. This distinction was acknowledged by University leadership who, at our institutions, paused formal evaluation of teaching surveys in the 2020–2021 period. For these reasons, we have made no attempt to directly compare student feedback from online teaching to that received in traditional classes in the pre-pandemic period.
Despite the challenges of the pandemic years, key benefits for our students and our teaching practice have been the increased flexibility offered by online resources, increased participation of a diverse range of students in our geoscience subjects, and improved assessment design (e.g., Bennett et al., Citation2017). Although at different institutions in the same city, we largely tackled the challenges independently, only realizing the extent of missed opportunities for collaboration in the post-lockdown period. It is imperative that the geoscience education community effectively capitalize on the collective of creative work done developing online teaching resources during the COVID-19 pandemic. We recommend a focus on enhanced collaborative teaching communities and networks for sharing the range of mature teaching resources now available. These resources, developed globally, have the potential to support student engagement with geoscience more broadly, open our discipline to students with diverse learning and accessibility needs, and improve teaching quality for in-person, online, and hybrid teaching programs.
Virtual field excursions
As noted by Waldron et al. (Citation2016), Earth science is unique among scientific sub-disciplines for its dependence on field data for many aspects of geological problem-solving. In geology, field studies are known to be significant in fostering the development of spatial cognition skills (e.g., Orion et al., Citation1997), spatio-temporal relationships, and geological reasoning (e.g., Dolphin et al., Citation2019; Elkins & Elkins, Citation2007; Hannula, Citation2019). Undergraduate fieldwork is an excellent example of fully immersive (e.g., Mogk & Goodwin, Citation2012; Petcovic et al., Citation2014), “authentic” work-integrated learning (e.g., Viskupic et al., Citation2021). Moreover, in addition to disciplinary skills, fieldwork also provides students with opportunities to gain transferable skills in problem-solving, decision-making, leadership, and teamwork (e.g., Nyarko & Petcovic, Citation2023; Peasland et al., Citation2019). However, despite the documented benefits of field teaching, many students and instructors perceive field studies to be inherently exclusionary (e.g., Guillaume et al., Citation2023).
In Australia, every undergraduate Bachelor of Science geology program includes fieldwork at Levels 2 and 3 (and often also at Level 1) and a field course is a capstone option in all geology majors. Remote delivery of teaching during the Melbourne lockdowns meant that traditional undergraduate field work was not possible in 2020 and for much of 2021. In common with many Earth science educators globally (e.g., Peace et al., Citation2021; Rotzien et al., Citation2021) the provision of a field experience during the pandemic was an important focus for us. Moreover, given that positive experiences on field excursions are often instrumental in students choosing to major in Earth science (e.g., Hoyer & Hastie, Citation2023; LaDue & Pacheco, Citation2013; Manner, Citation1995), overriding aims of our approaches to virtual excursion delivery were student engagement and enjoyment.
Technologies such as gigapan photography, panosphere photography, virtual reality, and/or augmented reality have seen rapid development over the past decade, and exploration of their potential use in geoscience teaching was underway before the COVID-19 pandemic (e.g., Benson, Citation2010; Bursztyn et al., Citation2017; Dolphin et al., Citation2019; Friess et al., Citation2016; Klippel et al., Citation2019; Stainfield et al., Citation2000). However efforts to develop virtual field experiences necessarily increased significantly as the pandemic began, and there is an increasingly rich literature on these approaches (e.g., Alías et al., Citation2023; Fleming, Citation2022; Gregory et al., Citation2022; Horota et al., Citation2023; Rader et al., Citation2021). The merits of virtual excursions have been evaluated by a range of authors, and although a range of advantages and disadvantages have been identified, there is mixed evidence for the effectiveness of virtual field experiences on student learning (e.g., Ruberto et al., Citation2023).
Notably, the severe travel restrictions in force in Melbourne meant that it was not even possible for us to visit field sites to take photos or record videos, let alone the specialized imagery required for digital outcrop models or immersive technologies. This, together with the urgent, immediate need for replacement excursions in 2020 meant that we were only able to develop “low-tech” virtual excursions using preexisting photos and videos, together with publicly available imagery, such as that available on Google Earth Pro. In this way, our “virtual excursions” differed markedly from immersive virtual excursions described by others (e.g., Guillaume et al., Citation2023; Harknett et al., Citation2022; Peace et al., Citation2021; Ruberto et al., Citation2023).
Level 1 introductory geology and physical geography
In Australia, very few primary or secondary schools teach Earth science, and this is particularly the case for schools in Victoria. Consequently, students who take first-year geoscience subjects often have very limited prior knowledge, and many take introductory Earth science without the intention to complete a geoscience major. Both Monash University and the University of Melbourne include field teaching at Level 1, in part to provide a memorable integrated learning experience that encourages students to continue in the discipline. At Monash University, introductory geology and physical geography students (subject code EAE1022) normally attend a two-day residential camp. This camp was replaced by the “Staycation” report designed to connect the three themes of the subject (Earth, Atmosphere, and Environment) and to replicate many of the skills learned on the field trip, but which could be undertaken at any location, including within the confines of a house or apartment for those who were in mandatory isolation. Together the three tasks were designed to develop most of the skills students would have learned on the in-person trip with learning outcomes focused on field observations, note-taking, data collection and analysis, and report writing.
For the Earth theme, students were asked to find, photograph, describe, and name (using the methods learned in the lectures including a first-order igneous, sedimentary, or metamorphic description and classification) “a rock” available to them in their current circumstances. Students were also asked to describe “the journey” of their rock from where it formed to where it was found. The definition of “rock” was purposefully broadened to include minerals (including gems in jewelry), and items derived from or obtained from rocks (e.g., glass or metals), and creativity in the description of the journey was encouraged. The atmosphere theme task required students to keep a weather journal for 7 consecutive days and report their observations in comparison to both online synoptic charts and the expected weather for the time of year at their location. The environment theme task required students to research their local climate zone, soil properties, and typical annual weather data, and then observe and map different plant groups within a 5 km radius of their location. Observations of past local environments using archived satellite image data provided a historical perspective that students synthesized with their own observations and location-specific climate change forecasts to summarize the current and future state of their local built and vegetated environment.
Level 2 and level 3 structural geology
Students at the University of Melbourne attend a two-day field excursion early in Level 2 (as part of the subject GEOL20002) and a week-long field trip at the beginning of Level 3 (as part of the subject GEOL30002). The Level 2 excursion visits localities characterized by spectacular deformation structures in Paleozoic rocks as well as a range of rock types exhibiting different contact relationships. Aided by the availability of a series of narrated short videos fortuitously collected before the pandemic, the virtual excursion in 2020 attempted to replicate almost all of the intended learning outcomes of the in-person excursion. The Level 3 structural geology excursion was historically taught as a week-long regional trip visiting a range of locations in Eastern Victoria, including a two-and-a-half-day detailed mapping exercise at one location. The trip changed several years before the pandemic with regional locations replaced by a longer, more detailed field mapping exercise at the same single location. Given this focus, the virtual excursion did not attempt to replicate the in-person field trip, and components of the original regional excursion were re-introduced and combined with simplified synthetic mapping exercises designed to teach key mapping skills. Learning outcomes were focused on the regional synthesis of complex geology.
The virtual field excursions for both subjects were hosted using the Thinglink platform (www.thinglink.com) which allowed a range of still photographs, videos, and online resources to be linked to a Google Earth base map () to provide the regional context for the work. Students were provided with a detailed workbook that provided background information linked to lecture content, and questions and activities to complete at each virtual site. Activities included (1) considering field health and safety, including mental health on field work; (2) describing different rock types present at each locality, estimating their orientation, and interpreting their environments of deposition; (3) sketching brittle and ductile structural features—including those associated with polydeformation—to interpret regional stress orientation directions during deformation; (4) deducing contact relationships between rock units including sequencing of deformation, metamorphism and the intrusion of melt products; and (5) constructing schematic cross-sections.
Figure 1. Examples of materials available to students taking the Level 2 structural geology (GEOL20002) virtual field excursion at the University of Melbourne showing Thinglink basemap for one outcrop region with localities (A–E) and corresponding questions provided to students in their excursion workbook.
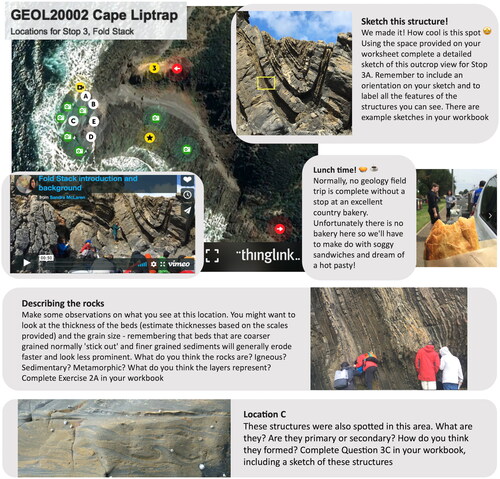
Due to timing constraints in 2020, students at both Level 2 and Level 3 worked independently on their virtual excursions outside scheduled class time. Both excursions were able to run in-person in 2021, but in 2022 the Level 3 structural geology virtual excursion was again used after the in-person excursion was abandoned due to positive COVID-19 cases. But in this case, students worked through the excursion materials together with their instructor during a 5-day intensive-style instruction period with consecutive dual delivery (face-to-face and online options) all-day classes that included directed active-learning on the excursion materials (particularly in small groups) as well as additional lecture material to clarify key concepts.
Level 2 volcanic landforms and igneous processes
This field excursion takes place within a Level 2 subject on igneous petrology and petrography (GEOL20003) at the University of Melbourne. The field excursion explores the Newer Volcanic Province—a globally significant, dormant, intraplate volcanic field—in western Victoria, Australia. Before the COVID-19 pandemic, the two-and-a-half day excursion introduced students to a range of volcanic landforms and lithologies, and key learning outcomes included the practise of recording scientific observations as well as making geological interpretations based on group discussion and problem-solving. Rather than replicate each individual component of the in-person field trip, the virtual excursion aimed to provide problem-solving challenges equivalent to those posed during the in-person trip. Students were provided with (1) a recorded 2-h lecture on volcanological principles; (2) a collection of high-quality images and descriptions related to volcanic phenomena, including, but not limited to, those that they would have observed on the field excursion; (3) a .kmz (Google Earth) file containing a sequence of localities, similar to the localities visited on the in-person excursion; and (4) a selection of field photographs from the localities in the .kmz file.
shows one example of the material provided to students. The example was used to investigate the Harman’s Valley lava flows showing the emphasis on geological problem-solving. During remote teaching, and after completing a series of similar exercises, students met in small groups with the instructor for an online discussion session after which they completed a short summative essay evaluating a variety of hypotheses, both plausible and implausible, that might explain the existence of the enigmatic Newer Volcanic Province as an intraplate volcanic field. The essay question assessed students’ understanding of core principles in igneous petrology, and of the Newer Volcanic Province in the context of its regional tectonic setting.
Figure 2. Examples of materials available to students taking the Level 2 volcanic landforms and igneous processes (GEOL20003) virtual field excursion at the University of Melbourne. (a) Waypoints for localities on the Harman’s Valley lava flows and their source, Mt Napier, in the Newer Volcanics Province of western Victoria. (b) Photograph of Mt Napier and the Harman’s Valley flows taken from Harman’s Valley Lookout waypoint in (a), with scale and direction to be inferred by students. Students were required to sketch the margin of the Harman’s Valley flow series as they interpreted it from Google Earth (the margin is visible, unmarked, in ), to deduce and demonstrate how the younger flows follow a river valley that cuts through older lavas. (c) View of Mt Napier’s scoria cone from Google Earth. (d) Photograph of a tumulus found in the Harman’s Valley lava flows at the waypoint shown in (a). Students characterized the shape of Mt Napier to help deduce its origin and investigated a series of sinkhole-like features in the Harman’s Valley based on their geomorphology to evaluate hypotheses regarding the nature of tumuli in the lava flow.
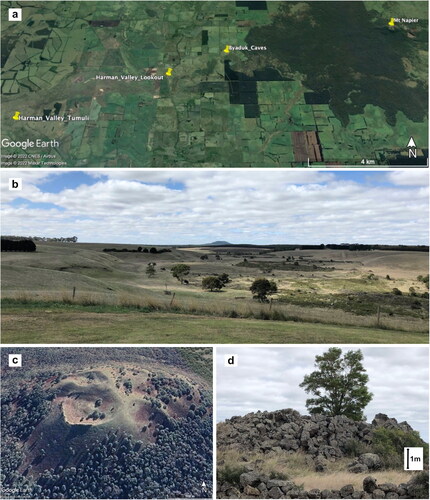
Student perspectives
A voluntary survey of students in GEOL20002 and GEOL30002 who completed the structural geology virtual excursions in 2020 yielded 25 responses from a total enrollment of 39 (). Advantages noted by students included having more time to study the outcrop photos and not feeling time pressures at any outcrop (). Being able to “visit” and then “re-visit” regional locations were highlighted by 21/25 respondents, although this extra opportunity to compare outcrops from across a regional terrane was not reflected in the students’ self-reported ability to put their observations into regional context (; ). Students also noted that not having to purchase field equipment or pay excursion travel costs—known barriers to field trip participation (Giles et al., Citation2020)—were advantages (19/25 respondents; ). In response to the question “do you feel confident to undertake in-person fieldwork following the virtual excursion,” 9/12 Level 2 students agreed (). Interestingly, only 7/25 student respondents would have liked immersive imagery (such as gigapans or virtual outcrop models) added to the virtual excursion.
Figure 3. Pooled results of an informal student survey of Level 2 and Level 3 structural geology students who took the GEOL20002 and GEOL30002 virtual excursions in 2020. Note that as they had not previously had in-person field experience, only Level 2 students were asked about their level of confidence in approaching site fieldwork.
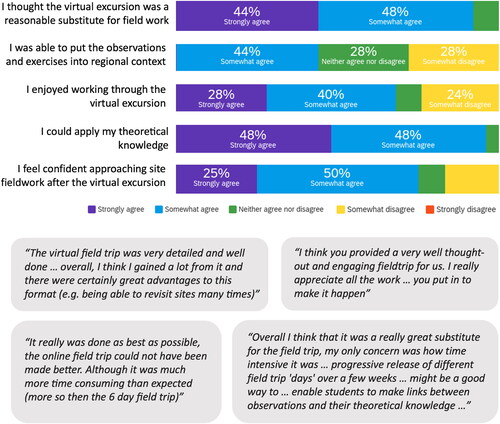
Table 1. Advantages of virtual excursions as reported by level 2 and level 3 structural geology students at the University of Melbourne in 2020.
In assessing the disadvantages of the virtual excursion, we were not surprised that students highlighted not being able to visit outcrops and landscapes and the attendant challenge of interpreting geology from 2D photos (). Students also noted the absence of social connections usually developed on excursions, less staff support, fewer peer-peer learning opportunities, and the impact of working in isolation (; ). Similar disadvantages have been reported in other studies (e.g., Çaliskan, Citation2011; Guillaume et al., Citation2023). Students suggested virtual excursions be released day-by-day to replicate an in-person trip () and when the Level 3 structural geology virtual excursion was again used in 2022, this approach yielded higher levels of engagement from students and more opportunities for the instructor to explicitly address conceptual linkages, misconceptions, and knowledge gaps at appropriate points.
Figure 4. University of Melbourne Level 3 and Level 2 structural geology student responses to the reflective prompt to “draw our class on a virtual excursion,” with most responses highlighting the sense of isolation many students felt working through the assignment.
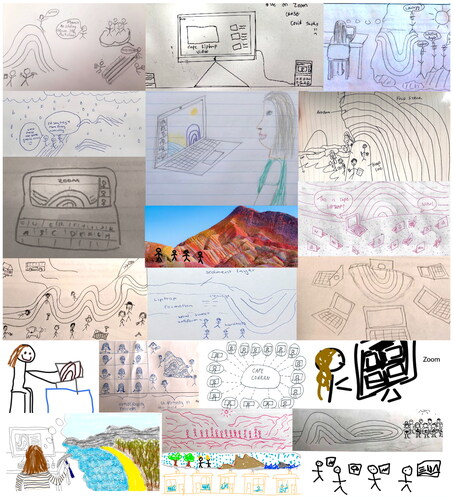
Table 2. Disadvantages of virtual excursions as reported by level 2 and level 3 structural geology students at the University of Melbourne in 2020.
End-of-semester University administered (but voluntary and informal) student surveys yielded feedback on the Level 1 virtual excursion and the Level 2 volcanic landforms virtual excursion (). Although students were appreciative of the efforts of the instructors in building the virtual excursions, and we observed them to have generally engaged thoughtfully with the learning tasks, there was no clear consensus on how the virtual excursions were received (). In comparing feedback across all three levels of our teaching, we noticed that generally students at Level 3 were less positive about the virtual excursion tasks and attribute this to their previous in-person field experiences making them aware of the profound differences in the approach.
Instructor perspectives
Development and the first use of the virtual excursions were extremely challenging, particularly as each of us was developing our new resources independently under intense time pressure. In 2020, the amount of preparation needed meant there was less time for us to work together with students on the assignments, and the inability to purpose-collect imagery or data meant newer approaches were not available to us. Moreover, the amount of additional scaffolding required to support students meant that their workload was onerous and some students, often working alone outside of class times, found the experience dispiriting. We noticed a clear contrast between our approach in 2020 and in later years where additional support was provided to students undertaking the virtual excursions and thus concur with the conclusion of Ruberto et al. (Citation2023) that the inclusion of active-learning tools in virtual field teaching is a strong predictor of success for students and instructors.
Like others (e.g., Bond & Cawood, Citation2021; Kastens & Ishikawa, Citation2006) we noted that the development of spatial cognition skills in non-immersive virtual environments was challenging for many students, and observing and interpreting details of the rocks and landscapes in the crucial third dimension remained difficult to replicate (Hoyer & Hastie, Citation2023; McLaughlin & Bailey, Citation2023). But training in the correspondence between 2D and 3D structures (e.g., LeClair, Citation2003) is an important step in the development of the visualization realm of spatial cognition (e.g., Ormand et al., Citation2017; Vasilyeva & Lourenco, Citation2012). Thus, still, field images in non-immersive virtual excursions may, if appropriately scaffolded, have unrealized potential to help students develop these critical skills.
Another significant disadvantage of the virtual excursions we identified was the profound difficulty in helping students to “see” and focus on the important details in the still images and videos provided. Even students who had some prior field experience were commonly distracted by unimportant detail that is, effectively, “unseen” by experienced geologists. Although this is always a problem as students learn to make observations in the field, it was particularly acute in the virtual environment. Fully immersive VR or panosphere-style images have been shown to be effective for higher-level students (e.g., Pugsley et al., Citation2022) but may not significantly improve the outcomes for commencing or less-experienced undergraduate students as such imagery can add higher fidelity that compounds distraction. The use of these technologies would need to be carefully scaffolded so that students are supported to practice their geological judgment in terms of deciding which features are important and relevant. In a second iteration of the virtual excursion, additional still images were provided as examples of how to “see” key geological features, but this still did not fully solve this issue.
Despite the disadvantages, across all three levels of our degree programs, we observed that some aspects of the field work could be replicated by the virtual excursion approach. In particular, a key aim of encouraging students to enjoy the virtual excursion was largely met (). Although we did not have the opportunity to rigorously evaluate our excursions in a formal way, subject final assessments showed that students had understood the content of the excursion and were able to relate the field work to the rest of the subject in similar ways as we observed in the pre-pandemic period. Moreover, we noticed no discernible difference in field reasoning for students who continued with geology subjects in the following years.
Assessment design improvements meant that the virtual excursions emphasized higher-level Bloom’s criteria, such as synthesis and analysis (Anderson et al., Citation2001; Bennett et al., Citation2017). Consequently, we observed students’ regional geological syntheses and regional-scale schematic cross-sections to be of a higher standard when compared to previous years. The potential for virtual excursions to connect student learning across a range of scales in this way has also been noted by others (e.g., Peace et al., Citation2021). Importantly, however, other skills could not be developed as well and additional supports were needed for hands-on field skills, such as collecting structural measurements, in subsequent classes.
For the University of Melbourne Level 2 volcanic landforms excursion, the success of the virtual excursion in helping students develop similar skills to those developed during the in-person trip has subsequently driven changes to the post-lockdown delivery of this course component. In 2022, an option to choose the virtual rather than the in-person excursion was provided, and 9/33 students elected to complete the virtual excursion. Reasons included illness, caution around COVID-19 exposure, chronic health conditions, and concerns about the physical and environmental challenges of in-person field work. For students who attend the in-person excursion, the exercises have been modified based on successful aspects of the virtual excursion, including the use of Google Earth in the field to give students a fuller three-dimensional view of the landscape. In this way, some of the most interesting elements of the virtual excursion are now blended with the in-person excursion, enhancing the in-person excursion and allowing all students to be assessed in comparable terms. This outcome supports previous research suggesting an integrated combination of in-person field work, virtual field work, and traditional teaching and learning activities may optimize outcomes opportunities for students (e.g., Friess et al., Citation2016; Guillaume et al., Citation2023).
Benefits of virtual excursions
Published literature on virtual field excursions often highlights their potential to increase diversity within our student cohort, as well as the accessibility of our discipline more generally (e.g., Bond & Cawood, Citation2021). Both are key challenges given the increasingly important role of trained Earth scientists in the solution of global resource and climate challenges, and the persistent global decline in geoscience student enrollments (e.g., Boatright et al., Citation2019; Cohen, Citation2022; Keane, Citation2021). Perceptions that geoscience is dominated by white men and that a geoscience career requires high levels of physical fitness and long hours of challenging field work are persistent barriers to accessibility and diversity in the profession (e.g., Atchison et al., Citation2019; Atchison & Libarkin, Citation2016; Bush & Mattox, Citation2020; Dutt, Citation2020) and contribute to the crisis of diversity among students taking geoscience subjects (e.g., Dowey et al., Citation2021; Giles et al., Citation2020; Huntoon & Lane, Citation2007). Indeed, a range of authors has suggested that such perceptions may mean students self-select away from geoscience (e.g., Bernard & Cooperdock, Citation2018; Carter et al., Citation2021; Stokes et al., Citation2015).
Fieldwork is a particular barrier to racially and ethnically diverse groups, neuro-diverse students, students with disabilities, and students from lower-socio-economic groups (e.g., Carrera et al., Citation2023; Hall et al., Citation2004; Marshall & Thatcher, Citation2019; Mol & Atchison, Citation2019). Thus, virtual excursions and/or the provision of online pre-fieldwork training modules have the potential to promote inclusion in Earth science and to increase opportunities for historically excluded groups and particularly those who have limited capacity to attend courses on campus or to complete fieldwork (e.g., Guillaume et al., Citation2023). Although we have not offered virtual excursions for sufficient time to assess any change in the diversity of our student group, student acknowledgment of monetary savings on field excursion costs as well as the number of students self-selecting our Level 2 volcanic landforms virtual excursion option in 2022 suggests at least some improvement in inclusion.
We also note that the widespread availability of virtual field excursions expands the range of teaching and learning activities available to students regardless of geographic location. For example, the spectacular rocks studied in the University of Melbourne virtual excursions documented above are available to our students simply by geological circumstance. Many areas of the world do not have such geology locally, so students are less likely to learn about these features.
Petrology and petrography teaching
Petrology is a key component of many geology subjects and at Levels 2 and 3 of the geology major, many subjects also include a component of petrography. In our igneous and metamorphic geology subjects in particular, petrology and petrography are a high proportion of class time with students learning from rock samples and thin sections in every practical class. These requirements posed obvious challenges for remote delivery of teaching, a challenge in common with other geoscience educators globally (e.g., Engel et al., Citation2023) as well within the biological and medical sciences (e.g., Amer & Nemenqani, Citation2020; Caruso, Citation2021).
At Monash University, small Level 3 class sizes (<20 enrollments) meant that rock samples were able to be posted directly to students during the lockdown period. For larger classes at Level 2, this was not possible so a combination of still photos (at a range of magnification) as well as videos were made to illustrate each sample (). This combination was necessary for mineral properties, such as cleavage, to be correctly observed.
Figure 6. Petrology and petrography teaching. (a) At Monash University and at The University of Melbourne, staff took home a microscope with camera attached, which enabled live demonstration of the properties of minerals down a microscope during classes; (b) Staff used high-resolution document cameras to demonstrate minerals and textures in rocks. At Monash University, students were posted the rock samples so they could use the videos to find the features in their own samples; (c) Image of the camera feed from the microscope during a synchronous online practical session; (d) Example of thin section images provided to students: for each field of view the stage was rotated through 90° with an image taken every 10° in plane polarized light and in cross-polarized light, producing 20 images. Here all photomicrographs are in cross-polarized light except the first and last, which are in plane-polarized light; (e) Representative positive student feedback from petrography classes; (f) Representative negative student feedback from petrography classes.
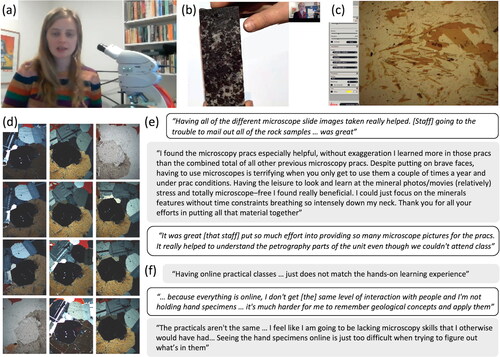
A much greater challenge was that students were not able to be supplied with petrographic microscopes for home use. Both at the University of Melbourne and Monash University modified forms of low-tech “virtual microscopes” were utilized. For Monash University, a “virtual microscope” was a series of plane-polarized and cross-polarized photomicrographs, taken every 10° as the microscope stage was rotated through 90°, allowing students to self-determine pleochroism, birefringence, and angles of extinction (). The Macquarie University online virtual petrographic microscope (https://imagematrix.science.mq.edu.au/; Tetley & Daczko, Citation2014) was also used. This platform provides a high-resolution photo of a large thin section area and the ability to zoom and rotate the photos, somewhat replicating the experience of manual microscope operation.
At the University of Melbourne, Level 2 igneous and metamorphic petrology and petrography were mainly taught using photographs and narrated movies, created by the instructors, of the hand specimens and thin sections in the teaching collection. This imagery was supplemented by the Open University’s Virtual Microscope (Whalley et al., Citation2011; www.virtualmicroscope.org), Frank K. Mazdab’s rockPTX resource (www.rockptx.com), Alessandro Da Mommio’s exceptional Thin Section library (www.alexstrekeisen.it) and SketchFab (www.sketchfab.com). As with the virtual excursion materials, our petrography and petrology resources were developed independently at each institution.
Student perspectives
During online teaching, students informally expressed appreciation for the effort involved in creating the online experience, but overall feedback in end-of-semester student evaluations was much less positive than our typical in-person petrography classes. Although some students found the virtual environment beneficial to their learning—as they were not constrained by timetabled access to rock samples and/or microscopes ()—many students did not, reporting the use of still images and videos frustrating and confusing. A significant number of students at both our institutions expressed concern about the impact of the virtual learning environment on their developing practical skills and worried that they would not be able to apply what they had learned from images to real specimens on their return to campus.
Instructor perspectives
For many rocks and minerals, the images we took of class specimens were in some ways more successful than a physical specimen, since students could view and discuss them at a much larger scale. However, the loss of tactile information, such as an impression of density or texture, was significant. Moreover, some minerals, such as biotite, were challenging even to photograph successfully. A related problem was revealed when collecting thin-section imagery with the in-built cameras of some microscopes producing an inaccurate and inconsistent impression of color.
A more pernicious problem was that providing images and movies produced a curated view of the samples. As with field work, in teaching petrography, one of the objectives is that students should learn to discern for themselves which features: (1) are key to identification; (2) can be used to provide additional interpretation, and (3) are “noise.” With limited time for movie editing, and constraints on the movie length and download size imposed by online learning management systems, the movies focused on the important features rather than providing an opportunity for students to practice making these judgments. As a result, the intended learning outcomes of petrography teaching at both our institutions changed significantly and were focused on questions about specific features seen in the thin section movies and images, rather than the more advanced skill of writing petrographic descriptions.
The challenges of teaching petrology and petrography online meant we made a range of assessment changes and saw benefits from a more flexible approach to deadlines and timing of course delivery. For example, before the pandemic, petrology classes at Monash University were assessed by the submission of practical materials at the end of each weekly class. During remote delivery of teaching, two weeks of practical material was combined into one longer practical, and students had two weeks to work on the problem set before assessment. This did not reduce the overall workload, but it did give students additional time between weekly classes, reducing stress for students () and staff and allowing more time for students to make connections between lectures and practical material. We also note that in assessing our petrography subjects, online assessment had one great advantage over in-person assessment in that all students saw the same sample material, rather than each student seeing only one sample from a non-identical class set. Student self-evaluations were typically more negative than the evaluation of the instructors, and we observed that most students were able to achieve the modified learning outcomes and subsequently apply and develop their knowledge appropriately in later on-campus classes.
Discussion
As noted by Bernard et al. (Citation2009), both learning gains and student-instructor engagement are favored by high levels of student-student, student-instructor, and student-content interaction. In the online environment, Martin and Bolliger (Citation2018) showed that student-instructor engagement was perceived by students to be the most influential factor in their learning. Our experiences during lockdown support this suggestion, and the additional time needed for preparation of new teaching materials meant we were not able to spend as much time with students as we would have liked, particularly affecting the virtual field excursions as noted above. The immersive teaching experience during lockdown has prompted us to reengage with the importance of these connections in facilitating learning, regardless of the mode of delivery of the subject content. Given that our experience is not unique, it is important that University leadership also acknowledge the importance of student-instructor connections and value the time for these to be developed.
Perhaps most significantly, our experiences of teaching through the COVID-19 pandemic have brought the general challenges of Earth science teaching into sharper focus. In Australia, Earth science is a very small discipline in terms of student and instructor numbers, and is taught across dispersed regions without the level of resourcing available to educators in more popular disciplines, such as chemistry and physics, and biology (e.g., Royal Australian Chemical Institute Chemistry Education Division). By coincidence, the informal Australasian Universities Geoscience Educators Network (AUGEN), first established in 2012, had moved to a formal organizational structure just before the pandemic. Before the pandemic, the group organized one annual meeting, but the ready availability of online meeting tools during the pandemic meant the newly established AUGEN committee could meet monthly and themed-talks and networking events, open to all members, were held every 2–3 months during 2020 and 2021. These events provided collaborative networking opportunities that were otherwise unavailable. The distribution of monthly AUGEN newsletters provided further peer support. Building on the momentum that began in the pandemic, AUGEN has continued to expand its program of regular events and has recently established a platform for sharing teaching materials. Given the relatively small Australasian geoscience education community together with the focus of many universities (including our own) on research excellence, collaborative peer networks are an important source of support for instructors at all levels.
Having reflected on work in the pandemic years, an imperative for our community now should be to capitalize on the time already invested and the wealth of research related to online teaching globally. The genuine goodwill and collaborative spirit of geoscience educators place our community well to move forward and improve many aspects of our offerings. Key goals should be: (1) documentation of our approaches to online teaching; and (2) development of accessible repositories for teaching materials that can be used or adapted by others (subject to any copyright requirements of individual universities where educators work). The benefits of sharing strategies and resources are significant and can only enhance the standard and reach of geoscience teaching everywhere. We note the excellent materials available from the National Association of Geoscience Teachers (NAGT—https://nagt.org/index.html) and new teaching resource-sharing initiatives within AUGEN (https://www.augenteam.net/), however, there is potential for much more. For virtual excursions, for example, a Google Earth style library that allows educators to browse global locations and to view available imagery and worksheets/assignments should be an aspiration for the global geoscience education community. Similarly, global community support for open-access virtual petrographic microscope platforms would provide significant benefits for instructors and students worldwide.
Predictions of profound long-term change to higher education following the COVID-19 pandemic (e.g., Arday, Citation2022; Baré et al., Citation2021; Eringfeld, Citation2021) were only partially realized at our institutions. From Semester 1, 2023, Monash University made a permanent shift to have all lecture content delivered asynchronously using prerecorded videos while practical and tutorial classes remain in-person on campus. In contrast at the same time The University of Melbourne returned to pre-pandemic teaching norms, with no online or blended learning options for lecture, practical, and tutorial sessions. However, regardless of delivery mode, the resources we have developed and the insights we have gained from our remote teaching experiences have enhanced our individual and collective teaching practice. Our field teaching now embraces tools and imagery developed for online learning to provide a hybrid active-learning framework for our students. Similarly, petrography resources developed for online learning are now provided as additional support for revision and review complementing in-person laboratory classes. Our assessment practices now emphasize geological synthesis and problem-solving skills, rather than simple recall of materials. Our observations of the utility of “low-tech” approaches to remote delivery of teaching are potentially significant as they show that large monetary or technological investments are not necessarily needed for effective teaching and learning online. In these ways, the COVID-19 pandemic has brought about real change as we develop and use new approaches to add value to our existing teaching practice. Like others in the community, we are optimistic about the benefits these approaches and resources will have for improving the diversity of our student cohort and for engagement with Earth science more generally.
Supplemental Material
Download Zip (8.6 MB)Acknowledgments
We gratefully acknowledge the patience, understanding, and support of the students in our classes through the challenging years of 2020 and 2021. Sincere thanks to three anonymous reviewers and to the editorial team—particularly Kim Hannula—for supportive and insightful feedback which substantially improved our contribution.
Disclosure statement
No potential conflict of interest was reported by the author(s).
References
- Alammary, A., Sheard, J., & Carbone, A. (2014). Blended learning in higher education: Three different design approaches. Australasian Journal of Educational Technology, 30(4), 440–454. https://doi.org/10.14742/ajet.693
- Alías, G., Sendrós, A., Aulinas, M., Bordonau, J., Domènech, C., Masana, E., & Martín-Martín, J. D. (2023). The impact of online teaching on Geology degree programs during COVID-19: A case study from the University of Barcelona (Spain). Journal of Geoscience Education, 1–15. https://doi.org/10.1080/10899995.2023.2165858
- Amer, M. G., & Nemenqani, D. M. (2020). Successful use of virtual microscopy in the assessment of practical histology during pandemic COVID-19: A descriptive study. Journal of Microscopy and Ultrastructure, 8(4), 156–161. https://doi.org/10.4103/JMAU.JMAU_67_20
- Anderson, L. W., Krathwohl, D. R., Airasian, P. W., Cruikshank, K. A., Mayer, R., Pintrich, P. R., Raths, J., & Wittrock, M. C. (2001). A taxonomy for learning, teaching, and assessing: A revision of bloom’s taxonomy of educational objectives (1st ed.). Longman.
- Arday, J. (2022). Covid-19 and higher education: The times they are A’Changin. Educational Review, 74(3), 365–377. https://doi.org/10.1080/00131911.2022.2076462
- Atchison, C. L., & Libarkin, J. C. (2016). Professionally held perceptions about the accessibility of the geosciences. Geosphere, 12(4), 1154–1165. https://doi.org/10.1130/GES01264.1
- Atchison, C. L., Marshall, A. M., & Collins, T. D. (2019). A multiple case study of inclusive learning communities enabling active participation in geoscience field courses for students with physical disabilities. Journal of Geoscience Education, 67(4), 472–486. https://doi.org/10.1080/10899995.2019.1600962
- Australian Department of Education (2020). Selected higher education statistics – 2020 Student data. Department of Education. Retrieved from https://www.education.gov.au/higher-education-statistics/student-data
- Baré, E., Beard, J., Marshman, I., & Tjia, T. (2021). Does the COVID-19 emergency create an opportunity to reform the Australian University Workforce? Melbourne CSHE. Retrieved from https://melbourne-cshe.unimelb.edu.au/fellow-voices/evolving-australian-university-workforce
- Bennett, S., Agostinho, S., & Lockyer, L. (2017). The process of designing for learning: Understanding university teachers’ design work. Educational Technology Research and Development, 65(1), 125–145. https://doi.org/10.1007/s11423-016-9469-y
- Benson, R. G. (2010). The campus mine: An adaptable instruction approach using simulated underground geology in a campus building to improve geospatial reasoning before fieldwork. Journal of Geoscience Education, 58(5), 253–261. https://doi.org/10.5408/1.3559688
- Bernard, R. E., & Cooperdock, E. H. G. (2018). No progress on diversity in 40 years. Nature Geoscience, 11(5), 292–295. https://doi.org/10.1038/s41561-018-0116-6
- Bernard, R. M., Abrami, P. C., Borokhovski, E., Wade, C. A., Tamim, R. M., Surkes, M. A., & Bethel, E. C. (2009). A meta-analysis of three types of interaction treatments in distance education. Review of Educational Research, 79(3), 1243–1289. https://doi.org/10.3102/0034654309333844
- Bernard, R., Abrami, P., Lou, Y., Borokhovski, E., Wade, A., Wozney, L., Wallet, P., Fiset, M., & Huang, B. (2004). How does distance education compare to classroom instruction? A meta-analysis of the empirical literature. Review of Educational Research, 74(3), 379–439. https://doi.org/10.3102/00346543074003379
- Boatright, D., Davies-Vollum, S., & King, C. (2019). Earth science education: The current state of play. Geoscientist, 29(8), 16–19. https://doi.org/10.1144/geosci2019-045
- Bond, C. E., & Cawood, A. J. (2021). A role for virtual outcrop models in blended learning – Improved 3D thinking, positive perceptions of learning and the potential for greater equality, diversity and inclusivity in. Geoscience Communication, 4(2), 233–244. https://doi.org/10.5194/gc-4-233-2021
- Bursztyn, N., Walker, A., Shelton, B., & Pederson, J. (2017). Assessment of student learning using augmented-reality Grand Canyon field trips for mobile smart devices. Geosphere, 13(2), 260–268. https://doi.org/10.1130/GES01404.1
- Bush, P., & Mattox, S. (2020). Decadal review: How gender and race of geoscientists are portrayed in physical geology textbooks. Journal of Geoscience Education, 68(1), 2–7. https://doi.org/10.1080/10899995.2019.1621715
- Butterworth, P., Schurer, S., Trinh, T.-A., Vera-Toscano, E., & Wooden, M. (2022). Effect of lockdown on mental health in Australia: Evidence from a natural experiment analysing a longitudinal probability sample survey. The Lancet. Public Health, 7(5), e427–e436. https://doi.org/10.1016/S2468-2667(22)00082-2
- Çaliskan, O. (2011). Virtual field trips in education of Earth and environmental sciences. Procedia-Social and Behavioral Sciences, 15, 3239–3243. https://doi.org/10.1016/j.sbspro.2011.04.278
- Carrera, A., Luckie, T., & Cooperdock, E. H. G. (2023). Extreme underrepresentation of first-generation college students in the geosciences: An intersectional issue. Journal of Geoscience Education, 1–12. https://doi.org/10.1080/10899995.2023.2187233
- Carter, S. C., Griffith, E. M., Jorgensen, T. A., Coifman, K. G., & Griffith, W. A. (2021). Highlighting altruism in geoscience careers aligns with diverse US student ideals better than emphasizing working outdoors. Communications Earth & Environment, 2(1), 213. https://doi.org/10.1038/s43247-021-00287-4
- Caruso, M. C. (2021). Virtual microscopy and other technologies for teaching histology during Covid-19. Anatomical Sciences Education, 14(1), 19–21. https://doi.org/10.1002/ase.2038
- Cohen, D. R. (2022). Australian geoscience tertiary education profile 2003–2021 – AGC. Australian Geoscience Council Reports. Retrieved from https://www.agc.org.au/wp-content/uploads/2023/01/AGC-Tertiary-Education-Profile-2003-2021-Report.pdf
- Crawford-Ferre, H. G., & Wiest, L. R. (2012). Effective online instruction in higher education. Quarterly Review of Distance Education. Retrieved from https://www.learntechlib.org/p/131979/
- Currie, H. N. (2020). Mindful well-being and learning. Journal of Chemical Education, 97(9), 2393–2396. https://doi.org/10.1021/acs.jchemed.0c00777
- Czeisler, M. É., Wiley, J. F., Facer-Childs, E. R., Robbins, R., Weaver, M. D., Barger, L. K., Czeisler, C. A., Howard, M. E., & Rajaratnam, S. M. W. (2021). Mental health, substance use, and suicidal ideation during a prolonged COVID-19-related lockdown in a region with low SARS-CoV-2 prevalence. Journal of Psychiatric Research, 140, 533–544. https://doi.org/10.1016/j.jpsychires.2021.05.080
- Dodd, R. H., Dadaczynski, K., Okan, O., McCaffery, K. J., & Pickles, K. (2021). Psychological wellbeing and academic experience of university students in Australia during COVID-19. International Journal of Environmental Research and Public Health, 18, 866. https://doi.org/10.3390/ijerph18030866
- Dolphin, G., Dutchak, A., Karchewski, B., & Cooper, J. (2019). Virtual field experiences in introductory geology: Addressing a capacity problem, but finding a pedagogical one. Journal of Geoscience Education, 67(2), 114–130. https://doi.org/10.1080/10899995.2018.1547034
- Dowey, N., Barclay, J., Fernando, B., Giles, S., Houghton, J., Jackson, C., Khatwa, A., Lawrence, A., Mills, K., Newton, A., Rogers, S., & Williams, R. (2021). A UK perspective on tackling the geoscience racial diversity crisis in the Global North. Nature Geoscience, 14(5), 256–259. https://doi.org/10.1038/s41561-021-00737-w
- Dutt, K. (2020). Race and racism in the geosciences. Nature Geoscience, 13(1), 2–3. https://doi.org/10.1038/s41561-019-0519-z
- Elkins, J. T., & Elkins, N. M. L. (2007). Teaching geology in the field: Significant geoscience concept inventory gains in entirely field-based introductory geology courses. Journal of Geoscience Education, 55(2), 126–132. https://doi.org/10.5408/1089-9995-55.2.126
- Engel, K. T., Davidson, J., Jolley, A., Kennedy, B., & Nichols, A. R. L. (2023). Development of a virtual microscope with integrated feedback for blended geology labs. Journal of Geoscience Education, 1–15. https://doi.org/10.1080/10899995.2023.2202285
- Eringfeld, S. (2021). Higher education and its post-coronial future: Utopian hopes and dystopian fears at Cambridge University during Covid-19. Studies in Higher Education, 46(1), 146–157. https://doi.org/10.1080/03075079.2020.1859681
- Fleming, Z. (2022). Using virtual outcrop models and google earth to teach structural geology concepts. Journal of Structural Geology, 156, 104537. https://doi.org/10.1016/j.jsg.2022.104537
- Friess, D. A., Oliver, G. J. H., Quak, M. S. Y., & Lau, A. Y. A. (2016). Incorporating “virtual” and “real world” field trips into introductory geography modules. Journal of Geography in Higher Education, 40(4), 546–564. https://doi.org/10.1080/03098265.2016.1174818
- Giles, S., Jackson, C., & Stephen, N. (2020). Barriers to fieldwork in undergraduate geoscience degrees. Nature Reviews Earth & Environment, 1(2), 77–78. https://doi.org/10.1038/s43017-020-0022-5
- Gregory, D. D., Tomes, H. E., Panasiuk, S. L., & Andersen, A. J. (2022). Building an online field course using digital and physical tools including VR field sites and virtual core logging. Journal of Geoscience Education, 70(1), 85–100. https://doi.org/10.1080/10899995.2021.1946361
- Guillaume, L., Laurent, V., & Genge, M. J. (2023). Immersive and interactive three-dimensional virtual fieldwork: Assessing the student learning experience and value to improve inclusivity of geosciences degrees. Journal of Geoscience Education, 1–14. https://doi.org/10.1080/10899995.2023.2200361
- Hall, T., Healey, M., & Harrison, M. (2004). Fieldwork and disabled students: Discourses of exclusion and inclusion. Journal of Geography in Higher Education, 28(2), 255–280. https://doi.org/10.1080/0309826042000242495
- Hannula, K. A. (2019). Do geology field courses improve penetrative thinking? Journal of Geoscience Education, 67(2), 143–160. https://doi.org/10.1080/10899995.2018.1548004
- Harknett, J., Whitworth, M., Rust, D., Krokos, M., Kearl, M., Tibaldi, A., Bonali, F. L., Van Wyk de Vries, B., Antoniou, V., Nomikou, P., Reitano, D., Falsaperla, S., Vitello, F., & Becciani, U. (2022). The use of immersive virtual reality for teaching fieldwork skills in complex structural terrains. Journal of Structural Geology, 163, 104681. https://doi.org/10.1016/j.jsg.2022.104681
- Hodges, C., Moore, S., Lockee, B., Trust, T., & Bond, A. (2020, March 17). The difference between emergency remote teaching and online learning. EDUCAUSE Review. Retrieved from https://er.educause.edu/articles/2020/3/the-difference-between-emergency-remote-teaching-and-online-learning
- Horota, R. K., Senger, K., Rodes, N., Betlem, P., Smyrak-Sikora, A., Jonassen, M. O., Kramer, D., & Braathen, A. (2023). West Spitsbergen fold and thrust belt: A digital educational data package for teaching structural geology. Journal of Structural Geology, 167, 104781. https://doi.org/10.1016/j.jsg.2022.104781
- Hoyer, L., & Hastie, W. W. (2023). Geoscience undergraduate students’ perceptions of how field work and practical skills influence their conceptual understanding and subject interest. Journal of Geoscience Education, 71(2), 158–176. https://doi.org/10.1080/10899995.2022.2110630
- Huntoon, J. E., & Lane, M. J. (2007). Diversity in the geosciences and successful strategies for increasing diversity. Journal of Geoscience Education, 55(6), 447–457. https://doi.org/10.5408/1089-9995-55.6.447
- Kastens, K. A., & Ishikawa, T. (2006). Spatial thinking in the geosciences and cognitive sciences: A cross-disciplinary look at the intersection of the two fields. Geological Society of America Special Papers, 413, 53–76. https://doi.org/10.1130/2006.2413(05)
- Keane, C. (2021). U.S. geoscience enrollments and degrees collapse in 2019–2020. American Geosciences Institute. Retrieved from https://www.americangeosciences.org/geoscience-currents/us-geoscience-enrollments-and-degrees-collapse-2019-2020
- King, H. (2012). Student difficulties in learning geoscience. Planet, 25(1), 40–47. https://doi.org/10.11120/plan.2012.00250040
- Klippel, A., Zhao, J., Jackson, K. L., La Femina, P., Stubbs, C., Wetzel, R., Blair, J., Wallgrün, J. O., & Oprean, D. (2019). Transforming earth science education through immersive experiences: Delivering on a long held promise. Journal of Educational Computing Research, 57(7), 1745–1771. https://doi.org/10.1177/0735633119854025
- LaDue, N. D., & Pacheco, H. A. (2013). Critical experiences for field geologists: Emergent themes in interest development. Journal of Geoscience Education, 61(4), 428–436. https://doi.org/10.5408/12-375.1
- Latchem, C. (2018). Australia. In A. Qayyum and O. Zawacki-Richter (Eds.), Open and distance education in Australia, Europe and the Americas. Springer. https://doi.org/10.1007/978-981-13-0298-5_2
- LeClair, E. (2003). Alphatome-enhancing spatial reasoning. Journal of College Science Teaching, 33(1), 26–31.
- Manner, B. M. (1995). Field studies benefit students and teachers. Journal of Geological Education, 43(2), 128–131. https://doi.org/10.5408/0022-1368-43.2.128
- Marshall, A. M., & Thatcher, S. (2019). Creating spaces for geoscientists with disabilities to thrive. EOS, 100. https://doi.org/10.1029/2019EO136434
- Martin, F., & Bolliger, D. U. (2018). Engagement matters: Student perceptions on the importance of engagement strategies in the online learning environment. Online Learning, 22(1), 205–222. https://doi.org/10.24059/olj.v22i1.1092
- Martin, L. (2020). The student experience of online learning in Australian higher …. Tertiary Education Quality Standards Agency. Retrieved from https://www.teqsa.gov.au/sites/default/files/student-experience-of-online-learning-in-australian-he-during-covid-19.pdf?v=1606442611
- McLaughlin, J. A., & Bailey, J. M. (2023). Students need more practice with spatial thinking in geoscience education: A systematic review of the literature. Studies in Science Education, 59(2), 147–204. https://doi.org/10.1080/03057267.2022.2029305
- Mogk, D. W., & Goodwin, C. (2012). Learning in the Field: Synthesis of research on thinking and learning in the geosciences. In K. A. Kastens & C. A. Manduca (Eds.), Earth and mind II: A synthesis of research on thinking and learning in the geosciences (pp. 131–163). Geological Society of America Special Paper 486.
- Mol, L., & Atchison, C. (2019). Image is everything: Educator awareness of perceived barriers for students with physical disabilities in geoscience degree programs. Journal of Geography in Higher Education, 43(4), 544–567. https://doi.org/10.1080/03098265.2019.1660862
- Nyarko, S. C., & Petcovic, H. L. (2023). Do students develop teamwork skills during geoscience fieldwork? A case study of a hydrogeology field course. Journal of Geoscience Education, 71(2), 145–157. https://doi.org/10.1080/10899995.2022.2107368
- Orion, N., Ben-Chaim, D., & Kali, Y. (1997). Relationship between earth-science education and spatial visualization. Journal of Geoscience Education, 45(2), 129–132. https://doi.org/10.5408/1089-9995-45.2.129
- Ormand, C. J., Shipley, T. F., Tikoff, B., Dutrow, B., Goodwin, L. B., Hickson, T., Atit, K., Gagnier, K., & Resnick, I. (2017). The spatial thinking workbook: A research-validated spatial skills curriculum for geology majors. Journal of Geoscience Education, 65(4), 423–434. https://doi.org/10.5408/16-210.1
- Peace, A. L., Gabriel, J. J., & Eyles, C. (2021). Geoscience fieldwork in the age of COVID-19 and beyond: Commentary on the development of a virtual geological field trip to Whitefish Falls, Ontario, Canada. Geosciences, 11(12), 489. https://doi.org/10.3390/geosciences11120489
- Peasland, E. L., Henri, D. C., Morrell, L. J., & Scott, G. W. (2019). The influence of fieldwork design on student perceptions of skills development during field courses. International Journal of Science Education, 41(17), 2369–2388. https://doi.org/10.1080/09500693.2019.1679906
- Petcovic, H. L., Stokes, A., & Caulkins, J. L. (2014). Geoscientists’ perceptions of the value of undergraduate field education. GSA Today, 24(7), 4–10. https://doi.org/10.1130/GSATG196A.1
- Pugsley, J. H., Howell, J. A., Hartley, A., Buckley, S. J., Brackenridge, R., Schofield, N., Maxwell, G., Chmielewska, M., Ringdal, K., Naumann, N., & Vanbiervliet, J. (2022). Virtual field trips utilizing virtual outcrop: Construction, delivery and implications for the future. Geoscience Communication, 5(3), 227–249. https://doi.org/10.5194/gc-5-227-2022
- Rader, E., Love, R., Reano, D., Dousay, T. A., & Wingerter, N. (2021). Pandemic minecrafting: An analysis of the perceptions of and lessons learned from a gamified virtual geology field camp. Geoscience Communication, 4(4), 475–492. https://doi.org/10.5194/gc-4-475-2021
- Rotzien, J. R., Sincavage, R., Pellowski, C., Gavillot, Y., Filkorn, H., Cooper, S., Shannon, J., Yildiz, U., Sawyer, F., & Uzunlar, N. (2021). Field-based geoscience education during the COVID-19 pandemic: Planning, execution, outcomes, and forecasts. GSA Today, 31(3–4), 4–10. https://doi.org/10.1130/GSATG483A.1
- Ruberto, T., Mead, C., Anbar, A. D., & Semken, S. (2023). Comparison of in-person and virtual Grand Canyon undergraduate field trip learning outcomes. Journal of Geoscience Education, 1–17. https://doi.org/10.1080/10899995.2023.2186067
- Simonson, M., Schlosser, C., & Orellana, A. (2011). Distance education research: A review of the literature. Journal of Computing in Higher Education, 23(2–3), 124–142. https://doi.org/10.1007/s12528-011-9045-8
- Stainfield, J., Fisher, P., Ford, B., & Solem, M. (2000). International virtual field trips: A new direction? Journal of Geography in Higher Education, 24(2), 255–262. https://doi.org/10.1080/713677387
- Stokes, P. J., Levine, R., & Flessa, K. W. (2015). Choosing the geoscience major: Important factors, race/ethnicity, and gender. Journal of Geoscience Education, 63(3), 250–263. https://doi.org/10.5408/14-038.1
- Tetley, M. G., & Daczko, N. R. (2014). Virtual petrographic microscope: A multi-platform education and research software tool to analyse rock thin-sections. Australian Journal of Earth Sciences, 61(4), 631–637. https://doi.org/10.1080/08120099.2014.886624
- Vally, H., & Bennett, C. (2021). Covid in Victoria: 262 days in Lockdown, 3 stunning successes and 4 avoidable failures. The Conversation. Retrieved from https://theconversation.com/covid-in-victoria-262-days-in-lockdown-3-stunning-successes-and-4-avoidable-failures-172408
- Vasilyeva, M., & Lourenco, S. F. (2012). Development of spatial cognition. Wiley Interdisciplinary Reviews. Cognitive Science, 3(3), 349–362. https://doi.org/10.1002/wcs.1171
- Viskupic, K., Egger, A. E., McFadden, R. R., & Schmitz, M. D. (2021). Comparing desired workforce skills and reported teaching practices to model students’ experiences in undergraduate geoscience programs. Journal of Geoscience Education, 69(1), 27–42. https://doi.org/10.1080/10899995.2020.1779568
- Waldron, J. W. F., Locock, A. J., & Pujadas-Botey, A. (2016). Building an outdoor classroom for field geology: The geoscience garden. Journal of Geoscience Education, 64(3), 215–230. https://doi.org/10.5408/15-133.1
- Whalley, P., Kelley, S., & Tindle, A. (2011). The role of the virtual microscope in distance learning. Open Learning: The Journal of Open and Distance Learning, 26(2), 127–134. https://doi.org/10.1080/02680513.2011.567460