Abstract
The development of on-line sensors for compositional analysis during cheese manufacture is desirable for improved quality control. Dielectric properties of a food product are principally determined by its moisture and salt content. This indicates that dielectric spectroscopy may offer a rapid, on-line and non-destructive method for the determination of moisture and salt content of process cheese. However limited information is available in the literature on the dielectric properties of process cheese. Therefore the aims of this study are to investigate the dielectric properties of process cheese samples over a range of compositional parameters and to assess the potential of dielectric spectroscopy to improve process control during process cheese manufacture. Dielectric spectra of process cheese samples were measured using a coaxial line probe between 300 MHz and 3 GHz. A clear tend was observed between higher moisture content and increases in the dielectric constant. Inorganic salt content was found to have a major influence on the loss factor. The dielectric data obtained was used to develop chemometric models for the prediction of moisture and inorganic salt content of two experimental sets of process cheese samples (exp A and exp B). The root mean square error of prediction (RMSEP) for the models developed to predict moisture content were 0.524% (w/w) (exp A), and 0.423% (w/w) (exp B), while the RMSEP of the inorganic salt models were 0.220% (w/w) (exp A), and 0.263% (w/w) (exp B). It was concluded that dielectric spectroscopy has potential application for compositional analysis in process cheese manufacture.
INTRODUCTION
There is an increasing demand for improved measurement and control techniques in cheese manufacture.[Citation1] Evaluation of cheese quality involves measurement of compositional parameters including moisture and salt content. However determination of composition is usually laboratory based and involves time-consuming and labour intensive techniques. Therefore the development of an on-line/at-line sensor, which allows for the objective, rapid and non-destructive determination of cheese composition would greatly enhance overall process control. Dielectric properties of a food material are primarily determined by their composition. Therefore dielectric properties should provide an insight into the key compositional parameters of a product. The properties of interest are the dielectric constant (ϵ′) and the loss factor (ϵ″).
The dielectric constant, ϵ′, is a measure of the capacity of a material to store electrical energy. The loss factor, ϵ″, relates to the ability of a material to dissipate energy. These properties are essentially dominated by molecular rotation of polar molecules and ionic interactions. Therefore the dielectric properties of food materials will principally be determined by moisture and salt content.[Citation2] This suggests a potential application of dielectric spectroscopy for the simultaneous determination of these compositional parameters in process cheese. Several studies have demonstrated the potential of using dielectric spectroscopy to determine the quality and composition of food products including meats,[Citation3–5] fruits,[Citation6,Citation7] dairy,[Citation8–10] and agricultural products.[Citation11] While Kudra et al. determined the dielectric properties of milk and its constituents at 2450 MHz,[Citation9] Green was one of the first to provide chemical analysis and dielectric measurements of cheese at high frequencies (750 MHz–12.4 GHz).[Citation10] In his study, Cheddar cheese samples were dried from 35% to 0% moisture content on a wet basis. Decreases in both the dielectric constant and loss factor were clearly evident.[Citation10] An increase in the salt content was indicated by a rapidly increasing loss factor at low frequencies. Green also found that the permittivity of the fat fraction was constant between 2.6 and 12.4 GHz with both a low dielectric constant and loss factor recorded. Further work has led to the development of an on-line dielectric sensor by Keam Holdem Associates for the determination of moisture and salt content in cheese.[Citation12,Citation13]
Mean differences between the predicted and actual results for moisture and salt content were approximately 0.5% and 0.2% respectively. However these studies focused solely on natural cheese.[Citation12,Citation13] With an increasing demand for improved measurement and control techniques in cheese manufacture, dielectric spectroscopy offers the potential for low cost, accurate sensing, which could achieve near instantaneous measurements of critical process control parameters. However there is limited information available on the dielectric properties of process cheese. Therefore the aims of this study are to investigate the dielectric properties of process cheese samples over a range of compositional parameters and to assess the potential of dielectric spectroscopy to improve process control during process cheese manufacture.
MATERIALS AND METHODS
Process Cheese Samples
Process cheese samples (n = 40) were manufactured at the Dairy Products Research Centre, Cork, Ireland in two separate experiments (exp A and exp B). Exp A samples were manufactured using direct steam injection, while indirect steam heating was employed in producing exp B samples. All samples were produced in a UMM/SK 5 Universal Cooker (Stephan Food Processing Technology, Germany). Exp A was a 5 × 3 factorial design with 9 duplicates. Exp B was a 3 × 3 factorial design with 7 duplicates. Exp A and exp B samples were manufactured on different dates.
The ingredients used were cheddar cheese, Irish commercial salted butter, water and emulsifying salts (disodium phosphate). The amount of each ingredient used in the production of the experimental samples is presented in . The total weight of each cheese manufactured was 3.1 kg. Ingredients were mixed in the cooker and then heated to 80° C, by the heating method specified, with constant stirring (knife at 300 rpm and baffle mixer at 80 rpm) for 2 mins. The resulting mixture was poured into plastic containers of food commercial grade with a capacity of 225 g, lidded, cooled and placed in storage at 4° C. The ingredients were varied as indicated in to give a range of compositions. The target compositions shown in , were selected as to extend beyond those used commercially by process cheese manufacturers.
Table 1 Formula for the production of 3.1 kg of each process cheese samples.
Table 2 Process cheese compositional targets (% w/w).
Compositional Analysis
The method used to determine the moisture content of the process cheese samples was drying in an air-oven for 16 h at 100° C. Finely cut and mixed cheese samples (∼5 g) were accurately weighed and spread uniformly in an aluminium dish. Cheese samples were dried overnight (∼16 h) at 100° C. Dried samples were removed from the oven, covered and cooled to room temperature in a desiccator (∼1 h) and weighed. The moisture content was calculated as the percentage loss in weight. Analyses were performed in duplicate with the mean being taken as the final result. The precision of moisture determinations was expressed as the standard deviation of the differences between the replicates (SDD). Inorganic salt content in the process cheese was calculated as the amount of disodium phosphate included plus the amount of sodium chloride in the butter and natural cheese added to the blend. The salt content of the butter and cheese was determined from the manufactures specifications and had salt contents of 2% (w/w) and 1.77% (w/w) respectively.
Dielectric Measurements
Dielectric measurements were made using a HP 85070 open-ended coaxial line probe, a HP network analyser (Hewlett Packard, CA, USA), a PC and associated software (). The system was calibrated using three known standards, air, a short circuit and distilled water at 25° C. Dielectric properties are temperature dependent, therefore samples were left out overnight to equilibrate to room temperature (18–22° C). The temperature of each sample was recorded immediately prior to the dielectric measurement using a digital thermocouple temperature probe (Sensor-Tech Ltd., Ireland). The cheese samples were raised on a ramp until in constant contact with the surface of the probe. The network analyser was used to automatically obtain dielectric spectra for each of the samples at 51 logarithmically spaced frequency points between 300 MHz and 3 GHz. Dielectric spectra were collected in triplicate for each sample, which each spectra measured at a different position on the sample.
Statistical Analysis
The standard deviations of the replicates across the dielectric spectra were calculated. In general the standard deviations at any frequency was less than 4% and this was used as a guide to identifying and rejecting outliers.[Citation4] Outliers are the result of poor or uneven contact between the probe and the sample or the presence of air bubbles close to the probe. Statistical analysis of the relationships between the dielectric properties and compositional values were carried out using SAS V 8.02 (SAS Institute Inc., USA). Chemometric models were developed using The Unscrambler software version 8.0 (CAMO Ltd, Norway). The calibration models were generated by partial least squares (PLS) regression and confirmed by full cross validation. Regressions were carried out using two response variables, moisture and inorganic salt content. The proficiency of the developed models to predict the level of these elements was expressed as the optimum number of PLS Loadings (L), root mean square error of prediction (RMSEP) [Eq. (Equation1)] and the coefficient of determination (R2).
I = number of samples, N = number of elements, y = response variable, F = y residual.
The optimal number of loadings was determined from the RMSEP curve. The number of loadings to be used was indicated by the first local minimum. Practical utility of the calibrations was assessed using the range error ratio (RER). Values for this ratio are calculated by dividing the range in content of a given compositional parameter by the prediction error for that parameter.[Citation14]
RESULTS AND DISCUSSION
Compositional Analysis
A summary of the compositional analysis results is presented in . Samples were analysed at 2 and/or 4 weeks post manufacture as outlined in . In exp A the moisture content ranged from 37.08–50.24% (w/w) whereas in exp B the moisture content was 37.5–48.4% (w/w). In both cases the inorganic salt content ranged from 2.5–4.7% (w/w). The precision of the laboratory results was determined as the standard deviation of the difference between the duplicates (SDD). The reproducibility of the moisture content results at week 2 for exps A and B were ± 0.41% and ± 0.1% respectively, while for exp A at week 4 it was ± 0.21%.
Table 3 Mean compositional analysis results for process cheese samples (% w/w).
Table 4 Overview of experimental analysis and dielectric spectra obtained
Dielectric Spectra
Dielectric spectra of all samples were recorded at 2 and/or 4 weeks post manufacture as detailed in . This resulted in a total of 55 spectra for chemometric analysis. and represent sample complex (i.e., ϵ′ and ϵ″) dielectric spectra of process cheese obtained in this study. Both figures illustrate the frequency dependent nature of the dielectric properties. The significant decreases in the loss factor over the frequency range tested may be attributed to the presence of salts introducing ionic conductivity.
Influence of Moisture Content on the Dielectric Properties
Previous studies would suggest that the polar rotation of molecules will dominate the dielectric spectrum.[Citation15] Therefore water, as a polar solvent, should primarily influence the dielectric properties of a food material. Initially the effect of moisture at two frequencies (948 MHz and 2460 MHz) was investigated. These frequencies were chosen due to their proximity to the two frequencies allocated for industrial use, namely 915 MHz and 2450 MHz. The significance of the relationship between moisture content and ϵ′ or ϵ″ was often similar at both frequencies (). However, the coefficient of determination (R2) was lower at 948 MHz () and hence that data is not further explored here. Therefore all further analysis of the influence of moisture content on the dielectric parameters was carried out at 2460 MHz.
Table 5 Coefficient of determination (R2) of correlations between compositional and dielectric parameters at two frequencies.
illustrates the relationship between moisture content and ϵ′ for exp A samples. It is clear from that at higher moisture contents, ϵ′ of the samples increase (R2 = 0.92). This correlation was also highly significant (). Although indicates that moisture significantly influenced ϵ″, the correlation coefficient was very low and was not illustrated (R2 = 0.32). Therefore ϵ′ will be primarily dominated by the moisture content of the process cheese samples. It was noted in that one sample (1 at 4 weeks) would seem to be an outlier. This experimental dielectric measurement is behaving in a significantly different manner to the rest of the set, which includes the same sample analysed at 2 weeks. Therefore we can attribute this outlier to errors in the dielectric measurement, which are most likely due to the presence of bubbles near to the probe.[Citation4]
The same positive relationships between ϵ′ and moisture content observed for the steam injected samples in was also apparent for the indirectly heated samples in exp B. illustrates that ϵ′ was principally affected by the moisture content of the samples (R2 = 0.99). The less significant association between ϵ″ and the moisture content (R2 = 0.38) () was again evident and hence is not reproduced here. These results suggest that in the cases of both cheese products the dielectric constant was almost entirely determined by moisture content. These findings correspond with those of Green who determined that ϵ′ was dominated by the moisture content of Cheddar cheese.[Citation10]
Influence of Inorganic Salt Content on the Dielectric Properties
To determine the effect of inorganic salt on the dielectric properties the data was treated as outlined above. In this case however, the effect of inorganic salt on the dielectric parameters was more apparent at the lower frequency of 948 MHz. This is to be expected due to the dominant effect of ionic conductivity on ϵ″ at lower frequencies already observed in . Therefore the following analysis of the influence of inorganic salt content on the dielectric properties took place at 948 MHz. demonstrates the correlation between the loss factor and inorganic salt content at 948 MHz for exp A. It illustrates that although this positive relationship is statistically significant, the coefficient of determination is low (R2 = 0.40) () and therefore a combination of factors are interacting to influence ϵ″. Moisture content is expected to have some influence on ϵ″ due to the significant influence of moisture on this property as detected in .
In order to further explore this, the samples were subdivided into groups based on moisture content in 3% intervals (). The correlations observed in each of these ranges was an improvement on that seen when salt was taken independently (R2 = 0.90–0.80). It was also noted that again sample 1 at week 4 recorded a lower loss factor than expected ().
Figure 7 Influence of salt content on the loss factor at 948 MHz in 4 moisture ranges for exp A samples.
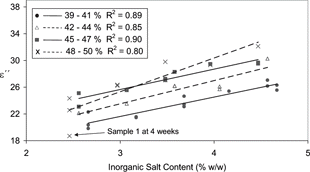
There was a limited positive relationship observed between the loss factor and the inorganic salt content in exp B (R2 = 0.53, ). This correlation was also less significant than the corresponding exp A correlation (). It was also noted that no significant relationship between moisture content and ϵ″ existed for this smaller sample set (). As expected in both sets of samples, no association was observed between the ϵ′ data and inorganic salt content. Therefore inorganic salt content will only influence ϵ″. However in contrast to the dominant effect of moisture on the ϵ′, ϵ″ would seem to be determined by a combination of the salt and the moisture content.
Development of Moisture and Inorganic Salt Content Prediction Models
Models were developed to predict both moisture and inorganic salt content of the two experimental sets of process cheese samples. The initial investigation has demonstrated correlations between the ϵ′ data and moisture content and ϵ″ data and inorganic salt content at discrete frequencies. However previous research has indicated that multivariate statistical techniques such as partial least squares (PLS) regression, which can utilise the entire spectrum, can improve the prediction accuracy of models.[Citation11] Therefore PLS regression was used to develop the calibration models for moisture and inorganic salt content. The full ϵ′ spectra were used to predict moisture content, while the full ϵ″ spectra were utilised in the prediction of inorganic salt content. PLS is a data compression method, which will find a few linear combinations (loadings) of the 51-point dielectric spectra. Only these loadings are used in the regression equation. This results in only the most relevant part of the variation in the spectra being used for regression. The parameter to be predicted, y, is computed using Eq. (Equation2), where a is the number of loadings, t is the loading scores and q is the y loadings.
The models developed for the steam injected samples (exp A) included 38 spectra as sample 1 at week 4 was removed due to its obvious outlying position ( and ). No samples were removed from the indirectly heated sample set (exp B). The moisture prediction models developed for exp A [Eq. (Equation3)] and exp B [Eq. (Equation4)] corresponded well with the available published data for natural cheese in which, the mean difference between the laboratory reference method and dielectrically determined moisture contents ranged from 0.4–0.8%.[Citation12,Citation13]
The exp A model had an RMSEP of 0.524% (w/w) and R2 of 0.98 (), while the exp B moisture model had an associated error of 0.423% (w/w) and R2 of 0.98 (). The range error ratio (RER) is a method of standardising the RMSEP by relating it to the range of the reference data[Citation14]. RER values of less than 6 indicate very poor classification and are not recommended for any application. An RER of 7–20 indicates that the model classification is poor to fair and could be used in a screening application. Finally a model that has an RER of 21–30 would illustrate good classification suggesting a role in a quality control application. The practical utility of the moisture content predication models were deemed to be very high (i.e., RER > 20) with the RER of the exp A and exp B models calculated as 25 and 26 respectively. It was noted that the exp A model, which involved a larger data set, was relatively more complex as it incorporated 5 loadings in comparison with the 3 loadings used in the exp B model [Eqs. (Equation3) and (Equation4)]. However the equations are relatively simple and hence should be sufficiently robust to yield good prediction results in the future.
Figure 9 Correlation plot for moisture content of the dielectric predicted and the reference data for the PLS model of exp A.
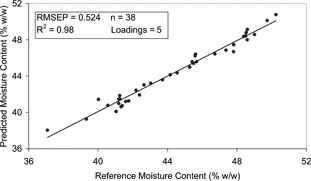
Figure 10 Correlation plot for moisture content of the dielectric predicted and the reference data for the PLS model of exp B.
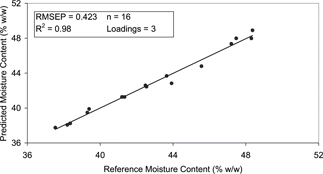
The ability of the developed models to predict inorganic salt content was found to correlate well with published reports in which salt content of Cheddar cheese was predicted with a mean difference of between 0.1–0.2%.[Citation16] represents the results obtained for the exp A salt prediction model. The model developed had an RMSEP of 0.220% (w/w) and R2 of 0.91 (). Similar results were observed in the exp B model with inorganic salt content predicted with an RMSEP of 0.263% (w/w) and R2 of 0.90 (). Both models were similar in their complexity with the equations incorporating 4 and 3 loadings in exp A [Eq. (Equation5)] and exp B [Eq. (Equation6)], respectively.
Figure 11 Correlation plot for inorganic salt content of the dielectric predicted and the reference data for the PLS model of exp A.
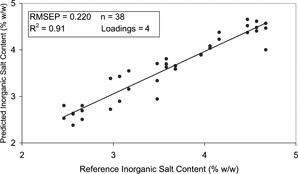
Figure 12 Correlation plot for inorganic salt content of the dielectric predicted and the reference data for the PLS model of exp B.
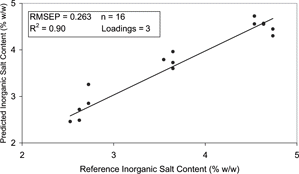
The exp A model was also deemed to have a poor to fair practical utility with a RER of 10. The RER of the exp B model was calculated as 8 and therefore also had a poor to fair practical utility. In order to improve the practical utility of the models, the salt content of the samples should be experimentally determined rather than based on the salt content of the ingredients added.
CONCLUSIONS
The development of on-line sensors for compositional analysis during cheese manufacture is desirable for improved quality control. The results of this study demonstrate the potential use of dielectric spectroscopy in such an application. Both directly and indirectly heated process cheese samples were found to have similar dielectric properties. In both experiments the moisture content of the samples was found to almost entirely dominate the dielectric constant. This resulted in a positive relationship between increasing moisture content and the dielectric constant. Although increases in the inorganic salt content resulted in an increasing loss factor, moisture content was also found to have an underlying effect on this property. It was determined that the dielectric constant and loss factor in conjunction with PLS regression could be used to predict moisture and inorganic salt content of process cheese products.
The moisture models developed were found to be of very high practical utility as indicated by the RER statistic. The models predicted the moisture content of the samples with a RMSEP of 0.524 and 0.423% (w/w). The inorganic salt prediction models were also demonstrated to be useful. The RMSEP associated with the inorganic salt models were 0.220 and 0.263% (w/w). However the practical utility of the models could be improved through experimental determination of salt content as opposed to the computations, based on ingredients added, used in this study. It was concluded that this technology has the potential to offer rapid, non-destructive and real-time measurement of inorganic salt and moisture content in process cheese manufacture.
ACKNOWLEDGMENT
The authors wish to acknowledge financial support from the Irish Department of Agriculture and Food under the Food Institutional Research Measure (FIRM), supported through EU and national funds.
Notes
****p < 0.0001.
**p < 0.01.
*p < 0.05.
12. Keam Holdem Associates Ltd. On-line measurement of cheese curd moisture on the draining belt. Application Note KHA0413. 1–4. Auckland, New Zealand,1999a.
13. Keam Holdem Associates Ltd. On-line measurement of moisture and salt content of 20 kg cheese blocks. Application Note KHA0414. 1–2. Auckland, New Zealand, 1999b.
16. Keam Holdem Associates Ltd. Salt measurement in dairy products. Application Note KHA0415, 1–2. Auckland, New Zealand, 1999c.
REFERENCES
- Blazquez , C. , Downey , G. , O'Donnell , C. and Howard , T.V. 2004 . Prediction of moisture, fat and inorganic salts in processed cheese by near infrared reflectance spectroscopy and multivariate data analysis . J. Near Infrared Spectrosc. , 12 : 149 – 158 .
- Herve , A.-G. , Tang , J. , Luedecke , L. and Feng , H. 1998 . Dielectric properties of cottage cheese and surface treatment using microwaves . J. Food Eng. , 37 : 309 – 410 . [CROSSREF]
- Kent , M. and Anderson , D. 1996 . Dielectric studies of added water in poultry meat and scallops . J. Food Eng. , 28 : 239 – 259 . [CROSSREF]
- Kent , M. , Knochel , R. , Dascher , F. and Berger , U-K. 2001 . Composition of foods including added water using microwave dielectric spectra . Food Control , 12 : 467 – 482 . [CROSSREF]
- Kent , M. , Peymann , A. , Gabriel , C. and Knight , A. 2002 . Determination of added water in pork product using microwave dielectric spectroscopy . Food Control , 13 : 143 – 149 . [CROSSREF]
- Nelson , S.O. , Forbus , W.R. and Lawrence , C.K. 1995 . Assessment of microwave permittivity for sensing peach maturity . Trans. ASAE , 38 : 579 – 585 .
- Garcia , A. , Torres , J.L. , Prieto , E. and De Blas , M. 2001 . Dielectric properties of grape juice at 0.2 and 3 ghz . J. Food Eng. , 48 : 203 – 211 . [CROSSREF]
- Shiinoki , Y. , Motouri , Y. and Kensuke , I. 1998 . On line monitoring of moisture and salt contents by the microwave transmission method in a continuous salted butter making process . J. Food Eng. , 38 : 153 – 157 . [CROSSREF]
- Kudra , T. , Raghavan , V. , Akyel , C. , Bosisio , R. and van de Voort , F. 1992 . Electromagnetic properties of milk and its constituents at 2.45 GHz . J. Microwave Power Electromagn. Energy. , 27 : 199 – 204 .
- Green , A.D. 1997 . Measurements of the dielectric properties of cheddar cheese . J. Microwave Power Electromagn. Energy , 32 : 16 – 27 .
- Lawerence , K. , Windham , W.R. and Nelson , S.O. 1998 . Sensing wheat moisture content independent of density . Trans. ASAE , 41 : 693 – 699 .
- 12. Keam Holdem Associates Ltd. On-line measurement of cheese curd moisture on the draining belt. Application Note KHA0413. 1–4. Auckland, New Zealand,1999a.
- 13. Keam Holdem Associates Ltd. On-line measurement of moisture and salt content of 20 kg cheese blocks. Application Note KHA0414. 1–2. Auckland, New Zealand, 1999b.
- Williams , P.C. 2001 . “ Implementation of near-infrared technology ” . In Near-Infrared Technology in the Agriculture and Food Industries , 2nd , Edited by: Williams , P and Norris , K. 165 Minnesota, , USA : American Association of Cereal Chemists, Inc. .
- Ryynänen , S. 1995 . The electromagnetic properties of food materials: a review of the basic principles . J. Food Eng. , 26 : 409 – 429 . [CROSSREF]
- 16. Keam Holdem Associates Ltd. Salt measurement in dairy products. Application Note KHA0415, 1–2. Auckland, New Zealand, 1999c.