Abstract
Acoustic/EMG system is presented to better measure the human perception of food texture through the use of sensory systems that monitor the mastication process. The sound transducer and EMG electrode system provide the means by which to measure the oral tactile sensation experienced by both masseter muscles and the auditory signals produced during mastication. Human testing was successfully preformed and signal data was recorded from both the EMG and acoustic sensors. The auditory signals, experienced by the subject during mastication, were recorded by a microphone placed in the ear. Maximum signal, total accumulative power and slope of accumulative power were extracted from the data and strong trends were seen, which corresponded to expected food texture within food groups. These parameters were able to distinguish between all products and similar trends were seen between EMG and acoustic results. The use of an acoustic sensor has the potential to drastically reduce commercial design costs and simplify data interpretation.
INTRODUCTION
The processes of food textural analysis and objective human testing have been tried and tested over many years and have formed the basis for the product analysis in many companies. Though these methods have been proven to be effective to a certain extent, the food industry is consistently looking for new ways to expand on and improve their testing techniques. Currently food textural analysis is primarily done through instrumental texture profile analysis (TPA) and sensory texture measurements. TPA could not be used to identify all components of the texture. TPA instrumentation can be used instead of human trials as a cheap, quantitative, repeatable, and efficient alternative. But TPA do not mimic the conditions inside the mouth during mastication and therefore are poor predictors of deterioration of food throughout the natural mastication process. Also TPA does not include psychological aspects of the texture. Sensory texture measurements conversely do incorporate the dynamics of the human mastication process using the perception of chewing, but they contain natural bias that is unavoidable even with well-trained panellists. Texture assessment will also depend on individual capability of subjects to analyze and translate their perceptions. Ideally food texture should be measured during unimpeded mastication by an objectionless sensor that would allow for repeatable quantitative data. Both of the current commonly favored methods fall short of meeting this objective. Therefore, the objective of this article is to provide a system that will better represent the human perception of food during mastication then previous methods.
The human perception of food texture can be broken down into three major categories: visual, tactile, and auditory. Visual perception of texture is a result of previous experience with similar foods. For example, viscosity of a fluid can be visually evaluated as it is poured into a container or tilted.Citation[1] Visual evaluation of food texture creates expectations and may introduce bias into the subsequent auditory and tactile evaluation of texture. Tactile texture can be broken into two major categories: oral and hand. Oral tactile perception of food is a response to muscle contractions and nerve impulses within the mouth, especially on the tongue. It can be further categorized into size and shape, mouthfeel characteristics, and phase change in the oral cavity. Size and shape of particles within food, and of the entire sample, are important in the evaluation of food texture. Hand tactile texture is rarely examined in food industry but does play a role in perception of food.Citation[1] Manipulation of food prior to oral evaluation may improve subject interpretation of food texture as handling occurs during normal consumption. There are two major sensory characteristics associated with the auditory perception of food sounds: the evenness and loudness of the sound. Continuous sounds are perceived as tearing or grinding while discontinuous sounds are perceived as popping or snapping. Sound loudness can increase the perception of crunchiness, crispiness, crackliness, sharpness, brittleness, hardness, and snappiness.Citation[1]
Electromyographic (EMG) analysis is a common means used to pick up the action potential of the motor units of a muscle to measure the strength of contraction.Citation[2] EMG allows the study of changes during the masticatory process. These changes in EMG parameters allow a better assessment of sensory characteristics than mechanical measurements. EMG based texture studies describe changes or differences in the signals.Citation[3] EMG can be used to collect data such as the mean EMG peak activity, the duration of the chewing cycle, the chewing rate, and the relative contraction time, which have been correlated to physical characteristics of the food such as its size and texture.Citation[4] Throughout the literature EMG has been used to characterize food texture and determine muscle response to various food textures. Correlation has been reported between food hardness and chewing time, number of chews, and peak amplitude of masseter.Citation4–6 Food hardness and fracturability have also been related to masseter total energy and peak energy of the first bite.Citation[6] Hard foods have a greater amplitude spike and longer duration of muscle activity per bite compared to soft foods.Citation[7] The foods fibrous and granulous nature affected chewing rate, often more than food hardness.Citation[5]
As with EMG signals, acoustic signals have also been linked to food characteristics. Low pitch sounds less than 1.9 kHz are typically associated with crunchiness and high pitch frequencies higher than 1.9 kHz were associated with crispiness. The low pitch sounds of a crunchy food heard while chewing are conducted through the bones of the skull and jaw to the ear. The human skull and jaw bone resonate at about 160 Hz and the sounds in this frequency range are therefore amplified.Citation[1] Crispy foods have been characterized by air-conducted high-frequency sounds of about 5 kHz while crunchy foods had a peak air-conducted frequency of 1.25 to 2 kHz.Citation[1] During mastication, texture differences between food samples can be determined by analyzing the generated frequency between 0 and 6000 Hz.Citation[8] Chewing sounds were analyzed to detect crispness differences among apples by calculating amplitude, energy, frequency content after fast Fourier transformation of sound weaves for bite and chew.Citation[9] In the frequency domain, the high values in the teeth signals between 700 and 900 Hz and to a lesser extent between 1200 and 1400 Hz affected the signals. The energy of first bite gave mostly a better indication of crispiness than the penetrometer firmness.
METHODOLOGY
Selection of Microphone and Muscle of Mastication for EMG Electrodes
An electret microphone was used due to its wide-range uniform frequency response, excellent transient response, and small size. The main muscles of mastication are the masseter, temporalis, and internal and external ptergyoideus muscles.Citation[10] Of these muscles only the temporalis, and masseter muscles are suitable for analysis using surface EMG electrodes.Citation[2] The posterior temporalis would require the shaving of hair above the ears to facilitate the placement of electrodes. Only the masseter muscle response was measured to limit subject discomfort, system complexity and practical commercial application.
Basic Design
The preliminary design consisted of measuring the activity of both masseter muscles with bi-polar surface electrodes and an electret microphone placed at the opening of the ear cannel through the use of an ear clip headset. The activity of the masseter muscles were measured with disposable Cleartrode™ Pregelled Electrodes (Multi BioSensors, Inc.), 1 cm diameter, bio-polar, silver-silver chloride electrodes and Noraxon TeleMyos EMG system. The EMG signal undergoes a total amplification gain of 2000. The data were sampled with the MyoResearch Version 2.11.90.81.200 (Noraxon USA, Inc.) at 6000 Hz. To attach the electret microphone to the ear clip headset, the speaker was removed from the left ear clip headset and a hole was drilled in the casing to allow the electret microphone to be put in place. A variable gain UK009—Electret Microphone Mini Amplifier with built-in bias voltage and adjustable gain and AOSafety Professional Hearing Protection Headset were added to avoid background noise. The Headset was modified so that only the acoustic data coming to one ear was dampened. All components were attached via 863 coax cable. A 9 V battery was needed to power the amplifier and provided the bias voltage. The electret microphone system was attached via a BNC connector to the Noraxon TeleMyos EMG systems BNC connector board, to allow for synchronous collection of EMG and acoustic signals.
Food Samples
Food samples were chosen to encompass a large range of food attributes and to test the specificity of the system. Foods were purchased from local food stores. Primary foods tested were cheese strings, un-pealed carrots, and celery. The carrot and celery samples were presented in lengths of between 8.2 cm to 13.5 cm sliced longitudinally, and the cheese strings were presented in their commercial form. Subjects were allowed to take a natural bite of these products to allow for a more natural chewing response to these types of foods. Secondary foods tested were at the extremes of the scale: gummy food (Gummy Bears and Nibs), and chips (Pringles and Sun Chips), these foods were presented in their natural state. The purpose behind their inclusion in the testing set was to determine if the acoustic system was sensitive enough to obtain significant data from closely related foods from the extremes of the spectrum.
Subject Selection and Preparation
Four subjects (3 males and 1 female, mean age of 23.75) with full dentition and no signs of jaw dysfunction were selected.
EMG electrode placement
Subject was asked to clench teeth and pulse masseter muscle. Muscle was palpated with fingertips to determine location of the muscle belly. Once the area was located it was scrubbed with paper towel and then with isopropyl-rubbing alcohol using Q-Tips. Two electrodes were then placed on each masseter muscle over the most active section found, approximately 0.5 cm apart. A ground electrode was placed over the bonny prominence of the right elbow. Leads were taped off to allow for a comfortable range of motion and limited pull on the electrodes.
Microphone placement
Ear clip headset was positioned around the left ear and the microphone was angled so as to point into the ear canal. The testers then held the ear clip headset in place while the subject put the noise dampening headset in place.
Sampling procedure
The Noraxon TeleMyos EMG system warmed up for 15 minutes prior to testing. Maximum force readings were obtained using an approximately 1 cm thick rectangle of paper towel. The paper towel was placed between the back molars of the left and right sides individually, and maximum force readings were taken for the left and right masseter muscles with a rest period between. This provided maximum force normalization data for the EMG signals, which would allow for comparison between data of the same subject with slightly different electrode placement if trials were to be repeated. The subjects were also asked to relax all jaw muscles and stay completely silent for a baseline reading of both acoustic and EMG signals for noise removal. All samples were included in triplicate and presented to the subject in randomized order. Each sample was handed to the subject and once total silence was achieved a visual cue was used to start the mastication process and data was collected until final swallowing. Subject was allowed to rest for 1 minute between samples and to take water at their discretion. The first three samples were repeated to limit the initial enhanced awareness of the system and the mastication process.
Data Analysis
Signal processing
Data from the microphone and left and right EMG electrodes were recorded as voltage versus time (Noraxon Inc. MyoResearch Version 2.11) at a sampling rate of 6 kHz. Real time signals appeared in the form seen in . The data were analyzed in the following manner using Matlab software:
Figure 1 Real time signals from subject 1 for Sun chip 1: A) right EMG (similar for left EMG), and B) acoustic.
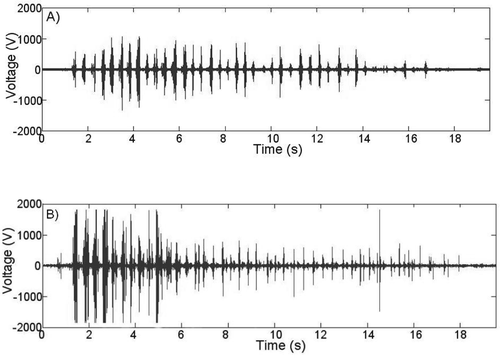
1 Rectified EMG and acoustic signals with baseline removal
Rectification was done by taking the absolute value of the original signal. Relaxed and silent trials were rectified and the average voltage was removed from the respective EMG and acoustic rectified signals (). This was done to remove the noise present in the system.
2 Merge of right and left EMG moving average signals
Rectified signals of right and left EMG were added together to form a total EMG signal (). This was done to allow for simplified analysis of bilateral chewing.
3 Moving average of EMG and acoustic signals
Moving averages were tested at 300, 600, and 900 samples to filter out the noise components of the signal to create a smooth graph with more distinct bite separation. The 600 sample filter was used for final data analysis because it created adequate bite separation without removing too much of the high frequency signal ( and ).
4 Normalization of total EMG signals moving average signals
Maximum force data from the left and right EMG signals were rectified and the maximum signal values were calculated. The right and left maximum values were summed and used as a normalization value for EMG total signals. Each total signal value was divided by the average maximum force value and multiplied by 100 to give a percent of maximum muscle force (). Acoustic signals were not normalized because no true quantitative maximum value was measured during trials.
5 Final noise removal from total EMG and acoustic signals
It was found that for some trials the baseline removal did not eliminate all of the noise, and with some trials some negative data points were produced from the baseline removal due to less noise in these trials then during the baseline trial. These phenomenons resulted in a shift of the data away from the zero axes. To zero these trials, the minimum signal value for each subject was determined, and this value was subtracted from all moving average data, resulting in the zeroing of the graphs.
Parameters Analyzed
1 Moving average graphs of total EMG and acoustic signals
Maximum signal.
2 Accumulative power graphs of total EMG and acoustic signals
Total accumulative power, and slope of the accumulation of power which was calculated between 20 and 80% of the total accumulative power.
RESULTS AND DISCUSSION
Maximum Signal
The maximum signal was obtained from the maximum peak signal of the moving average graphs and the results are shown in –. The maximum signal proved to be a good indicator of all product trends excluding the gummy products. Increasing upwards trends can be seen for the cheese-celery-carrots and Pringles-Sun Chips groups, for both EMG and acoustic signals. Significant differences were seen between the products of these groups. For the Gummy-Nibs group neither the EMG nor acoustic system was able to distinguish between these two products using maximum signal. This could have been due to the small product size and the tendency to stick to the teeth. EMG maximum signals may be the result of tightly squishing the product between the teeth, which would cause the major forces to become shear forces. With the acoustic data, the maximum peaks may have been caused by the teeth colliding or grinding and not the noise generated by the product being deformed. This did not seem to occur in the soft cheese product due to a much larger sample size and reduced product-teeth adhesion.
For the products tested, both maximum EMG and acoustic signals provided remarkably similar indications of food texture, and product differentiation. Due to the characteristics of EMG and acoustic signals, maximum EMG signals would be better at distinguishing between products that have a strong resistance to deformation and maximum acoustic signal would be better at distinguishing between products that produce significant noise signals during deformation. Significant samples size is required to reduce teeth to teeth contact. These two sets of data would compliment each other very well for samples such as tough liquorish that will generate a strong muscle force reaction but little sound or a sticky brittle product that may generate a strong noise signal during deformation but will take little muscle force.
Total Accumulative Energy
EMG total accumulative energy is a measure of how much energy is used during the entire mastication process. This relates to the hardness and resilience of a food. The results are shown in . When comparing between product groups a strong trend is observed. Cheese, Celery, and Carrot sticks have an increasing total accumulative energy as expected. The two chip products resulted in expected values with the harder less crumbly Sun Chip requiring more energy to masticate. The gummy products did not have a large difference between samples partly because of the type of chewing required for this product type. A significant amount of energy is exerted trying to pick the partially chewed pieces between the teeth with the tongue. Larger sample sizes could be used to reduce this effect in future trials. Despite this the firmer Nibs did require noticeably more energy to chew then the softer Gummy Bears as would be expected. Acoustic total accumulative energy is a measure of how much acoustical energy is produced during the entire mastication process. Acoustic results for accumulative energy are displayed in . All of the trends described above for EMG are seen in the acoustic data, which implies a very strong correlation between total accumulative energy for EMG and acoustic signals. Even Gummy Bears and Nibs were able to be identified using the acoustic total accumulated energy.
Slope of Accumulative Energy
The slope of accumulative energy was less consistent then maximum signal or total accumulative energy as an indicator of food texture, as seen from –. The EMG and acoustic slopes provided a weak determination between celery and carrots, and Gummy Bears and Nibs, but worked sufficiently well for determination between Pringles and Sun Chips, cheese and carrot, and cheese and celery. The EMG signal and acoustic signal showed opposite trends for Gummy Bears and Nibs and for celery and carrots. This parameter may not be as strong an indicator of food texture. It was hoped that if two products had a similar total accumulated energy that the slope would be able to distinguish between them.
Prototype Performance
The Acoustic/EMG system was able to determine between samples of different texture during natural mastication. For all parameters analyzed it was found that the Acoustic system could be used independently of the EMG system, though it should be noted that there may be products that were not tested here that may elicit enhanced data from using the whole Acoustic/EMG System. Use of the Acoustic system independently of the EMG System would drastically decrease the cost of the system.
The system provided an enhanced, unbiased measure of human perception of food texture, which is believed to be better than commonly used TPA and sensory texture analysis. For maximal measurement of the human perception to food texture the full Acoustic/EMG System should be used, as the EMG system most closely measure human tactile perception and the acoustic system auditory perception. Though as stated previously, these systems provide very similar measurements of food texture.
The system posed minimal interference with the mastication process. It did not cause significant enhanced awareness of the mastication greater then that, which would likely be inherent in any mastication measurement technique. There was a slight increase in awareness due to the covering of one ear, which slightly enhanced the sounds of mastication. The system was relatively easy to use.
The energy of the first bite (from EMG or acoustic signals) will provide a good indication of the crispness for solid compact foods like apple.Citation[9] Crisper samples will provide more total noise. The correlations with sensory textural attributes (such as crispness, crunchiness) of various foods could be determined after combining the information from various frequency bands after data segmentation. The correlations could also be developed among sensory textural attributes and various parameters suggested in this study, i.e., maximum EMG or acoustic signal, total energy accumulated, slope of the accumulated energy, etc.
CONCLUSIONS
The EMG/acoustic system was capable of quantifying differences in food texture. This system can produce repeatable, quantitative and unbiased measurement unlike sensory texture measurements. This system was capable of better representing the human perception of food during mastication then TPA. Similar trends were found in both EMG and acoustic parameters and both methods were able to distinguish between foods of different textures.
ACKNOWLEDGMENTS
Assistances provided by Dr. M. Oliver for the use of EMG system, Mr. Greg Northy and Dr. B. Zhao for their assistance in data acquisition and analysis, and Mr. Allan Miller and Mr. Bill Verspagen for their technical help are appreciated.
REFERENCES
- Lawless , H.T. and Heymann , H. 1999 . Sensory Evaluation of Food: Principles and Practices , New York : Aspen Publishers .
- Moller , E. 1974 . “ Action of the muscles of mastication ” . In Frontiers of Oral Physiology Volume 1: Physiology of Mastication , Edited by: Kawamura , Y. 121 – 157 . Basel, , Switzerland : S. Karger .
- Gonzalez , R. , Montoya , I. and Carcel , J. 2001 . Review: The use of electromyography on food texture assessment . Food Sci. Tech. Int. , 7 : 461 – 471 . [CSA]
- Karkazis , H.C. and Kossioni , A.E. 1997 . Re-examination of the surface EMG activity of the masseter muscle in young adults during chewing of two test foods . J. Oral Rehabilitation , 24 : 216 – 223 . [CSA] [CROSSREF]
- Karkazis , H.C. and Kossioni , A.E. 1998 . Surface EMG activity of the masseter muscle in denture wearers during chewing of hard and soft food . J. Oral Rehabilitation , 25 : 8 – 14 . [CSA] [CROSSREF]
- Sun , X.S. , Wang , F.T. , Carson , L. and Setser , C. 2001 . Electronic sensing system for food texture characterization . Trans. Amer. Soc. Agric. Engr. , 44 : 623 – 630 . [CSA]
- Peyron , M.A. , Maskawi , K. , Woda , A. , Tanguay , R. and Lund , J.P. 1997 . Effects of food texture and sample thickness on mandibular movement and hardness assessment during biting in man . J. Dent. Res. , 76 : 789 – 795 . [INFOTRIEVE] [CSA]
- De Beliea , N. , Sivertsvikb , M. and De Baerdemaekerc , J. 2003 . Difference in chewing sounds of dry-crisp snaks by multi-variate data analysis . J. Sound & Vibration , 266 : 625 – 643 . [INFOTRIEVE] [CSA] [CROSSREF]
- De Belle , N. , Harker , F.R. and De Baerdemaeker , J. 2002 . Crispness judgement of Royal Gala apples based on chewing sounds . Biosystems Engg , 81 : 297 – 303 . [CSA] [CROSSREF]
- Gray, H. Anatomy of the Human Body; Lea and Febiger: Philadelphia, PA, 1918; Accessed through Bartleby.com, 2003. www.bartleby.com/107/