Abstract
The efficiency of sonication and microfluidization to produce nano-emulsions were evaluated in this study. The purpose was to produce an oil-in-water nano-emulsion of d-limonene to apply it in the next step for nano-particle encapsulation. In the entrapment and retention of volatiles or for the microencapsulation efficiency, emulsion size is one of the critical factors. In this study, a bench-top sonicator and an air-driven microfluidizer were used to prepare the emulsions. Results show that, while both methods were capable of producing nano-emulsions of the size range of 150–700 nm, the microfluidizer produced emulsions with narrower size distributions and sonication was more convenient in terms of operation and cleaning. In general, the size of the emulsions decreased with increasing sonication time, or the microfluidization pressure and duration. However, for both sonication and microfluidization, optimal conditions were necessary for emulsification beyond which the emulsion sizes would either increase or have little change with further processing.
INTRODUCTION
Nano-emulsions are defined as emulsion systems mostly covering the size range of 50 to 200 nm (transparent) or up to 500 nm that have a milky appearance.[Citation1] The attraction of nano-emulsions for application in various industrial fields, for example, in personal care and cosmetics, health care, pharmaceuticals, and agrochemicals, is their very small droplet size and their high kinetic stability. Nano-emulsions can be prepared by low-energy emulsification methods or spontaneous emulsification such as phase inversion temperature (PIT) or phase inversion composition that are usually used in pharmaceutical and cosmetic industries.[Citation2] In most cases, however, submicron emulsions can be prepared using a high-energy input such as ultrasonication or by high-pressure homogenization and microfluidization.
The Microfluidizer® was originally designed by the Arthur D. Little Co., but was later taken over by the Microfluidics Corp.[Citation3] This equipment has been traditionally used in the pharmaceutical industry to make pharmaceutical emulsions and has been used to produce flavor emulsions or homogenized milk only in the last few years. It works on the principle of dividing a pressure stream into two parts, passing each part through a fine orifice, and directing the flows at each other in the heart of the microfluidizer, i.e., the interaction chamber. The microfluidizer uses a high pressure to guide the flow stream through microchannels toward the impingement area, which creates a very high shearing action that provides an exceptionally fine emulsion. Within the interaction chamber, cavitation, along with shear and impact, reduces emulsion droplet size.[Citation4]
Various studies have shown that emulsification by microfluidization is superior to the other types of conventional homogenization. The distributions of particle sizes produced by a microfluidizer appear to be narrower and smaller than the products of traditional homogenization. Pinnamaneni et al.[Citation5] found the microfluidizer to be more effective than the homogenizer in producing stable oil in water submicron emulsions at low emulsifier concentrations. Also, submicron emulsions prepared by the microfluidization process had smaller droplet diameters and exhibited less droplet diameter growth over time compared to high speed homogenization. Some workers have also studied the use of this device in the homogenization of dairy model emulsions.[Citation6–11] They examined the dependence of the droplet diameter on the emulsification pressure, temperature, and some product ingredients. Analysis of their data permits prediction of the fat globule size parameters as a function of process and formulation conditions.
Also, emulsification was one of the first applications of powerful ultrasound, and the first related patent was taken out more than fifty years ago.[Citation12] Since then, many scientists and industrialists have used different types of ultrasound devices to make emulsions. Cavitation is the main phenomenon responsible for ultrasonically induced effects.[Citation13] Cavitation is the formation and collapse of vapor cavities in a flowing liquid. Such a vapor cavity forms when the local pressure is reduced to that of the vapor at the temperature of the flowing liquid because of local velocity changes. The collapse of these cavities causes powerful shock waves to radiate throughout the solution in proximity to the radiating face of the tip, thereby breaking the dispersed liquid. A two-step mechanism has been proposed for ultrasound emulsification:[Citation14] At the first step, a combination of interfacial waves and instability leads to the eruption of dispersed phase droplets into the continuous phase; and the second step consists of breaking droplets up through cavitation near the interface. The intense effect (disruption and mixing) of shock waves explains the very small droplet size, and the most influential parameters are those affecting cavitation phenomena.
Emulsification is one of the important steps in microencapsulation technology. The emulsions are usually produced by high-pressure homogenizers and their droplet size is normally more than one micron. The effect of emulsion sizes larger than 1 micron on the efficiency and surface oil content of microcapsules have been studied by different workers.[Citation15, Citation16] Even the emulsions produced by two different methods such as ultrasonication and microfluidization can have different effects on the microencapsulation efficiency as have been studied by Mongenot et al.[Citation17] The effect of sub-micron emulsions on the efficiency and surface oil content of microcapsules is unclear and there have been no studies on the application of nano-emulsions in the microencapsulation of volatiles such as d-limonene. We decided to define the encapsulation of submicron particles (droplets) in larger particles as “nano-particle encapsulation.” It is different from nano-encapsulation in that the final product size of the particles is not in the nano range.
The present study investigated the production of submicron emulsions of oil-in-water type with ultrasonication and microfluidization. During preparation, several parameters including microfluidization pressure and cycles, ultrasonication time in highest power input, and emulsion composition were varied to determine their influence on the droplet size of the emulsions. These sub-micron emulsions would be used in the next step to produce microcapsules containing nano-particles (droplets) by spray drying (not undertaken in this study).
MATERIALS AND METHODS
Materials
Whey protein concentrate (WPC) and d-limonene were supplied by Steggall Nutrition (Brisbane, QLD, Australia) and Quest International (Gold Coast, QLD, Australia), respectively. Modified starch (Hi-Cap) was purchased from National Starch and maltodextrin (DE 17) from Fieldose Company.
Experimental Parameters
In order to study the effect of dispersed-phase concentration on the emulsion size, two levels of d-limonene concentration, 20 and 25% of the total solids, which are typical concentrations in microencapsulation by spray drying, were selected. The continuous phase of the emulsions consisted of WPC, HI-cap and maltodextrin (aqueous solutions of 40% solids), which are also used extensively in the microencapsulation of volatiles and non-volatiles.[Citation3] Three different pressures each with 3 cycles and 3 different times were selected for microfluidization and ultrasonication respectively. The experimental conditions are summarized in .
Table 1 Experimental parameters.
Coarse Emulsion Preparation
All emulsions used were of the oil-in-water type. The aqueous phase was a solution of maltodextrin with Hi-Cap or WPC, while the oil phase consisted of d-limonene. Hydrated solution of wall materials were prepared with distilled water one day before emulsion preparation and kept overnight in water bath to warrant a full saturation of the polymer molecules. The total concentration of dissolved solid was 40% (w/w) that was composed of 30% maltodextrin, 10% Hi-Cap or WPC, and 60% distilled water. Coarse emulsions containing different compositions of d-limonene and maltodextrin with Hi-Cap or WPC were prepared using a high-speed IKA blender (IKA Works Co, Malaysia). The d-limonene content of the emulsions was in two levels of 20 and 25% of the total existing solids ().
Microfluidization
Previously prepared coarse emulsion was passed through an air-driven microfluidizer (Model M-110 L, Microfluidics, US) operating from 20 to 124 MPa. This equipment included a pneumatic pump, a filter, and an interaction chamber. The pump could generate a pressure up to 124 MPa from the compressed air supply. This system could be operated continuously or recycled via a closed loop to have different cycles. Emulsions were homogenized at different pressure and different cycles (). The interaction chamber of the microfluidizer was cooled with tap water in order to alleviate the temperature rise. A thermometer was placed in the reserviour of the samples just after the discharge port to monitor temperature fluctuations during microfluidization. The temperature rise was from 27°C for 35 MPa after 3 cycles up to 41°C for 105 MPa after 3 cycles. For each pressure, 500 mL sample was prepared and passed through a microfluidizer at the set pressure for one cycle. Then one third of the microfluidized sample was taken away for size analysis, and the remaining volume was passing through microfluidizer again for the second cycle and the same procedure was followed for the third cycle. The experiments were duplicated.
Ultrasonication
Emulsification by sonication was performed using a 24 KHz sonicator (Dr Hielscher series, Model UP 400S). This system consisted of a generator, a converter, and a horn tip. The generator converted the conventional alternating electrical current from 60 Hz to 24 KHz. The converter transformed this energy to mechanical vibrations. The heart of the converter was a lead zirconate titanate piezoelectric quartz crystal that could expand and contract under an alternating current. The converter, therefore, vibrated and transmitted this motion to the horn tip, which was 22 mm in diameter and could vibrate at peak-to-peak amplitude of 100 µm at full power (100%). The horn tip was immersed in the coarse emulsion after the sonication was turned on to the highest power. All experiments were performed in a 500 mL glass beaker. A thermometer was placed in the side of beaker for the determination of temperature rise during sonication. The temperature rise was 32°C after 20 seconds and 39°C after 60 seconds. Each experiment was duplicated.
Emulsion Droplet Size Analysis
The size distribution of the oil droplets were determined by the laser light scattering method using Mastersizer 2000 (Malvern Instruments, UK). Two measurements were done for each sample. The system could detect particle sizes ranging from 0.02 to 2000 µm. The mean diameter of the droplets was expressed as the Sauter diameter , representing a surface average diameter).
In general, volume mean diameter ()represents the existence of big particles while surface mean diameter (D32) is associated with the smaller particles. When there is a big difference between D32 and D43, it means that the size distribution is a bimodal with two peaks and when they are close together, it shows that we have only one peak. So, by considering volume size distribution of the particles, there can be a misunderstanding of the average mean diameter especially when there are a lot of small particles and their contribution to the volume is not as much as big particles such as in our case. But if the frequency size distribution is used, it can represent large number of small particles, thus the surface mean diameter is more appropriate to consider.[Citation18]
The emulsion size was measured half an hour after emulsification by ultrasound or microfluidizer to cancel any creaming or coalescence effect. About 1 to 5 mL of the emulsion was added to 800 mL water whilst being gently stirred and recirculated in the Mastersizer cell. Particle size distribution was presented as volume percentage vs. droplet diameter. The volume size distribution was calculated from the intensity of the light diffracted at each angle using Mie theory. The analysis requires the relative refractive indices of the dispersed and continuous phases, in our case, d-limonene and water, respectively. The refractive index of d-limonene, measured using a digital refractometer, was found to be 1.40 and that for water 1.33. The width or “span” of the droplet or particle size distributions is calculated from the following formula:[Citation19]
RESULTS AND DISCUSSION
Comparison of Emulsions Produced by Microfluidization vs. Ultrasonication
In microfluidization, droplet diameter of the emulsions is a function of the emulsification pressure, temperature, and product composition. In this study, experiments have conducted to examine the dependence of emulsion droplet size on system pressure and number of cycles of processing and also emulsion composition. The results are presented in .
Table 2 Effect of microfluidization conditions on the emulsion size of d-Limonene with two different compositions of continuous phase (40% solids).
Generally, increasing the microfluidization pressure and number of cycles would result in emulsions of finer sizes. However, this is apparently not the case for results shown in and . In , the Sauter diameter (D32) before emulsification was around 10 µm and 400 nm for Hi-cap and WPC emulsions, respectively. Microfluidization reduced D32 for both emulsions, when treatment pressure was increased from 35 to 70 MPa. Above 70 MPa, D32 increased with increasing pressure.
This phenomenon, where the particle size increases with increasing the input energy, can be referred to as “over-processing” as stated by Desrumaux et al.[Citation20] This could be attributed to poorer function of the emulsifiers, and an increase in the Brownian motion, hence higher probability of collision and coalescence at higher energy input. In these conditions, the droplet size distribution of the emulsion is a result of the competition between two opposite processes, drop breakage and drop-drop coalescence. Fresh interface is created whenever a droplet is formed from breakage of an original droplet. Between its formation and its subsequent encounter with the other droplets, some surfactant will adsorb onto this fresh interface. If the timescale of collision is shorter than the timescale of adsorption, the fresh interface of the newly formed droplets will not be fully covered with surfactant and leads to coalescence. So, drop coalescence will depend on the relative rates of surfactant adsorption and drop collision. In our case, at high pressures of microfluidization, the rate of drop collision is greater than drop breakage. Since the newly formed droplets are not completely covered by the surfactant, a higher rate of collision represents a greater coalescence rate, leading to an increase in the droplet size (over-processing). This can be prevented by selecting appropriate surfactant and adjusting its concentration.
The results of the emulsions prepared by ultrasonication are summarized in and . By looking at these results, it can be explained that emulsification by ultrasound is more straight forward and without “over-processing” than with microfluidizer especially with the emulsions containing Hi-Cap. For Hi-Cap emulsions produced by ultrasound, the emulsion size is decreasing until 40 seconds of sonication, but after that the emulsion size is to some extent stable in around 500 nm.
Table 3 Effect of ultrasonication conditions on the emulsion size of d-Limonene with two different compositions of continuous phase (40% solids).
The results presented in and indicate that increased pressure and number of recirculations (cycles) in the case of microfluidization did not change the span (distribution width) of the emulsions, whereas the emulsion distribution was getting narrower and span was getting smaller with increasing the sonication time ( and ). Nevertheless, microfluidization produced narrower size distributions (less span) than ultrasound.
Figure 3 Size distribution of the emulsions produced by (a) microfluidizer for one cycle at 3 different pressures and (b) Ultrasound at the highest power for 3 different times. Emulsion was containing d-limonene (20% of total solids) as the dispersed phase and hydrated solution of Hi-Cap/MD (40% solids) as the continuous phase.
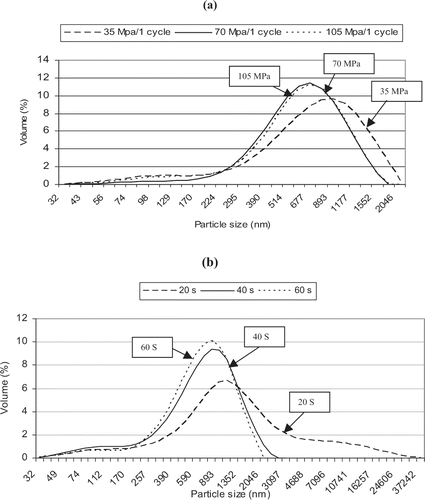
Effect of the Dispersed-phase Concentration on the Nano-emulsion Formation
From , it can be seen that reducing the amount of dispersed phase or d‐limonene leads to a decrease in the size of microfluidized emulsions especially with WPC emulsions. For example, the emulsions size of WPC composition after microfluidization at 70 MPa with 25% of d-Limonene for 2 cycles is 7407 nm, while the size for 20% d-Limonene is 125 nm. Another result that is worth noting is that emulsions of WPC with higher amounts of d-Limonene have a narrower size distribution than emulsions with lower amounts of d-limonene, while the distribution of Hi-Cap emulsion seems to be independent of the oil content.
On the other hand, with WPC emulsions produced by ultrasound, again the concentration of d-Limonene is a critical factor. For higher (> 20%) amount of d-limonene, emulsion size is over 800 nm at the beginning and decreased to about 750 nm with increasing sonication time (). For WPC emulsions with lower d-limonene (20%), the starting emulsion size was smaller than 300 nm and increased slightly with increasing sonication time. All of the WPC emulsions with 25% of d-limonene homogenized by microfluidizer had an emulsion size of more than 1 µm while the same emulsions homogenized by ultrasound have an emulsion size lower than 1 µm. Therefore, ultrasonication is more efficient than microfluidization to produce nano-emulsions of WPC with higher amount of d-limonene.
The droplet size distribution for ultrasound emulsions was bimodal. It means that coalescence was happening. Also, D43 of the WPC emulsions with 25% d-limonene by both emulsification methods, i.e., ultrasonication and microfluidization, was the same and higher than 1 micron (Data not shown). Lower D32 of the WPC emulsions with ultrasound was because of their bimodal distribution of these emulsions and skewing minor parts of the curve toward smaller sizes. In fact, there is not much difference between WPC emulsions with higher concentration of d-limonene homogenized by microfluidizer or ultrasound if considering volume-mean diameter (D43) and verifying that WPC concentration was critical in higher amount of d-limonene. But in case of lower amount of d-limonene and emulsions with Hi-Cap, there is not much difference in terms of emulsion size between ultrasonication and microfluidization. In general, sonication was more convenient regarding operation and cleaning. On the other hand, size distribution of the emulsions produced by microfluidizer was narrower than emulsions prepared by the ultrasonication (). Increasing the time of ultrasonication decreases the width of the distribution (reflected in a decrease in the span as can be seen in and ).
Emulsifying Properties of WPC vs. Hi-Cap
An additional important result from is that WPC functioned better than Hi-Cap as an emulsifier and stabiliser since the size of WPC emulsions with 20% oil is on average about 350 nm lower than Hi-Cap emulsions. This means that WPC can be adsorbed on the fresh oil-water interface better than Hi-Cap. However, WPC emulsions were very sensitive to the concentration of dispersed phase. When the oil concentration was 25%, no nano-emulsions can be produced with WPC irrespective of the process pressure and cycles.
This can be explained by the works of Tcholakova et al.[Citation21] They found that, with WPC emulsions, the dependence of D32 on the initial protein concentration consisted of two distinct regions: a significant decrease of droplet size at low surfactant concentrations and a plateau region at high surfactant concentrations. Therefore, when the d-limonene concentration was 25% of the solids, the droplet size was highly dependent on the concentration of WPC. Because it could not cover the fresh interface, droplet size can not be decreased. On the other hand, when the oil concentration was lower, there is a plateau pattern in D32, which suggests the concentration of WPC was sufficient and not a critical factor.
CONCLUSION
Microfluidization and ultrasonication were used to produce nano-emulsions. The emulsion droplet size decreased initially with increasing microfluidizer pressure and number of cycles. However, there was a critical point above which the emulsions become “over-processed.” The optimum conditions to produce nano-emulsions by microfluidizer were moderate pressure and number of passes through the equipment. In our particular case, the optimum pressure and process cycles are about 60–70 MPa with 1 to 2 cycles. A high-power ultrasound was able to produce nano-emulsions with nearly the same emulsion size as the microfluidizer, but sonication produced emulsions with wider and bimodal size distributions. Sonication was better in terms of operation and cleaning. For WPC emulsions, the concentration of the dispersed phase (d-limonene) was a critical factor. Using 20% oil, it was possible to produce a nano-emulsion of WPC with 243 nm of emulsion size after 20 seconds sonication and 125 nm emulsion size after microfluidization in 70 MPa for 2 cycles. For Hi-Cap emulsions, the average droplet size was bigger than WPC emulsions. For example, the lowest emulsion size for Hi-Cap emulsions with microfluidizer was 445 nm (35 MPa and 1 cycle) and 460 nm with sonication for 60 seconds. Finally, the size distributions of Hi-Cap emulsions were narrower than those of WPC emulsions.
REFERENCES
- Tadros , T. , Izquierdo , R. , Esquena , J. and Solans , C. 2004 . Formation and stability of nano-emulsions . Advances in Colloid and Interface Science , : 108 – 09 . 303 – 318 .
- Sonneville-Aubrun , O. , Simonnet , J.T. and L'Alloret , F. 2004 . Nanoemulsions: a new vehicle for skincare products . Advances in Colloid and Interface Science , : 108 – 09 . 145 – 149 .
- Reineccius , G.A. 1994 . “ Flavour Manufacturing ” . In Source Book of Flavours , Edited by: Reineccius , G.A. 572 – 576 . New York : Chapman and Hall .
- Maa , Y.F. and Hsu , C.C. 1999 . Performance of Sonication and microfluidization for liquid-liquid emulsification . Pharmaceutical Development and Technology , 4 ( 2 ) : 233 – 240 . [INFOTRIEVE] [CROSSREF]
- Pinnamaneni , S. , Das , N.G. and Das , S.K. 2003 . Comparison of oil-in-water emulsions manufactured by microfluidization and homogenization . Pharmazie , 58 ( 8 ) : 554 – 558 . [INFOTRIEVE]
- Robin , O. , Remillard , N. and Paquin , P. 1993 . Influence of Major Process and Formulation Parameters on Microfluidized Fat Globule Size Distribution and Example of a Practical Consequence . Colloids and Surfaces a-Physicochemical and Engineering Aspects , 80 ( 2–3 ) : 211 – 222 . [CROSSREF]
- Dalgleish , D.G. , Tosh , S.M. and West , S. 1996 . Beyond homogenization: The formation of very small emulsion droplets during the processing of milk by a Microfluidizer . Netherlands Milk and Dairy Journal , 50 ( 2 ) : 135 – 148 .
- McCrae , C.H. 1994 . Homogenization of Milk Emulsions – Use of Microfluidizer . Journal of the Society of Dairy Technology , 47 ( 1 ) : 28 – 31 .
- Strawbridge , K.B. , Ray , E. , Hallett , F.R. , Tosh , S.M. and Dalgleish , D.G. 1995 . Measurement of Particle-Size Distributions in Milk Homogenized by a Microfluidizer—Estimation of Populations of Particles with Radii Less-Than 100 Nm . Journal of Colloid and Interface Science , 171 ( 2 ) : 392 – 398 . [CROSSREF]
- Robin , O. , Kalab , M. , Britten , M. and Paquin , P. 1996 . Microfluidization of model dairy emulsions .2. Influence of composition and process factors on the protein surface concentration . Lait , 76 ( 6 ) : 551 – 570 .
- Robin , O. , Blanchot , V. , Vuillemard , J.C. and Paquin , P. 1992 . Microfluidization of Dairy Model Emulsions .1. Preparation of Emulsions and Influence of Processing and Formulation on the Size Distribution of Milk-Fat Globules . Lait , 72 ( 6 ) : 511 – 531 .
- Abismail , B. , Canselier , J.P. , Wilhelm , A.M. , Delmas , H. and Gourdon , C. 1999 . Emulsification by ultrasound: drop size distribution and stability . Ultrasonics Sonochemistry , 6 ( 1–2 ) : 75 – 83 . [INFOTRIEVE] [CROSSREF]
- Jayasooriya , S.D. , Bhandari , B.R. , Torley , P. and D'Arcy , B.R. 2004 . Effect of High Power Ultrasound Waves on Properties of Meat: A Review . International Journal of Food Properties , 7 ( 2 ) : 301 – 319 . [CROSSREF]
- Canselier , J.R. , Delmas , H. , Wilhelm , A.M. and Abismail , B. 2002 . Ultrasound emulsification – An overview . Journal of Dispersion Science and Technology , 23 ( 1–3 ) : 333 – 349 .
- Soottitantawat , A. , Yoshii , H. , Furuta , T. , Ohkawara , M. and Linko , P. 2003 . Microencapsulation by spray drying: Influence of emulsion size on the retention of volatile compounds . Journal of Food Science , 68 ( 7 ) : 2256 – 2262 .
- Risch , S.J. and Reineccius , G.A. 1988 . Spray-Dried Orange Oil—Effect of Emulsion Size on Flavour Retention and Shelf Stability . Acs Symposium Series , 370 : 67 – 77 .
- Mongenot , N. , Charrier , S. and Chalier , P. 2000 . Effect of ultrasound emulsification on cheese aroma encapsulation by carbohydrates . Journal of Agricultural and Food Chemistry , 48 ( 3 ) : 861 – 867 . [INFOTRIEVE] [CROSSREF]
- McClements , D.J. 1999 . Food Emulsions; Principles, Practice, and Techniques , 1st , CRC Press .
- Elversson , J. , Millqvist-Fureby , A. , Alderborn , G. and Elofsson , U. 2003 . Droplet and particle size relationship and shell thickness of inhalable lactose particles during spray drying . Journal of Pharmaceutical Sciences , 92 ( 4 ) : 900 – 910 . [INFOTRIEVE] [CROSSREF]
- Desrumaux , A. and Marcand , J. 2002 . Formation of sunflower oil emulsions stabilized by whey proteins with high-pressure homogenization (up to 350 MPa): effect of pressure on emulsion characteristics . International Journal of Food Science and Technology , 37 : 263 – 269 . [CROSSREF]
- Tcholakova , S. , Denkov , N.D. and Danner , T. 2004 . Role of surfactant type and concentration for the mean drop size during emulsification in turbulent flow . Langmuir , 20 : 7444 – 7458 . [INFOTRIEVE] [CROSSREF]