Abstract
The rheology of process cheese during heating and cooling was examined by measuring the transient and dynamic linear viscoelastic properties of regular fat, lower moisture and an 80% reduced-fat, higher moisture pasteurized process cheese from 10 to 50°C. The dynamic (stress and frequency sweep) and transient (creep and recovery) rheological properties of the reduced-fat process cheese were found to be higher than that of regular-fat process cheese, indicating that fat content changed rheological properties more than moisture content. The temperature-dependent frequency dispersions of storage and loss moduli (dynamic mechanical spectra) were fitted with a power-law model, and master curves (at a reference temperature of 30°C) and shift factors were obtained by shifting the temperature-dependent frequency dispersion of dynamic mechanical spectra. The relaxation spectra (moduli, viscosities and relaxation times) of both cheeses were obtained from the master curves using the generalized Maxwell model and nonlinear regression. The viscosity distribution of corresponding Maxwell model elements were higher for the reduced-fat cheese by a factor of 1.6–4.7 compared to the regular-fat cheese, indicating that the higher moisture content in the reduced-fat process cheese did not loosen the protein matrix or soften the cheese even though higher moisture is recommended to cheese manufacturers in order to compensate for some textural defects in reduced-fat cheeses.
INTRODUCTION
Cheese is increasingly used as an ingredient in prepared foods to add texture, flavor, and color. Therefore, texture and melting characteristics of cheese are the most important factors in determining quality of cheese for a particular product application,[Citation1,Citation2] consumer acceptability, and quality.[Citation3] Cheese is a viscoelastic material and can be regarded as a dispersed phase of fat globules embedded in a continuous protein matrix. The rheological properties of cheese are affected by composition and structural characteristics, and they undergo notable physical and chemical changes during aging.[Citation4]
Dynamic and transient testing are the most important and fundamental methods for determining rheological properties of viscoelastic materials, and they have been used extensively to probe the structure of cheese.[Citation5] But determining the rheological properties are complicated by structural non-homogeneity.[Citation6] Taneya et al.[Citation7] investigated the effects of maturation on the softening properties of heated natural (Gouda and Cheddar) and process cheese by measuring dynamic viscoelastic properties. Nolan et al.[Citation8] used dynamic rheological properties to distinguish between imitation and natural low-moisture, part-skim Mozzarella by measuring the viscoelastic moduli and dynamic viscosities. Tunick et al.[Citation9] used viscoelastic properties to distinguish the textural differences between Cheddar and Cheshire cheeses. Nolan et al.[Citation10] compared rheological behavior of Cheddar and pasteurized process American cheeses by studying the dynamic rheological properties. Ustunol et al.[Citation11] used the dynamic complex modulus of Cheddar cheese with varying fat content as an index of meltability, and they reported a good correlation with meltability obtained from Arnott's test. Diefes et al.[Citation12] performed dynamic tests to compare rheological properties of low-moisture, part-skim Mozzarella cheese subjected to freezing storage and refrigerated storage. Hsieh et al.[Citation13] used dynamic tests to evaluate effect of temperature on the functional properties of Mozzarella cheese containing various protein fillers. Venugopal and Muthukumarappan[Citation14] studied the dynamic rheological properties of Cheddar cheese during heating and cooling (25°C to 60°C and back) with different fat and moisture levels for up to 24 weeks of aging. They found that proteolysis during ripening of cheese led to a decrease in the storage and loss modulus at higher temperatures but the differences were not statistically significant at lower temperatures. Joshi et al.[Citation15] studied the effect of calcium, storage, and test temperature on the linear viscoelastic properties of part-skim Mozzarella cheese, and they found the elastic or storage modulus to be always higher than the viscous or loss modulus for temperatures up to 45°C.
The consumer acceptance of many prepared, ready-to-eat foods like grilled cheese sandwiches and pizzas depends on the melting quality of the cheeses used as ingredient. Past studies have mainly focused on the effect of proteolysis, chemical composition, and cheese making procedures on the meltability of cheese.[Citation16–24] Feedback from the cheese industry has indicated that temperature history during heating may also affect melting characteristics of cheeses. Wang et al.[Citation25] studied the effect of temperature on the melting characteristics of Mozzarella cheese using a modified squeezing flow apparatus. They found the biaxial elongation viscosity (BEV) decreased with increasing temperature, indicating thermal softening of Mozzarella cheese. Similar results were reported by Ak and Gunasekaran.[Citation26] Kim[Citation27] observed that, depending on the holding time, the viscosity of Cheddar cheese held at 60°C changed significantly. This was attributed to protein aggregation by hydrophobic interactions among the caseins. Kuo et al.[Citation28] studied the effect of heat treatments at 60°C for different holding times on the meltability of Cheddar cheese of different composition and a low-moisture, part-skim Mozzarella cheese at 1, 3, 6, and 12 weeks of aging using a modified squeeze flow apparatus. They found that, over all ages, the mean meltabilities of both Cheddar and Mozzarella cheeses decreased significantly as holding time increased.
Venugopal and Muthukumarappan[Citation29] examined the functionality of cheese by studying the stress relaxation characteristics of Cheddar cheese with different fat and moisture levels for up to 24 weeks of aging. Results from the stress relaxation tests (using Peleg and generalized Maxwell models) were found to be more useful in differentiating the cheese characteristics as compared to results from TPA and dynamic rheological tests. Joshi et al.[Citation30] evaluated processed cheese for instrumental textural profile analysis (TPA) using a universal testing machine and a rheometer. They attributed higher values of hardness and viscoelasticity to higher protein and lower fat content in the cheeses. Everard et al.[Citation31] correlated the meltability of different process cheese determined by sensory analysis, computer vision, and the Olson and Price test.[Citation32] They found very little evidence to show that process cheese meltability changed between 2 and 4 weeks of aging.
The objective of this study was to investigate the transient and dynamic linear viscoelastic properties of regular- and 80% reduced-fat pasteurized process cheeses during heating and cooling. Most of the literature available on cheese rheology is for natural cheeses like Mozzarella and Cheddar but there is very little literature on systematic rheological evaluation of process cheese.
MATERIALS AND METHODS
Regular- and 80% reduced-fat pasteurized process cheese were obtained from Borden Dairy Foods Ltd., Plymouth, Wisconsin, USA in vacuum-packaged plastic bags and stored at 6 to 8°C for rheological measurements. The chemical composition data for the cheeses are listed in .
Table 1 Chemical composition of pasteurized process cheese.
Dynamic and Transient Rheological Measurements
The dynamic and transient linear viscoelastic properties of regular- and 80% reduced-fat pasteurized process cheese were measured using a Bohlin CVO 50 rheometer (Bohlin Instruments Inc., New Jersey, USA) with a PP20 measuring system. In order to prevent slippage, fine sand paper was glued to the upper plate of the rheometer. Disk-shaped samples (mean thickness of 2.5 mm and diamater of 20 mm) were cut from refrigerated cheese blocks using a borer and a cutter. The sliced specimens were enclosed into plastic bags to prevent dehydration. The temperature of the lower plate of the measuring system was maintained by circulating distilled water from a constant temperature water bath. The sample was placed on the lower plate and then the upper plate was brought in contact with the sample. The exposed surface of the sample was coated with mineral oil to minimize sample drying during measurement. The sample was held for 4 to 5 minutes after loading to allow relaxation and attain temperature equilibrium. The dynamic rheological measurements were made at temperatures ranging from 10°C to 50°C, and the transient rheological measurements were made at temperatures ranging from 10°C to 40°C. The dynamic rheological data obtained included the storage or elastic modulus (G′), the loss or viscous modulus (G″) and the complex viscosity (η*). For this study, stress sweep measurements were made at a frequency of 9.43 rad/s (1.5 Hz) to determine the region of linear viscoelasticity.
The frequency sweep measurements were performed within the linear viscoelastic region over two decades of frequencies (0.1 to 10 Hz and back). The frequency sweep measurements during heating and cooling were made at a frequency of 9.43 rad/s (1.5 Hz) within the linear viscoelastic region. The samples were heated from 10°C to 40°C at the rate of 3°C per minute, held at 40°C for 30 minutes, and then cooled back to 10°C at the rate of 3°C per minute.
In creep tests, a constant shear stress (σ = 100 Pa) was applied and the resultant strain was followed/measured as a function of time. The results were expressed in terms of creep compliance (Jt ) defined as:
RESULTS AND DISCUSSION
Stress Sweep Test (Region of Linear Viscoelasticity)
The storage modulus (G′) of regular- and 80% reduced-fat pasteurized process cheeses are presented in For both cheeses, the linear region of viscoelasticity (constant G′) decreased with increasing temperature. The lowest value of about 100 Pa in shear stress (strain = 0.029 or 2.9%) was obtained for the linear region at a temperature of 50°C and an angular frequency (ω) of 9.43 rad/s (or 1.5 Hz).
Figure 1 Region of linear viscoelasticity (variation of storage with shear stress) for regular-fat and 80% reduced-fat pasteurized process cheese (ω = 9.43 rad/s or 1.5 Hz).
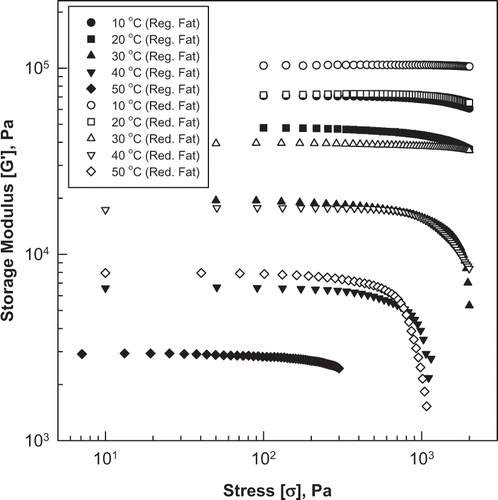
From , it can be seen that G′ decreased with increasing temperature due to thermal softening. Also, the regular-fat process cheese became softer (lower G′) compared to the 80% reduced-fat process cheese. The trends with G″ were similar to that of G′.
Frequency Sweep Test (Dynamic Viscoelastic Properties)
shows the frequency dispersion of storage modulus (G′) of both process cheeses as a function of temperature. We can see that, at any given point, G′ is greater for 80% reduced-fat process cheese compared to regular-fat process cheese despite the fact that the 80% reduced-fat process cheese had higher moisture content than the regular-fat process cheese (). It has been observed that elasticity and firmness increase with reduction in fat, and cheeses become softer with increase in bound water.[Citation33] Reduction in moisture alters the casein matrix in cheese and results in a firmer structure. Water acts as a lubricant or a plasticizer between different proteins and therefore, increasing moisture content increases textural properties like softness and makes it more meltable. It is not uncommon for cheese manufacturers to compensate for textural defects or shortcomings in reduced-fat cheeses by increasing the moisture content.
Figure 2 Frequency dispersion of storage modulus at different temperatures for regular-and 80% reduced-fat pasteurized process cheese (stress = 100 Pa).
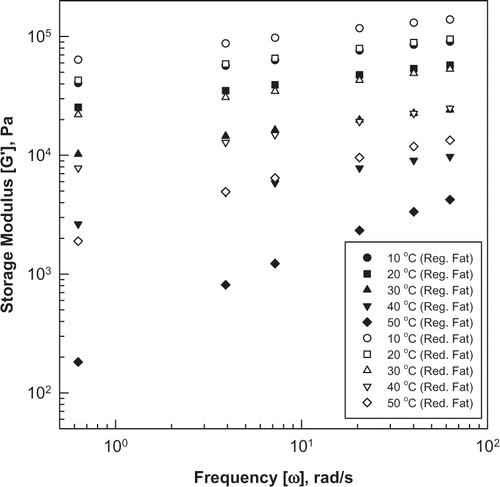
The combined effect of fat and moisture on the textural characteristics of cheese is very significant. The fat content changes the rheological properties more than moisture content, with a synergistic effect causing the differences to become more pronounced if both fat and moisture change. Subramanian and Gunasekaran[Citation34–35]studied the small amplitude oscillatory shear (SAOS) behavior of two different types of part-skim Mozzarella cheese and found that the low-fat, part-skim Mozzarella containing 8.45% fat and 53.5% moisture had higher dynamic viscoelastic properties (G′ and G″) compared to the low-moisture, part-skim Mozzarella containing 22.3% fat and 47% moisture. The experimental measurements from this study indicate a similar trend for pasteurized process cheese; the 80% reduced-fat process cheese containing 4.76% fat and 55.3% moisture has higher dynamic viscoelastic properties compared to the regular-fat process cheese containing 23.81% fat and 51.1% moisture.
shows the frequency dispersion of the loss tangent (tan δ = G″/G′) for both types of process cheese obtained from the frequency sweep measurements (). At lower temperatures, the dynamic storage or elastic modulus (G′) was greater than the dynamic loss or viscous modulus (G″) at all measured frequencies for both regular- and 80% reduced-fat process cheese. This indicates a dominant contribution of the elastic component to the viscoelasticity of cheese, a behavior typical for a viscoelastic solid.[Citation36] Similar responses have been reported by other researchers, including melted cheese.[Citation8,Citation12,Citation13,Citation37] We found that both G′ and G″ decreased with increasing temperature due to thermal softening. But G′ decreased at a faster rate than G″, and the change was more pronounced for a regular-fat process cheese at a temperature of 50°C and lower frequencies. At this temperature, the regular-fat process cheese behaved more like a viscoelastic solid (tan δ < 1) at higher frequencies but rapidly changed to a viscoelastic liquid-like behavior (tan δ > 1) at middle and lower frequencies.
Figure 3a Frequency dispersion of loss tangent at different temperatures for regular-fat pasteurized process cheese (stress = 100 Pa).
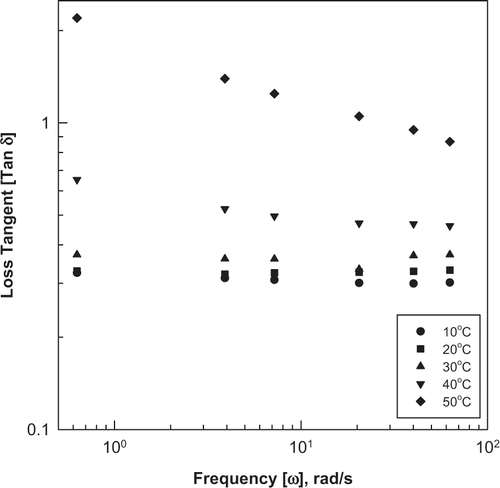
Figure 3b Frequency dispersion of loss tangent at different temperatures for 80% reduced-fat pasteurized process cheese (stress = 100 Pa).
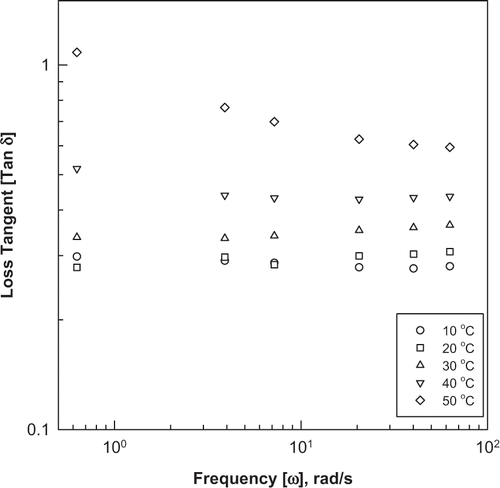
From we can see that, within the linear viscoelastic region, the frequency dispersions of the dynamic mechanical spectra (G′ and G″) of regular- and 80% reduced-fat process cheeses are more or less straight lines with different slopes. Therefore, each set of data can be fitted by a power-law equation as follows:
lists the storage and loss moduli power-law model parameters for regular- and 80% reduced-fat process cheeses as a function of temperature. We found that, at the same temperature, the low-frequency power-law model parameters for storage (or elastic) and loss (or viscous) modulus were higher for 80% reduced-fat process cheese compared to regular-fat process cheese but a comparison of power-law model indices for storage (or elastic) and loss or (viscous) modulus for both regular- and 80% reduced-fat process cheeses did not exhibit any discernable trend. The low-frequency power-law model parameters (“a” and “c”) decreased with increasing temperature, whereas the power-law model indices (“b” and “d”) increased with increasing temperature.
Table 2 Power-law model parameters for regular-fat and 80% reduced-fat pasteurized process cheese.
Creep and Recovery Test (Transient Viscoelastic Properties)
The instantaneous creep and recovery compliances (Jt ) as a function of time (t) for regular- and 80% reduced-fat pasteurized process cheeses within the linear viscoelastic region (shear stress = 100 Pa) at temperatures of 10, 20, 30 and 40°C are shown in . Both types of cheese gave a creep and recovery compliance-time curve indicative of a viscoelastic behavior. It can be seen that there is a small amount of instantaneous or retarded elasticity, and the Newtonian flow contributes significantly to the overall compliance (Jt ). Consequently, there was no full recovery even 125 s after the shearing stress was removed.
Figure 4 Creep and recovery compliance curves at different temperatures for regular- and 80% reduced-fat pasteurized process cheese (stress = 100 Pa).
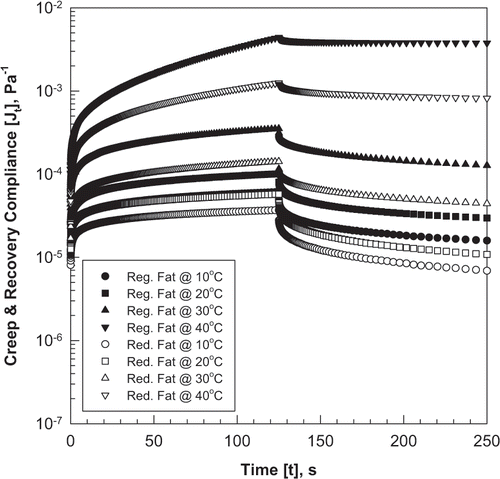
The instantaneous creep and recovery compliances of regular-fat process cheese were higher compared to that of 80% reduced-fat process cheese at the same temperature and time, again indicating increased softness. Also, the instantaneous creep and recovery compliances increased with temperature due to thermal softening. This was consistent with the measured data obtained from dynamic tests (stress and frequency sweep).
Dynamic Viscoelastic Properties During Heating and Cooling
shows the temperature dispersion of dynamic viscoelastic properties (G′ and G″) during heating and cooling for both cheeses at a frequency of 9.43 rad/s (1.5 Hz). At any given temperature, the values of storage and loss moduli were higher for the 80% reduced-fat process cheese compared to the regular-fat process cheese. This is consistent with data obtained from dynamic (stress and frequency sweeps) and transient (creep and recovery) tests. The temperature dispersion of loss or viscous modulus (G″) formed a hysteresis-type loop during heating and melting for both regular- and 80% reduced-fat process cheese, and G″ was always lower during the cooling compared to heating. The loss modulus is generally more associated with fat and moisture content in cheese, whereas the storage modulus (G′) is generally more associated with the protein network matrix structure in cheese. Unlike the loss modulus, the temperature dispersion of storage modulus did not form a hysteresis-type loop even though G′ during heating was higher than G′ during cooling for both regular- and 80% reduced-fat process cheese. Also, there was a slight drop in the value of G′ at the end of the both the heating cycle (at T = 40°C) and the cooling cycle (at T = 10°C), indicating a permanent change in the texture due to structural breakdown in the protein matrix/mineral mesh caused by the holding time between the heating and cooling cycle.
Figure 5 Temperature dispersion of dynamic viscoelastic modulus (storage and loss) during heating and cooling for regular- and 80% reduced-fat pasteurized process cheese (stress = 100 Pa, frequency = 9.43 rad/s, heating and cooling rate = 3°C/min, holding time between heating and cooling = 30 min).
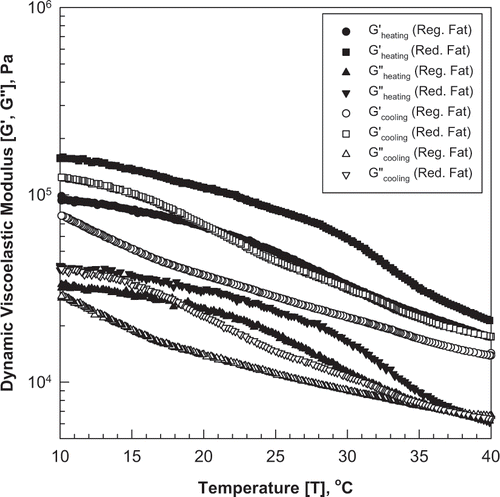
shows the temperature dispersion of the mechanical loss angle (δ) during heating and cooling of regular- and 80% reduced-fat process cheese. The loss angle was higher during cooling than during heating for both cheeses, indicating a structural breakdown in the protein matrix/mineral mesh. Also, the loss angle during heating and cooling cycles was higher for the regular-fat process cheese compared to the 80% reduced-fat process cheese. This is consistent with the loss tangent obtained from frequency sweep measurements ( ).
Master Curves from Frequency Dispersion of Dynamic Viscoelastic Properties
shows the frequency dispersion of G′ at different temperatures for both cheeses. The storage and loss moduli corresponding to various temperatures are similar, i.e., there are significant regions of overlap, and they can be superimposed to obtain a master curve using the temperature-frequency superposition principle on the basis of a standard or reference temperature.[Citation35] For this study a reference temperature (TR ) of 30°C was chosen and the dynamic moduli (G′ and G″) shifted along the frequency axis to obtain a master curve. The higher temperature data (40 and 50°C) moves towards lower frequencies on the master curve, and lower temperature data (10 and 20°C) moves towards higher frequencies on the master curve.
Applying the temperature-frequency superposition method to both cheeses, master curves were obtained as shown in . The master curves span a frequency range of about 10 decades (3 × 10−4 − 3 × 106 rad/s) compared to the experimental range of about 2 decades (0.628–62.8 rad/s). The low and high end of the master curve is not experimentally measurable because of sample slippage and noise at extremely low frequencies and physical stability of the measurement apparatus at extremely high frequencies. Note that, in the entire shifted frequency region, the 80% reduced-fat process cheese exhibits a higher frequency dispersion of storage and loss moduli compared to the regular-far process cheese (). This was because the measured frequency dispersion of storage and loss moduli (unshifted data) of the 80% reduced-fat process cheese was higher than that of the regular-fat process cheese. Also, both regular- and 80% reduced-fat process cheese exhibit a broader frequency dispersion of the storage or elastic modulus (G′) in the lower and higher frequency regions compared to the frequency dispersion of the loss or viscous modulus (G″). The cross over point (the point at which G′ = G″ or tan δ = 1) occurred at a shifted frequency of about 2.5 × 10−3 rad/s for regular-fat pasteurized process cheese, and at about 1 × 10−3 rad/s for 80% reduced-fat pasteurized process cheese. This is consistent with the fact that cheese behaves more like a viscoelastic liquid than a viscoelastic solid at higher temperatures (which corresponds to the lower frequency region on the master curve).
Figure 7 Dynamic viscoelastic modulus (storage and loss) master curves for regular- and 80% reduced-fat pasteurized process cheese (Tref = 30°C) using experimental data and generalized Maxwell model.
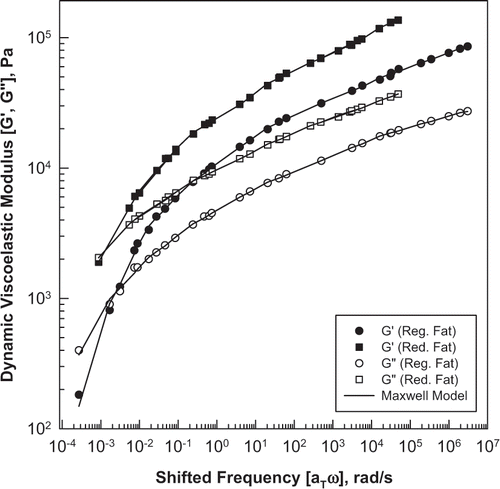
shows a plot of shift factor (aT ) as a function of temperature (1/T) obtained from the master curves for regular- and 80% reduced-fat pasteurized process cheese. At any age, the shift factor decreases linearly with increasing temperature. For any temperature (T), the shift factor (aT ) can be described by an Arrhenius type of equation as follows
Figure 8 Dynamic viscoelastic modulus (storage and loss) master curve shift factors for regular- and 80% reduced-fat pasteurized process cheese (Tref = 30°C).
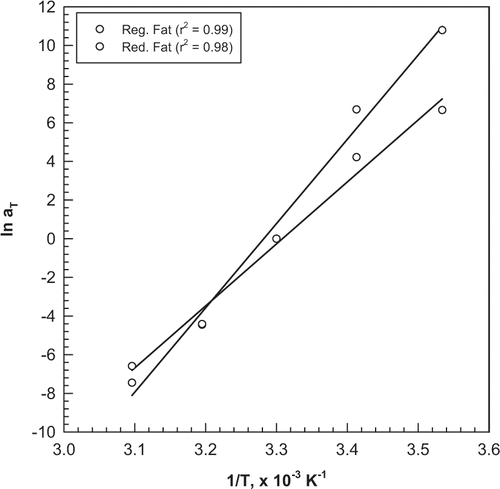
From the above equation, we can see that the slope of the straight line plot of aT vs 1/T is given by the activation energy term (−ΔEa/R). From , the activation energy terms for regular- and 80% reduced-fat pasteurized process cheese were computed to be 44102 K (r2 = 0.989) and 32045 K (r2 = 0.986), respectively. Thus, at a reference temperature of 30°C, the activation energy for regular-fat pasteurized process cheese was found to be higher than that of 80% reduced-fat pasteurized process cheese.
Relaxation Spectra from Master Curves of Dynamic Viscoelastic Properties
The response of a viscoelastic material to small deformation is related to the dynamic mechanical spectra (G′ and G″). The storage or elastic modulus (G′) and loss or viscous modulus ( G″ ) of a generalized Maxwell model are given by the following expressions:
Although this technique has been used to obtain relaxation spectrum parameters for polymer melts and solutions,[Citation38,Citation39] it has not been used extensively to study the rheological characteristics of cheeses. Subramanian and Gunasekaran[Citation35] used this technique to obtain age-dependent relaxation spectra of low-moisture and low-fat, part-skim Mozzarella cheeses from storage modulus master curves. In this study, nonlinear regression analyses, using PC-STATGRAPHICS PLUS™ package, were conducted on dynamic storage and loss moduli master curves for both regular- and 80% reduced-fat process cheese to obtain relaxation spectrum parameters for the generalized Maxwell model. The procedure was as follows:
(1) We began by selecting a set of relaxation times λk for the spectrum. The spacing of the relaxation times was conveniently chosen to be decade intervals in order to reduce the amount of computation and have greater accuracy of fitting. The following constraints were imposed:
(2) Once the relaxation time spectrum was chosen, best fit viscosities ηk were obtained for each relaxation time. This was performed by minimizing the difference between measured moduli and predicted moduli at N frequencies ωj . Care was taken to ensure that the fitted values of ηk were always positive in order to make physical sense.
shows the fit of generalized Maxwell model to the master curves for both regular- and 80% reduced-fat process cheese, and the values of the relaxation spectrum parameters are listed in . Such a behavior is also shown by polymer melts like low-density polyethylene.[Citation40] In , the low-frequency region (high temperature unshifted data) is dominated by the long relaxation times and the high-frequency region (corresponds to low temperature unshifted data) is controlled by the short relaxation times. Also, the regular-fat process cheese has lower values of relaxation time compared to 80% reduced-fat process cheese because of lower storage moduli at each temperature. Although this method is quite accurate in describing the dynamic linear viscoelastic properties (using the generalized Maxwell model) of cheese in the mid- and high-frequency range, care should be taken while using the master curve data in the low frequency region. Once the Maxwell model parameters are obtained, other linear viscoelastic properties like complex viscosity and loss modulus can be evaluated.[Citation38]
Table 3 Generalized Maxwell Model parameters of pasteurized process cheese.
A comparison of corresponding Maxwell elements of regular- and 80% reduced-fat process cheese indicated that the 80% reduced-fat process cheese indicated that the 80% reduced-fat process cheese had higher dynamic viscosity than the regular-fat process cheese. Thus, 80% reduced-fat process cheese could be described as more solid-like than regular-fat process cheese.
CONCLUSIONS
Within the linear viscoelastic region of 100 Pa stress, the synergistic effect of fat reduction and moisture enhancement on the dynamic and transient rheological properties during heating and cooling were examined for a regular- and a reduced-fat pasteurized process cheese. We found that the 80% reduced-fat pasteurized process cheese (containing 4.76% fat and 55.3% moisture) had higher dynamic and transient linear viscoelastic properties compared to the regular-fat pasteurized process cheese (containing 23.81% fat and 51.1% moisture). A reduction in fat content altered the rheological properties more than an enhancement in the moisture content. The higher moisture content of the reduced-fat process cheese did not loosen the protein matrix or soften the cheese in order to compensate for textural defects associated with reduced- and low-fat cheese due to structural changes in the protein/mineral mesh. Master curves obtained from the frequency dispersion of dynamic mechanical spectra (storage and loss moduli) were successfully modeled using the generalized Maxwell model, and parameters like Newtonian viscosity (ηk) and relaxation time (λk) were obtained at a reference temperature of 30°C. By comparing the corresponding generalized Maxwell model elements, we found that the 80% reduced-fat process cheese had values of dynamic viscosity 1.6 to 4.7 times higher than the regular-fat process cheese.
ACKNOWLEDGMENTS
The authors wish to thank the Dairy Management Inc., Wisconsin Milk Marketing Board, University of Wisconsin-Madison Center for Dairy Research, and Natural Sciences and Engineering Research Council (NSERC) of Canada for their financial support, and the University of Wisconsin-Madison's Food Science department, Chemical Engineering department and the Rheology Research Center for their cooperation.
Notes
Gupta, S.K. Changes in the chemical and physical properties of the proteins in Cheddar and Gouda cheese during aging. Ph.D. thesis, University of Illinois, Urbana-Champaign, USA, 1972.
Kim, S. Physicochemical changes occurring in Cheddar cheese during heating. Ph.D. thesis, University of Wisconsin, Madison, USA, 1999.
Luyten, H. The rheological and fracture properties of Gouda cheese. Ph.D. thesis, Wageningen Agriculture University, The Netherlands, 1988.
REFERENCES
- Park , J. , Rosenau , J.R. and Peleg , M. 1984 . Comparison of four procedures of cheese meltability evaluation . Journal of Food Science , 49 : 1158 – 1162 . 1170
- Kindstedt , P.S. 1993 . Effect of manufacturing factors, composition and proteolysis on the functional characteristics of Mozzarella cheese . Critical Reviews in Food Science and Nutrition , 33 ( 2 ) : 167 – 182 . [INFOTRIEVE]
- McEwan , J.A. , Moore , J.D. and Colwill , J.S. 1989 . The sensory characteristics of Cheddar cheese and their relationship with acceptability . Journal of Society of Dairy Technology , 4 : 112 – 117 .
- Fox , P.F. 1989 . Proteolysis during cheese manufacture and ripening . Journal of Dairy Science , 72 : 1379 – 1400 .
- Gunasekaran , S. and Ak , M.M. 2003 . Cheese Rheology and Texture , New York : CRC Press .
- Walstra , P. and Van Vliet , T. 1982 . Rheology of cheese . International Dairy Federation Bulletin , 153 : 22 – 27 .
- Taneya , S. , Izutsu , T. and Sone , T. 1979 . “ Dynamic viscoelasticity of natural and processed cheese ” . In Food Texture and Rheology , Edited by: Sherman , P. London : Academic Press .
- Nolan , E.J. , Holsinger , V.H. and Shieh , J.J. 1989 . Dynamic rheological properties of natural and imitation Mozzarella cheese . Journal of Texture Studies , 20 : 179 – 189 .
- Tunick , M.H. , Nolan , E.J. , Shieh , J.J. , Basch , J.J. , Thompson , M.P. , Maleeff , B.E. and Holsinger , V.H. 1990 . Cheddar and Cheshire cheese rheology . Journal of Dairy Science , 73 : 1671 – 1675 .
- Nolan , E.J. , Shieh , J.J. and Holsinger , V.H. 1990 . “ A comparison of some rheological properties of Cheddar and pasteurized process American cheese ” . In Engineering and Food: Physical Properties and Process Control , Edited by: Spiess , W.E.L. and Schubert , H. Vol. 1 , London : Elsevier Applied Science .
- Ustunol , Z. , Kawachi , K. and Steffe , J. 1994 . Arnott test correlates with dynamic rheological properties for determining cheddar cheese meltability . Journal Food Science , 59 : 970 – 971 .
- Diefes , H.A. , Rizvi , S.S.H. and Bartsch , J.A. 1993 . Rheological behavior of frozen and thawed low-moisture, part-skim Mozzarella cheese . Journal of Food Science , 58 : 764 – 769 .
- Hsieh , Y.L. , Yun , J.J. and Rao , M.A. 1993 . Rheological properties of Mozzarella cheese filled with dairy, egg, soy protein and gelatin . Journal of Food Science , 58 : 1001 – 1004 .
- Venugopal , V. and Muthukumarappan , K. 2003 . Rheological properties of Cheddar cheese during heating and cooling . International Journal of Food Properties , 6 : 99 – 114 . [CROSSREF]
- Joshi , N.S. , Muthukumarappan , K. and Dave , R.I. 2004 . Viscoelastic properties of part-skim Mozzarella cheese: Effect of calcium, storage, and test temperature . International Journal of Food Properties , 7 : 239 – 252 . [CROSSREF]
- Arnott , D.R. , Morris , D.A. and Combs , W.B. 1957 . Effect of certain chemical factors on the melting quality of process cheese . Journal of Dairy Science , 40 : 957 – 963 .
- Gupta, S.K. Changes in the chemical and physical properties of the proteins in Cheddar and Gouda cheese during aging. Ph.D. thesis, University of Illinois, Urbana-Champaign, USA, 1972.
- Tunick , M.H. , Malin , E.L. , Smith , P.W. and Holsinger , V.H. 1995 . Effect of skim milk homogenization on proteolysis and rheology of Mozzarella cheese . International Dairy Journal , 5 : 483 – 491 . [CROSSREF]
- Bogenrief , D.D and Olson , N.F. 1995 . Hydrolysis of β-casein increases Cheddar cheese meltability . Milchwissenchaft , 50 : 678 – 682 .
- Kindstedt , P.S. , Yun , J.J. , Barbano , D.M. and Larose , K.L. 1995 . Mozzarella cheese: Impact of coagulant concentration on chemical composition, proteolysis and functional properties . Journal of Dairy Science , 78 : 2591 – 2597 .
- Yun , J.J. , Barbano , D.M. , Larose , K.L. and Kindstedt , P.S. 1995 . Mozzarella cheese: Impact of whey pH at draining on chemical composition, proteolysis and functional properties . Journal of Dairy Science , 78 : 1 – 7 .
- Yun , J.J. , Barbano , D.M. , Kiely , L.J. and Kindstedt , P.S. 1995 . Mozzarella cheese: Impact of rod: coccus ratio on composition, proteolysis and functional properties . Journal of Dairy Science , 78 : 751 – 760 . b
- Hong , Y.H. , Yun , J.J. , Barbano , D.M. , Larose , K.L. and Kindstedt , P.S. 1998 . Mozzarella cheese: Impact of three commercial culture strains on composition, proteolysis and functional properties . Australian Journal of Dairy Technology , 53 ( 3 ) : 163 – 169 .
- Madsen , J.S. and Qvist , K.B. 1998 . Mozzarella made by ultrafiltration . Australian Journal of Dairy Technology , 53 ( 2 ) : 112
- Wang , Y.-C. , Muthukumarappan , K. , Ak , M.M. and Gunasekaran , S. 1998 . A device for evaluating melt/flow characteristics of cheeses . Journal of Texture Studies , 29 : 43 – 55 .
- Ak , M.M. and Gunasekaran , S. 1995 . Evaluating rheological properties of Mozzarella cheese by the squeezing flow method . Journal of Texture Studies , 26 : 695 – 711 .
- Kim, S. Physicochemical changes occurring in Cheddar cheese during heating. Ph.D. thesis, University of Wisconsin, Madison, USA, 1999.
- Kuo , M.-I. , Wang , Y.-C. , Gunasekaran , S. and Olson , N.F. 2001 . Effect of Heat Treatments on the Meltability of Cheeses . Journal of Dairy Science , 84 : 1937 – 1943 . [INFOTRIEVE]
- Venugopal , V. and Muthukumarappan , K. 2001 . Stress relaxation characteristics of Cheddar cheese . International Journal of Food Properties , 4 : 469 – 484 . [CROSSREF]
- Joshi , N.S. , Jhala , R.P. , Muthukumarappan , K. , Acharya , M.R. and Mistry , V.V. 2004 . Textural and rheological properties of processed cheese . International Journal of Food Properties , 7 : 519 – 530 . [CROSSREF]
- Everard , C.M. , O'Donnell , C.P. , Fagan , C.C. , Sheehan , E.M. , Delahunty , C.M. and O'Callaghan , D.J. 2005 . Correlation between process cheese meltability determined by sensory analysis, computer vision method, and Olson and Price test . International Journal of Food Properties , 8 : 267 – 275 . [CROSSREF]
- Olson , N.F. and Price , W.V. 1958 . A melting test for pasteurized process cheese spreads . Journal of Dairy Science , 41 : 999 – 1000 .
- Luyten, H. The rheological and fracture properties of Gouda cheese. Ph.D. thesis, Wageningen Agriculture University, The Netherlands, 1988.
- Subramanian , R. and Gunasekaran , S. 1997 . Small amplitude oscillatory shear studies on Mozzarella cheese. Part I. Region of Linear Viscoelasticity . Journal of Texture Studies , 28 : 633 – 642 .
- Subramanian , R. and Gunasekaran , S. 1997 . Small amplitude oscillatory shear studies on Mozzarella cheese. Part II. Relaxation Spectrum . Journal of Texture Studies , 28 : 643 – 656 .
- Rao , M.A. and Steffe , J.F. 1992 . Viscoelasticity of Foods , New York : Elsevier Applied Science .
- Ak , M.M and Gunasekaran , S. 1996 . Dynamic rheological properties of Mozzarella cheese during refrigerated storage . Journal of Food Science , 61 ( 3 ) : 566 – 569 .
- Bird , R.B. , Armstrong , R.C. and Hassager , O. 1985 . Dynamics of Polymeric Liquids , Vol. 1 , New York : Wiley-Interscience Publications .
- Papanastasiou , A.C. , Scriven , L.E. and Macosko , C.W. 1983 . An integral constitutive equation for mixed flows viscoelastic consideration . Journal of Rheology , 27 : 387 – 410 . [CROSSREF]
- Laun , H.M. 1978 . Description of the non-linear shear behaviour of a low-density polyethylene melt by means of an experimentally determined strain dependent memory function . Rheologica Acta , 17 : 1 – 15 . [CROSSREF]