Abstract
For sorptional data to be useful in simulation and design purposes, they must be represented by equations valid in the conditions usually found in industrial practice. In this regard sorptional models that include the influence of temperature on desorption and sorption equilibrium values are most valuable. In this article, water desorption and sorption isotherms of rose hip fruits (Rosa Eglanteria) were experimentally determined by the gravimetric method, and from these the isosteric heat of sorption was calculated. According to the ANOVA test carried out for this fruits, no significant differences were found between experimental desorption and adsorption isotherms. Seven models were tested to mathematically represent the moisture content as a function of water activity (aw) in the aw range of 0.11 to 0.85 and temperatures of 20, 40, and 60°C, for further use in process simulation.
The five-parameter GAB model was most accurate, with an MRE of −2,9 % and R2 = 0.989. The values obtained for the isosteric heat of sorption were fitted with a previous published equation, with an MRE% = −0.05. The isosteric heat of sorption derived from the GAB five parameters equation, for the corresponding monolayer moisture content, only differed by 1.25% with the calculated in this paper.
INTRODUCTION
Water is an essential component of most fruits and plays an important role in food preservation.[Citation1] Knowledge of the relationship between water content in a foodstuff, and the equilibrium relative humidity of the surrounding atmosphere, or food water activity is very useful to predict drying endpoint for food stability, to design new products, and to improve dehydration processes through design and optimization with adequate models.[Citation2,Citation3] Adsorption isotherms are also practical to provide information during storage handling of dehydrated products and during product reconstitution. Another key property is the isosteric heat of sorption of bound water in the food, which is higher than that for pure water and constitutes the minimum energy demand for dehydration. For this purpose equilibrium isotherms and heat of sorption curves are particularly useful when expressed as equations valid in practical conditions that can be incorporated in simulation models to improve and/or optimize dehydration processes and equipment. In this regard, models relating equilibrium moisture content or heat of sorption with water activity and temperature are especially useful.
There is a good literature reserve of equations relating water content with water activity, many of them including the influence of temperature.[Citation4,Citation5,Citation6,Citation7,Citation8,Citation9,Citation10,Citation11] However, despite various efforts, no equation is of true general use since water activity depends on food composition and of the interaction of several components with water in thermodynamic equilibrium conditions.[Citation2] That is, different foods with the same chemical composition are expected to have different equilibrium moisture contents if their physical structures are not alike. Moreover, in a given food, not all the range of water activity corresponds to sorptional mechanisms, because at very high water activity water may be held in capillaries, or exist in dilute solutions.[Citation12]
By this concept, fruits of diverse varieties kept at the same water activity may present variable moisture contents. Therefore, equilibrium data must be available even for chemically similar fruits to verify the applicability of existing equations and, eventually, to determine specific parameters for each cultivar. The objectives of this work were to obtain experimental equilibrium moistures for rose hip as a function of water activity for three temperatures, to compare the values with predictions from selected isotherm equations taken from literature sources and to calculate and model the sorption isosteric heat derived from the equilibrium data.
MATERIALS AND METHODS
Raw Material
Desorption
Fresh fruit, kept for 24 hours at 0°C in forced-air cold stores were used. According to their refractometric soluble solid content, Rose hip fruits were classified as fruit of 16 °Brix. This is a typical value for freshly harvested fruits in the Andean Patagonic Valleys at Parallel 42°, Argentina.
Adsorption
The raw material employed was the same as those used for desorption. Samples were dehydrated first at 70°C in vacuum oven until constant weight and then placed in a desiccator containing silica gel to allow the fruit to equilibrate any residual moisture gradient before being used.
Initial moisture and dry matter contents
From the classified sample, 50 fruits were cut in small pieces and mixed. Three replicates 5 g each, randomly selected, were placed in vacuum oven set at a temperature of 70°C with an absolute pressure of 10 mm Hg up to constant weight. Weights were determined using an analytical balance. Moisture and dry solids content are averages of the replicates and presented in .
Table 1 Characteristics of fruits and salts utilized to obtain atmospheres of known equilibrium relative humidity or aw.
Equilibrium isotherms
They were determined according to the gravimetric method suggested by the European Cooperative Project, COST′90.[Citation13] The experimental apparatus consisted of three natural convection ovens set to controlled temperatures of 20, 40, and 60 ± 0.5°C. In each oven, nine sealed containers were placed, each with a specific saturated salt solution that provides a constant relative humidity, as shown in . Three small subsamples of 2 g were placed in each container, being them initially wet for desorption tests and dry for adsorption experiments. The sample was obtained from a set of 50 selected fruits processed as indicated in the preceding section. Initial and successive weights were obtained in analytical balance, by removing the sample from the oven at the times recommended by COST··90. Equilibrium was considered to be attained after finding three consecutive weights within the balance error. Equilibrium moisture content was calculated by differences of the first and last weight, considering constant the dry matter content. Results reported are average of the three subsamples of each container at any of the temperatures tested.
RESULTS AND DISCUSSION
Desorption
In , experimental values obtained for the equilibrium moisture contents in rose hip are plotted as a function of water activity for the three temperatures tested during desorption. Van den Berg and Bruin[Citation14] have revised 77 equations for food isotherms, and find that some were related with the BET or GAB models while others belonged to the empirical category, with 2 or 3 parameters at constant temperature. Authors recommended the GAB equation for having parameters with physical meaning and good predictions up to aw = 0.90, and indicated that one or two of the three GAB can be temperature-dependent, through Arrhenius-type relationships. In turn, Lomauro et al.[Citation15] have used 88 experimental isotherms belonging to dairy products, coffee, tea, dry fruits, oilseeds, spices and starch-rich foods. Among other conclusions, they found that the GAB model for physical adsorption in multilayers accurately predicted some 75% of the isotherms to which it was fitted, but the experimental data were limited to a temperature of 25°C and to an aw range of 0.24–0.96. On the other hand, van den Berg[Citation16] listed a series of requirements for isotherm equations: (a) The experimental curve must be mathematically described for practical applications as drying, packaging and storage. (b) The equation must be relatively simple with a limited number of parameters. (c) The parameters must have physical meaning, (d) The parameters must be temperature-dependent. (e) The equation must allow for correction to account for hysteresis effects.
Figure 1 Experimental equilibrium moistures expressed on a dry basis (Xdb) as a function of water activity (aw) for rose hip fruits during desorption, and the predictions of the five-parameter GAB model fitted in this work.
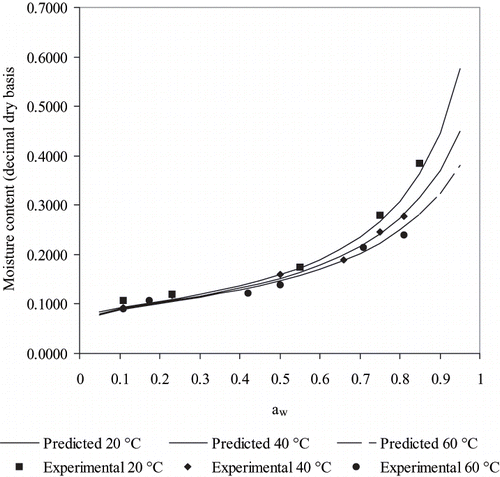
Upon these recommendations and in order to model the behavior of equilibrium isotherms, the models of: Crapiste and Rotstein;[Citation17] Three and five-parameter GAB; Ratti;[Citation8] Iglesias and Chirife;[Citation18] Strohman and Yoerger[Citation19] and Pfost et al.,[Citation28] previously used in other fruits, were tested (The corresponding mathematical expressions are presented in ). Using the non-linear regression feature of the Systat v.5.02 statistical software for Windows, the parameters fitted for the seven models applied to the data at 40 and 60°C are presented in . In addition, and to evaluate the behavior of models, an analysis of maximum, minimum and average percentage errors was carried out, together with statistical parameters of average residual and standard percentage error. These were calculated as:
Table 2 Parameters obtained for regression of experimental data for the seven models tested.
Table 3 Error analysis for obtaining parameters of the different models evaluated in with corresponding regression coefficients.
The trend of the results obtained () is similar to those from published data by other authors in other fruits.[Citation17,Citation20,Citation19,Citation21,Citation22,Citation23,Citation24] In the same figure, the equilibrium moisture is observed to increase at constant aw for decreasing temperature, as indicated by Chirife et al.[Citation25] and Giner.[Citation3] Chirife et al.[Citation25] has indicated that adsorption is favored by lower temperatures because of its exothermic nature. The equilibrium moisture content of fruits for a water activity threshold of 0.7 is particularly important because most microorganisms cannot grow in this condition. However, complete stability cannot be ensured at this water activity because other processes as enzymic and Maillard browning may be active and would require further drying or refrigerated to keep color and nutritive properties.[Citation26,Citation1] Nonetheless, setting a water activity of 0.7, safe moisture contents for microbial stability can be obtained from to be 0.235 at 20°C; 0.216 at 40°C and 0.203 decimal, dry basis at 60°C. Therefore, as safe moisture contents increase for decreasing temperature, dehydrated fruit kept in refrigerated storage would require less dehydration for microbial stability (For instance, using the selected model at 10°C for a water activity of 0.7 gives a safe moisture content of 0.247 decimal, dry basis). This analysis shows the benefits of refrigerated storage for dehydrated foods, which is interesting when considering advantages and disadvantages of specific facilities for packaging and storing dehydrated foods.[Citation3]
Adsorption
presents experimental equilibrium moisture contents obtained by adsorption for rose hip as a function of water activity at the three temperatures tested. Values so obtained are observed to be similar to those measured by desorption and, for this reason, an statistical analysis of variance (ANOVA) was carried out at a significance level of 5%. Values for desorption and adsorption were not found to be significantly different as can be seen in . On these grounds, the same model (five-parameter GAB) with the same parameters can describe equilibrium adsorption and desorption isotherms. Other authors have already stated that hysteresis may not appear in equilibrium isotherms for all foods.[Citation27,Citation28,Citation5,Citation29,Citation30] Iglesias and Chirife[Citation31] have published a thorough revision of equilibrium isotherms, and reported a marked hysteresis effect in various products such as apple, dextrin, pistachio, and sugar. Moreover, differences of equlibrium moisture contents caused by hysteresis in one research can be as considerable as the differences between the isotherms reported by various authors, as it is the case in coffee, colagen, figs, fish protein, peanut, milk, mushroom, and pears. Various hypothesis have been formulated to explain the hysteresis phenomenon. Labuza[Citation32] has attributed it to sugar supersaturation, while Chinachoti and Steimberg[Citation33] to the crystalline state of sugars. Tsami et al.[Citation24] considered that hysteresis is caused by the change in composition of the product during storage and/or dehydration. However, as the sorption isotherms of some products having soluble solids as pear and figs do not show any considerable hysteresis, another hypothesis can be proposed,[Citation30] which is related to non-equilibrium conditions of water inside the foods. Water diffusivity in foods is often lower than 5 × 10−9 m2/s, so either for samples losing moisture through the surface (dehydration) or adsorbing through it (rehydration), steep moisture gradients are established inside the food so equilibrium moisture can be different to that obtained in totally equilibrated samples, i.e., in samples with flat internal gradients. This may suggest that hysteresis may or may not appear in the same sample. Furthermore, in samples where the hysteresis effect is moderate, it can be masked by the experimental error of the method used. To this end, to minimize errors, samples used in the adsorption tests were allowed to reach equilibrium in a closed container for 24 hours. From the point of view of the product stability Fennema[Citation1] proposes that in the presence of hysteresis the water activity ensuring microbial stability for the adsorption branch, of lower moisture content, would be not guarantee stability for the desorption branch, of higher moisture content, by molecular mobility considerations. Therefore, if a safe moisture content is calculated with the desorption branch of the isotherm, the water activity threshold used must be lower than that for adsorption.
Figure 2 Experimental equilibrium moisture contents, expressed on a dry basis (Xdb) as function of water activity, in rose hip fruits during adsorption and predictions of the five-parameter GAB model fitted in this work for desorption.
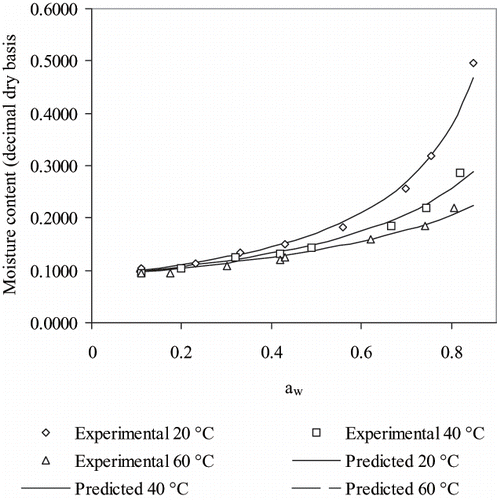
Table 4 Statistical analysis on experimental values of equilibrium moisture contents arrived at by desorption and adsorption.
Isosteric Heat of Sorption
The isosteric heat of sorption Q, is the energy per unit mass required to remove water from a material and incorporates the binding energy between water molecules and the food dry matter.[Citation34] Therefore, it is logically related to the amount of energy required in a dehydration process. The net isosteric heat of sorption q represents the difference of the heat of sorption Q and that for pure water evaporation Lw, and can be evaluated by means of the Clausius-Clapeyron equation, in method sometimes called Clausius-Clapeyron — sorption isotherm method,[Citation3] which is expressed by:[Citation34,Citation35,Citation36]
The derivative placed in the left hand side of EquationEq. (3) can be evaluated with a sorption isotherm model used to calculate q. Otherwise, if mathematical manipulations are complicated, or a model is not available, the value of q can be obtained from the slope of the ln aw vs 1/T plot. EquationEq. (3) is based on the assumption that q is independent of temperature, despite it is not always true it is often accepted as such.[Citation34,Citation37] For this last method, the experimental sorption curve must be available at least for three temperatures. Based on the integrated form of EquationEq. (3), Mulet et al.[Citation36] used an expression by Riedel[Citation38] relating aw with temperature and proposed a very simple expression to obtain q:
Being R the gas constant (8.314 kJ/kmol K), aw1 and aw2 water activities evaluated at temperatures T1 and T2 , respectively. A and b are regression parameters from EquationEq. (5). By combining EquationEqs. (4) and Equation(5), Mulet et.al.[Citation36] have obtained:
Where D = A R. Therefore, q is calculated at different moisture contents by repeated application of the ln aw vs 1/T plot. Then, by further fitting of EquationEq. (6) to the data of Q vs Xbs, the parameters D and b are found. In , Q values, obtained from the equlibrium curves of moisture dry basis vs aw (observed values), are plotted together with predictions by EquationEq. (6) using the parameters fitted in this work. Values of the parameters obtained in this work are: D = 799.56 kJ/kg and b = 7.662 kg dry solids/kg water. Maximum and minimum errors % were 1.31 and – 2.47, respectively, the mean relative error being, MRE % = −0.05.
Figure 3 Isosteric heat of sorption as a function of the moisture content for rose hip fruits, and predictions by Eq. (13).
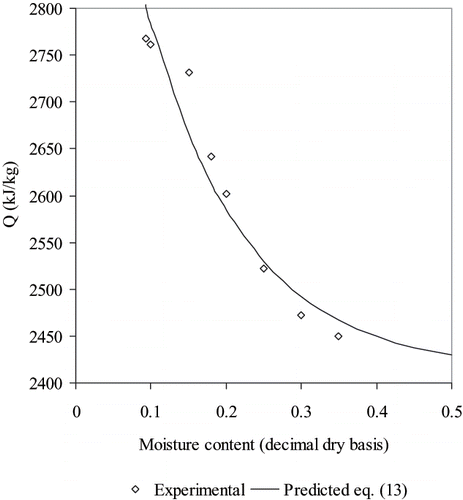
In analysis carried out by Giner (3) and Mulet et.al.,[Citation34] maximum values of Q are reported to be between 1.14 to 1.20 times the heat of vaporization of pure water. In this work, for the value obtained for the minimum moisture measured Xdb = 0.093, the ratio was 1.147, close to the conclusions by the previous authors. Moreover, the monolayer moisture content obtained by the five-parameter GAB model was of 0.0887 kg water/kg dry solid, and, from the same model, the monolayer heat of sorption can be obtained using the correlations for C and K from the five-parameter GAB model (), where:
While Hm and Hn are the monolayer and multilayer heats of sorption, respectively. If both expressions are combined in EquationEq. (7) by solving through the common parameter, Hn (33), the following is obtained:
By replacing the values from the fitting ΔHc and ΔHk (C3 and C5 in ), and that for Lw in EquationEq. (8), Hm is found to be 2855.6 kJ/kg. In turn, from EquationEq. (6) used at the monolayer moisture, a value of 2820.3 kJ/kg is obtained which is within 1,25 % from that obtained by EquationEq. (8).
CONCLUSIONS
Equilibrium moisture contents were experimentally determined during water desorption and adsorption in rose hip fruits at 20, 40, and 60°C. By comparing adsorption and desorption values under the experimental conditions assessed here, no statistically significant hysteresis phenomenon was detected. For this reason, experimental values for adsorption and desorption were fitted with the same seven previously published models. Among the predictive models tested, the five-parameter GAB model was selected based on its best statistical parameters of goodness of fit for the prediction of experimental data of sorption in rose hip. The selected model mathematically represents experimental equilibrium moistures as a function of water activity and represented adequately effect of temperature in the range studied. Predictions at a temperature lower than used in the fitting gave an acceptable agreement as well. The experimental values mentioned above were used to calculate the isosteric heat of sorption for rose hip fruits. Values obtained were interpreted with a simple, previously published model, giving a satisfactory correlation. Moreover, the heat corresponding to the monolayer moisture content calculated in this work only differed by 1.25% from that obtained using the GAB model in the same conditions. Values of the heat of sorption to heat of vaporization obtained here are in agreement with published values for different fruits.
Notes
3. Giner, S.A. Diseño de secadoras continuas de trigo. Simulación de la transferencia de calor y materia y de perdidas de calidad. Tesis Doctoral. Departamento de Química e Ingeniería Química, Facultad de Ingeniería, Universidad Nacional de La Plata, La Plata, Argentina, 1999, Cap. 4; 1–39
8. Ratti, C. Diseño de Secaderos de Productos Frutihortícolas. Tesis Doctoral. Departamento de Química e Ingeniería Química. Planta Piloto de Ingeniería Química, Universidad Nacional del Sur, Bahía Blanca, Argentina, 1991; 27–57
20. Pfost, H.B.; Maurer, S.G.; Chung, D.S.; Milliken, G.A. Summarizing and Reporting Equilibrium Moisture Data for Grains, ASAE paper No. 76–3520. St. Joseph, Michigan, 1976
25. Chirife, J.; Resnik, S.; Suarez, C. Fundamentos de secado y almacenaje de granos. Curso de postgrado. Departamento de Industrias. Facultad de Ciencias Exactas y Naturales. Universidad de Buenos Aires – Argentina, 1985
REFERENCES
- Fennema , O.E. 2000 . Química de los alimentos , 2nd , Edited by: Acribia , S.A. 19 – 109 . Zaragoza, , España : Tipo Línea .
- Welti-Chanes , J. and Vergara-B , F. 1997 . “ Actividad de agua. Concepto y aplicación en alimentos con alto contenido de humedad ” . In Temas en tecnología de alimentos , 11 – 43 . Aguilera, México : J.M. Instituto Politécnico Nacional . Programa Iberoamericano de ciencia y tecnología para el desarrollo: CYTED, Ed.
- 3. Giner, S.A. Diseño de secadoras continuas de trigo. Simulación de la transferencia de calor y materia y de perdidas de calidad. Tesis Doctoral. Departamento de Química e Ingeniería Química, Facultad de Ingeniería, Universidad Nacional de La Plata, La Plata, Argentina, 1999, Cap. 4; 1–39
- Brunauer , S. , Emmet , P.H. and Teller , E. 1938 . Adsorption of Gases in Multimolecular Layers . J. Am. Chem. Soc , 60 : 309 – 315 .
- Chirife , J. , Iglesias , H.A. and Boquet , R. 1977 . Some Characteristics of the Heat of Water Vapor Sorption in Dried Foodstuffs . J. Food Tech , 12 : 605 – 611 .
- Henderson , S.M. 1952 . A Basic Concept of Equilibrium Moisture . Agric. Eng , 33 : 29 – 37 .
- Leung , H.K. 1986 . Water Activity and Other Colligative Properties of Foods , Edited by: Okos , M. R. 95 – 109 . Delhi, MI : A. S. A. E. .
- 8. Ratti, C. Diseño de Secaderos de Productos Frutihortícolas. Tesis Doctoral. Departamento de Química e Ingeniería Química. Planta Piloto de Ingeniería Química, Universidad Nacional del Sur, Bahía Blanca, Argentina, 1991; 27–57
- Rizvi , S.S.H. 1986 . “ Thermodynamic Properties of Foods in Dehydration ” . In Engineering Properties of Foods , Edited by: Rao , M.A. and Rizvi , S.S.H. 133 – 214 . New York : Marcel Dekker, Inc. .
- Thompson , T.L. , Peart , R.M. and Foster , G.H. 1968 . Mathematical Simulation of Corn Drying . A New Model. T. ASAE , 24 ( 3 ) : 582 – 588 .
- Toledo , R.T. 1991 . Fundamentals of Food Process Engineering , 2nd , New York : VanNostrand Reinhold .
- Crapiste , G.H. and Rotstein , E. 1997 . “ Design and Performance Evaluation of Dryers ” . In Handbook of Food Engineering Practice , Edited by: Valentas , K.J. , Rotstein , E. and Singh , R.P. 125 – 165 . Boca Raton and New York : CRC Press . Cap. 4
- Spiess , W.E.L. and Wolf , W. 1983 . “ The Results of the COST··90 Project on Water Activity ” . In Physical Properties of Foods , Edited by: Jowitt , R. 65 London : Applied Science Publishers .
- van Den Berg , C. and Bruin , S. 1981 . “ Water Activity and its Estimation in Foods System ” . In Water Activity: Influences on Food Quality , Edited by: Rockland , L.B. and Stewart , G. F. 1 – 61 . New York : Academic Press .
- Lomauro , C.J. , Bakshi , A.S. and Labuza , T.P. 1985 . Evaluation of Food Moisture Sorption Isotherm Equation. Part II: Milk, Coffee, Tea, Nuts, Oilseeds, Spices, and Starchy Foods . Lebensm-Wiss. U-Technol , 18 : 118 – 124 .
- van Den Berg , C. 1986 . “ Water Activity ” . In Concentration and Drying of Foods , Edited by: MacCarthy , D. 11 – 61 . London : Elsevier Applied Sciences Publisher .
- Crapiste , G.H. and Rostein , E. 1986 . “ Sorptional Equilibrium at Changing Moisture Contents ” . In Drying of solids , Edited by: Mujumdar , A. S. Wiley Elastern Ltd .
- Iglesias , H.A. and Chirife , J. 1976 . Handbook of Food Isotherms , 1 – 319 . New York : Academic Press .
- Strohman , R.D. and Yoerger , R.R. 1967 . A New Equilibrium Moisture-Content Equation . T. ASAE , 10 : 675 – 681 .
- 20. Pfost, H.B.; Maurer, S.G.; Chung, D.S.; Milliken, G.A. Summarizing and Reporting Equilibrium Moisture Data for Grains, ASAE paper No. 76–3520. St. Joseph, Michigan, 1976
- Krokida , M.K. , Kiranoudis , C.T. , Maroulis , Z.B. and Marinos-Kouris , D. 2000 . Drying Related Properties of Apple . Dry. Technol , 18 ( 6 ) : 1251 – 1267 .
- Veltchev , Z.N. and Menkov , N.D. 2000 . Desorption Isotherms of Apples at Several temperatures . Dry. Technol , 18 ( 4,5 ) : 1127 – 1137 .
- Roman , G.N. , Urbicain , M.J and Rotstein , E. 1982 . Moisture Equilibrium in Apples at Several Temperatures. Experimental Data and Theoretical Considerations . J. Food Sci , 47 : 1484 – 1488 . 1507
- Tsami , E. , Marinos-Kouris , D. and Maroulis , Z.B. 1990 . Water Sorption Isotherms of Raisins, Currants, Figs, Prunes and Apricots . J. Food Sci , 55 ( 6 ) : 1594 – 1597 . 1625
- 25. Chirife, J.; Resnik, S.; Suarez, C. Fundamentos de secado y almacenaje de granos. Curso de postgrado. Departamento de Industrias. Facultad de Ciencias Exactas y Naturales. Universidad de Buenos Aires – Argentina, 1985
- Chirife , J. and Iglesias , H.A. 1984 . “ Consideraciones Sobre la Cinética de Secado de Granos y las Humedades de Equilibrio durante el Almacenaje ” . In La refrigeración como medio para disminuir las pérdidas post-cosecha , 5 – 61 . Buenos Aires : PNITA-SECyT and OEA . Tomo II
- Ross , K.D. 1975 . Estimation of Water Activity in Intermediate Moisture Foods . Food Tech , 3 : 26 – 34 .
- Norrish , R.S. 1966 . An Equation for the Activity Coefficients and Equilibrium Relative Humidities of Water in Confectionery Syrups . J. Food Tech , 1 : 25 – 39 .
- Vega-Mercado , H. , Romanach , B. and Barbosa-Cánovas , G.V. 1994 . Prediction of Water Activity in Food Systems. A Computer Program for Predicting Water Activity in Multicomponent Foods . Rev. Esp. Cienc. Tecnol. Aliment , 34 ( 4 ) : 427 – 440 .
- Herman , E. , De la Cruz , J. and García , M.A. 1999 . Prediction of Pineaple Sorption Isotherms Using the Ross Equation . Dry. Technol , 17 ( 4,5 ) : 915 – 923 .
- Iglesias , H.A. and Chirife , J. 1982 . Handbook of Food Isotherms: Water Sorption Parameters for Food and Food Components. Food Science and Technology a Series of Monographs , 125 – 132 . New York : Academic Press .
- Labuza , T.P. 1984 . “ Moisture Sorption: Practical Aspects of Isotherm Measurement and Use ” . St. Paul, MN : Am. Assoc. Cereal Chemists .
- Chinachoti , P. and Steimberg , M.P. 1986 . Moisture Hysteresis is Due to Amorphous Sugar . J. Food Sci , 53 ( 4 ) : 1150 – 1155 .
- Mulet , A. , García-Pascual , P. , Sanjuán , N. and García-reverter , J. 2002 . Equilibrium Isotherms and Isosteric Heats of Morel (Morchella esculenta) . J. Food Eng , 53 : 75 – 81 .
- Sánchez , E.S. , Sanjuán , N. , Simal , S. and Rosselló , C. 1997 . Calorimetric Techniques Applied to the Determination of Isosteric Heat of Desorption for Potato . J. Sci. Food Agric , 74 : 57 – 63 .
- Mulet , A. , García-reverter , J. , Sanjuán , N. and Bon , J. 1999 . Sorption Isosteric Heat Determination by Thermal Analysis and Sorption Isotherms . J. Food Sci , 64 ( 1 ) : 64 – 68 .
- Iglesias , H.A. , Chirife , J. and Ferro Fontán , C. 1989 . On the Temperature Dependence of Isosteric Heats of Water Sorption in Dehydrated Foods . J. food Sci , 54 ( 6 ) : 1620 – 1631 .
- Riedel , L. 1997 . Calorimetric Measurement of Heat of Hydration of Foods . Chemie Mikrob U-Technologie Lebens , 5 : 97 – 101 .