Abstract
Whey protein concentrate, whey protein isolate, and nonfat dry milk may be processed through a twin-screw extruder to produce ingredients for protein-fortified food. The products ranged from rigid to flexible to soft, and small amplitude oscillatory shear measurements showed that these properties varied with extrusion temperature and moisture content. The whey proteins showed different effects than nonfat dry milk due to the type and amount of protein present. The characteristics of extruded milk powders can be manipulated through processing parameters to obtain texturized products with the desired rheological properties.
INTRODUCTION
Whey proteins include all of the essential amino acids, and are easily digested. Whey protein concentrates (WPC) containing around 80% protein are designed to compete with other protein ingredients in food, such as egg white proteins.[Citation1] Whey protein isolate (WPI), which contains about 90% protein and is clear in solution, is used in nutritional supplements and protein-fortified beverages.[Citation1] Both WPC and WPI function as emulsification, gelation, and foam formation agents, and are used to alter solubility, viscosity, and water holding capacity of food.[Citation2] They are also being used in applications such as sports nutrition[Citation3] appetite suppression,[Citation4] and a derivatization procedure for producing a cold gelling WPI, which will affect foaming and emulsifying characteristics of foods to which it is added, has been developed.[Citation5,Citation6] The two major whey proteins, β-lactoglobulin and α-lactalbumin, are responsible for much of the functionality of the food to which they are added.[Citation1] This functionality is governed by composition, degree of hydration, intermolecular interactions, and other factors, which in turn are influenced by concentration and temperature.[Citation2]
Proteins may be texturized with applied heat in twin-screw extruders, which use two intermeshing screws rotating in tandem to convey, knead, and shear the product.[Citation7] Extrusion processing at elevated temperatures modifies the conformational structure of proteins through partial denaturation, which exposes amino acids and peptides that are normally concealed in the native protein.[Citation8] Whey contains two major proteins, β-lactoglobulin at a concentration of 50–70% with a denaturation temperature of 78°C, and α-lactalbumin at a concentration of 15–25% with a denaturation temperature of 62°C.[Citation9,Citation10] Globular proteins such as β-lactoglobulin and α-lactalbumin are also susceptible to unfolding and denaturation because of shear, an effect that has been observed by Onwulata et al.[Citation11] in an extruder at temperatures as low as 50°C, increasing by an order of magnitude every 25°C. The resulting changes in the characteristics of the proteins, which may be characterized by rheological measurements, create the possibility for their use in a wide range of products such as high-protein snacks and meat replacers. Food processors would be able to take advantage of the differences in the physical properties of extruded whey protein to obtain products with more desirable functional properties.
Extruding whey protein solutions with corn and soy to obtain a product with a more desirable combination of amino acids was first attempted by Aguilera and Kosikowski.[Citation12] Extrusion of corn flour and whey powder was performed by Cuddy and Zall to improve baking characteristics of bread, cookies, and muffins.[Citation13] More recently, the Dairy Processing & Products Research Unit, USDA, has co-extruded whey with corn, potato, and rice flours,[Citation14,Citation15] and corn meal and wheat fiber[Citation16] to increase the protein content of snacks. Research has turned toward using texturized whey protein alone, at 25% by weight, to modify food.[Citation11] The aim of this portion of our research is to determine how the rheological behavior of extruded whey proteins is affected by temperature and moisture when compared with extruded nonfat dry milk (NDM).
MATERIALS AND METHODS
NDM was obtained from Land O'Lakes (Carlisle, PA). WPC (WPC-80) was purchased from Davisco Foods International, Inc. (Le Sueur, MN). WPI (Provon 190) was purchased from Glanbia Ingredients, Inc. (Monroe, WI). All of these powders were manufactured using membrane technology, leaving around 80% of the proteins in their native, undenatured state. The percentages of components of the starting materials, according to the manufacturers' specifications, are shown in .
Table 1 Composition (g/100 g sample) of nonfat dry milk (NDM), whey protein concentrate (WPC), and whey protein isolate (WPI), from manufacturers' specifications.
The powder was extruded in a ZSK30 twin-screw extruder (Krupp, Werner & Pfleiderer Co., Ramsey, NJ) consisting of 9 barrel zones, each with individual temperature control. The screw configuration has been described previously.[Citation12,Citation14] The die was fitted with two circular inserts of 3.18 mm diameter each. Feed was conveyed into the extruder with a series 6300 digital type 35 twin screw volumetric feeder (K-tron Corp., Pitman, NJ) at a constant setting of 600 rpm, yielding a feed rate of 78 g/min. Water was added in zone 3 with an electromagnetic dosing pump (Milton Roy, Acton, MA) at the settings shown in . NDM was extruded at pump settings of 30, 40, 50, 60, and 70. WPC and WPI were extruded at pump settings of 20, 30, 40, 50, and 60. Product was extruded at 50, 75, or 100°C, as measured at zone 9. Samples were collected after 25 minutes of processing and stored at 4°C until analyzed. Duplicate experiments were performed.
Table 2 Addition of water during extrusion process.
The moisture content of the extruded products was determined in triplicate by AOAC Method 925.10.[Citation17] Water solubility index (WSI), which is unitless, was determined in triplicate by the method of Anderson et al.[Citation18] Small amplitude oscillatory shear analyses were conducted in triplicate at room temperature using an AR-2000 rheometer (TA Instruments, New Castle, DE) with parallel aluminum plates. Specimens were cut into disks measuring 25 mm in diameter and 2.5–4.0 mm thick. Strain sweeps were performed to determine the linear viscoelastic range of the sample, which was always between 0.2 and 0.8% strain. Frequency sweeps were then run from 1 to 100 rad/s. Values for elastic or storage modulus (G′) and viscous or loss modulus (G″) were obtained at 10 rad/s. Loss modulus, the unitless ratio of G″ to G′, was also calculated. Pooled standard deviations (averages of the standard deviations of experimental groups) and linear regressions were calculated by the Statistical Analysis System package (SAS Institute, Inc., Cary, NC).
RESULTS AND DISCUSSION
Moisture
Within each extrusion temperature, the moisture content of the product increased with the water pump setting (). The differences in moisture exhibited between the lower and higher pump settings far exceeded the standard deviations. Extruded NDM exhibited the narrowest moisture range (36.9–58.9%), followed by WPC (30.9–59.5%), and WPI (34.4–67.6%). Previous investigations about water sorption of milk powders showed that water is preferentially bound by casein and whey proteins, with lactose being relatively nonhygroscopic.[Citation19–21] NDM powder (which contains 32% protein and 52% lactose) would be expected to adsorb < 0.3 g water/g dry material,[Citation20] whereas WPC and WPI powders (which contain at least 77% protein and no more than 7% lactose) would be expected to adsorb > 1.0 g water/g dry material.[Citation21] The NDM therefore absorbed less water at each pump setting than either WPC or WPI, and the extruded NDM products exhibited the smallest range of moisture content. The moisture levels of the extruded NDM samples decreased as the extrusion temperature increased from 50 to 75°C. On the other hand, the levels increased when the temperature was raised from 75 to 100°C, but the differences were never greater than 6.5 percentage points. The WSI of the extruded NDM was always within a range between 15.1 and 17.2, and was unaffected by extrusion temperature or pump setting ().
Table 3 Grams of moisture per 100 g sample in extruded nonfat dry milk (NDM), whey protein concentrate (WPC), and whey protein isolate (WPI) samples, with pooled standard deviations (PSD).
Table 4 Water solubility index values for extruded nonfat dry milk (NDM), whey protein concentrate (WPC), and whey protein isolate (WPI) samples, with pooled standard deviations (PSD).
The water binding capacity of native milk proteins decreases with increasing temperature;[Citation2] this effect has also been observed with heat- and-shear-treated WPC.[Citation20] Heat denaturation and shear denaturation of β-lactoglobulin and α-lactalbumin unfold the protein, causing hydrophobic amino acid residues to be exposed[Citation7] and aggregates of protein to be formed from linkages of disulfide and sulfhydryl groups.[Citation22,Citation23] It is also possible that the proteins assume a molten globule structure,[Citation24] an intermediate stage that is characterized by a compact structure and an elevated degree of hydration.[Citation25] Within each pump setting, the moisture content of the extruded WPI samples decreased (beyond the standard deviation) as the extrusion temperature increased () because of the decrease in water adsorption of the powder inside the extruder.[Citation22] The moisture content of the WPC samples decreased or exhibited slight (within the standard deviation) increases as the extrusion temperature increased; there was an obvious increase in moisture content with temperature at the pump setting of 60. Lactose stabilizes whey proteins against heat denaturation,[Citation24] an effect that increases with lactose concentration.[Citation22,Citation26] WPC, which contains 7% lactose, was therefore less heat susceptible than WPI, which contains < 2% lactose. The extruded WPI thus contained less moisture when the extrusion temperature was raised but the moisture content of the WPC did not change significantly.
When the extruded WPC and WPI products were placed in water, the WSI increased (beyond the standard deviation) with extrusion temperature for each pump setting (), and tended to be inversely proportional to moisture content. Once the whey protein products were out of the extruder, their reduced moisture content allowed them to undergo more water uptake, with this effect becoming more pronounced as the extrusion temperature increased.
Elastic Modulus
Dynamic rheological measurements, which are performed under small amplitude oscillatory conditions, are often used to examine short-range interactions in proteins.[Citation27] The NDM sample extruded at 75°C and a water pump setting of 30 contained 36.9% moisture and exhibited a G′ value of 228.6 kPa, indicating a strong rigid internal structure. Three samples contained 40–44% moisture and had G′ values between 42 and 49 kPa. The other eleven samples contained 48–59% moisture and displayed G′ values between 11.4 and 40.1 kPa, indicative of a rather flexible internal structure. and show the dependence of G′ on moisture content of extruded NDM. Casein, which comprises about 80% of the protein in NDM, has a random coil structure and does not denature below 100°C. The G′ values of the 100°C samples were relatively constant across the range of pump settings, and did not display a significant correlation with moisture. The extruded NDM samples remained solid throughout the temperature and moisture ranges, with differences being less than the standard deviation.
Figure 1 Relationships between percent moisture and log of the elastic modulus (log G′) in nonfat dry milk samples extruded at 50°C (circles, solid line), 75°C (squares, dashed line), and 100°C (triangles, broken line). R2 values for the 50, 75, and 100 °C linear regression lines are 0.77, 0.88, and 0.41, respectively.
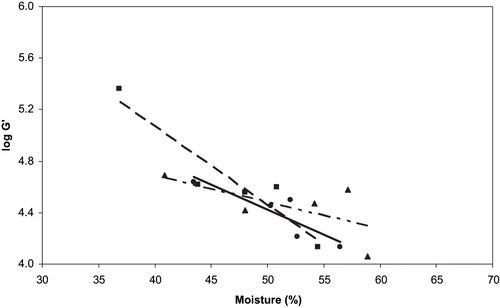
Figure 2 Relationships between log of the elastic modulus (log G′) and extrusion temperature of extruded nonfat dry milk samples, normalized to 50% moisture, with standard deviation bars.
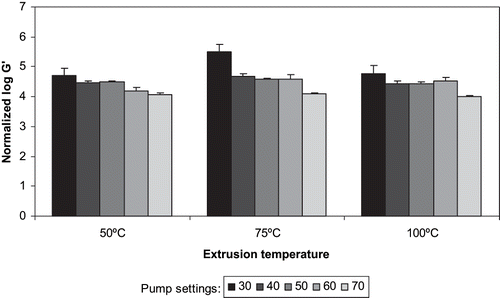
At 50 and 75°C, the G′ values of extruded WPC and WPI decreased as the moisture content and the pump setting increased (). The samples were transformed from sticky to soft to fluid with increased moisture. The most pronounced changes were found in the WPC extruded at 50°C, where the G′ values dropped from 710 kPa to 14 Pa as the moisture content increased from 35 to 54% (), and the pump setting increased from 20 to 60 (). The changes in G′ reflect heat- and shear-induced denaturation taking place in these samples, leading to the formation of an aggregate protein structure,[Citation22,Citation23] a molten globule structure,[Citation24] or a combination of both. The moduli fluctuated at 100°C ( and ), where all of the whey protein was denatured by heat and shear, and all of the samples were solid. The values for G″ displayed the same trends for all samples and temperatures.
Figure 3 Relationships between percent moisture and log of the elastic modulus (log G′) in whey protein concentrate samples extruded at 50°C (circles, solid line), 75°C (squares, dashed line), and 100°C (triangles, broken line). R2 values for the 50, 75, and 100°C linear regression lines are 0.86, 0.81, and 0.20, respectively.
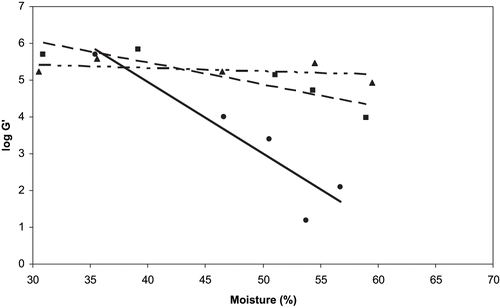
Figure 4 Relationships between log of the elastic modulus (log G′) and extrusion temperature of extruded whey protein concentrate samples, normalized to 50% moisture, with standard deviation bars.
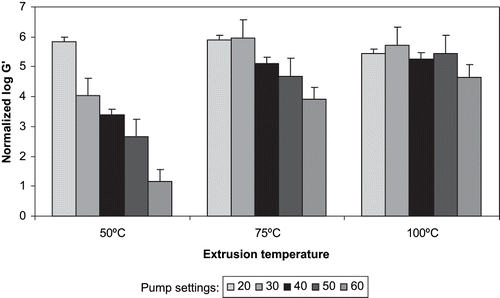
Figure 5 Relationships between percent moisture and log of the elastic modulus (log G′) in whey protein isolate samples extruded at 50°C (circles, solid line), 75°C (squares, dashed line), and 100°C (triangles, broken line). R2 values for the 50, 75, and 100°C linear regression lines are 0.76, 0.92, and 0.70, respectively.
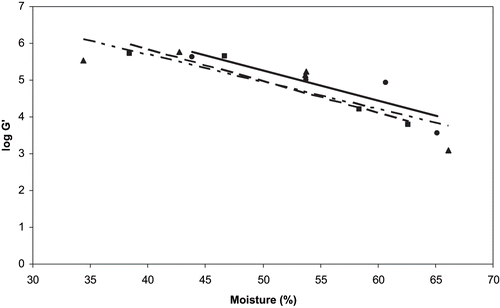
Loss Modulus
Loss modulus, an indication of the solid/liquid nature of a sample, fell between 0.20 and 0.27 for the NDM samples, all of which were quite solid (). The small (within the standard deviation) ranges of values reflect the small moisture effect or lactose effect within the temperature range. Many of the 50°C WPC and WPI samples were liquid-like, and therefore gave loss modulus values greater than 0.5. The lower moisture content at 100°C is reflected by loss modulus values under 0.3. All of the samples were solid when extruded at 100°C, and almost all were solid at 75°C, but there was a wide solid/liquid variation at 50°C. These results reflect the increase in shear denaturation and heat denaturation with extrusion temperature.
Table 5 Loss modulus values for extruded nonfat dry milk (NDM), whey protein concentrate (WPC), and whey protein isolate (WPI) samples, with pooled standard deviations (PSD).
Extruded NDM, WPC, and WPI can all be used as food ingredients depending upon the properties desired, and the type and amount of protein a manufacturer wishes to include. NDM produced a solid product with less moisture than the whey proteins when extruded at 50 or 75°C, and with higher WSI when extruded at 50°C. Extruded WPI contained more moisture than the other extruded proteins, and was usually less solid-like. When extruded at 50°C, the moisture content of WPC was close to that of NDM, but the WSI was lower and the product became fluid-like as the pump setting increased. At the other temperatures, the WPC moisture content was lower than that of WPI and the WSI was higher than that of NDM. Whey proteins are now being included in a number of food products, and modification by extrusion may allow for more applications and greater utilization.
CONCLUSIONS
Adjustment of water addition and temperature led to differences in rheology, moisture, and solubility of extruded milk proteins. The moisture content of extruded NDM, WPC, and WPI increased with water pump setting. Extruded NDM, nearly all of which is casein and lactose, remained solid within a relatively narrow range of G′ and loss modulus values. Extruded WPC and WPI, which contained 77–90% protein, liquefied when extruded at 50°C, became soft at 75°C and high moisture content, and were solid at 100°C, reflecting the level of heat-induced and shear-induced denaturation. The range of physical properties exhibited by extruded milk powders will allow food processors and ingredient manufacturers to tailor their protein-fortified products according to their customers' needs.
ACKNOWLEDGMENTS
The authors thank Zerlina Muir and Eric Tilman for their valuable contributions. References to brand or firm name are for information only, and do not constitute endorsement by the U.S. Department of Agriculture over others of a similar nature not mentioned.
REFERENCES
- Foegeding , E.A. and Luck , P.J. 2003 . “ Whey Protein Products ” . In Encyclopedia of Dairy Sciences , Edited by: Roginski , H. , Fuquay , J.W. and Fox , P.F. Vol. 3 , 1957 – 1960 . New York : Academic Press .
- Herceg , Z. and Lelas , V. 2005 . The Influence of Temperature and Solid Matter Content on the Viscosity of Whey Protein Concentrates and Skim Milk Powder Before and After Tribomechanical Treatment . J. Food Eng , 66 : 433 – 438 .
- Luff , S. 2005 . Products for Athletes . Food Prod. Design , 15 ( 5 ) : 49 – 52 .
- Child , R. 2005 . The Wheys of Weight Loss . Funct. Foods Nutraceut , 5 ( 3 ) : 62 – 71 .
- Resch , J.J. and Daubert , C.R. 2002 . Rheological and Physicochemical Properties of Derivatized Whey Protein Concentrate Powders . Int. J. Food Prop , 5 : 419 – 434 .
- Firebaugh , J.J. and Daubert , C.R. 2005 . Emulsifying and Foaming Properties of a Derivatized Whey Protein Food Ingredient . Int. J. Food Prop , 8 : 243 – 253 .
- Harper , J.M. 1986 . Extrusion Texturization of Foods . Food Technol , 40 ( 3 ) : 70 – 76 .
- Kim , C.H. and Maga , J.A. 1987 . Properties of Extruded Whey Protein Concentrate and Cereal Flour Blends . Lebensm.-Wiss. Technol , 20 : 311 – 318 .
- Singh , H. and Havea , P. 2003 . “ Thermal Denaturation, Aggregation and Gelation of Whey Proteins ” . In Advanced Dairy Chemistry , 3rd , Edited by: Fox , P.F. and McSweeney , P.L.H. Vol. 1 , 1261 – 1287 . New York : Kluwer Academic/Plenum Publishers. .
- Paulsson , M. and Dejmek , P. 1990 . Thermal Denaturation of Whey Proteins in Mixture with Caseins Studied by Differential Scanning Calorimetry . J. Dairy Sci , 73 : 590 – 600 .
- Onwulata , C.I. , Konstance , R.P. , Cooke , P. H. and Farrell , H.M. Jr. 2003 . Functionality of Extrusion-texturized Whey Proteins . J. Dairy Sci , 86 : 3775 – 3782 .
- Aguilera , J.M. and Kosikowski , F.V. 1978 . Extrusion and Roll-cooking of Corn-soy-whey Mixtures . J. Food Sci , 43 : 225 – 227 . 230
- Cuddy , M.E. and Zall , R.R. 1982 . Performance of Lipid-dried Acid Whey in Extruded and Baked Products . Food Technol , 36 ( 1 ) : 54 – 59 .
- Onwulata , C.I. , Konstance , R.P. , Smith , P.W and Holsinger , V.H. 1998 . Physical Properties of Extruded Products as Affected by Cheese Whey . J. Food Sci , 63 : 814 – 818 .
- Onwulata , C.I. , Konstance , R.P. , Smith , P.W and Holsinger , V.H. 2001 . Co-extrusion of Dietary Fiber and Milk Proteins in Expanded Corn products . Lebensm. Wiss. Technol , 34 : 424 – 429 .
- Onwulata , C.I. , Smith , P.W. , Konstance , R.P. and Holsinger , V.H. 2001 . Incorporation of Whey Products in Extruded Corn, Potato or Rice Snacks . Food Res. Int , 34 : 679 – 687 .
- AOAC International . 1998 . Official Methods of Analysis , 16th , Gaithersburg, MD : AOAC International .
- Anderson , R.A. , Conway , H.F. , Pfeifer , V.F. and Griffin , E.J. Jr. 1969 . Gelatinization of Corn Grits by Roll and Extrusion Cooking . Cereal Sci. Today , 14 : 4 – 12 .
- Berlin , E. , Anderson , B.A. and Pallansch , M.J. 1968 . Water Vapor Sorption Properties of Various Dried Milks and Wheys . J. Dairy Sci , 51 : 1339 – 1344 .
- Berlin , E. , Anderson , B.A. and Pallansch , M.J. 1968 . Comparison of Water Vapor Sorption by Milk Powder Components . J. Dairy Sci , 51 : 1912 – 1915 .
- Berlin , E. , Kliman , P.G. , Anderson , B.A. and Pallansch , M.J. 1973 . Water Binding in Whey Protein Concentrates . J. Dairy Sci , 56 : 984 – 987 .
- Spiegel , T. 1999 . Whey Protein Aggregation Under Shear Conditions — Effects of Lactose and Heating Temperature on Aggregate Size and Structure . Int. J. Food Sci. Technol , 34 : 523 – 531 .
- Havea , P. , Singh , H. , Creamer , L.K. and Campanella , O.H. 1998 . Electrophoretic Characterization of the Protein Products Formed During Heat Treatment of Whey Protein Concentrate Solutions . J. Dairy Res , 65 : 79 – 91 .
- Morr , C.V. and Ha , E.Y.W. 1993 . Whey Protein Concentrates and Isolates: Processing and Functional Properties . Crit. Rev. Food Sci , 33 : 431 – 476 .
- Farrell , H.M. Jr. , Qi , P.X. , Brown , E.M. , Cooke , P.H. , Tunick , M.H. , Wickham , E.D. and Unruh , J.J. 2002 . Molten Globule Structures in Milk Proteins: Implications for Potential New Structure-function Relationships . J. Dairy Sci , 85 : 459 – 471 .
- Nielsen , M.A. , Coulter , S.T. , Morr , C.V. and Rosenau , J.R. 1973 . Four Factor Response Surface Experimental Design for Evaluating the Role of Processing Variables upon Protein Denaturation in Heated Whey Systems . J. Dairy Sci , 56 : 76 – 83 .
- Tunick , M.H. 2000 . Rheology of Dairy Foods that Gel, Stretch, and Fracture . J. Dairy Sci , 83 : 1892 – 1898 .