Abstract
Stability of vitamin C in fortified formula food at constant temperature, as affected by the water activity (aw ) and glass transition (Tg ), was investigated in an attempt to differentiate the effects of aw and Tg on the degradation reaction. Fortified formula samples, equilibrated at different moisture contents/water activity levels, were stored for more than 10 weeks at the room temperature, 23°C. The water activity and glass transition temperatures as a function of moisture content were determined for fortified formula to compare the two distinct criteria of food stability. The adsorption experiments, which were carried out at controlled water activities, at 23°C using an isopiestic method, were modeled using the GAB equation. The glass transition temperatures, which were measured by modulated differential scanning calorimeter, were modeled using the Gordon-Taylor equation. The loss of vitamin C followed a zero-order kinetic model. The vitamin C retention after storage of 73 days at water activities of 0.11 and 0.87 was 40 and 7%, respectively. The rate constants ranged from 5.8 to 10 mgd−1, depending on the water activities. The constants were correlated with water activities and glass transition temperatures. Results indicated that reactions rates correlated with both water activity and the glass transition temperatures. In the storage and processing of fortified food products, both the water activity and glass transition should be recognized as important criteria for gaining a better understanding of vitamin stability.
INTRODUCTION
In recent years, vitamins have been incorporated into more and more varieties of food formulations as the market for neutraceuticals and supplemented food keeps on growing. Vitamin C is one of the most frequently added vitamins in commercially available fortified formulas. L-Ascorbic acid is the main biologically active form of vitamin C. As an antioxidant, vitamin C plays several roles in human health. It is now well established that it helps in neutralizing free radicals, which play an important role in cell degradation. It readily scavenges reactive oxygen and nitrogen species, and thereby, prevents oxidative damage to important biological macromolecules such as DNA, lipids, and protein. It also reduces redox active transition metal ions in the active sites of specific biosynthesis enzymes and allows for lowering of blood pressure.[Citation1]
Vitamin C is unstable and its degradation depends upon many factors such as oxygen, heat, light, storage temperature, and storage time.[Citation2] The stability of vitamin C has been evaluated in fresh fruits during processing and storage.[Citation2–7] The stability of vitamin C in fortified formula has not been evaluated using water activity and glass transition concepts.
Water activity has traditionally been used to describe microbial and chemical stability of food products.[Citation8–10] During the last two decades, attempts have also been made to apply glass transition concept to explain the physical, chemical, and microbial stability of food, biological, and pharmaceutical product.[Citation11–20] In the glassy state, reactions that depend on molecular diffusion, such as chemical and enzymatic changes, are reduced significantly. Despite the research effort, the significance and the mechanism of the glass transition as an indicator of chemical and biochemical stability is still not clear.[Citation18] Due to the interrelation among factors (i.e. temperature, water activity, and pH) influencing chemical reactions, it is difficult to interpret the results.[Citation21] Food materials are heterogeneous systems; these may contain local regions of different molecular mobility. The use of a single glass transition temperature measured using existing methods of mechanical and thermal measurements may not be taken as an absolute reference for stability.[Citation22]
Few studies have compared both criteria (i.e., water activity and glass transition) from the viewpoint of product stability. Roos[Citation23] evaluated these concepts in relation to the molecular weight of materials of a homologous family. He defined the critical water content that depressed the glass transition temperature to 25°C. It was indicated that increasing molecular weight of the material required a rise in critical water activity from 0.07 to 0.71 in order to depress the Tg to ambient temperature. Schaller-Povolny et al.[Citation24] compared water activity and glass transition data of four different molecular weights of inulin. Similarly, their study argued that increasing molecular weight increased the critical water activity from 0.44 to 0.61 required at a given Tg . These studies compared the a w/Tg requirement at ambient temperature (i.e., between 23 and 25°C). Sablani et al.[Citation25] examined the two concepts with several food materials. They indicated that based on the sorption isotherm criterion, for most food materials (expect sugar rich fruits), the predictions of the glass transition model overestimate the stable temperature range at each level of solids.
Most of the studies reported have used model food systems to evaluate chemical changes while applying glass transition and water activity concepts.[Citation15,Citation16,Citation18,Citation33] Information on stability of bioactive compounds in food materials such as fruits, vegetables, and dairy products; and, neutraceuticals formulations as influenced by water activity and glass transition is scarce.
The objective of the present article was to evaluate the storage stability of vitamin C in fortified formula using water activity and glass transition concepts. This would enhance the knowledge of the necessary requirements for proper storage conditions and chemically stable formulations.
MATERIALS AND METHODS
Material and Vitamin C Analysis
A commercial fortified formula manufactured by a multinational company was selected for the evaluation of the stability of vitamin C during storage at room temperature. The shelf life of fortified formula as specified on the label was 2 years. At the time of purchase, the formula powder was four months old and its initial moisture content was 5.4% (w.b.). The ingredients of the fortified formula listed on the bottle label were milk solids, wheat flour, malted barley, malt extract, sugar, 16 different minerals, and vitamins. Immediately after purchase, the formula samples were equilibrated at different moisture contents using water activity jars. These were then stored at room temperature for 73 days. The moisture content of equilibrated samples was calculated gravimetrically by drying samples in the air convection drier at 105°C for at least 24 hours.[Citation26] The vitamin C content in fortified powder was determined using a titration method with 2–6 dichloro-phenol-indophenol reagents.[Citation26] The loss of vitamin C in fortified formula was modeled using the standard equation of zero- and first-order reaction given below:
Sorption Isotherms
The water sorption isotherm of fortified formula powder was obtained using an isopiestic method.[Citation28,Citation29] Five replicates of powder were placed in open weighing bottles and stored in air-sealed glass jars while maintaining equilibrium relative humidity with saturated salt solutions. The salts were: LiCl, CH3COOK, MgCl2, K2CO3, Mg(NO3)2, NaBr, SrCl2, and KCl (BDH, Laboratory Supplies, Poole, England). Relative humidity values for these solutions were obtained from Greenspan,[Citation29] and Spiess and Wolf.[Citation30] The jars were kept at room temperature of 23°C.
The most commonly used BET and GAB equations were employed for modeling of the sorption isotherm.[Citation28] The BET equation is given by:
Thermal Analysis
A modulated differential scanning calorimeter (MDSC, Q1000, TA Instruments, New Castle, DE) equipped with a mechanical cooling system was used to perform experiments. Temperature modulation (sinusoidal oscillation) of MDSC separates total heat flow into its reversing (thermodynamic) and non-reversing (kinetic) components. A mechanical refrigerated cooling system was used to chill the sample up to –90°C. The calorimeter was calibrated according to the instruction provided by TA instruments user manual by checking temperature and enthalpy of fusion of indium and sapphire as standards. Fortified formula powder samples (5–10 mg) equilibrated at different water activities were placed in aluminum pans and were cooled to –90°C at 5°C/min, and equilibrated for 10 minutes. After equilibration it was scanned from –90°C to 90°C at a rate of 5°C/min. Each thermogram was analyzed for the onset, mid, and end of glass transition, and mid values were used. Three replicates were used for the measurement of glass transition temperatures at each water activity/moisture content.
An empirical equation proposed by Gordon and Taylor[Citation32] was used to predict the glass transition temperature of mixtures:
RESULTS AND DISCUSSION
Water Sorption and Glass Transition Data
Experimental water sorption and glass transition data for fortified formula is presented in and also plotted in and . The constants of BET and GAB models are shown in . These constants can be used to predict water sorption isotherms at room temperature. The monolayer moisture content values were 6.31 and 9.95% (d.b.) in the BET and GAB models, respectively. The monolayer moisture content estimated by GAB model was higher than the BET monolayer moisture content. The BET monolayer is an effective method for estimating the amount of water bound to specific polar sites in dehydrated systems. The product at its monolayer moisture should be stable against microbial and chemical deterioration.[Citation10] The values of Tgs and k for fortified formula powder were found to be 46.5°C and 2.18, respectively.
Table 1 Water activity and corresponding equilibrium moisture content (EMC, g H2O/g of dry matter) and initial (Tgi ), mid (Tgm ), and end (Tge ) glass transition temperatures of fortified formula powder
Table 2 BET and GAB model parameters of water adsorption isotherms of fortified formula powder at room temperature
Water activity and glass transition concepts were closely examined for formula powder ( and ). The water activity concept suggests that a product is most stable at its monolayer moisture content, which depends on chemical composition and temperature of the material.[Citation9] The glass transition concept puts forward that a product formulation is stable at or below the corresponding glass transition temperature. Based on the sorption isotherm criterion, the predictions of the glass transition model closely matched the stable temperature. For instance, the sorption isotherm at 23°C indicates that formula powder would be stable at 94.1% solids (corresponds to BET monolayer) ( and ). At this level of solids, the glass transition curve produced using the Gordon and Taylor equation yields a Tg value of 24.7°C. The glass transition temperature of 23°C corresponds to a solid content fraction of 0.936 and a water activity value of 0.225 that is very much within the range of stable water activity. Sablani et al.[Citation25] have reported that based on the sorption isotherm criterion, for most food materials, except sugar rich fruits, the predictions of the glass transition model overestimate the stable temperature range at each level of solids.
Table 3 Comparing sorption isotherm and glass transition models using monolayer aw as the basis for stability
Stability of Vitamin C
The initial concentration of vitamin C in fortified formula powder was 690 ± 55 mg/100 g of dry formula. After 73 days of storage, vitamin C contents of samples decreased to 273 and 8 mg/100 g of dry formula at water activity of 0.11 and 0.87, respectively. The change in concentration of vitamin C with storage time at different water activities is shown in . The destruction of vitamin C occurred at all the water activities; however, the rate of degradation was lower at reduced level of water activity. The degradation of vitamin C was modeled using zero and first order kinetics. The values of R2 varied from 0.78 to 0.98 in case of for first order model and it varied from 0.91 to 0.99 for zero-order model. Hence the reaction rate constants of degradation of vitamin C were used from zero-order kinetic model (). The rate constants ranged from 5.8 to 10 mgd−1 depending on water activities.
Figure 4 Loss of vitamin C in fortified formula stored at room temperature at different water activities.
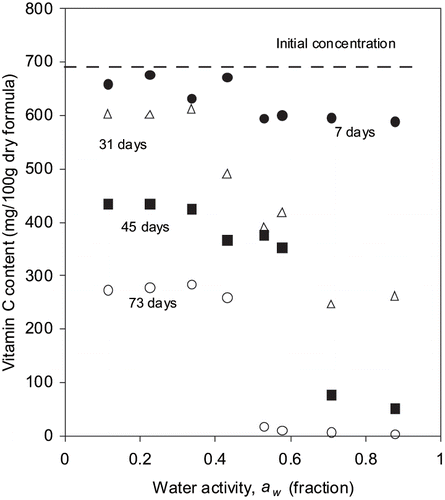
Figure 5 Zero-order plot of vitamin C loss in fortified formula stored at room temperature at different water activities.
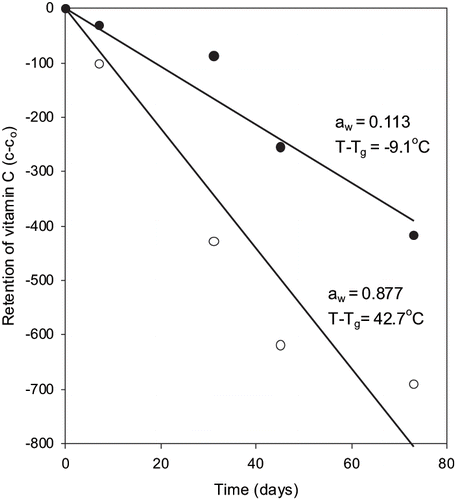
There have been studies reported that degradation of vitamin C followed zero-order,[Citation3] first order,[Citation2,Citation5] or second-order reaction.[Citation4] The reaction rate constant calculated at different moisture levels were plotted as a function of water activity and the distance from the glass transition temperature (i.e. T-Tg ) (). The reaction rate decreased significantly (P < 0.5) with decreasing water activity and T-Tg . However, at very low water activity (aw <0.2; corresponding to the storage temperature below glass transition), the reaction rate did not approach to zero. Overall, the reactions rates correlated very well with both water activity and the glass transition temperatures. The reaction rate constant was minimal around monolayer moisture content and as water activity increased the reaction rate increased. The condition of monolayer moisture content also corresponded with the glassy state of the formula powder. That is at monolayer moisture content the glass transition temperature of formula powder was above the storage temperature of 23°C. The formula powder at monolayer moisture was in the glassy state when stored at the room temperature. Both water activity and glass transition concepts predicted similar conditions of moisture/solids level and temperature for safe storage. In the literature, the water activity and glass transition data have been compared for stability of aspartame and thiamin in model food systems, i.e., polyvinylpyrrolidone of different molecular weight.[Citation15] Bell and Hageman[Citation15] observed that aspartame degradation depended on the water activity rather than upon the state of the system. While both aw and Tg appeared to affect thiamin stability in solids with glass transition considerations having larger effect on the reaction.[Citation33] The article suggests that aw or Tg criteria alone can be used to evaluate stability of vitamin C in fortified formula powder.
CONCLUSIONS
Vitamin C in fortified formula decreased more rapidly with increasing water activity and distance from glass transition temperature. The loss of vitamin C in fortified formula at different moisture levels was described by zero-order reaction. Both aw and Tg affected the stability of vitamin C. In the storage and processing of fortified food products, both the water activity and glass transition should be recognized as important criteria for gaining a better understanding of vitamin stability. Research on the stability of other nutrients in different food systems as affected by water activity and glass transition would provide additional insight into the factors influencing their degradation during storage.
NOMENCLATURE
a w | = |
Water activity (fraction) |
B | = |
Constant related to net heat of sorption in EquationEq. 1 |
C | = |
Constant related to first layer heat of sorption in EquationEq. 2 |
i | = |
ith experimental data |
K | = |
Constant related to heat of sorption of multi-layer in EquationEq. 2 |
M w | = |
Moisture content (fraction, gH2O/g dry matter, d.b.) |
M b | = |
Monolayer moisture content in BET equation (g/g dry matter) |
M g | = |
Monolayer moisture content in GAB equation (g/g dry matter) |
k | = |
Gordon-Taylor constant parameter |
T | = |
Temperature (K) |
X w | = |
Moisture content (fraction, gH2O/g sample, w.b.) |
REFERENCES
- Davies , M.B. , Austin , J. and Partridge , D.A. 1991 . Vitamin C: Its Chemistry and Biochemistry , London : Royal Society of Chemistry .
- Buedurlu , H.S. , Koca , N. and Karadeniz , F. 2006 . Degradation of Vitamin C in Citrus Concentrates during Storage . Journal of Food Engineering , 74 : 211 – 216 .
- Laing , B.M. , Schlueter , D.L. and Labuza , T.P. 1978 . Degradation Kinetics of Ascorbic Acid at High Temperature and Water Activity . Journal of Food Science , 43 : 1440 – 1443 .
- Robertson , G.L. and Samaniego , C.M.L. 1986 . Effect of Initial Dissolved Oxygen Levels on the Degradation of Ascorbic Acid and the Browning of Lemon Juice During Storage . Journal of Food Science , 51 : 184 – 187 .
- Johnson , J.R. , Braddock , R.J. and Chen , C.S. 1995 . Kinetics of Ascorbic Acid Loss and Nonenzymatic Browning in Orange Juice Serum: Experimental Rate Constants . Journal of Food Science , 60 : 502 – 505 .
- Lee , H.S. and Coates , G.A. 1999 . Vitamin C in Frozen, Fresh Squeezed Unpasteurized, Polyethylene-Bottled Orange Juice: A Storage Study . Food Chemistry , 65 : 165 – 168 .
- Biolatto , A. , Salitto , V. , Cantet , R.J.C. and Pensel , N.A. 2005 . Influence of Different Postharvest Treatments on Notional Quality of Grapefruits . Lebensmittel Wissenschaft-und Technologie (lwt) , 38 : 131 – 134 .
- Labuza , T.P. 1980 . The Effects of Water Activity on Reaction Kinetics of Food Deterioration . Food Technology , 34 : 36 – 41 . 59
- Rockland , L.B. and Nishi , S.K. 1980 . Influence of Water Activity on Food Product Quality and Stability . Food Technology , 34 : 42 – 51 .
- Rahman , M.S. and Labuza , T.P. 1999 . “ Water Activity and Food Preservation ” . In Handbook of Food Preservation , Edited by: Rahman , M.S. 339 – 382 . New York : Marcel Dekker .
- Roos , Y.H. and Karel , M. 1991 . Applying State Diagrams to Food Processing and Development . Food Technology , 45 : 66 – 71 .
- Slade , L. and Levine , H. 1991 . Beyond Water Activity: Recent Advances Based on an Alternative Approach to the Assessment of Food Quality and Safety . Critical Reviews in Food Science and Nutrition , 30 : 115 – 360 .
- LeMeste , M. , Huang , V.T. , Panama , J. , Anderson , G. and Lentz , R. 1992 . Glass Transition of Bread . Cereal Foods Worlds , 37 : 264 – 267 .
- Chuy , L.E. and Labuza , T.P. 1994 . Caking and Stickiness of Dairy Based Food Powders as Related to the Glass Transition . Journal of Food Science , 59 : 43 – 46 .
- Bell , L.N. and Hageman , M.J. 1994 . Differentiating Between the Effects of Water Activity and Glass Transition Dependent Mobility on a Solid State Reaction: Aspartame Degradation . Journal of Agricultural and Food Chemistry , 42 : 2398 – 2401 .
- Chen , Y.-H. , Aull , J.L. and Bell , L.N. 1999 . Solid-State Tyrosinase Stability as Affected by Water Activity and Glass Transition . Food Research International , 32 : 467 – 472 .
- Adhikari , B. , Howes , T. , Bhandari , B.R. and Truong , V. 2001 . Stickiness in Foods: A Review of Mechanisms and Test Methods . International Journal of Food Properties , 4 : 1 – 33 .
- Lievonen , S.M. and Roos , Y.H. 2003 . Comparison of Dielectric Properties and Non-Enzymatic Browning Kinetics around Glass Transition . Innovative Food Science and Emerging Technologies , 4 : 297 – 305 .
- Khalloufi , S. and Ratti , C. 2003 . Quality Deterioration of Freeze Dried Foods as Explained by Their Glass Transition Temperature and Internal Structure . Journal of Food Science , 68 : 892 – 903 .
- Roe , K.D. and Labuza , T.P. 2005 . Glass Transition and Crystallization of Amorphous Trehalose-Sucrose Mixtures . International Journal of Food Properties , 8 : 559 – 574 .
- Bell , L.N. and Hageman , M.J. 1995 . Water Activity and Glass Transition Effects on Solid State Chemical Reactions . Journal of Food Quality , 18 : 141 – 147 .
- Champion , D. , Le Meste , M. and Simatos , D. 2000 . Towards an Improved Understanding of Glass Transition and Relaxation in Foods: Molecular Mobility in the Glass Transition Range . Trends in Food Science and Technology , 11 : 41 – 55 .
- Roos , Y.H. 1993 . Water Activity and Physical State Effects on Amorphous Food Stability . Journal of Food Processing and Preservation , 16 : 433 – 447 .
- Schaller-Povolny , L.A. , Smith , D.E. and Labuza , T.P. 2000 . Effect of Water Content and Molecular Weight on the Moisture Isotherms and Glass Transition Properties of Inulin . International Journal of Food Properties , 3 : 173 – 192 .
- Sablani , S.S. , Kasapis , S. and Rahman , M.S. 2006 . Evaluating Water Activity and Glass Transition for Stability of Dried Foods . Journal of Food Engineering , 78 : 266 – 271 .
- Association of Official Analytical Chemists . 1990 . Official Methods of Analysis of the AOAC , Edited by: Helrich , K. Arlington : AOAC .
- Rahman , M.S. 1995 . Food Properties Handbook , Boca Raton, FL : CRC Press .
- Sablani , S.S. , Rahman , M.S. and Labuza , T.P. 2001 . “ Measurement of Water Activity using Isopiestic Method ” . In Current Protocols in Food Analytical Chemistry , Edited by: Wrolstad , R.E. Vol. 1 , A2.3.1 – A2.3.10 . New York : John-Wiley and Sons, Inc. .
- Greenspan , L. 1977 . Humidity Fixed Points of Binary Saturated Aqueous Solutions . Journal of Research and National Bureau of Standards (A): Physics and Chemistry , 81A ( 1 ) : 89
- Spiess , W.E.L. and Wolf , W. 1987 . “ Critical Evaluation of Methods to Determine Moisture Sorption Isotherms ” . In Water Activity: Theory and Applications to Foods , Edited by: Rockland , L.B. and Beuchat , L.R. 215 – 234 . New York : Mercel Dekker .
- Rahman , M.S. 2005 . Dried Food Properties: Challenges Ahead . Drying Technology-An International Journal , 23 ( 4 ) : 695 – 715 .
- Gordon , M. and Taylor , J.S. 1952 . Ideal Copolymers and the Second Order Transitions of Synthetic Rubbers. I. Non-Crystalline Copolymers . Journal of Applied Chemistry , 2 : 493 – 500 .
- Bell , L.N. and White , K.L. 2000 . Thiamin Stability in Solids as Affected by the Glass Transition . Journal of Food Science , 65 : 498 – 501 .