Abstract
Onion slices (0.5 cm thick) and tomato fruits, fresh and osmotically dehydrated in sugar (600 g/l), salt (300 g/l) or mixed salt and sugar (45/15, w/w) solutions, were air dried at 60°C and drying constants determined. The air drying occurred in two falling rate periods, characterised each by a drying constant. The drying rate and the moisture diffusivity of osmosed samples were higher than that of fresh samples. The overall drying behaviour of osmosed onion slices was not influenced by osmotic conditions (solution and time), while the drying behaviour of osmosed tomato samples depended on osmotic solution and pre-treatment time. 15 minutes and 20 hours of pre osmosis seemed more convenient before drying of onion slices and tomato respectively. In this respect, the structural modification of cells of tomato membrane during extended pre treatment seemed to explain the variation in the drying behaviour.
INTRODUCTION
Sun drying is among the most affordable techniques used by farmers to extend shelf-life of foods. The principle of the technique is based on the removal of free water from foods, in order to prevent both enzymatic and microbial degradation. Since the biological components of foods are susceptible to heat degradation, many dried foods present physical and organoleptic denaturation, which results in the reduction of their market value. In this respect, different drying techniques have been developed towards water removal with care to preserve the chemical and physical integrity of the food.
Many scientific works have proven the interest of osmotic dehydration of food before drying.[Citation1,Citation2,Citation3,Citation4,Citation5] The osmotic treatment has the advantage of maintaining organoleptic (colour, texture, aroma) and nutritional (vitamins, minerals) characteristics of the food. The treatment consists in immersing the product in a concentrated solution, which absorbs water from the product by osmosis. The transfer of water is accompanied by exchange of solubles between the product and the solution. After this initial osmotic step, the osmosed product can therefore be dried using a conventional drying method such as hot air drying, to produce a shelf stable, dried food.
Osmotic process is a very suitable pre-treatment prior to air drying of fruits and vegetables, since it inhibits polyphenol oxidase and prevent the oxidative browning.[Citation6] The simultaneous material exchange between the food product and the osmotic solution is accompanied by significant shrinkage, deformation and flow interaction.[Citation7,Citation8,Citation9,Citation10] These changes in the composition and structure of the food during the osmotic step can influence the air drying behaviour later. Different studies have reported reduction of drying rate after osmotic dehydration of foods using sucrose solution, and have attributed the decrease in drying rate to the presence of infused sucrose.[Citation2,Citation11]
A previous study of material transfer during osmotic dehydration of onion slices and tomato fruits in different osmotic solutions (sodium chloride, sucrose and mixture of sodium chloride and sucrose) showed a predominance of soluble gain over solubles, with better control of solute penetration when mixed osmotic solution was used. In addition, extended treatment, beyond 15 minutes and 20 hours, respectively, for onion slices and tomato, resulted in structural alteration of cells, and disturbance of material exchanges.[Citation10]
The present study aims at examining the air drying behaviour of the above osmosed onion slices and tomato fruits. Farmers of the Lake Chad region of Central Africa have developed local production of dried onion slices and tomato powder, but the market quality of these dried products is reduced by their dark colour.[Citation12] Therefore the introduction of an osmotic step in the drying process appears a good opportunity for quality improvement.
MATERIAL AND METHODS
Source and Preparation of Onion Slices and Tomato Fruits
Onion fruits (Allium cepa), purchased from local market (Ngaoundéré, Cameroon), were bulbs of 8–12 cm longitudinal diameter, and 6–8 cm transversal diameter. The external envelopes of the bulbs were removed, and the flesh was washed using distilled water, then sliced horizontally in order to obtain rings of 0.5 cm thickness. Red tomato fruits (Lycopersicon esculentum), cultivar “Roma,” were harvested from a local farm (Ngaoundéré, Cameroon). All fruits had ovoid shape with a length of 5.2 ± 0.4 cm, a median diameter of 3.3 ± 0.2 cm, and a weight of 45 ± 2 g. The selected fruits were washed in distilled water and used for assays during the week following harvesting. Tomatoes were treated without slicing.
Osmotic Pre-treatment
Samples were osmosed in sealed glass jars at 25°C for onion slices and 60°C for tomato fruits, under permanent agitation (80 rotation/min), using a product/solution ratio of 1/10 (w/w). The osmotic media consisted of solutions of commercial sucrose (600 g/l), commercial sodium chloride (300 g/l), or a mixture of sucrose and NaCl (45/15, w/w). For each solution, samples were immersed for 5, 10, 15, 30, 60, and 120 minutes for onion slices, and 1, 5, 10, 20, 40, 60, 80, and 100 hours for tomatoes.[Citation10]
Drying Experiments
Untreated and osmosed samples were placed in simple and aerated layers on pre-weighed drying trays, and dried at 60°C in a cross flow cabinet dryer (Heraeus T6), with an air flow rate of 0.13 ms−1. Drying trays were periodically weighed during the drying process. For each sample, trays were prepared in triplicate, and from the three values of tray weight, average of sample moisture were determined as a function of time. These values were used to construct the drying curves.
Theoretical Approach
Assuming the description of the drying behaviour of the samples by Fick's diffusion equation and the analytical solution given by Crank,[Citation13] the removable moisture ratio (Hr) of the samples during drying was expressed using the simplified equation of Perry et al.[Citation14] and Henderson and Perry:[Citation15]
Where Ht (gH2O.g−1 DM) is the time dependent moisture content of the sample; H0 is the initial moisture content of the sample; He is the equilibrium moisture content (at the end the drying process); D (m2 h−1) is the apparent moisture diffusivity; t (h) is the drying time; and, k (h−1) is the drying constant, obtained from the plot of lnHr vs. t. Since the drying experiments are conducted for long time until equilibrium rate is approached, it is assumed that at equilibrium, the drying rate is zero;[Citation3] i.e.:
Therefore, from plots of near equilibrium, final values of vs.H0, and through regression analyses, He values were determined as the point where the graph cuts the Ht axis.[Citation3] The values obtained were included in EquationEq. (1) to determine drying constants.
The apparent moisture diffusivities D, during the drying process were estimated at various moisture contents by the method of Perry et al.,[Citation14] as described by Sankat et al.[Citation3] For a given value of Hr, the theoretical value of is calculated from EquationEq. 1, and the predicted experimental value of t obtained from the linear regression of Hr
vs. t. Then D is obtained from:
where subscripts “th” and “exp” refer to theoretical and experimental values, respectively.
RESULTS AND DISCUSSION
Drying curves of untreated and osmosed onion slices and tomato fruits are shown respectively on and . The difference in initial moisture content of osmosed products reflects the varying degrees of water loss during osmotic pre-treatment and indicates reduction of residual water content with increasing of osmotic treatment time.[Citation9] In this respect, initial water content is higher in the sugar osmosed samples than in the salt and mixed osmosed ones. In spite of these initial differences, all curves show convergence after 20 hours and 60 hours of drying, respectively, for onion slices and tomato.
The predicted values of equilibrium moisture contents (He) attained at the end of the drying process () show that in onion slices, He decreases with the increase in pre- osmosis time, whatever the osmotic solution used. When osmotic pre-treatment was done for more than 15 minutes in a given osmotic solution, the decrease in He was not significantly different. He values of tomato samples are relatively comparable when pre-treatment has been less than 20 hours. Above this pre-treatment time, He values become irregular.
Table 1 Equilibrium moisture contents (g H20.g−1 DM) of dried, fresh, and osmosed onion slices and tomato.
The previously mentioned behaviour in He values could be related to the structural alteration of cells and disturbance of material exchange previously observed for extended osmotic treatments. The cell alteration occurred after 15 minutes and 20 hours of osmosis, respectively, for onion slices and tomato.[Citation10] It could be hypothesized that the destruction of the proteins of cells membrane by osmotic shock, after extended osmotic treatment,[Citation16] disturbed the water-binding capacity of the macromolecules. For short and medium time osmosed products, i.e., equal or less than 15 minutes and 20 hours, respectively, for onion slices and tomatoes, the equilibrium moisture contents at the end of the drying process are comparable to the untreated samples; which indicate that the cell structure of these samples are relatively intact.
The drying rate curves (,) show two periods of drying. In the early period of drying, when moisture content is the highest, drying rates start with the highest values, dependent upon the level of osmotic pre-treatment, then decline rapidly. After this initial period of rapid decline, the drying rate curves continue to decline, but more gradually and in a linear fashion, to equilibrium conditions. In this second period, the differences in equivalent drying rate between fresh and osmosed samples are not easily perceptible. The break point separating the two falling rate periods occurs at moisture contents of about 2g H2O.g−1 (DM basis) and 0.75g H2O.g−1 (DM basis), respectively, for onion slices () and tomato ().
From linear regression analyses of the drying rate (lnHr vs. t), considering transient moisture content values in each of the two falling rate periods, drying constants k1 and k2 were established (EquationEq. 1) for all drying runs (, ). k1 and k2 represent the drying constants respectively in the first falling rate period, when drying rates are high, and in the second falling rate period, when decline in drying rate is linear and drying curves are about the same. In all drying runs, k1 is higher than k2, confirming a higher drying rate in the first falling rate period.
Table 2 Drying constants k1 and k2, during the air drying of onion slices as a function of osmotic pre-treatment time and solution.
Table 3 Drying constants k1 and k2, during the air drying of tomato fruits as a function of osmotic pre-treatment time and solution.
In onion slices, for each falling-rate period, drying constants of osmosed samples are relatively comparable for all osmotic pre-treatment times and solutions used. Therefore, k1 and k2 means have been calculated (). In addition, it should be noted that the drying of untreated onion slices does not show two falling-rate periods (k1 is almost equal to k2), indicating that in this case, drying occurs in one falling rate. In tomato (), k1 constants are comparable and higher than k2 for samples pre-osmosed during 5h and 10h. During this drying time, drying rate is in general higher for salt pre-osmosed samples than for sucrose pre-osmosed samples and than for mixed pre-osmosed ones. Above this pre-treatment time, there is a change in the trend of drying constant values for all pre-osmosis solutions, with tendency of k1 values to increase for sucrose and mixed pre-osmosed samples, while for salt pre-osmosed tomato samples the drying tends to occur in one falling period (k1 ≈ K2). The incrustation of sugar molecules at the surface of cytoplasm during long period of osmotic pre-treatment and the resulting alteration of cell membrane[Citation10,Citation17] may explain the increase in drying rate for sucrose and mixed pre-osmosed tomato samples. The presence of salt in mixed solution seems to have contributed to limit the incrustation of sugar and the physical alteration of cell membrane during osmotic pre-treatment,[Citation7] which may be the reason why the increase in drying rate is lower for mixed solution pre-osmosed tomato samples than in sucrose pre-osmosed ones.
The calculation of apparent moisture diffusivity of onion slices during drying, as a function of samples moisture content () shows that during drying, moisture diffusivity decreases with the decrease of moisture content. This behaviour is normal since moisture migration becomes increasingly difficult as the consequence of toughness and hardness of sample structure during drying. Meanwhile, though residual water is higher in untreated onion than in pre-osmosed one, the moisture diffusivity during drying is higher in the later; the minimum predicted diffusivity value in this case is around 1.5 × 10−8 m2h−1 when moisture content tends towards zero, while in unosmosed onion slice, the minimum diffusivity is around 0 when the moisture content is still around 8 gH2O g−1 DM (). This observation indicates that pre-osmosed dry sample contains less residual moisture than untreated dry sample.
Figure 5 Changes in apparent moisture diffusivity in onion slices as a function of residual moisture content during the drying process.
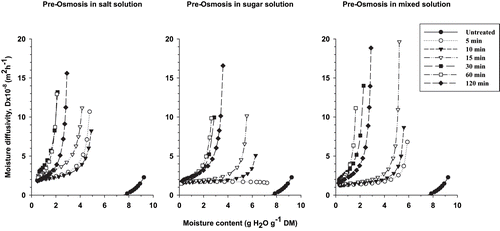
During the decrease of apparent diffusivity, for a given moisture content of pre-osmosed onion, D is higher as the pre-treatment time increases, particularly between 5 minutes to 60 minutes of pre-osmosis. Above 60 minutes, D decreases. In addition, from the trend of moisture diffusivity (), pre-osmosed samples can be divided in 2 homogenous groups characterised by their pre-treatment time (5 to 15 minutes and above 15 minutes), and their comparable diffusivity towards equilibrium, particularly for sugar and mixed pre osmosed samples. These observations are related to the trend of equilibrium moisture content (); He decreased as pre-osmosis time increased up to 15 minutes, and remained constant for samples pre treated above 15 minutes. From 60 minutes of pre-treatment, change in trend of He and D values may be attributed to alteration of cells membrane due to long osmotic pre-treatment.
CONCLUSION
This study has shown that extension of osmotic pre-treatment results in reduction of equilibrium moisture content at the end of air drying. This reduction in He value seems to be related to the alteration of the cells membrane during long osmotic pre-treatment. In this respect, and considering the mastering of the drying process, 15 minutes of osmosis seems an acceptable pre-treatment time before drying of onion slices, while for tomato, pre-osmosis should end before 20 hours.
Air drying occurs in two falling rate periods, whose constants depend on the nature of the food material. For onion slices, osmotic pre-treatment increases the drying constants; but since the cells membrane do not seem to be significantly affected by osmotic pre-treatment, the drying constants are not significantly influenced neither by the osmotic solution, nor by the time of pre-treatment. On the other hand, for tomato, the structure of cells membrane are more affected by osmotic conditions (time and solution) and results thus in variation of drying constant values.
With respect to the structural effect of osmotic pre-treatment on the drying behaviour of food, the study of the ultra structure of cells both during osmosis and air drying represents a research opportunity for better understanding of the behaviour of food materials during these treatments.
REFERENCES
- Jackson , T.H. and Mohammed , B.B. 1971 . The Shambat Process. New Development Arising from the Osmotic Dehydration of Fruits and Vegetables . Sudan Journal of Food Science and Technology , 3 : 18 – 22 . (1971)
- Islam , M.N. and Flink , J.N. 1982 . Dehydration of Potatoes. II. Osmotic Concentration and its Effect on Air Drying Behaviour . Journal of Food Technology , 31 : 123 – 125 .
- Sankat , C.K. , Castaigne , F. and Rohanie , M. 1996 . The Air Drying Behaviour of Fresh and Osmotically Dehydrated Banana Slice . International Journal of Food Science and Technology , 31 : 123 – 135 .
- Jiokap , N.Y. , Nuadje , G.B. , Raoult-Wack , A.L. and Giroux , F. 2001 . Comportement de Certains Fruits Tropicaux Traités Par Déshydratation-imprégnation Par Immersion Dans Une Solution De Saccharose . Fruits , 56 ( 2 ) : 75 – 83 .
- Moreno-Castillo , E.J. , Gonzales-Garcia , R. , Grajales-Lagunes , A. , Ruiz-Cabrera , M.A. and Abud-Achilla , M. 2005 . Water Diffusivity and Color of Cactus Pear Fruits (Opuntia Ficus indica) Subjected to Osmotic Dehydration . International Journal of Food Properties , 8 ( 2 ) : 323 – 336 .
- Ponting , J.D. 1973 . Osmotic Dehydration of Fruits—Recent Modifications and Applications . Process Biochemistry , 8 : 18 – 20 .
- Lenart , A. and Flink , J.N. 1984 . Osmotic Concentration of Potatoes: Criteria for the End Point of the Osmotic Effect . Journal of Food Technology , 19 : 65 – 89 .
- Fito , P. and Chiralt , A. 1997 . “ Osmotic Dehydration: An Approach to the Modelling of Solid–liquid Food Operations ” . In Food Engineering 2000 , Edited by: Fito , P. , Ortega-Rodriguez , E. and Barbosa-Canovas , G. New York : Chapman & Hall, International Thomson Publishing .
- Shi , J. and Le Maguer , M. 2003 . Mass Transfer in Cellular Material at Solid–liquid Contacting Interface . LWT Food Science and Technology , 36 : 3 – 11 .
- Passo Tsamo , C.V. , Bilame , A-F. , Ndjouenkeu , R. and Jiokap Nono , Y. 2005 . Study of Material Transfer During Osmotic Dehydration of Onion Slices (Allium Cepa) and Tomato Fruits (Lycopersicon Esculentum) . LWT Food Science and Technology , 38 : 495 – 500 .
- Rahaman , M.D.S. and Lamb , J. 1991 . Air Drying Behaviour of Fresh and Osmotically Dehydrated Pineapple . Journal of Food Process Engineering , 14 : 163 – 171 .
- Kenfack , H. , Ndjouenkeu , R. , Ngongang , D. , Ferré , T. and Koumaro , M. . Evaluation de la Production et de la Commercialisation de la Poudre de Tomate au Nord Cameroun et au Tchad . Proceedings of the International Workshop on Drying and Improvement of Shea and Canarium . Dec 1–3 1999 , Ngaoundere, Cameroon. Séminaire International sur le Séchage et la Valorisation du Karité et de L'aïélé , Edited by: Kapseu , C. and Kayem , J. pp. 381 – 387 . Cameroun : ENSAI, Université de Ngaoundéré .
- Crank , J. 1975 . Mathematics of Diffusion , London : Oxford University Press .
- Perry , R.H. , Green , D.W. and Maloney , J.O. 1984 . Perry's Chemical Engineers' Handbook , New York : McGraw Hill .
- Henderson , S.M. and Perry , R.L. 1976 . Agricultural Process Engineering , Connecticut : AVI Publishing Co. Inc. .
- Berkaloff , A. , Bourguet , J. , Favard , P. and Lacroix , J.C. 1977 . Biologie et physiologie cellulaire I. Membrane plasmique , 270 Paris : Herman ETC .
- Isse , M.G. and Schubert , H. . Osmotic Dehydration of Mango: Mass Transfer Between Mango and Syrup . Proceedings of Fourth World Congress of Chemical Engineering . Edited by: Behrens , D. pp. 728 – 745 . Francfurt : Bechema .