Abstract
Linear models based on water activity measurement were developed to predict the crystalline fraction of lactose present in the supersaturated crystal-solution mixture of lactose and whey. By this method, it was possible to measure the crystalline fraction in the mixture even if the sample is opaque or coloured, which would be difficult to measure by the conventional refractometric method. To calculate the fraction of lactose crystallized, the differential water activity of the crystallized mixture and non-crystalline supersaturated solution needs to be determined. For the pure lactose, the predictive linear equation was Δ L = 1874.4 Δ aw, whereas for whey it was Δ L = 1155.2 Δ aw, where Δ L is the amount of α-lactose monohydrate crystals (g 100g−1 water) that is in the crystallized solution, and Δ aw is the differential water activity after and prior to crystallization. Other equations such as Raoult's. Norrish, and Money-Born were also tested to predict the water activity of supersaturated solutions of lactose or whey.
Keywords:
INTRODUCTION
Many dairy or non-dairy food product systems contain low molecular weight carbohydrates such as sugars and acids, which can crystallize out from the mixture if they exist in a supersaturated state. The supersaturation is obtained by concentration or drying. In a composite food system, the crystallization is delayed if there are hinderances at the molecular level, such as intermolecular interactions. The solute-solvent interactions are also influenced by the presence of other components. Liquid food systems may be a mixture of crystalline solids suspended in a solution of the same species; the crystalline phase in this mixture is a non-solute component. At the equilibrium solubility concentration, the chemical potentials are the same for the crystal and the solution.[Citation1,Citation2] Therefore, the water activity of a saturated solution is constant, independent of the proportion of the crystalline and liquid phases. The water activity decreases as the solution becomes supersaturated. From the supersaturation state, being a thermodynamically non-equilibrium state, the excess solutes above saturation eventually crystallized out, depending on the temperature, time, and other intrinsic factors of the food systems. During crystallization, as the solute becomes crystallized, the water activity of the solution increases up to the level of a saturated solution. This is due to the expulsion of water molecules from hydrated sites during molecular rearrangement, and/or the decreased level of interaction of crystalline solids with water. A thermodynamic equilibrium condition is achieved only when the water activity of the system is reduced to a constant value at the saturation point. Each individual compound possesses its own equilibrium water activity value at saturation.
Haneock and Zografi[Citation3] described that it is possible to quantify the degree of crystallinity of solid food materials in a semicrystalline mixture. This is based on the large difference in the degree of water absorption (solute-water interaction) between the amorphous and crystalline fractions. Since water activity of a solution is a function of concentration of solutes, it can also be used as a tool to quantify the crystalline fraction present in the liquid systems.[Citation1] In this case, the water activity of an equilibrium saturated state in a given solute-solvent system should be known. Bhandari and Bareyre[Citation4] sucessfully developed a method to detect the crystalline fraction of glucose in a superstaurated glucose crystal-solution slurry. This was based on the fact that the difference of water activity between the superstaurated crystallized solution and the initial supersaturated solution is a function of amount of solids crystallized out. Zamora and Chirife[Citation5] also reported that change in water activity in honey corresponded to that caused by glucose crystallisation, but no empirical relationship was provided.
Lactose is a disaccharide and shows unique behaviour as compared to other common sugars such as sucrose, glucose, or fructose. In a supersaturated state, a lactose solution is unstable and if the supersaturation degree is high (in the labile area), a spontaneous crystallization of lactose can occur. In a solution, lactose exists in two interchangeable forms: α-lactose and β-lactose. The proportion of these two isomers depends on the temperature of the solution. The β form is more soluble than the α form (at 20°C the α:β proportion is about 38:62). The ratio increases with temperature (40:60 at 60°C and 43:57 at 100°C). These 2 forms equilibrate in a solution, but at temperatures below 93.5 °C, lactose crystallizes in the α-monohydrate form.[Citation6] Thus, it is important to understand the equilibrium condition of these two isomers. During crystallization, the equilibrium of α to β is maintained due to mutarotation.
Currently, the extent of crystallization of pure lactose or lactose-rich dairy products such as whey is determined by measuring the refractive index of the liquid phase being crystallized. Although this is the most convenient method, it may be difficult to give accurate and reliable results for thick and dark-coloured slurry or solutions. Thus, the objective for this article was to investigate the use of the water activity difference method to estimate the crystalline fraction present in the supersaturated lactose and whey crystal-solution mixtures. The water activity prediction models such as Raoults, Norrish, and Money-Born are also tested for their suitability to predict the water activity of a supersaturated solution of both dairy products.
MATERIALS AND METHODS
Determination of the Equilibration Time
As mentioned previously, lactose exists in 2 forms in solution: α-lactose and β-lactose. When α-lactose monohydrate is added to water, dissolution is limited by the solubility of the α-form and mutarotation to β form occurs to maintain the equilibrium. The time required to mutarotate α to β form depends on the temperature; therefore, α-lactose can become supersaturated instantaneously.[Citation7] Since the solubility of β-lactose is higher than that of α-lactose, the solubility of the mixture changes with time as the α form is converted to β form. According to the literature, the maximum solubility of lactose (α+β) in solution at 20°C is around 19 g 100 g−1 of water.[Citation8] However, the α-lactose at the initial stage will be soluble in much lesser quantity than this (about 7.4 g 100 g−1 water). In order to measure the time of equilibration of a lactose solution prepared from solid crystalline lactose, 20 g of α-lactose monohydrate (99% purity, 5% water. AnalaR, BDH Laboratory Supplies. Poole, BH15 ITD England) were mixed with 100 g of distilled water, and the solid content of the liquid phase was measured once per day with a refractometer. The lactose-water slurry was manually agitated intermittently and before each measurement during the entire period of equilibration.
Preparation of the Saturated Solution of Lactose
Based on the equilibration time determined in previous exercise, the saturated solutions of lactose was obtained by adding slightly excess amount of lactose monohydrates to 100 g of water (20°C) with constant agitation during 3 days to reach the final solubility value as verified with a refractometer. Excess crystals which were still in solution were removed by filtration (Whatman filter paper). This saturated lactose solution was maintained at 20°C for further experiments.
Addition of α-Lactose Monohydrate to the Saturated Solution
In order to prepare supersaturated solutions of lactose, various amounts (5, 10, 15, 20, 25, 30, 35 g) of α-lactose monohydrate were added to saturated lactose solutions. Then, these mixtures were heated in a water bath (Thermoline Scientific Equipment Pty. Ltd., QLD, Brisbane, Australia) at 60°C to dissolve the lactose and manually agitated every 5 minutes until total disappearance of lactose crystals. These solutions were prepared in bottles with lids on to avoid evaporation of water during heating in the water bath. At 60°C, the solubility of lactose was about 58.7 g of lactose 100 g1 of water.
The lactose content of lactose solutions was checked with a refractometer to verify if all crystals were dissolved. Then, the solutions were cooled down to 20°C to obtain supersaturated solutions. In our case, it is not important to maintain the equilibrium between α and β forms above saturation level so long as it does not contain any crystals, although it is not reported elsewhere how the equilibrium between α and β forms are maintained in thermodynamically non-equilibrium supersaturated solution. The water activity of all solutions was measured at 20°C. We observed no crystallisation within the period of cooling or water activity measurement of these supersaturated solution. All the experiments were undertaken in triplicate.
Preparation of Supersaturated Lactose Solution from Whey Powder
Whey powder (75% lactose, w/w) was obtained from Dairy Farmer's Unity Whey Powder, Lidcombe, NSW, Australia. The quantities of whey powder dissolved in water were calculated in order to give the same amount of lactose as in the experiment with pure lactose described previously. Thus, 32.6, 39.3, 45.8, 52.5, 59.2, 65.8, 72.5 g of whey powder were dissolved in 100 g of water at 60°C. Whey solutions were maintained for the same time as in the case of lactose solution preparation to assure that all the lactose crystals were dissolved. Then, solutions were cooled down to 20°C to measure the water activity at the same temperature. All the experiments were undertaken in triplicate.
Determination of Water Activity
All the water activity measurements of saturated and supersaturated lactose and whey solutions were performed using Aqualab 3TE (Decagon Devices Inc., Pullman, WA 99163, USA) water activity meter which had a sensitivity of 0.001. Each water activity measurement was made in triplicate at 20°C. The Aqualab was calibrated with a standard KCI solution (0.5 M in water, aw = 0.984) furnished and following the procedure given by the manufacturer. There was no variation of the water activity of the triplicate representative samples measured at different times once the equipment was calibrated.
RESULTS AND DISCUSSION
Equilibration Time of Lactose in Solution
The dissolution of α-lactose involves three sequential processes: initial dissolution; mutarotation of some α-lactose to the β form to maintain the equilibration; further dissolution of the α-lactose monohydrate due to its mutarotation to more soluble β-form. The mutarotation to β-form is a slow process; therefore, it requires a study to determine the time needed to reach this equilibration. shows that the solubility of the lactose increased continuously until 3 days and remained constant there after. This suggests that the time required to reach the equilibration is around 3 days at 20°C. The water activity will be independent of the α or β form. In the same , it is shown that the water activity of the solution decreased with the time as more lactose dissolved in the solution. The water activities were also calculated by using the Norrish equation.[Citation4]
Figure 1 Changes in the solubility of lactose and water activity of the lactose solution over time (at 20°C). The values presented are the average of three replications (measured values were the same within replicates).
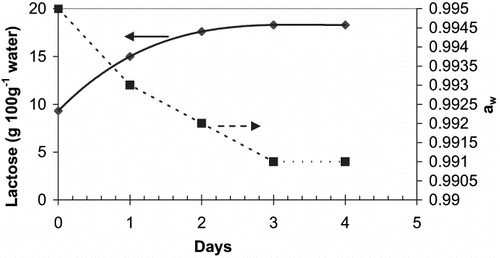
The Brix value measured with a refractometer at the equilibrium was 15.5% (w/w) which corresponds to approximately 18.34 g lactose per 100 g of water or 19.5 g α-lactose monohydrates per 100 g of water. This solubility value was slightly lower than the literature values reported (19.2 g 100 g−1 water). Considering this result, all lactose or whey solutions were kept at least for 3 days at 20°C to reach equilibrium.
Water Activity of Saturated Lactose and Whey Solution
The difference between chemical potentials of the solution components (solute-solvent) of the supersaturated lactose solution induce crystallisation, which can occur very quickly depending on the supersaturation degree of the solution. That crystallisation continued until the equilibrium of the chemical potentials reaches the saturation state of the solution. At the supersaturation state, lactose-water molecules interactions result in a lower water vapour pressure above the solution than at the saturation state. Thus, during the crystallization, the water activity of the solution increased until its limit, which corresponds to the saturated state. Regardless of presence of crystals, the water activity of the saturated solution will always be the same. At the equivalent concentration of lactose, this limiting water activity at saturation at 20°C was found to be 0.989 for a lactose solution, and 0.980 for a whey powder solution (). The water activity of whey was, thus, less than that of the lactose solution. This is possibly due to the water activity depressing effect of minerals present in the whey. Whey powder contains water activity reducing agents like minerals such as phosphorus, potassium, calcium and magnesium which accounts for up to 8% of the total solids (w/w). At saturation, the difference in water activity between whey and lactose solution was 0.009; this value increased to 0.019 for 50.4 g of anhydrous lactose 100 g−1 of water (supersaturation state). The difference increases with the lactose content because the more is added whey, the more there will be water activity reducing agents in the solution. The effect of presence of large protein molecules in the presence of excess water on water activity can be considered negligible.[Citation9]
Water Activity of Supersaturated Solutions of Lactose and Whey and Linear Prediction Model
Saturated lactose solutions with various amounts of dissolved α-lactose monohydrate solids to obtain a supersaturated state showed a good linear correlation (R2 = 0.9954. ) between the lactose content and the water activity. The water activity decreased as the lactose content in the supersaturated solution increased. Thus, it is possible to predict the lactose crystallisation rate in a random mixture of supersaturated lactose-crystal-solution slurry using the equation derived from the data in . If the water activity of the crystallized solution at any time and supersaturation solution prior to crystallization (or by redissolving the crystals) are known, we can predict how much lactose crystals are present in the solution at that particular time by using the following EquationEq. 1:
Here, ΔL is the amount of lactose crystals (g 100g−1 water) that is in the crystal-solution mixture at a given time: aw1 is the water activity of the supersaturated crystal-solution mixture at the same time at 20°C; and, aw2 is the water activity of the supersaturated solution after dissolution of crystals at the same temperature.
In order to verify the reliablility of the linear model, a supersaturated solution was prepared dissolving 54.5 g of α-lactose monohydrates in water at 60°C followed by cooling to 20°C. This solution was then kept at 20°C for 6 days to allow the excess lactose to crystallize out over this time. The solid content and water activity of the solution were measured once a day. shows experimental water activity and expected water activity calculated by the linear model. From this table, it can be seen that the lactose content in the solution decreases while lactose is being crystallized out. The predicted and measured water activity values were very close [Mean Absolute Error (MAE): 0.037%]. The linear model thus appears to fit very well and can accurately predict the lactose crystalline fraction in a crystal-lactose mixture. This experiment was not undertaken for whey because the solution was too opaque to measure the solid content by refractometer.
Table 1 Measured and predicted water activities of crystallizing solution of supersaturated lactose and whey
Prediction Model for the Crystalline Lactose Fraction in Whey Solution
A similar experiment was undertaken with the whey solutions containing the same amount of lactose as in the case of pure lactose solutions of the previous experiment. The linear model also demonstrated a strong correlation (R2 = 0.9973. ) between the water activity and the crystallized lactose content (EquationEq. 2).
Where ΔL is the amount of lactose crystals in the supersaturated lactose whey solution at the beginning; aw1 is the water activity of whey solution at 20°C with crystals; and, aw2 is the water activity of the lactose supersaturated whey solution.
The slope of the line for whey solutions was less steep than the lactose one (in EquationEq. 1). Inverse of the slope (1 slope−1) indicates the change in the water activity per unit amount of lactose crystallized out. The inverse of slope for whey is higher than that for the pure lactose solution. Since whey contains components other than lactose, this variation is expected to be due to lactose-solvent-minerals interactions in the solution. Bhandari and Bareyre[Citation1] reported an even less steep slope (846.97) for glucose indicating that the change in the water activity will be higher for a monosaccharide than for a disaccharide, for the same amount of solids crystallized out.
In the dairy industry, the extent of crystallisation of lactose is usually measured by a refractometer. However, this method is not reliable for solutions containing a heavy amount of crystals or dark-coloured solutions. Thus, the method described here can be useful to quantify the amount of lactose crystals present in such mixtures. It should be kept in mind, however, that the sensitivity of the water activity meter will still be a critical factor. Use of higher precision water activity meters (< 0.001) will provide even better predictability.
Generic Prediction Models for Water Activity of Supersaturated Lactose and Whey Solutions
Three common models were tested to predict the water activity of supersaturated lactose solutions: Raoult's law. Norrish equation, and Money-Born equations.
Raoult's law
Raoult's law is based on the fact that the water activity of an ideal solution at low solute concentration is equal to the mole fraction of water in the solution. At higher solute concentration, a correction factor may apply. In the case of lactose solutions, the mean absolute percentage error was quite low (MAE: 0.28%, ), and this model overestimated the water activity of lactose solution. For whey solutions, the mean absolute error was higher (MAE: 1.71%, ) than the errors obtained from other models. Raoult's law again overestimated the water activity. This can be explained by the effect of other water activity reducing agents (such as minerals) present in the whey. Because of the unknown composition of these compounds, it is difficult to incorporate the effect of these compounds into Raoult's law calculation.
Table 2 Water activity prediction of supersaturated lactose solutions by Raoult, Norrish, and Money-Born models
Norrish equation
The Norrish equation takes into account each mole fraction of the components of the solution to calculate the water activity. It can be calculated using the following EquationEq. 3.[Citation10]
where, Xw is the water mole fraction; ki the Norrish constant for the solute i; and, Xsi, the mole fraction of the solute i. The k value used for lactose is −10.29. The Norrish model was the best of the three predictive models tested for lactose solutions (MAE lactose: 0.12%. ). It could be used to predict the water activity of supersaturated lactose solutions. For whey solutions, this model overestimates the water activity values (MAE: 1.38%, ) as compared to Money-Born equation.
Money-Born equation
Money-Born equation is an empirical model inspired by the Raoult's law which states that the water vapour pressure above the solution is inversly proportional to the solute concentration. The water activity can be calculated with the following EquationEq. 4.[Citation4]
where, Nsi is the mole of the solute i per gram of water in solution. This model showed the highest mean absolute error % among three models for lactose solutions (MAE lactose: 0.58%, ) and underestimated the water activity. But for whey solutions, this was the best (MAE: 0.83%, ) as compared to other two.
The Norrish model appears to be the most reliable of the three models tested for the prediction of the water activity of supersaturated lactose solutions, but for simplicity of calculation, the linear model can be more useful, particularly if used for predicting the crystallization fraction in a mixture. For whey solutions, those three models will not be as accurate because they do not take into account of the other water activity reducing agents contained in the whey. The linear model was accurate and reliable for prediction of the water activity of supersaturated solution.
CONCLUSION
The crystalline fraction of lactose in supersaturated lactose and whey solutions can be determined by measuring the water activity before and after the dissolution of crystals contained in solutions and using the linear models given in this article. This method is reliable, can be replicated, and the calculation of the crystalline fraction is very simple.
REFERENCES
- Hartel , R.W. and Shastry , A.V. 1991 . Sugar Crystallisation in Food Products . Critical Reviews in Food Science and Nutrition , 1 ( 1 ) : 49 – 112 .
- Bhandari , B.R. , D'Arcy , B.R. , Kelly , C. and and , Rheology . 1999 . Crystallisation Kinetics of Honey: Present Status . International Journal of Food Properties , 2 ( 3 ) : 217 – 226 .
- Haneock , B.C. and Zografi , G. 1997 . Characteristies and Significance of the Amorphous State in Pharmaceutical Systems . Journal of Pharmaceutical Science , 86 : 1 – 12 .
- Bhandari , B. and Bareyre , I. 2003 . Estimation of Crystalline Phase Present in the Glucose Crystal-Solution Mixture by Water Activity Measurement . Lebensmittel-Wissenchaft und-Technologie , 36 ( 7 ) : 729 – 733 .
- Zamora , M.C. and Chirife , J. 2006 . Determination of Water Activity Change Due to Crystallization in Honeys from Argentina . Food Control , 17 : 59 – 64 .
- Visser , R.A. 1981 . Supersaturation of α-Lactose in Aqueous Solutions in Mautarotation Equilibrium . Netherlands Milk Dairy Journal , 36 : 89 – 101 .
- Lowe , E.K. and Paterson , A.H.J. 1998 . A Mathematical Model for Lactose Dissolution, part II. Dissolution Below the Alpha Lactose Solubility Limit . Journal of Food Engineering , 38 ( 1 ) : 15 – 25 .
- Raghavan , S.L. , Ristic , R.I. , Sheen , D.B. and Sherwood , J.N. 2000 . The Bulk Crystallization of α-Lactose Monohydrate from Aqueous Solution . Journal of Pharmaceutical Sciences , 90 ( 7 ) : 823 – 832 .
- Chirife , J. , Resnik , S.L. and Ferro-Fontan , C. 1985 . Application of Ross Equation for Prediction of Water Activity in Intermediate Moisture Food Systems Containing a Non-Solute Solid . Journal of Food Technology , 20 ( 6 ) : 773 – 779 .
- Labuza , T.P. 1984 . Moisture Sorption: Practical Aspects of Isotherm Measurement and Use , St. Paul, MN : American Association of Cereal Chemists . 1992.