Abstract
Differential scanning calorimetry study of frozen state transitions of mixtures of lactose, proteins, cornstarch, and water revealed that maximum freeze-concentration was achieved by annealing at temperatures T′m-1. The onsets of glass transition of maximally freeze-concentrated solids, T′g, were lower and onsets of ice melting, T′m, were higher for mixtures of proteins and polysaccharide than those of lactose. The established state diagrams showed solids concentrations C′g, of the maximally freeze-concentrated systems of, 82, 78, 78, 78, and 75% for lactose, lactose/albumin, lactose/gelatin, lactose/cornstarch, and lactose/cornstarch/gelatin solutions, respectively. The state diagrams established with experimental and predicted Tg values are useful in characterization of thermal phenomena and physical state of the systems at various water contents and in the freeze-concentrated state.
INTRODUCTION
The stability of frozen foods depends on the physical state of their constituent solids. Frozen food matrices are divided into two phases: (i) crystallized ice; and (ii) unfrozen solutes phase.[Citation1,Citation2] The solids constituents of frozen food matrix concentrated in an amorphous unfrozen solute phase.[Citation1,Citation2,Citation3,Citation4,Citation5,Citation6,Citation7] The amorphous state is a nonequilibrium state and undergoes various time-dependent changes.[Citation1,Citation2,Citation3,Citation4,Citation6,Citation8,Citation9] Frozen foods may exhibit stability if maximum amount of ice is separated and a maximally freeze-concentrated state is formed.[Citation10,Citation3]
Transitions of amorphous solute phase which have been used to define the quality of frozen foods are glass transition Tg′ and onset of ice melting Tm′, temperatures in maximally freeze concentrated state.[Citation4,Citation11,Citation12,Citation13,Citation14,Citation15] The onset of ice melting in a maximally freeze-concentrated system, Tm′, was considered as glass transition of the maximally freeze-concentrated solids, Tg′, by some authors.[Citation10] The Tg′ depends on composition of a frozen system.[Citation6,Citation16,Citation17,Citation18] The researchers [Citation4,Citation19] proposed annealing for maximal freeze-concentration in a frozen system.
Differential scanning calorimetry (DSC), dynamic mechanical thermal analysis (DMTA), dynamic mechanical analysis (DMA) and dielectrical thermal analyser (DETA) have been commonly used for detecting state transitions of frozen systems.[Citation20,Citation21] Typical thermal behaviour of maximally freeze-concentrated systems shows constant Tg′ and Tm′ values, and no devitrification exotherm.[Citation4,Citation22,23] Where as non-annealed, rapidly cooled frozen systems may retain high amounts of unfrozen water in an unfrozen solute phase. Such partially freeze-concentrated systems show a low Tg value and often a devitrification peak above Tm′.[Citation4,Citation6,Citation12,Citation22,Citation7]
State diagrams are often used to describe the physical state and to determine the concentration of solutes, Cg′, in the maximally freeze-concentrated unfrozen phase.[Citation4,Citation10,Citation12,Citation13] State diagrams also serve as “stability maps” for frozen and freeze-dried foods.[Citation6,Citation8] It has been described by [Citation13,Citation15] that below the Tg′ the viscosity becomes high enough to kinetically reduce rates of chemical reactions. An increase in temperature above the Tg′ may result in ice melting and increase the amount of unfrozen water and decrease viscosity.[Citation15,Citation18] An increased molecular mobility causes a decrease to viscosity and leads to enhanced rates of diffusion-controlled reactions.[Citation15,Citation18,Citation24,Citation25]
Studies of carbohydrate, carbohydrate/polysaccharide, and carbohydrate/protein mixtures as model systems help to understand the thermal behaviour of several categories of frozen foods, such as fruits, frozen dairy desserts, and other types of sugar-protein food systems.[Citation7] For example Tg′ and Tm′ can be used to relate loss of quality including changes in texture, aroma as well as caking and stickiness of freeze-dried foods.[Citation10,Citation11,Citation26,Citation27] The present study was carried out with the objectives to investigate frozen state transitions of lactose, lactose/albumen, lactose/gelatin, lactose/cornstarch, and lactose/cornstarch/gelatin model systems and to understand effects of the presence of food polymers on ice formation in a lactose system.
MATERIALS AND METHODS
Materials
α-lactose, gelatin (type B: from bovine skin), egg albumin (chicken egg, grade II), and corn starch were products of Sigma-Aldrich Chemie Gmbh, 89552 Steinheim, Germany. These and distilled water were used in the experiments.
Preparation of Solutions
Lactose, albumin, gelatin, and cornstarch were weighed with water to prepare solutions of 10%, 20%, and 30% of total solids (w/w). All model systems had different components in an equal ratio of lactose, proteins and cornstarch that is sugar/protein (1:1), sugar/cornstarch (1:1), and sugar/protein/cornstarch (1:1:1). Water bath at 60°C was used to obtain clear solutions of lactose and lactose/gelatin mixture. For making lactose/albumen solutions, the heated lactose solutions were cooled to room temperature after which egg albumen was added, and the mixture was well stirred to obtain a clear solution at room temperature. Lactose/gelatin mixture was rewarmed using water bath to facilitate filling of DSC pans for thermal analysis and glass vials for freeze-drying. Mixtures of lactose/cornstarch samples were heated in water bath slightly above 85°C and held at this temperature for 5 min to complete gelatinization of starch before transfer to freeze-drying glass vials. All solutions were cooled at room temperature and any water lost as a result of evaporation was added back on an analytical balance. Samples with cornstarch for DSC were gelatinized in DSC furnace before the study of their thermal behaviour.
Preparation of Anhydrous Samples
For preparation of anhydrous samples, petri dishes were used. Aliquots of model solutions were filled into petri dishes up to an approximate layer thickness of 0.5–1 cm. Samples in petri dishes were immediately pre-frozen at −20°C for 12 h and then transferred to a −80°C freezer for 24 h. From −80°C freezer, samples were rapidly transferred to a freeze-drier (within less than 1 min). The materials in petri plates were freeze-dried for 3 days at ice temperature of −45°C and corresponding to chamber pressure < 0.1 mbar using a Finn-Aqua Lyovac GT2 (Germany) freeze-drier. The freeze-dried samples were stored for 5 days at room temperature in vacuum desiccators over P2O5 for further dehydration. After P2O5 treatment, samples were crushed to a fine powder with a mortar and pestle. Powdered samples of 5–25 mg were filled in DSC pans and placed again over P2O5 at room temperature for 3 days for further removal of residual moisture.
Differential Scanning Calorimetry (DSC)
Phase and state transitions of amorphous lactose, lactose/protein (1:1), lactose/cornstarch (1:1), and lactose/protein/cornstarch (1:1:1) mixtures were analysed by using differential scanning calorimetry (DSC, Mettler Toledo 821e with liquid N2 cooling). The instrument was calibrated for heat flow and temperature using n-hexane (m.p. −95°C, ΔH m 151.8 Jg−1), gallium (m.p. 29.8°C, ΔH m 80 Jg−1), water (m.p. 0°C, ΔH m 334.5 Jg−1), indium (m.p.156.6°C, ΔH m 28.45 Jg−1), and mercury (m.p. −38.8, ΔH m 11.4 Jg−1).[Citation28] The thermograms were analysed for onset temperature of glass transition, Tg′, onset temperature of ice melting, Tm′, and latent heat of melting (ΔHm) using Stare, version 6.0 (Mettler Toledo, Switzerland) thermal analysis software as reported by Roos and Karel.[Citation11] The glass transition temperature of the maximally freeze concentrated phase, Tg′, is an endothermic change as a result of a change in heat capacity and it was determined from the intersection of tangents to the baseline.[Citation11,Citation12] The onset temperature of the transition preceding the ice melting endotherm was defined as the ice melting onset temperature, Tm′,[Citation2,Citation3,Citation4,Citation11,Citation12] and its value was determined from the intersection of the tangents to the baseline and to the leading side of the transition.[Citation2,Citation3,Citation4,Citation11,Citation12,Citation25]
To determine frozen state behaviour, samples (10–35 mg) of each material were accurately weighed using Mettler Toledo AG245 balance into 40 μl DSC aluminum pans (Mettler Toledo 27331, Switzerland). Pans with samples were hermetically sealed. An empty aluminum pan was used as a reference in all measurements. Before cooling in the calorimetric cell, the samples with cornstarch were first heated to 85°C and isothermally held at this temperature for 5 min to complete gelatinization of starch.
Annealing procedure
Authors[Citation2,Citation11] reported that annealing of frozen system slightly below Tm′ allowed maximum ice formation and gave constant Tg′, Tm′ and Cg′ for frozen systems. We used annealing procedure as described by,[Citation28] for maximal freeze concentration in our samples.
To determine Tg, 5–25 mg of freeze-dried powdered samples were stored in open DSC pans for 144 h at room temperature in evacuated desiccators over P2O5 and various saturated salt solutions: LiCl, CH3COOK, MgCl2, K2CO3, Mg(NO3)2, NaNO2, and NaCl (Sigma-Aldrich Chemie Gmbh, 89552 Steinheim, Germany) with respective relative water vapor pressures (RVP) of 11.4, 23.1, 33.2, 44.1, 54.5, 65.6, and 76.1%, giving an aw of 0.01 × percentage of RVP at equilibrium, as used by.[Citation28] Water activity of the saturated salt solutions was confirmed with an Aqua Lab CX-2 (Decagon Devices, Inc. Pullman, Washington, USA) water activity meter. After equilibration, the pans were hermitically sealed. Triplicate samples of each material were analysed.
The samples were scanned first over the glass transition temperature range at 5°C min−1, followed by cooling at 10°C min−1 to below glass transition, and a second heating scan at 5°C/min was run to well above the glass transition temperature. Anhydrous samples were scanned using pans with punctured lids to allow evaporation of any residual water during the measurement. Samples with different water contents were scanned in hermetically sealed pans. Transition temperatures (Tg onset) were determined using STARe thermal analysis software, version 6.0 (Mettler Toledo, Switzerland).
The Gordon and Taylor equation[Citation29] was fitted to the experimental Tg data as reported by.[Citation28] The value for k was the mean derived from the experimental data on Tg of various water contents calculated as reported by Jouppila and Roos.[Citation21] The Gordon and Taylor equation was applied in this study for predicting water plasticization of lactose, lactose/protein, lactose/cornstarch and lactose/protein/cornstarch mixtures, considering that all solid components contributing to the observed glass transition were miscible and formed a single phase.[Citation13,Citation28,Citation30]
Statistical Analysis
A 1-way ANOVA followed by multiple range (LSD) test were performed using SPSS 11.0 (SPSS Inc., Chicago, III., U.S.A.) to compare the Tg, Tg′, and Tm′ values of 5 different compositions. The level of confidence required for significance was selected as P ≤ 0.05.
RESULTS AND DISCUSSION
Effect of Water on Glass Transition
The Tg value of amorphous sugars or food products containing such sugars at low water contents can be used in stability control.[Citation6,Citation22,Citation31] The Tg of anhydrous amorphous lactose was found at 105°C, being slightly higher than was reported by.[Citation2,Citation6,Citation32] Hill et al.[Citation33] reported an onset Tg value for lactose at 114, 113, and 83°C for open pan, non-hermetic sealed pans, and hermetic sealed pans respectively. The higher Tg for punctured, open or non-hermetically sealed pans was explained by loss of residual water from samples during a DSC analysis as a result of evaporation.[Citation28,Citation30,Citation33]
It was observed that all model systems showed single glass transition after second scan. Matveev et al.[Citation34] reported that in a heterogeneous multi-component food systems one glass transition may indicate compatibility and miscibility of components. We found that lactose/albumin, lactose/gelatin, lactose/cornstarch and lactose/cornstarch/gelatin mixtures had a significantly (P ≤ 0.05) different Tg values. It was also found that the Tg values of all model systems were significantly (P ≤ 0.05) different from the Tg value of lactose. All systems had higher Tg than that of lactose in their anhydrous state. The higher Tg of mixtures of lactose/proteins/polysaccharide was expected as the higher molecular weight polymeric compounds increase the average molecular weight of the system. Similar observation was reported by several authors for mixtures of sugars and polymeric substances.[Citation15,Citation28,Citation30,Citation35,Citation36]
It has been widely reported in literature that water is a plasticizer and it reduces the Tg of a hydrophilic system. Similar observations we made for our freeze-dried amorphous model systems, lactose/proteins, lactose/polysaccharide and lactose/polysaccharide/proteins.
In DSC analysis, an endothermic relaxation peak was associated with the glass transition of pure lactose, even in an immediate rescan. Such relaxation was not observed in lactose/protein and lactose/cornstarch mixtures ().
Figure 1 DSC thermograms (Second scan) of 20% freeze-dried anhydrous, lactose, lactose/albumin (1:1), lactose/gelatin (1:1), lactose/cornstarch (1:1), and lactose/gelatin/cornstarch (1:1:1). The arrow indicates the onset of Tg.
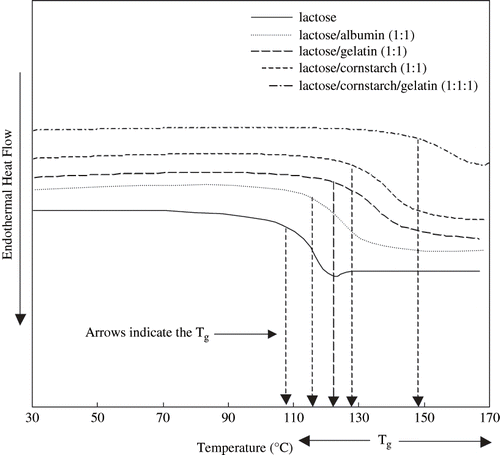
The experimental Tg values of lactose, lactose/albumin, lactose/gelatin, lactose/cornstarch, and lactose/cornstarch/gelatin mixtures were successfully predicted using the Gordon-Taylor equation. The Tg values followed the curve predicted by the Gordon-Taylor equation at various sorbed water contents after exposure to RVP ranging from 0 to 0.76%. The constant, k, for lactose, lactose/albumin, lactose/gelatin, lactose/cornstarch, and lactose/cornstarch/gelatin mixtures were 6.5, 6.4, 6.6, 6.5, and 5.5, respectively. Water plasticization properties of lactose and lactose protein and cornstarch mixtures with their respective experimental data are shown in .
Figure 2 State diagrams for lactose, lactose/albumin (1:1), lactose/gelatin (1:1), lactose/cornstarch, and lactose/cornstarch/gelatin (1:1:1). Tg curve predicted by the Gordon and Taylor equation (Gordon and Taylor, 1952). Below Tg all solutions are in the glassy state. Maximally freeze concentrated solutions show constant Tg′ (onset of glass transition) and Tm′ (onset ice melting temperature) values. The Tm′ of solutions was at the endpoint region of Tg′. All solution show maximum concentration of unfrozen phase at Cg′.
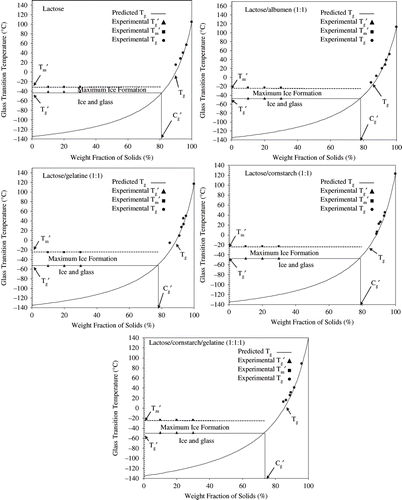
Thermal Behavior of Frozen Systems
It has been reported that freeze-concentrated frozen systems have constant initial concentration independent Tg′ and Tm′.[Citation2,Citation4,Citation12] The importance of freeze-concentration has been described in terms of frozen food stability by different authors.[Citation6,Citation13,Citation22,Citation14,Citation15,Citation24,Citation25,Citation37] We found that isothermal annealing of model systems at a temperature Tm′−1 () gave concentration independent, constant Tg′, and Tm′ values. The thermal graphs of annealed solutions did not show devitrification exotherm on rewarming (), which was another indication of maximal freeze-concentration in the systems. Similar characteristics for a maximally freeze-concentrated system had been defined by.[Citation22] We found that Tg′ and Tm′ values of lactose solutions () agreed with previously reported data[Citation6,Citation12,Citation13] and were significantly (P ≤ 0.05) different from lactose/polymer systems ().
Table 1 Anhydrous onset of glass transition temperature (Tg) as well as onset of glass transition temperature (Tg′), onset of ice melting temperature (Tm′), and solute concentration value (Cg′) of maximally freeze-concentrated solutions of lactose, lactose protein, and lactose cornstarch mixtures. A 15 minute annealing time was used for all solutions
Figure 3 Thermograms of 20%, lactose, lactose/albumin (1:1), lactose/gelatin (1:1), lactose/cornstarch (1:1), and lactose/gelatin/cornstarch (1:1:1) solutions isothermally annealed for 15 minutes before determination of the transitions during rewarming from −100 to 20°C at 5°C min−1.
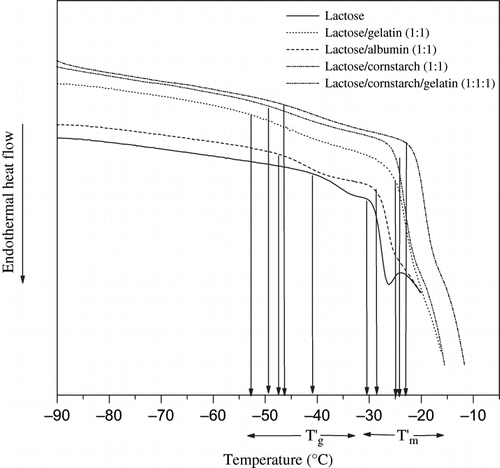
Lactose and sucrose are widely used in mixtures in frozen dairy products with proteins and polysaccharide. Researchers[Citation7] described that a study of sugars in mixed model systems with proteins and polysaccharides may be the best way to model the thermal behaviour of several categories of frozen foods, such as frozen fruits and frozen dairy desserts. For example the physical properties of frozen systems, like firmness or texture in dairy products are strongly affected by sugars.[Citation2,Citation6,Citation7,Citation32] We found that lactose-protein-cornstarch model systems behaved significantly differently from lactose solutions. However, one observation common to all model systems was that the Tg′ value of lactose/polymer solutions was lower and the Tm′ value was higher than those of lactose solutions (). Hence, the transition temperature range (between Tg′ and Tm′) of the mixtures was broader (). The comparison between the model systems showed that lactose/gelatin had the highest broadness of the transition range, where as albumin had the lowest broadness of the transition range. The broadness of the transition range in the presence of high molecular weight polymeric materials was also reported by other authors .[Citation12,Citation14,Citation15,Citation25,Citation28,Citation34,Citation37,Citation39] We assumed that broadness of transitions may be due to the high viscosity of unfrozen phase during melting, which produces restriction toward melting.
Another important process, which is directly related to high viscosity of unfrozen phase is ice formation.[Citation11] reported that high viscosity of unfrozen phase at a low temperature led to less ice formation in a frozen system and resulted in lower Tg′ value. Various authors[Citation2,Citation14,Citation37,Citation40] reported that polymeric substances increased viscosity of unfrozen phase at lower temperatures, which kinetically restricted ice formation in carbohydrate solutions. The positive affects of high viscosity of an unfrozen phase described by authors[Citation7,Citation37,Citation38,Citation41,Citation42] in terms of stabilization of the ice phase and prevent ice recrystallization in frozen products. Whereas[Citation25] described the importance of high viscosity of an unfrozen phase in controlling diffusion controlled reactions in frozen foods.
The properties of polymeric materials at lower temperatures, which affect ice formation in frozen systems have been described by several authors. The authors[Citation10,Citation43] suggested that at low temperatures polymeric substances undergo chain entanglement, leading to formation of a visco-elastic network around ice crystals and gelation. The authors[Citation44] recently reported that possible mechanism behind gelation of polymers is steric blocking of the interface or in other words inhibition of solute transport to and from the ice interface.
In state diagram a point of intersection of Tg′ curve on Tg curve and corresponding value at x-axis (percent of weight fraction of solids) gives a Cg′ value or level of maximum freeze-concentration in a system (). Our results showed that Tg at varying water contents could be described by Gordon and Taylor equation as reported by.[Citation28] We found that all model systems showed lower values to Cg′ than lactose, which suggested that a critical viscosity value of the unfrozen phase controlled the extent of concentration. It has been reported that the predicted value of viscosity in glassy unfrozen phase may be 1011–1012 Pas which does not allow ice formation.[Citation8,Citation10,Citation22] Further, it was predicted that in carbohydrate solution minimum viscosity of unfrozen phase required for ice formation was 107 Pas, but at this viscosity ice formation was very slow.[Citation22]
It was reported that polymeric components such as polysaccharides increase ice nucleation in frozen systems, but at the same time increased viscosity of unfrozen phase may play a role to control ice formation.[Citation10,Citation37,Citation45] Further, it has been described that increased viscosity of the unfrozen phase limits diffusion of water to the surface of growing ice crystals, this also supports the critical viscosity concept. We found that maximally freeze-concentrated lactose had Cg′ value of 82%, which was higher than the mixed model systems of lactose/albumin, lactose/gelatin, lactose/cornstarch, and lactose/cornstarch/gelatin. The model systems lactose/albumin, lactose/gelatin, lactose/cornstarch had Cg′ value of 78%, where as lactose/cornstarch/gelatin had Cg′ value of 75% (, Table-1). The Cg′ of lactose solutions was similar as reported by[Citation6,Citation12,Citation13] and slightly less than Cruz et al.[Citation46] who reported Cg′ value of lactose 83.3%. A C′g value of 72.9% and 73% for cornstarch was reported[Citation12,Citation21] respectively. The higher Cg′ value of lactose than lactose/albumen, lactose/gelatin, lactose/cornstarch, and lactose/cornstarch/gelatin indicated lower concentration of solute in unfrozen phase and delayed ice formation during annealing than lactose. It was defined by[Citation6,Citation10,Citation24,Citation25] that high amounts of unfrozen water or less concentration of solutes in unfrozen phase may results in physicochemical reactions to occur in frozen systems and hence deteriorate the quality of frozen foods.
CONCLUSIONS
Study of frozen foods as model food systems can be used to predict the physical state of constituent components in frozen foods. Annealing at Tm′−1 allowed maximal freeze-concentration in carbohydrate/polymers solutions and gave constant Tg′ and Tm′ values, and no devitrification peak was observed. The polymeric components in lactose solutions tend to reduce the onset of Tg′ and increase the Tm′ values and the solutions therefore showed broad transitions. Broad transitions suggested resistance of amorphous phase to melting. The Tg′ and Tm′ values of all model systems were significantly different (P ≤ 0.05) from each other. Where as the Tg′ value of the lactose/albumin, lactose/cornstarch, and lactose/cornstarch/gelatin showed non-significant differences (P ≤ 0.05). There was no indication of devitrification in lactose/polymer mixed systems. The state diagrams based on the experimental data and predicted Tg values gave valuable information for studies of physical behaviour of lactose, lactose/albumen, lactose/gelatin, lactose/cornstarch and lactose/gelatin/cornstarch systems. At Tg′ lactose solution had the highest value, 82% for Cg′ and higher than lactose/polymer mixed systems. The lactose/polymer solutions, lactose/albumin, lactose/gelatin, lactose/cornstarch, gave Cg′ values of 78% at Tg′, where as lactose/cornstarch/gelatin system gave a Cg′ value of 75%. The lower Cg′ value of lactose/polymer mixed systems than of lactose solution suggested a lower level of freeze-concentration.
REFERENCES
- Simatos , D. , Faure , M. , Bonjour , E. and Couach , M. 1975 . The Physical State of Water at Low Temperatures in Plasma with Different Water Contents as Studied by Differential Thermal Analysis and Differential Scanning as Studied by Differential Thermal Analysis and Differential Scanning Calorimetry . Cryobiology , 12 : 202 – 208 .
- Roos , Y.H. and Karel , M. 1991 . Amorphous State and Delayed Ice Formation in Sucrose Solutions . Int. J. Food Sci. and Technol. , 26 : 553 – 566 .
- Roos , Y.H. and Karel , M. 1991 . Nonequilibrium Ice Formation in Carbohydrate Solutions . Cryo-lett. , 12 : 367 – 376 .
- Roos , Y.H. and Karel , M. 1991 . Water and Molecular Weight Effects on Glass Transitions in Amorphous Carbohydrates and Carbohydrate Solution . J. Food Sci. , 56 ( 6 ) : 1676 – 1681 .
- Le Meste , M. , Champion , D. , Roudaut , G. , Blond , G. and Simatos , D. 2002 . Glass Transition and Food Technology : A Critical Appraisal . J. Food Sci. , 67 ( 7 ) : 2444 – 2458 .
- Roos , Y.H. 1995 . Phase Transitions in Foods , 360 San Diego, CA : Academic Press .
- Goff , H.D. and Sahagian , M.E. 1996 . Glass Transitions in Aqueous Carbohydrate Solutions and Their Relevance to Frozen Food Stability . Thermonica Acta. , 280/281 : 449 – 464 .
- Rahman , M.S. 2004 . State Diagram of Date Flesh Using Differential Scanning Calorimetry (DSC) . International Journal of Food Properties , 7 ( 3 ) : 407 – 428 .
- Slade , L. and Levine , H. 1991 . Beyond Water Activity: Recent Advances Based on an Alternative Approach to the Assessment of Food Quality and Safety . Crit. Rev. Food Sci. Nutr. , 30 : 115 – 359 .
- Levine , H. and Slade , L. 1988 . Principle of “Cryostabilization” Technology from Structure/property Relationships of Carbohydrate/water Systems-A Review . Cyro-Lett. , 9 : 21 – 63 .
- Roos , Y.H. and Karel , M. 1991 . Phase Transitions of Mixtures of Amorphous Polysaccharides and Sugars . Biotechnol Prog. , 7 : 49 – 53 .
- Roos , Y.H. and Karel , M. 1991 . Phase Transitions of Amorphous Sucrose and Frozen Sucrose Solutions . J Food Sci. , 56 ( 1 ) : 266 – 267 .
- Roos , Y.H. 1995 . Characterization of Food Polymers Using State Diagrams . J. Food Eng. , 24 : 339 – 360 .
- Blond , G. and Simatos , D. 1998 . Optimal Thermal Treatments to Obtain Reproducible DSC Thermograms with Sucrose + Dextran Frozen Solutions . Food Hydrocolloids , 12 : 133 – 139 .
- Biliaderis , C.G , Swan , R.S. and Arvanitoyannis , I. 1999 . Physicochemical Properties of Commercial Starch Hydrolyzates in the Frozen State . Food Chem. , 64 : 537 – 546 .
- Iglesias , H.A. and Chirife , J. 1978 . Delayed Crystallization of Amorphous Sucrose in Humidified Freeze Dried Model Systems . J. Food Technol. , 13 : 137 – 144 .
- Simatos , D. and Karel , M. 1988 . “ Characterization of the Condition of Water in Foods Physicochemical Aspects ” . In Food Preservation by Moisture Control , Edited by: Seow , C.C. 1 – 39 . London : Elsevier Applied Science .
- Le Meste , M. and Huang , V. 1992 . Thermomechanical Properties of Frozen Sucrose Solutions . J. Food Sci. , 57 ( 5 ) : 1230 – 1233 .
- Roos , Y.H. , Karel , M. and Kokini , J.L. 1996 . Glass Transitions in Low Moisture and Frozen Foods: Effects on Shelf Life and Quality . Food Technol. , 50 ( 11 ) : 95 – 108 .
- Roos , Y.H. 1987 . Effect of Moisture on the Thermal Behaviour of Strawberries Studied Using Differential Scanning Calorimetry . J. Food Sci. , 52 ( 1 ) : 146 – 149 .
- Jouppila , K. and Roos , Y.H. 1994 . Glass Transition and Crystallization in Milk Powders . J. Dairy Sci. , 77 : 2907 – 2915 .
- Roos , Y.H. and Karel , M. 1991 . Plasticizing Effect of Water on Thermal Behaviour and Crystallization of Amorphous Food Models . J. Food Sci. , 56 : 38 – 43 .
- Franks , F. , Asquith , M.H. , Hammond , C.C. , Skaer , H.B. and Echlin , P. 1977 . Polymeric Cryoprotectants in the Preservation of Biological Ultra Structure. I. Low Temperature States of Aqueous Solutions of Hydrophilic Polymers . Journal of Microscopy, , 110 : 223
- Herrera , J.R. , Pastoriza , L. and Smapedro , G. 2000 . Inhibition of Formaldehyde Production in Frozen-stored Minced Blue Whiting (Micromesistius Poutassou) Muscle by Cryostabilizers: An Approach from the Glassy State Theory . J. Agric. Food Chem. , 48 : 5256 – 5262 .
- Herrera , J.R. and Roos , Y.H. 2001 . A Kinetic Study on Formaldehyde Production in Cryostabilized Water-soluble Fish Muscle Extracts . Inn. Food Sci. Emerging Technol. , 1 : 227 – 235 .
- Levi , G. and Karel , M. 1995 . Volumetric Shrinkage (Collapse) in Freeze-dried Carbohydrates Above Their Glass Transition Temperature . Food Res. Int. , 28 ( 2 ) : 145 – 151 .
- Roos , Y.H. 1997 . Frozen State Transitions in Relation to Freeze-drying . J. Thermal Anal. , 48 : 535 – 544 .
- Singh , K.J. and Roos , Y.H. 2005 . Frozen State Transitions of Sucrose/Protein/Cornstarch Mixtures . Journal of Food Science , 70 : E198 – E204 .
- Gordon , M. and Taylor , J.S. 1952 . Ideal Copolymers and the Second-order Transitions of Synthetic Rubbers. I. Non-Crystalline Copolymers . J. Appl. Chem. , 2 : 493 – 500 .
- Haque , K. and Roos , Y.H. 2004 . Water Plasticization and Crystallization of Lactose in Spray-dried Lactose/protein Mixtures . J. Food Sci. , 69 ( 1 ) : 23 – 29 .
- Roos , Y.H. and Karel , M. 1990 . Differential Scanning Calorimetry Study of Phase Transitions Affecting the Quality of Dehydrated Materials . Biotechnol Prog. , 6 : 159 – 163 .
- Mazzobre , M.F. , Soto , G. , Aguilera , J.M. and Buera , M.P. 2001 . Crystallization Kinetics of Lactose in Systems Co-lyofilized with Trehalose. Analysis by Differential Scanning Calorimetry . Food Res. Int. , 34 : 903 – 911 .
- Hill , V.L. , Craig , D.Q.M. and Feely , L.C. 1998 . Characterisation of Spray-dried Lactase Using Modulated Differential Scanning Calorimetry . Int. J. Pharmaceutics , 161 : 95 – 107 .
- Matveev , Y.I. , Grinberg , V.Y. , Sochava , I.V. and Tolstoguzov , V.B. 1997 . Glass Transition Temperature of Protein. Calculation Based on the Additive Contribution Method and Experimental Data . Food Hydrocolloids , 11 ( 2 ) : 125 – 133 .
- Roos , Y.H. and Karel , M. 1992 . Crystallization of Amorphous Lactose . J. Food Sci. , 57 : 775 – 777 .
- Shamblin , S.L. , Huang , E.Y. and Zografi , G. 1996 . The Effects of Co-lyophilized Polymeric Additives on the Glass Transition Temperature and Crystallization of Amorphous Lactose . J. Thermal Anal. , 47 : 1567 – 1579 .
- Goff , H.D. , Caldwell , K.B. and Stanley , D.W. 1993 . The Influence of Polysaccharides on the Glass Transition in Frozen Sucrose Solutions and Ice Cream . J. Dairy Sci. , 76 : 1268 – 1277 .
- Goff , H.D. and Sahagian , M.E. 1996 . “ Freezing of Dairy products ” . In Freezing Effects on Food Quality , Edited by: Jeremiah , L.E. 299 – 335 . New York : Marcel Dekker Inc .
- Kasapis , S. , Insaf , M.A. , Marhoobi , A. and Khan , A.J. 2000 . Viscous Solutions, Networks and the Glass Transition in High Sugar Galactomannan and κ-carrageenan Mixtures . Int. J. Biol. Macromol. , 27 : 13 – 26 .
- Muhr , A.H. and Blanshard , J.M.V. 1986 . Effects of Polysaccharide Stabilizers on the Rate of Growth of Ice . J. Food Technology , 21 : 683 – 710 .
- Sutton , R.L. , Cooke , D. and Russel , A. 1997 . Recrystallization in Sugar/stabilizer Solutions as Affected by Molecular Structure . J. Food Sci. , 62 ( 6 ) : 1145 – 1149 .
- Goff , H.D. , Verespej , E. and Smith , A.K. 1999 . A Study of Fat and Air Structures in Ice Cream . Int. Dairy Journal. , 9 : 817 – 829 .
- Wang , S.T. , Barringer , S.A. and Hasen , P.M.T. 1998 . Effects of Carboxymethylcellulose and Guar Gum on Ice Crystal Propagation in a Sucrose-lactose Solution . Food Hydrocolloids , 12 : 211 – 215 .
- Regand , A. and Goff , H.D. 2003 . Structure and Ice Recrystallization in Frozen Stabilized Ice Cream Model Systems . Food Hydrocolloids , 17 : 95 – 102 .
- Carrington , A.K. , Goff , H.D. and Stanley , D.W. 1996 . Structure and Stability of the Glassy State in Rapidly and Slowly Cooled Carbohydrate Solutions . Food Res. Int. , 29 ( 2 ) : 207 – 213 .
- Cruz , I.B. , Oliveira , J.C. and MacInnes , W.M. 2001 . Dynamic Mechanical Thermal Analysis of Aqueous Sugar Solutions Containing Fructose, Glucose, Maltose and Lactose . Int. J. Food Sci. Technol. , 36 : 539 – 550 .