Abstract
Concentration, gel strength (Bloom), and pH effects on both melting and gelling temperatures of gelatin were studied using small amplitude oscillatory rheology. Temperature sweeps were applied to gelatin gel samples for heating and cooling at fixed frequencies. Results showed that melting temperatures were higher than gelling temperatures, and both increased with increasing concentration at pH from 3 to 6 for all gel strength. For constant gelatin concentration and pH, as gel strength increased, melting temperatures decreased, whereas gelling temperatures increased. A mathematical model was obtained which correlates melting and gelling temperatures, respectively, with pH and concentration at fixed Bloom degrees. All gelatin gels showed storage modulus higher (2 to 10 kPa) than loss modulus (50 to 500 Pa).
INTRODUCTION
Gelatin, a protein food ingredient obtained by the thermal denaturation of collagen, is an important industrial gelling biopolymer due to its ability to form thermally reversible gels with water.[Citation1] It is widely utilized in the food industry to increase the viscosity of aqueous systems[Citation2] such as in dessert formulations. The gel melting (Tm) temperature (<35°C) gives gelatin products unique organoleptic properties and flavor release.[Citation3] In confectionery it is used as the gelling binder in gummy products, wine gums, and so on; it has applications in the pharmaceutical industry in the elaborations of capsules and tablets; and in the photographical industry as film preparations, colored papers, and x-ray films, and so on.
Gelatin is an amphoteric protein, it has both positive and negative charges on the molecule, with isoelectric point between 5 and 9 depending on raw material and method of manufacture.[Citation1] Interstitial collagen molecules are composed of three α-chains intertwined in the collagen triple helix. This structure is due to the continuous repeat of the proline-glycine-hydroxyproline sequence. These molecules present both intra and inter-molecular covalent crosslinks. Since gelatin is derived from denatured collagen, its properties and gelling abilities depend on all these parameters.[Citation3,Citation4] The most important properties that characterize a gelatin for food applications are gel strength, viscosity, and both melting and gelling temperature. These properties are affected by various factors such as concentration, pH, gelling time, and salt content.[Citation5] One of the most important attributes of gelatin is its gel strength; the standard method to determine gelatin gel strength is called the Bloom test, which is the force in grams required to press a 12.5 mm diameter plunger 4 mm into 112 g of a standard 62/3% w/v gelatin gel at 10°C. This characteristic is called Bloom strength.
During the processing of aqueous gelling systems, sol-gel transitions usually occur, which cause very complex rheological behavior. Varying environmental parameters such as time, temperature, and concentration, often lead to dramatic changes in the viscoelastic behavior. For the rheological characterization of such sol-gel transitions, different methods and techniques have been applied.
Rheologically, the gel point follows the expression:[Citation6]
where G′ and G″ are the storage and loss modulus, respectively; δ is the phase angle; ω is the angular frequency;[Citation8] and Γ is the gamma function. Independence of δ from the frequency as obtained with EquationEq. (4) allows the gel point detection using this technique of dynamic testing with small amplitude oscillatory rheology. Arenaz and Lozano[Citation9] determined the gel point temperature of pectin samples with an oscillatory pressure testing device. Small amplitude oscillatory rheology offers a good opportunity for non destructive testing to observe structural changes.[Citation8] Where the curves corresponding to the loss (G′) and storage (G″) modulus crossover corresponds to the melting temperature for the heating process and to the gelling temperature, Tg, for the cooling process, respectively.[Citation10] Gudmundsson[Citation11] studied rheological properties of gelatin obtained from different types of fish using this technique to obtain Tm and Tg.
Knowledge of Tm and Tg of gelatin gels is important in food product development because of its influence in sensorial attributes such as consistency and melting in the mouth during the eating process.[Citation8] Also, the interactions with other food components are influenced by the food system conditions such as pH, temperature and the gelatin strength.[Citation8] Therefore, based upon the reasons mentioned above, the objectives of this work were i) to study of the effects of concentration, gel strength, and pH on the gel point of gelatin gels using small amplitude oscillatory testing; ii) to find a mathematical model, which allows correlation of melting and gelling temperatures, respectively, with pH and concentration with gel strength as a parameter.
MATERIALS AND METHODS
Materials
Gelatin powder samples (gelatin strengths of 180, 220, and 240 Bloom) from different batches were obtained from a producer (Floramatic™ S.A., Chile).
Procedure
Gelatin samples were prepared at 5, 7, and 10% (w/v) at pH values from 3 to 9. Gelatin powder was added to distilled water at room temperature and dissolved by increasing the controlled water bath temperature to 40°C while stirring. When the sample reached 40°C either a NaOH solution (0.5M) or HCl solution (0.5M) was added drop by drop until the desired pH, controlled by a pH-meter (Cole Parmer, USA), was reached. The sample was removed from the controlled water bath and left to cool at room temperature and afterwards stored at 10°C for approximately 12 h. The formed gel was cut into 40 mm diameter cylinders with 1.2 mm height.
Rheological Testing
A rheometer (Carri Med, CSL2 100, TA Instruments, England) using a 40 mm diameter parallel plate fixture was used to carry out the experiments. The lower plate is equipped with a Peltier temperature control system. A 800 μm gap between the two parallel plates was applied for small amplitude oscillatory measurements. The cylindrical gelatin sample was placed on the lower plate, and the plate was then raised to the desired gap. To ensure that the samples reached and maintained the working temperature, a routine temperature stabilization provided by the instrument software was applied. Preliminary tests, to determine the strain, which assures the region of linear viscoelasticity, were performed in strain sweep mode, with an oscillatory shear stress sweep range from 8 × 10−3 to 80 Pa at a frequency of 1 Hz and temperature of 10°C.
Temperature Variation
To determine gelatin melting temperature, temperature sweeps from 10 to 40°C with a heating rate of 1°C/min at frequencies of 1, 5, 10, 15, and 20 Hz were applied. To determine gelatin gelling temperature, temperature sweeps from 40 to 10°C with a cooling rate of 1°C/min at frequencies of 1, 5, 10, 15, and 20 Hz were applied. In both cases, the strain applied was that corresponding to linear viscoelasticity.
Gelatin Melting and Gelling Temperature Determination
When the curves corresponding to the loss (G′) and storage (G″) modulus crossover, this corresponded to Tm for the heating process and to Tg for the cooling process, respectively.[Citation10]
Statistical Analysis
A 5 × 3 × 3 statistical design was applied and the results were analyzed by response surface methodology with the aid of Statgraphics® 4.0 plus for Windows software (Statistical Graphics Corp. 1994–1997). The experimental factors were: temperature, gelatin concentration (w/v), and frequency; response variables were storage (G′) and loss (G″) modulus. Results were analyzed with Microsoft® Excel 2000 software (Microsoft Corporation, 1983–1999). Treatment averages were considered significantly different at P < 0.05.
Mathematical Modeling
To obtain an adequate mathematical model, which allows correlation of melting and gelling temperatures, respectively, with pH and concentration at fixed Bloom; parameters of tested equations were obtained by non-linear regressions with the program SYSTAT (Version 8, SPSS Inc., 1998), using as objective function [EquationEq. (5)] the minimization of the relative deviations.[Citation12]
RESULTS AND DISCUSSION
Linear Viscoelastic Region
The first task of this study was to determine the region of linear viscoelasticity and strain was found to be in the range 0.05 to 0.19% which is usual in materials like gels. This value is within those obtained by other researchers, for shark gels the strain was 0.5%,[Citation13] and within those found for wheat dough[Citation14–17]. It was experimentally verified that this strain value did not significantly vary (P < 0.05) over the temperature range applied in this study.
Frequency sweeps at 10°C of different gelatin gel strengths in the range from 1 to 25 Hz are given in for pH 6. Tm and Tg are not affected by the applied frequencies. The storage modulus G′ increased with increasing gel concentration for all gelatin gel strengths; this increase was more obvious for 10%(w/v) concentration. In this study, all gelatin gel samples showed viscoelastic behavior, with storage modulus G′ values higher than the loss modulus G″. G′ values varied from 2000 to 10000 Pa and G″ varied from 50 a 500 Pa showing predominance of a solid-like behavior.
Figure 1 Storage modulus G′ during frequency sweep of gelatin gel at 10°C, pH 3, and 0.06% strain. Gelatin gel strength: a) 180 Bloom; b) 220 Bloom; c) 240 Bloom. Gelatin concentrations (w/v): ▪: 5%; ▴:7%; ♦: 10%.
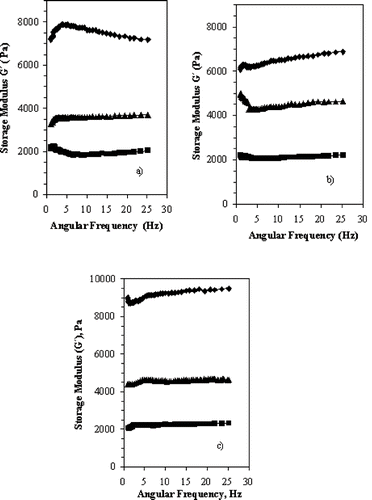
also shows the effect of gelatin gel strength on G′, where G′ changed from 7182 Pa for 180 Bloom to 6889 Pa for 220 Bloom and to 9492 Pa for 240 Bloom for 10%(w/v) concentration when considering a frequency of 25 Hz, whereas for 5%(w/v) concentration G′ changed from 2039 Pa for 180 Bloom to 2214 Pa for 220 Bloom and to 2323 Pa for 240 Bloom at the same frequency of 25 Hz.
Melting and Gelling Temperatures
Melting temperature (Tm ) determinations are shown in , which were obtained from where storage modulus (G′) and loss modulus (G″) crossover during the heating stage. Tg determinations were similar to Tm determinations using, in this case, the cooling stage. These tests were performed at different frequencies, in duplicate, using the method described by Michon et al.[Citation10] It was found that frequency variation did not influence neither Tm nor Tg ; therefore, these values are expressed as the average for the applied frequencies. At Tm and Tg gelatin gels showed a constant loss tangent value over the frequency range applied in this study. Melting and gelling temperature dependence on gel strength, gelatin concentration and pH are shown in and
Figure 2 Melting temperature Tm determination through storage modulus (G′) and loss modulus (G″) variations during temperature sweeps at a heating rate of 1°C/min for 180 Bloom gelatin gels at 1 Hz, pH 3, and 0.06% strain. Gelatin concentrations (w/v): a): 5%; b): 7%, and c): 10%.
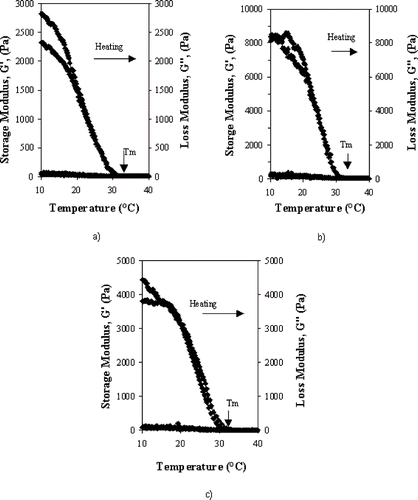
Table 1 Melting (Tm) temperature dependence on gel strength, gelatin concentration, and pHFootnote∗
Table 2 Gelling (Tg) temperature dependence on gel strength, gelatin concentration, and pHFootnote∗
Gel Strength Effect
For a given gelatin concentration and fixed pH as gelatin gel strength (Bloom) increased Tm decreased (). It could be expected that as gelatin gel strength increases, melting temperature also increases, due to the extra energy required to de-crosslink the chains; but, at this point it is necessary to point out that the gelatin gel strength was characterized as detailed in the introduction section for standard conditions (force in grams required to press a 12.5 mm diameter plunger 4 mm into 112 g of a standard 62/3% w/v gelatin gel at 10°C), and the actual rheological measurements were carried out at different conditions. According to the literature,[Citation3] there is no simple relationship between gel strength and concentration.
also shows that for a given gelatin gel strength and fixed pH, as gelatin concentration increased Tm also increased, due to the extra energy required to de-crosslink the chains. From it can be seen that gel strength showed a more obvious effect on Tm from 180 Bloom to 220 Bloom with 1.4°C decrease on average Tm value, whereas from 220 Bloom to 240 Bloom there was a small decrease (0.3°C) on average Tm value. When analyzing the effect of gel strength on the gelling temperature Tg, the behavior is opposite to the Tm case. For a given gelatin concentration and fixed pH as gelatin gel strength increased Tg increased ().
Gelatin Concentration Effect
For a given gelatin gel strength and fixed pH as gelatin concentration increased both Tm and Tg increased ( and ). Gelatin concentration showed a stronger influence on Tg than on Tm as seen in the case of 180 Bloom where Tm increased 1.22°C when concentration increased from 5% to 10% at pH 6 () whereas Tg increased 4.33°C for the same concentration change and pH (). In the range of gelatin concentration of this study, the average change for Tm was 2.1°C and for Tg was 4.2°C.
The concentration effect on Tm and Tg may be explained due to the fact that when gelatin concentration increases there are higher protein-protein and protein–dissolvent interactions with higher number of bonds created requiring higher temperatures to break them, therefore Tm increases; on the other hand, higher concentrations allows bond formation at higher temperatures, meaning Tg increases. From and it is observed that Tm is higher than Tg for all the variables considered in this study. The average difference between Tm and Tg is 10.53°C ± 0.73. This difference in temperature is due to the fact that during the gelatin gel preparation there was a resting period of 12 hs at refrigerated temperature, the period in which hydrogen bonds — promoting triple helix formation (physical aggregation) causing gel formation— are favored, which means that is more difficult to break them (‘sol’ transition) than to form them (‘gel’ transition).
pH Effect
The effect of pH on Tm at different gelatin concentrations is shown on The effect of pH is more obvious in the pH range from 3 to 6 where Tm increased as pH increased, whereas in the pH range from 6 to 9 Tm increased slightly. Tm decreased slightly with pH when gel strength increased at a given gelatin concentration. The exception in this study occurred when working at 10% (w/v) gelatin concentrations with pH values higher than 5. The effect of pH on Tg at different gelatin concentrations is shown on . The effect of pH is more obvious in the range from 3 to 6 where Tg increased as pH increased. The more stable Tm and Tg values for the pH range from 5 to 9 is related to the isoelectric point of gelatin, which corresponds to the same range from 5 to 9.[Citation1]
Mathematical Modeling
A mathematical model [EquationEq. (6)] was obtained for Tm and Tg which allows to correlate melting and gelling temperatures, respectively, with pH and concentration at fixed Bloom degrees, and it can be written as:
where T is the melting or gelling temperature; Conc is the gelatin concentration (w/v); pH is the corresponding pH of gelatin gel at a given gel strength; a, b, c, and d are the parameters of EquationEq. (6) given in and for Tm and Tg, respectively. This equation gave the higher mean corrected R-square for the adjusted equation, the lower asymptotic standard error (A.S.E.) for each parameter, and the closest Wald confidence interval at 95% for each parameter for a given gel strength, as shown in and
Table 3 Parameters of EquationEq. (6) for melting temperature Tm at different gelatin gel strengthFootnote∗
Table 4 Parameters of EquationEq. 6 for gelling temperature Tg at different gelatin gel strengthFootnote∗
CONCLUSIONS
It can be concluded that melting temperatures were higher than gelling temperatures, with an average difference of 10.53°C ± 0.73. Melting and gelling temperature values increased with increasing concentration for all gelatin strength and pH values in the range from 3 to 6. Both Tm and Tg increased with increasing gelatin concentrations. It was found that Tm and Tg are not affected by the applied frequencies. Both Tm and Tg were notoriously affected by pH in the range from 3 to 6. All gelatin gels showed storage modulus values higher than loss modulus for temperatures lower than Tm. For a given gelatin concentration and fixed pH as gelatin gel strength increased Tm decreased, whereas Tg increased. A mathematical model was obtained, which allows to correlate melting and gelling temperatures, respectively, with pH and concentration at fixed gel strength.
ACKNOWLEDGMENTS
This research was funded by project DICYT-USACH 080371- OL.
Notes
1. Fennema, O. Química de los Alimentos, 2° Edición; Ed. Acribia, S.A., Ed.; Zaragoza, España, 2000; 155, 220, 466.
REFERENCES
- 1. Fennema, O. Química de los Alimentos, 2° Edición; Ed. Acribia, S.A., Ed.; Zaragoza, España, 2000; 155, 220, 466.
- Ahmed , J. , Ramaswamy , H.S. and Ngadi , M.O. 2005 . Rheological Characteristics of Arabic Gum in Combination with Guar and Xanthan Gum Using Response Surface Methodology: Effect of Temperature and Concentration . International Journal of Food Properties , 8 : 179 – 192 .
- Ledward , D.A. 2000 . “ Gelatin ” . In Handbook of Hydrocolloids , Edited by: Phillips , G.O. and Williams , P.A. 67 – 86 . Boston : Woodhead Publishing Limited .
- Johnston-Banks , F.A. 1990 . “ Gelatin ” . In Food Gels , Edited by: Harris , P. 233 – 289 . New York : Elsevier Applied Science Publishers .
- Rao , M.A. 1999 . “ Rheology of Fluid and Semisolid Foods ” . In Principles and Applications , 1 – 21 . Maryland, , USA : Aspen Publishers, Inc . 25–54, 319–330.
- Winter , H.H. , Morganelli , P. and Chambon , F. 1988 . Stoichiometry Effects on Rheology of Model Polyurethanes at the Gel Point . Macromolecules , 21 : 532 – 535 .
- Horst , R.H. and Winter , H.H. 2000 . Stable Critical Gels of a Crystallizing Copolymer of Ethene and 1-Butene . Macromolecules , 33 : 130 – 136 .
- Steffe , J. 1996 . Rheological Methods in Food Process Engineering , 1 – 30 . Michigan : Freeman Press . 25–54.
- Arenaz , M.F. and Lozano , J.E. 1998 . Measurement of Gel Point Temperature and Modulus of Pectin Gels . J. Food Sci , 63 : 979 – 982 .
- Michon , C. , Cuvalier , G. and Launay , B. 1993 . Concentration Dependence of Critical Viscoelastic Properties of Gelatin at the Gel Point . Rheol. Acta , 32 : 94 – 103 .
- Gudmundsson , M. 2002 . Rheological Properties of Fish Gelatin . J. Food Sci , 67 : 2172 – 2175 .
- Pedreschi , F. , Hernández , P. , Figueroa , C. and Moyano , P. 2005 . Modelling Water Loss during Frying of Potato Slices . International Journal of Food Properties , 8 : 289 – 299 .
- Venugopal , V. , Kakatkar , A. , Bongirwar , D.R. , Karthikeyan , M. , Mathew , S. and Shamasundar , B.A. 2002 . Gelation of Shark Meat under Acidic Conditions: Physicochemical and Rheological Characterization of the Gel . J. Food Sci , 67 : 2681 – 2686 .
- Campos , D.T. , Steffe , J.F. and Ng , P.K.W. 1997 . Rheological Behavior of Undeveloped and Developed Wheat Dough . Cereal Chemistry , 74 : 489 – 494 .
- Dus , S.J. and Kokini , J.L. 1990 . Prediction of Nonlinear Viscoelastic Properties of a Hard Wheat Flour Dough using the Bird-Carreau Constitutive Model . J. Rheol , 34 : 1069 – 1084 .
- Hibberd , E.E. and Wallace , W.J. 1996 . Dynamic Viscoelastic Behavior of Wheat Flour Doughs . I. Linear aspects. Rheology Acta , 5 : 193 – 198 .
- Weipert , D. 1990 . The Benefits of Basic Rheometry in Studying Dough Rheology . Cereal Chemistry , 67 : 311 – 317 .