Abstract
Thermal degradation kinetics of chlorophyll ‘a’, ‘b’ and total chlorophyll in mint leaves puree were investigated as function of pH (4.5–8.5) and processing temperature (80–145°C), respectively. Mint puree was processed at 80°to 100°C at pH 4.5, while that at pH 5.5 to 8.5 was processed at 105°to 145°C. Chlorophyll degradation followed the first order reaction kinetics. Good agreement was found between estimated and experimental chlorophyll retention in all cases (R2 > 0.86; MRQE < 0.27). Activation energies ranged from 6.45 to 47.67 kJ/mol. Reaction rate and activation energy data indicated that chlorophylls were more stable at alkaline pH. Transition state theory was applied to estimate the enthalpy, entropy and Gibbs free energy of activation. Enthalpy (ΔH#) ranged from 3.14 to 44.66 kJ/mol, while entropy (ΔS#) ranged from −0.157 to −0.266 kJ/(mol K). The overall free energy change was 105.76 kJ/mol. Results indicated that, the compensation effect did not exist for chlorophyll degradation in mint puree during thermal processing.
INTRODUCTION
Mint (Mentha arvensis) belongs to herb species and is valued for its aromatic component. Various species of mint have been used since times immemorial for infusions in beverages and as culinary flavoring. The mint plant yields both the fresh green herb and essential oil which have rich medicinal properties and are used in pharmaceutical, perfumery, cosmetic products, religious rituals, and soon. They are valued as piquant flavoring agents and also as appetite stimulants, muscle relaxant and analgesic. Recently, Choudhary[Citation1] described strong antioxidant properties of Indian mint leaves. Drying of mint leaves for culinary use in is unsuitable, as it leads to significant losses of aromatic components and its attractive green color.
Retention of green color in processed green vegetables is a challenge to food processors.[Citation2] The color change from bright green to olive brown during processing is attributed by conversion of chlorophylls to pheophytins due to the loss of central magnesium ion. Several investigators have studied the rate and extent of chlorophyll conversion[Citation3,Citation4,Citation5,Citation6] Most of those studies revealed that both pigment and visual color degradation during thermal processing follows the first order reaction kinetics.[Citation6] Application of various techniques have been attempted to preserve green color of vegetables. Researchers tried to retard the formation of pheophytin by minimizing heat exposure by applying HTST technique,[Citation7] or by combining HTST with pH adjustments.[Citation8,Citation9] Gupte et al.[Citation8] reported that although the use of magnesium carbonate in combination with HTST (149°C) processing initially improved the chlorophyll retention in pureed spinach, the effect was unstable during storage. Magnesium compounds also resulted in the formation of hard white crystals of magnesium-ammonium-phosphate.[Citation10] Extent of chlorophyll degradation depends on the thermal severity of the process. The possibility of reducing the severity of the process from a chemical point of view, while, maintaining a microbiologically equivalent heat dose has been investigated.[Citation7,Citation11] It is well known that acidic foods require lesser degree of thermal processing for their preservation as heat resistant food spoilage microorganisms are unable to thrive at acidic pH. In thermal processing, it is possible to achieve different time-temperature combinations with same lethal effects but resulting in different quality losses. It is, therefore, quite interesting to study the effect of wide pH ranges that may be combined with less severe thermal process, leading to maximum retention of chlorophyll in thermally processed food.
Because of the safety and quality factors (microorganisms, color, flavor, and nutrients), care must be taken to avoid either under-processing or over-processing of foods. In order to balance the benefits of thermal processing without compromising the safety, a good predictive model is required. Evaluation of a thermal process and determination of the optimum processing conditions involve the development of mathematical models that accurately predict the progress of a chemical or biochemical reaction occurring in homogenous liquid or semi-solid phase.[Citation12] Experimental studies and application of simplified models to predict and interpret kinetic parameters (reaction order, rate constant, and activation energy) have been utilized by process industries to improve the process efficiency.[Citation13] Systematic study of chlorophyll degradation kinetics during thermal processing of vegetables at different levels of pH is lacking.
Thermodynamic Functions
There are numbers of equilibrium and rate processes in which plotting enthalpic changes against entropic changes produced by a systematic variation of solvent composition, reactant molecule change, or pH gives a straight line.[Citation14] The relationship between enthalpy and entropy is termed as enthalpy-entropy compensation (ΔH/ΔS)) and the slope of this line is called the compensation temperature (Tβ). The existence of compensation suggests that each thermal degradation is carried out by the same mechanism. It allows the forecasting of the activation energy, if the frequency factor can be deduced by some means.[Citation15] The enthalpy– entropy compensation has been the object of controversy because some authors[Citation15,Citation16] believe that the straight line behavior of the ΔH and ΔS data is fortuitous because of error spread effects. Krug et al.[Citation16,Citation17] published a statistical approach of testing for true compensation. They demonstrated that if the errors were high, the slope of the compensation plot would be within 95% confidence interval of harmonic mean of temperatures represented by . Wold and Exner[Citation18] derived a statistical relationship in which, if compensation occurs, all ln(k) vs 1/T curves should intersect at 1/Tβ. Most researchers assume compensation phenomena same as the isokinetic effect. However, Liu & Guo[Citation19] pointed that the compensation effect and isokinetic effect can be different and the correlation between ΔH and ΔS is not a good evidence for existence of isokinetic effect. The existence of the true compensation effect for chlorophyll degradation during thermal processing has been suggested by Labuza[Citation20] and Canjura et al.[Citation21] for broccoli and spinach puree, respectively. Labuza[Citation20] advocated that (ΔH/ΔS) analysis could lead to useful predictions of rates and activation energies from several conditions and could explain the change in activation energy without the need to postulate a change in the mechanism. Two criteria have been suggested for applying ΔH/ΔS to food systems: (i) data at some constant pH or water activity as a function of temperature; and (ii) data at some constant temperature as a function of water activity or pH. Canjura et al.[Citation21] indicated the importance of the study of chlorophyll degradation at different pH values to demonstrate the existence of true compensation effect on degradation of these compounds.
The objectives of this research were: (i) to determine the degradation kinetics of chlorophyll ‘a’, ‘b’ and total chlorophyll in mint leaves puree at temperatures varying from 80° to 145°C considering pasteurization to sterilization time-temperature combinations; (ii) to study the stability of chlorophylls at broad pH ranges (4.5 to 8.5) on during thermal processing. Kinetic parameters were determined for prediction of chlorophylls in thermally processed mint puree as a function of processing time, temperature and pH; and (iii) the existence of true compensation effect was tested for chlorophyll degradation during thermal processing.
MATERIALS AND METHODS
Fresh mint was procured locally. The leaves were de-stemmed, washed with running water and drained. The leaves were then blanched at 85°C for 3.5 min, cooled immediately to room temperature and comminuted in a wet grinder having two motor driven round stones hinged in the centre, rolling over a flattened granite stone base (Vijai Company, Chennai, India). The comminuted puree was passed through a sieve of 1.19 mm aperture size and stored in freezer (−7°C) till further use. Before the experiment, the puree was thawed overnight in refrigerator at 5 ± 1°C. The inherent pH of the puree was 5.6 ± 0.15. Adjustment of pH was done by adding either 10% citric acid or 1N sodium hydroxide solution.
Mint leaves puree was filled to the brim in each culture tube (o.d. = 1.25 cm, height = 10.5 cm) and covered with rubber and teflon lined screw cap (JSGW, Ambala, India). A constant temperature oil bath (±0.1°C, Narang Scientific Works, New Delhi, India) was used for thermal treatment of mint puree at 80–145°C. The come-up time (time taken by the sample to reach the desired temperature at its geometric centre) for each temperature level was determined by inserting a thermocouple in the centre of the tube (). Thermal treatment times were adjusted for the come-up times at each temperature. Experiments were designed to allow adequate heating time so that concentrations of chlorophyll a and b would have changed by at least one log cycle, which is necessary to distinguish a first order reaction from a zero or second order reaction.[Citation22] Experimental conditions were established to provide a wide temperature range, i.e., 80–100°C for puree at pH 4.5 and 105–145° C for puree at pH 5.5 to 8.5. It is well known that highly acidic foods require thermal processing below 100°C, while foods above pH 4.6 require thermal treatment at greater than 121°C for their commercial sterilizaion. Temperature and time intervals at pH 5.5–8.5 were as follows: 105–115°C (0–240 min); 125° (0–120 min), 135°, and 145°C (0–60 min). The puree at pH 4.5 was heated at temperatures of 80 to 100°C for up to 240 min. Thermal processing of puree was done in triplicates. Heat-treated puree was cooled immediately in chilled water bath and stored in refrigerator until used for pigment extraction.
Table 1 Come-up-time for desired temperature attainment in culture tubes
The pigments were extracted using 80% acetone following the procedure of Jones et al.[Citation23] () Amber colored volumetric flasks were used during extraction and dilution steps. The absorbance of chlorophyll extracts was measured using UV-Vis spectrophotometer (UV 2401 PC, Shimadzu Co., Singapore) at 646.5 and 664 nm.
Kinetic Data Analysis
It has been established by several researchers[Citation5,Citation21,Citation24] that degradation of chlorophyll follows the first-order kinetic model.
Integration of EquationEq. (1) yields,
where, C is the measured chlorophyll ‘a,’ ‘b,’ or total chlorophyll (μmol/L); Co is the initial chlorophyll content of the puree at zero min; t is the heating time (min); and k is the rate constant for chlorophyll degradation (min−1). The Arrhenius equation can be used to include the effect of temperature on the reaction rate constant.
where, ko is the pre-exponential factor (min−1); E is activation energy for chlorophylls degradation (kJ/mol); R is universal gas constant (8.314 J/mol.K); and T is absolute temperature (K). The accuracy of the fit of the model was tested by mean relative quadratic error (M.R.Q.E.):
Thermodynamic Parameters
The statistical thermodynamic version of activated complex theory has motivated an empirical approach in which the activation process is expressed in terms of thermodynamic functions. According to Eyring equation of the activated complex theory,[Citation25]
where, κ is transmission factor (dimensionless), ks is Boltzmann constant (1.38 × 10−23 J/K) and h is Plank's constant (6.63 × 10−34 Js), and K# is equilibrium constant of the activation complex (dimensionless). The # on K indicates that the energetic mode along the reaction coordinate has been excluded from the formulation of the equilibrium constant.
Because, G = H – TS, the Gibb's energy of activation can be divided into entropy of activation (ΔS#) and enthalpy of activation (ΔH#) by writing
The transmission coefficient is assumed to be unity and is ignored in the analysis. On combining Equationequations (8) and Equation(9),
Linearization of the Equationequation (10) yields,
Therefore, from regression of ln(k/T) vs (1/T), ΔH#, ΔS# and ΔGo# could be computed.
RESULTS AND DISCUSSION
Kinetics of Thermal Degradation of Chlorophylls
Linear regression of EquationEq. (2) was carried out using least squares technique and the coefficients were determined. The obtained coefficients k, R2, and standard error (S.E.) for chlorophyll degradation are reported in . Normalized concentration curves for thermal degradation of chlorophyll in mint puree at pH 4.5, heated at 80 to 100°C are shown in . A representative curve for the pH 5.5 to 8.5, which was heated at 105° to 145°C is shown in . Solid lines in these figures represent the values computed using the model [EquationEq. (2)]. The R2 values ranged between 0.858 and 0.999, while standard error values were less than 0.737 in all cases. The accuracy of fit of the first order model was calculated by MRQE using EquationEq. (4). The MRQE values were less than 0.27. The excellent agreement between experimental and calculated values demonstrated the applicability of the first order rate kinetic equation to describe the degradation of chlorophylls of mint leaves puree during thermal processing. In general, the degradation rate (k) for chlorophyll ‘a’ at pH 4.5, 5.5, and 7.5 was higher than chlorophyll ‘b’. This is in agreement with the results of several researchers[Citation21,Citation26,Citation27] who reported that the relative degradation of chlorophyll ‘a’ was 2–10 times faster than chlorophyll ‘b.’ It is pertinent to mention that all these investigators worked on purees with an unmodified pH, in the range of 5.5–6.8. To be specific, Canjura et al.[Citation21] worked on pea puree at pH 6.0–6.2; La Jollo et al.[Citation26] on spinach at pH 5.4–6.2, and Schwartz & Lorenzo[Citation27] on spinach at pH 5.2–6.2.[Citation7] However, rate of degradation for chlorophyll ‘b’ was found to be higher than chlorophyll ‘a’ in mint leaves puree at pH 6.5 and 8.5 ().
Table 2 Computed values of coefficients of Equationequation (2) for chlorophylls (a, b, and total) degradation
Effect of Temperature on Degradation Rate Constant
Linear regression of EquationEq. (3) was performed to compute frequency factor and activation energy. reports activation energies, coefficients of determination (R2) and standard errors at pH 4.5–8.5. The R2 values ranged between 0.877 and 0.991, while MRQE value were less than 0.11 and the standard error values were less than 0.174 in all cases. Arrhenius plots were obtained for mint puree at the five pH levels (). The estimated activation energy of chlorophylls at pH 6.5 (22–37 kJ/mol) lies in the reported range.[Citation22] The activation energy for chlorophyll ‘a’ in heat processed mint puree was found to be less than chlorophyll b at pH 5.5 to 8.5. These observations are in agreement with the results reported by Canjura et al.[Citation21] This is, however, in variation with other reports, which, indicated that activation energy of chlorophyll ‘b’ was less than that of ‘a’ form.[Citation3,Citation28] These variations may be attributed to differences in materials, temperature ranges and analytical methods used to quantify the pigments.[Citation21] Results of this study indicated that the activation energy for chlorophyll ‘a’ was more than that for chlorophyll ‘b’ at pH 4.5.
Table 3 Activation energy and frequency factor values for thermally processed mint puree at pH 4.5–8.5 and 80°–145°C
Figure 3 The Arrhenius relationship between chlorophyll degradation rate constant and processing temperature of mint puree at a) pH 4.5; b) pH 5.6; c) pH 6.5; d) pH 7.5; and e) pH 8.5.
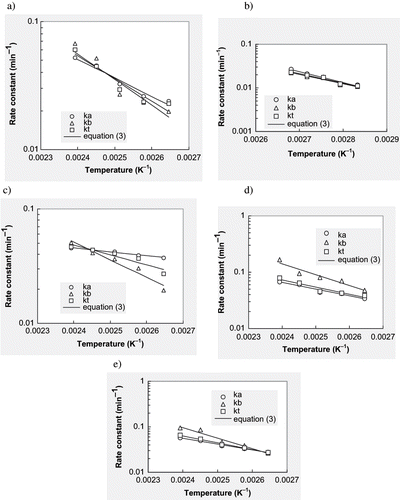
Higher activation energy implies that a smaller temperature change is required to degrade a specific compound more rapidly. It may therefore be inferred that chlorophylls ‘a’ and ‘b’ were most heat stable at pH 7.5 (). This was found to be in contradiction with the generally perceived notion that higher the alkalinity more is the chlorophyll stability. This contradiction may be attributed to the fact that in earlier works, the alkaline pH was attained with the addition of organic or inorganic salts of alkaline earth metals (mainly MgCO3) to aid in replenishment of Mg2+ ions removed during thermal degradation.[Citation2] However, in the present study, we intended to study the effect of only alkalinity without the compensatory effect of any additional magnesium salt to replenish the degraded chlorophylls, and the pH was adjusted using 1N NaOH solution. The possibility of solvation effect or reaction medium playing a major role in lowering the enthalpy cannot be ruled out. Acid-base catalysis during the chlorophyll degradation reaction at other pH levels may also be a reason for this behavior.
Thermodynamic Parameters
The enthalpy and entropy values of activation computed using Equationequation (11) are reported in . Enthalpy (ΔH#) ranged from 3.14 to 44.67 kJ/mol while entropy (ΔS#) ranged from −0.157 to −0.266 kJ/(mol.K). The enthalpy and entropy values for thermal degradation of chlorophyll in spinach puree ranged between 3.39 and 33.96 kJ/mol and −0.0124 and −0.064 kJ/(mol.K),[Citation9,Citation21] respectively. An attempt was initially made to plot ΔS#-ΔH# plots for chlorophyll ‘a,’ chlorophyll ‘b,’ and total chlorophyll individually. The computed compensation temperatures were 381.13, 372.73 and 388.23 K for chlorophyll ‘a,’ ‘b,’ and total chlorophyll, respectively. The corresponding free energy values were 104.95, 103.79, and 106.75 kJ/mol. It was observed that the slope (Tβ) and the intercept (ΔG) of equation were similar for the three cases. This observation is supported by Leffler & Grunwald[Citation29] who stated that free energy (ΔG°) varies little for a set of similar reactions of a homologous series or for the same reaction in a solvent of changing pH or dielectric strength. Therefore, the data corresponding to Ca, Cb and Ct was combined together to determine the average values of compensation temperature and free energy of activation. shows the enthalpy/entropy plot for chlorophyll degradation during thermal processing of mint leaves puree (R2 = 0.9955). The average values of Tβ and ΔG°# were 383.99K and 105.76kJ/mol, respectively ().
Table 4 Entropy, enthalpy, and free energy values of activation for chlorophylls in thermally processed mint puree
Compensation Effect
Various tests are proposed by researchers[Citation14,Citation16,Citation17,Citation18] ascertain the existence of true compensation effect of enthalpy and entropy. According to Krug et al.,[Citation16] harmonic mean temperature should not lie in the 95% confidence interval of Tβ. The harmonic mean temperatures for our experimental conditions were 362.86 K (pH 4.5) and 397.48 K (pH 5.5 to 8.5), which lie in the 95% confidence interval of Tβ (354–407 K). Therefore, the condition for existence of true compensation effect was not satisfied. Krug et al.[Citation16] invalidated the existence of compensation effect in a large number of chemical reactions reported in the literature using the 95% confidence interval test. A test recommended by Wold and Exner[Citation18] and Liu and Guo[Citation19] was also conducted to locate the intersection point of Arrhenius plots. The Arrhenius lines did not intersect at a common point and were found to be different from Tβ in general. This shows the absence of isokinetic effect for chlorophyll degradation during thermal processing of mint leaves puree of pH 4.5–8.5 at processing temperature 80–145°C. Labuza[Citation20] and Canjura et al.[Citation21] however, mentioned the existence of true compensation for chlorophyll degradation at varying water activity and during thermal processing in spinach puree respectively. It may therefore be inferred that the enthalpy-entropy compensation is essentially a statistical artifact. This is supported by the statistical analysis of Krug et al.[Citation16] and Leffler[Citation14] because the data used in the correlation are estimators of the corresponding parameters.
CONCLUSION
The thermal degradation of chlorophylls ‘a,’ ‘b,’ and total followed the first order reaction kinetics (R2 > 0.85). The rate constants were related to the inverse of temperature (T−1) by Arrhenius equation (R2 > 0.87). The activation energy values indicated greater heat stability of chlorophyll ‘b.’ Chlorophyll in mint leaves puree was found to be most heat stable at pH 7.5 (E = 16.659 kJ/mol). The enthalpy and entropy of activation followed a linear relationship (R2 = 0.9955) but did not indicate true thermodynamic compensation effect (Tβ ≈ Thm).
NOMENCLATURE
t | = |
Heating time (min) | ||||||||||||||
k | = |
Rate constant for chlorophyll degradation (min−1) | ||||||||||||||
ko | = |
Frequency factor (min−1) | ||||||||||||||
E | = |
Activation energy (kJ/mol) | ||||||||||||||
R | = |
Universal gas constant (8.314 J/mol.K) | ||||||||||||||
T | = |
Absolute temperature (K) | ||||||||||||||
κ | = |
Transmission factor | ||||||||||||||
ks | = |
Boltzmann constant (1.380658 × 10−23 JK−1) | ||||||||||||||
K# | = |
Equilibrium constant of the activation complex (dimensionless) | ||||||||||||||
h | = |
Plank's constant (6.626 × 10−34 Js) | ||||||||||||||
ΔGo # | = |
Gibbs free energy of activation in the standard state | ||||||||||||||
ΔS # | = |
Entropy of activation | ||||||||||||||
ΔH # | = |
Enthalpy of activation
|
REFERENCES
- Choudhury , R.P. , Kumar , A. and Garg , A.N. 2006 . Analysis of Indian Mint (Mentha spicata) for Essential, Trace and Toxic Elements and its Antioxidant Behaviour . Journal of Pharmaceutical & Biomedical Analysis , 41 : 825 – 832 .
- Ahmed , J. , Shivhare , U.S. and Kaur , M. 2002 . Thermal Colour Degradation Kinetics of Mango Puree . International Journal of Food Properties , 5 ( 2 ) : 359 – 366 .
- Gupte , S.M. and Francis , F.J. 1964 . Effect of pH Adjustment and High-temperature Short-time Processing on Color and Pigment Retention in Spinach Puree . Food Technology , 10 : 141 – 144 .
- Shin , S. and Bhowmik , S.R. 1995 . Thermal Kinetics of Color Changes in Pea Puree . Journal of Food Engineering , 24 : 77 – 86 .
- Steet , J.A. and Tong , C.H. 1996 . Degradation Kinetics of Green Color and Chlorophylls in Peas by Colorimetry and HPLC . Journal of Food Science , 61 : 924 – 927 . 931
- Weemaes , C. , Ooms , V. , Indrawati , Ludikhuyze , L. , Broek , I.V. , Loey , A.V. and Hendrickx , M. 1999 . Pressure-temperature Degradation of Green Color in Broccoli Juice . Journal of Food Science , 64 ( 3 ) : 504 – 508 .
- Tan , C.T. and Francis , F.J. 1962 . Effect of Processing Temperatures on Pigments and Color of Spinach . Journal of Food Science , 27 : 232 – 241 .
- Gupte , S.M. , El Bisi , H.M. and Francis , F.J. 1963 . Kinetics of Thermal Degradation of Chlorophyll in Spinach Puree . Journal of Food Science , 29 : 379
- Clydesdale , F.M. , Fleischman , D.L. and Francis , F.J. 1970 . Maintenance of Color in Processed Green Vegetables . Journal of Food Product Development , 4 ( 5 ) : 127
- Eheart , M.S. and Odubi , D. 1973 . Use of Ammonium Compounds for Chlorophyll Retention in Frozen Green Vegetables . Journal of Food Science , 38 : 202 – 205 .
- Kala , A. and Prakash , J. 2006 . Nutrient Composition and Sensory Profile of Differently Cooked Green Leafy Vegetables . International Journal of Food Properties , 7 ( 3 ) : 659 – 669 .
- Banga , I.R. , Alonso , A.A. , Galardo , J.M. and Perez-Martin , R.I. 1993 . Mathematical Modeling and Simulation of Thermal Processing of Anisotropic and Non-homogenous Conduction-heated Foods: Application to Canned Tuna . Journal of Food Engineering , 18 : 69 – 387 .
- Ahmed , J. and Shivhare , U.S. 2005 . “ Thermal processing of Vegetables ” . In Thermal Processing: New Technologies and Quality Issues , Edited by: Da Wen , Sun . New York : CRC Press .
- Leffler , J.E. 1966 . The Interpretation of Enthalpy and Entropy Data . Journal of Organic Chemistry , 31 : 533
- Suh , H.J. , Noh , D.O. , Kang , C.S. , Kim , J.M. and Lee , S.W. 2003 . Thermal Kinetics of Color Degradation of Mulberry Juice Extract . Nahrung/Food , 2 : 132 – 135 .
- Krug , R.R. , Hunter , W.G. and Grieger , R.A. 1976 . Enthalpy-Entropy Compensation. 1. Some Fundamental Statistical Problems Associated with the Analysis of van't Hoff and Arrhenius Data . Journal of Physical Chemistry , 80 ( 21 ) : 2335
- Krug , R.R. , Hunter , W.G. and Grieger , R.A. 1976 . Enthalpy-Entropy Compensation. 2. Separation of the Chemical from the Statistical Effect . Journal of Physical Chemistry , 80 ( 21 ) : 2342
- Wold , S. and Exner , O. 1973 . Statistics of the Enthalpy-Entropy Relationship. IV. Temperature Dependent Activation Parameters . Chemica Scripta , 3 : 5
- Liu , L. and Guo , Q.X. 2001 . Isokinetic Relationship, Isoequilibrium Relationship and Enthalpy-Entropy Compensation . Chemical Reviews , 101 : 673 – 695 .
- Labuza , T.P. 1980 . Enthalpy/Entropy Compensation in Food Reactions . Food Technology , 34 : 67 – 77 .
- Canjura , F.L. , Schwartz , S.J. and Nunes , R.V. 1991 . Degradation Kinetics of Chlorophylls and Chlorophyllides . Journal of Food Science , 56 : 1639 – 1643 .
- Lund , D.B. 1977 . Design of Thermal Process for Maximizing Nutrient Retention . Food Technology , 31 : 71 – 78 .
- Jones , I.D. , White , R.C. , Gibbs , E. , Butler , L.S. and Nelson , L.A. 1977 . Experimental Formation of Zinc and Copper Complexes of Chlorophyll Derivatives in Vegetable Tissue by Thermal Processing . Journal of Agricultural and Food Chemistry , 25 ( 1 ) : 149 – 153 .
- Ahmed , J. , Shivhare , U.S. and Ramaswamy , H.S.A . 2002 . Fractional Conversion Kinetic Model for Thermal Degradation of Color in Red Chili Puree and Paste . LWT Food Science and Technology , 35 : 497 – 503 .
- Atkins , P. and dePaula , J. 2002 . “ Molecular Reaction Dynamism. ” . In Atkin's Physical Chemistry , 7th , 956 – 961 . New York, NY : Oxford University Press .
- La Jollo , F. , Tannenbaum , S.R. and Labuza , T.P. 1971 . Reactions of Limited Water Concentrations. Chlorophyll Degradation . Journal of Food Science , 36 : 850
- Schwartz , S.J. and Lorenzo , T.V. Chlorophyll Stability during Continuous Aseptic Processing and Storage . Journal of Food Science , 1991 ( 56 ) 1059
- Schwartz , S.J. and von Elbe , J.H. 1983 . Kinetics of Chlorophyll Degradation to Pyropheophytin in Vegetables . Journal of Food Science , 48 : 1303 – 1306 .
- Leffler , J. and Grunwald , E. 1963 . Rates and Equilibria of Organic Reactions , New York : John Wiley & Sons .