Abstract
The effect of sodium chloride and/or sucrose content on the denaturation (Td) and the glass transition (Tg) temperatures of osmotically dehydrated tilapia fillets using binary or ternary solutions at 20°C, was evaluated. DSC analyses revealed that myofibrillar proteins denaturation temperatures and enthalpies decreased with increase in muscle salt content. Sugar produced a stabilizing effect on denaturation of the fillets proteins. When ternary solutions were used, sucrose did not protect the proteins against the ionic stress produced by the salt. Tg was affected by the residual moisture content of the samples. Influence of NaCl was important at low water activity values. Sucrose content did not influence on Tg.
INTRODUCTION
Osmotic dehydration is a process used for the concentration of water-rich foodstuffs and consists of immersing food pieces in concentrated solutions of salt, sugar, acids, and so on. When food is immersed in such a solution, a water flux occurs from the food to the solution and generally a flux of solute also occurs from the solution to the food. Thus, this process can also cause an increase in the solute content of the food. As a consequence, osmotic dehydration has also been considered as an impregnation process.[Citation1,Citation2]
Osmotic dehydration can be used as a fish salting process, and is still widely applied in many countries due to its relative simplicity and low cost. In order to salting fish by this wet process, a hypertonic sodium chloride solution is normally used.[Citation3–5] Sodium chloride has a strong effect in the reduction of the water activity, despite its limited capacity in reducing the moisture content. This behaviour is due to salt impregnation, which can also cause considerable changes in the fish protein quality. For similar purposes, other low molecular weight compounds such as sucrose may also be used.
In contrast to the sodium chloride solutions, sucrose solutions produce a greater reduction in moisture content and lower solute impregnation during the osmotic dehydration process of meats. As sucrose is not a compound normally present in animal tissues, muscle cell permeability to sucrose could be very low. Also, many authors consider that a slight increase in solute molecular weight reduces the gain in solute for an equivalent water loss, which results in a greater weight reduction.[Citation6]
One might expect that the combination of two solutes, such as sucrose and sodium chloride, could provide the advantages of both, such as a high moisture reduction with a low impregnation rate,[Citation6,Citation2,Citation7] thus reducing the loss in fish protein quality. Changes in meat solute concentration can affect the configuration of muscle proteins causing denaturation, which can be considered as any modification of the protein structure (secondary, tertiary, or quaternary), without necessarily breaking the peptide chains in their primary structure. Denaturation causes an important reduction in protein solubility and consequently in the water binding capacity of the meat.
Protein denaturation is an irreversible endothermic order-disorder transition,[Citation8] which has been studied by thermal analysis.[Citation9–12] Park and Lanier[Citation11] studied the behaviour of the Tilapia myofibrillar proteins, such as myosin and actin, during the surimi process, and observed that the denaturation temperature (Td) of myosin remained fairly constant at around 58°C during the reduction of the particle size and subsequent leaching to produce surimi, whilst that of the actin Td decreased. Hastings et al.[Citation10] used DSC to verify that fish myosin was susceptible to denaturation during frozen storage and dehydration.
Meanwhile, although denaturation is an important property of the moist product, glass transition, is the most important for dried products.[Citation13–20] Glass transition is a second order phase change from a stable glassy solid to a rubbery (less stable) state.[Citation8] Knowledge of the glass transition temperature (Tg) is very important in stability studies and to determine the best storage condition for a dried product. Tg is also affected by the moisture content and/or solute concentration of foods.[Citation14] The most common method used to determine glass transition is the differential scanning calorimetry (DSC) that detects the change in heat capacity occurring over the transition temperature range.[Citation15] Aguilera et al.[Citation16] studied the glass transition temperature of hydrolysed fish protein and the collapse in structure, observing that an increase in water activity (aw) from 0 to 0.64 caused a decrease in Tg from 79 to −43°C. Inoue and Ishikawa,[Citation17] in a trial with fresh tuna (Thunnus obesus) meat and its filtrate, showed a great influence of the salt content on the glass transition temperature. Thus, considering the lack of information on the properties of tilapia muscle after osmotic dehydration using concentrated solutions of sucrose and sodium chloride, the objective of this work was to study the influence of sodium chloride and/or sucrose concentrations on protein denaturation and on the glass transition temperature of osmotically dehydrated tilapia fillets, as determined by differential scanning calorimetry (DSC).
MATERIAL AND METHODS
Nile tilapias (Oreochromis niloticus), stored on ice, were acquired at a local market and processed into fillets within 24 h after capture. The physical characteristics of each fish and fillet were as follows: fish weight, 300–350 g; fish length, 23–25 cm; average fillet thickness 1.33–1.55 cm and fillet density 1.065–1.072 g/cm.3
Osmotic Dehydration
Aqueous solutions of sucrose (66.67 g/100 g of water or 40% w/w), sodium chloride (35.14 g/100 g of water or 26%, w/w) and mixed solutions of sodium chloride and sucrose (66.67 g sucrose plus 35.14 g NaCl in 100 g of water) were used for the osmotic dehydration of the fillets. These concentrations were chosen based on previous work showing these values to be those leading to a higher mass transfer in the osmotic dehydration of tilapia fillets.[Citation7] The solutions were prepared using commercial sugar and salt and distilled water.
Tilapia fillets (Oreochromis niloticus) were soaked in the previously described binary or ternary solutions at a constant temperature of 20°C and a 1:10 product to solution ratio. Fillet samples were removed from the solution at regular intervals in order to obtain samples with increasing solute concentration. They were superficially drained and then gently dried with absorbent paper tissue and stored for 12 h at 4°C with the purpose of obtaining uniform distribution of the water and solutes in the samples. The fillets were then ground and aliquots taken for analysis. The sodium chloride content was determined by quantification of the Na+ ions using a flame absorption photometer (Analyser 910M, São Paulo, SP, Brazil). The sucrose content was determined by the Lane and Eynon method[Citation18] and the moisture content by oven drying to constant weight at 105°C.[Citation5]
Thermal Analyses
The state transitions of the fish fillets were studied with a temperature modulated differential scanning calorimeter (TMDSC-TA 2920, TA Instruments Inc., New Castle, DE, USA), using 25 mL/min dry helium as the purging gas. For trials at very low temperatures (≤ −70°C), a liquid nitrogen-cooling accessory was used to reduce the cell temperature but otherwise, a mechanical refrigeration system (RCS) was applied. The temperature and melting calibrations were performed with indium [mp = 156.6°C and ΔHm= 28.5 J/g] and milli-Q deionised water [mp = 0.0°C and ΔHm=333 J/g].
For the determination of the denaturation properties, the TMDSC was operated in the conventional mode from 15 to 90°C, with a heating rate of 10°C/min and with one empty pan as reference. Immediately after osmotic dehydration of the filets, samples of 10 to 15 mg (± 0.01 mg) were closed in hermetic aluminium pans (20 μl). The denaturation temperature (Td) and enthalpy (ΔHd) were considered as those corresponding to the maximum peak and to the area of the endothermic peak, respectively, both using the Universal Analysis V.2.5H software (TA Instruments Inc., New Castle, DE, USA). All analyses were run in triplicate. In order to study glass transition temperatures, the TMDSC was operated from -130 to 200°C in the dynamic mode with a heating rate of 3.5°C/min, period of 40 s and amplitude of 0.5°C, also with one empty pan as reference. Before heating, the pans were cooled to 8°C/min. The modulation conditions were selected according to the Lissajous figures method, as described by Georget et al.[Citation19]
According to Reading et al.,[Citation21] Baroni et al.,[Citation20] and Bell and Touma,[Citation22] the glass transition temperature can be observed as an inflexion on the reversible heat flux baseline, resulting from a change in material heat capacity. Glass transition temperatures were calculated from deconvoluted reversing signals.[Citation20] Data were treated with Thermal Solutions Software (TA Instruments, New Castle, DE, USA).
For the measurement of Tg, fresh and osmotically dehydrated fillets were frozen in liquid nitrogen and then freeze-dried (Bench Freeze-dryer Heto-FD3, Germany, −55°C, 0.05 bar) for 48 h. The dried samples were crushed and maintained in desiccators with P2O5 until constant weight was reached. These samples were considered to be “water-free” and then placed in the DSC aluminium pans, weighed and equilibrated over saturated salt solutions (aw = 0.11 to 0.85) in desiccators at 25°C until equilibrium was reached.[Citation20] The final water content was calculated from the mass variation of the samples. GAB model (EquationEq. 1) was used to describe water sorption isotherms.
RESULTS AND DISCUSSION
The kinetics of solute (sodium chloride and sucrose) gain by the tilapia fillets in the first seven hours of osmotic dehydration can be observed in . This period corresponds to the highest rate of solute gain and water loss. The kinetics of solute uptake presented similar behaviour to that of other solute uptake kinetics for the same solutes, carried out at a different volume rate between the solution and fillet (4:1) in a previous study.[Citation7] It is very clear that when using ternary solutions, the presence of one solute causes a lower gain of the other. After osmotic dehydrations, the solute concentrations of fillets were 14.3 g NaCl/100 g of sample and 11.7 g of sucrose/100 g of sample obtained using binary (NaCl or sucrose-water) solutions; and 7.1 g of NaCl/100 g sample and 7.6 g of sucrose/100 g of sample for the ternary (NaCl-water-sucrose) solutions.
Figure 1 The kinetics of the sodium chloride (NaCl) and sucrose contents of tilapia fillets during osmotic dehydration using binary solutions of NaCl and sucrose or ternary solutions (NaCl-water-sucrose). w.b. = wet basis.
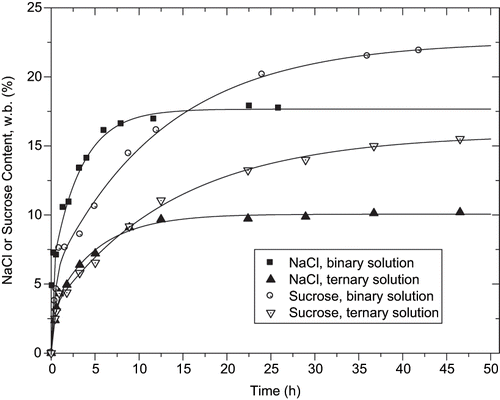
Denaturation Properties
DSC thermograms of tilapia fillets, both fresh and osmotically dehydrated in sodium chloride solutions, are shown in . It can be observed that the thermogram of the fresh fillet presented two important first order transitions, like endothermic peaks, with a less intense transition, like a shoulder. These curves are typical of the thermal behaviour of fish muscle proteins, as reported by several authors.[Citation11,Citation23–26] The first (lower temperature) endothermic transition with a peak temperature at 54.7 ± 0.2°C, may be associated with denaturation of the myosin (), and the second, at 75.2 ± 0.1°C, was due to that of the actin, both being contractile myofibrillar proteins. These results agree with those obtained by Monterrey-Quintero and Sobral,[Citation24] who reported the denaturation temperatures of myosin and actin from tilapia muscle as being 54 and 76°C, respectively. However, Park and Lanier,[Citation11] also working with tilapia muscle, found a different value for the denaturation temperature of myosin (59°C), but a similar value for that of actin (74°C). According to Monterrey-Quintero and Sobral,[Citation26] the observed intermediary transition is due to the denaturation of stromatic proteins such as collagen.
Figure 2 (A) DSC thermograms of tilapia muscle with different sodium chloride contents; (B) DSC thermograms of tilapia muscle with different sucrose contents; and (C) DSC thermogram of tilapia muscle with different NaCl and sucrose contents. Exothermal heat flow up.
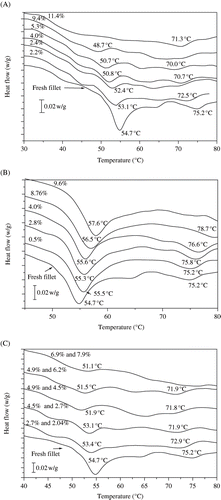
Table 1 Denaturation temperature (Td) and enthalpy (ΔH) of tilapia fillets after different impregnation treatments, measured by DSC
The effect of increasing sodium chloride concentration in the muscle on denaturation temperatures (Td) is evident from ; the denaturation temperatures of myosin and actin shifted towards lower values together with a reduction in denaturation enthalpy (). This reduction in Td is assumed to be due to a loss of protein stability (Park and Lanier[Citation24]). Nevertheless, actin proved to be more stable than myosin, showing a reduction in Td from 75 to 71°C as against 54 to 43°C for myosin, the salt content increasing from 0 to 11.4g/100 g fillet. At the higher muscle sodium chloride content (11.4g/100 g fillet), the endothermic transition due to myosin denaturation disappeared ().
On the other hand, the reduction in denaturation enthalpy is an indication that a fraction of the proteins was cold denaturated by contact with the solute. Similar results can be observed in the work of Furukawa et al.[Citation27] The conformational structure, and consequently the thermal stability of proteins, can be effectively affected by its chemical environment, such as the ionic strength.[Citation21] Harwalkar and Ma[Citation28] showed different behaviour, with an increase in the denaturation temperature of oat globulin as the sodium chloride content increased. According to Danilenko et al.,[Citation29] the improvement in heat stability of this kind of protein can be attributed to an augmentation of hydrophobic interactions in its native conformation. The difference in structure between globular and myofibrillar proteins may explain this different behaviour in the presence of salt.[Citation30]
DSC thermograms of tilapia fillet osmotically dehydrated in sucrose solutions are shown in . The presence of this sugar in the tilapia muscle, contrary to that observed with sodium chloride, increased the peak area corresponding to myosin denaturation, hiding the intermediary transition that appears between the peaks of the main proteins. The denaturation temperature of both proteins slightly increased, suggesting their stabilization. The same behaviour was also observed by Park and Lanier[Citation31] in the calorimetric study of tilapia muscle with 8% of sucrose, where the denaturation temperature of the myosin increased by 2.5°C. Wimmer et al.[Citation32] explained that the increase in the Td of a protein could be caused by the organisation of solvent (water) molecules around the protein molecules, producing an increase in hydrophobic interactions in these macromolecules. In other words, sucrose causes a decrease in surface hydrophobicity of the protein.[Citation33] The DSC thermograms of tilapia fillets soaked in ternary solutions (water-NaCl-sucrose) can be observed in . The overall behaviour is very similar to that observed with pure chloride sodium, as shown in , despite the presence of sucrose. Thus, the destabilizing effect of salt is more important than the stabilizing effect of sucrose. Comparing similar solute concentrations in the fillets, it can be observed that the myosin Td obtained from fillets dehydrated in ternary solutions with concentrations to the order of 4.9g NaCl/100 g fillet and 6.2 g sucrose/100 g fillet, was 51.9°C, while for fillets with 5.3 g NaCl/100 g fillet and 4–8 g sucrose/100 g fillet dehydrated in binary solutions, the myosin Td was 50.7°C and 56.0°C, respectively. Therefore, it must be pointed out that the presence of sucrose did not protect the protein against the ionic strength of the salt. Park and Lanier[Citation31] also observed this behaviour, as did Furukawa et al.,[Citation27] who reported that sucrose addition did not restore the protein stability displaced by the salt.
Glass Transition
Sample conditioning at different water activities led to the water vapour sorption isotherms at 25°C shown in . The obtained parameters as well as the determination coefficients are shown on . The behaviour of these sorption isotherms can be explained in two regions. Up to water activity (aw) values around 0.5, the sample equilibrium moisture content (Xeq) was almost the same. On the other hand, for aw values above 0.5, the sample moisture content increased markedly with increasing water activity, depending on the presence of solutes. A clear synergistic effect can be observed when both solutes are used in the fillets.
Table 2 GAB parameters for Tilapia fillet
All figures show midpoint glass transition temperature. shows that with samples conditioned at low water activities (0.11), the effect of impregnated solutes on the Tg was not very clear, but for high aw values (), the samples presented different Tg values. It can be seen that salt and sucrose had a plasticizing and antiplasticizing effect, respectively, since the glass transition of samples osmotically dehydrated with these solutions occurred at lower and higher temperatures respectively, when compared to samples with no solute. Also, the effect observed in samples osmotically dehydrated in ternary solutions was intermediary. This behaviour was obviously also affected by samples moisture content. For samples conditioned at aw = 0.11, the final equilibrium moisture contents were very close, remaining between 0.03 and 0.07, whilst at high aw, the sample moisture content was very different, as can be seen in . Thus, it can be considered that the effect of the impregnated solute on Tg was indirect, affecting the respective equilibrium moisture content of the samples.
Figure 4 (A) Thermograms of fresh and osmotically dehydrated tilapia fillets in different solutions at aw= 0.11; and (B) thermograms of fresh and osmotically dehydrated tilapia fillets in different solutions at aw= 0.85. Exothermal heat flow up.
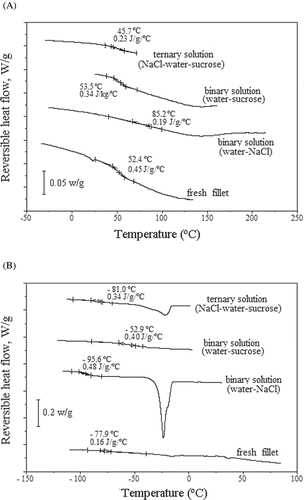
The glass transitions calculated as the initial, mid and end transitions temperatures, according to Rahman et al.,[Citation34] are shown in . The effect of water activity on the Tg of osmotically dehydrated samples can be better observed in . In spite of the wide temperature range, it can be observed, as a general tendency, that Tg shifts towards lower values as a consequence of increases in the water activity, due to the plasticizing effect of water.[Citation8] Nevertheless, at high water activity this shift became more clear and sharp due to the samples moisture content increasing. For fillets osmotically dehydrated in NaCl and sucrose aqueous solutions, this behaviour was more evident, which is a characteristic of a multicomponent system.[Citation35]
Figure 5 (A) Thermograms of osmotically dehydrated tilapia fillets in aqueous NaCl solutions at different aw values; (B) thermograms of osmotically dehydrated tilapia fillets in aqueous sucrose solutions at different aw values; and (C) thermograms of osmotically dehydrated tilapia fillets in ternary solutions (NaCl-water-sucrose). Exothermal heat flow up.
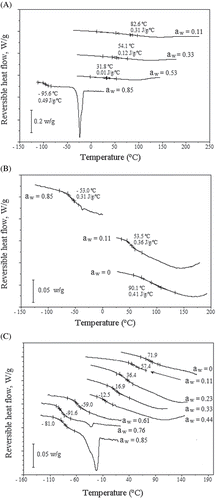
Table 3 Glass transition temperature of Tilapia fillet after different impregnation treatments, measured by DSC
For the fillets osmotically dehydrated in sodium chloride solutions (), Tg decreased from 83 to −96°C when aw increased from 0.11 (Xeq = 0.03, d.b.) to 0.85 (Xeq = 0.88, d.b.). However, for osmotically dehydrated fillets in sucrose solutions (), the Tg temperature only decreased to −53°C as the water activity increased from 0 to 0.85, because the equilibrium moisture content reached a less important value (Xeq = 0.33, d.b.) than that obtained with sodium chloride solutions. An intermediary behavior was clearly observed for samples dehydrated in ternary solutions (), for which Tg decreased from 72 to −81°C, as aw increased from 0 to 0.82 (Xeq = 0.63, d.b.). In , for untreated fillets, conditioned at several different final relative moistures, can be observed similar behavior to the thermograms of .
The plasticizing effect of sample moisture content can be better visualized in . In some cases, such as for samples osmotically dehydrated with the salt and ternary solutions, the glass curves were clearly visible.[Citation36] Unfortunately, for other treatments, there was not enough data to observe the parabolic decay of Tg as a function of moisture content. The Tg values determined in this work agree with those observed by Inohue and Ishikawa[Citation17] for a tuna fish variety, but were higher than those measured by Aguilera et al.[Citation16] for hydrolysed fish protein, certainly due to the lower molecular weight of these proteins. The Tg values determined in this work suggest that the dehydrated tilapia fillets can be adequately stored at room temperature with a water activity of 0.44. The difference between storage and glass transition temperatures (T-Tg) has been used as a fitting parameter to describe the rate of product physical change.[Citation37] Deteriorative changes can be prevented as a consequence of the reduction in T- Tg, resulting in an increase in the system viscosity and consequent decrease in molecular mobility.
CONCLUSIONS
The salt migrating into the fillets caused an important destabilisation of the muscle proteins. The denaturation temperatures and denaturation enthalpies of the tilapia myofibrillar proteins decreased with increase in sodium chloride content of the fillets. Myosin was more sensitive to salt than actin and sucrose presented a stabilisation effect. Myosin and actin denaturation temperatures slightly increased with an increase in sucrose content of the muscle. For samples dehydrated in ternary solutions, the denaturation temperature of the myofibrillar proteins decreased with increase in sodium chloride content, i.e., the sucrose did not protect the proteins against the destabilizing effect of the salt. On the other hand, the effect of solutes on the protein glass transition temperatures was indirect, affecting the sample equilibrium moisture content. The most significant effect on the glass transition temperature was observed for samples dehydrated in salt solutions, presenting higher equilibrium moisture contents after conditioning.
ACKNOWLEDGMENTS
This work was supported by grants from FAPESP (Fundação de Amparo a Pesquisa do Estado de São Paulo), the Brazilian National Research Council (CNPq), and CAPES-Grices (Brazil-Portugal International Cooperation Project).
Notes
5. Medina-Vivanco, M. Estudo da difusão de cloreto de sódio no filé de tilápia (Oreochromis niloticus) utilizando volumes limitados de salmoura. Dissertação (Mestrado em Engenharia de Alimentos), Faculdade de Engenharia de Alimentos, Universidade Estadual de Campinas, 1998, 103.
30. Cheftel, J.C.; Cuq, J.L. Aminoacidos, Peptidos y Proteinas. Quimica de los Alimentos; Department of Food Science, University of Wisconsin-Madison; O.R. Fennema, Director; Editorial Zaragoza. 1984, 275–412.
REFERENCES
- Raoult-Wack , A.L. , Guilbert , S. , Le Maguer , M. and Rios , G. 1991 . Simultaneous Water and Solute Transport in Shrinking Media—Part 1. . Application to Dewatering and Impregnation Soaking Process Analysis (Osmotic Dehydration) Drying Technol , 9 ( 3 ) : 589 – 612 .
- Bohuon , P. , Collignan , A. , Rios , G.M. and Raoult-Wack , A.L. 1998 . Soaking Process in Ternary Liquids: Experimental Study of Mass Transport Under Natural and Forced Convection . J Food Eng , 37 : 451 – 469 .
- Del Valle , F.R. and Nickerson , J.T. 1967 . Studies on Salting and Drying Fish I: Equilibrium Considerations in Salting . J. Food Sci , 32 : 173 – 179 .
- Zugarramurdi , A. and Lupin , H. 1976 . Estudio Sobre el Salado de Anchoíta. I. Equilibrio y Perfiles de Concentración. . Rev. Lat. Am. J. Chem. Eng. Appl. Chem , 6 : 79 – 90 .
- 5. Medina-Vivanco, M. Estudo da difusão de cloreto de sódio no filé de tilápia (Oreochromis niloticus) utilizando volumes limitados de salmoura. Dissertação (Mestrado em Engenharia de Alimentos), Faculdade de Engenharia de Alimentos, Universidade Estadual de Campinas, 1998, 103.
- Collignan , A. and Raoult-Wack , A.L. 1994 . Dewatering and Salting of Cod by Immersion in Concentrated Sugar/Salt Solutions . LWT Food Sci.Technol , 27 : 259 – 264 .
- Medina-Vivanco , M. , Sobral , P.J.A. and Hubinger , M.D. 2002 . Osmotic Dehydration of Tilapia Fillets in Limited Volume of Ternary Solutions . Chem. Eng. J , 86 : 199 – 205 .
- Roos , Y. 1995 . Characterization of Food Polymers Using State Diagrams . J. Food Eng , 24 : 339 – 360 .
- Wright , D.J. , Leach , I.B. and Wilding , P. 1977 . Differential Scanning Calorimetric Studies of Muscle and Its Constituent Proteins . J. Sci. Food Agric , 28 : 557 – 564 .
- Hastings , R.J. , Rodger , G.W. , Park , R. , Matthews , A.D. and Anderson , M.E. 1985 . Differential scanning calorimetry of fish muscle: The effect of processing and species variation . J. Food Sci , 50 : 503 – 510 .
- Park , J.W. and Lanier , T.C. 1989 . Scanning Calorimetric Behavior of Tilapia Myosin and Actin Due to Processing of Muscle and Protein Purification . J. Food Sci , 54 : 49 – 51 .
- Sobral , P.J.A. , Ocuno , D. and Savastano Jr , H. 1998 . Preparo de proteínas miofibrilares de carne e elaboração de biofilmes com dois tipos de ácidos: Propriedades Mecânicas . Braz. J. Food Technol , 1 : 44 – 52 .
- Rahman , M.S. , Kasapis , S. , Guizani , N. and Al-Amri , O.S. 2003 . State Diagram of Tuna Meet: Freezing Curve and Glass Transition . J. Food Eng , 57 : 321 – 326 .
- Champion , D. , Le Meste , M. and Simatos , D. 2000 . Towards an Improved Understanding of Glass Transitions and Relaxation in Foods: Molecular Mobility in the Glass Transition Range . Trends Food Sci. Tech , 11 : 41 – 55 .
- Rahman , M.S. 2004 . State Diagram of Date Flesh Using Differential Scanning Calorimetry (DSC). . Int. J. Food Prop , 7 ( 3 ) : 407 – 428 .
- Aguilera , J.M. , Levi , G. and Karel , M. 1993 . Effect of Water Content on the Glass Transition and Caking of Fish Proteins Hydrolizates . Biotechnol. Prog , 9 : 651 – 654 .
- Inoue , C. and Ishikawa , M. 1997 . Glass Transition of Tuna Flesh at Low Temperature and Effects of Salt . J. Food Sci , 62 ( 3 ) : 496 – 499 .
- Ranganna , S. 1977 . Manual of Analysis of Fruit and Vegetable Products , 634 Mysure, New Delhi : Limited McGraw-Hill Publishing Company . Central Food Technological Research Institute:
- Georget , D.M.R. , Smith , A.C. and Waldrow , K.W. 1999 . Thermal Transitions in Freeze-dried Carrot and Its Cell Wall Components . Thermochim. Acta , 332 : 203 – 211 .
- Baroni , A.F. , Sereno , A.M. and Hubinger , M.D. 2003 . Thermal Transitions of Osmotically Dehydrated Tomato by Modulated Temperature Differential Scanning Calorimetry . Thermochim. Acta , 395 ( 1–2 ) : 237 – 249 .
- Reading , M. , Elliot , D. and Hill , V.L. 1993 . A New Approach to the Calorimetric Investigation of Physical and Chemical Transitions . J. Therm. Anal. Calorim , 40 : 949 – 955 .
- Bell , L.N. and Touma , D.E. 1996 . Glass Transition Temperatures Determined Using a Temperature-cycling Differential Scanning Calorimeter . J. Food Sci , 61 ( 4 ) : 807 – 810 .
- Wright , D.J. and Wilding , P. 1984 . Differential Scanning Calorimetric Study of Muscle and Its Proteins: Myosin and Its Subfragments . J. Sci. Food Agric , 35 : 357 – 372 .
- Park , J.W. and Lanier , T.C. 1987 . Combined Effect of Phosphates and Sugar or Polyol on Protein Stabilization of Fish Myofibrils . J. Food Sci , 52 : 1509 – 1513 .
- Ma , C.Y. and Harwalkar , V.R. 1991 . Thermal Analysis of Food Proteins . Adv. Food Nutrition Res , 35 : 317 – 367 .
- Monterrey-Quintero , E.S. and Sobral , P.J.A. 2000 . Preparo e Caracterização de Proteínas Miofibrilares de Tilapia do Nilo (Oreochromis niloticus) para Elaboração de Biofilmes . Pesqui Agropecu Bras , 35 ( 1 ) : 179 – 190 .
- Furukawa , V.A. , Sobral , P.J.A. , Habitante , A.M. and Gomes , J.D.F. 2004 . Análise Térmica da Carne de Coelhos . Ciên & Tecnol Alimentos , 24 ( 2 ) : 265 – 269 .
- Harwalkar , V.R. and Ma , C.Y. 1987 . Study of Thermal Properties of Oat Globulin by Differential Scanning Calorimetry . J. Food Sci , 52 : 394
- Danilenko , A. , Rogova , E. , Bikbov , T. , Grinberg , V.Y. and Tolstogusov , V. 1985 . Stability of 11S Globulin from Vicia Faba Seeds. Studies Using Differential Scanning Microcalorimetry . Int. J. Pept. Res. Ther , 26 : 5
- 30. Cheftel, J.C.; Cuq, J.L. Aminoacidos, Peptidos y Proteinas. Quimica de los Alimentos; Department of Food Science, University of Wisconsin-Madison; O.R. Fennema, Director; Editorial Zaragoza. 1984, 275–412.
- Park , J.W. and Lainer , T.C. 1990 . Effects of Salt and Sucrose Addition on Thermal Denaturation and Aggregation of Water-leached Fish Muscle . J. Food Biochem , 14 : 395 – 404 .
- Wimmer , R. , Olsson , M. , Petersen , M.T.T. , Hatti-Kaul , R. , Petersen , S.B. and Muller , N. 1997 . Towards a Molecular Level Understanding of Protein Stabilization: The Interaction between Lysozyme and Sorbitol . J.Biotechnol , 55 : 85 – 100 .
- Ismond , M.A.H. , Murray , E.D. and Arntfield , S.D. 1988 . The Role of Noncovalent Forces and Micelle Formation by Vicilin from Vicia Faba III. The Effect of Urea, Guanidine Hydrochloride and Sucrose on Protein Interactions . Food Chem , 29 : 189
- Rahman , M.S. , Sablani , S.S. , Al-Habsi , N. , Al-Maskri , S. and Al-Belushi , R. 2005 . State Diagram of Freeze-dried Garlic Powder by Differential Scanning Calorimetry and Cooling Curve Methods . J. Food Sci , 70 ( 2 ) : E135 – E141 .
- Hashimoto , T. , Hagiwara , T. , Suzuki , T. and Takai , R. 2003 . Study on the Glass Transition of Katsuobushi(Boiled and Dried Bonito Fish Stick) by Differential Scanning Calorimetry and Dynamic Mechanical Analysis . Fisheries Science , 69 : 1290 – 1297 .
- Sobral , P.J.A. , Monterrey-Quintero , E.S. and Habitante , A.M.Q.B. 2002 . Glass Transition of Nile Tilapia Myofibrillar Protein Films Plasticized by Glycerin and Water . J. Therm. Anal. Calorim , 67 ( 2 ) : 499 – 504 .
- Roos , Y. and Karel , M. 1992 . Cristallization of Amorphous Lactose . J. Food Sci , 57 ( 3 ) : 775 – 777 .