Abstract
The aim of this research was to investigate the effect of mineral fortification on the textural and oxidative stability of reduced-fat spreads produced using κ-carrageenan. Model systems were prepared containing varying amounts of zinc, copper, and iron. The hardness of the spreads stored at 5°C and 25°C was assessed over time using a penetrometer (20° cone), and it was observed that samples were generally weaker compared to the controls. Samples containing the transition minerals iron and copper were very susceptible to oxidation, becoming rancid rapidly. Low levels of zinc addition, between 2–10% of the recommended daily allowance (RDA), led to spreads with acceptable oxidative stability and a textural profile comparable to the controls over the storage period examined.
INTRODUCTION
Butter and margarine, the traditional oil continuous products for spreading, contain 80% fat. The large fat phase determines the sensory attributes by flavour release, thickness, sweetness, smoothness, and ‘melt in the mouth’ behaviour. It also dictates the physical properties, rheological characteristics, chemical reactions and microbiological stability of traditional products.[Citation1 ,Citation2] Additionally, such products derive much of their structure from the fat phase, and few other ingredients were used to tailor the properties of these spreads.[Citation3,Citation4] In the last three decades a number of economic, medical and social factors have all contributed to the decline in butter consumption, amongst which are widespread availability of fridges, trends towards healthier lifestyles and diets, and very high fat consumption, particularly in the Western world.[Citation4–7] Attempts have been made, therefore, to produce spreads of a high dietary value containing half, a quarter or even less of the fat of butter which, however, retain its desired appearance, texture, flavour and sensory characteristics.[Citation2,Citation7] In products containing less than 20% fat the emulsion phase inverts, and the fat phase is dispersed within the aqueous matrix. Both fat- and water- continuous preparations contain a disproportionate aqueous phase which will flow unless it is structured somehow.[Citation8] Spreads have to combine two properties that are somewhat difficult to reconcile in practice. On the one hand, a spread requires a relative firm consistency, and a convenient way to do this is by creating a network in the product. On the other hand, a more or less plastic rheology is desirable so that the product does not become much thinner during spreading.[Citation4] Today, biopolymers such as protein (from egg, milk, gelatin), intact and modified starch, and/or polysaccharides (e.g., carrageenan, alginate, pectin) are used to provide structure to the aqueous phase of these food systems. Since no biopolymer on its own can provide the required structure alongside a ‘plastic’ flow, mixtures have been used to achieve the desired combination of properties.[Citation8]
Another major nutritional issue in addition to the high fat intake, both in developing and developed countries is that an appreciable fraction of the population, particularly young children, adolescents, the elderly, and women of child-bearing age can suffer from nutrient deficiencies at borderline or pathological levels.[Citation9] Several strategies have been adopted at times in an attempt to address this micronutrient malnutrition.[Citation10,Citation11] Micronutrient fortification is now a highly relevant tool worldwide for overcoming micronutrient deficiency,[Citation12] and continues to be one of the main strategies employed in tackling those global.[Citation11,Citation13] Indeed mandatory fortification programmes are used throughout the world using various food vehicles in delivering a series of nutrients.[Citation10] in an attempt to meet the recommended intakes.[Citation14,Citation15] Usually the cheapest approach is staple food fortification, however, fortification of spreads has recently been proposed and attempted.[Citation16]
In this research, an attempt was made to utilise the properties of κ-carrageenan, a polysaccharide, in terms of mineral binding in addition to its ‘structuring’ contribution and explore the potential of producing reduced-fat spreads that have been fortified with zinc, copper and iron, whose intakes are traditional and emerging causes for concern.[Citation1,Citation2] Carrageenans are high-molecular weight linear sulphated polysaccharides extracted from many species of red seaweed,[Citation17] comprising three major fractions (κ-, ι-iota and λ-lambda). κ-carrageenan exists as random coils at high temperatures, a coil-to-helix transition occurs on cooling below a certain temperature, which is dependent on the ionic environment.[Citation17] Cations contribute to the formation of electrostatic bonds between the negatively charged double-helices, thereby causing aggregation. The coil-to-helix transition temperature is directly related to cation concentration.[Citation18] It has been suggested that the presence of divalent cations such as Ca2+ may link the negatively charged polyions and that these Ca2+ bridges reinforce the gel network structure.[Citation19] Although research has been carried out on the effects of various cations,[Citation20] little is known about the effects of divalent cations from different metals such as zinc or copper or for that matter the effects of trivalent cations from metals such as iron. Fortifying low-fat spreads with those cations is expected to have an effect on both the oxidative and textural stability of the spreads, therefore the peroxide values of the produced spreads were monitored over the course of storage and their textural stability was evaluated using the penetrometer, a tool first used for the evaluation of spreads and butters in 1959,[Citation21] but still very widely used, [Citation6],Citation22–25 This is a simple, compression and shear type test which can give an unequivocal characterisation of the mechanical properties of the product.[Citation26]
MATERIALS AND METHODS
Production of Reduced-fat Spreads
A ten kg reduced-fat spread (60% fat) with a commercial-type formulation was manufactured with the following formulation: an oil phase containing 5860 g vegetable oil blend (code BDG:WPGLTC, Cargill, France) and 60 g monoglyceride emulsifier (Paalsgaard 0291, Grinstead A/S, Braband, Denmark); and an aqueous phase containing 3816 g distilled and deionised water, 170 g salt (Salt Union, Cheshire, England), 50 g sodium caseinate (Dairygold Foods, Mitchelstown, Co Cork, Ireland), 25 g sodium alginate (Grinstead Alginate FD 155, Danisco, Denmark), 10 g buttermilk powder (Tipperary Co-op, Tipperary, Ireland) and 2.5 g citric acid (Univar Ireland Ltd., Dublin, Ireland).
Using the same manufacturing process, a reduced-fat spread was prepared by replacing the aqueous phase with 5975 g water and 25 g κ-carrageenan (Deltagel P379; Kerry Biosciences, Carragiline, Co. Cork, Ireland). The κ-carrageenan contained 5.8% potassium, 0.4% sodium, 0.4% calcium and 0.4% magnesium. A series of fortified reduced-fat spreads were also prepared by including iron, zinc or copper at levels of 0.08-20 mM (ion concentration in final product). The iron (iron (III) chloride), zinc (zinc chloride), and copper (copper (II) chloride dihydrate) were reagent grade or better and obtained from BDH Chemicals (Poole, England). The levels of addition were such as to cover 0-100% of the recommended RDA,[Citation13,Citation14]
The pilot scale processing was carried out in a Perfector scraped surface heat exchanger (Gerstenberg and Agger, Copenhagen, Denmark) with a 25-litre jacketed tank connected to a 3-piston pump, which fed the Perfector unit. The initial mixing of the oil and aqueous phases was carried out in a Silverson mixer (model AX3, Silverson Machines Ltd., Waterside, Chesham, Bucks, England). The production process is presented schematically in .
Peroxide Value
Peroxide values were measured to determine oxidation according to the official AOAC method.[Citation27] All determinations were carried out in duplicate, after storage at 5°C for 1, 8, and 15 days, unless values were in the region of 5 mEq O/kg in which cases the samples were considered spoilt and were discarded.
Measurement of the Hardness of Spreads with Cone Penetrometer
A Seta 1719 Universal Penetrometer and Controller MK.VI supplied by Stanhope-Seta Ltd. Station Road, Chertsey, Surrey, KT16 8BG, England, was used for measurement of hardness. A 20° stainless steel cone was used, which weighed 76.54 g. Release time was 5 s. Samples were held at testing temperature for at least 24 hours before testing. Penetration readings (in tenths of a millimetre) were obtained and yield values were calculated using EquationEq. 1:
where C is the yield value in kg/m 2; W is the weight of cone and shaft assembly in grams; K is a factor dependant on the angle of the cone (for a 20 degree cone K = 19000); and P is the penetration depth (mm) reached in 5 seconds. All measurements were performed in triplicate.
Statistical Analysis
Analysis of variance (ANOVA) was carried out using SigmaStat (version 3.0; Jandel Scientific, Corte Madera, CA, USA). Student-Newman-Keuls pairwise-comparison test was used to determine differences between treatment means. Treatment means were considered significantly different at P ≤ 0.05.
RESULTS AND DISCUSSION
Oxidative Stability
The peroxide values of samples stored at 5°C were measured and the results are summarised in . For the industry the samples are considered rancid and unacceptable when peroxide values (PV) are over 5, while ideally PV should be below 1–1.5. In this trial, therefore we stopped measuring and discarded the samples once the PV was in the region of 5. In addition to the fortified reduced-fat spreads, a control containing κ-carageenan but no mineral salts and a control based on a commercial recipe were also tested. As can be seen from , the commercial recipe spread was the most resistant to oxidation. Amongst the rest of the sample, the κ-carageenan control exhibited the greatest stability against oxidation, following the trend suggested previously by Keogh [Citation28] for spreads stored at 4°C. The addition of Fe in any level caused unacceptable rancidity levels in the spreads even after one day of storage, while after 7 days all samples were completely spoilt. Spreads containing lower concentrations of Cu (0.08–4 mM) gave acceptable PV after one day of storage, however after 8 days all samples containing >4 mM were rancid, while the lower concentration also had peroxide values that would not be acceptable in an industrial setting. The effect of added Zn in the peroxide values of the reduced-fat spreads was much lower. Indeed the samples containing 0.3 mM were comparable with the κ-carageenan control throughout the storage period, but still had higher values than the commercial control. Briend[Citation16] has suggested that limited contact with oxygen and humidity, and absence of water are all very favourable factors for obtaining a prolonged shelf life for a fortified spread by increasing its resistance to oxidation. In our case however it would appear that the added ions negated these factors, with the trivalent Fe exhibiting, as anticipated, the most susceptible profile. Transition metals such as copper and iron are in particular expected to catalyse, and thus accelerate the breakdown of oxidation products.[Citation29,Citation30] This breakdown is leading to a series of secondary oxidation products which are associated with the deterioration of the organoleptic properties, and in particular aldehydes, due to their very low flavour threshold.[Citation30]
Table 1 Peroxide values of reduced–fat spreads
Overall, the results suggested that mineral fortified reduced-fat spreads were inferior to the controls, with only those containing lower concentrations of Zn having acceptable PV after 15 days storage. The carrageenan-metal complexes are known to form gels,[Citation17–19] and this was confirmed by the reduced-fat spread production, however, it is believed that in our case the binding was not complete thus allowing free metal ions in the spreads which in turn promoted rancidity through autoxidation. Of course some well established methods exist for the prevention of oxidation[Citation31] and a future approach could include the addition to the spreads of a variety of antioxidants.[Citation30,Citation31]
Spread Hardness
The texture of spreads is mostly determined by the properties of the fat phase.[Citation4] In the production of spreads, crystallisation of fat occurs on cooling at lower temperatures. The agglomerated fat crystals dispersed in liquid oil play a critical role in determining the texture/consistency of the system.[Citation4], Citation32 The textural stability of the spreads was monitored using the penetrometer. The results for the samples stored at 5°C are presented in , , and . The significances of the differences are presented in . For the Zn-containing samples stored at 5°C () a clear trend was observed whereby the yield values increased between 1–14 days storage. No further increase was observed upon storage for a third week (days 14–21), however, over the 21 days of storage the control samples (no Zn added) continued to strengthen. Maximum strength was observed for spreads containing 1.5 mM Zn. For samples measured after one and seven days the addition of any amount of Zn had a weakening effect when compared with the control, and this was also true for samples stored for 21 days. It would therefore appear that addition of Zn had a weakening effect on the spreads and, furthermore, no additional hardening was noted after the second week of storage (). These differences between control and Zn-containing samples could be due to the different extent of fat crystallisation. Indeed, for samples stored at 5°C crystallisation of fat would strengthen the product's matrix (while such strengthening does not appear with samples stored at 25°C). The presence of Zn would induce oxidation, which in turn would counteract some of the strengthening caused due to the crystallisation. Importantly, the zinc-induced oxidation prohibits further hardening of the samples after two weeks of storage.
Figure 2 Effect of Zn fortification on the textural stability of reduced-fat spreads stored at (a) 5 and (b) 25°C for 1, 7, 14, and 21 days. Average of three measurements, error bars represent standard deviation.
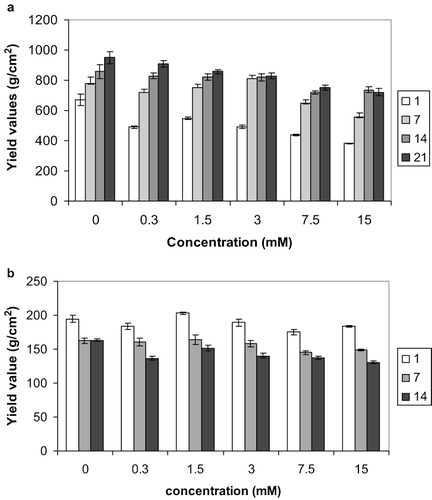
Figure 3 Effect of Cu fortification on the textural stability of reduced-fat spreads stored at (a) 5 and (b) 25°C for 1, 7, 14, and 21 days. Average of three measurements, error bars represent standard deviation.
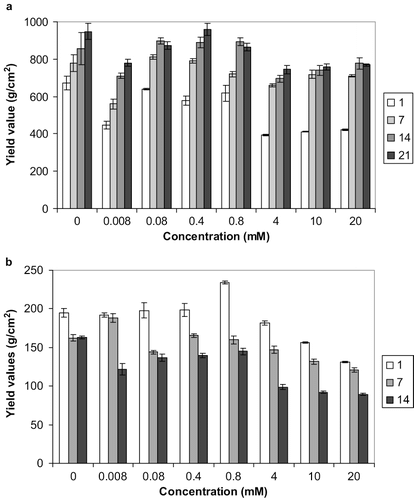
Table 2 Statistical significance of mean yield values differences for reduced fat spreads fortified with various amounts of minerals and stored at 5 and 25 °C. Different letters indicate significant difference (p > 0.05). Letters a-f refer to differences between concentrations within a certain storage time (comparison across rows), and letters w-z refer to differences over storage within a concentration (comparison within columns)
Figure 4 Effect of Fe fortification on the textural stability of reduced-fat spreads stored at (a) 5 and (b) 25°C for 1, 7, 14, and 21 days. Average of three measurements, error bars represent standard deviation.
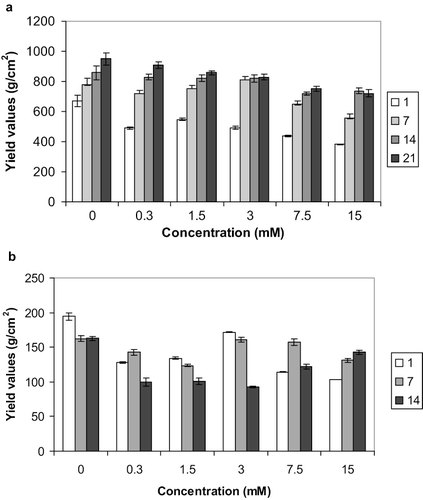
The samples fortified with Cu and stored at 5°C () all exhibited a weaker behaviour than the control after 1 day of storage (). Samples throughout the Cu concentration range got stronger with storage up to 14 days, but upon storage for a third week (days 14–21) there was no clear pattern as some samples kept strengthening while others did not. The stronger samples were those containing 0.4mM Cu, which were comparable with the non-mineral containing control throughout the storage period (). The spreads containing the highest concentrations of Cu (4, 10, and 20 mM) weakened noticeably compared with the control after one day storage but seemed to recover most of the yield values upon further storage, without however reaching the levels of the control.
Fe-fortified spreads when stored at 5°C () exhibited a different behaviour when compared with the Zn- and Cu- fortified samples. These samples strengthened throughout the storage period of 21 days, the only exception being the sample with the highest Fe concentration (15 mM). The highest yield values were observed for samples containing 0.3 mM Fe, while increasing Fe concentration lead to progressively lower values over the storage period. When compared to the control, Fe-containing samples were consistently weaker, throughout the trials ().
In contrast, samples stored at 25°C showed a different behaviour (, , ). Their highest yield values were observed after 1 day's storage and all Zn-containing spreads consistently became weaker upon prolonged storage (). The control samples behaved differently, in that they appeared to be unaffected and did not deteriorate further after the first week of storage, i.e., there was no significant (P ≤ 0.05) difference in the yield values for samples stored for 7 and 14 days (). As for the samples stored at 5°C, the highest yield values, corresponding to the stronger samples, were observed for the spreads containing 1.5 mM Zn. Notably, for the samples stored at 25°C, spreads containing Zn were not weaker than the control for the first week, but did appear to be weaker after 14 days of storage (). With storage, the fat crystals are probably the main emulsion stabilising factor in the spreads, so storage at higher temperatures results in elimination of this emulsion stabilising effect of the fat crystals.[Citation33] This is a result of the commercial necessity whereby a spread would be required to lose some of its consistency in the mouth, which is caused by melting of the fat phase as well as dispersal of the spread.[Citation4] This behaviour becomes more prominent at higher temperatures, which more closely resemble the temperature of the mouth.
The Cu-containing samples stored at 25°C (), as for the case of Zn, weakened throughout the storage period, but noticeably the 0.8 mM Cu-containing sample was stronger than the control after 1 day storage. Interestingly the sample containing the lowest amount of Cu (0.008 mM) had the highest yield values after 7-day storage. For spreads containing iron stored at 25°C (Fig.4b), no obvious pattern was observed. Some of the samples progressively weakened, while others strengthened over time. The highest yield values after one and seven days storage were exhibited by the spread containing 3mM Fe, while after 14 days the sample with the maximum content of Fe (15 mM) was the strongest. All samples however were consistently weaker (lower yield values) than the control ().
CONCLUSIONS
The results confirmed that it was possible to use of κ-carageenan for the binding of mineral salts. The reduced-fat spreads produced, however, appeared to be more susceptible to oxidation, particularly those containing Cu and Fe. Samples containing 0.3 and 1.5mM Zn (corresponding to 2 and 10% of the recommended RDA, based on a 25g serving) were affected much less by oxidation, while also exhibiting comparable textural stability compared with the non-mineral containing spread. It would appear, therefore, that reduced-fat spreads fortified with Zn can be readily produced with acceptable oxidative stability without any major detrimental effects texturally. The combined effect of mineral fortification and antioxidant addition needs to be established through further work.
ACKNOWLEDGMENTS
The authors would like to acknowledge the help of J. Roche in the preparation of the spreads, and J. O'Reilly for useful comments and advice.
REFERENCES
- Subramanian , R. , Muthukumarappan , K. and Gunasekaran , S. 2006 . Linear Viscoelastic Properties of Regular- and Reduced-Fat Pasteurized Process Cheese During Heating and Cooling . Int. J. Food Properties , 9 ( 3 ) : 377 – 393 .
- Chronakis , I.S. and Kasapis , S. 1995 . A Rheological Study on the Application of Carbohydrate-Protein Incompatibility to the Development of Low Fat Commercial Spreads . Carbohydr. Polym. , 28 ( 4 ) : 367 – 373 .
- Domagala , J. , Sady , M. , Grega , T. and Bonczar , G. 2005 . The Influence of Storage Time on Rheological Properties and Texture of Yoghurts with the Addition of Oat-Maltodextrin as the Fat Substitute . Int. J. Food Properties , 8 ( 3 ) : 439 – 448 .
- Bot , A. and Vervoort , S. 2006 . “ Hydrocolloid Functionality in Spreads and Related Products ” . In Gums and Stabilisers for the Food Industry 13 , Edited by: Williams , P.A. and Phillips , P.A. 381 – 394 . Cambridge : Royal Society of Chemistry .
- Keogh , M. K. 1993 . “ The Stability to Inversion of a Concentrated Water-in-Oil Emulsion ” . Cork, Ireland : PhD Thesis; University College Cork .
- Patel , A.A. and Gupta , S.K. 1988 . Studies on a Soy-Based Low-Fat Spread . J. Food Sci. , 53 ( 2 ) : 455 – 459 .
- Keogh , M. 1995 . “ Chemistry and Technology of Milk Fat Spreads ” . In Advanced Dairy Chemistry, Volume 2 Lipids; Fox, P.F , 213 – 246 . London : Chapman & Hall .
- Evangeliou , V. , Alevisopoulos , S. and Kasapis , S. 1997 . Application of Stress-Controlled Analysis to the Development of Low Fat Spreads . Journal of Texture Studies , 28 : 319 – 335 .
- Richardson , D.P. 1990 . Food Fortification . Proc. Nutr. Soc. , 49 : 39 – 50 .
- Darnton-Hill , I. and Nalubola , R. 2002 . Fortification Strategies to Meet Micronutrient Needs: Successes and Failures . Proc. Nutr. Soc. , 61 : 231 – 241 .
- Fletcher , R.J. , Bell , I.P. and Lambert , J.P. 2004 . Public Health Aspects of Food Fortification: A Question of Balance . Proc. Nutr. Soc. , 63 : 605 – 614 .
- Rosado , J.L. 2003 . Zinc and Copper: Proposed Fortification Levels and Recommended Zinc Compounds . J. Nutr. , 133 ( 9 ) : 2985S – 2989S .
- FAO . 1996 . “ Food Fortification: Technology and Quality Control ” . In Fao Food and Nutrition , Vol. 60 , Rome : FAO .
- FAO/WHO . 1998 . Vitamin and Mineral Requirements in Human Nutrition: Report of a Joint Fao/Who Expert Consultation , 2nd , Bangkok : FAO/WHO .
- Scientific Committee on Food (Scf) Tolerable Upper Intake Levels for Vitamins and Minerals. 2003 http://europa.eu.int/comm/food/fs/sc/scf/out80_en.html (Accessed: 22 May 2007 ).
- Briend , A. 2001 . Highly Nutrient Dense Spreads: A New Approach to Delivering Multiple Micronutrients to High Risk Groups . Br. J. Nutr. , 85 ( Suppl. 2 ) : S175 – S179 .
- Viebke , C. , Borgstrom , J. and Piculell , L. 1995 . Characterisation of Kappa- and Iota-Carrageenan Coils and Helices by Malls/Gpc . Carbohydr. Polym. , 27 ( 2 ) : 145 – 154 .
- Rochas , C. and Rinaudo , M. 1980 . Activity Coefficients of Counterions and Conformation in Kappa-Carrageenan Systems Biopolymers . 19 ( 9 ) : 1675 – 1687 .
- Vega , C. , Dalgleish , D.G. and Goff , H.D. 2005 . Effect of [Kappa]-Carrageenan Addition to Dairy Emulsions Containing Sodium Caseinate and Locust Bean Gum . Food Hydrocolloids , 19 ( 2 ) : 187 – 195 .
- Hermansson , A.M. , Eriksson , E. and Jordansson , E. 1991 . Effects of Potassium, Sodium and Calcium on the Microstructure and Rheological Behaviour of Kappa-Carrageenan Gels . 16 : 297 – 320 .
- Haighton , A. J. 1959 . The Measurement of the Hardness of Margarine and Fats with Cone Penetrometers . J. Am. Oil Chem. Soc. , 36 : 345
- Wright , A.J. , Scanlon , M.G. , Hartel , R.W. and Marangoni , A.G. 2001 . Rheological Properties of Milkfat and Butter . J. Food Sci. , 66 ( 8 ) : 1056 – 1071 .
- Staniewski , B. , Szpendowski , J. , Panfil-Kuncewicz , H. , Malkus , J. and Bohdziewicz , K. 2006 . The Application of an Ap4/2 Conical Penetrometer for the Evaluation of Spreadability of Selected Table Fats . Milchwissenschaft , 61 ( 3 ) : 292 – 296 .
- Kim , B.H. , Shewfelt , R.L. , Lee , H. and Akoh , C.C. 2005 . Sensory Evaluation of Butterfat-Vegetable Oil Blend Spread Prepared with Structured Lipid Containing Canola Oil and Caprylic Acid . J. Food Sci. , 70 ( 7 ) : s406 – s412 .
- Strohmaier , W. , Klostermeyer , H. , Deuritz , P. and Windhab , E. 1992 . Comparison of Different Methods to Determine the Spreadability and Firmness of Processed Cheese . Z. Lebensm. -Unter. Forsch. , 194 : 531 – 535 .
- Van Vliet , T. 1999 . “ Rheological Classification of Foods and Instrumental Techniques for Their Study ” . In Food Texture: Measurement and Perception , Edited by: Rosenthal , A.J . 65 – 96 . Gaithersburg, MD : Aspen Publishers, Inc .
- Association of Official Analytical Chemists . 1997;9 . “ Method 965.33 ” . In Official Methods of Analysis: Association of Analytical Chemists , Gaithersburg, MD : AOAC .
- Keogh , M.K. , Quigley , T. , Connolly , J.F. and Phelan , J.A. 1988 . Anhydrous Milk Fat: 4.Low-Fat Spreads . Irish Journal of Food Science and Technology , 12 : 53 – 75 .
- Lundberg , W.O. 1962 . “ Mechanisms of Lipid Oxidation ” . In Lipids and Their Oxidation , Edited by: Schultz , H.W. 31 – 51 . Westport, CT : AVI Publishing Company .
- Chow , C.K. and Gupta , M.K. 1994 . “ Treatment, Oxidation and Health Aspects of Fats and Oils ” . In Technological Advances in Improved and Alternative Sources of Lipids , Edited by: Kamel , B.S. and Kakuda , Y. 329 – 359 . Glasgow : Blackie Academic and Professional .
- Gutcho , M. 1979 . “ Improving Stability of Fats and Oils ” . In Edible Oils and Fats Recent Developments , 53 – 76 . Park Ridge, NJ : Noyes Data Corporation .
- Precht , D. 1988 . “ Fat Crystal Structure in Cream and Butter ” . In Crystallization and Polymorphism of Fats and Fatty Acids , Edited by: Garti , N. and Sato , N. K. 305 – 361 . New York : Marcel Dekker .
- Moran , D.J.P. 1990 . The Development of Yellow Spreads . Dairy Industries International , 5 : 41 – 43 .