Abstract
In this review, briefly the role of structure of food dispersions in their rheological properties and how it affects rheological measurement are discussed. Besides processed food dispersions, starch dispersions during and after gelatinization are considered. The discussion points out to the benefit of studying rheological properties of dispersions with known composition and structural characteristics. Lastly, we discuss frequency shift factor for superposing the temperature and shear dependent viscosity data during gelatinization of starch dispersions to obtain master viscosity curves.
INTRODUCTION
There are many commercially important processed-food dispersions of solid particles in liquid media, such as: ketchup, mustard, tomato puree, and baby foods. In addition, dispersions of starch granules before and after gelatinization are also important. In this brief review, the role of structure of those dispersions and factors affecting measured rheological properties are considered. We assume that the reader is familiar with basic terms of rheology and geometries used in measurement of rheological properties. We urge the interested researcher to consult the original works that formed the basis of this review. The viscosity (or apparent viscosity) of a dispersion can be expressed in terms of the relative viscosity, η r :
The η r of a dispersion is strongly dependent on the volume fraction, φ, of the solids in it. One commonly used equation that takes in to account the effect of volume fraction on the η r of concentrated dispersions is[Citation1]:
STRUCTURAL FACTORS AFFECTING MEASUREMENT OF RHEOLOGICAL PROPERTIES
Rheological properties of a food dispersion are strongly influenced by its physical structure. Thus, every attempt should be made to minimize unintended changes to a dispersion's structure. One common disruption of a dispersion's structure occurs during set up of a rheological test using a well-defined measuring geometry (e.g., concentric cylinder, cone-plate, parallel disk). Often, in recognition of the disruption of structure during loading, a reasonable time is allowed for the sample structure to recover before rheological measurements are conducted. However, the original structure of the sample may never be recovered due to irreversible changes. In addition, errors are introduced in the calculated values of shear rates due to slip at the solid walls of the measuring geometry. This is because in the derivation of the expressions for shear rates for all test geometries, a no slip boundary condition at all viscometer walls is used.[Citation2] However, with many dispersions (e.g., mustard paste, ketchup, tomato concentrates, vegetable purees), a thin layer of clear, low-viscosity, fluid forms at a solid boundary. While procedures exist to correct for the slip velocity, they are time-consuming.[Citation3,Citation4] In addition, the measuring geometry's gap should be of the order of about 10 times that of a particle diameter. Thus, if a dispersion contains relatively large solid particles, both the narrow-gap concentric cylinder (CC) and cone-plate geometries would not be suitable.
VANE-IN-CUP GEOMETRY FOR FLOW PROPERTIES
Yield Stress Using a Vane
The vane-in-cup (VIC) geometry has been used for about 20 years to obtain reliable values of yield stress of foods[Citation2] using the method introduced by Dzuy and Boger.[Citation5] It will be shown later that the VIC geometry can also be used to measure flow properties because the vane acts as an effective cylinder. One important requirement in both viscosity and YS tests is that the vane should have at least four blades. A typical vane with six blades is shown in .[Citation6] With minimum precautions, insertion of a vane into a test sample in a container can be done with minimum disruption of the sample. In addition, one need not be concerned with the effect of slip. Typical values of the ratio of vane diameter to cup diameter for YS measurements are: 1.81–2.0; i.e., there should be sufficient sample between the vane blade tip and the container wall to allow unimpeded breakage of the food's structural bonds near the edges of the blades, and at the top and bottom of the vane.
Figure 1 Schematic diagram of a vane with six blades that can be used for measurement of yield stress and flow behavior of food dispersions.
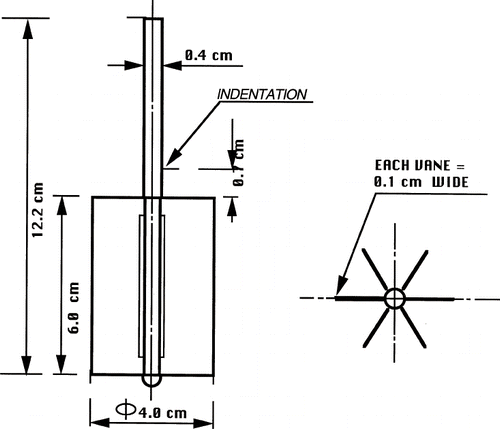
In a vane YS test, the vane is rotated at a low speed, and the torque is recorded as a function of time (). Comparison of controlled-stress and controlled-strain tests showed that the latter is preferable because of the maximum torque can be determined unambiguously.[Citation7] From the value of the maximum torque, Tm , the vane YS, σ0v , is calculated using the equation:
Figure 2 Torque vs. time curve when a vane is rotated at a low rpm. Yield stress can be calculated from the maximum value of the torque.
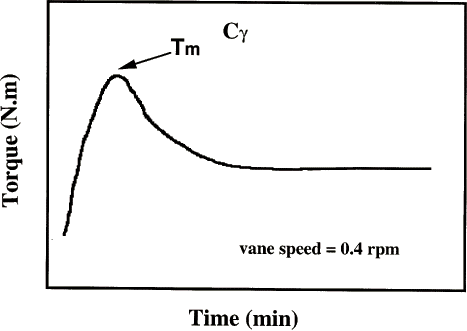
In EquationEq. (3), Dv is the diameter of the vane and H is its height. It was suggested that data obtained from creep experiments using a vane may be also used to determine yield stress.[Citation8] Recognizing that the YS of a dispersion with undisrupted structure (static YS) differs from that with a disrupted structure (dynamic YS), additional useful information on the structure of a dispersion can be obtained.[Citation9]
Determination of Flow Behavior Using a Vane
From numerical simulation of flow of power law fluids in a narrow-gap VIC geometry (ri = 8.5 mm, ro = 9.5 mm), Barnes and Carnali[Citation10] suggested that for shear-thinning fluids with the flow behavior index <0.5, the fluid within the blades is trapped and turns with the rotating vane. Thus, for a given rotational speed of the vane, the shear stress at the cup wall is equal to that in a conventional CC viscometer geometry and identical flow curves would be obtained. In contrast, for a flow test, the VIC geometry should imitate a CC geometry. The shear stress and the Newtonian shear rate are determined as with a CC geometry[Citation2]:
STRUCTURE OF STARCH DISPERSIONS
Starch is an abundantly available source of food and its two principal components, amylose and amylopectin, exist in the form of granules of about 15 μm in size. The swelling characteristic of starch granules depends primarily on the type and source of the starch. Due to differences mainly in their crystallite patterns, tuber starches are prone to disruption to a greater extent compared to cereal starches.[Citation11,Citation12] Yet, in high amylopectin cereal starches like waxy maize starch with ca. 99% amylopectin, granules tend to hydrate with ease, swell rapidly, and rupture to a great extent.[Citation13] As a result, the starch paste loses viscosity relatively easily producing weak bodied, stringy and cohesive pastes. Cross-linking treatment is intended to add chemical bonds at random locations in a granule. Cross-linked waxy maize starch pastes are more viscous and heavy bodied, and are less likely to breakdown with extended cooking times, high acidic conditions or severe agitation.[Citation14]
Some of the measurement problems with starch dispersions (SDs) are in part due to the tendency of starch granules to settle during gelatinization, a first-order phase transition,[Citation2] that occurs over a temperature range of about 10°C. For example, one can use Stoke's law to calculate the settling velocity of a single starch granule, assuming for starch ρ s = 1.63 g cm−3, granule radius = 10 μm, and for water: η= 0.0007 mPa s in the 30–60 °C range, ρ w = 1.00 g cm−3. Further, it can be shown that a single granule can settle over 6 cm in about 5 minutes.
During gelatinization of native starches, because the granules swell continuously and eventually begin to rupture, so that their volume fraction also changes continuously; consequently, the apparent viscosity also changes in a corresponding manner. The mass fraction of starch granules in a dispersion can be calculated from the starch concentration, c, and the granule swelling factor, Q, as cQ.; the factor, Q, should be determined for each SD as described elsewhere.[Citation15] Values of the maximum volume fraction, φ max , of starch granules that have been used in viscosity equations [e.g., EquationEq. 2] have been about 0.74.
ISOTHERMAL HEATING OF STARCH DISPERSIONS
In order to create gelatinized dispersions, raw SDs should be heated while keeping the starch granules suspended. However, it should be noted that vigorous agitation damages the starch granules. Two experimental approaches to studying rheological behavior of SDs have been used: (1) isothermal heating under gentle agitation in a container using either a magnetic bar[Citation15] or a rotating flask,[Citation16] cooling the gelatinized dispersion, and studying its rheological behavior after transferring it to a viscometer/rheometer, and (2) concurrent rheological measurement during non-isothermal (continuous) heating either in a small-gap (500 μm), parallel disc, geometry in which convection currents induce agitation, or a CC geometry together with careful preparation of the dispersion to minimize starch granules settling in the gap of that geometry. We adapted the technique of Keetles and van Vliet[Citation17] for obtaining apparent viscosity data at high temperatures (>90 °C).[Citation18] It should be noted that isothermal heating experiments are valuable in evaluating the viscosity behavior of a SD (e.g., the effect of the type and concentration of starch).
NON-ISOTHERMAL HEATING OF STARCH DISPERSIONS
While non-isothermal or continuous heating experiments are relatively time-consuming, they allow for obtaining simultaneously rheological data as a function of temperature and shear rate. Such thermo rheological data are useful in experimental and simulation studies on heat transfer to foods containing starch.[Citation19–22]
Small-Amplitude Oscillatory Test
A parallel plate geometry, with a wide gap (∼500 μm), can be used to obtain rheological data during heating of SDs. However, during a flow test, some of the sample in the gap may be lost due to the prevalent centrifugal force in continuous rotation. Therefore, it would be best to use small-amplitude oscillatory tests. In general, in our studies[Citation23,Citation24], a small volume of the SD (∼0.64 mL) with virtually no air bubbles was transferred to the bottom plate of a rheometer (AR1000, TA Instruments, New Castle, DE) using a micropipette. Water evaporation from the sample that occurs at high temperatures was minimized by placing paraffin oil at the edge of the steel plate soon after gelatinization began. Each sample was heated from 55°C to 97°C at a specific heating rate (e.g., 2.1°C min−1), and the effect of oscillatory shear rate, ω, on the complex viscosity, η*, of each SD was examined.
The η* versus T profiles at the different frequencies were similar in shape as illustrated for an 8% cross-linked waxy maize SD in . Further, by employing the scaling factor: (w/w
r
)
b
, the reduced complex viscosity; , was calculated using the equation[Citation23]:
Figure 3 Complex viscosity vs. temperature curves obtained at different oscillatory frequencies on an 8% cross-linked waxy maize starch dispersion.
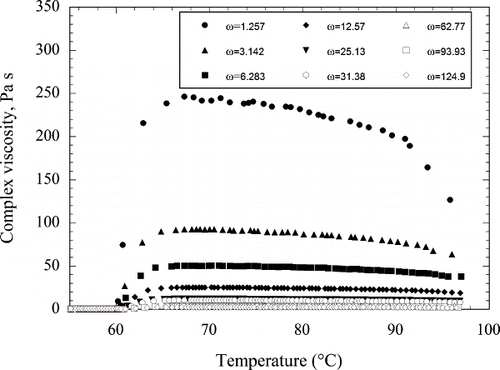
Figure 4 Master curves of reduced complex viscosity vs. temperature of the 8% starch dispersions: corn, cross-linked waxy maize, tapioca, and waxy rice. The reference shear rate is 6.28 rad s−1.
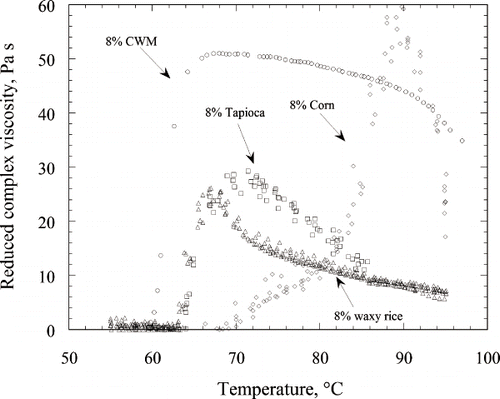
The data in provide insights in to the effects of starch composition and granule on the temperature-dependent (thermo) rheological behavior of SDs. The non-isothermal technique using the parallel plate geometry is also well suited for SDs of high starch concentrations because they tend to stay in place; the master curve obtained on a 45.5% rice SD at a heating rate of 4.0 °C min−1 is shown in .[Citation25] Clearly, in and , the effect of temperature on the viscosity of the SDs over the entire temperature range cannot be described by the well-known Arrhenius relationship. Further, the superposition of data obtained at different oscillatory frequencies results in thermo rheological data useful in experimental and simulation studies on heat transfer to the SDs. However, the Arrhenius relationship would be applicable for the viscosity of SDs that have been completely gelatinized.
Rheological Behavior of Starch Dispersions at High Temperature
Compared to the cone-plate and the parallel plate geometries, one advantage of the CC system is that it holds the SD in a well-confined space during application of shear making it amenable to continuous shear experiments and minimal loss of water by evaporation. Therefore, it was used together with a pressurized system to obtain apparent viscosity data at temperatures from ambient to above boiling point of water.[Citation18] In this system, the drive motor, torque unit, CC unit (MVI, ri = 20 mm, ro = 21 mm, h = 60 mm), and temperature control vessel of a Haake RV2 viscometer (Haake Inc., Secaucus, NJ) were placed in a pressure chamber. The rheological measurements were performed under a pressure of about 0.23 MPa (2.3 Atmospheres). To minimize settling of starch granules in the gap of the CC geometry, the sample preparation procedure of Keetles and van Vliet[Citation17] with minor modification was used: (1) 250 mL of 5% cross-linked waxy maize (CWM) starch was heated to 64 °C and cooled to 25 °C to which ungelatinized 250 mL of 5% CWM starch was added; and (2) the entire dispersion, made up of mostly ungelatinized granules suspended in a medium of granules heated to 64 °C, was then poured in to the cup (MVI) of the CC geometry. Apparent viscosity data as a function of temperature were recorded at constant RPM settings (); the duration of each experiment was limited to about 20 mins to minimize loss of water from the SD sample. Because of continuous shearing, the viscosity-temperature profiles in are relatively flat. Further, one can see shear-thickening effect at several shear rates that has been reported during continuous shearing of gelatinized SDs.[Citation15]
CONCLUSION
Use of viscometric flow geometries, such as the concentric cylinder and the cone-plate, may introduce errors in measured values due to structure disruption and wall slip. However, the vane-in-cup geometry is well suited for the determination of both yield stress and flow rheological data of food dispersions. If proper precautions to avoid starch granule settling are taken, rheological data can be obtained on starch dispersions undergoing gelatinization. Because the complex viscosities versus temperature data at different shear rates are similar, master curves of reduced viscosity can be derived.
REFERENCES
- Metzner , A.B. 1985 . Rheology of suspensions in polymeric liquids . J. Rheol. , 29 ( 6 ) : 739 – 775 .
- Rao , M.A. 2007 . Rheology of Fluid and Semisolid Foods: Principles and Applications , 2nd , 481 New York : Springer .
- Qiu , C-G. and Rao , M.A. 1989 . Effect of dispersed phase on the slip coefficient of apple sauce in a concentric cylinder viscometer . J. Texture Studies , 20 : 57 – 70 .
- Grikshtas , R. and Rao , M.A. 1993 . Determination of slip velocities in a concentric cylinder viscometer with Mooney and Kiljanski methods . J. Texture Studies , 24 : 173 – 184 .
- Dzuy , N.Q. and Boger , D.V. 1985 . Direct yield stress measurement with the vane method . J. Rheol. , 29 : 335 – 347 .
- Qiu , C-G. and Rao , M.A. 1988 . Role of pulp content and particle size in yield stress of apple sauce . J. Food Sci. , 53 : 1165 – 1170 .
- Yoo , B. , Rao , M.A. and Steffe , J.F. 1995 . Yield stress of food suspensions with the vane method at controlled shear rate and shear stress . J. Texture Studies , 26 : 1 – 10 .
- Baravian , C. , LaLante , A. and Parker , A. Vane rheometry with a large, finite gap . Applied Rheol. , 2002 ( 12 ) 81 – 87 .
- Genovese , D.B. and Rao , M.A. 2005 . Components of vane yield stress of structured food dispersions . J Food Sci. , 70 ( 8 ) : E498 – 504 .
- Barnes , H.A. and Carnali , J.O. 1990 . The vane-in-cup as a novel rheometer geometry for shear thinning and thixotropic materials . J. Rheol. , 34 : 841 – 865 .
- Lineback , D.R. 1984 . The starch granule: organization and properties . Bakers Dig. , 58 ( 2 ) : 18 – 21 . 16
- Swinkels , J.J.M. 1985 . Composition and properties of commercial native starches . Starch/Stärke , 37 ( 1 ) : 1 – 5 .
- Lineback , D.R. 1986 . Current concepts of starch structure and its impact on properties . J. Japan. Soc. Starch Sci. , 33 ( 1 ) : 80 – 88 .
- Langan , R.E. 1986 . “ Food Industry ” . In Modified Starches: Properties and Uses , Edited by: Wurzburg , O. B. 199 – 212 . Florida , Boca Raton : CRC Press, Inc .
- Okechukwu , P.E. and Rao , M.A. 1995 . Influence of granule size on viscosity of corn starch suspension . J. Texture Studies , 26 : 501 – 516 .
- Evans , I.D. and Haisman , D.R. 1979 . Rheology of gelatinized starch suspensions . J. Texture Studies , 10 : 347 – 370 .
- Keetels , C.J.A.M. and Van Vliet , T. 1993 . “ Mechanical properties of concentrated starch system during heating, cooling and storage ” . In Food colloids and polymers: stability and mechanical properties , Edited by: Dickinson , E. and Walstra , P. 266 – 271 . Cambridge : The Royal Society of Chemistry .
- Rao , M.A. , Cooley , H.J. and Liao , H.-J. 1999 . High temperature rheology of tomato puree and starch dispersion with a direct-drive viscometer . J. Food Process Eng. , 22 : 29 – 40 .
- Yang , W.H. and Rao , M.A. 1998 . Transient natural convection heat transfer to starch dispersion in a cylindrical container: numerical solution and experiment . J. Food Eng. , 36 : 395 – 415 .
- Liao , H.-J. , Rao , M.A. and Datta , A.K. 2000 . Role of thermorheological behavior in simulation of continuous sterilization of a starch dispersion . IChemE Transactions Part C - Food and Bioproducts Processing , 78 ( C1 ) : 48 – 56 .
- Tattiyakul , J. , Rao , M.A. and Datta , A.K. 2002 . Heat transfer to three canned fluids of different thermo-rheological behavior under intermittent agitation . IChemE Transactions Part C - Food and Bioproducts Processing , 80 : 20 – 27 .
- Kelder , J.D.H. , Ptasinski , K.J. and Kerkhof , P.J.A.M. 2004 . Starch gelatinization in coiled heaters . Biotechnol. Progress , 20 : 921 – 929 .
- Yang , W.H. and Rao , M.A. 1998 . Complex viscosity-temperature master curve of cornstarch dispersion during gelatinization . J. Food Process Eng. , 21 : 191 – 207 .
- Tattiyakul , J. and Rao , M.A. 2000 . Rheological behavior of cross-linked waxy maize starch dispersions during and after heating . Carbohyd. Polym. , 43 ( 3 ) : 215 – 222 .
- Liao , H.-J. , Tattiyakul , J. and Rao , M.A. 1999 . Superposition of complex viscosity curves during gelatinization of starch dispersion and dough . J. Food Process Eng. , 22 : 215 – 234 .