Abstract
The effect of calcium lactate on the cellular structure and mechanical properties of osmodehydrated melon pieces with sucrose solutions was studied. Samples were treated with sucrose solutions (40 or 60°Brix) containing calcium lactate (0 to 20 g kg−1). The salt had a remarkable effect on the stress at failure and the cellular structure of fruits, resulting in structural preservation when used at concentrations of up to 10 g kg−1. Salt concentrations above 15 g kg−1 as well as the treatment performed with sucrose solution alone promoted cytoplasm plasmolysis and cell wall damages. Fruits processed with sucrose solutions at 60°Brix with or without calcium lactate showed good sensory acceptance, while fruits treated with sucrose solutions at 40°Brix presented statistically significant lower scores at calcium concentrations above 15 g kg−1.
INTRODUCTION
The consumption of lightly processed food has promoted the development of new technologies based on mild processing, aiming to improve its resistance to degradation and maintain the sensory and nutritional characteristics of the fresh fruit. In this context, osmotic dehydration has been widely used to obtain minimally processed or intermediate moisture products, extending their shelf life. Osmodehydrated products are not microbiologically stable, and therefore, subsequent treatment, such as air-drying,[Citation1,Citation2] atmospheres and refrigerated storage, amongst other processing alternatives, is necessary for long-term preservation.[Citation3]
Osmotic dehydration is a process based on the concentration of water rich foodstuffs, and it consists of immersing food pieces in concentrated aqueous solutions, mainly sugar and salt solutions. Throughout the process, two types of counter current mass transfer occur: water outflow from the product to the solution and solute incorporation from the solution to the food. Leakage of the product's own solute is also observed, which is quantitatively negligible with respect to the other two fluxes, but important to the final product composition. Thus, osmotic dehydration allows for changes in the food system matrix, by adding desired amounts of active components, such as texture enhancers, nutrients or additives.Citation[4]
Texture is one of the most important quality attributes of osmodehydrated products. Over the last few years, the importance of texture as a quality attribute has considerably increased due to changes in consumer eating habits.Citation[5] The determination of textural properties is very important to understand the food structure and how it is affected by the osmotic process.Citation[6] Water loss and sugar uptake occurring during the osmotic dehydration promote changes in optical and mechanical properties, altering the product appearance and texture characteristics.Citation[7] The main alterations induced by osmotic treatment which affect the mechanical behavior of plant tissue are: loss of cell turgor pressure, splitting and degradation of the middle lamella, alterations in cell wall resistance, deformation and/or cell wall failure, lysis of membranes (plasmalema and tonoplast), cellular collapse, plasmolysis and tissue shrinkage. On the other hand, these changes in the mechanical properties of plant tissue take place at different rates, depending on the process conditions and product characteristics.Citation8–10
Calcium salts have been employed before or after harvest in order to delay ripening and senescence and to prevent physiological disorders, extending the shelf life of several fruits and vegetables, and recently the use of calcium salts aimed at tissue structural preservation has also been studied. The action of calcium salts on fruit texture and structural characteristics can be explained based on the cross-links or bridges formed between calcium ions and free carboxyl groups on the pectin chains, resulting in cell wall strengthening. Calcium forms complexes with cell wall and middle lamella pectin, improving structural integrity and promoting greater tissue firmness. Furthermore, calcium salts can be used as a cross-linking agents in order to produce stronger pectin or alginate films.Citation11–12 Two main techniques for calcium application to fresh-like minimally processed fruits and vegetables have been reported: calcium salt dips in combination with mild heat treatments and impregnation methods with the addition of calcium salts to the osmotic solution under vacuum or atmospheric conditions.Citation[12] In addition, treatments with calcium salts have a significant effect on mass transfer rates during the osmotic dehydration process.Citation[13]
Luna-Guzman & BarretCitation[14] compared the effect of calcium chloride and calcium lactate dips on fresh-cut cantaloupe texture, respiration, and sensory evaluation. According to the authors, both salts were effective in maintaining melon firmness, but calcium chloride imparted an undesirable bitterness to the fruit pieces. Therefore, calcium lactate has been proposed as a good alternative to calcium chloride because it avoids the bitterness and off-flavors.Citation[10] This salt has been used as a firming agent in concentrations varying from 5 g kg−1 to 20 g kg−1 for fruits like peaches,Citation[15] mangoes,Citation[16] apples,Citation[17] and guavas.Citation[10] The objective of this work was to study the effect of calcium lactate on the mechanical properties (stress and strain at failure, relaxation time, and residual stress), cellular structure (light microscopy) and sensory characteristics (acceptance tests) of melon cubes osmodehydrated with sucrose solutions, aiming at fruits structure maintenance. The influence of calcium lactate on mass transfer rates during the osmotic process was also evaluated.
MATERIAL AND METHODS
Material and Sample Preparation
Ripe melons (Cucumis melo L.) of the Cucumis melo inodorus variety, Gold Mine cultivar (), purchased at a local market (CEASA – Campinas – Brazil), were selected based on their ripeness level (9–10°Brix), shape and size (17.1 ± 0.7 cm length, 13.2 ± 0.6 cm diameter and 2200.0 ± 158.0 g mass). Chemical analyses were performed according to AOAC.Citation[18] The soluble solids content was determined as °Brix (g of solids/100 g of product) using a bench refractometer (Zeiss, Jena, Germany). All measurements were made in triplicate.
Table 1 Fresh melon composition (Cucumis melo inodorus variety. Gold Mine cultivar)
The fruits were washed with tap water and immersed in a 533 mg/L solution of peracetic acid in a mixture of hydrogen peroxide with glacial acetic acid (TSUNAMI 100®) containing 80 mg/L of peracetic acid (Ecolab Química Ltda., São Paulo, Brazil) for 3 minutes. The melons were then manually peeled, the seeds removed, and the pulp cut into 20 mm cubes and weighed.
Osmotic Dehydration
Sucrose (Copersucar União, Piracicaba, Brazil) and calcium lactate (LabSynth São Paulo, Brazil) were used as the dehydrating agents. The osmotic solutions were prepared with distilled water and sucrose (40 and 60°Brix) with or without the addition of 5, 10, 15, and 20 g kg−1 of calcium lactate. The melon cubes were immersed in the osmotic solutions using a product:solution mass ratio of 1:10. Fruit and solution (6 pieces per flask) were placed in a thermostatic shaker (Model TE 420, Tecnal, Piracicaba, Brazil) under controlled conditions of temperature (30°C) and agitation (120 rpm) for to two hours, process conditions previously optimized. After the osmotic process, the osmodehydrated melon pieces were drained, rinsed with distilled water and placed on absorbent paper to remove excess solution before being weighed.
The samples were named FR (without treatment), S40 (osmotically dehydrated in a 40°Brix sucrose solution), S60 (osmotically dehydrated in a 60°Brix sucrose solution), S40CA5 to S40CA20 (osmotically dehydrated in a 40°Brix sucrose solution with the addition of 5 – 20 g kg−1 of calcium lactate), and S60CA5 to S60CA20 (osmotically dehydrated in a 60°Brix sucrose solution with the addition of 5 – 20 g kg−1 of calcium lactate). The FR and S samples were used as controls in order to evaluate the effects of osmotic dehydration and calcium lactate addition, respectively, on the melon properties.
Weight Reduction (WR), Water Loss (WL), and Solids Gain (SG) Determinations
Weight reduction (EquationEq. 1) was evaluated by weighing the samples before and after the osmotic treatment, while water loss (EquationEq. 2) and solids gain (EquationEq. 3) were calculated from the sample moisture content, determined according to AOACCitation[18] method 934.06. Three melon cubes from each treatment, taken from different fruits, were used for these measurements and the mean values were reported (%).
where: w0 is the initial sample weight (g) and wf is the final sample weight (g);
where: ww0 is the initial weight of water in the sample (g) and wwf is the final weight of water in the sample (g);
where: ws0 is the initial solids weight of the sample (g) and wsf is the final solids weight of the sample (g).
Calcium Content Determination
The calcium concentrations of the fresh and osmodehydrated samples were determined using an inductively coupled plasma atomic emission spectrometer (ICP 2000, Baird, MA, USA) according to AOACCitation[18] method 991.25. The calcium determination was carried out in triplicate and the mean values reported.
Mechanical Properties
The mechanical properties were analyzed by uniaxial compression tests using a Universal Testing Machine (TA.XT2i Texture Analyzer, Stable Micro Systems, Surrey, England). The measurements were made using a 60 mm diameter lubricated acrylic plate, and the other experimental conditions are described below. The stress at failure, relaxation time and residual stress measurements were determined in quintuplicate.
Stress at Failure
The stress at failure tests was performed at a compression speed of 1 mm/s and 80% sample deformation. The force and height values obtained from this test were converted to Hencky stress (σH) and strain (ϵH), according to EquationEqs. (4) and Equation(5), and the stress at failure was determined from the peak of the stress-strain curve.Citation[19]
where F(t), A(t) and H(t) represent the force (N), product area (m2) and height (m) at time t, respectively, and H0 is the initial product height (m).
Relaxation Time and Residual Stress
These tests were carried out with samples kept under a strain of 5% for 600 s with initial speed of 7 mm/s. The resulting stress-relaxation curves (EquationEq. 6) were linearised according to Peleg.Citation[20] The relaxation time (τ) and residual stress (Sr) were determined from the constants k1 (viscous element) and k2 (elastic element) for the moment at which σt = 0.75σo,Citation[21] according to EquationEqs. (7) and Equation(8).
Light Microscopy
Samples (∼5mm × 3mm × 3mm) from the fleshy tissue of fresh and osmotically dehydrated melons were fixed in 40 g kg−1 glutaraldehyde in phosphate buffer (pH 7.0) with 40 g kg−1 of added sucrose and dehydrated in a graded ethanol series.Citation[22] The dehydrated samples were embedded in hydroxyethyl methacrylate historesin (Leica Microsystems, Jung, Heidelberg, Germany) and sectioned using a rotary microtome (820 Spencer Microtome, American Optical Corporation, New York, USA). Sample sections measuring 10 μm were stained with toluidine blue (0.5 g kg−1) in acetate buffer (pH 4.7)Citation[23] and examined using an Olympus BX 51 light microscope (Olympus Optical Co., Tokyo, Japan). Two samples of the same fruit, from each treatment, were used for the microscopic evaluation.
Sensory Preference
Sensory acceptance tests were carried out in a standardized test room, one day after the osmotic process. The samples were presented in completely balanced blocks, in a monadic way, using white saucers labelled with random three-digit number codes. Appearance, aroma, flavor, texture and overall impression of the samples were evaluated by 35 melon consumers, representative of the target public, using a 9 cm unstructured hedonic linear scale anchored with ‘I dislike very much’ on the left side and ‘I like very much’ on the right side. An average score of 4.5 was considered to be the limit of acceptability.Citation[24]
Statistical Analysis
All the results were statistically analysed by the analysis of variance (ANOVA) using the software STATISTICA® 5.0 (StatSoft, Inc, Tulsa, USA), in order to determine the significant differences between the sample means. The means analysis was performed using the Tukey´s test procedure at p ≤ 0.05.
RESULTS AND DISCUSSION
Calcium Content, Weight Reduction, Water Loss, and Solids Gain
illustrates the calcium content of the fresh fruit and the osmotically dehydrated samples after two hours. The calcium content of the fresh melon was 0.894 ± 0.008 g kg−1 dry matter (mean value ± standard deviation). The addition of calcium lactate to the osmotic solution resulted in a significant increase (p ≤ 0.05) in the calcium content of the treated fruits, reaching values of 3.831 ± 0.030 g kg−1 dry matter. Fruits osmotically dehydrated with sucrose solutions at 40°Brix presented a final calcium content between 1.245 ± 0.006 and 3.831 ± 0.030 g kg−1 dry matter, while the calcium content values for the fruits processed at 60°Brix varied in the range from 1.142 ± 0.008 to 2.540 ± 0.019 g kg−1 dry matter.
Figure 1 The calcium content of melon subjected to different treatments. FR: without osmotic treatment; S40: osmotically dehydrated in a 40°Brix sucrose solution; S40CA5 to S40CA20: osmotically dehydrated in a 40°Brix sucrose solution with the addition of 5–20 g kg−1 of calcium lactate; S60: osmotically dehydrated in a 60°Brix sucrose solution; S60CA5 to S60CA20: osmotically dehydrated in a 60°Brix sucrose solution with the addition of 5 – 20 g kg−1 of calcium lactate. Mean separation by the Tukey test. Different letters indicate statistically significant differences at p ≤ 0.05.
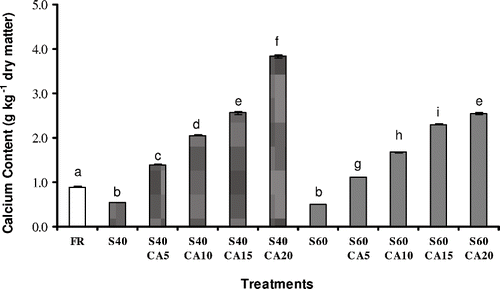
Mastrangelo et al.Citation[9] working with the osmotic dehydration of melon pieces using glucose (50°Brix) and calcium lactate (1 to 10 g kg−1) as the osmotic agents, observed calcium impregnation of approximately 1 g kg−1 for the fruits treated under atmospheric or vacuum conditions. According to Lewicki et al.,Citation[13] the osmotic process for tomatoes pre-treated in 20 g kg−1 of CaCl2 for 24 hours, preserved more than 50% of the fruit calcium content. Moreover, in the osmotic treatment with the addition of calcium (20 g kg−1 CaCl2), a considerable increase in tomato calcium content was also verified, showing values around seven-fold higher than the raw tomato.
As can be seen in , an increase in salt concentration in the osmotic solution resulted in greater calcium ion incorporation. In addition, the combination of a lower sucrose concentration (40°Brix) and the addition of calcium lactate promoted an increase in salt uptake, when compared to the sucrose treatments at 60°Brix. The samples treated with sucrose solutions at 40°Brix and 20 g kg−1 of calcium lactate presented a final salt content approximately five-fold higher than that of the fresh fruit. Torres et al.Citation[16] working with the osmotic dehydration of mango pieces using sucrose (45 or 65°Brix) and calcium lactate (0 – 20 g kg−1) as osmotic agents, with the application or otherwise of a vacuum pulse at the beginning of the process, observed increasing calcium impregnation of the fruits as the salt concentration of the solution increased. The calcium gain was greater in the treatments at 45°Brix, as a consequence of the lower solution viscosity observed in this condition. According to the authors, the substantial increase in solution viscosity at 65°Brix acts as a barrier to mass transfer from the solution to the fruit, due to the formation of a superficial solute layer around the fruit.
The osmotic process using sucrose solutions at 60°Brix caused greater water loss (≅28%) and weight loss values (≅19%). According to Moreno-Castillo et al.,Citation[25] higher sugar concentration in the solution increases the osmotic pressure gradient at the product/solution interface, enhancing the mass transfer rates. In the osmotic dehydration of melon cubes using sucrose, glucose or manitol (30–70ºBrix) and food-grade marine salt (0–15%) as dehydrating agents, a significant increase in water loss rates was verified for the osmodehydrated samples with higher salt and sugar concentrations.Citation[26] On the other hand, the solids gain rates decreased as the sugar concentration in the osmotic solution increased for the treatments with or without calcium lactate addition (). A similar behaviour was also noticed in some studies with mangoesCitation[16] and kiwis.Citation[27] As previously discussed, this fact can be associated with the increase in solution viscosity in the experiments at 60°Brix, hindering sugar uptake.
Figure 2 Weight reduction (WR), water loss (WL) and solids gain (SG) of melon pieces subjected to different treatments. (A) osmotic dehydration in a 40°Brix sucrose solution with or without the addition of calcium lactate; (B) osmotic dehydration in a 60°Brix sucrose solution with or without the addition of calcium lactate. Mean separation by the Tukey test. Different letters indicate statistically significant differences at p ≤ 0.05.
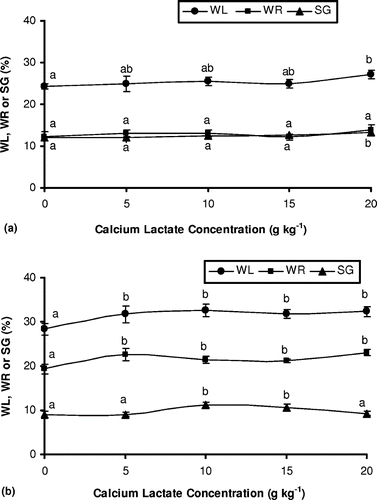
The addition of calcium salts to the osmotic solution provided a statistically significant increase in the sample water loss rates, for the fruits treated with sucrose solution at 60°Brix (p ≤ 0.05). However, as the calcium concentration increased from 5 to 20 g kg−1 the sample water content remained practically constant, not showing significant differences. For the treatments at 40°Brix, a considerable augmentation in water loss rates was only verified for the processed fruits with 20 g kg−1 of calcium lactate. With respect to weight reduction, the results obtained presented a similar behavior to that of the water loss values. Higher weight reduction rates were verified with the addition of calcium salts to the solution, but this weight loss increase was statistically significant for the treatments at 60°Brix.
Samples osmodehydrated with sucrose at 60°Brix showed an increasing trend to gain solids with the addition of calcium lactate (), reaching the maximum solids incorporation of about 11% in the osmotic treatment with 10 g kg−1 of calcium lactate. For calcium salt concentrations above 15 g kg−1, a significant reduction in solids gain was shown. According to Sereno et al.,Citation[28] the use of calcium salts in the osmotic solution can hinder solids gains, a fact attributed to its influence on the fruit cellular structure, causing some alterations in cell membrane permeability. In the present study, a small increase in fruit solids content was observed in the samples osmotically dehydrated with sucrose solutions at 40°Brix as a result of the addition of calcium salts to the osmotic solution. These differences were only significant for the highest calcium concentration used (20 g kg−1), with a solids uptake of about 13% ().
Lewicki et al.Citation[13] also reported an increase in water and sugar diffusion in tomatoes pre-treated with calcium chloride and subjected to osmotic and drying processes. The formation of an open structure due to the calcium bridges formed between the pectin molecules and calcium ions favored mass transport, resulting in greater water loss and solids uptake in the final product. In similar studies, Pereira et al.Citation[10] and Mastrantonio et al.Citation[29] verified the influence of calcium salts in the osmotic dehydration of guavas, using sucrose or maltose solutions with the addition of calcium lactate. According to the authors, the combined effect of the sugar and calcium salts in the osmotic solution promoted a greater dehydrating effect, which can be related to the increasing osmotic pressure gradient observed in more concentrated solutions. On the other hand, Barrera et al.Citation[30] noticed that the ability of calcium to interact with the vegetal cellular matrix by forming bonds between the pectin and other cell wall components hindered the mass transfer fluxes, due to the formation of a more resistant cell wall. In addition, Torres et al. Citation[16] verified that the addition of calcium lactate (up to 20 g kg−1) to the osmotic solution containing sucrose (45 to 65°Brix), caused a reduction in water loss in treated mango samples. For Del Valle et al.,Citation[31] calcium cross-linking in cell walls should have increased mass transfer resistance to water outflow and sugar uptake in osmodehydrated apple pieces pre-treated with calcium chloride, but this behavior was not observed. The higher water loss and sugar gain rates in these treatments were attributed to membrane damage as a result of osmotic shock.
MECHANICAL PROPERTIES
Stress and Deformation at Failure
shows the stress at failure values for fresh and processed samples. The relatively large deviations in these measurements were probably due to heterogeneity amongst the fruits, and a lack of internal structure uniformity in these materials. The mechanical properties of a biological material are determined by its structure and cell wall constituents, as well as by the process conditions, ripeness levels and harvest time. Considerable variability in the raw materials was also observed by Pereira et al.Citation[32] and Lewicki & LukaszukCitation[21] in their studies with guavas and apples, respectively.
Figure 3 Stress at failure of melon pieces subjected to different treatments. FR: without osmotic treatment; S40: osmotically dehydrated in a 40°Brix sucrose solution; S40CA5 to S40CA20: osmotically dehydrated in a 40°Brix sucrose solution with the addition of 5–20 g kg−1 of calcium lactate; S60: osmotically dehydrated in a 60°Brix sucrose solution; S60CA5 to S60CA20: osmotically dehydrated in a 60°Brix sucrose solution with the addition of 5 – 20 g kg−1 of calcium lactate. Mean separation by the Tukey test. Different letters indicate statistically significant differences at p ≤ 0.05.
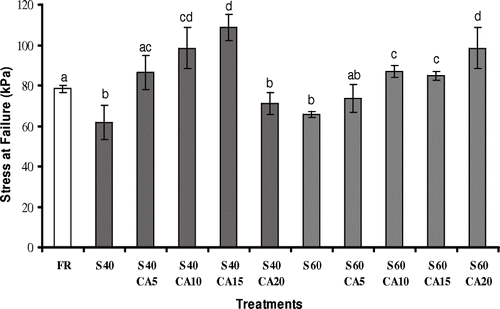
The stress at failure value (σH) for the fresh sample was 78.37 ± 1.77 kPa. The osmotic process with sucrose solutions at 40 or 60°Brix caused a significant decrease in the stress at rupture values (61.68 ± 8.47 kPa and 65.68 ± 1.64 kPa, respectively), which is in agreement with the results obtained by Lewicki & Lukaszuk,Citation[21] Prinzivalli et al.,[7] and Chiralt et al.Citation[8] According to the authors, textural changes can be correlated to the water loss and sugar uptake rates. Furthermore, there is a direct relationship between softening, chemical, and enzymatic factors, such as protopectin dissolution, loss of cell turgor pressure and degradation of the middle lamella induced by the osmotic dehydration process. In another study with melon pieces, Sanjinez-Argandona et al.Citation[33] verified a decrease in stress at rupture values for the samples osmodehydrated with sucrose and weak acids (citric or lactic acid). In this case, the reduction in stress at rupture was mainly associated with the sugar uptake rates, resulting in a less rigid product.
The addition of calcium lactate to the osmotic solution provided a strong influence on the sample stress at rupture values. For the fruits subjected to an osmotic treatment with sucrose at 40°Brix, as the calcium concentration increased from 5 to 15 g kg−1, so the hardness of the treated melon pieces increased, but the samples treated with 20 g kg−1 of calcium lactate showed a considerable decrease (p ≤ 0.05) in the stress at failure values. In this case, it is possible that the calcium lactate addition to the osmotic solution may have caused a higher dehydrating effect, promoting an significant (p ≤ 0.05) increase in water loss and solids uptake rates (), which resulted in a loss in firmness, altering the fruit texture quality. Additionally, this behavior could be a consequence of some structural damage caused by the mass transfer fluxes during the osmotic process.Citation[34] Pereira et al.Citation[10] studying the osmotic dehydration of guava with sucrose (60°Brix) and calcium chloride (5 to 25 g kg−1), verified a calcium ion incorporation resulting in a 3- to 10-fold higher calcium content than that found in the fresh fruit. For the samples treated with calcium chloride at concentrations higher than 5 g kg−1, rupture did not occur and some structural changes were also observed.
For melon pieces treated with sucrose solutions at 60°Brix, the increase in salt concentration in the osmotic solution resulted in higher stress at rupture values, and was statistically significant only for calcium concentrations greater than 10 g kg−1 (). The strain at failure was also influenced by the osmotic dehydration, with or without the addition of calcium lactate to the osmotic solution (). Fresh fruit showed a strain at failure of 0.289 ± 0.026, while osmodehydrated samples in sucrose solutions presented greater values than the fresh ones (p ≤ 0.05). Nevertheless, the effect of calcium lactate on the strain at failure was not pronounced. Torres et al.Citation[16] also failed to find a significant influence of the use of calcium lactate (0 to 20 g kg−1) as the dehydrating agent, on the strain at failure value of osmodehydrated mango pieces. On the other hand, an increase in the strain at failure after the osmotic process was reported in similar studies with kiwiCitation[8] and guavaCitation[10].
Figure 4 Strain at failure of melon pieces subjected to different treatments. FR: without osmotic treatment; S40: osmotically dehydrated in a 40°Brix sucrose solution; S40CA5 to S40CA20: osmotically dehydrated in a 40°Brix sucrose solution with the addition of 5–20 g kg−1 of calcium lactate; S60: osmotically dehydrated in a 60°Brix sucrose solution; S60CA5 to S60CA20: osmotically dehydrated in a 60°Brix sucrose solution with the addition of 5 – 20 g kg−1 of calcium lactate. Mean separation by the Tukey test. Different letters indicate statistically significant differences at p ≤ 0.05.
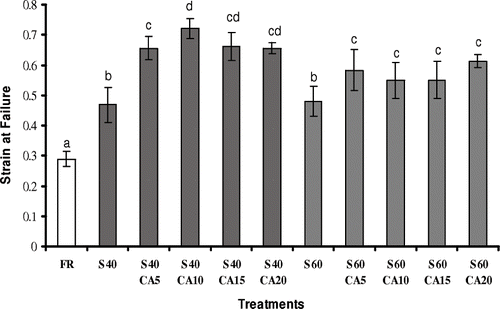
According to Mayor et al.Citation[35] and Krokida et al.,Citation[36] the decrease in moisture content throughout the osmotic process causes an increase in strain at failure of osmodehydrated samples. This fact is associated with the water reduction and sugar gain fluxes, showing that sugar incorporation during the osmotic process provides structural plasticity, affecting the mechanical properties of the fruit directly, due to the loss in elasticity. In the present work, the treatments with sucrose solutions at 40°Brix resulted in higher strain at failure values, which could be related to the greater sugar () and salt () incorporations observed under these osmotic conditions.
Relaxation Time and Residual Stress
The viscoelastic behaviour of the melon samples was evaluated from the relaxation time (τ) and residual stress (Sr) parameters. Fresh samples showed τ = 12.37 ± 1.01 s and Sr = 0.430 ± 0.033. These rheological properties are indirectly related to texture and, consequently, reflect the macro and microscopic food features. The conception of relaxation time is complex, but can be explained as the time taken for a macromolecule to be stretched out when subjected to a determined deformation rate, or the ratio between the viscous and elastic characteristics. Hence, higher relaxation times imply greater effort to deform the material structure. Residual stress represents the measurement of the elastic part of a viscoelastic material and is equal to the stress that is not relaxed, even after an infinite relaxation time. Greater values for this parameter are associated with a more solid-like or more rigid material.Citation[37]
As can be seen in , there were statistical differences in the relaxation times (p ≤ 0.05) amongst the fresh and processed samples. Osmodehydrated melon pieces showed relaxation time results about five-fold lower than fresh ones, whereas the residual stress values (data not shown) were almost negligible for the osmotically dehydrated fruits. These results indicated that the elastic fresh fruit characteristics were not maintained throughout the osmotic dehydration, even for the treatments carried out with calcium lactate addition. According to Chiralt et al.,Citation[8] relaxation measurements indicated that the process sharply decreased the elastic component of the viscoelastic material. In addition, Lewicki and LukaszukCitation[21] verified a reduction in the relaxation time and residual stress results in tomatoes pre-treated with calcium and subjected to osmotic and drying processes. For the authors, osmodehydrated samples presented a lower resistance to deformation and more viscous characteristics in comparison to fruits with no osmotic treatment. Moreover, the sugar gains occurring during the osmotic process caused structural plasticity, reflecting directly on the fruit rheological behavior, due to the simultaneous loss of elasticity and increase in viscous nature of the osmodehydrated products. Since the mass transfer rates occurring throughout the osmotic process have a great influence on the viscoelastic characteristics of the osmotically dehydrated products, the significant reduction in relaxation time values during the process can also be related to the osmotic treatment time. This is better observed at the beginning of the process (in the first two hours), when the mass transfer fluxes are more intense, leading to very low relaxation time results and almost null residual stress values.
Figure 5 Relaxation time of melon pieces subjected to different treatments. FR: without osmotic treatment; S40: osmotically dehydrated in a 40°Brix sucrose solution; S40CA5 to S40CA20: osmotically dehydrated in a 40°Brix sucrose solution with the addition of 5–20 g kg−1 of calcium lactate; S60: osmotically dehydrated in a 60°Brix sucrose solution; S60CA5 to S60CA20: osmotically dehydrated in a 60°Brix sucrose solution with the addition of 5 – 20 g kg−1 of calcium lactate. Mean separation by the Tukey test. Different letters indicate statistically significant differences at p ≤ 0.05.
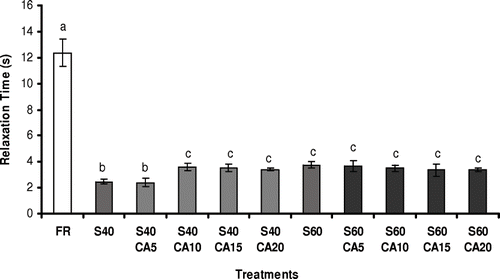
In a similar work with melon cylinders, Mastrangelo et al. Citation[9] observed that the fruits subjected to vacuum or atmospheric osmotic treatment with glucose and calcium lactate as the osmotic agents, presented relaxation times remarkably smaller than those found for raw melon. In addition, the results obtained for the residual stress of the fresh samples were more than eight times higher than those obtained for the osmodehydrated melon pieces, showing the high internal fracturing suffered by the fruits during the process. Pereira et al. Citation[10] reported some alterations in the viscoelastic behavior of osmodehydrated guava halves, but these modifications were less severe in comparison to the melon results, due to the structural differences between the two fruits. According to the authors, the addition of calcium lactate to the osmotic solution caused a decrease in relaxation time and residual stress values of the treated samples. On the other hand, the use of calcium chloride avoided the decrease in relaxation time, but had no significant effect on the residual stress values.
Structural Changes
illustrates a transversal section of fresh melon mesocarp. The fresh tissue showed turgid and round shaped cells with a well-defined, consistent, thin cell wall (solid arrow). The vacuole (V) occupied the majority of the cell volume and contained the larger portion of the cell water content. The high moisture content of fresh melon tissue (> 92%) resulted in vacuole enlargement, pushing the cytoplasm with its organelles towards the cell periphery and hindering its delimitation.
Figure 6 Micrograph of fresh melon mesocarp (parenchyma tissue). Scale bar = 140μm. V = vacuole; IE = intercellular spaces; solid arrow = cell wall.
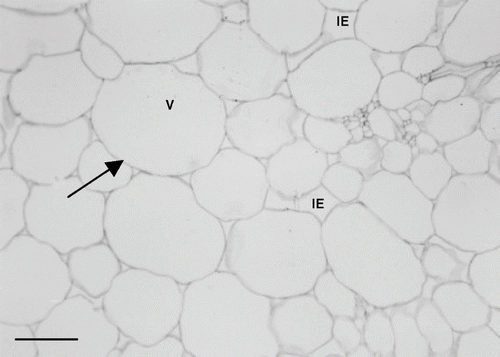
shows the cellular structure of osmodehydrated melon in sucrose solutions, with or without calcium lactate. All the processed samples showed a reduction in intercellular space and an increase in cell-to-cell contact, due to the tissue shrinkage and mass transfer fluxes observed in osmotic treatments, in which the sugars tend to occupy the intercellular spaces. This fact was observed in all the osmotic conditions, with the exception of the treatment with sucrose solution at 40°Brix and 20 g kg−1 of calcium lactate (). Several papers have also reported a decrease in intercellular spaces as a consequence of the osmotic process in apple,Citation38–39 melon,Citation[9] and strawberryCitation[7].
Figure 7 Micrographs of melon mesocarp (parenchyma tissue) subjected to different treatments. (a) osmodehydrated in a 40°°Brix sucrose solution; (b), (c), (d) and (e) osmodehydrated in a 40˚Brix sucrose solution with the addition of 5, 10, 15 and 20 g kg−1 of calcium lactate; (f) osmodehydrated in a 60°Brix sucrose solution; (g), (h), (i) and (j) osmodehydrated in a 60°Brix sucrose solution with the addition of 5, 10, 15 and 20 g kg−1 of calcium lactate. Scale Bar = 140μm. solid arrows = cell wall damage; dashed arrows = cellular plasmolysis.
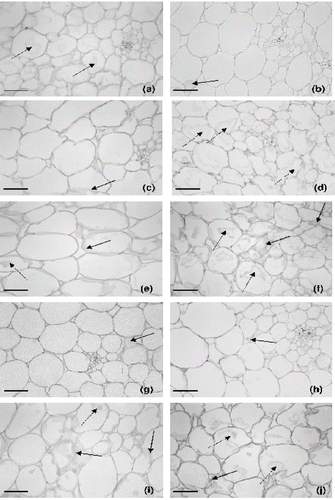
The osmotic dehydration in sucrose solutions caused intense cellular plasmolysis (dashed arrows) ( and f). The cells were more deformed, contracted, and collapsed due to the water loss throughout the osmotic process, promoting loss of cell turgor pressure, alterations in the middle lamella and cell wall damage (solid arrows). Comparing both experiments without the addition of calcium, structural damage was more intense in the osmotic treatment at 60°Brix, which can be correlated with its more severe nature and to the dissolution of cell wall pectin during the osmotic process, leading to a loss of cellular cohesion and consequently tissue disintegration.Citation[34] Prinzivalli et al. Citation[7] working with osmotic dehydration of strawberry slices at 25°C in 60°Brix sucrose solution during 6 hours observed a turgor loss with a gradual detachment and breakdown of the tissue along theprocess. Besides, a loss of cells shape and cytoplasm retraction was also noticed after 2 hours of osmotic treatment.
The addition of calcium lactate to the osmotic solution promoted the structural preservation of the melon tissue, when used in concentrations below 10 g kg−1 for sucrose treatments at 40 or 60°Brix (, 7c, 7g, and 7h). The negative impact of the osmotic process on cell tissue integrity and the beneficial effects of the use of calcium lactate in osmotic dehydration have been reported by some researchers in similar studies with strawberry,Citation[7] mango,Citation[16] and apple.[Citation30,Citation34] Fruits treated with 5 g kg−1 of calcium lactate presented a cellular structure more similar to that of fresh samples ( and g). Nevertheless, a calcium concentration of 15 g kg−1 caused severe damage to melon tissue, such as cytoplasm plasmolysis, loss of cell wall resistance, increase in intercellular space and turgor loss, resulting in remarkable modifications in cell shape and size (, 7i, and 7j). Although the fruits treated under this condition showed higher stress at rupture values (), and consequently a strengthening of the cell wall, a beneficial effect of the use of calcium lactate on cell structure maintenance was not verified. Samples osmodehydrated with sucrose solutions (40°Brix) and calcium lactate (20 g kg−1) also showed intense structural injury (). According to Quiles et al.,Citation[34] such tissue damage may have caused loss of cell cohesion, contributing to the decrease in stress at rupture values, as observed in . The osmotic process results in cell de-bonding, due to the loss in adhesion between adjacent cell walls.[Citation8,Citation40]Furthermore, this behavior can be attributed to the great calcium uptake observed under this condition (), causing some structural damage to the melon tissue. Pereira et al.Citation[10] attributed the damage to the cell structure of osmodehydrated guava to the elevated calcium incorporation verified when calcium chloride was used at concentrations higher than 5 g kg−1. Thus in the present work, it is possible that the higher calcium ion incorporation could have contributed to the intense cell damage in the osmotic treatments using calcium salts at concentrations above 15 g kg−1.
Despite severe cellular plasmolysis caused by the water loss, as verified with calcium lactate concentrations above 15 g kg−1, the water content of the fruit remained practically constant as the salt concentration increased (). Thus the osmotic process allowed the water to flow out to the spaces between the cytoplasm and cell wall (periplasmatic space), but the rigid, structured cell wall provided by the calcium ions and pectin linkages hindered the loss of water from these spaces, with no alterations in the total fruit water content.
Sensory Preference
Melon pieces osmodehydrated in sucrose solutions at 60°Brix with or without the addition of calcium showed good sensory acceptance for all the sensory attributes evaluated (). On the other hand, slightly lower scores were observed for samples treated with sucrose solutions at 40°Brix with calcium lactate concentrations above 15 g kg−1, although the average scores were above the acceptability limit (4.5). Texture and flavor were the attributes most influenced, contributing directly to the statistically lower (p ≤ 0.05) overall impression scores for these treatments. The poor sensory acceptance of fruits processed under these conditions can be related to the intense effect of the calcium lactate on the melon mechanical properties, which was consistent with the light microscopy results, showing considerable cell damage when the salt was employed at concentrations greater than 15 g kg−1. According to some consumers, these osmodehydrated fruits were more rigid and sweeter as compared to fresh and other processed samples. The lower scores obtained for flavor were probably linked to the higher solids uptake observed for sucrose treatments at 40°Brix, making the product sweeter and consequently significantly affecting the consumer scores (p ≤ 0.05) under these conditions.
Table 2 Sensory acceptance scores of melon pieces subjected to different osmotic treatments, in relation to appearance, aroma, flavor, texture and overall impression
Nevertheless, the good acceptance of samples osmodehydrated with sucrose solutions at 60°Brix was correlated with the relatively lower solids gain occurring during the osmotic process (), resulting in a fruit with a more agreeable flavor. Additionally, the statistically significant reduction in calcium incorporation on melon tissue and the changes in mechanical properties results also showed a great influence on sensory acceptance (p ≤ 0.05). The samples treated with sucrose at 60°Brix plus calcium lactate had a significantly higher score for the attribute of texture than the samples processed with sucrose at 40°Brix and calcium concentrations above 15 g kg−1 (p ≤ 0.05). The evaluation of the cellular structure agreed with the sensory analysis results only for the treatments performed with sucrose solutions at 40°Brix, where the samples better accepted by the consumers were those processed using 5 and 10 g kg−1 of calcium lactate. For the treatments with more concentrated sucrose solutions (60°Brix), despite some structural damage observed with calcium lactate concentrations above 15 g kg−1, these alterations did not significantly influence product sensory acceptance.
For the attribute of appearance, fruits osmotically dehydrated without the addition of calcium lactate presented higher scores (statistically significant at p ≤ 0.05) than samples treated with the addition of the calcium salt. This fact can be explained by the higher water loss rates observed in the osmotic experiments carried out with calcium lactate, causing remarkable modifications in fruit appearance, due to the greater tissue shrinkage verified under these conditions.
CONCLUSIONS
The addition of calcium lactate to the sucrose solutions showed a strong influence on the mechanical properties and cellular structure of osmodehydrated melon pieces, promoting preservation of the tissue structure when the salt was used in concentrations up to 10 g kg−1. Stress at rupture values showed a firming effect provided by the calcium lactate, which could be related to cell wall strengthening and to the more structured cellular arrangement of the fruits osmodehydrated fruits with the addition of the calcium salt. The relaxation time and residual stress data indicated that the elastic and viscous fresh fruit characteristics were not maintained throughout osmotic dehydration, even for the treatments carried out with the addition of calcium lactate. The use of calcium lactate as a dehydrating agent also influenced the mass transfer rates during the osmotic process, causing a remarkable increase in the water loss flux under all the conditions evaluated. Furthermore, the combination of sucrose treatments at 40°Brix with the addition of calcium lactate at concentrations above 15 g kg−1 provided greater calcium ion incorporation, resulting in a fruit calcium content 3 to 5-fold higher than that of the fresh sample. However, this behavior was not favorable for product quality, since the texture and flavor attributes were considerably affected in the sensory analysis. Fruits processed with sucrose solutions at 60°Brix with or without the addition of calcium lactate showed high acceptance for all the attributes evaluated, while for the treatments with sucrose solutions at 40°Brix, lower sensory acceptance scores were observed in the samples treated with calcium lactate concentrations above 15 g kg1. Despite some physical alterations observed in the osmodehydrated products, the osmotic dehydration process with sucrose and calcium lactate solutions was efficient in the maintenance of the final product characteristics. However, shelf-life studies should be carried out in the future to verify the storage stability of these products.
ACKNOWLEDGMENTS
The authors would like to thank CNPq, Capes-Grices, and Fapesp for their financial support.
REFERENCES
- Gonzalez-Martinez , C. , Chafer , M. , Xue , K. and Chiralt , A. 2006 . Effect of the Osmotic Pre-Treatment on the Convective Air Drying Kinetics of Pear Var. Blanquilla . International Journal of Food Properties , 9 ( 3 ) : 541 – 549 .
- Tsamo , C.V.P. , Bilame , A.F. and Ndjouenkeu , R. 2006 . Air Drying Behaviour of Fresh and Osmotically Dehydrated Onion Slices (Allium cepa) and Tomato Fruits (Lycopersicon esculentum) . International Journal of Food Properties , 9 ( 4 ) : 877 – 888 .
- Torreggiani , , D. and Bertolo , G. 2001 . Osmotic Pre-Treatments in Fruit Processing: Chemical, Physical and Structural Effects . Journal of Food Engineering , 49 ( 2–3 ) : 247 – 253 .
- Buggenhout , S.V. , Grauwet , T. , Loey , A.V. and Hendrickx , M. 2008 . Use of Pectinmethylesterase and Calcium in Osmotic Dehydration and Osmodehydrofreezing of Strawberries . European Food Research and Technology , 226 ( 5 ) : 1145 – 1154 .
- Rahman , M.S. 2005 . Dried Food Properties: Challenges Ahead . Drying Technology , 23 ( 4 ) : 695 – 715 .
- Telis , V.R.N. , Telis-Romero , J. and Gabas , A.L. 2005 . Solids Rheology for Dehydrated Food and Biological Materials . Drying Technology , 23 ( 4 ) : 759 – 780 .
- Prinzivalli , C. , Brambilla , A. , Maffi , D. , Lo Scalzo , R. and Torreggiani , D. 2006 . Effect of Osmosis Time on Structure, Texture and Pectin Composition of Strawberry Tissue . European Food Research and Technology , 224 ( 1 ) : 119 – 127 .
- Chiralt , A. , Martinez-Navarrete , N. , Martinez-Monzó , J. , Talens , P. and Moraga , G. 2001 . Changes in Mechanical Properties throughout Osmotic Processes: Cryoprotectant Effect . Journal of Food Engineering , 49 ( 2–3 ) : 129 – 135 .
- Mastrangelo , M.M. , Rojas , A.M. , Castro , M.A. , Gerschenson , L.N. and Alzamora , S.M. 2000 . Texture and Structure of Glucose-Infused Melon . Journal of the Science of Food and Agriculture , 80 ( 6 ) : 769 – 776 .
- Pereira , L.M. , Carmello-Guerreiro , S.M. , Bolini , H.M.A. , Cunha , R.L. and Hubinger , M.D. 2007 . Effect of Calcium Salts on the Texture, Structure and Sensory Acceptance of Osmotically Dehydrated Guavas . Journal of the Science of Food and Agriculture , 87 ( 6 ) : 1149 – 1156 .
- Rico , D. , Martin-Diana , A.B. , Barat , J.M. and Barry-Ryan , C. 2007 . Extending and Measuring the Quality of Fresh-Cut Fruit and Vegetables: a Review . Trends in Food Science and Technology , 18 ( 7 ) : 373 – 386 .
- Martin-Diana , A.B. , Rico , D. , Frias , J.M. , Barat , J.M. , Henehan , G.T.M. and Barry-Ryan , C. 2007 . Calcium for Extending the Shelf Life of Fresh Whole and Minimally Processed Fruits and Vegetables: A Review . Trends in Food Science and Technology , 18 ( 4 ) : 210 – 218 .
- Lewicki , P.P. , Vu Le , H. and Pomaranska-Lazuca , W. 2002 . Effect of Pre-Treatment on Convective Drying of Tomatoes . Journal of Food Engineering , 54 ( 2 ) : 141 – 146 .
- Luna-Guzman , I. and Barret , D.M. 2000 . Comparison of Calcium Chloride and Calcium Lactate Effectiveness in Maintainig Shelf Stability and Quality of Fresh-Cut Cantaloupe . Postharvest Biology and Technology , 19 ( 1 ) : 61 – 72 .
- Manganaris , G.A. , Vasilakakis , M. , Diamantidis , G. and Mignani , I. 2007 . Effect of Post-Harvest Calcium Application on Tissue Calcium Concentration, Quality Attributes Incidence of Flesh Browning and Cell Wall Physicochemical Aspects of Peach Fruits . Food Chemistry , 100 ( 4 ) : 1385 – 1392 .
- Torres , J.D. , Talens , P. , Escriche , I. and Chiralt , A . 2006 . Influence of Process Conditions on Mechanical Properties of Osmotically Dehydrated Mango . Journal of Food Engineering , 74 ( 2 ) : 240 – 246 .
- Anino , S.V. , Salvatori , D.M. and Alzamora , S.M. 2006 . Changes in Calcium Level and Mechanical Properties of Apple Tissue due to Impregnation with Calcium Salts . Food Research International , 39 ( 2 ) : 154 – 164 .
- Association of Official Analytical Chemists . 2002 . Official Method of Analysis of the Association of Official Analytical Chemists , 17th , Arlington , , USA : AOAC .
- Costell , E. , Fisman , S.M. and Durán , L. 1997 . “ Physical Properties I – Rheology of Solids and Texture ” . In Themes in Food Technology , Edited by: Aguilera , J.M . 215 – 260 . Mexico City, Mexico , , Spanish : National Polytechnic Institute .
- Peleg , M. 1980 . Linearization of Relaxation and Creep Curves of Solid Biological Materials . Journal of Rheology , 24 ( 4 ) : 451 – 463 .
- Lewicki , P.P. and Lukaszuk , A. 2000 . Effect of Osmotic Dewatering on Rheological Properties of Apple Subjected to Convective Drying . Journal of Food Engineering , 45 ( 3 ) : 119 – 126 .
- Luza , J.G. , Gorsel , Van , R.; Polito , V.S. and Kader , A.A. 1992 . Chilling Injury in Peaches: A Cytochemical and Ultrastructural Cell Wall Study . Journal of the American Society for Horticultural Science , 117 ( 1 ) : 114 – 118 .
- O'Brien , T.P. , Feder , N. and Mccully , M.E. 1964 . Polychromatic Staining of Plant Cell Walls by Toluidine Blue . Protoplasma , 59 ( 2 ) : 368 – 373 .
- Meilgaard , M. , Civille , G.V. and Carr , B.T. 1999 . Sensory Evaluation Techniques , 3rd , 387 Boca Raton, FL : CRC Press Inc .
- Moreno-Castillo , E. J. , González-Garcia , R. , Grajales-Lagunes , A. , Ruiz-Cabrera , M. A. and Abud-Archila , M. 2005 . Water Diffusivity and Color of Cactus Pear Fruits (Opuntia Ficus Indica) Subjected to Osmotic Dehydration . International Journal of Food Properties. , 8 ( 2 ) : 323 – 336 .
- Rodrigues , , S. and Fernandes , F.A.N. 2007 . Image Analysis of Osmotically Dehydrated Fruits: Melons Dehydrated in a Ternary System . European Food Research and Technology , 225 ( 5–6 ) : 685 – 691 .
- Talens , P. , Martinez-Navarrete , N. , Fito , , P. and Chiralt , A. 2002 . Changes in Optical and Mechanical Properties during Osmodehydrofreezing of Kiwi Fruit . Innovative Food Science and Emerging Technologies , 3 ( 2 ) : 191 – 199 .
- Sereno , A.M. , Moreira , R. and Martinez , E. 2001 . Mass Transfer Coefficients during Osmotic Dehydration of Apple in Single and Combined Aqueous Solutions of Sugar and Salt . Journal of Food Engineering , 47 ( 1 ) : 43 – 49 .
- Mastrantonio , S.D.S. , Pereira , L.M. and Hubinger , M.D. 2005 . Osmotic Dehydration Kinetics of Guavas in Maltose Solutions with Calcium Salt . Alimentos e Nutrição , 16 ( 4 ) : 309 – 314 .
- Barrera , C. , Betoret , N. and Fito , P. 2004 . Influence on the Osmotic Dehydration Kinetics of apple Slices (var. Granny Smith) . Journal of Food Engineering , 65 ( 1 ) : 9 – 14 .
- Del Valle , J.M. , Cuadros , T.R.M. and Aguilera , J.M. 1998 . Glass Transitions and Shrinkage during Drying and Storage of Osmosed Apple Pieces . Food Research International , 31 ( 3 ) : 191 – 204 .
- Pereira , L.M. , Rodrigues , A.C.C. , Sarantópoulos , C.I.G.L. , Junqueira , V.C.A. , Cunha , R.L. and Hubinger , M.D. 2004 . Influence of Modified Atmosphere Packaging and Osmotic Dehydration of Minimally Processed Guavas . Journal of Food Science , 69 ( 4 ) : 172 – 177 .
- Sanjinez-Argandona , E.J. , Nishiyama , C. and Hubinger , M.D. 2002 . Quality of osmotically dehydrated melon in sucrose soltions with addition of acids . Pesquisa Agropecuária Brasileira , 37 ( 17 ) : 1803 – 1810 . (in Portuguese)
- Quiles , A. , Hernando , I. , Pérez-Munuera , I. , Llorca , E. , Larrea , V. and Lluch , M.A. 2004 . The Effect of Calcium and Cellular Permeabilization on the Structure of the Parenchyma of Osmotic Dehydrated ‘Granny Smith’ Apple . Journal of the Science of Food and Agriculture , 84 ( 13 ) : 1765 – 1770 .
- Mayor , L. , Cunha , R.L. and Sereno , A.M. 2007 . Relation between Mechanical Properties and Structural Changes during Osmotic Dehydration of Pumpkin . Food Research International , 40 ( 4 ) : 448 – 460 .
- Krokida , M.K. , Karathanos , V.T. and Maroulis , Z.N. 2000 . Effect of Osmotic Dehydration on Viscoelastic Properties of Apple and Banana . Drying Technology , 18 ( 4–5 ) : 951 – 966 .
- Steffe , J.F. 1996 . Rheological methods in food process engineering , 2nd , 418 East Lansing , Michigan : Freeman Press .
- Martinez , V.Y. , Nieto , A.B. , Castro , M.A. , Salvatori , D. and Alzamora , S.M. 2007 . Viscoelastic characteristics of Granny Smith Apple during Glucose Osmotic Dehydration . Journal of Food Engineering , 83 ( 3 ) : 394 – 403 .
- Nieto , A.B. , Salvatori , D.M. , Castro , M.A. and Alzamora , S.M. 2004 . Structural Changes in Apple Tissue during Glucose and Sucrose Osmotic Dehydration: Shrinkage, Porosity, Density and Microscopic Features . Journal of Food Engineering , 61 ( 2 ) : 269 – 278 .
- Lewicki , P.P. and Porzecka-Pawlak , R. 2005 . Effect of Osmotic Dewatering on Apple Tissue Structure . Journal of Food Engineering , 66 ( 1 ) : 43 – 50 .