Abstract
TMCT (Thermal Mechanical Compression Test) was applied to measure the Tg-r (glass-rubber transition temperature) of rice flour and individual rice kernels at low moisture content (2.4–19.5% wet basis). As expected, Tg-r decreased with increasing moisture content. The Tg-r temperatures measured by TMCT were comparable with those measured by DSC (Differential Scanning Calorimetry), TMA (Thermo-mechanical Analysis), and DMTA (Dynamic Mechanical Thermal Analysis) as reported in the literature. These results indicated that the TMCT technique could be applied to measure the glass-rubber transition of a single grain of rice or the rice in powder form. The sensitivity of the test was observed higher for the rice kernel than rice flour. TMCT technique has the advantage of usefulness and cost-effectiveness compared with the other techniques.
INTRODUCTION
Determination of the glass transition temperature (T g ) of rice, corresponding to its water content, has become very important since the glass transition concept has been applied in order to explain rice fissure formation during drying.Citation[1,Citation2] Most of the studies involved in estimating T g of granular starch were carried out on the water-starch systemCitation3–6 rather than on native starch or individual kernel. It has been pointed out that DSC (Differential Scanning Calorimetry), a common method to determine glass transition temperature, could not detect the T g of low moisture, high molecular weight biological polymers such as starch systems. This is due to several drawbacks related to the nature of native starch at molecular and micro-structural levels and small change in the heat capacity during the transition from one state to another.Citation[3,Citation6] These two factors result in broadening of the thermal event, which makes it difficult to detect the step changes in specific heat. For such materials, detection of changes in the mechanical properties has been proved more sensitive than the changes in the heat capacity.Citation[7] Therefore, TMA (Thermo-mechanical Analysis) and DMTA (Dynamic Mechanical Thermal Analysis) are employed to detect the glass transition event.Citation[8,Citation9] Recently, Rahman et al.Citation[10] has published a comparative study between various methods used to determine the glass transition temperature by considering spaghetti as a model food material. TMA has been applied to develop the state diagram for mapping glass transition temperature at different moisture levels in brown riceCitation[8]. DMTA has also been employed to measure the glass transition temperature of brown rice kernels at low moisture content range (5–20% wet basis).Citation[9] However, in both methods a complex sample preparation technique is required, for example, covering rice kernel with Al2O3 powder, to prevent moisture loss when heating rice kernels.Citation[9]
The recent TMCT (Thermal Mechanical Compression Test) device, which was developed by Bhandari and co-workers at The University of Queensland, Australia, has a potential to measure the physical state transition temperature of any solid materials without much sample preparation step.Citation11–13 This technique is based on the force-deformation or stress-strain response of a material under compression during heating. The amorphous state of material will be transformed from a glassy to rubbery state under the compression and heating, thereby allowing the phase change to be detected by a sudden displacement of the compression probe. The transition is termed as glass-rubber transition (T g-r ) since the probe displacement occurs due to the change in the viscosity of material at the interface of heating surface. This is similar to a creep test but under thermal scanning condition. The sample cell is designed to be compatible with mechanical analyzers such as Instron or Texture Analyser. Any mechanical changes that occur on the particle surface can be sensitively detected, owing to material being in contact with a large surface area under the compression probe. Furthermore, moisture loss can be minimized since the contact surface of sample is covered by the compression probe. To note, only the change in the mechanical property of the sample surface that is in contact with the heating surface is enough to detect the transition temperature. This TMCT has been applied to detect the glass-rubber transition temperature (T g-r ) of various dry food materials, i.e. skim milk, whey, honey, and apple juice powders, pasta, and starch powders against standard DSC and TMA methods.Citation11–13 The objective of this work was to investigate the applicability of TMCT to measure glass-rubber transition temperatures of individual rice kernel and rice flour. The values obtained by this method will be compared with the literature values, which are obtained by TMA and DMTA techniques.
METHODOLOGY
TMCT System
illustrates the TMCT system comprising of a thermally controlled aluminium sample block (50 × 50 × 25 cm), which is heated normally at a rate of 30°C/min through heating elements inserted into the sample block. The Texture Analyser TA-XTplus (Stable Microsystems, UK) records the probe position and compression force at specific time, and temperature through 35 mm cylindrical probe and temperature probe, respectively.
In this test, the probe displacement or movement is detected when there is a transformation of physical state of the sample from the glassy to rubbery state. The onset temperature at this point is referred as ‘glass-rubber transition temperature’ (T g-r ). TMCT is designed to detect glass-rubber transition by heat scanning only. The samples should already be in glass state at ambient condition. It is not possible to measure the reversible transition event while cooling.
Sample preparation
The measurements were done using single kernel of rice or the flour from the same rice sample. Milled YRM64 rice flour was dried in a vacuum oven at 40°C for 24 h. The sample was then cooled down in a desiccator and stored in tight container at 25°C for further use. Saturated salts at different water activities were prepared at 25°C as indicated by Bell and LabuzaCitation[14]. Aluminum pans each containing a thin layer of 5 g dried milled rice flour was transferred into vacuum desiccators. Samples were allowed to equilibrate at 25°C for at least 3 weeks. Moisture content of rice flour was determined according to AOAC method (32.1.02)Citation[15].
Sample data correction and testing procedure
All the tests were carried out under creep mode of TA-TXplus (Stable Microsystems, UK). A 35 mm cylindrical compression probe was used. Constant force (stress) 49.033 N was applied to the sample and corresponding deformation (strain) was measured with time. Data acquisition rate was 1 pps (point per second). Due to the possible expansion of the block and the probe during heat scanning, there can be a movement of the probe, which will underestimate the result. Therefore, a blank heat scanning was carried out. The blank data were used to correct the data obtained from the sample scanning. Pre-dried maltodextrin (DE6) was used as a blank material for data correctionCitation[13]. Approximately 1 g of maltodextrin was spread thinly on the sample cell and held under the probe at the force of 49.033 N for 300 seconds before the thermal scanning. The maltodextrin was then scanned from room temperature to 200°C at a heating rate of 30°C/min. During this thermal scanning, the displacement distances of the probe were recorded. The procedure to conduct TMCT for equilibrated rice flour was similar to maltodextrin, except the scanning temperature up to 150°C was used because rice flour is likely to decompose at higher temperature. Each sample was carried out in triplicate and average value was used as glass-rubber transition temperature. Representative curves obtained during thermal scanning are presented in . The changes in the temperature and corresponding probe displacement can be seen in this figure.
Determination of glass-rubber transition temperature
The temperature-distance-temperature data was extracted for further analysis. The corrected curve for each sample was obtained by subtracting the displacement blank data from the sample data. The temperature vs. subtracted displacement was plotted to estimate the T g-r by performing a non-linear regression as shown in .
RESULTS AND DISCUSSION
TMCT curves of YRM64 milled rice flour at some selected moisture contents are presented in . The slopes were steeper at higher moisture contents indicating that moisture content possibly weakens the intermolecular forces of materials in solid state. Our attempt to measure the transition event using a DSC was not successful. No change could be detected in the DSC thermograms (results not presented). As stated earlier, the sharp changes in the mechanical property of the material allowed detecting the glass transition regions easier than using the DSC technique, which is based on specific heat change of material during this transition.
presents the dependence of T g-r on moisture content. It shows clearly that the T g-r decreases with increasing moisture content. This result emphasizes the role of water as strong plasticizer because the presence of water enhances the molecular mobility resulting in lower T g-r according to the free volume theory. The results presented in indicated that the T g-r value was not decreased sharply at higher moisture content, probably due to the limit of the plasticization effect of water on rice. In this case, additional water does not interact strongly with the starch or protein molecules in rice, therefore fails to decrease the T g-r rapidly. The system can behave as phase separated into water and solid. To predict glass-rubber transition temperature as a function of solid fraction of water, Gordon-Taylor model was used to fit the data. Note that, this model was fitted based on the measured data T g-r in the range of moisture content 2.4–19.5% wet basis. Due to the possible phase separation system at high moisture content, this equation cannot be extrapolated beyond the experimental range used in this investigation. The k value calculated from this model is 1.339. A low value of k indicates nearly a linear behavior of the model. T g-r (°K) can be predicted for rice flour by TMCT technique as following equation:
where, w 1 and w 2 are mass fraction of solid and water, respectively. The predicted T g-r in this study was compared to the published data on Tg of rice at the same moisture content range as presented in . At the moisture content range 12–16% wet basis, predicted T g-r (41.6–56.7°C) was comparable to T g (approximately 50°C) determined by DSC.Citation[16] At 14.4% wet basis the predicted T g-r in our study was 47.7°C, which was quite close to that of measured by TMA at the same moisture contentCitation[8]. The T gs defined by DSC and DMTA (around 45°C) at approximately 16% wet basis were higher than our measured T g-r (40.38°C)Citation[17,Citation9]. This discrepancy may be explained partly by the evaporation loss in test chamber in DMTA. In DMTA, the measured temperature is not the actual sample temperature but of the test chamber temperature, and the T g may be higher than the actual value. In addition, the T g-r measured by TMCT is an onset value, whereas in DSC and DMTA analyse it is generally reported as a mid-point value (between Tg-on and Tg-end).
Table 1 Published data on Tg of rice as compared to T g-r measured in this study
Independent tests with the same procedure to rice flour were applied to determine the T g-r of individual rice kernels in order to investigate if single rice kernel can be used directly instead of ground flour. It was found that glass-rubber transition temperature of single rice kernel could be measured by TMCT as shown in . The measured T g-r values of rice kernels at 17, 14, and 10% wet basis were 40.9, 48.6, and 54.8°C, respectively. It is interesting to note that the measured T g-r was almost identical for both individual rice kernel and rice flour at the same moisture content. Furthermore, rice kernel showed sharper changes in displacement compared to those changes in rice flour ().
Figure 7 Mechanical behavior of both rice kernel and rice flour plotted at the same moisture contents. RK: Rice kernel; RF: Rice flour.
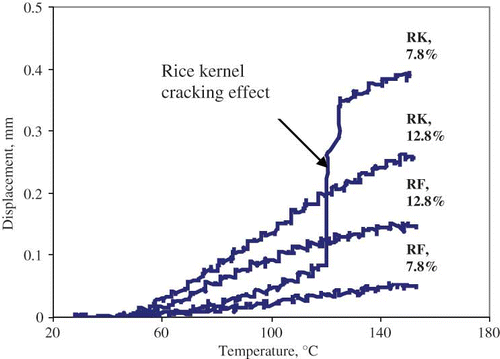
Another interesting observation was an occurrence of rice kernel cracking at low water content under compression during thermal scanning above the transition temperature. This showed a discontinuous change at 120°C in TMCT curve as represented in . It is possibly a consequence of temperature gradient within the rice kernel resulting in rubbery exterior and glassy interior. This translates into a mechanical stress enough to cause cracks in the kernel. The same mechanism has been understood to cause rice cracking during rewetting of the dried rice grain. At high water content, the temperature gradient might not be that large to cause such cracking under our experimental condition. The brittleness of the grain will also be low at higher moisture content.
CONCLUSION
In brief, thermal mechanical compression test was found to be applicable to determine the glass-rubber transition temperature of rice flour at a range of moisture content 2.4–19.5% wet basis, which is difficult to detect by common DSC method. The measured T g-r values in this study were not significantly different from those reported using TMA and DMTA techniques. It was also found that glass-rubber transition temperature of individual rice kernel could be measured directly by TMCT. This demonstrates the usefulness of this technique to measure the transition of not only rice grain but also other cereals or legumes when conventional DSC is not available or DSC does not provide adequate sensitivity.
REFERENCES
- Cnossen , A.G. , Siebenmorgen , T.J. , Yang , W. and Bautista , R.C. 2001 . An application of glass transition temperature to explain rice kernel fissure occurrence during the drying process . Drying Technology , 19 ( 8 ) : 1661 – 1682 .
- Perdon , A. , Sienbenmorgen , T.J. and Mauromoustakos , A. 2000 . Glassy state transition and rice drying: development of a brown rice state diagram . Cereal Chemistry , 77 ( 6 ) : 708 – 713 .
- Biliaderis , C.G. , Page , C.M. , Maurice , T.J. and Juliano , B.O. 1986 . Thermal characterization of rice starches: a polymer approach to phase transitions of granular starch . Journal of Agriculture and Food Chemistry , 34 : 6 – 14 .
- Chung , H.-J. , Lee , E.-J. and Lim , S.-T. 2002 . Comparison in glass transition and enthalpy relaxation between native and gelatinized rice starches . Carbohydrate Polymers. , 48 : 287 – 298 .
- Huang , R.-M. , Chang , W.-H. , Chang , Y.-H. and Lii , C.-Y. 1994 . Phase transitions of rice starch and flour gels. Cereal Chemistry . 71 ( 2 ) : 202 – 207 .
- Zelenak , K.J. and Hoseney , R.C. 1987 . The glass transition of starch . Cereal Chemistry. , 64 ( 2 ) : 121 – 124 .
- Roos , Y.H. 1995 . Phase transitions in foods , 360 San Diego , , US : Academic Press .
- Sun , Z. , Yang , W. , Sienbenmorgen , T. J. , Stelwagen , A. and Cnossen , A.G. 2002 . Thermomechanical transitions of rice kernels . Cereal Chemistry. , 79 ( 3 ) : 349 – 353 .
- Sienbenmorgen , T.J. , Yang , W. and Sun , Z. 2004 . Glass transition temperature of rice kernels determined by Dynamic Mechanical Thermal Analysis . Transactions of the ASAE , 47 ( 3 ) : 835 – 839 .
- Rahman , M. S. , Al-Marhubi , I. M. and Al-Mahrouqi , A. 2007 . Measurement of glass transition temperature by mechanical (DMTA), thermal (DSC and MDSC), water diffusion and density methods: A comparison study . Chemical Physics Letters. , 440 : 372 – 377 .
- Bhandari , B. 2007 . “ Stickiness and Caking in Food Preservation ” . In Handbook of Food Preservation , Edited by: Rahman , S. M. 387 – 401 . Boca Raton : CRC Press .
- Boonyai , P. , Bhandari , B. and Howes , T. 2006 . Applications in Thermal Mechanical Compression Tests in Food Powder Analysis . International Journal of Food Properties , 9 ( 1 ) : 127 – 134 .
- Boonyai , P. , Howes , T. and Bhandari , B. 2007 . Instrumentation and testing of a thermal mechanical compression test for glass-rubber transition analysis of food powders . Journal of Food Engineering , 78 : 1333 – 1342 .
- Bell , L.N. and Labuza , T.P. 2000 . Moisture sorption: Practical aspects of isotherm measurement and use , 2nd , 122 St. Paul , , Minnesota, USA : American Associations of Cereal Chemists .
- Association of Official Analytical Chemists International . 1990 . “ Chapter 32: Cereal Foods ” . In Official Methods of Analysis , 15th , 1 Gaithersburg , MD : AOAC .
- Nehus , Z.T. 1997 . Milled rice breakage as influenced by environmental conditions, kernel moisture content, and starch thermal properties , Fayetteville , AR : Ms Thesis. The University of Arkansas .
- Cao , W. , Nishiyama , Y. and Koide , S. 2004 . Physicochemical, mechanical and thermal properties of brown rice grain with various moisture contents . International Journal of Food Science and Technology. , 39 : 899 – 906 .