Abstract
Soymilk was prepared from boiled and unboiled comminuted suspensions of dehulled soybean and water using pressurized homogenization (one pass and two pass). Particle size showed significant differences between soymilks homogenized by one pass and two pass. Apparent viscosities and total solid contents showed significant differences between boiled and unboiled treatments. Soymilks exhibited pseudoplastic and thixotropic behavior and Arrhenius model was fitted to express temperature dependence of apparent viscosity. Microscopic images showed hydrated, separated, uniformly distributed, and homogeneous particles in boiled two-pass soymilk as they were disrupted easily and it was selected as the best treatment for processing soymilk containing all of the soybean solids.
INTRODUCTION
Soymilk is an aqueous extract of dry and mature soybeans, which is similar to cow's milk in appearance.Citation[1] The Chinese method of preparation utilizes soaking of soybeans in cold water until fully hydrated followed by fine grinding in stone mill with added water, filtration of the slurry to remove the insoluble solids, and boiling is done to obtain soymilk of better flavor. This process yields 65% of soybean solids.Citation[1] Another soymilk manufacturing method with 90% utilization of soybean solids was reported by Hand et al.,Citation[2] which includes bean dehulling, fine grinding of steam-dried beans to make powder, and adding water to the powder to produce soymilk. Another process with no soak water losses of soybean soluble solids was developed by Mustakas and Mayberry,Citation[3] which includes moisture-conditioning of dehulled soybean flakes, and extrusion of bean mixture through an orifice at high temperature, high pressure and short time. The puffed, cooked, and dried material was finely ground to make soymilk by adding water. The Illinois process of producing soymilk utilized all the cotyledon solids except for 10–12% of water-soluble solids in the final product, which utilized all the proteins with high process yield in addition to an environmentally friendly process, which also avoided solids waste.Citation4–6 Even though there were immense advantages in the Illinois process, it is not widely accepted for manufacturing soymilk due to low physical stability and the presence of chalkiness in the soymilk.Citation[6] Chalkiness is a defect and is a sensory attribute of a liquid food, which is related to the sensation of chalk powder in the mouth and throat after the ingestion of the liquid food. This defect in soymilk can be attributed to the presence of fine, grainy particles, which fill the pores in mucous membranes of the mouth after its intake.Citation[5,Citation6] The lack of suspension stability (clear separated of serum layer at the top and opaque layer of settled solids at bottom of soymilk) can be prevented by filtration/centrifugation or by adding stabilizers. A separation index of 1 (means no visible separation) was obtained after five days of quiescent storage for soymilks processed at a homogenization pressure of 34.47 MPa (5000 psi) and for soymilk treated with temperature of 82ºC prior to homogenization at 24.13 MPa (3500 psi).Citation[7]
Thermal treatment utilized in commercial soymilk production gives assurance of food safety and extended shelf life in addition to inactivation of trypsin inhibitors and lipoxygenase (and thus the enzyme-induced off-flavors) which are biologically active compounds present in soy.Citation[5 Citation8–10 The adverse effects of thermal processing were also reported as sensory and nutritional quality losses of soymilk.Citation11–13 Preservation of soymilk characteristics to some extent can be done using 34.47–55.16 MPa (5000–8000 psi) pressurized homogenization of cotyledons with colloid milling and centrifugal clarification to improve mouthfeel and suspension stability.Citation[5,Citation7,Citation14] Soymilk was pasteurized by heating to 85ºC after the two homogenizations at 24.13 MPa (3500 psi) and prior to third homogenization with atmospheric pressure in the first stage and 3.44 MPa (500 psi) in the second stage.Citation[5] The third homogenization was to break clumps formed by heating and the study showed that chalkiness was significantly reduced by heating. By keeping a minimum homogenization, temperature of 82ºC (for one of the two homogenizations) promoted the soymilk stability with formation of lipid-protein complex during processing.Citation[7] Homogenizing twice improved the sensory acceptability of the Illinois soy beverage but homogenizing more than twice added undesirable off-flavors to it.Citation[4,Citation5,Citation15]
The composition of soymilk differs with the variety of soybean used and the processing method.Citation[11,Citation16,Citation17] This variation in solid content and its increase imparted increased viscosity to soymilk.Citation[4,Citation5,Citation18] Viscosity is an important functional property of soymilk and it is of vital importance when considering the commercialization of pressure treated soymilk.Citation[18,Citation19] Addition of hulls increased the viscosity of soymilk and dropped the flow behavior index, which indicated a greater deviation from Newtonian behavior.Citation[15] Son and SinghCitation[20] reported that the power law model was fitted best to the soymilk with different solids content and the effect of temperature on apparent viscosity was expressed using Arrhenius relationship. A product is normally exposed to different shear rates during industrial applications and it becomes vital to know its variation in viscosity with temperature at those shear rates which is required in the design of processing equipment.Citation[21]
Lakshmanan et al.Citation[19] reported that the peptide profiles were not affected though aggregates were observed in the soluble protein fraction in thermal- and pressure-treated samples of soymilk. They also reported increased emulsion stability and decreased hydrophobicity for high-pressure processed soymilk. The complexing of phospholipids and proteins in soymilk aids in preventing settling of suspended solids by reducing density difference between the particles and the serum.Citation[7] This coating of the particle by lipids strengthens the mutual attraction between hydrophilic phosphate groups and water. The effect of heating at 82ºC for 2 min before homogenization at 80 MPa on the rheological properties and microstructure of oil-in-water emulsions containing commercial fraction of soy protein concentrate and soybean oil was reported by Roesch and Corredig.Citation[22] All emulsions displayed a gel-like behavior, stability to creaming and no separation during quiescent storage for 20 days at 4ºC.
Very little information is available on full utilization of dehulled soy cotyledons, non-extracted pressurized soymilk, and its rheological and ultrastructural properties and particle size distribution. Comparison and correlation using experimental data has not been done on the multitude of influencing factors such as total solids, physical and ultrastructural properties, and particle size distribution of thermal-treated and pressurized soymilk, though several studies have reported assumptions of protein-lipid linkage based on data from sensory, stability, and electrophoresis analysis.Citation[4,Citation5,Citation7,Citation19] This study was undertaken with the objective to study the influence of number of passes of pressurized homogenization and preheating on the rheological and ultrastructural properties and particle size distribution of soymilk prepared from whole dehulled soybeans.
MATERIALS AND METHODS
Materials
Soybeans were provided by the Georgia Seed Development Commission, 2420 South Milledge Avenue, Athens, GA, USA. The soybean (Glycine max [L.] Merrill) variety used for this study was Benning, Group VII cultivar, harvested in 2005 from Davisboro, GA, USA. Soybeans were stored in sealed polyethylene-lined bags at 4ºC and 20%R H in the dark throughout the experiments (until they were processed into soymilk) to minimize the changes in composition. Deionized water (DW) was used throughout the experiments to prepare soymilk. Silk® Unsweetened (shelf-stable) soymilk (WhiteWave Foods Company, Broomfield, CO 800021) was purchased from the local grocery store.
Preparation of Soymilk
Soybeans were dehulled by heating at 154.4°C for 5 min on a pan in an Impinger oven (Lincoln Impinger Model 1450, Lincoln Foodservice Products, Inc., Fort Wayne, IN, USA). The tempered soybeans were coarse ground in a plate mill (Quaker City Mill Model 4-E, QCG Systems, LLC, Phoenixville, PA, USA) with the plates set far enough apart to crack the hulls but not to break the cotyledons. The hulls and the cotyledons were separated by air classification. Soymilk was prepared from whole dehulled soybeans by blanching in DW (dehulled soybean: DW was 1:5 w/w) at 60°C for 2½ h. The blanched cotyledons were drained and rinsed 3 times with DW and then adding DW (blanched dehulled soybean: DW was 1:3 w/w) the mixture was ground in a food processor (Robot Coupe Model RSI 10V, Robot Coupe USA, Inc., Jackson, MS, USA) at 2500 rpm for 2½ min. and at 3000 rpm for 2½ min. Then comminution was done in a Fitzpatrick mill (Model JT, The Fitzpatrick Company, Elmhurst, IL, USA) using 0.127 cm and 0.0508 cm screen. The comminuted suspension was homogenized at 96.53 MPa (14,000 psi) in a Gaulin one-stage homogenizer (Model 15MR-8TA, Gaulin, Everett, MA, USA). The process designations for these unboiled soymilks were: one-pass Gaulin homogenization: process A, and two-pass Gaulin homogenization: process B. Two more treatments were done by heating (boiling) the comminuted suspension at 98 ± 2°C for 15 min followed by Gaulin homogenization as described above. The process designations for these boiled soymilks are: one-pass Gaulin homogenization: process C, and two-pass Gaulin homogenization: process D. The whole experiment was triplicated.
Total Solid Content
The experimental soymilk samples and Silk® Unsweetened (shelf-stable) soymilk samples were analyzed for total solids using Halogen Moisture Analyzer (Model HR73, Mettler-Toledo, Inc., Columbus, OH). Three measurements were taken from the triplications. Proximate composition (protein, fat, crude fiber, moisture, ash, and carbohydrate (by difference)) of whole soybean and dehulled blanched bean were done at Feed and Environmental Water Laboratory, The University of Georgia, Athens, GA, USA. Duplicate measurements were taken for each sample.
Rheological Properties
Rheological properties were measured using a dynamic stress controlled rheometer (model SR5000, Rheometric Scientific, Inc., Piscataway, NJ, USA) with concentric cylinder geometry. Apparent viscosity (μapp) was measured using steady state shear test for all the treatments once. The total solid content of boiled suspension-made soymilk was made equal to that of the unboiled samples during analysis of each replication. Apparent viscosity measurements were done at 4, 10, and 25ºC to simulate different storage conditions and Arrhenius equation (EquationEq. [2]) was fitted to predict the apparent viscosity of soymilk at different temperatures. A new sample was used for each measurement. Temperature control at the measurement couette was done with recirculating heating and refrigerating fluid from a water bath (model F25, Julabo USA, Inc., Kutztown, PA, USA) attached to the rheometer. Flow behavior and consistency indices at different temperatures were determined by fitting the power law model (EquationEq. [1]). Time-dependence of the soymilk (for measuring settling during prolonged time period) was measured using transient shear rate ramp test with constant shear rate of 1000 s-1 at 10ºC for a particular time period. The power law model (EquationEq. [1]) was used to describe the non-Newtonian behavior of the soymilkCitation[23]:
where τ = shear stress (Pa); K = consistency coefficient (Pa.sn); γ = rate of shear (s−1); and n = flow behavior index. If n < 1 the fluid shows pseudoplastic behavior and if n > 1 the fluid shows dilatant behavior. For fluids having characteristics that fit in EquationEq. (1), the apparent viscosity (Pa.s), μapp, can be expressed in log transformation as:
The apparent viscosity is dependent on temperature and can be expressed by the Arrhenius equation as given belowCitation[23]:
where μapp stands for apparent viscosity (Pa.s) at absolute temperature T (K); μapp1 stands for apparent viscosity (Pa s) at T1 (K), Ea stands for the activation energy (J/g mol); and R stands for gas constant (8.314 J/g mol K). The EquationEq. (3) can be rearrangedCitation[23] to obtain the following straight line equation:
where A and B are constants and are intercept and slope respectively for the plot of ln(μapp) against (1/T). By equating the EquationEqs. (3) and (4), we get the slope (B) as Ea/R, from which the Ea can be calculated after the model is obtained. Three observations obtained from three replications were used for the analyses.
Particle Size Distribution
Soymilk particle-size distribution was measured using Malvern Laser Particle Size Analyzer, Mastersizer S with 300 mm lens (Malvern Instruments, Southborough, Mass.). Soymilk samples were dispersed in deionized water until an obscuration point (10–20%) was obtained in the diffractometer cell at a pump speed of 2500 rpm. An optical model based on the Mie theory of light scattering by spherical particles was applied to calculate the predicted scattering pattern with the refractive indices of the soymilk and water as follows: real refractive index, 1.47; imaginary refractive index, 0.00; refractive index of water, 1.33. The instrument software calculated the average volume-weighted diameter, D(4,3) = Σnidi 4/Σnidi 3 (where ni is the number of particles in a size class of diameter di), the surface-weighted mean diameter, D(3,2) = Σnidi 3/Σnidi 2, the diameter below which 90% of the volume of particles are found, D(v,0.99), the diameter below which 90% of the volume of particles are found, D(v,0.9), the diameter below which 80% of the volume of particles are found, D(v,0.8), the diameter below which 60% of the volume of particles are found, D(v,0.6), the diameter below which 50% of the volume of particles are found, D(v,0.5), the diameter below which 40% of the volume of particles are found, D(v,0.4), the diameter below which 20% of the volume of particles are found, D(v,0.2), the diameter below which 10% of the volume of particles are found, D(v,0.1), from the size distribution were calculated by the instrument software. The measurement of particle size distribution of Silk Unsweetened (shelf-stable) soymilk was also done in the same way to compare it with the particle size distribution of the experimental soymilk samples. Six measurements from three replications were used for the analyses.
Ultrastructural Properties
Ultrastructural observations were carried out for all treatments at the Center for Ultrastructural Research, University of Georgia, Athens, GA, USA. LEO 982 field emission scanning electron microscope (Zeiss SMT, Inc., Peabody, MA, USA) equipped with an Oxford EDX detector (Oxford Intstruments, Inc., Concord, MA, USA) and a Gatan Alto 2500 cryostage and cryoprep chamber (Gatan, UK; Oxford, UK) was used for examining the structural network of the soymilk. One drop of soymilk was placed on a specimen stub and covered with a stub cap, then rapidly frozen by immersing it in liquid nitrogen (approximately −206°C). After moving to the cryoprep chamber, the sample was fractured with a knife to provide a fresh surface. Residual ice present on the frozen sample was removed by sublimation at −100°C for 5 min, sputter-coated with gold to a thickness of approximately 20 nm, and placed in the sample chamber of the scanning electron microscope (SEM) on a cold stage maintained at −120ºC.
Distribution of fat and protein droplets was also determined with a Leica- TCS SP2 confocal laser scanning microscope (CLSM, Leica Microsystems Inc., Exton, PA, USA) used in reflectance, transmittance and fluorescence mode. Fluorescence dyes specific for lipid globules (lipid soluble Nile red fluorescent dye- NR, Sigma-Aldrich, St. Louis, MO, USA) and protein fraction (fluorescein isothiocyanate – FITC, Sigma-Aldrich, St. Louis, MO, USA) were added to the samples separately. The preparation of the working solutions of dyes was done as described by DamodaraswamyCitation[24]. NR stock solution was prepared with 0.5mg/ml in acetone. From this, a working solution for NR was made by combining 0.10 ml of stock solution to 100 ml of a 75:25 glycerol-water mixture. FITC stock solution was prepared with 0.25% FITC in water. This was further diluted to 1% in water to produce a working solution. The amount of each dye solution and soymilk sample used in the micro slide for analysis were: 25 μL NR, 10 μL FITC, and 25 μL soymilk. The sample was put on a pre-cleaned glass micro slide, stains were added to the sample, and a cover slip was placed over it. CLSM was equipped with a 63x (numerical aperture 1.4), oil immersion objective lens, which provided good control in the z-direction in addition to optical sections in both xy- and xz-directions. Images were taken separately for each dye with a single sample. Excitation for both dyes was by argon/krypton laser with excitation wavelengths of 568 and 488 nm for fat and protein, respectively. Fat globules were pseudo-colored red and protein particles were colored green.
Statistical Analysis
Statistical significance of treatment factors and its interactions were determined according to two-way factorial design with three replications of each treatment using SAS version 9.1 (SAS Inst., Inc., Cary, N.C.). The factors were homogenization temperature and number of passes; each at two levels. Analysis of variance was used to study the effects of treatment factors on response variables (particle size diameter and total solids) quantifying soymilk properties.
Similarly, three-way factorial design with three replications was used to study the statistical significance of treatment factors and the interactions on the rheological properties (apparent viscosity, consistency index, and flow behavior index). The three factors were preheating, number of passes, and measurement temperature. Student's t-test was used further for pair wise comparison between the means of each variable. Silk® Unsweetened (shelf-stable) soymilk was used as a control for statistical comparison with the experiment samples for the response variables of total solids and particle size distribution.
RESULTS AND DISCUSSION
Soymilk processing was done starting from whole bean with proximate composition of 36.62% protein, 19.66% fat, 9.31% crude fiber, 7.76% moisture, 5.53% ash, and 21.12% carbohydrate (by difference). The dehulled blanched beans had proximate analysis of 18.08% protein, 6.17% fat, 5.40% crude fiber, 64.60% moisture, 1.13% ash, and 4.62% carbohydrate (by difference). The process B (unboiled two pass) soymilk had proximate analysis of 3.68% protein, 1.58% fat, 0.26% crude fiber, 92.51% moisture, 0.24% ash, and 1.73% carbohydrate (by difference). The process D (boiled two pass) had proximate analysis of 4.24% protein, 1.78% fat, 0.27% crude fiber, 91.49% moisture, 0.27% ash, and 1.95% carbohydrate (by difference).
Total Solids Content of Soymilk
Total solids content is a key property, which influences other properties such as sensory, physical, and rheological properties as well. Total solids content of the soymilk samples are given in . There was a significant difference (p < 0.0019) in solids content between boiled and unboiled samples. The total solids content of boiled soymilks were 0.79 times greater than that of unboiled soymilks. This difference affected the apparent viscosity of these two samples. This agreed with the results of previous studies done on soymilkCitation[4,Citation5,Citation18,Citation19]. Silk® Unsweetened (shelf-stable) soymilk displayed a similar total solids content of 7.55 ± 0.5% which was not significantly different from unboiled soymilk samples. The results of Kuntz et al.Citation[5] showed that chalkiness is a function of solid content. They reported that the least chalkiness score was obtained for the soymilks with 6–8% solid content. In the present study, the solid content of the unboiled soymilk is near 8% and that of the boiled soymilk was higher solid content due to moisture evaporation during boiling. Priepke et al.Citation[7] reported after testing the total solids top to bottom ratio for soymilks with total solid content 2.39–8.85% that after long term storage, settling is more likely to occur in beverages with lower solid content than with higher solid content. In addition to that, when the extracted total lipid was added back to the soymilk, it gave the same suspension stability (separation index of 1) as the unextracted Illinois soy beverage.
Table 1 Effect of various processes on total solids and flow properties of soymilk
Rheological Characteristics of Soymilk
As expected, the apparent viscosity (μapp) decreased significantly (by a factor of 1.08 as temperature changed from 4 to 10ºC change, and by a factor of 1.43 for 10 to 25ºC temperature change) (p < 0.0001). Silk® Unsweetened (shelf-stable) had an apparent viscosity of 7.38 ± 0.23 mPa.s at 10ºC, which was significantly different from the soymilks obtained from the four processes in this study. Apparent viscosity of soymilk for four treatments is shown in . Apparent viscosity decreased significantly (p < 0.0005) with increase in number of passes (). The results agreed to those obtained by Forster and Ferrier.Citation[15] Analysis of variance showed significant interaction between the factors boiling and number of passes. The interaction plot and t-test revealed that the boiled soymilk had significantly lower apparent viscosity than unboiled soymilk (by a factor of 1.27) when the soymilk was passed once through homogenizer at 96.53 MPa during preparation (p < 0.0001). However, this was not apparent in two-pass homogenization () where the reduction factor was only 1.06. The reduction in μapp may be due to the additional shearing during the second pass, which also decreased the mean particle size. The quick orientation of smaller particles in the direction of shear force might have helped in lowering the apparent viscosity.Citation[15] Similar results were reported by Lakshmanan et al.Citation[19] for soymilk at pH 7 with no significant difference in viscosity between the controls and pressure homogenized soymilks, however thermal-treated soymilks displayed higher viscosity than controls. Puppo et al.Citation[25] explained the differences in soymilk viscosity between the heat- and pressure-treated soymilks as due to the rupture in hydrogen-bonded networks that are affected by heating and not by pressure. Results of Kuntz et al.Citation[5] showed that viscosity did not have any effect on perception of chalkiness in soymilk. This also agreed with results by Nelson et al.Citation[4] that the stability of the soy beverage was not related to its viscosity. They reported a viscosity of 14 mPa.s (at 25ºC for 4.6% protein concentration) for the Illinois soy beverage. Rosenthal and othersCitation[6] reported similar results with enzyme treated soymilk as having the same apparent viscosity (approximately 10 mPa.s, measurement temperature and method not mentioned) as the doubly homogenized (34.47 MPa, 60ºC) soymilk. The highest physical stability and the sensorial attribute “body” of the enzyme treated soymilk were exhibited by the highest apparent viscosity obtained for a particular treatment. Iwuoha and UmunnakweCitation[16] reported higher apparent viscosity of soymilk from blanched wet-dehulled seeds, soymilk from unblanched wet-dehulled, soymilk from toasted dry-dehulled, and soymilk from large flour particles as 38.00, 50.00, 45.00, 45.00 mPa.s respectively for freshly processed soymilks with measurement temperature at 30ºC and moisture contents 91.56, 88.09, 90.51, 91.00% for the respective treatment samples. The rheological characteristics reported earlier were done using single shear rateCitation[5,Citation16]; for a Newtonian fluid the viscosity results obtained using single shear rate are appropriate but it may give deficient or conflicting information on non-Newtonian fluids and its behavior.Citation[15]
Figure 1 Influence of various processes on apparent viscosity of soymilk. Process A (unboiled/one pass) __◊__, R2 = 1.00; process B (unboiled/two pass)__ _ _Δ_ _ __, R2 = 1; process C(boiled/ one pass) _ _ _ _□_ _ _ _, R2 = 0.99; and process D (boiled/two pass)_ _∗_ _, R2 = 0.92.
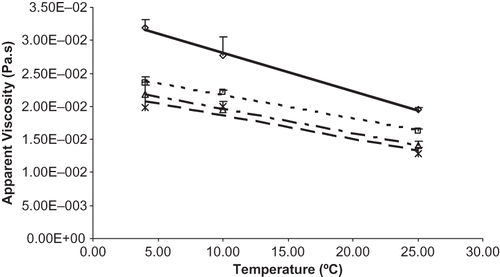
The flow behavior of soymilk was best explained by the power law model EquationEq. (1). All soymilk treatments showed non-Newtonian pseudoplastic behavior. The values of K and n can be substituted in EquationEq. (2) from for defining flow behavior of each soymilk sample. Data by Son and SinghCitation[20] showed similar results for 13% and 16% solids soymilk, although Casson and power law models fitted good for soymilk with 9% solids. Ease of pumping the beverage is the advantage of a pseudoplastic behavior as reduced power will be required to pump the beverage at higher speeds, an advantage in industrial applications.Citation[15,Citation20]
Analysis of variance for the consistency index showed significant effect of measurement temperature (p < 0.0001); however, the number of passes and boiling had significant interaction (p < 0.0046). The difference between consistency indices of boiled and unboiled samples was decreased with increase in number of passes. It was noticed that the number of passes significantly changed the consistency index of both boiled and unboiled products (p-values 0.03 and 0.05). In the viscometric evaluation of Illinois soy beverage, a consistency index of 0.67 Pa sn and flow behavior index of 0.73 was obtained when the product was prepared without hulls and the total solids content was 7%Citation[15]. The consistency index increased exponentially (0.02, 0.14, and 0.70 Pa sn at 25ºC for 9%, 13%, and 16% total solids) with increasing concentration and decreased with increasing temperature (0.02, 0.01, and 0.008 Pa sn at 25, 45, and 65ºC for 9% total solids) for aseptically processed soymilk under turbulent conditions.Citation[20]
Like consistency index the flow behavior index was also affected by the temperature of analysis (p < 0.0060). Increasing the number of passes, significantly decreased the flow behavior index for boiled sample (p < 0.0001), however, this was not evident for unboiled samples. There was no noticeable difference in flow behavior index between boiled and unboiled either at one or two passes. Son and SinghCitation[20] reported decreasing flow behavior index with increasing total solids content (0.77, 0.67, and 0.58 at 25ºC for 9%, 13%, and 16% total solids) and increased with increasing temperature (0.77, 0.77, and 0.77 at 25, 45, and 65ºC for 9% total solids). Flow behavior index (0.82) did not appreciably change with measurement temperature, and it was considered that there was no influence of measurement temperature on the degree of non-Newtonian behavior of soymilkCitation[15]. The values of n agreed with Lakshmanan et al.,Citation[19] who reported a more pronounced pseudoplastic behavior with n values ranging from 0.45 to 0.49 for 1:6 (soybean-to-water ratio) soymilk and 0.56 to 0.57 for 1:8 soymilk at pH 6 for the pressure- and thermal-treated soymilks.
Apparent viscosity changes of the soymilk over time were determined at a constant shear rate (1000 s−1) at 10ºC and the curve obtained is shown in for the soymilks obtained by processes C and D. During the first 200 s apparent viscosity decreased, showing that the soymilk was thixotropic, and it became relatively constant later on when the shear rate was kept constant. Similar results reported earlier explained that the identical curve given by soymilk after a rest period of 900 s with a second analysis using same constant shear rate indicated that shear-induced structural changes on the soymilk were reversible.Citation[6,Citation15] From Priepke et al.Citation[7] a separation index of 1 (means no visible separation) was obtained after 5 days of quiescent storage for soymilks processed at a homogenization pressure of 34.47 MPa (5000 psi). Same result was obtained for soymilks treated with temperature of 82ºC prior to one-pass homogenization at 24.13 MPa (3500 psi) with formation of lipid-protein complex during processing.Citation[7] The thixotropic behavior shown by soymilk may indicate that the beverage will have less tendency to settle, increasing its shelf life and improving mouthfeel by reducing the chalkiness.Citation[15]
Figure 2 Thixotropic behavior of soymilk, at constant shear rate of 1000 s−1 and 10ºC, shows the resistance to settling by keeping constant apparent viscosity after 200 s. Process C (boiled/one pass)_◊_ and process D (boiled/ two pass)_▄_.
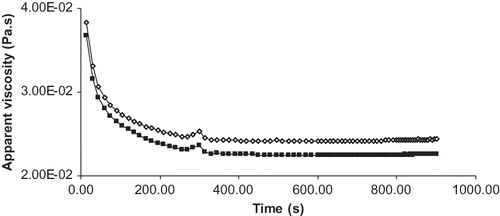
The temperature dependence of the soymilk apparent viscosity was correlated and expressed in terms of the Arrhenius model (). Arrhenius relationship EquationEq. (4) was examined for all treatments and it showed that apparent viscosity decreased exponentially with increase in temperature. This was in agreement with the results of Son and SinghCitation[20] who also reported that the exponential increase in apparent viscosity with increase in concentration at all measurement temperatures indicated protein-protein interaction. The activation energy, Ea, was calculated as 401.47 (0.096), 252.75 (0.060), 244.32 (0.058), and 245.58 (0.059) J/mol (kcal/mol) for processes A, B, C, and D respectively. Son and Singh reported activation energy for soymilks with 9%, 13%, and 16% solid content as 43542.72 (10.40), 29307.60 (7.00), and 28470.24 (6.80) J/mol (kcal/mol), respectively. Though substantiating earlier reports it can be correlated with the present rheological properties results that there seems to be no or little chalkiness and no settling for the process D soymilk; however it is difficult to establish such a correlation that homogenization temperature and pressurized double homogenization reduced the chalkiness and settling of soymilk without testing the sensory and physical properties of soymilk.
Figure 3 Arrhenius model for soymilk showing temperature dependency of apparent viscosity. Process A (unboiled/one pass)___¤_ __, y = 48.29x – 0.14, R2 = 0.99; process B (unboiled/two pass)_ _▄_ _, y = 30.40x – 0.09, R2 = 1; process C (boiled/one pass)__ __Δ__ __, y = 29.39x – 0.09, R2 = 0.99; and process D (boiled/two pass) ____×___, y = 29.54x – 0.09, R2 = 0.91.
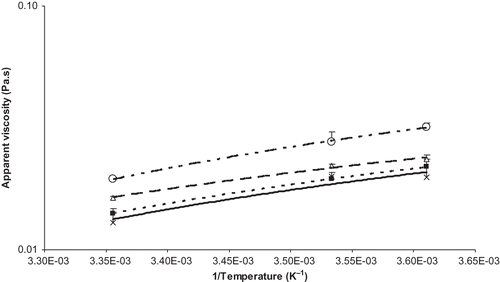
Particle Size Distribution
Particle diameter decreased significantly (p < 0.05) with two passes (). There was no significant difference (p > 0.05) in the particle size between the boiled and unboiled soymilk samples. The soymilk particle size was decreased by a factor of ∼1.50 (, ) in the second homogenization pass. The results of Roesch and CorredigCitation[22] agreed with this and they attributed the presence of large particles in the image taken with integrated light scattering was partly due to large soy fiber structures. From microscopy observations, those fiber particles gave an average size between 50 and 100 μm and their size did not seem to be affected by heat treatment or by high-pressure homogenization. This partly agreed to the results of Kuntz and others,Citation[5] which showed that homogenization temperature (∼82°C) had a significant effect on chalkiness of soymilk which might have resulted from the more extensive hydration and tenderization of the tissue during heating. This high temperature resulted in greater disruption of tissue particles during pressurized homogenization and reduced the particle size, which, in turn, reduced the chalkiness of soymilk. Particle size measurements using Coulter Counter resulted with 80% of particles with size range 3.4–7.3 μm and 10% of the particles above 10 μm in the Illinois soy beverage.Citation[4] They further explained that particles sizes were well above the upper limit of colloidal particles and it was considered to be in the range of unhomogenized milk. The large particle size of the Illinois beverage does not represent a true colloidal system and so the particle size distribution was not the single factor for good stability of the beverage. From previous reports and by comparing our results to particle size distribution of Silk® Unsweetened (shelf-stable) soymilk which is far below [D(4,3) was higher than Silk® Unsweetened (shelf-stable) soymilk by a factor of 12.03, 7.93, 11.83, and 7.91 for processes A, B, C, and D, respectively] the particle size obtained for any of the treatments (). It seems that particle size reduction is of utmost importance to commercialize soymilk processed using any of these treatments. Roesch and CorredigCitation[22] reported a similar result for oil-in-water emulsion with soy protein concentrate (SPC) and soybean oil that after homogenization and/or heat treatment, the suspensions were still characterized by a wide distribution of large particles, but the mean particle size was smaller than that for untreated SPC. In that case, heat treatment alone seemed to produce a similar effect on the particle size distribution as homogenization alone. Our results showed that although the process D produced the smallest particle diameter [D(4,3) ∼29 μm], only 40% of the particles were less than 25 μm. Thus, more research is needed on how to reduce particle size and narrowing the particle size distribution before soymilk from whole soybeans can be successfully commercialized.
Table 2 Effect of different processes on particle size distribution of soymilk
Ultrastructural Properties
Cryo-scanning electron microscope (cryo-SEM) images (, , and ) showed the homogeneity and hydration of the Process D sample (). The preheating treatment before homogenization tenderized and hydrated the particles. All the other processes (image for Process C soymilk is not given) did not impart a uniform structure to the soymilk which was apparent from the aggregates of fat globules in the images () ( and ). Magnification of the images was limited to 10,000 × for all the process samples except for process D that allowed magnification of 100,000 × (image not shown). This reiterates the minute particle size and the homogeneity of particles in process D soymilk. The result is also in agreement with the particle-size distribution results obtained by laser light scattering, which showed that process D soymilk had the smallest particle size among the treatments. The agglomerated particles were not separated by one-pass homogenization. Aggregates were ruptured only by the combined heating and pressurized double homogenization (). Similar results were obtained by Roesch and Corredig,Citation[22] in which the network formed in the heated oil-in water emulsions containing SPC and soybean oil seemed to have a smoother surface than that present in unheated emulsions. They explained that it was due to the enhanced unfolding of the globular protein caused by the high pressure homogenization, the high shear in the presence of higher SPC or/and the heat treatment applied. Wagner et al.Citation[26] explained that hydration in soymilk was due to thermal treatment which causes the proteins to open due to denaturation forming aggregates through disulphide bonds and hydrophobic interactions, which results in higher water binding capacity. Lakshmanan et al.Citation[19] showed that at pH 6 pressurized soymilk exhibited higher water-binding capacity than thermal-treated samples. Previous reportsCitation[4,Citation5,Citation7] assumed that hydrophilic protein-lipid complexes were formed during processing to tenderize the beans and when combined with pressurized homogenization the beverage was stable and had excellent mouthfeel. The microstructure exhibited by the cryo-SEM images, suggests will impart excellent mouthfeel and physical stability of the process D soymilk.
Figure 5 Cryogenic scanning electron microscope image of Process A (one-pass homogenization at 96.53 MPa with no preheating) soymilk, the arrows show that the particles are still coalescing, unhydrated without any pattern of distribution, and large in size.
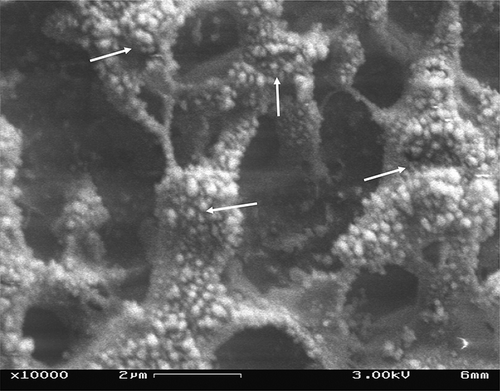
Figure 6 Cryogenic scanning electron microscope image of Process B (double-pass homogenization at 96.53 MPa with no preheating) soymilk, the arrows show that though the particles are separated, they are unhydrated with rough surface and not having uniform particle size. These larger particles may be fat globules coalesced and are not completely absorbed in the protein matrix.
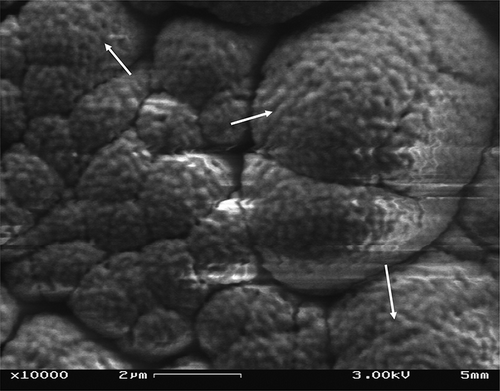
Figure 7 Cryogenic scanning electron microscope image of Process D (double-pass homogenization at 96.53 MPa with homogenization temperature of 98 ± 2ºC) soymilk, shows that the particles are completely separated, hydrated with smooth surface, and having uniform particle size and distribution. The fat and protein particles are difficult to be identified separately. Hydration due to heat treatment and small particle size due to pressurized double homogenization helped in dispersion and uniform arrangement of particles in the fat-protein matrix.
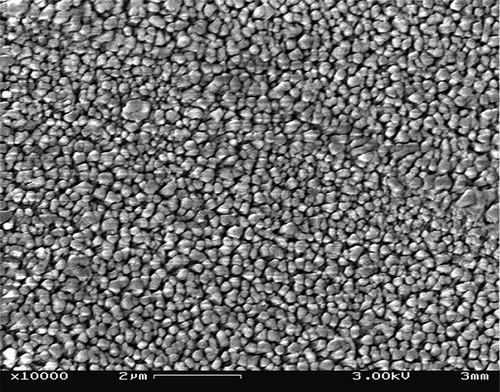
CLSM images obtained showed fat globules in fluorescent red color and protein particles in fluorescent green color. CLSM images ( and ) showed that in the heat-treated samples (image for Process C soymilk not shown; for Process D soymilk), fat globules (red) were distributed more uniformly than that in the other samples. When gelled proteins were adsorbed at the oil/water interface the droplets are protected against creaming, flocculation, and coalescence, while the stability of the emulsion increases.Citation[19] The fat globules seemed to coalesce to form big particle size in the two unboiled samples ( for Process A soymilk; image for Process B soymilk not shown). Protein particles are not visible as separate particles and are not identifiable as they are distributed uniformly in the sample. shows a non-uniform distribution of fat particles and the particles are seen to have coalesced. The non-uniform particle size displayed by cryo-SEM image of process B () and the coalesced structure of process A () along with the results of particle size distribution obtained by laser light scattering collaborate the CLSM images of samples from the respective treatments. Similar result was made by Lopez et al.Citation[27] from the CSLM images of heated curd grains and the particle size obtained by laser light scattering. Process C and D soymilk images showed small sized fat globules (no coalescence) with homogeneous size and uniform distribution. The protein droplets (green) were very small and were difficult to identify due to the very small size it attained and uniform distribution in the image (). Process D gave uniform particle size and uniform distribution of fat globules and protein particles and the fat globules can be seen separately in this image. Thus better attachment of protein at the oil-water interface may have occurred due to protein unfolding and hydrophobic sites were exposed because of pressurized homogenization.Citation[19] The results also agreed to the report by Puppo et al.Citation[28] in which high pressure processing treatment of soy protein isolate, β-7S and A-11S polypeptides displayed increased ability to anchor at the oil-water interface. Similar results were reported by Priepke et al.Citation[7] on Illinois soy beverage in which a protein-lipid film with an almost constant protein-lipid ratio was formed. They further assumed with this evidence that lipid-protein complex was formed in the Illinois soy beverage. Nelson et al.Citation[4] made similar assumptions after testing the composition of freeze-dehydrated soy beverage which resulted in no oil recovery by Soxhlet method and 1.7% oil recovery using sulfuric acid digestion. As the same fat-protein ratio as in soybeans (20:40) was obtained for the soy beverage (1.7:3.1), they assumed that the oil in that beverage was bound by complex chemical formation. CLSM images of mozzarella cheese made from homogenized and unhomogenized milk mixture variationsCitation[29] showed smaller and highly emulsified fat globules embedded in protein matrix for cheese from homogenized milk (image displayed similarity to ), and larger pools of fat within voids in the protein matrix for cheese from homogenized milk and for cheese from 1:1 mixture of the homogenized and unhomogenized milks (images similar to ). This confirms that the fat-protein network was formed in process D soymilk, which was obvious from the CLSM and cryo-SEM images and the particle size distribution. So, in the present study, process D has been confirmed as the best process among the treatments and the soymilk with full essential solids, and excellent rheological ad ultrastructural characteristics can be obtained by this process.
Figure 8 Confocal laser scanning microscope images of Process A (single-pass homogenization at 96.53 MPa with no homogenization temperature) soymilk; fat (seen as light colored small globules) is colored red with Nile red and protein (seen as dark colored background) is colored green with fluorescein isothiocyanate; the arrows show bigger sized fat globules; circles A show the clustering of fat globules; circles B show the spaces where fat globules are not present or are very few. Protein particles are not seen separately as they are distributed uniformly in the sample. This treatment did not give a uniform distribution of particles and the particles are seen coalesced in the image. (Figure available in color online.)
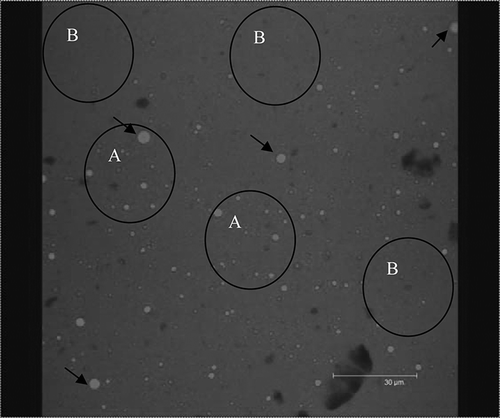
Figure 9 Confocal laser scanning microscope images of Process D (double-pass homogenization at 96.53 MPa with homogenization temperature of 98 ± 2ºC) soymilk; fat (seen as light colored minute globules) is colored red with Nile red and protein (seen as the dark background) is colored green with fluorescein isothiocyanate; there is no clustering of fat globules and they are very small in size. This treatment gives uniform particle size and uniform distribution to fat globules and protein particles and the fat globules are seen separated in the image. (Figures available in color online.)
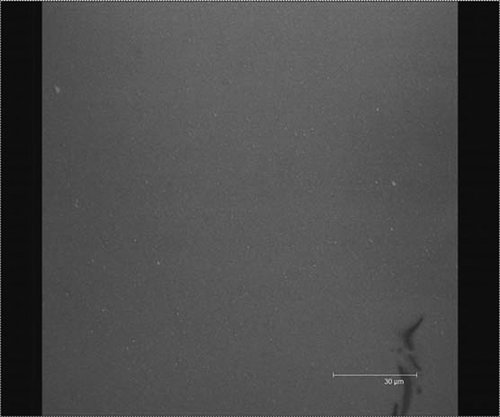
CONCLUSION
Soymilk with all the essential soybean solids were produced by softening of the cotyledons by hydration and cooking, adequate comminution, and heating prior to pressurized homogenization. The product flow properties, ultrastructural properties, and particle size distribution were a function of the processing parameters. Heating the coarsely comminuted slurry prior to homogenization resulted in softening and hydration of soy particles. The size of particles in soymilk is reduced when the homogenization was done twice. Pressurized homogenization helped to decrease the size of solid particles and disperse it uniformly. The ultrastructural images helped immensely to define and verify the structure and particle size distribution obtained for each treatment. The results of the rheological and ultrastructural properties and particle size distribution of soymilk validated that preheating and pressurized double homogenization are very important factors in producing excellent quality soymilk and its commercialization. The results may lead to the use of high-pressure homogenization in the processing of soymilk with whole soybean solids in the milk.
ACKNOWLEDGMENTS
This project was supported by National Research Initiative Grant no. 2005–35503–15374 from the USDA Cooperative State Research, Education and Extension Service NCGP program. This paper was presented in the Institute of Food Technologists 2006 annual meeting at Orlando, FL, USA as a poster. Dr. Diana Hartle, Reference Instruction Librarian, Reference Department, Science Library, The University of Georgia, Athens, GA, USA, for her support with the EndNote reference used in this paper.
REFERENCES
- Lo , W.Y. , Steinkraus , K.H. , Hand , D.B. , Hackler , L.R. and Wilkens , W.F. 1968 . Soaking Soybeans before Extraction as it affects Chemical Composition and Yield of Soymilk . Food Technology , 22 : 1188 – 1190 .
- Hand , D.B. , Steinkraus , K.H. , Van Buren , J.P. , Hackler , L.R. , el Rawi , I. and Pallesan , H.R. 1964 . Pilot Plant Studies on Soymilk . Food Technology , 18 : 139 – 142 .
- Mustakas , G.C. and Mayberry , D.H. 1964 . Simplified Full-Fat Soyflour Process . Food Engineering. , 36 ( 10 ) : 52
- Nelson , A.I. , Steinberg , M.I. and Wei , L.S. 1976 . Illinois Process for Preparation of Soymilk . Journal of Food Science , 41 ( 1 ) : 57 – 61 .
- Kuntz , D.A. , Nelson , A.I. , Steinberg , M.P. and Wei , L.S. 1978 . Control of Chalkiness in Soymilk . Journal of Food Science , 43 ( 4 ) : 1279 – 1283 .
- Rosenthal , A. , Deliza , R. , Cabral , L.M.C. , Cabral , L.C. , Farias , C.A.A. and Domingues , A.M. 2003 . Effect of Enzymatic Treatment and Filtration on Sensory Characteristics and Physical Stability of Soymilk . Food Control , 14 : 187 – 192 .
- Priepke , P.E. , Wei , L.S. , Nelson , A.I. and Steinberg , M.P. 1980 . Suspension Stability of Illinois Soybean Beverage . Journal of Food Science , 45 ( 2 ) : 242 – 245 .
- Wilkens , W.F. , Mattick , L.R. and Hand , D.B. 1967 . Effects of Processing Method on Oxidative Off-Flavors of Soybean Milk . Food Technology , 21 : 1630
- Liener , I. 1981 . Factors Affecting the Nutritional Quality of Soya Products . Journal of the American Oil Chemists’ Society , 58 ( 3 ) : 406 – 415 .
- Kumar , V. , Rani , A. , Tindwani , C. and Jain , M. 2003 . Lipoxygenase Isozymes and Trypsin Inhibitor Activities in Soybean as Influenced by Growing Locations . Food Chemistry , 83 : 79 – 83 .
- Kwok , K.-C. and Niranjan , K. 1995 . Review: Effect of Thermal Processing on Soymilk . International Journal of Food Science & Technology , 30 ( 3 ) : 263 – 295 .
- Chauhan , G.S. , Singh , J.D. and Tomar , N.S. 1998 . Nutritional Changes in Soymilk Subjected to Different Physical and Chemical Treatments . Journal of Food Science and Technology , 35 : 271 – 273 .
- Sancho , F. , Lambert , Y. , Demazeau , G. , Largeteau , A. , Bouvier , J.M. and Narbonne , J.F. 1999 . Effect of Ultra High Hydrostatic Pressure on Hydrosoluble Vitamins . Journal of Food Engineering , 39 : 247 – 253 .
- Mustakas , G.C. and Mayberry , D.H. 1974 . A New Soy Lipid-Protein Concentrate for Beverages . Cereal Science Today , 19 ( 2 ) : 62
- Forster , L.L. and Ferrier , L.K. 1979 . Viscometric Characteristics of Whole Soybean Milk . Journal of Food Science , 44 ( 2 ) : 583 – 585 . 90.
- Iwuoha , C.I. and Umunnakwe , K.E. 1997 . Chemical, Physical and Sensory Characteristics of Soymilk as Affected by Processing Method, Temperature and Duration of Storage . Food Chemistry , 59 : 373 – 379 .
- Mullin , W.J. , Fregeau-Reid , J.A. , Butler , M. , Poysa , V. , Woodrow , L. , Jessop , D.B. and Raymond , D. 2001 . An Interlaboratory Test of a Procedure to Assess Soybean Quality for Soymilk and Tofu Production . Food Research International , 34 : 669 – 677 .
- Cheng , Y. , Shimizu , N. and Kimura , T. 2005 . The Viscoelastic Properties of Soybean Curd (Tofu) as Affected by Soymilk Concentration and Type of Coagulant . International Journal of Food Science and Technology , 40 : 385 – 390 .
- Lakshmanan , R. , de Lamballerie , M. and Jung , S. 2006 . Effect of Soybean-to-Water Ratio and pH on Pressurized Soymilk Properties . Journal of Food Science , 71 ( 9 ) : E384 – E391 .
- Son , S.M. and Singh , R.K. 1998 . Rheological Behavior of Aseptically Processed Soybean Milk under Turbulent Flow Conditions . International Journal of Food Properties , 1 ( 1 ) : 57 – 70 .
- Ditchfield , C. , Tadini , C.C. , Singh , R. and Toledo , R.T. 2004 . Rheological Properties of Banana Puree at High Temperatures . International Journal of Food Properties , 7 ( 3 ) : 571 – 584 .
- Roesch , R.R. and Corredig , M. 2003 . Texture and Microstructure of Emulsions Prepared with Soy Protein Concentrate by High-Pressure Homogenization . Lebensmittel-Wissenschaft und-Technologie , 36 ( 1 ) : 113 – 124 .
- Toledo , R.T. 2007 . “ Flow of Fluids ” . In Fundamentals of Food Process Engineering , Edited by: Toledo , R.T . 183 – 221 . New York : Springer .
- Damodaraswamy , J. 2005 . Rheology and Microstructure of Ready-to-Eat Retorted Egg Product, MS Thesis , Athens, GA : University of Georgia .
- Puppo , C. , Chapleau , N. , Speroni , F. , deLamballerie-Anton , M. , Michel , F. , Añón , C. and Anton , M. 2004 . Physicochemical Modifications of High-Pressure-Treated Soybean Protein Isolates . Journal of Agricultural and Food Chemistry , 52 ( 6 ) : 1564 – 1571 .
- Wagner , J.R. , Sorgentini , D.A. and Anon , M.C. 1992 . Effect of Physical and Chemical Factors on Rheological Behavior of Commercial Soy Protein Isolates: Protein Concentration, Water Imbibing Capacity, Salt Addition, and Thermal Treatment . Journal of Agricultural and Food Chemistry , 40 ( 10 ) : 1930 – 1937 .
- Lopez , C. , Briard-Bion , V. , Camier , B. and Gassi , J.-Y. 2006 . Milk Fat Thermal Properties and Solid Fat Content in Emmental Cheese: A Differential Scanning Calorimetry Study . Journal of Dairy Science , 89 : 2894 – 2910 .
- Puppo , M.C. , Speroni , F. , Chapleau , N. , de Lamballerie , M. and Anon , M.C. 2005 . Anton, M. Effect of High-Pressure Treatment on Emulsifying Properties of Soybean Proteins . Food Hydrocolloids , 19 ( 2 ) : 289 – 296 .
- Rowney , M.K. , Hickey , M.W. , Roupas , P. and Everett , D.W. 2003 . The Effect of Homogenization and Milk Fat Fractions on the Functionality of Mozzarella Cheese . Journal of Dairy Science , 86 ( 3 ) : 712 – 718 .