Abstract
Seeds of different bean types of Phaseolus vulargis display different rates of water imbibition. A processing treatment consisting of tempering and heating was used to improve the rate of water uptake in beans. Chemical properties of unprocessed and processed seed coats were examined. Seeds that readily imbibed water presented lower levels of fatty acids and phenolics in the seed coat. Processing to improve water uptake also caused a decrease in total phenolics content of the seed coats. These results link improved water uptake with decreased lipid and total phenolics content in the seed coat.
INTRODUCTION
The water uptake behavior of seeds belonging to the Leguminoseae family, including Phaseolus vulgaris has been studied extensively in the literature as poor hydration behavior is commonly observed in seeds belonging to this family.Citation[1] Seeds that readily imbibe water are termed “soft” or permeable while poorly hydrating seeds exhibit a barrier to water uptake or slow water uptake.Citation[2] Hydration prior to cooking plays an important role in determining the length of cooking time and quality of processed legumes.Citation[3] The long time required to soak legumes in order to reduce cooking time is one of the negative attributes associated with processing legumes.
The problem of seed permeability has been studied in the literature with respect to the physical, morphological, and chemical characteristics of the seed coat. Published literature indicated that the seed coat is the principle barrier to water uptake.Citation[1] Ross, Arntfield, Cenkowski, Beta, and FulcherCitation[4] have provided a review of the literature on seed permeability, with respect to seed coat structure, from a food science and a plant anatomy perspective. Briefly, work in food science has accounted for physical differences between impermeable and permeable seeds while work in botany has investigated differences between the morphology and anatomy of permeable and impermeable seeds. In the food science literature the importance of seed coat thickness, seed coat to whole seed ratio, and surface area have been cited as being important factors regarding seed coat permeability.Citation[5,Citation6]
Literature from a botanical perspective has implicated the cuticle of the palisade layer as the key factor that determines the permeability of the seed coat.Citation1–9Additionally, there are numerous reports in the literature citing chemical differences in the seed coats of seeds that imbibe water rapidly and those that exhibit delayed water uptake,Citation[1,Citation5,Citation9–11 however no universal explanation has been reported. Phenolics substances may affect water uptake.Citation[10 Citation,11] Wild type pea species with naturally impermeable seed coats possessed high phenolic content while cultivated pea species had a low phenolic content.Citation[11] Sievwright and ShipeCitation[12]noted that phenolics, particularly tannins, are responsible for reduced water uptake, heat penetration, cell separation and deformation characteristics of cooked beans.
The negative aspects of seed coat phenolics in terms of poor water uptake and the anti-nutritional properties have been well documented in the literature; however a diet containing dry beans has been linked to reduced risk of diabetes, cardiovascular disease, obesity, and colon cancer Citation[13] The health promoting qualities of dry beans have been linked to the phenolic compounds present in the seed coat, as these phenolic compounds possess anti-carcinogenic and antioxidant properties.Citation[14] Despite the importance of seed coat phenolics in terms of water uptake properties and antioxidant properties, little is known on how processing to improve water uptake affects these properties.
Food science research has not focused on relating the lipid chemistry of the seed coat and water uptake, however, research in botany and plant science has linked loss of lipids in the seed coat to create permeable seeds.Citation[7] The surface of a legume seed is covered by a hydrophobic cuticle layer, which consists of a complex mixture of lipids including cutin, suberin, and waxes with different fatty acid compositions. Arechavaleta-Medina and SnyderCitation[1] implicated the cuticular layer of the soybean as a key factor in water uptake behavior, noting it was the site of the moisture barrier. Egerton-WarburtonCitation[15] indicated that rapidly hydrating seeds possessed seed coats containing cuticular waxes whose structures had been plasticized and thus possessed an altered hydrophobicity. Shao et al.Citation[2] documented that well hydrating soybean seeds possessed seed coats lacking mid-chain hydroxylated fatty acids while the seed coats from impermeable seeds contained a disproportionately high amount of hydroxylated fatty acids. Zeng et al.Citation[7] hypothesized that the thermal degradation of the lipid component of the seed coats during field growing conditions is primarily responsible for rendering impermeable seeds permeable. The work by Ross et al.Citation[4] demonstrated that the rate of water uptake of whole bean seeds could be increased by subjecting the whole bean seeds to a moist heat treatment. Whether or how the seed coat lipids changed during moist heat processing conditions is unknown.
This work strived to address the chemical changes in the seed coat as affected by processing in relation to water uptake along with identifying chemical differences between bean varieties with different water uptake profiles. The phenolic content including non-tannin and tannin phenolic content, antioxidant activity, and fatty acid content of the seed coat from different beans varieties were determined. Also, the concomitant changes in these components as affected by processing were addressed in relation to water uptake behavior. Characterizing the chemical composition of the seed coat helped to improve understanding of seed coat permeability.
MATERIALS AND METHODS
A portion of the water uptake data cited in this present work was obtained from the work of Ross et al.Citation[4]; however, it is pertinent to briefly describe the samples and methods used for processing both the AC Ole and Galley bean seeds.
Samples
Pinto (AC Ole) and Navy (Galley) beans were obtained from the Agriculture and Agri-Food Canada Station in Morden, Manitoba (crop year 2005). All seed samples were originally stored under ambient conditions.
Processing Regimes for Modifying Water Uptake
AC Ole pinto beans demonstrate a lag and thus delayed water uptake while Galley navy beans exhibit an inherently rapid rate of water uptake. Both the Pinto (AC Ole) and Navy (Galley) beans were processed with the aim of modifying water uptake. Although Galley beans do not exhibit a lag period in their hydration profile, they were processed in order to investigate any chemical changes induced by processing. Based on the work of Ross et al.,Citation[4] a processing regime consisting of cyclic heating and cooling was used to alter water uptake rates of the AC Ole pinto and Galley navy beans. Samples were processed in a tempered state (16% moisture content) and were subjected to cyclic heating and cooling. In the cyclic heating and cooling regime, samples were heated to the desired temperature and held for 30 min in an air convection oven. The samples were removed from the oven and cooled until the surface temperature reached 7°C. This procedure was performed three times in total. AC Ole seeds were heated at both 60 and 160°C. Galley seeds were heated at 60°C. The abrasion treatment used by Ross et al.Citation[4] was used to remove the seed coats from the seeds. Briefly, a plastic jar (12 cm diameter by 14.5 cm height) was lined with sandpaper. Whole seeds and marbles were placed in the jar; the marbles were added to provide weight. The jar containing the whole seeds and marbles was placed on a Ball Mill (Norten, Akron, OH) and rotated for 40 min.
Water Uptake Measurements
Water uptake measurements were performed in the same manner as given by Ross et al.Citation[4] Briefly, after processing, the beans were subjected to water uptake measurements using 10 seeds. The initial mass of the seeds was determined and the seeds were placed at the bottom of a 300 ml beaker. Distilled water was allowed to equilibrate to room temperature (24°C) for 16 h prior to testing. 200 ml of distilled water was added to the seeds. Every 30 min, the seeds were removed from the beaker, blotted on a paper towel to remove surface water, and weighed. Water uptake was calculated as the difference between the starting mass and mass at the selected time points.
Chemical Analysis
Seed coat removal
To perform various chemical analyses, seed coats were removed from the whole seeds. To facilitate seed coat removal, the seeds were frozen with liquid nitrogen. Once the seeds were frozen, a rolling pin was used to crack the seeds. This caused the seed coat to detach from the cotyledon. Forceps were used to separate seed coats from the cotyledon. The seed coats were placed on paper towels and allowed to equilibrate to room temperature. Upon reaching room temperature the seed coats were ground for 30 seconds with a coffee grinder (Persona, Toronto, ON). The ground seed coats were placed in plastic bags and stored at −20°C until chemical analysis. All chemical analyses were performed within one month of seed coat removal.
Extraction
Seed coat (0.05 g) from the native AC Ole and Galley and seeds tempered to 16% moisture content and subjected to heat treatment at 60°C (AC Ole and Galley) or 160°C (AC Ole) was added to 4 mL of 100% methanol. Both unprocessed and processed seed coat material were extracted with methanol at room temperature for two hours on a wrist-action shaker (Burrel, Pittsburgh, PA, USA). The mixture was centrifuged at 1358 × g for 10 min on a table centrifuge (GLC-1, Sorval, Newton, CT, USA) and the supernatant was utilized for determination of the total phenolics and antioxidant activity. An acidified methanol based extraction was performed on the native and thermally processed seed coat material in order to be consistent with determination of total phenolics cited in the literature.Citation[16] In this method, seed coat (0.05 g) from both the native and processed AC Ole and Galley seeds was added to 4 ml of acidified methanol (HCl:Methanol:Water, 1:80:10, v/v) and was further processed in the same manner as the methanol extraction.
Determination of total phenolics
The total phenolic content was determined using the Folin-Ciocalteau method based on the work of Singleton & RossiCitation[17] as modified by Gao et al.Citation[17] It should be noted that dilutions were based on preliminary work to ensure that absorbance readings were within the range of the standard curve utilized for quantification of phenolics. Methanolic extract from the seed coat of both the native AC Ole seeds and AC Ole seeds tempered to 16% moisture content and heated at 60°C was diluted five-fold prior to analysis. The acidified methanol extract from the seed coat of both the native AC Ole seeds and AC Ole seeds tempered to 16% moisture content and subjected to heat treatment at 60°C or 160°C was diluted 10-fold as was the methanolic extract from the AC Ole seeds tempered to 16% moisture content and subjected to heat treatment at 160°C. The methanol and acidified methanol extracts obtained from the seed coat of native and processed Galley seeds were tested without dilution. Depending on the extract either methanol or acidified methanol was used as a blank and ferulic acid (Sigma-Aldrich) was used as standard. The results were expressed on dry basis as mg of ferulic acid equivalents per gram of sample. Measurements of each extract were carried out in duplicate and expressed on a dry weight basis.
Determination of tannins
Tannin content in each sample was determined using insoluble polyvinyl-polypirrolidon (PVPP), which binds tannins as described by Rakic et al.Citation[18] An aliquot (2 mL) of the undiluted methanol and acidified methanol extract from the seed coat of both the native and AC Ole seeds and AC Ole seeds tempered to 16% moisture content and heated at 60°C was added to 100 mg of PVPP (Sigma-Aldrich). An aliquot (2 mL) of five-fold diluted methanol and acidified methanol extract from the seed coat of AC Ole seeds tempered to 16% moisture content and heated at 160°C was added to 100 mg of PVPP. An aliquot (2 mL) of undiluted methanol and acidified methanol extract from the seed coats of native and processed Galley seeds was added to 0.05 g PVPP. After addition of the extract to the PVPP, the mixture was vortexed, left for 15 minutes at 4°C and then centrifuged at 1358 × g for 10 min on a table centrifuge (GLC-1, Sorval, Newton, CT, USA). In the clear supernatant, non-tannin phenolics were determined in the same way as total phenolics. Tannin content was calculated as the difference between total phenolics and non-tannin phenolics. Again, it should be noted that dilutions were based on preliminary work to ensure that absorbance readings were within the range of the standard curve utilized for quantification of phenolics. Also preliminary experiments were performed to optimize the amount of PVPP used to ensure that the total phenolics present were not in excess of the added PVPP.
Antioxidant activity
For measuring the antioxidant activity, a modified version of the method of Brand-Williams, Cuvelier & BersetCitation[19] was used. Methanolic extracts from the seed coat of both the native and AC Ole seeds and AC Ole seeds tempered to 16% moisture content and heated at 60 and 160°C were diluted 10 fold prior to analysis. Undiluted methanol extracts from the seed coat of both native and processed Galley beans seeds were used for analysis. One hundred μL of these extracts was reacted with 3.9 mL of 2,2-diphenyl-1-picrylhydrazyl (DPPH•) solution (6 × 10−5 M in methanol). The decreasing absorbance was monitored at 515 nm with a Biochrom-Novapspec Plus visible light spectrophotometer (Biochrom Ltd., Cambridge UK) in the dark at 0 and 30 minutes. The reference consisted of 100 μL of methanol in 3.9 mL of DPPH• solution. The results were expressed on the basis of a Trolox (6-hydroxy-2,5,7,8-tetramethychroman-2-carboxyllic acid, Sigma-Aldrich) standard curve. Initially, 0.0625 g of Trolox was dissolved in 25 mL of methanol. The stock solution of Trolox was subjected to serial dilutions to generate a standard curve with the range of 0 to 250 μM for the reaction of Trolox and DPPH•. The data was converted to activity in terms of μmoles Trolox equivalents (TE)/100 g of sample, which is in accordance with the method of Miller et al.Citation[20] All antioxidant determinations of each extract were carried out in duplicate and expressed on a dry weight basis.
Lipid extraction
Lipids were extracted from the seed coat using the Soxhlet method as given by Zeng et al.Citation[7] A sample of at least 1 g of ground seed coat was put into a Soxhlet thimble. Samples were extracted for 6 h with 120 mL of hexane. This solvent was evaporated to constant weight using a rotary evaporator and the extracted lipids were weighed. Each sample was extracted in triplicate.
Fatty acid analysis
The lipid samples were converted to fatty acid methyl esters (FAMEs) as indicated by Zeng et al.Citation[7] Each of the lipid samples obtained from the seed coat lipid extracts (10–30 mg) were dissolved in 2 mL of hexane. Then 1.5 mL of methanolic potassium hydroxide (2 M) was added to the lipids of the samples to produce FAMEs. After mixing for 1 min and standing for 15 min, the hexane layer containing the FAMEs was separated and transferred into glass vials. The combined fatty acid methyl ester derivatives were analyzed using a gas chromatograph (Agilent Technologies 6890N GC System) equipped with a flame ionization detector using a DB-Wax (122-7032) high resolution gas chromatography column (30 m × 0.25 mm × 0.25 μm; length, inner diameter, pore size). The column pressure was set at 200 kPa. The injector temperature was set at 250°C and the FID oven temperature was set at 285°C. Samples (5 μL) were injected in splittless mode and simultaneously eluted with the following scheme: the initial oven temperature was 170°C and was held for 2 min, followed by an increase to 220°C (5°C/min), and a 5 min hold at 220°C. The temperature was increased to 230°C at 2°C/min. The final temperature (230°C) was held for 6 min for a total run time of 28 min. Helium and hydrogen were used for the carrier and detector gases, respectively. Compounds were identified on the basis of their co-elution with authentic standards. An 11 component FAME mix (Supelco) was used as the standard. Quantification was based on FID peak areas and final results were expressed as mg fatty acid per gram seed coat on a dry weight basis.
Statistical Analysis
All data were analyzed using analysis of variance (ANOVA) and Tukey mean separation with the Statistical Analysis System (SAS) Program (SAS Institute, Carey, NC). Significance was accepted at p ≤ 0.05.
RESULTS AND DISCUSSION
Effect of Processing on Seed Coat Fatty Acid and Water Uptake
shows the effect of bean type and processing on fatty acid content. Only five fatty acids present in the standard were identified in detectable quantities from the seed coat extracts. a shows a representative chromatogram for the standard FAME mixture and b shows a representative chromatogram for the FAMEs obtain from seed coat. It should be noted here that a peak eluting approximately 6 seconds prior to the oleic acid peak (C18:1 n9-cis) was not present in the FAME standard used for identification and quantification was tentatively identified as elaidic acid (C18:1 n9-trans, the trans isomer of oleic acid) based on the work of Gunstone and Herslof.Citation[21]
Table 1 Effect of processing and bean type on fatty acid content1
Figure 1 (a) Representative chromatogram for the standard FAME mixture, where 1 = hexane (1.036 min); 2 = myristic acid (C14:0, 3.372 min); 3 = palmitic acid (C16:0, 5.311min); 4 = stearic acid (C18:0, 7.891 min); 5 = oleic acid (C18:1 n9-cis, 8.342 min); 6 = linoleic acid (C18:2, 8.938min); 7 = linolenic acid (C18:3, 9.876 min); 8 = archidic acid (C20:0, 10.759 min); 9 = cis-11-eicosenoic acid (C20:1, 11.080 min); 10 = behenic acid (C22:0, 13.944 min); 11 = erucic acid (C22:1, 14.423 min); and 12 = lignoceric acid (C24:0, 18.659 min); and (b) representative chromatogram for the FAMEs obtained from seed coat, where 1 = hexane (1.028 min); 2 = palmitic acid (C16:0, 5.323min); 3 = stearic acid (C18:0, 7.875 min); 4 = elaidic acid (C18:1 n9-trans, 8.199 min); 5 = oleic acid (C18:1 n9-cis, 8.299 min); 6 = linoleic acid (C18:2, 8.926 min); and 7 = linolenic acid (C18:3, 9.924 min).
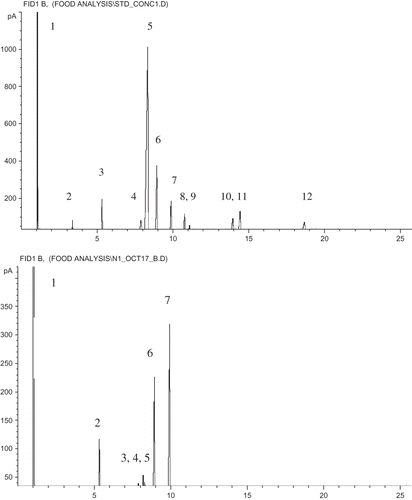
Sasaki et al.Citation[22] characterized the composition of fatty acids in the triglycerols of Adzuki beans, Kidney beans, Scarlet runner beans, along with peas and identified the key fatty acids as: palmitic, stearic, oleic, linoleic, and linolenic acid, which is in agreement with the results of this work. Comparing the fatty acid composition of the unprocessed Pinto bean seed coat (AC Ole: Native) against the fatty acid composition of the unprocessed Navy bean seed coat (Galley: Native), there was significantly more palmitic acid, stearic acid, oleic acid, linoleic acid, and linolenic acid present in the unprocessed AC Ole bean compared to the unprocessed Galley bean. The processed Navy bean (Galley) tempered at 16% and heated at 60°C was the only bean that showed an appreciable amount of myristic acid. The processed Galley bean showed significantly more palmitic acid than the unprocessed Galley bean and was at a level comparable the level present in the pinto bean (AC Ole). Stearic acid was also detected in greatest quantity in the processed Galley bean compared to the other beans. The results seem to show that processing caused an increase in the amount of saturated fatty acids present in the Galley beans.
The work of Seena et al.Citation[23] studied the effect of processing on fat levels in legume seeds and indicated that the amount of crude fat increased when mangrove seeds were treated with dry roasting and pressure cooking. The fatty acid profile of the mangrove seeds depended on the processing methods, which is consistent with our work on beans. Also Gernah et al.Citation[24] indicated that the fat content of African locust beans increased with fermentation processing and attributed the increase in fat content to the release of fat from the carbohydrate matrix as well as the generation of fatty acids due to hydrolysis. Similarly for the Galley seeds, the combination of water and heat may have converted bound lipids to free lipids during processing; however, the same results were not observed for the processed pinto beans (AC Ole) as processing did not have a significant effect on the amounts of saturated fatty acids detected. This implies that seed type and processing affected saturated fatty acid content. Regarding unsaturated fatty acids, the unprocessed Pinto bean (AC Ole-native) showed significantly higher amounts of oleic, linoleic, and linolenic acid relative to the processed Pinto beans and unprocessed and processed Galley beans.
Yoshida et al.Citation[25] determined the content of fatty acids in the triacylglycerols isolated from the seed coat of unprocessed and microwaved soy beans with respect to saturated fatty acids (myristic, palmitic, and stearic) and unsaturated fatty acids (oleic, linoleic, and linolenic). They found a decrease in all of the fatty acids as the time of exposure to microwaves increased. This finding was in partial agreement with our data, as previously discussed, as differences in the amounts of saturated and unsaturated fatty acids present in the seed coat were detected as a result of processing.
Zeng et al.Citation[7] stated that the process of creating permeable seeds during growing conditions includes both physical and chemical changes in the seed coat lipids. Heat provided from the environment during growing conditions likely caused the polymeric structure of the lipids to change through a weakening of the hydrophobic interactions. This would likely make lipids vulnerable to degradation. The presence of water and the application of heat and water likely caused thermal degradation and hydrolysis of the lipids in the seed coat to free fatty acids.Citation[7] Past work of Ross et al.Citation[4] showed that the application of heat in the presence of water improved the water uptake profiles of pinto beans (AC Ole and Pintoba) along with black beans (CDC Jet) while the application of dry heat did not significantly improve water uptake. Hydrolysis of lipids in the seed coat to fatty acids, which requires water, likely contributed to the improved water uptake observed in the tempered and heat treated seeds compared the hydration behavior seen in the unprocessed seeds and seeds subjected to dry heat only. Importantly, this work demonstrates a link of a decrease in lipid content with an increase in water permeability.
It should be noted that in this work the fatty acids identified and quantified from FAMEs, were obtained from hydrolysis of the triglycerides present in the seed coat. Thus the amount of identified fatty acids present in the seed coat decreased and this was associated with a decrease in total lipids. Although the amount of the identified fatty acids derived from the triglyceride lipids present in the seed coat decreased, it is possible that the amount of free fatty acids present in the seed coat increased. The work of Yoshida et al.Citation[25] indicated that the total amount of lipids present in the microwaved soybean seed coats was less than the amount of lipids present in the unprocessed soy bean. While they stated that the fatty acids obtained from the triacylglycerides decreased as microwave heating time increased, they also noted that the amount of lipids components obtained from other sources, which included free fatty acids, increased. Their work did not explicitly measure free fatty acids but indicated that these lipid components along with others were substantially produced in the seed coats during microwave heating. It is likely that there was an increase in free fatty acids present in the seed coats of the processed AC Ole seeds and Galley seeds. These free fatty acids were not esterified with the methanolic potassium hydroxide used to convert the extracted lipid to fatty acid methyl esters as this method does not successfully produce fatty acid methyl esters from free fatty acids if appreciable amount of free fatty acids are.Citation[26 Citation,27]. Future work should include quantification of free fatty acids present in unprocessed and processed bean seed coats.
Curiously, the seed coat of the 16% and 160°C processed AC Ole seeds presented significantly lower levels of oleic, linoleic, and linolenic fatty acid compared to seed coats from the unprocessed and 16% and 60°C processed AC Ole seeds. Based on these results, and the conclusions of Zeng et al.,Citation[7] it might be expected that rapid water uptake behavior would be observed. The water uptake curves for the processed and unprocessed beans examined in this study indicate otherwise. a depicts the water uptake profiles for the unprocessed and processed AC Ole seeds. a shows that the 16% tempered and 160°C processed AC Ole seeds demonstrated poorer water uptake than the unprocessed and 16% 60°C processed AC Ole seeds. The amount of water absorbed at the 8 hour time point was significantly less for the 16% tempered and 160°C heated seeds than the unprocessed and 16% tempered and 60°C heated seeds (p ≤ 0.05). However, it is well known that deterioration of quality in dry beans may result from changes in both the seed coat and/or the cotyledon.Citation[4] Therefore, to identify the cause of the decreased water uptake, seeds were abraded to eliminate the effect of the seed coats as abrasion was shown by Ross et al.Citation[4] to be effective method of removing the effect of seed coat on imbibition. There was no significant difference in the amount of water imbibed by the abraded and non-abraded 16% tempered and 160°C heated seeds at the 8 h time point (p ≤ 0.05). However there was significantly more water absorbed at the 8 h time point by the abraded unprocessed AC Ole seeds than the abraded 16% tempered and 160°C heated AC Ole seeds (p ≤ 0.05). These results imply that the decreased water absorption observed for the 16% tempered and 160°C heated seeds was caused by the cotyledon of the seeds and not the seed coat. Heating the seeds at 160°C impeded water uptake into the cotyledon even though the fatty acid content of the seed coat was decreased. The effect of decreased fatty acid content in the seed coat was masked by the deterioration experienced by the cotyledon caused by heating at 160°C.
Figure 2 (a) Water uptake behavior of unprocessed and processed AC ole seeds; and (b) water uptake behavior of unprocessed and processed galley seeds.
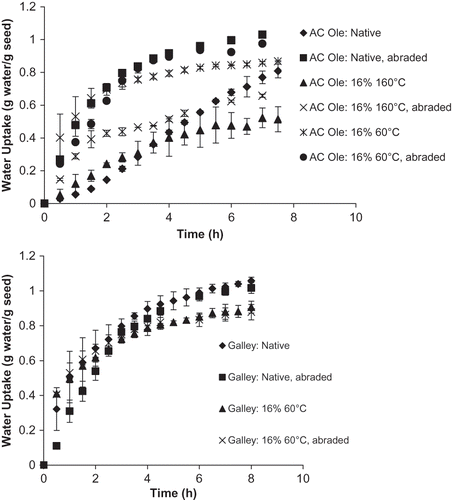
b shows the water uptake behavior of the unprocessed and 16% tempered and 60°C heated Galley seeds in an abraded and non-abraded condition. b shows that there was no significant affect of processing on imbibition as there was no significant difference (p ≤ 0.05) between the amount of water absorbed at the 0.5, 1, 2, 4, and 8 h time points for the unprocessed and processed Galley beans in an abraded state. There were no significant differences in water uptake (p ≤ 0.05) between the unprocessed and processed Galley beans in a non-abraded state at the 0.5, 1, 2, and 4 h time points. There was a significant difference at the 8 h time point. The unprocessed Galley bean showed significantly more water uptake than the processed Galley seed. This result implies that the seed coat has an affect at the 8-h time point. Possibly the increase in saturated fatty acids had a deleterious affect water absorption at prolonged soaking times.
provides further proof that an improvement in water uptake may be linked to a decrease in fatty acid content of the seed coat. shows slopes calculated from the hydration curves for the various seeds. Slopes were calculated from the initial straight line portion of the curve (0 to 2 h time points). These slopes were normalized relative to the amount of water absorbed for each bean type and processing condition at the 8 h time point (i.e., normalized slopemaximum). Additionally, the slopes were normalized relative to the amount of water absorbed for each bean type in their native unprocessed condition at the 8-h time point (i.e., normalized slopecommon). The slopes for the Galley seeds at various processing conditions were generally higher (p ≤ 0.05) than the non-abraded unprocessed AC Ole seeds. This result corresponds with the Galley seeds having lower seed coat fatty acid content than the non-abraded unprocessed AC Ole seeds. The slopes for all of the Galley seeds at various processing conditions were not significantly different (p ≤ 0.05). For the AC Ole seeds, the slopes of the unprocessed non-abraded AC Ole seeds were significantly lower than the slopes of the AC Ole seed processed at different conditions except the 16% tempered 160°C heated non-abraded AC Ole seeds (p ≤ 0.05). Again, the effect of lowered fatty acid content of the seed coat of the 16% tempered 160°C heated AC Ole seeds may be masked by cotyledon effects and this will be further discussed.
Table 2 Effect of processing and bean type on water uptake behavior1
The normalized slopemaximum for the Galley seeds at each processing condition did not show significant differences (p ≤ 0.05) while the non-abraded, unprocessed AC Ole seeds showed a significantly lower normalized slopemaximum than all of the other AC Ole seeds processed at different conditions (p ≤ 0.05). Treating the data in this manner helped to eliminate the effect of cotyledon noted previously for the AC Ole sample tempered to 16% and heated at 160°C and allowed for the improvement in water uptake due to a decrease in fatty acid content of the seed coat to be observed. However, the normalized slopecommon for the non-abraded unprocessed AC Ole seeds is not significantly different than the normalized slopecommon for the 16% tempered 160°C heated seeds yet it is significantly different than the normalized slopecommon for the 16% tempered 60°C heated seeds (p ≤ 0.05). This supports the masking effect of the cotyledon on decreased fatty acid content in seed coat for the sample heated to 160°C.
Effect of Processing on Seed Coat Phenolics and Antioxidant Activity
The total phenolics, non-tannin phenolics, and the tannins of the methanol and acidified methanol extract for the unprocessed and processed AC Ole and Galley seed coats are presented in . The highest yield of total phenolics was obtained from the acidified methanol extracts of the unprocessed and processed AC Ole and Galley seeds. The seed coats of the AC Ole pinto beans showed greater total phenolics than the seed coats of the Galley navy beans. This result was expected as the literature has indicated a direct relationship between seed coat color and total phenolics.Citation[28] Also, seeds that showed lower methanol soluble total phenolics content exhibited faster water uptake when cotyledon affects are taken into account. supports this as the normalized slopemaximum for the non-abraded AC Ole seeds that were tempered to 16% and heated to 160°C was significantly greater than the normalized slopemaximum for the non-abraded unprocessed AC Ole seeds (p ≤ 0.05). Phenolics, particularly tannins have been noted to be responsible for reduced water uptake in beans.Citation[12] Interestingly, tannins are currently being formulated into particle board composites in order to impede water uptake.Citation[29]
Table 3 Effect of processing and bean type on phenolic content1
Extraction solvent also had an effect on the amount of total phenolics extracted as there were significantly more phenolics detected in the acidified methanol extract compared to the methanol extract. Despande and CheryanCitation[30] used both acidified and unacidified methanol to extract phenolics from multiple dry bean varieties and indicated that there was no definite pattern with regard to solvent and extraction of phenolics and bean variety. They indicated that the amount of phenolics detected in the different extraction solvents was dependent upon the bean type. Higher amounts of phenolics present in the acidified methanol extracts may be due to the fact that some phenolics are bound to the grain component and are not readily released. The extraction of such phenolics is likely dependent on an acid catalyzed reaction to release.Citation[30] Additionally, SiddhurajuCitation[31] noted that more phenolics were detected in the methanolic extract of the seed coats of Tamarind than aqueous acetone extracts. The poorer extractability observed in the aqueous acetone extracts may have been caused by the presence of insoluble phenolic-protein and/or phenolic-carbohydrate compounds. The bean seed coats in the present study may have contained insoluble phenolic-protein and/or phenolic-carbohydrate and the acidified methanol extraction solvent was more effective in releasing these phenolics compared to methanol. Thus, the acidified methanol extracts exhibited more total phenolics than the methanol extracts.
Regarding the methanol extracts, processing decreased the amount of total phenolics present in the extract for the AC Ole pinto Galley beans. SiddhurajuCitation[32] also noted that more total phenolics were found in unprocessed moth bean seed extracts compared to dry heated (processed) moth bean seed extracts. Alternatively, the acidified methanol extracts for both the processed and unprocessed seed coats from Galley beans were not significantly different (p ≤ 0.05). The acidified methanol extracts from the unprocessed AC Ole seed coats and the 16% tempered and 60°C processed AC Ole seed coats were not significantly different from each other but showed significantly more total phenolics than the 16% tempered 160°C processed AC Ole seed coats (p ≤ 0.05).
In general, the methanol and acidified methanol extracts from AC Ole were primarily tannins and the extracts from Galley were non-tannins. The exception to this was AC Ole heated to 160°C where the non-tannin levels were higher. This result was difficult to interpret, however it is believed that under the high temperature treatment of 160°C, most of the tannins present in the seed coat of the 16% tempered AC Ole beans were oxidized or became complexed with other seed components such as proteins. Deshpande and CheryanCitation[30] attributed a loss in assayable tannins to oxidation or polymerization. Sievwright and ShipeCitation[12] also indicated that both polymerization of tannins and their binding to proteins would reduce the measurable amount of tannins. It is likely that the tannins present in the 16% tempered and 160°C heated AC Ole seed coats were polymerized and/or were bound to proteins in the seed coat possibly due to the Maillard reaction, which is promoted at temperatures above 60°C.Citation[33] These tannins were therefore unavailable to bind to PVPP. In the PVPP assay, tannin content was calculated as the difference between total and non-tannin phenolics content. Thus, if the tannins present were unable to be measured by the assay, this would be manifested as an artificially high non-tannin phenolic content. It is possible that this artifact was not as pronounced in the acidified methanol extracts as the acid may have released the bound tannins present in the seed coat via acid hydrolysis.
The free radical scavenging activities of the methanol extracts of the unprocessed and processed AC Ole and Galley bean seed coats were determined with the DPPH• free radical assay, and the results are shown in . There was significantly more (p ≤ 0.05) antioxidant activity associated with the unprocessed and processed seed coats of the AC Ole seed coats compared to the unprocessed and processed Galley seed coats. This trend is in agreement with the total phenolics data and literature has shown a correlation between total phenolics and antioxidant activity.Citation[34,Citation35] There is significantly more antioxidant activity associated with the unprocessed AC Ole seed coats compared against the 16% tempered and 60°C and 160°C heated AC Ole seed coats, which were not significantly different from each other (p ≤ 0.05). These results are also in agreement with the methanol extract total phenolics data observed for the methanol extracts. The antioxidant results further prove that the higher non-tannin phenolics values observed for the 16% tempered and 160°C heated AC Ole methanolic seed coat extracts was artificially inflated due to the enhanced polylmerization of tannins preventing an accurate determination of tannins using PVPP. Antiradical scavenging activity is related to the substitution of the hydroxyl groups of the aromatic rings in the phenolic compounds and therefore would contribute to hydrogen donating ability.Citation[31] If the high non-tannin phenolics values associated with the 16% tempered 160°C heated methanolic AC Ole seed coats extracts was not an artifact of the tannin measuring assay, the antioxidant activities of the methanol extracts of 16% tempered and 60°C and 160°C heated AC Ole seed coats would likely be significantly different.
Table 4 Effect of processing and bean type on antioxidant activity1
CONCLUSIONS
Evidence from the present study has implicated the importance of the fatty acid content of the seed coat on water uptake behavior of legume seeds. Navy beans (Galley variety) possess a naturally rapid water uptake profile compared to pinto beans (AC Ole variety). Galley navy beans contain lower levels of unsaturated fatty acids than AC Ole pinto beans. Processing of the AC Ole pinto beans caused a reduction in fatty acid content of seed coat of these beans. These beans, when cotyledon effects were accounted for, exhibited an improved water uptake profile. The results from this work imply that there is a causal relationship between fatty acid content of the seed coat and water uptake behavior, which has not yet been reported in food science literature. Extraction solvent had an effect on the amount of phenolics measured and the different bean types also showed different phenolics content. The acidified methanol extracts contained more total phenolics than the methanolic extracts for all of the unprocessed and processed bean seed coats. Seed coats of the navy bean variety Galley had lower levels of total phenolics and tannins than the seed coats of the pinto bean variety AC Ole. Different processing conditions created differences in structure between bean seed coat tannins that could affect their quantification. The seed coats from the 16% tempered and 60°C heated AC Ole seeds demonstrated a 24 and 1% decrease in total phenolics content in methanolic and acidified methanol extracts, respectively. The seed coats from the 16% tempered and 60°C heated AC Ole seeds showed 40% decrease in antioxidant activity compared to that of the unprocessed AC Ole seeds. Antioxidant activity was positively correlated to total phenolics content. Seeds that showed lower methanol soluble total phenolics content and lower antioxidant activity exhibited faster water uptake. In all, the results from this work indicate that a simple moist heat treatment can be used to improve the water uptake in beans through altering the fatty acid content and total phenolics content of the seed coat. Although the link between fatty acid content of the seed coat and water uptake behavior has not yet been reported in food science literature, based on this work, it is difficult to state whether fatty acid content or total phenolic content affects water uptake more. Future work should aspire to determine the relative importance of fatty acid content and total phenolic content on water uptake.
REFERENCES
- Arechavaleta-Medina , F. and Snyder , H.E. 1981 . Water Imbibition by Normal and Hard Soybeans . J. Am. Oil Chem. Soc. , 58 ( 11 ) : 976 – 979 .
- Shao , S. , Meyer , C.J. , Ma , F. , Peterson , C.A. and Bernards , M.A. 2007 . The Outermost Cuticle of Soybean Seeds: Chemical Composition and Function During Imbibition . J. Exp. Bot. , 58 ( 5 ) : 1071 – 1082 .
- Abu-Ghannam , N. and McKenna , B. 1997 . The Application of Peleg's Equation to Model Water Absorption During the Soaking of Red Kidney Beans (Phaseolus vulgaris L.) . J. Food Eng. , 32 : 391 – 401 .
- Ross , K.A. , Arntfield , S.D. , Beta , T. , Cenkowski , S. and Fulcher , R.G. 2008 . Understanding and Modifying Water Uptake of Seed Coats Through Characterizing the Glass Transition. Int . J. Food Prop. , 11 ( 3 ) : 544 – 560 .
- Reyes-Moreno , C. , Carabez-Trejo , A. , Paredes-Lopez , O. and Ordorica-Falomir , C. 1994 . Physicochemical and Structural Properties of Two Bean Varieties which Differ in Cooking Time and the HTC Characteristic . Lebensm-Wiss. u-Technol. , 27 : 331 – 336 .
- Sefa-Dedeh , S. and Stanley , D.W. 1979 . The Relationship of Microstructure of Cowpeas to Water Absorption and Dehulling Properties . Cereal Chem. , 56 ( 4 ) : 379 – 386 .
- Zeng , L.W. , Cocks , P.S. , Kailis , S.G. and Kuo , J. 2005 . The Role of Fractures and Lipids in the Seed Coat in the Loss of Hardseededness of Six Mediterranean Legume Species . J. Agr. Sci. , 143 : 43 – 55 .
- Ma , F. , Cholewa , E. , Mohamed , T. , Peterson , C.A. and Gijzen , M. 2004 . Cracks in the Palisade Cuticle of Soybean Seed Coats Correlate with their Permeability to Water . Ann. Bot. , 94 : 213 – 228 .
- Rangaswamy , N.S. and Nandakumar , L. 1985 . Correlative Studies on Seed Coat Structure, Chemical Composition, and Impermeability in the Legume Rhynchosia minima . Bot. Gaz. , 146 ( 4 ) : 501 – 509 .
- Marbach , I.. and Mayer , A.M. 1975 . Changes in Catechol Oxidase and Permeability to Water in Seed Coats of Pisum elatius during Seed Development and Maturation . Plant Physiol. , 56 : 93 – 96 .
- Marbach , I. and Mayer , A.M. 1974 . Permeability of Seed Coats to Water as Related to Drying Conditions and Metabolism of Phenolics . Plant Physiol. , 54 : 817 – 820 .
- Sievwright , C.A. and Shipe , W.F. 1986 . Effect of Storage Conditions and Chemical Treatments on Firmness, In Vitro Protein Digestibility, Condensed Tannins, Phytic Acid and Divalent Cations of Cooked Black Beans (Phaseolus vulgaris) . J Food Sci. , 51 ( 4 ) : 982 – 987 . 1986
- Rocha-Guzman , N.E. , Gonzales-Laredo , R.F. , Ibarra-Perez , F.J. , Nava-Berumen , C.A and Gallegos-Infante , J. 2007 . Effect of pressure cooking on the antioxidant activity of extracts from three common bean (Phaseolus vulgaris L.) cultivars . Food Chem. , 100 : 31 – 35 .
- Oomah , B.D. , Cardador-Martinez , A. and Loarca-Pina , G. 2005 . Phenolics and Antioxidant Activities in Common Beans (Phaseolus vulgaris L.) . J. Sci. Food Agr. , 85 : 935 – 942 . 2005
- Egerton-Warburton , L.M. 1998 . A Smoke Induced Alteration of the Sub-Testa Cuticle in Seed of the Post Fire Recruiter, Emmenanthe penduliflora Benth. (Hydrophyllaceae) . J. Exp. Bot. , 49 ( 325 ) : 1317 – 1327 .
- Gao , L. , Wang , S. , Oomah , B. D. and Mazza , G. 2002 . “ Wheat quality: Antioxidant Activity of Wheat Millstreams ” . In Wheat Quality Elucidation , Edited by: Ng , P. and Wrigley , C. W. 219 – 233 . St. Paul, MN : AACC International .
- Singleton , V. L. and Rossi , J. A. 1965 . Colorimetry of Total Phenolics with Phosphomolybdic-phosphotungistic Acid Reagents. Amer . J. Enol. Viticult. , 16 : 144 – 158 .
- Rakic , S. , Petrovic , S. , Kukic , J. , Jadranin , M. , Tesevic , V. , Povrenovic , D. and Siler-Marinkovic , S. 2007 . Influence of Thermal Treatment on Phenolic Compounds and Antioxidant Properties of Oak Acorns from Serbia . Food Chem. , 104 : 830 – 834 .
- Brand-Williams , W. , Cuvelier , M. E. and Berset , C. 1995 . Use of a Free Radical Method to Evaluate Antioxidant Activity . Lebensm-Wiss. u-Technol. , 28 : 25 – 30 .
- Miller , H.E. , Rigelhof , F. , Marquart , L. , Prakash , A. and Kanter , M. 2000 . Antioxidant Content of Whole Grain Breakfast Cereals, Fruits, and Vegetables . J. Amer. Coll. Nutr. , 19 ( 3 ) : 312S – 319S .
- Gunstone , F.D. and Herslof , B.G. 2004 . Lipid Glossary 2 , 152 Bridgewater, , England : The Oily Press .
- Sasaki , S. , Oshnishi , M. , Mano , Y. , Komjima , M. , Ito , S. and Fujino , Y. 1989 . Characterization of Triaglycerol Species in Oil-Poor Beans. Agr. Biol . Chem. Tokyo , 53 ( 7 ) : 2021 – 2023 .
- Seena , S. , Sridhar , K.R. , Arun , A.B. and Young , C.C. 2006 . Effect of Roasting and Pressure Cooking on Nutritional and Protein Quality of Seed of Mangrove Legume Canavalia cathartica from Southwest Coast of India . J. Food Compos. Anal. , 19 : 284 – 293 . 2006
- Gernah , D.I. , Inyang , C.U. and Ezeora , N.L. 2007 . Incubation and Fermentation of African Locust Beans (Parkia biglobosa) in Production of “Dawadawa.” . J. Food Process. Pres. , 31 : 227 – 239 .
- Yoshida , H. , Takagi , S. and Hirakawa , Y. 2000 . Molecular Species of Triacylglycerols in the Seed Coats of Soybeans (Glycine max L.) Following Microwave Treatment . Food Chem. , 70 : 63 – 69 .
- Martinez-Castro , I.. , Alonsi , L. and Juarez , M. 1986 . Gas Chromatographic Analysis of Free Fatty Acids and Glycerides of Milk Fat using Tetramethylammonium Hydroxide as Catalyst . Chromatographia. , 21 ( 1 ) : 37 – 40 . 1986
- Christopherson , S.W. and Glass , R.L. 1969 . Preparation of Milk Fat Methyl Esters by Alcoholysis in an Essentially Non-Alcoholic Solution . J. Dairy Sci. , 52 ( 8 ) : 1289 – 1290 . 1969
- Barampama , L. and Simard , P. 1993 . Oligosaccharides, Antinutritional Factors and Protein Digestibility of Dry Beans as Affected by Processing . J. Food Sci. , 59 ( 5 ) : 833 – 837 .
- Bisanda , E.T.N. , Ogola , W.O. and Tesha , J.V. 2003 . Characterization of Tannin Resin Blends for Particle Board Applications . Cement Concrete Comp. , 25 : 593 – 598 . 2003
- Despande , S.S. and Cheryan , M. 1985 . Evaluation of Vanillin Assay for Tannin Analysis of Dry Beans . J. Food Sci. , 50 : 905 – 910 .
- Siddhuraju , P. 2007 . Antioxidant Activity of Polyphenolic Compounds Extracted From Defatted Raw and Dry Heated Tamarindus Indica Seed Coat. Lebensm-Wiss . u-Technol. , 40 : 982 – 990 .
- Siddhuraju , P. 2006 . The Antioxidant Activity and Free Radical Scavenging Capacity of Phenolics of Raw and Dry Heated Moth Bean (Vigna aconitifolia) (Jacq.) Marechal Seed Extracts . Food Chem. , 99 : 149 – 157 .
- Pagani , M.A. , Resmini , P. and Pellegrino , L. 1992 . Technological Parameters Affecting the Maillard Reaction in Pasta Processing . Tecnica Molitoria. , 43 : 577 – 592 .
- Mandic , A.I. , Dilas , S.M. , Letkovic , G.S. , Jasna , M. , Canadanovic-Brunet , J.M. and Tumbas , V.T. 2008 . Polyphenolic Composition and Antioxidant Activities of Grape Seed Extract . Int. J. Food Prop. , 11 ( 4 ) : 713 – 726 .
- Cano , A. and Arnao , M. B. 2005 . Hydrophilic and Lipophilic Antioxidant Activity in Different Leaves of Three Lettuce Varieties . Int. J. Food Prop. , 8 ( 3 ) : 521 – 528 .