Abstract
Three insoluble dietary fibers (cellulose, wheat, and oat) were incorporated into an extruded-expanded product containing 32% whey protein at three concentrations (18, 36, and 48%) to determine the influence of fiber on extrudate properties. As the concentration of fiber in the extrudates increased, there was a significant decrease in expansion ratio, air cell size, water soluble carbohydrate, and water solubility index, and a significant increase in extrudate density, breaking force, moisture content, and water absorption index. The main effects observed were attributed primarily to a decrease in the amount of normal cornstarch.
Keywords:
INTRODUCTION
Extrusion processing is used for the production of cereals, snacks and other human and animal foods. The incorporation of whey protein into extruded-expanded products has gained popularity due to the possibility of producing a high-protein snack food that will fit into the functional food category.[Citation1–8] The appeal of functional foods is in the ability of a food product to provide health benefits beyond basic nutrition.[Citation9] Dairy whey protein and plant fiber are very nutritious and relatively inexpensive functional food ingredients that are incorporated into a variety of nutritious snack foods.
Dietary fiber and whey products, such as whey protein concentrate (WPC), have been incorporated into extruded-expanded snack products with varying levels of success. The main disadvantages inherent with whey addition are reduced cross-sectional expansion, increased hardness, heightened non-enzymatic browning, and difficulty in extrusion.[Citation1,Citation2,Citation6,Citation10] Recently, Allen et al.[Citation1] extruded WPC (80% protein) at varying protein levels with different types of starches. It was found that starch type (normal cornstarch or waxy cornstarch) had the greatest impact on extrudate physical properties. The use of waxy cornstarch at whey protein concentrations from 32–40% yielded extrudates with greater expansion and lower bulk densities than those with normal cornstarch.
High fiber concentrations have been shown to decrease expansion and increase density and breaking force[Citation11–14] while low fiber concentrations (10–12%) have been shown to improve extrudate expansion.[Citation3,Citation15] Jin et al.[Citation16] contributed reduced radial expansion to a thickening of the air cell walls. The purity of fiber directly influences expansion properties.[Citation17] Fibers with the lowest level of protein and lipid in conjunction with smallest particle size allow for the highest degree of expansion.[Citation17] Plant fibers can contain cellulose, hemicelluose, pectic substances, and lignin. Previous research has shown the hemicellulose and pectic fractions can be solubilized during extrusion.[Citation18–20]
Whey protein and dietary fiber both tend to have detrimental effects on extrudate properties such as decreased expansion ratio, air cell size, and increased hardness and density. The possibility to extrude both dietary fiber and WPC in a snack food exists. The success or acceptability of the extrudate will depend on the ability to manipulate extrusion conditions, product formulation, and the intended use of the extrudate. This study investigated the influence of fiber type (cellulose, wheat, and oat) and fiber concentration (18, 36, and 48% wt/wt) on the chemical and physical properties of extruded-expanded products containing 32% whey protein.
MATERIALS AND METHODS
Sample Preparation and Fiber Characterization
An extruded-expanded product was produced containing 32% total protein from whey WPC containing 80% protein (WPC80) (Grande Custom Ingredients, Lomira, WI, containing 80% protein, 4% water, 5% fat, 3.5% lactose, and 4.5% minerals), 50% normal cornstarch (Argo, Bedford Park, Ill, containing 75% amylopectin and 25% amylose), and 10% pregelatinized waxy cornstarch (National Starch and Chemical, Bridgewater, NJ) as the standard control containing 0% total dietary fiber (TDF). Initially, four sources of soluble fiber and four sources of insoluble fiber were screened at a concentration of 18% for suitability in an extruded-expanded product. Selection of fibers was based on ease of extrusion (absence of surging or clogging extruder) and visible radial expansion. The three fibers selected were Vitacel Powdered Cellulose L601 FCC, Vitacel Oat fiber HF600, and Vitacel Wheat Fiber WF600 (all from J. Rettenmaier USA LP, Schoolcraft, MI) containing 97 (95.5 % insoluble), 96 (>90% insoluble) and 97% (94.5% insoluble) TDF.
The water and 0.1M NaOH fiber soluble fractions were determined on 1 gram of each fiber in triplicate using a modification of the method described by Kuan and Liong.[Citation21] The fibers were dissolved in 40 ml water and 10 ml ethanol and incubated at 60°C for 1 h. Samples were filtered (Whatman glass microfiber filters) and rinsed with 15 ml ethanol, 15 ml acetone and 15 ml water sequentially. The filtrate and filter were dried for 12 h at 60°C and weighed. The amount of fiber soluble in water and 0.1 M NaOH was determined by weight difference.
Fibers were separately blended with the normal cornstarch, pregelatinized waxy cornstarch and WPC80 to form final %TDF concentrations of 18, 36, and 48% (wt/wt) (). Adjustments were made in the normal cornstarch concentration to account for different %TDF content of each fiber sample. Treatments were extruded in block form with each fiber type representing one block. Each fiber concentration was extruded in random order within each block. Each fiber type, fiber concentration and control was extruded in triplicate yielding 10 treatments (three fiber types, three fiber concentrations, and one control in triplicate) for a total of 30 samples. All samples were extruded as described below.
Table 1 Composition of dry blends used for extrusion at three different fiber concentrations
Extrusion
Samples were extruded employing a bench-top scale APV Baker MP-19TC twin-screw extruder (APV Maker, Inc., Grand Rapids, MI). Dry feed was added to the extruder and mixed with liquid feed (0.1 M NaOH) in the barrel.[Citation1,Citation22] Liquid feed rate was held constant at 5.6 g/min. Temperature zones along the barrel were controlled and monitored with CAL3200 Autotune temperature controllers (Cal Controllers, Inc., Libertyville, IL). The five barrel temperature zones (25, 25,115, 125, 135° C) were set for all extrusion runs with the fifth zone, 135°C, as the die exit. Other independent extrusion conditions of dry feed rate and screw speed were initially set at 500 rpm for dry feed rate, and 200 rpm for screw speed. The exit die was conical with a 2.5 mm diameter. Independent extrusion parameters of dry feed rate and screw speed were determined for each fiber concentration and used to optimize product extrusion based on a constant liquid feed rate of 5.6 g/min. All set and observed independent extrusion parameters were recorded. Food coloring was added to the barrel at the dry feed inlet for each treatment run and the length of time for the dye to exit was used to determine residence time. Four, 20-second samples randomly collected during each extrusion run were weighed to determine product flow rate. The extruder was cleaned with water between extrusion runs. Specific mechanical energy was calculated in as described by Grenus et al.[Citation15] using 2 kW as the motor power of the MPF-19 extruder.
Physical Properties
All physical properties were determined on extrudates dried for 16 h at 70°C. The average air cell size was determined from five extrudates from each treatment embedded in melted household wax and digitally photographed. The air cell surface area was determined with the Magnetic Lasso tool in Adobe Photoshop (Adobe Systems, Inc., CA) generating a total pixel count within the outlined circumference as described previously.[Citation1] Pixel counts of standard paper squares were used to make a standard curve. Total pixel counts of the air cells were compared to the standard curve to determine air cell size.
Breaking force and density were calculated from shear (Salter 235 device with a Warner Bratzler shear cell, GR Electric Manufacturing, KS), length, diameter and weight of 10 extrudates in each treatment as previously reported.[Citation1] The ratio of the cross sectional area of each extrudate to the area of the die exit was used for expansion ratio calculations.
Chemical Properties
The moisture content of extrudates was determined as the percent weight difference on extrudates immediately after extrusion and after drying for 16 h at 70°C. The water adsorption index (WAI) and water solubility index (WSI) were determined according to Jin et al.[Citation16] with modifications described by Taylor et al.[Citation22] and Allen et al.[Citation1] WAI values were reported as grams of water absorbed per 100 grams sample. WSI values are reported as grams of sample solubilized per 100 grams sample. WSI and WAI analysis were done in triplicate for each replicate. Extrudate water soluble protein, total soluble protein (1% w/v sodium dodecyl sulfate and 1% v/v beta-mercaptoethanol) and water soluble carbohydrate were determined as previously described.[Citation1,Citation23]
Statistical Analysis
Treatment means for physical and chemical properties were calculated and analyzed using the proc glm function in Statistical Analysis Software (SAS) version 9.0 (SAS Institute, Inc., Cary, NC). Analysis of variance (ANOVA) was used to compare treatment means and identify statistically significant differences at the 95% confidence level. Fiber concentration, fiber type and fiber type/fiber concentration interactions were set as fixed factors and were analyzed based on ANOVA results.
RESULTS AND DISCUSSION
Extrusion and Fiber Characteristics
Screening of soluble and insoluble fibers resulted in three insoluble fiber sources that were extruded into expanded products. The soluble fibers tested did not result in extrudate products because of seizing and clogging in the extruder that may be related to the thickening and gelling effects observed with soluble fibers. Previous research on the effects of fiber on extruded-expanded products focused on the use of insoluble fiber sources,[Citation1,Citation15,Citation16] although the use of ingredients (barley or okara) containing both soluble and insoluble fiber have also been researched.[Citation11,Citation14]
Each fiber concentration (oat, wheat, and cellulose) used had a unique extrusion condition varying only slightly among fiber types (). Liquid feed was held constant at 5.6 g/min for each extrusion run and optimized extrusion was obtained for each fiber concentration defined as an absence of product surging, indicating a state of equilibrium, and minimal elastic recoil (spring back after stretching) of exiting extrudate. The specific mechanical energy (SME) increased with an increase in fiber compared to the control, which may be due to an increase in viscosity in samples with more fiber. The decrease in die pressure was presumably due to the decrease in dry feed and product flow rates.
Table 2 Extrusion parameter ranges
All fibers contained predominantly insoluble fiber (>90% insoluble) with the oat and wheat fibers containing 26% hemicellulose and 74% cellulose. The cellulose and wheat fibers used had similar water retention capacity, 1100% and 1050% respectively, while the oat fiber had a lower water retention capacity (800%). The fibers had similar bulk densities (range of 166–280 g/L) and particle sizes (15–60% > 32 microns, 3% > 100 microns) (J. Rettenmaier product information sheets).
The water and base (0.1 M NaOH) soluble fractions of the fibers were tested. The amount of fiber soluble in water was 1.5, 0.8, and 0.5% and the amount soluble in base was 20.2, 19.6, and 1% for oat, wheat and cellulose respectively. Fiber is defined as a group of polysaccharides that are resistant to digestion by humans. The primary components of fiber include cellulose, hemicellulose, pectins, lignins, gums, and mucilages. The oat and wheat fibers in this study contained hemicellulose, which, unlike cellulose, is not crystalline, consists of short chain length sugars, is branched, and is more susceptible to dextrinization in the presence of dilute acids, bases and during extrusion conditions of high shear and temperature.[Citation17,Citation18,Citation19,Citation20] The fiber fraction solubilized with base in the oat and wheat fibers was presumably the hemicellulose fraction.
Physical Properties
Extrudate properties exhibited significant statistical differences based on the amount of fiber in the extrudate (18, 36, 48%) and, to some degree, by the type of fiber (cellulose, wheat, and oat) used. Generally, as the amount of fiber in the extrudate increased, negative effects on physical properties were observed. These findings were consistent with previous research.[Citation11,Citation14,Citation16,Citation24] Expansion ratio was significantly influenced by fiber concentration and the fiber concentration/fiber type interaction, while air cell size was significantly influenced by fiber concentration. shows a representative sample of extrudates produced with wheat fiber.
Figure 1 Pictures of (a) control extrudate, extrudates containing wheat fiber at (b) 18%, (c) 36%, and (d) 48%.
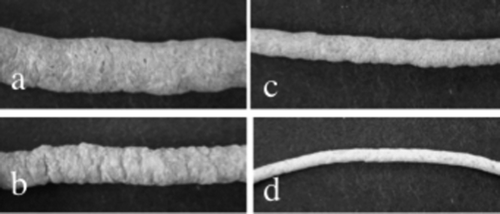
Expansion ratio () and air cell size () both decreased with an increase in the amount of fiber present in the extrudate. All fiber types and concentrations were significantly lower than the controls which are shown on the y axis except for the air cell size 0% TDF control mean which was 0.222 and a letter of ‘c’. Limited expansion was observed at the 48% TDF concentration, with few air cells present (). Generally, as the amount of fiber increased in the extrudates, regardless of fiber type, extrudate air cells were smaller and more numerous. Fiber has been shown to reduce air cell size, reduce extrudate expansion, and cause premature rupture of air cells.[Citation17,Citation24,Citation25] Various researchers have shown that the influence of fiber on expansion is concentration dependent with limited amounts of fiber (10–12%) increasing expansion, but expansion was decreased at higher fiber concentrations.[Citation3,Citation15] Above a critical concentration, the fiber molecules disrupt the continuous melt structure, reducing expansion.[Citation26]
Figure 2 Expansion ratio, air cell size, density and breaking force of extrudates (♦ is cellulose, ▪ is wheat, ▴ is oat). Controls (•) (0% TDF) are shown on the y axis except for air cell size, which has a mean of 0.222 and a letter sharing of ‘c’. Points are means of all three replicates. Means sharing a letter are not different at p > 0.05.
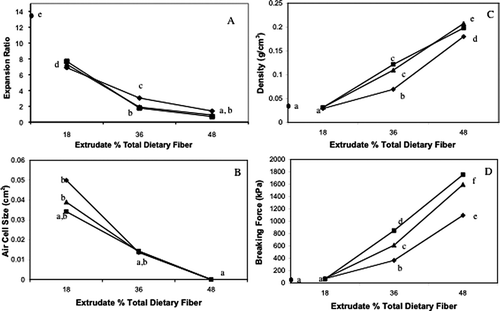
As reviewed by Moraru et al.,[Citation26] extrudate expansion is complex and is the consequence of several events including biopolymer structural transformations, nucleation, bubble growth, and bubble collapse. It is widely recognized that starch plays the major role in expansion and the lower limit of starch content for good expansion is 60–70%. In this research, we are below the lower limited of starch necessary for good expansion, except in the control. Therefore, the type of fiber may be less significant than the amount of starch for extrudate expansion.
Extrudate density () and breaking force () were both influenced by fiber type, fiber concentration and the fiber type/fiber concentration interactions. At fiber concentrations of 48%, there was approximately a four times increase in density compared to the 0%TDF control and the 18% TDF extrudates. Each fiber type had an increase in extrudate density and breaking force with an increase in the amount of fiber in the extrudate. Thus, as breaking force and density increased, extrudate expansion ratio and air cell size decreased. The cellulose extrudates showed slightly higher expansion ratios at 36 and 48% TDF concentrations (). A more expanded product would take less force to shear and have a lower density. There was no difference among samples with added fiber and the control for breaking force or density at 18% TDF.
All physical properties were significantly correlated with each other (). Air cell size was negatively correlated to density and breaking force. Density and breaking force were positively correlated with one another. As the fiber concentration increased, less expansion was seen, with a decrease in air cell size leading to a denser, harder extrudate. Expansion ratio was negatively correlated to density and breaking force and positively correlated to air cell size.
Table 3 Correlations among physical properties. Bold text indicates significant (p < 0.05) coefficients
Chemical Properties
Moisture content of extrudates was significantly influenced by the concentration of fiber. Both WAI and WSI were significantly influenced by the fiber concentration, fiber type, and fiber concentration/fiber type interactions. Water-soluble carbohydrate was significantly influenced by fiber concentration, fiber type and fiber concentration/fiber type interaction, respectively.
Generally, moisture content () increased with increasing fiber concentrations in the extrudate. With moisture content, there was no difference among fiber types at each fiber concentration. The 48% TDF concentration was statistically different from the other fiber concentrations. The dry feed rate at this fiber concentration was approximately 34% lower than the control (), which could have contributed to the high moisture content of the 48% TDF extrudates.
Figure 3 Moisture content, WAI, WSI, and water soluble carbohydrate of extrudates (♦ is cellulose, ▪ is wheat, ▴ is oat). Controls (•) (0% TDF) are shown on the y axis. Points are means of all three replicates. Means sharing a letter are not different at p > 0.05.
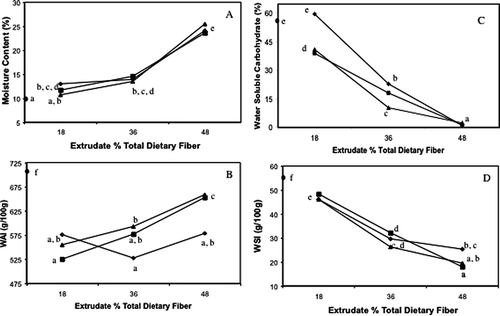
The WAI is the amount of water an extrudate absorbs and is usually dependent on the starch present in the extrudates. All fiber samples showed WAI values lower than the control, which may be due to the decrease in normal corn starch in all samples containing fiber. Wheat and oat fiber extrudates showed similar WAI values, which were higher than the cellulose extrudates. This may be due to the composition of the fibers, with the oat and wheat fibers containing hemicellulose.
The amount of soluble carbohydrate in each sample correlated to the amount of starch (normal and waxy), not fiber, () () except for extrudates containing cellulose that tended to have more water-soluble carbohydrate than the sum of the normal and waxy starch concentrations. The higher amount of water-soluble carbohydrate in the cellulose samples may be due to the partial dextrinization of cellulose to glucose, but it is unclear why this did not occur in the wheat and oat extrudates.
The WSI, a measure of the ability of extrudates to be solubilized by water, decreased with an increase in fiber (). This is consistent with the findings of Jin et al.[Citation16] who reported than an increase in fiber in extrudates from 20% up to 40% caused a decrease in WSI. All fibers had significantly less WSI values than the control at all fiber concentrations. Comparing the WSI data to water soluble carbohydrate () and water soluble protein (), the decrease in WSI with an increase in fiber is related to the starch content. The contribution of lactose and minerals to WSI from WPC80 is 8% and the contribution of water soluble protein to WSI is less than 4.5%, therefore the WSI is related to the solubilization of starch.
Figure 4 Total soluble protein and water soluble protein of extrudates (♦ is cellulose, ▪ is wheat, ▴ is Oat). Controls (•) (0% TDF) are shown on the y axis. Points are means of all three replicates. Means sharing a letter are not different at p > 0.05.
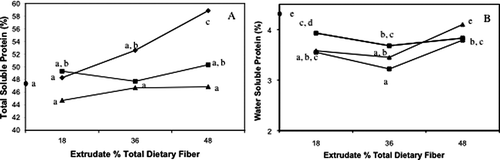
The total percentage of protein in extrudates resolubilized after extrusion (total soluble protein) () was significantly influenced by fiber concentration and fiber type. There were no statistical differences among the control and the oat and wheat extrudates at any concentration while the cellulose extrudates showed higher total soluble protein at 48% cellulose. Cellulose fiber at 48% TDF showed the only significant difference from the control. Generally, extrudates had between 45 and 60% of the whey protein resolubilized after extrusion, which is similar to results reported by Allen et al.[Citation1] for extrudates containing 32% whey protein and normal corn starch. This is indicative of the whey protein's involvement in some form of protein-protein (or protein:carbohydrate) covalent bonding or cross-linking since it was expected that 100% of the protein in extrudates would be resolubilized after extrusion in a solution containing sodium dodecylsulfate (which denatures proteins) and β-mercaptoethanol (which cleaves disulfide bonds). More protein cross-linking in the oat and wheat extrudates may be the result of dextrinized hemicellulose facilitating protein:protein or protein:starch crosslinks. Cellulose extrudates exhibited more total soluble protein at 48% TDF, therefore less cross-links, which may be due to the absence of both starch and hemicellulose at the highest cellulose concentration.
Water-soluble protein was significantly influenced by fiber concentration, fiber type and fiber concentration/fiber type interactions. Water-soluble protein for the control and oat fiber at 48% TDF was significantly higher than all other extrudates. On average, only 3–4% of the whey protein was solubilized in water. This indicates that the majority of whey proteins are involved in both covalent and non-covalent interactions that are formed during extrusion that are not disrupted by water.
There were limited correlations among chemical properties (). Moisture content was negatively correlated to WSI. Water soluble protein and WAI were both positively correlated to WSI. WAI was also positively correlated to water soluble protein. These correlations may be due to the lack of starch in the extrudates.
Table 4 Correlations among chemical properties. Bold text indicates significant (p < 0.05) coefficients
Correlations Among Chemical and Physical Properties
Moisture content and WSI () were correlated to all four physical properties (expansion ratio, air cell size, density, and breaking force). WSI was influenced by the starch content of the extrudates, and the amount of starch influences the expansion ratio and air cell size which in turn influence the density and breaking force. Moisture content also influences expansion ratio since moisture is needed to gelatinize starch and for vaporization at die exit creating an expanded product. Since expansion ratio had low correlations to total and water soluble protein (), it was concluded that increased fiber (with an associated decrease in starch) was responsible for decreased expansion ratio.
Table 5 Correlations among physical and chemical properties. Bold text indicates significant (p < 0.05) coefficients
CONCLUSIONS
Statistical differences were found among extrudates dependent on the concentration and type of fiber incorporated. There was not always a linear association with the amount of fiber added and extrudate properties. Generally, the greatest differences in physical and chemical properties were found in extrudates with the highest fiber (48%) concentration. Chemical and physical properties were generally comparable between the control and extrudates with 18% TDF. Oat and wheat extrudates were very similar for most properties tested which may be due to the presence of hemicellulose in these fibers. Hemicellulose is susceptible to degradation under the conditions of high pressure extrusion and alkaline conditions which may have influenced the amount of protein cross-linking, WAI and water soluble carbohydrate, The greatest factor affecting all properties was the amount of fiber added to the extrudates. It is possible that the effects of fiber inclusion such as decreased air cell size, expansion ratio and increased density and breaking strength is due to the absence or decrease of starch. The addition of dietary fiber at approximately 18% in whey protein-extruded products results in limited detrimental affects on the physical and chemical properties of the extrudates.
ACKNOWLEDGMENTS
This research was supported by Dairy Management Inc, the Utah State University Western Dairy Center (Logan, UT 84322-8700), and the Utah State University Agricultural Experiment Station (Logan, UT 84322-4810). Approved as journal paper number 7834.
REFERENCES
- Allen , K.E. , Carpenter , C.E. and Walsh , M.K. 2007 . Influence of protein level and starch type on an extrusion-expanded whey product . Int. J. Food Sci. Technol. , 42 : 953 – 960 .
- Onwulata , C.I. , Smith , P.W. , Konstance , R.P. and Holsinger , V.H. 1998 . Physical properties of extruded products as affected by cheese whey . J. Food Sci. , 63 : 814 – 818 .
- Onwulata , C.I. , Konstance , R.P. , Strange , E.D. , Smith , P.W. and Holsinger , V.H. 2001 . Co-extrusion of dietary fiber and milk proteins in expanded corn products . Lebensm. Wiss. Technol. , 34 ( 7 ) : 424 – 429 .
- Alavi , S.H. , Gogoi , B.K. , Khan , M. , Bowman , B.J. and Rizvi , S.S.H. 1999 . Structural properties of protein-stabilized starch-based supercritical fluid extrudates . Food Res. Int. , 32 : 107 – 118 .
- Kim , C.H. and Magna , J.A. 1987 . Properties of extruded whey protein concentrate and cereal flour blends . Lebensm. Wiss. Technol. , 20 : 311 – 318 .
- Martinez-Serna , M.D. and Villota , R. 1992 . “ Reactivity, functionality and extrusion performance of native and chemically modified whey proteins ” . In Food Extrusion Science and Technology , Edited by: Kokini , J.L. , Ho , C.T. and Karwe , M.V. 387 – 414 . New York : Marcel Dekker, Inc .
- Matthey , F.P. and Hanna , M.A. 1997 . Physical and functional properties of twin-screw extruded whey protein concentrate-corn starch blends . Lebensm. Wiss. Technol. , 30 ( 4 ) : 359 – 366 .
- Sokhey , A.S. , Rizvi , S.S.H. and Mulvaney , S.J. 1996 . Application of supercritical fluid extrusion to cereal processing . Cereal Foods World , 41 : 29 – 34 .
- Deis, R.C. The Facts on functional Foods. Food Product Design, July 2003 http://www.foodproductdesign.com/articles/0703CS.html (http://www.foodproductdesign.com/articles/0703CS.html) (Accessed: 14 January 2008 ).
- Cuddy , M.E. and Zall , R.R. 1982 . Performance of lipid-dried acid whey in extruded and baked products . Food Technol. , 36 ( 1 ) : 54 – 59 .
- Burglund , P.T. , Fastnaught , C.E. and Holm , E.T. 1994 . Physiochemical and sensory evaluation of extruded high-fiber barley cereals . Cereal Chem. , 71 ( 1 ) : 91 – 95 .
- Huber , G.R. 1991 . Carbohydrates in extrusion processing . Food Technol. , 45 ( 3 ) : 160 – 161 . 168
- Camire , M.E. and King , C.C. 1991 . Protein and fiber supplementation effects on extruded cornmeal snack quality . J. Food Sci. , 56 ( 3 ) : 760 – 763 .
- Rinaldi , V.E.A. , Ng , P.K.W. and Bennink , M.R. 2000 . Effects of extrusion on dietary fiber and isoflavone contents of wheat extrudates enriched with wet okara. Cereal Chem . 77 ( 2 ) : 237 – 240 .
- Grenus , K.M. , Hsieh , F. and Huff , H.E. 1993 . Extrusion and extrudate properties of rice flour . J. Food Eng. , 18 ( 3 ) : 229 – 245 .
- Jin , Z. , Hsieh , F. and Huff , H.E. 1995 . Effects of soy fiber, salt, sugar and screw speed on the physical properties and microstructure of corn meal extrudate . J. Cereal Sci. , 22 : 185 – 194 .
- Huber , G.R. 2001 . “ Snack foods from cooking extruders ” . In Snack Foods Processing , Edited by: Lusas , E.W. and Rooney , L.W. 315 – 367 . Pennsylvania : Technomic Publishing Company, Inc .
- Ng , A , Lecain , S. , Parker , M.L. , Smith , A.C. and Waldron , K.W. 1999 . Modification of cell-wall polymers of onion waste III Effect of extrusion-cooking on cell-wall material of outer fleshy tissue . Carbohydrate Polymers , 39 : 341 – 349 .
- Ralet , M.C. , Della Valle , G. and Thibault , J.F. 1993 . Raw and extruded fibre from pea hulls. Part 1: Composition and physico-chemical properties . Carbohydrate Polymers , 20 : 17 – 23 .
- Qian , J.Y. and Ding , X. 1996 . Effect of twin-screw extrusion on the functional properties of soya fibre . J. Sci. Food Agric. , 71 : 64 – 68 .
- Kuan , Y.H. and Liong , M.T. 2008 . Chemical and physiochemical characterization of agrowaste fibrous materials and residues . J. Agric. Food Chem. , 56 : 9252 – 9257 .
- Taylor , D. , Carpenter , C. and Walsh , M.K. 2006 . Influence of sulfonation on the properties of expanded extrudates containing 32% whey protein . J. Food Sci. , 71 ( 2 ) : 17 – 24 .
- Dubois , M. , Giles , K.A. , Hamilton , J.K. , Rebers , P.A. and Smith , F. 1956 . Colorimetric method for determination of sugars and related substances . Anal. Chem. , 28 : 350 – 356 .
- Lue , S. , Hsieh , F. and Huff , H.E. 1991 . Extrusion cooking of corn meal and sugar beet fiber: Effects on expansion properties, starch gelatinization, and dietary fiber content . Cereal Chem. , 68 ( 3 ) : 227 – 234 .
- Moore , D. , Sanci , A. , Van Hecke , E. and Bouvier , J.M. 1990 . Effect of ingredients on the physical structure and properties of extrudates . J. Food Sci. , 55 ( 5 ) : 1383 – 1381 .
- Moraru , C.I. and Kokini , J.L. 2003 . Nucleation and expansion during extrusion and microwave and heating of cereal foods . Comp. Reviews Food Sci. Food Safety , 2 : 120 – 138 .