Abstract
The effect of extrusion conditions, including feed rate (2.52–6.84 kg/h), feed moisture content (13–19% wet basis), screw speed (150–250 rpm), and extrusion temperature (150–260°C) on the mechanical properties of corn/legume-based extrudates was studied. White bean and lentil were used in mixtures with corn flour at a ratio of 10:90 up to 90:10 (corn:legume). Simple power models were used to correlate breaking stress and corresponding strain with extrusion conditions and material characteristics. The influence of feed rate on the extrudates mechanical properties was incorporated in the mean residence time. The breaking stress of extrudates decreased with temperature, residence time, and corn to legume ratio, and it increased with feed moisture content. The corresponding strain showed an opposite trend. Screw speed did not affect the extrudate properties. The use of lentil flour led to a product with higher breaking stress. Furthermore, in a previous work, the porosity of these products was modeled and, now, it was found that breaking stress and porosity of the extrudates could be correlated by an exponential relationship.
INTRODUCTION
The increasing demand for all types of non-meat, high-protein products has resulted in new and highly profitable ventures for food manufacturers. Proteins derived from plant sources are becoming one of the food industry's fast growing and innovative ingredient segments. Plant proteins have increased functionality, a bland flavor profile, additional nutritional benefits, and a low-cost advantage as both meat extenders and meat alternatives. Legumes, with their high amount of protein (∼18–25%) can be considered as the best and the cheapest source of plant protein. Although there are several methods for processing plant proteins into texturized products, the use of twin-screw extrusion cooking has proven to be instrumental in the development of many new industry opportunities.
Extrusion cooking is unique among thermal processes in that the material is subjected to intense mechanical shear; moistened, starchy or proteinaceous foods are worked into viscous, plastic-like dough and cooked before being forced through the die. The intense structural disruption and mixing facilitates reactions otherwise limited by the diffusion of reactants and products.[Citation1] Some results of cooking during the extrusion process are the gelatinization of starch, denaturation of proteins, inactivation of many native enzymes, which cause deterioration of foods during storage, inactivation of antinutrient factors, and reduction of microbial counts in the final product.[Citation2,Citation3] Product quality can vary considerably, depending on the extrusion processing conditions such as extruder type, screw configuration, feed moisture, temperature profile in the barrel sections, screw speed, and feed rate. The influence of processing conditions during extrusion on the product quality of starch and starch/legumes-based blends has been well.[Citation4–9]
The acceptance of snacks is critical because of the specific quality attributes that attract people. The various sensory attributes of snack foods are appearance, texture, taste, color, and flavor. Among them, texture is one of the most important and this is particularly true for snack food. Crispness is perceived through a combination of tactile, kinesthetic, visual, and auditory sensation, and it represents the key attribute of dry snack products. Crispness is also associated with the rapid drop of force during the mastication process that, in turn, is based on fracture propagation in brittle materials. When a force is applied to brittle snacks, rupture of the cellular structure occurs and, in line with some deformation, generates a typical sound contributing to the crispness sensation. A crispy material usually generates an irregular force-deformation curve.[Citation10] The most common methods to determine the texture of extruded snack foods are compression, puncture, bending and shearing tests, of which the compression test is the most usual.[Citation11–13] The effect of extrusion conditions and material characteristics on the mechanical properties of starch and starch/protein-based extrudates has been discussed extensively during the last decade.[Citation4,Citation12,Citation14,Citation15]. However, the relationships developed were not applied over the whole range of the mentioned materials and process conditions. The relationship between extrusion conditions and physicochemical properties provides a basis for effective product development.
The objective of this study was to investigate the breaking stress changes of two corn/legume mixtures (including white bean and lentil) as a function of process conditions (temperature, feed rate, screw speed) and material characteristics (moisture content and corn to legume ratio). Simple mathematical models were developed for the prediction of breaking stress and the corresponding strain of extrudates as a function of process conditions and material characteristics.
MATHEMATICAL MODELING
Mathematical Models
The mathematical models used to predict the breaking stress and the corresponding strain are summarized on . The models for breaking stress and corresponding strain involve six parameters: the breaking stress and corresponding strain at reference conditions (σ B,0 and ϵ B,0 ), the exponents for residence time (n τ and m τ), the exponents for temperature (nT and mT ), the exponents for feed moisture content (nX and mX ), the exponents for corn to legume ratio (nC and mC ), and the exponents for screw rotation speed (nN and mN ).
Table 1 Mathematical models
The breaking stress and strain influence upon process conditions, such as, product temperature, residence time and screw speed is expressed by the nT , mT , n τ, m τ, nN , mN exponents, respectively, while the influence of material characteristics such as feed moisture content and corn to legume ratio is expressed by the nx , m x and nc , mc exponents, respectively.
The effect of the feed rate, on the extrudates mechanical properties, is incorporated into the mean residence time, assuming that the latter is expressed as:
Regression Analysis
The values of the required parameters are determined by fitting the proposed model to the experimental data. This can be done by minimizing the following residual sum of squares:
The standard deviation between experimental and predicted values (SR ) and the standard experimental error (SE ) can be calculated from the following equations:
Following the regression analysis procedure, all six parameters can be determined simultaneously. However, not all of these parameters affect the sum of squares of the residuals to the same degree. In order to distinguish between the ones that are important for the accurate prediction of the mechanical properties, the following procedure was adopted. Firstly, the minimum change in the sum of squares (SST σ, SST ϵ) was estimated for all six parameters and the mean deviation (S R,σ, S R,ϵ) was evaluated. Secondly, omitting one parameter at a time, the values of S R,σ, S R,ϵ were evaluated for all combinations of the five remaining parameters. In this way, the parameter chosen to be eliminated was the one whose elimination produced the minimum S R,σ S R,ϵ. Continuing the former procedure, the minimum values of S R,σ, S R,ϵ were evaluated for 4, 3, 2, and 1 parameters, respectively. In a typical plot of SR and SE as a function of the number of parameters is shown. The group of parameters which gives a lack of fit (S R,σ, S R,ϵ) close to the mean experimental error (S E,σ, S E,ϵ) is the one that involves the required number of parameters for the prediction of the mechanical properties.
MATERIALS AND METHODS
Sample Preparation
Yellow corn flour was mixed with legume flour (white bean and lentil) and 0.5 g salt per 100 g of mixture was added for taste reasons. Material characteristics are shown on . Distilled water was added to adjust the moisture content. All the ingredients of each preparation were put in a mixer for 15 min, to ensure a homogeneous mixture. After mixing, the samples were packed under vacuum and stored until experimentation time.
Table 2 Extrusion conditions and material characteristics
Extrusion Cooking
A co-rotating twin-screw extruder (Prism Eurolab, model KX-16HC, Staffordshire, UK) was used. The screw geometry was length 40 cm, diameter 16 mm, maximum rotation speed 500 rpm. It was used a cylindrical die with diameter 3 mm and length 17.5 mm. The material was fed into the extruder using a volumetric feeder. The extruder had five temperature control zones. The temperature during extrusion was adjusted by varying the temperature in the barrel, screw, and die using electric heaters. The extrusion conditions are shown on . Steady-state conditions were reached after 20 min, after which samples collected, were dried in air and stored for further properties' measurements.
Residence time is calculated using EquationEq. (3). The void fraction has been correlated with the extrusion conditions and is given by the following equation, which is applicable to this particular extruder type [16]:
Compression Test
The compression tests were performed at room temperature (25°C) using a Zwick Universal Testing Machine (Zwick, model 1120, Ulm, Germany). The samples were compressed between two parallel plates of diameter 10 cm each, at a crosshead speed of 0.5 mm/s, with 100 N load cell. Force and deformation were recorded and the resulting stress-strain compression curves were constructed. A drop of ≥1 N force during compression was considered as the major fracture. At the fracture point, breaking stress was calculated as:
Corresponding strain was calculated as:
RESULTS AND DISCUSSION
Typical compression curves of legume extrudates are presented in . The compression curve indicates the presence of three zones. In the first zone, there is a sharp rise of stress with deformation, which may be attributed to the toughness of the product. In the second zone, multiple fractures occur, as evidenced by several ups and downs in the curve, which still show a marginal increasing trend. The third zone is characterized by a sharp increase of the stress with an increase in the extent of compression. The breaking stress can be considered as the end of the linear part of the compression curve (first zone).
Figure 2 Typical stress-strain curve for corn legume extudates at 200°C, 200 rpm, 4.68 kg/h, 16% feed moisture content and 50% corn to legume ratio (CB: corn/white bean, CL: corn/lentil).
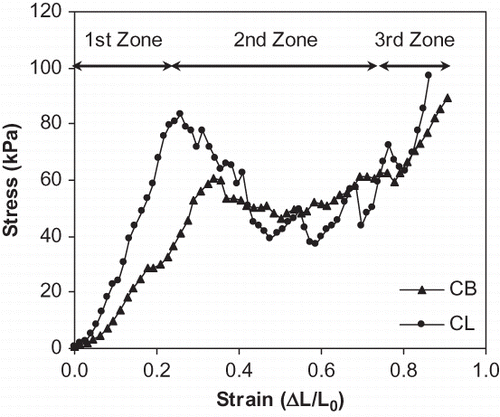
The application of regression analysis for each type of sample showed that the optimum number of parameters, which affect breaking stress and corresponding strain, is five. These parameters are σ B0, nT , n τ, nx , and nc for breaking stress and ϵ B0 , mT , m τ, mx , and mc, for the corresponding strain. The screw rotation speed did not affect significantly the mechanical properties of the extrudates. The corresponding parameter estimates for all materials are shown on . In , mechanical properties of the extrudates are shown as a function of mean residence time, feed moisture content, extrusion temperature, and corn to legume ratio. Solid lines are used for predicted values of mechanical properties using the mathematical model and the parameters of . summarizes experimental and predicted values of mechanical properties for all extrudates.
Table 3 Results of parameter estimation
Table 4 Experimental and predicted values of mechanical properties of corn-legume based extrudates
Figure 3 Breaking stress and corresponding strain of extrudates as a function of residence time and temperature (CB: corn/white bean; CL: corn/lentil).
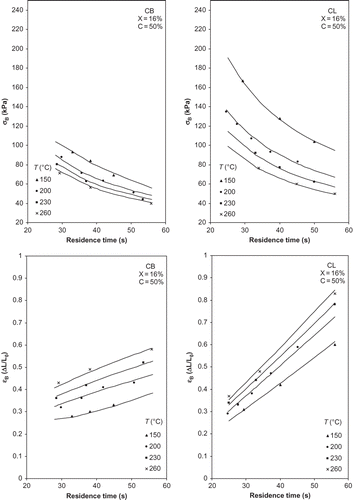
Figure 4 Breaking stress and corresponding strain of extrudates as a function of feed moisture content and corn to legume ratio (CB: corn/white bean, CL: corn/lentil).
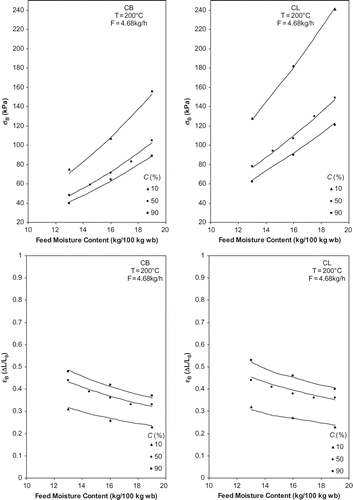
Figure 5 Comparative figures of breaking stress for all extrudates (CB: corn/white bean, CL: corn/lentil). (a) Breaking stress as a function of residence time. (b) Breaking stress as a function of corn/legume ratio. (c) Breaking stress as a function of temperature. (d) Breaking stress as a function of feed moisture content.
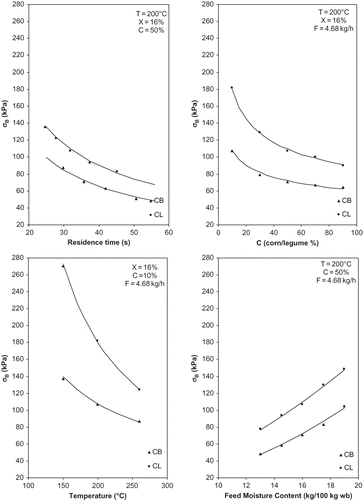
Figure 6 Comparative figures of corresponding strain for all extrudates (CB: corn/white bean, CL: corn/lentil). (a) Corresponding strain as a function of residence time. (b) Corresponding strain as a function of corn/legume ratio. (c) Corresponding strain as a function of temperature. (d) Corresponding strain as a function of feed moisture content.
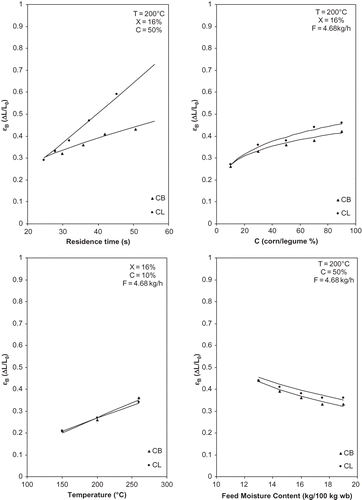
shows that the breaking stress of the extrudates decreased with residence time, while the corresponding strain increased. That is, increased feed rate led to an increase in breaking stress and a decrease in strain (EquationEq. 3). Increased feed rate would influence the degree of fill, inducing the degradation of amylopectin networks, and change the melt rheological characteristics, thus leading to a greater elastic effect and changed product's porosity.[Citation2,Citation4,Citation17] In particular, a rise in feed rate leads to an increased final viscosity and, consequently, to a decreased porosity. The latter may be responsible for the increased breaking stress and the reduction of the corresponding strain.
The extrusion temperature seems to affect the mechanical properties of corn/legume-based extrudates. As can be seen in , the breaking stress decreased with temperature, while the corresponding strain increased. Similar results were found in the past.[Citation4,Citation6,Citation9] Increasing the temperature would decrease the melt viscosity, which favors the bubble growth and produces low density products with small and thin cells, thus increasing the crispness of extrudates and decreasing breaking stress.
The effect of the feed moisture content during extrusion cooking is important as it greatly affects the extrudate texture. indicates that the breaking stress of the extrudates increased with increasing feed moisture content and the corresponding strain decreased. These trends are in agreement with previous works.[Citation9–11,Citation14,Citation15] It is known that the decrease of moisture content in extrusion cooking tends to increase the specific mechanical energy and, consequently, favors the macromolecular degradation of the starch through dextrinisation. The resulting melt gives more fragile structures, which leads to low-resistance cell walls and more structural fractures. Furthermore water leads to plasticization of food materials, which makes the snack, behave like a semi-solid mass rather than a brittle material. Hence, extrudates with high moisture content show enhanced levels of strain tolerance during compression with delayed fracture or even absence of fracture or failure.[Citation10]
The addition of legume flour had a significant effect on the extrudates mechanical properties. As the corn to legume ratio increased the breaking stress decreased, while the corresponding strain increased (). Therefore, the addition of legume caused an increase of the breaking stress of the extrudates. This trend can be attributed to the legume proteins. Proteins can affect the water distribution in the produced matrix through their macromolecular structure and conformation, which affect the extensional properties of the extruded melts. They also contribute to extensive networking through covalent and non-bonding interactions that take place during extrusion. Similar results have been observed by other investigators.[Citation6,Citation13,Citation15]
and make clear that the product containing lentil flour had higher values of breaking stress and corresponding strain than the product with white bean. Furthermore, a stronger influence of temperature on lentil extrudate breaking stress was observed. shows the similarity of the corresponding strain of the two products. The main differentiation is that the lentil extrudate strain is more strongly affected by the residence time. In general speaking, differences in values observed among different legume blends could be attributed to differences in legume proteins and, especially, in protein quality.
It has been proven that the processing conditions and the material characteristic affect the porosity of corn-legume based extrudates.[Citation16] The mechanical properties of extrudates, including breaking stress and corresponding strain, have in general been found to correlate positively with the extrudates' apparent density; increased apparent density corresponds to an increased breaking stress.[Citation6,Citation18,Citation19] It is known that porosity and apparent density are negatively correlated.[Citation16] The correlation of the extrudate porosity with breaking stress and corresponding strain showed that the properties had an exponential correlation. An increase in porosity can lead to a product with a lower breaking stress. depicts these trends.
CONCLUSIONS
Mechanical properties of expanded corn-legume snacks produced on a twin-screw extruder depend on several process conditions. Extrusion temperature and residence time as well as material characteristics (feed moisture content, corn to legume ratio) have a significant effect on the extrudate breaking stress and the corresponding strain. Breaking stress of extrudates was found to decrease with temperature and residence time and to increase with feed moisture content and the ratio of legume. An opposite trend was observed for the corresponding strain. The use of lentil in mixtures for the production of snacks leads to a product with a higher breaking stress than the white bean. In addition, a stronger influence of temperature on the breaking stress was observed. With regard to the corresponding strain, the product with lentil has a slightly higher value than the others have, and it exhibits a stronger influence on residence time. The breaking stress and the porosity of the extrudates were correlated by an exponential relationship. An increase in porosity can lead to a product with lower breaking stress. A similar exponential relationship was produced between porosity and strain at fracture with the opposite trend. In conclusion, the addition of legumes (protein source) leads to harder extruded products. The breaking stress and the porosity are related to the crispness of the product, which is the most important textural attribute of ready-to-eat extruded snacks.
NOMENCLATURE
= |
|
ACKNOWLEDGMENTS
The project is co-funded by the European Social Fund (75%) and National Resources (25%) - EPEAEK II – PYTHAGORAS. The authors wish to thank Mr. Panagiotis Mermigas and Mr. Panagiotis Michailidis for technical assistance.
REFERENCES
- Bjorck , I. and Asp , N.G. 1983 . The effects of extrusion cooking on nutritional value-A literature review . Journal of Food Engineering , 2 ( 4 ) : 281 – 308 .
- Edwards , R.H. , Becker , R. , Mossman , A.P. , Gray , G.M. and Whitehand , L.C. 1994 . Twin-Screw Extrusion Cooking of Small White Beans (Phaseolus vulgaris) . Lebensmittel-Wissenschaft und-Technologie , 27 ( 5 ) : 472 – 481 .
- Steel , C.J. , Sgarbieri , V.C. and Jackix , M.H. 1995 . Use of Extrusion Technology To Overcome Undesirable Properties of Hard-To-Cook Dry Beans (Phaseolus vulgaris L.) . J. Agric. Food Chem. , 43 ( 9 ) : 2487 – 2492 .
- Ding , Q.-B. , Ainsworth , P. , Tucker , G. and Marson , H. 2005 . The effect of extrusion conditions on the physicochemical properties and sensory characteristics of rice-based expanded snacks . Journal of Food Engineering , 66 ( 3 ) : 283 – 289 .
- Ahmed , Z.S. 1999 . Physico-chemical, structural and sensory quality of corn-based flax-snack . Nahrung/Food , 43 ( 4 ) : 253 – 258 .
- Bhattacharya , S. 1997 . Twin-screw extrusion of rice-green gram blend: Extrusion and extrudate characteristics . Journal of Food Engineering , 32 ( 1 ) : 83 – 99 .
- Chauhan , G.S. and Bains , G.S. 1988 . Effect of some extruder variables on physico-chemical properties of extruded rice-legume blends . Food Chemistry , 27 ( 3 ) : 213 – 224 .
- Sun , Y. and Muthukumarappan , K. 2002 . Changes in functionality of soy-based extrudates during single screw extrusion processing . International Journal of Food Properties , 5 ( 2 ) : 379 – 389 .
- Sebio , L. and Chang , Y.K. 2000 . Effects of selected process parameters in extrusion of yam flour (Dioscorea rotundata) on physicochemical properties of the extrudates . Nahrung/Food , 44 ( 2 ) : 96 – 101 .
- Mazumder , P. , Roopa , B.S. and Bhattacharya , S. 2007 . Textural attributes of a model snack food at different moisture contents . Journal of Food Engineering , 79 ( 2 ) : 511 – 516 .
- Van Hecke , E. , Allaf , K. and Bouvier , J.M. 1998 . Texture and structure of crispy-puffed food products part II: Mechanical properties in puncture . Journal of Texture Studies , 29 ( 6 ) : 617 – 632 .
- Onwulata , C.I. , Smith , P.W. , Konstance , R.P. and Holsinger , V.H. 2001 . Incorporation of whey products in extruded corn, potato or rice snacks . Food Research International , 34 ( 8 ) : 679 – 687 .
- Bhattacharya , M. , Hanna , M.A. and Kaufman , R.E. 1986 . Textural Properties of Extruded Plant Protein Blends . Journal of Food Science , 51 ( 4 ) : 988 – 993 .
- Ryu , G.H. and Ng , P.K.W. 2001 . Effects of Selected Process Parameters on Expansion and Mechanical Properties of Wheat Flour and Whole Cornmeal Extrudates . Starch - Stärke , 53 ( 4-5 ) : 147 – 154 .
- Fernandez-Gutierrez , J.A. , Martin-Martinez , E.S. , Martinez-Bustos , F. and Cruz-Orea , A. 2004 . Physicochemical Properties of Casein-Starch Interaction Obtained by Extrusion Process . Starch - Starke , 56 ( 5 ) : 190 – 198 .
- Lazou , A.E. , Michailidis , P.A. , Thymi , S. , Krokida , M.K. and Bisharat , G.I. 2007 . Structural Properties of Corn-Legume Based Extrudates as a Function of Processing Conditions and Raw Material Characteristics . International Journal of Food Properties , 10 ( 4 ) : 721 – 738 .
- Ilo , S. , Tomschik , U. , Berghofer , E. and Mundigler , N. 1996 . The Effect of Extrusion Operating Conditions on the Apparent Viscosity and the Properties of Extrudates in Twin-Screw Extrusion Cooking of Maize Grits . Lebensmittel-Wissenschaft und-Technologie , 29 ( 7 ) : 593 – 598 .
- Hutchinson , R.J. , Siodlak , G.D.E. and Smith , A.C. 1987 . Influence of processing variables on the mechanical properties of extruded maize . Journal of Materials Science , 22 ( 11 ) : 3956 – 3962 .
- Hayter , A.L. and Smith , A.C. 1988 . The mechanical properties of extruded food foams . Journal of Materials Science , 23 ( 2 ) : 736 – 743 .