Abstract
The properties of grain of Mauritia and Prestige barley varieties were determined for moisture content of 12.5 and 15.5% wet basis. Broad variations were observed in the relationship between the moisture content of grain, its variety, and the geometric properties of barley. Most of the geometric properties of barley were dependent on both the cultivar studied and the moisture content of grain, while selected characteristics were dependent on moisture content of grain or barley cultivar only. The values of displacement, force and energy required for kernel rapture were lower for Mauritia variety than Prestige variety and were not dependent on the moisture content of grain.
INTRODUCTION
Barley is one of the leading grain crops produced in the world with growing worldwide recognized as a source of food and many valuable nutritional components that enrich the human diet. Barley grain is used primarily for malting and livestock feeding, and the straw is also an important feedstuff when more desirable forages are not available. Most barley is grown for malt due to the price premium. Numerous research reports have been published on the composition of barley grain,[Citation1] its color[Citation2] and starch[Citation3] characteristics, as well as the functional,[Citation4–6] geometric,[Citation7,Citation8] and thermo-geometric[Citation9] properties of barley grain and malt.
If the moisture content of barley during harvest is low (below 14.0% w.b.), grain has to be aerated during storage. But if its moisture content exceeds 14.0%, grain should be dried before storage. The effect of drying or storage on the quality of barley is of considerable importance to the barley industry. It is evident that drying can adversely influence barley quality, whereas storage can either reduce barley quality[Citation10] or increase maltability.[Citation11] To design equipment for aeration and storage, various geometric properties have to be investigated as a function of moisture content. In order to optimize the equipment design for harvesting, handling, drying, storing, and other processes involving barley grain, its geometric properties have to be analyzed. Thermal capacity and conductivity influence the rate of heating and cooling of grain during drying, aeration, and storage, while diffusivity affects the rate of moisture migration in grain. In order to determine moisture diffusivity of grain, the shape of a single kernel must be known. Some seeds have a spherical shape,[Citation12] but this is not the case with cereals. For some cereals, the ellipsoid is a good approximation of a single kernel's shape. Gaston et al.[Citation13] showed that the ellipsoidal geometry of a kernel is useful for the determination of moisture diffusivity of wheat. Size and shape are important in designing separating, harvesting, sizing, and grinding machines. Bulk density and porosity affect the structural loads; the angle of repose is important in designing storage and transport structures. The seed's coefficient of friction against various surfaces also has to be determined for the needs of designing conveying, transporting, and storing structures.[Citation14] The knowledge of mechanical characteristics of grain supports improvements in its milling technology.[Citation15]
Each year, more than 200 new barley varieties are entered into the official variety testing system in different European countries. Around 100 of the introduced varieties are usually claimed to possess malting and brewing qualities, but only 10 to 20 varieties are used in malting barley production.[Citation16] Since published data on the geometric properties of barley grain commercially produced in Europe is scarce, the objective of this study was to investigate selected geometric and mechanical properties of the grain of barley varieties commercially grown in Poland, namely, their shape and size, apparent volume, 1000 grain mass, bulk density, apparent density, porosity, angle of repose, static coefficients of friction on various surfaces, as well as deformation, rupture, and energy required for rupture of kernels.
MATERIALS AND METHODS
Material
The barley grains used in this study were two commercially produced barley cultivars with a different malting quality index: Mauritia (index 7.0) and Prestige (index 5.9), harvested in 2006 in NE Poland. Cultivar malting quality index is presented as a 9-point scale with 1 as the lowest and 9 as the highest quality and is calculated based on the following malt attributes: malt extractivity, wort viscosity, the degree of final attenuation, Kolbach index, and diastatic power according to Research Centre for Cultivar Testing in Poland.[Citation17] The moisture content of the collected samples was 14.5 and 15.0% w.b. for cv. Mauritia and Prestige, respectively. Directly after harvesting, the investigated grain was cleaned and stored in a refrigerator at 10°C.
Two-grain humidity levels were investigated in this study. The samples with 17.5 ± 0.2% moisture content were prepared by adding amounts of distilled water calculated based on the sample's initial moisture content according to AACC method 26–95.[Citation18] The samples with moisture content of 12.5 ± 0.2% were prepared by drying in a laboratory scale spouted-bed drier at 35°C.[Citation19] After drying or conditioning, every sample was sealed in separate polyethylene bags. All samples were stored in a refrigerator at 10°C for 4 days to allow the moisture to distribute uniformly throughout the sample. The required amount of the material was removed from the refrigerator and warmed up to room temperature (usually 20°C) at least 12 h prior to the experiment.[Citation13] The geometric properties of grain were investigated at moisture levels of 12.5 and 17.5% w.b. At least ten replications of each test were performed for each moisture level.
Geometric Characteristics
The computer image analysis technique was applied to determine the projection area, length, and width of a single kernel of barley. Barley kernels were placed in four rows and nine columns on five arrays of black plastic, kernel crease downward. Kernel images were obtained using an Epson 4490 flat scanner. The details for scanner settings were as follows: image size 2754 × 4172 pixels; scan resolution 400 dpi; and depth of image 24 bits, as described by Zapotoczny et al.[Citation20] The length (L) and width (W) of a single kernel were derived from the projected area of a single kernel. The thickness (T) of a single kernel was measured with a micrometer to an accuracy of 0.01 mm. The number of kernels used in each analysis was 180.
Assuming that the shape of a single kernel is similar to the shape of a triaxial ellipsoid of axis equal to L (the longest), W (intermediate) and T (shortest), several geometric characteristics of a kernel can be calculated,[Citation13] i.e., geometric surface area, Agg , and volume, Vgg , of an ellipsoid, according to the following Equation1–4).
The so called ‘‘corrected’’ surface area, Ag , which is the difference between Vg and Vgg , where Vg is the measured apparent volume of a kernel, can be calculated based on the following formula:
Percentile differences in apparent volume between the kernels applied during the experiments and their ellipsoidal models were described with the following formula:
The third power of the sphericity index, f, can be defined as the ratio of the volume of the solids to the volume of the circumscribed sphere having the diameter equal to the longest intercept of the ellipsoid, as illustrated by the following formula[Citation7]:
Based on Mohsenin's[Citation7] findings, the aspect ratio, Ra , can be calculated with the following formula:
The apparent density of a solid is defined as the weight per unit volume of a material including voids inherent in the material as tested. The kernel apparent density of barley, ρ g , was measured by the hydrostatic method with the use of 100 kernels. Two different results of sample mass measurement were applied to calculate apparent density, i.e., the first measurement, ma , performed in the air and the second measurement, m tol, obtained for a sample submerged in toluene. Apparent density was then calculated as follows:
The temperature of air and toluene was 20°C. Apparent density measurement was performed in 15 replications. Based on the results of apparent density measurements, the apparent volume, Vg , and the equivalent diameter, De , of a single kernel were determined as follows:
Bulk density, ρ bulk , was calculated as the ratio of the mass of a seed sample to its total volume. The bulk porosity porosity of a static bed of barley, ϵ, was calculated as follows[Citation7]:
To evaluate 1000 grains mass, 1000 grains randomly selected from the bulk were averaged. The mass of grain was measured using an electronic balance to an accuracy of 0.001 g. The angle of repose was determined by using a steel funnel consisting of a cone at the top and a cylinder at the bottom. The height of the cone part of the funnel was 150 mm, while its diameters were 200 and 25 mm at the top and bottom, respectively. The cylindrical part was 30 mm long and 25 mm in diameter. The funnel was placed at the center of a raised circular plate, filled with barley grain, and raised slowly until it was emptied and the grain formed a cone on a circular plate. The diameter, Dc , and height, Hc , of the cone was recorded. The angle of repose, Ar , was calculated by using the following formula:
The angle of repose was calculated based on the measurement of the cone's height and diameter. The static coefficients of friction of barley kernels against galvanized metal sheet, plywood and plexiglass were determined. The sample comprising several bound kernels was placed on an inclined surface on which the inclination angle was increased gradually. At the moment of movement, the angle was read from the scale. The friction coefficient was calculated by evaluating the tangent of the angle.
Terminal velocity and drag coefficient of barley kernels have been experimentally measured by suspending the particles in an air stream. Prior to the experiment, 2 kg of barley grain was divided into three fractions using two sieves of 2.5 and 2.75 mm orifice sizes. From each fraction, 100 g of grain was randomly selected to produce three samples of the same mass and different kernel sizes. Terminal velocity of barley grain was measured for each sample using the K-293 vertical wind tunnel (Petkus, Germany). Grain was placed in the loading tray and inserted into the wind tunnel with 50 × 50 mm cross-section and height of 700 mm. The air flow rate was adjusted until the sample was suspended in the air, and terminal air velocity was calculated with the use of the following equation:
The drag coefficient of a single kernel can be calculated with the use of the following equation[Citation7]:
Mechanical Properties
Quasi-static compression tests of a single barley kernel were performed. All tests were carried out using an Instron Universal Testing Machine (High Wycombe, Bucks, UK), model 4301, operating in the compression mode, fitted with a parallel plate fixture and a 1 kN load cell. Cross-head speed of 5 mm/min was applied. A single barley kernel was randomly selected from the batch and placed on the bottom parallel plate, kernel crease downward. The mechanical behavior of barley grain dried to moisture content of 12.5% w.b. was expressed in terms of deformation, force, and energy required to attain the bioyield point and to reach the point of total rupture of a single kernel during the quasi-static compression test. The mechanical behavior of barley grain conditioned to moisture content of 17.5% w.b. was characterized by a monotonically rising force-deformation curve, which did not exhibit the bioyield point, or the total rapture point. Therefore, in order to compare the differences in the mechanical properties of barley grain with moisture content of 12.5 to 17.5% w.b., the force and energy required for the compression of barley kernels conditioned to 17.5% w.b. were derived for the same deformations, which had been applied to attain the bioyield point, as well as to achieve to total rapture point of kernels dried to 12.5% w.b. All compression tests were carried out in 35 replications.
Statistical Analysis
An analysis of variance (p ≤ 0.05) was performed to test the influence of the moisture content of grain and cultivar on the geometric and mechanical properties of barley grain. Duncan's parametric test was applied to perform the statistical analysis, and STATISTICA 7.1 software (StatSoft Inc., USA) was used.
RESULTS AND DISCUSSION
Geometric Characteristics
shows a summary of the geometric properties of Mauritia and Prestige barley grain varieties at two moisture content levels: 12.5 and 17.5% w.b. It was found that most of the properties listed in are dependent on moisture content, the barley cultivar or both factors, and that the higher the moisture content of barley, the higher the value of the observed property.
Table 1 Selected geometric properties of Mauritia and Prestige barley varieties.Footnote*
No significant differences were observed in kernel length between barley cultivars, but the differences between moisture content levels were significant and kernel length was higher at higher moisture content levels. The reverse was observed in respect of kernel width, which was influenced by grain cultivar but not by the moisture content level. Higher width was observed for Prestige cultivar. Moisture content did not affect the thickness of Prestige variety kernels, but it affected the Mauritia variety. The average length, width and thickness were in the range of 9.14–9.43, 3.79–3.93, and 2.68–2.78 mm, respectively, which is consistent with the data reported by Güner.[Citation21]
The sphericity index did not present significant differences between the moisture content of Mauritia cultivar, but significant differences were reported in the moisture content of Prestige cultivar. As regards both grain varieties, the higher the moisture content of grain, the lower sphericity and aspect ratio indices were observed. The mean sphericity values for Mauritia and Prestige barley cultivars were in the range of 49.2–49.5 and 49.8–50.7, respectively, and they fall within the range of 0.32–1 proposed by Mohsenin[Citation7] for most agricultural materials. The obtained sphericity results are consistent with the above proposal. As regards both of the applied moisture content levels, there were no significant differences in the equivalent diameter observed between cultivars. A similar tendency was reported for the volume of a single grain. The higher the applied moisture content level, the higher the equivalent diameter and volume of grain were noted, and the above results are consistent with the findings of Çalışır et al.[Citation22] for rapeseed.
The projected area of kernels of Mauritia variety barley grain did not differ with variations in moisture content of grain, but differences were reported for Prestiuge variety, and higher values of projected area were observed at higher moisture content levels. The average values of projected area of barley kernels varied between 25.4 and 26.5 mm2, subject to barley variety and grain moisture content. Similar values were reported for barley grain and lentil seeds at moisture content of 9% w.b.[Citation21]
In given drying conditions, the intensity of heat and mass transfer processes depend on both the shape and size of the dried solid. When diffusion of water within the particle limits the drying rate, larger particles dry more slowly than smaller particles of the same shape. Therefore, in order to derive the proper values of thermal conductivity and mass diffusivity based on experimental kinetic curves, the size and shape of the solid should be known and described precisely. The relatively low values of sphericity indices, as shown in , suggest that the shape of a single kernel differs significantly from the shape of a sphere. This is why the difference between the geometric characteristics of a single kernel of barley grain and its model assumed as an ellipsoid has been studied. It was found that both the geometric area and volume of a kernel, derived on the assumption of the kernel's ellipsoidal shape, depend on variety and moisture content of grain. The corrected kernel surface area was not affected by barley variety but it was dependent on the moisture content of grain, and the values observed for 17.5% moisture content were higher then the results for 12.5%. It was found that in respect of both barley varieties and moisture levels of grain applied in this study, the apparent volume of a single grain varied significantly from the geometric volume of a kernel. The assumption of barley kernels' ellipsoidal shape leads to an overestimation of a kernel's volume. Yet the average percentile differences in apparent volume between an average kernel investigated during the experiment and its ellipsoidal-shaped model were relatively low and ranged between 11.3%, which was observed for Prestige cultivar at 17.5% moisture content of grain, and 18.1%, which was reported for Mauritia variety at 12.5%. The clear difference between the equivalent diameter of a barley kernel and the short and intermediate intercepts of an ellipsoidal kernel model indicates that moisture and heat diffusivities of barley grain calculated on the basis of spherical and ellipsoidal shape should vary, as confirmed by Gaston et al.[Citation13] in respect of wheat.
Density and Porosity
The bulk density values of barley grain at moisture content of 12.5 and 17.5% varied between 596.8 and 646.5 kg/m3. The bulk density of Mauritia variety was not dependent on the moisture content of grain. The reverse was reported as regards the bulk density of Prestige variety, which was higher for 12.5% moisture content of grain. The bulk density of barley grain of Mauritia cultivar was significantly lower than that reported for Prestige cultivar. The bulk density of grain is useful in the design of silos and storage bins. Since the bulk density of Mauritia variety is below that of Prestige variety, Prestige variety would require a larger silo than Mauritia variety grain with the same weight. Significant differences in the apparent density of barley grain were observed between varieties and the moisture content of grain. The apparent density values of barley grain varied between 970.5 kg/m3 for Mauritia cultivar at 17.5% moisture content of grain to 1042.6 kg/m3 for Prestige cultivar at 12.5%. Pneumatic sorting tables are used to separate seeds of cereal crops by apparent density. Seeds of various impurities greatly differ from the seeds of cereal crops in both true and apparent density terms. The porosity values of Mauritia variety at 17.5% moisture content of grain and Prestige variety at 12.5 and 17.5% showed no significant differences. The corresponding values were 0.382, 0.380 and 0.376, respectively. The porosity of 0.407, observed for Mauritia variety at 12.5% moisture content of grain, was significantly higher. The reported values of bulk density and porosity of barley approximated the findings of Molenda & Horabik.[Citation8]
It was observed that moisture content had no significant effect (p ≤ 0.05) on the drag coefficient and terminal velocity of barley, but both properties were dependent on the variety of barley. Higher values of the drag coefficient and lover values of terminal velocity were observed for Mauritia cultivar in comparison with those reported for Prestige cultivar. The average drag coefficient and terminal velocity of barley were in the range of 0.387–0.440, and 7.96–9.74 m/s, respectively, which is consistent with the data reported by Güner[Citation21] for barley at moisture content of 9% w.b.
As regards the static coefficient of friction, as shown in , there were significant differences (p ≤ 0.05) between the studied cultivars, the moisture content of grain and the surfaces applied. The coefficient of friction on material surfaces ranged from 0.373 to 0.497 for all grain varieties, material surfaces and moisture contents. For Mauritia variety, the lowest and highest values of the static coefficient of friction were 0.373 (against plexiglass at 12.5% moisture content of grain) and 0.494 (against plywood at 17.5% moisture content of grain), while 0.384 (against plexiglass at 12.5% moisture content of grain) and 0.497 (against plywood at 17.5% moisture content of grain) were obtained for Prestige variety. In respect of Mauritia variety, there were no significant differences in the static coefficient of friction against plywood and plexiglass between different moisture content levels, while the static coefficient of friction against galvanized metal sheet rose with the increase in the moisture content of grain. As regards Prestige variety, the static coefficient of friction against plywood and plexiglass increased with the rise in the moisture content of grain, while the static coefficient of friction against galvanized metal sheet did not exhibit significant differences between different moisture content levels. Furthermore, as regards Mauritia variety at 12.5% and Prestige variety at 12.5 and 17.5% moisture content, no significant differences in the static coefficient of friction between all surfaces studied were observed. The values of the static coefficient of friction against galvanized metal sheet and wood were similar to the data reported for barley by Mohsenin[Citation7] but they exceeded the findings of Ghasemi Varnamkhasti et al.[Citation23] for rough rice. There are no published sources documenting the static coefficient of barley friction against plexiglass. The differences in the static coefficient of friction of barley against different materials as observed between published data and the results of this study could be caused by variations in the applied material roughness. For this reason, a further inquiry should be made into the average material roughness.
Table 2 Static coefficient of friction of Mauritia and Prestige barely varieties against different surfaces.Footnote* Footnote §
No differences were found in the angle of repose between the Mauritia variety grain with various moisture content, while the angle of repose in Prestige variety decreased with a drop in moisture content and was below that observed for Mauritia variety. The angle of repose of barley ranged from 18.1 to 19.7° for both grain varieties and moisture contents, and the above findings are consistent with the data reported by Mohsenin[Citation7] for pilling of barley. Thousand grain mass values ranged from 48.6 to 55.1 g for all grain varieties and moisture contents studied and were higher than those reported by Güner.[Citation21] It was found that for both barley varieties, the thousand grain mass of barley increased with the rise in moisture content of grain, and the values observed for Prestige variety were higher then those determined for Mauritia variety.
Mechanical Properties
shows the typical behavior of Mauritia and Prestige barley cultivars at 12.5 and 17.5% grain moisture content during a quasi-static compression test. At a grain moisture content of 12.5%, both a bioyield point—at which an increase in deformation is accompanied by a decrease in force, and a rapture point—at which a compressed kernel raptures under load, were observed in Mauritia and Prestige barley varieties. As demonstrated by in respect of both investigated varieties, grain conditioned to moisture content of 17.5% and subjected to a quasi-static compression test behaved differently than grain dried to 12.5%. It exhibited no bioyield point or rapture point, and its behavior was similar to that of elastic materials. To investigate the influence of moisture content on grain's mechanical behavior, the force and energy of both grain varieties were derived for deformations at which the bioyield point and rapture point of grain dried to 12.5% moisture content were observed.
Figure 1 A model force-deformation curve of quasi-static compression of a single kernel of Mauritia and Prestige barley cultivars with (a) 12.5% and (b) 17.5% grain moisture content.
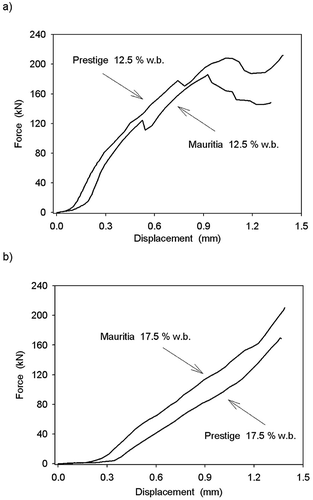
shows experimental values of force, deformation and energy required for kernel deformation up to the bioyield point, derived for Mauritia and Prestige barley varieties at 12.5 and 17.5% moisture content of grain. It was found that for Mauritia variety, the bioyield point occurs at higher deformation of 0.728 mm than in Prestige variety where it was observed at 0.616 mm. There were no significant differences in force values at bioyield point between the analyzed barley varieties, but the force values reported at that deformation was higher for Mauritia variety than for Prestige variety. Force values at the bioyield point ranged from 59.8 to 116.4 N. Force values at the bioyield point during a compression test for barley were reported by Mohsenin[Citation7] in the range of 53.4 to 57.8 N for grain moisture content similar to that investigated in this study. The same tendency was reported in respect of energy required to cause a given deformation. The energy required for kernel deformation up to the bioyield point ranged from 0.0147 to 0.0343 J. According to Mohsenin, [Citation7] the energy values required for barley kernel deformation up to the bioyield point were in the range of 0.0508 and 0.0576 J. The differences in the force and energy required for kernel deformation up to the bioyield point between the measured values and published data may be caused by significant variations in the applied cross-head speed, i.e., 5 mm/min in this study and 1.3 mm/min as indicated[Citation7] for barley, but all data has the same order of magnitude. The values of deformation, force, and compressive energy at bioyield point were lower than those reported by Gunasekaran and Paulsen[Citation24] for dried corn.
Table 3 Displacement, force and energy required for the deformation of kernels of Mauritia and Prestige barley varieties at the bioyield point.Footnote* , Footnote §
shows experimental values of force, deformation and energy required for kernel deformation up to the rapture point, obtained for Mauritia and Prestige barley varieties at 12.5 and 17.5% moisture content of grain. It was found that in the investigated barley varieties neither deformation at the rapture point nor rapture force were dependent on the moisture content of grain. Both kernel deformation at rapture point and the rapture force observed for Mauritia variety were lower than the indicators determined for Prestige variety. Kernel deformation at rapture point for Mauritia and Prestige varieties ranged from 1.180 to 1.321 mm, respectively, while rapture force ranged from 162.9 to 193.3 N, respectively. As regards the energy required for kernel rapture, there were significant differences (p ≤ 0.05) between the studied cultivars and the moisture content of grain. The energy required for kernel rapture ranged from 0.0722 to 0.1427 J. Higher values of the required rupture energy were observed for Prestige cultivar in comparisoe cultivar in comparison with those reported for Mauritia cultivar and for 17.5% grain moisture content in comparison with 12.5% grain moisture content. The values of rapture force are similar to those reported by Sadowska et al.[Citation25] for Sigma I wheat cultivar, but they are lower than the findings received for dried corn.[Citation13] The results postulated by the latter in respect of energy values required for dried corn rupture are higher than the barley-specific values indicated in this study.
Table 4 Displacement, force and energy required for the deformation of kernels of Mauritia and Prestige barley varieties at the rupture point.Footnote* Footnote §
CONCLUSIONS
This paper concludes with information on the engineering properties of Mauritia and Prestige varieties, which may be useful for designing much of the equipment used for barley processing. Wide variations were observed in the relationship between geometric and mechanical properties of barley grain, its moisture content and variety. Most of the geometric properties of barley were dependent on both the cultivar studied and the moisture content of grain, while selected characteristics were determined by moisture content or barley cultivar only. The effect of moisture content on the geometric and mechanical properties of barley grain should be investigated within a broader spectrum of change in the moisture content than observed in this study. Further tests are required to confirm the results reported by the authors of this study.
NOMENCLATURE
Ag | = |
Corrected kernel surface area (m2) | |||||||||||||||||||||||||
Agg | = |
Geometric area of ellipsoid (m2) | |||||||||||||||||||||||||
Ap | = |
Projected area (m2) | |||||||||||||||||||||||||
Ar | = |
Angle of repose (deg) | |||||||||||||||||||||||||
Cd | = |
Drag coefficient | |||||||||||||||||||||||||
Dc | = |
Diameter of cone (m) | |||||||||||||||||||||||||
De | = |
Equivalent diameter (m) | |||||||||||||||||||||||||
ErrV | = |
Percent error of the kernel apparent volume (%) | |||||||||||||||||||||||||
F | = |
Sphericity factor | |||||||||||||||||||||||||
F | = |
Inner area of the tunnel cross-section (m2) | |||||||||||||||||||||||||
g = 9.807 | = |
Acceleration of gravity (m/s2) | |||||||||||||||||||||||||
Hc | = |
Height of cone (m) | |||||||||||||||||||||||||
L, W, T | = |
Length, width, and thickness of a single kernel (m) | |||||||||||||||||||||||||
Ra | = |
Aspect ratio | |||||||||||||||||||||||||
M | = |
Mass (kg) | |||||||||||||||||||||||||
ma, mtol | = |
Mass of sample in air and submerged in toluene (kg) | |||||||||||||||||||||||||
V | = |
Volume (m3) | |||||||||||||||||||||||||
V | = |
Velocity (m/s) | |||||||||||||||||||||||||
Vg | = |
Apparent kernel volume (m3) | |||||||||||||||||||||||||
Vgg | = |
Geometric volume of ellipsoid (m3) | |||||||||||||||||||||||||
W | = |
Air flow rate (m3/s)
|
ACKNOWLEDGMENTS
This study was financially supported by the Polish Ministry of Scientific Research and Information Technology within the framework of grant no. 2 P06T 062 27. The authors would like to thank Dr. Krzysztof Jadwisinczak of the Faculty of Engineering at the University of Warmia and Mazury in Olsztyn, Poland, for his helpful cooperation in performing measurements of terminal velocities and drag coefficients.
REFERENCES
- Grove , A.V. , Hepton , J. and Hunt , C.W. 2003 . Chemical composition and ruminal fermentability of barley grain, hulls, and straw as affected by planting date, irrigation level, and variety . The Professional Animal Scientist , 19 ( 4 ) : 273 – 280 .
- Reuss, R. In Storage induced colour changes of barley grain, Proceedings of the 10th Australian Barley Technical Symposium, Canberra, ACT, Australia 16–20 September 2001, ISSN 1030-5408. http://www.cdesign.com.au/proceedings_abts2001/papers/23.%20Reuss.pdf (http://www.cdesign.com.au/proceedings_abts2001/papers/23.%20Reuss.pdf) (Accessed: 24 June 2010 ).
- Morrison , W.R. , Scott , D.C. and Karkalas , J. 2006 . Variation in the composition and physical properties of barley starches . Starch–Stärke , 38 ( 11 ) : 374 – 379 .
- Giural , H.S. and Gaur , S. 2005 . Instrumental texture of chapati as affected by barley flour, glycerol monostearate and sodium chloride . International Journal of Food Properties , 8 ( 2 ) : 377 – 385 .
- Ereifej , K.I. , Al-Mahasneh , M.A. and Rababah , T.M. 2006 . Effect of barley flour on quality of balady bread . International Journal of Food Properties , 9 ( 1 ) : 39 – 49 .
- Sidhu , J.S. , Kabir , Y. and Hoffman , F.G. 2007 . Functional foods from cereal grains . International Journal of Food Properties , 10 ( 2 ) : 231 – 244 .
- Mohsenin , N.N. 1980 . Physical properties of plant and animal materials , New York : Gordon and Breach Science Publishers . 678.
- Molenda, M.; Horabik, J. Mechanical properties of granular agro-materials and food powders for industrial practice. Part I. Characterization of mechanical properties of particulate solids for storage and handling. Institute of Agrophysics PAS: Lublin, 2005 http://www.ipan.lublin.pl/mat_coe/mat_coe24.pdf (http://www.ipan.lublin.pl/mat_coe/mat_coe24.pdf) (Accessed: 24 June 2010 ).
- Alagusundaram , K. , Jayas , D.S. , Muir , W.E. and White , N.D.G. 1991 . Thermal conductivity of bulk barley, lentils and peas . Transactions of the ASAE , 34 ( 4 ) : 1784 – 1788 .
- Woods , J.L. , Favier , J.F. and Briggs , D.E. 1994 . Predicting the germinative energy of dormant malting barley during storage . Journal of the Institute of Brewing , 100 : 257 – 269 .
- Woonton, B.; Jacobsen, J.; Sherkat, F.; Stuart, M. In Effect of post-harvest storage period on barley germination and malt quality. Proceedings of the 10th Australian Barley Technical Symposium, Canberra, ACT, Australia 16–20 September 2001, ISSN 1030-5408. http://www.cdesign.com.au/proceedings_abts2001/papers/P36.%20Wooton%20et%al.pdf (http://www.cdesign.com.au/proceedings_abts2001/papers/P36.%20Wooton%20et%al.pdf) (Accessed: 24 June 2010 ).
- Yalcin , I. and Ozarslan , C. . 2004 . Physical Properties of Vetch Seed . Biosystems Engineering , 88 ( 4 ) : 507 – 512 .
- Gaston , A.L. , Abalone , R.M. and Giner , S.A. 2002 . Wheat drying kinetics. Diffusivities for sphere and ellipsoid by finite elements . Journal of Food Engineering , 52 : 313 – 322 . 2002
- Altuntaş , E. , Özgöz , E. and Taşer , F. 2005 . Some physical properties of fenugreek (Trigonella foenum-graceum L.) seeds . Journal of Food Engineering , 71 : 37 – 43 .
- Engleson , J.A. and Fulcher , R.G. 2002 . Mechanical behavior of oats: the groat effects . Cereal Chemistry , 79 ( 6 ) : 787 – 789 .
- Larsen , J. Evaluation of new European malting barley varieties . Proceedings of the 10th Australian Barley Technical Symposium . Canberra, Australia.
- Najewski , A. 2005 . Barley varieties . Agro Serwis , 2 : 27 – 30 . In Polish
- American Association of Cereal Chemists (AACC) . 2000 . Approved methods of the AACC , 10th , 26 – 95 . 44 – 15A . St. Paul, MN : AACC .
- Markowski , M. , Sobieski , W. , Konopka , I. , Tańska , M. and Białobrzewski , I. 2007 . Drying characteristics of barley grain dried in a spouted-bed and combined IR-convection driers . Drying Technology , 25 : 1621 – 1632 .
- Zapotoczny , P. , Zielińska , M. and Nita , Z. 2008 . Application of image analysis for the varietal classification of barley: Morphological features . Journal of Cereal Science , 48 : 104 – 110 .
- Güner , M. 2007 . Pneumatic conveying characteristics of some agricultural seeds . Journal of Food Engineering , 80 ( 3 ) : 904 – 913 .
- Çalışır , S. , Marakoğlu , T. , Öğüt , H. and Öztürk , Ö. 2005 . Physical properties of rapeseed (Brassica napus oleifera L.) . Journal of Food Engineering , 69 : 61 – 66 .
- Ghasemi Varnamkhasti , M. , Mobli , H. , Jafari , A. , Keyhani , A.R. , Heidari Soltanabadi , M. , Rafiee , S. and Kheiralipour , K. 2007 . Some physical properties of rough rice (Oryza Sativa L.) grain . Journal of Cereal Sciences , 47 ( 3 ) : 496 – 501 .
- Gunasekaran , S. and Paulsen , M.R. 1985 . Breakage resistance of corn as a function of drying rates . Transactions of the ASAE , 28 ( 6 ) : 2071 – 2076 .
- Sadowska , J. , Blaszczak , W. , Jelinski , T. , Fornal , J. , Borkowska , H. and Styk , B. 2001 . Fertilization and technological quality of wheat grain . International Agrophysics , 15 : 279 – 285 .