Abstract
Cold-set gels of whey protein isolate (WPI) and of WPI plus tara gum (TG) were produced through the addition of magnesium chloride to heat-denatured (HD) WPI and WPI+TG solutions. The flow behaviour of the WPI, TG, and WPI+TG samples was analyzed: WPI solutions exhibit Newtonian behavior while the behavior of the TG and WPI+TG solutions can be described through the Cross and Carreau models. The mechanical characterization of the cold-set gels was done through puncture tests, and the Young's modulus for each cold-set gel was obtained. Statistical analysis of the mechanical data was made according to the Marquadt-Levenberg algorithm.
INTRODUCTION
Whey proteins are well known for their functional and nutritional properties, which lead to their incorporation in many food products.[Citation1] One of the most important features of whey proteins is their gelling capability, and for this reason many studies have been concerned with the gelation process. Food protein gels are usually formed during heating, and consequently this process is known as heat-set gelation.[Citation2–4] More recently, an alternative process called cold-set gelation, according to which the gelation occurs at ambient temperature, has been studied.[Citation5–11] The cold gelation of whey proteins has two major steps. First, a native solution of proteins is heated above 65°C for protein denaturation and aggregation. During heating, the processing conditions, like heating temperature and time, the environmental conditions, like pH, mineral content, and ionic strength, and, finally, the protein concentration must be carefully manipulated in order to ensure that the electrostatic repulsion is high enough to prevent the formation of a gel. In a second step, the gelation of the denatured solution is induced, at ambient temperature, usually by the addition of a salt whose cations screen the protein charges and lead to the decrease of the electrostatic repulsion between the molecules. In the case of divalent cations, salt addition may also improve the formation of salt bridges between negatively charged protein groups which means that much less salt concentrations is needed to obtain a gel. The salt used in the present study was magnesium chloride, since Mg2+ is an alternative to the most common used cations, like sodium,[Citation5,Citation11–13] calcium,[Citation5,Citation6,Citation8,Citation11,Citation12,Citation14] or iron.[Citation15] Besides, magnesium is a very important element for human health, since it is essential for nerves, muscles, and most other body cells to function. Magnesium is also a vital catalyst in enzyme activity, especially the activity of those enzymes involved in energy production; it also assists in calcium and potassium uptake.[Citation16]
In recent years, considerable interest has also been devoted to the study of protein-polysaccharide mixtures, particularly in order to increase their applicability in the food industry.[Citation17] Proteins and polysaccharides are the most important gelling and thickening agents; therefore, the interactions between these two kinds of biopolymers are of great importance in determining the processes of structure formation and the mechanical properties of new products. In this paper, besides the study of cold-set gels of WPI, mixed gels of WPI and tara gum were also analysed. Tara gum is a galactomannan that is obtained from the seeds of the tara shrub, Caesalpinia spinosa, which is a native of the northern regions of Africa and South America. It is described as having a backbone of (1–4)-linked β-D-mannopyranosyl units one third of which possess a single unit side chain of (1–6)-linked α-D-galactopyranose.[Citation18] Applications for TG are ice cream, cheese, bakery products, desserts, sauces and meat products.[Citation19]
The behavior of simple whey protein or mixed whey protein plus polysaccharide systems has been essentially studied in solution and during gel formation using rheological small deformation techniques.[Citation12] However, an important view of the food structure is related to the study of the breaking properties in large deformation, according to which the textural attributes are decisive for quality and consumer acceptance of the product. The objective of the present work was to analyse the impact of WPI and TG concentrations on the flow behaviour of WPI and WPI+TG native solutions and heat-denatured (HD) dispersions, as well as on the mechanical response of the cold-set gels obtained through the addition of Mg2+ to HD-WPI (or HD-WPI+TG) dispersions. The flow behavior of the WPI and WPI+TG solutions in the native form and after denaturation was analyzed in light of the Cross and Carreau models.[Citation20,Citation21] The large deformation behavior of simple gels of WPI and mixed gels of WPI+TG was assessed trough puncture tests. Values of fracturability, resistance to puncture, rupture strain, rigidity, and Young's modulus were obtained for each gel. A statistical analysis of the data was done in order to assess the influence of protein, tara gum, and magnesium concentrations on the gel properties.
MATERIALS AND METHODS
Materials
Whey protein isolate powder (LACPRODAN DI-9224, Lot no, 50M160218) was kindly supplied by Arla Foods Ingredients (Videbaek, Denmark). The protein content, determined by the Kjedahl method, was 93.5 wt%. According to the suppliers, the WPI total solids content was 94% (6% moisture), the ash content was 4.5% and the cation content was: sodium, 0.5%; phosphorus, 0.2%; chloride, 0.1%; potassium, 1.2% and calcium, 0.1% (all quantities are expressed in mass percentage). The tara gum was kindly supplied by Carob S.A (Spain), with the commercial reference no. 1760. The magnesium chloride, in the form of a hexahydrate salt (MgCl2.6H2O), the sodium azide (NaN3) and the potassium hydroxide (KOH) were obtained from Riedel-de-Haën (Sigma-Aldrich Laborchemikalien, Seelze, Germany), with weight purity greater than 99, 99, and 85%, respectively.
Methods
As it was stated above, this work intends to analyse the impact of WPI and TG concentrations on the viscoelastic behaviour of WPI and WPI + TG native solutions and heat-denatured (HD) dispersions, as well as on the mechanical response of the cold–set gels obtained through the addition of Mg2+ to HD-WPI (or HD-WPI+TG) dispersions. Preliminary studies allowed us to define the upper and lower limits of the WPI, Mg2+ and TG concentrations that would produce cold-set gels after heat denaturation of WPI or WPI + TG solutions at 75°C for 20 min. Based on those studies an experimental design was implemented.
Experimental Design and Statistical Analysis
The Box-Behnken experimental design[Citation22] was employed to investigate the interactive effect of WPI (6.0, 7.5, and 9.0 wt%), Mg2+ (25, 50, and 75 mM), and TG (0.20, 0.35, and 0.50 wt%). The experimental design consisted of 15 experimental points (with three independent variables and three levels, including three replicates at the central point) performed in random order (). The measured responses were fracturability, rupture strain, rigidity and Young's modulus.
Table 1 Box-Behnken experimental design matrix
The results obtained from Box-Behnken experimental design were analysed by multiple regressions, using the least squares regression methodology, to fit the following second-order equations to all dependent variables:
Preparation of WPI, TG, and WPI+TG Solutions
WPI solutions (6.2, 7.7, and 9.2 wt%, in protein) were prepared in beakers by weighing the appropriate amount of powder and adding distilled water, without reaching the final solutions weights. After 3 h of gentle mixing, the pH was adjusted to 7.0 with KOH 1M and/or HCl 1M. Two drops of sodium azide solution (2.0 wt%) were added in order to prevent bacterial growth. Finally, distilled water was added until the final weights were reached and the systems were kept under mixing for one more hour.
The tara gum stock solution was prepared as follows: the required amount of the powdered gum was gradually added to the appropriate amount of distilled water in order to obtain a solution with 1 wt% in TG. After 1 hour of vigorous stirring at room temperature, the solution was heated at 90°C for 30 min in a water bath, under continuous stirring. The non-dissolved material was removed by centrifugation at 30000 g for 1 h, at 25°C, and the concentration of the solution was determined from its dry matter content. TG solutions with 0.21, 0.36, 0.51, and 0.67 wt% concentrations were prepared from this stock solution by appropriate dilution with distilled water. To prepare the solutions of WPI+TG, the WPI powder was dispersed in the appropriated amount of tara gum stock solution. Then, distilled water was added, without reaching the final solutions weights, and from this point onward, the procedure was the same as that described above for WPI solutions.
Heat Denaturation and Cold-set Gel
The native solutions were denatured by heating at 75°C for 20 min, in a water bath. These conditions are known to provide a partial unfold and aggregation of the protein molecules without gelation.[Citation10] After heating, the dispersions were cooled in an ice bath in order to stop the denaturation process immediately. The aggregates formed during this stage remained soluble and stable dispersions were obtained which were stored at room temperature overnight. Then, 39.0 g of each dispersion were put inside beakers and gelation was induced through the addition of 1.00 g of magnesium chloride solution with the appropriate salt concentration. The gels were placed in a chamber of controlled temperature (25°C) during 48 h before being subjected to the texture tests.
Viscosity of the Solutions
The rheological measurements were performed at 25°C using a controlled stress rheometer AR-G2 (TA Instruments) fitted with a cone-and-plate geometry (4° cone angle, 60 mm diameter, 107 μm gap). The flow curves of the WPI, TG and WPI+TG native solutions and heat-denatured dispersions were determined by subjecting each sample to consecutive 60 seconds steps of constant shear stress. This step duration ensured the record of steady state shear rates and the corresponding viscosities (shear stress over shear rate) allowed us to build the steady state flow curves. All experiments were performed over a shear rate window that ranged from the lowest operational limit of the rheometer to a value that ensures the ‘destruction’ of the sample. In all experiments, the samples were covered with a thin layer of paraffin oil to prevent evaporation.
Penetration Tests on the Cold-set Gels
The measurements were performed with a TA–XT2 Texture Analyzer (Stable Micro Systems, Godalming, Surrey, UK), at room temperature. The plunger was cylindrical with a 10 mm diameter, and operated with a speed of 0.1 mm s−1. Tests were made directly in the beakers where the gels were penetrated down to 80% of the initial gel height. This deformation value was obtained through preliminary tests in order to ensure the rupture of the gel. To avoid friction, the plunger was lubricated with paraffin oil before each test. Data of force, distance and time were recorded, at a sampling rate of 50 pps (points per second). Taking into account that the texture tests have a great variability, eight replicates of each gel were tested being the results reported as the average. The following parameters were determined: fracturability [N], which is defined as the force at the first peak in the force vs. distance curve; rigidity [N m−1], which is the ratio between force and distance, at the fracturability point; puncture resistance [N], which is the force at the second peak in the force vs. distance curve; and rupture strain, which is the strain at the rupture point. If there is no second peak, the puncture resistance is equal to fracturability.[Citation27]
The measured force, F, and the depth of penetration, d, were manipulated in order to obtain the apparent Young's modulus, E, of each cold-set gel. The measured force, F, and the force due to buoyancy, Fb , were used to calculate the force corrected for buoyancy, Fcb .[Citation28] The E value is given through the slope of the initial linear increase of Fcb plotted as a function of the strain (δ = d / L, where L is the gel height). The low penetration velocity of the probe (0.1 mm/s) and the high sampling rate (50 points per second) of the reported experiments, allowed us to record “quasi-static” forces resulting from the very slow deformation of the gels, thus ensuring that the “time” factor during the experiment was not a problem, as it is stated by Oakenfull and Tanner.[Citation29]
RESULTS AND DISCUSSION
Flow Behaviour of the Solutions
Flow curves were performed at 25°C in order to investigate the shear, WPI and TG concentration effects on the apparent viscosity, ηap. The WPI native solutions and heat-denatured dispersions exhibited a clear Newtonian behaviour, in the shear rate window analysed. The experimental values are reported in , and, as it would be expected, the viscosity increased with the WPI concentration, since a raise in the WPI concentration means a higher number of macromolecules in solution, and, consequently, higher viscosity values. The heat-denatured dispersions presented always higher viscosity values than the correspondent native solutions, meaning that the unfolding of the protein molecules was effectively induced during the heating process.
Table 2 Values of apparent viscosity for native (ηap ND) and heat-denatured (ηap HD) WPI solutions, at 25°C
The flow curves corresponding to the TG solutions manifested typical rheological characteristics of a thickened fluid: a zero shear viscosity plateau at low shear rates and a shear-thinning regime at higher shear rates (). Nonlinear models namely, the Cross and Carreau models,[Citation20
Citation–21] were used to describe the apparent viscosity. These models describe the dependence of the apparent viscosity on the shear rate, , according to the EquationEqs. (2) and Equation(3), respectively:
Table 3 Cross (τ, m) and Carreau (λ, k) adjustable parameters for steady simple shearing, obtained for TG solutions, at 25°C
Figure 1 Flow curves for tara gum native (ND) solutions (open symbols) and heat denatured (full symbols) dispersions, for different concentration values, at 25°C. Symbols: (□) 0.20 wt%, (△) 0.35 wt%, (○) 0.50 wt%, and (⋄) 0.65 wt%. Full line represents predictions of the Cross model and dotted lines those of the Carreau model.
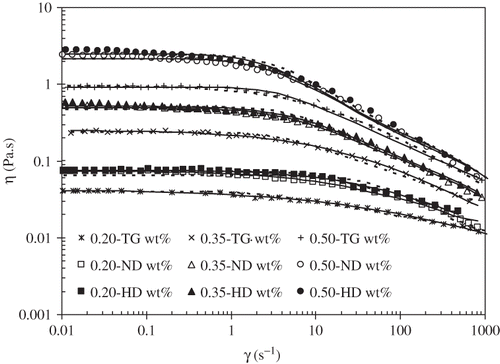
Figure 2 Shear rate/concentration superposition for tara gum, native (ND) solutions (open symbols) and heat denatured (full) dispersions, at 25°C. Master curve at the reference concentration of 0.20 wt% TG. Symbols: (□) 0.20 wt%, (△) 0.35 wt%, (○) 0.50 wt% and (⋄) 0.65 wt%.
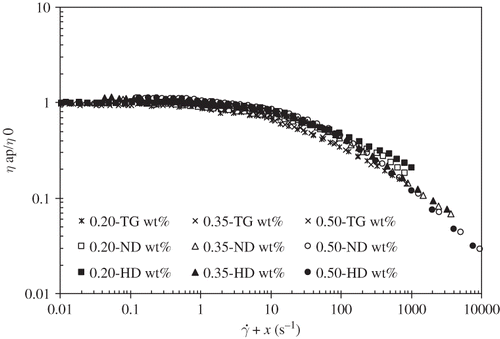
Figure 3 Horizontal (open symbols) and vertical (full symbols) shift factors as a function of the tara gum concentration, used to obtain the flow master curves of

The analysis of the flow curves correspondent to the WPI+TG solutions, with a WPI concentration of 7.5 wt% and a TG concentration that varied from 0.2 to 0.5 wt%, shows that the Newtonian behaviour exhibited by the WPI solutions was irremediably changed by the introduction of tara gum. The behaviour of the mixtures was conditioned by the presence of the galactomannan, as it can be seen in . Taking this into account, the flow curves of the WPI (native or heat denatured) + TG mixtures were correlated in light of the Cross and Carreau models, like was done before for TG solutions. The obtained parameters are reported in .
Table 4 Cross (τ, m) and Carreau (λ, k) adjustable parameters for steady simple shearing, of the native and heat-denatured mixed solutions of WPI+TG, at 25°C
Adopting the same procedure used for TG solutions, it was also possible to obtain a master flow curve for the mixtures WPI+TG, taking as reference the flow curve correspondent to 7.5 wt% WPI + 0.20 wt% TG. The vertical and horizontal shifts for the native and the correspondent heat-denatured mixtures of WPI+TG are the same, what is a consequence of the zero-shear viscosities and the time constants being analogous. These results are plotted in and .
Textural Characteristics of the Cold-set Gels
The puncture tests made on the cold-set gels allowed us to determine several mechanical properties for each of them. The values of fracturability, puncture resistance, rupture strain, rigidity and Young's modulus are reported in . According to the statistical procedure described in a previous section, the second-order polynomial model regression coefficients (EquationEq. 1) were determined for each response analysis, taking as independent variables the WPI, TG and Mg2+ concentrations. The results obtained are reported in . In all cases, the total regression is significant (p ≤ 0.05).
Table 5 Mechanical properties of the cold-set WPI and WPI+TG gels
Table 6a Regression coefficients of fracturability, puncture resistance, rupture strain, rigidity and Young's modulus
The analysis of variance reported in , shows that the linear WPI and TG concentrations are significant terms (p ≤ 0.05) for all the mechanical parameters determined. The linear Mg2+ concentration is significant only for the fracturability, puncture resistance and rigidity. The cross-products CWPI×CTG, CWPI×CMg2+ and CTG×CMg2+ are significant terms for all response analysis with four exceptions: CWPI×CTG and CTG×CMg2+ are not significant for the rupture strain, CTG×CMg2+ is not significant for puncture resistance and CWPI×CMg2+ is not significant for Young's modulus.
Table 6b analyses of variance (p value) of fracturability, puncture resistance, rupture strain, rigidity and Young's modulus
The rupture strain seems to present a critical value at the middle point, which means that increases with WPI concentration until 7.5 wt% and then starts to decrease; with Mg2+ and TG concentrations, the rupture strain firstly decreases till 50 mM and 0.35 wt%, respectively, and then reverts this tendency. The Young's modulus increases with the Mg2+concentration till 50 mM, and then starts to decrease. These results show that cold-set gels with a large spectrum of mechanical properties have been produced by manipulating their composition.
CONCLUSIONS
The cold gelation of whey protein isolate and whey protein isolate plus tara gum solutions is an interesting alternative to the traditional heat gelation for application in the food industry. The obtained gels have a large spectrum of textural properties, depending on the protein, salt or TG concentrations. These findings are of great practical importance, since the utilization of these gels in very different products can be envisaged. The viscoelastic behaviour of the WPI solutions is Newtonian, while for the TG solutions and for the WPI +TG native solutions (or HD dispersions) a typical rheological behaviour of a thickened fluid was observed, that could be described through the Cross and Carreau models. The viscosity of heat-denatured dispersions is higher than that of the correspondent native solutions, which ensures that protein unfolding was induced during the heating process. According to the statistical analysis of the results, it was verified that the linear WPI and TG concentrations are significant terms (p ≤ 0.05) for all the mechanical parameters determined, but the linear Mg2+ concentration is significant only for the fracturability, puncture resistance and rigidity.
REFERENCES
- De Wit , J.N. 1998 . Nutritional and Functional Characteristics of Whey Proteins in Food Products . J. Dairy Sci. , 81 ( 3 ) : 597 – 608 .
- Raikos , V. , Campbell , L. and Euston , S.R. 2007 . Rheology and Texture of Hen's Egg Protein Heat-Set Gels as Affected by pH and the Addition of Sugar and/or Salt . Food Hydrocolloid. , 21 ( 2 ) : 237 – 244 .
- Lopes , G.K. , Alviano , D.S. , Torres , D. , Gonçalves , M.P. and Andrade , C.T. 2006 . Gelation of Whey Protein Concentrate in the Presence of Partially Hydrolyzed Waxy Maize Starch and Urea at pH 7.5 . Colloid Polym. Sci. , 285 ( 2 ) : 203 – 210 .
- Puyol , P. , Pérez , M.D. and Home , D.S. 2001 . Heat-Induced Gelation of Whey Protein Isolates (WPI): Effect of NaCl and Protein Concentration . Food Hydrocolloid. , 15 ( 3 ) : 233 – 237 .
- Resh , J.J. and Daubert , C.R. 2002 . Rheological and Physicochemical Properties of Derivatized Whey Protein Concentrate Powders . Int. J. Food Prop. , 5 ( 2 ) : 419 – 434 .
- Bolder , S.G. , Hendrickx , H. , Sagis , L.M.C. and van der Linden , E. 2006 . Ca2+-Induced Cold-Set Gelation of Whey Protein Isolate Fibrils . Appl. Rheol. , 16 ( 5 ) : 258 – 264 .
- Sok Line , VL , Remondetto , GE and Subirade , M . 2005 . Cold Gelation of β-Lactoglobulin Oil-in-Water Emulsions . Food Hydrocolloid. , 19 ( 2 ) : 269 – 278 .
- Maltais , A. , Remondetto , G.E. , Gonzalez , R. and Subirade , M. 2005 . Formation of Soy Protein Isolate Cold-Set Gels: Protein and Salt Effects . J. Food Sci. , 70 ( 1 ) : C67 – C73 .
- Hongsprabhas , P. , Barbut , S. and Marangoni , A.G. 1999 . The Structure of Cold-Set Whey Protein Isolate Gels Prepared with Ca2+ . LWT - Food Sci. Technol. , 32 ( 4 ) : 196 – 202 .
- Bryant , C.M. and McClements , D.J. 2000 . Optimizing Preparation Conditions for Heat-Denatured Whey Protein Solutions to be Used as Cold-Gelling Ingredients . J. Food Sci. , 65 ( 2 ) : 259 – 263 .
- Bryant , C.M. and McClements , D.J. 2000 . Influence of NaCl and CaCl2 on Cold-Set Gelation of Heat-Denaturated Whey Protein . J. Food Sci. , 65 ( 5 ) : 801 – 804 .
- Bryant , C.M. and McClements , D.J. 2000 . Influence of Xanthan Gum on Physical Characteristics of Heat-Denaturated Whey Protein Solutions and Gels . Food Hydrocolloid. , 14 ( 4 ) : 383 – 390 .
- Marangoni , A.G. , Barbut , S. , McGauley , S.E. , Marcone , M. and Narine , S.S. 2000 . On The Structure of Particulate Gels - The Case of Salt-Induced Cold Gelation of Heat-Denaturated Whey Protein Isolate . Food Hydrocolloid. , 14 ( 1 ) : 61 – 74 .
- Veerman , C. , Baptist , H. , Sagis , L.M.C. and Van der Linden , E. 2003 . A New Multistep Ca2+-Induced Cold Gelation Process for β-Lactoglobulin . J. Agr. Food Chem. , 51 ( 13 ) : 3880 – 3885 .
- Remondetto , G.E. , Paquin , P. and Subirade , M. 2002 . Cold Gelation of β-Lactoglobulin in the Presence of Iron . J. Food Sci. , 67 ( 2 ) : 586 – 595 .
- Magnesium Information - Benefits, Deficiency, Food Sources http://www.healthvitaminsguide.com/minerals/magnesium.htm (http://www.healthvitaminsguide.com/minerals/magnesium.htm) (Accessed: 26 March 2009 ).
- Zazypkin , D.V. , Braudo , E.E. and Tolstoguzov , V.B. 1997 . Multicomponent Biopolymer Gels . Food Hydrocolloid. , 11 ( 2 ) : 159 – 170 .
- Daas , P.J.H. , Schols , H.A. and De Jongh , H.H.J. 2000 . On the Galactosyl Distribution of Commercial Galactomannans . Carbohyd. Res. , 329 ( 3 ) : 609 – 619 .
- Borzelleca , J.F. , LaDu , B.N. , Senti , F. and R; Egle , J.L. 1993 . Evaluation of the Safety of Tara Gum as a Food Ingredient: A Review of the Literature . J. Am. Coll. Toxicol. , 12 ( 1 ) : 81 – 89 .
- Cross , M.M. 1965 . Rheology of Non Newtonian Fluids: A New Flow Equation for Pseudoplastic Systems . J. Colloid Sci. , 20 : 417 – 437 .
- Carreau , P.J. 1972 . Rheological Equations from Molecular Network Theories . Trans. Soc. Rheol. , 16 ( 1 ) : 99 – 127 .
- Box , G.E.P. and Behnken , D.W. 1960 . Some New Three-Level Designs for the Study of Quantitative Variables . Technometrics , 2 : 455 – 475 .
- Box , G.E.P. 1952 . Multifactor Designs of First Order . Biometrika , 39 : 49 – 57 .
- Box , G.E.P. and Draper , N.R. 1959 . A basis for the selection of a response surface design . J. Amer. Stat. Assoc. , 54 : 622 – 654 .
- Box , G.E.P. and Wilson , K.B. 1957 . On the Experimental Attainment of Optimum Conditions . J. Roy. Statist. Soc. , B13 : 1 – 45 .
- Box , G.E.P. and Hunter , J.S. 1957 . Multifactor Experimental Design for Exploring Response Surfaces . Ann. Math. Statist. , 28 : 195 – 241 .
- Vázquez da Silva , M. , Mayor , L. , Lemos , M.A. , Sittikijyothin , W. , Gonçalves , M.P. and Sereno , A.M. Progress in Rheology of Biological and Synthetic Polymer Systems . September 2004 , Beja, Portugal. Edited by: Diogo , A.C. , Alvarenga , N.B. , Canada , J. , Ferro Palma , S. and Dias , J. pp. 9 – 11 . 151 – 156 . Beja, , Portugal : Instituto Politécnico de .
- Gregson , C.M. , Hill , S.E. , Mitchell , J.R. and Smewing , J. 1999 . Measurement of the Rheology of Polysaccharide Gels by Penetration . Carbohyd. Polym. , 38 ( 3 ) : 255 – 259 .
- Oakenfull , D.G. and Tanner , R.I. 2000 . Comment on Measurement of the Rheology of Polysaccharide Gels by Penetration by Gregson, C.M.; Hill, S.E.; Mitchell, J.R.; Smewing, J. Carbohyd . Polym. , 42 ( 1 ) : 95 – 95 .